Half a century of change in floristics and structure in an urban grassy woodland: implications for conservation management
Jamie B. Kirkpatrick

A
Abstract
Vegetation remnants enveloped by built up areas tend to drift away in their character from vegetation on similar sites in more extensive patches of native vegetation, but may stabilise in synthetic (novel) form with time in harmony with disturbance regimes and environmental change. Managers may be able to induce a synthetic state that maintains the nature conservation values of such remnants.
We examine the degree to which the nature conservation values of the the vegetation of a 19th century urban reserve in nipaluna (Hobart), lutruwita (Tasmania) changed between 1974 and 2022/23 in a period of climate change and changes in vegetation management, with the aim of discerning appropriate conservation management regimes.
Sixty-nine 1 × 15 m quadrats were randomly located in the non-cultivated part of the Domain in 1974 and roughly relocated for sampling in 1984, 1994, 2001 and 2022. Tree diameters were recorded using the point-centred quarter method in 1974, 1984, 1994, 2001 and 2023. The responses of native and exotic species richness, and the frequency of the more common species, to time and other predictor variables related to time since last fire, evidence of mowing at the time of the survey, and topography were determined using linear modelling, correlation, analysis of variance and chi-squared.
The native tree Allocasuarina verticillata was the only significantly varying consistent increaser of 67 taxa. The native perennial herbs Acaena echinata and Leptorhynchos squamatus and the non-native shrubby weed Chrysanthemoides monilifera were the only significantly varying consistent decreasers. However, several rare and threatened species too infrequent for statistical analysis also declined between 2022/23 and 2000. Twenty-four of the 67 taxa with sufficient data for analysis did not significantly vary among years. The percentage of native species in quadrats did not change through time. It was negatively related to mowing and the time since fire. Native tree stems 10–30 cm in diameter and those over 30 cm increased through time, whereas those less than 10 cm in diameter increased dramatically until 2000, then strongly decreased by 2023. Total stem density increased through time.
Mowing and fire regimes strongly influenced the distributions of a large proportion of native taxa independent of time and underlying environment, largely by controlling the density of the small tree layer, which can potentially form closed-forest without eucalypts.
It is possible to use fire, mowing and slashing to maintain nature conservation values in synthetic urban grassy woodland, although the maintenance of the rarest native species requires more specific and varied interventions.
Keywords: fire regimes, grassland, grassy woodland, mowing, nature conservation values, rare and threatened plants, synthetic vegetation, temporal change, vascular plants.
Introduction
The biotic characteristics and nature conservation management of native vegetation that is reserved or remnant within cities have been investigated since the 1960s, initially largely in Scandinavia, the United States and Australia (Florgård 2007). We now know that these fragments of wild vegetation in city and suburbia have a disproportionate importance for the conservation of nature (Miller and Hobbs 2002; Ives et al. 2016; Kendal et al. 2017) despite the urban pressures that beset them (Aronson et al. 2014) and the typical deterioration of nature from the pre-urbanisation state (Hahs et al. 2009). This deterioration includes increasing richness and cover of non-native plants in ‘synthetic communities/ecosystems’ (Odum 1962; Bridgewater and Backshall 1981), later to be relabelled as ‘novel ecosystems’ (Hobbs et al. 2006).
Bridgewater (1988, pp. 139–140) defined synthetic communities as ‘composed of species native to the local landscape region, in ratios differing from those of native communities or a mixture of native species with those native to other landscape regions in the same or different continents; both types of which are capable of persistence through several disturbance cycles’. He went on to hypothesise: ‘While there is no doubt some synthetic communities are unstable phases in an ultimately degrading landscape, there are many other examples where the communities are no less stable than native communities, and, with appropriate management, become part of the landscape matrix.’ (Bridgewater 1988, p. 139).
It is highly important for conservation management of remnants to both understand the changes in state of nature conservation values and the management regimes needed to prevent their ongoing degradation. The most critical of these nature conservation values are the elements of biodiversity and geodiversity that most depend on a remnant for their future (Kirkpatrick and Kiernan 2006). Increases in non-native species richness and cover are not considered to be a major threat in this context (Kirkpatrick and Gilfedder 1995; Rohwer and Marris 2021). However, a loss of native species, whether at the quadrat or remnant scale, is a strong indication of deterioration in nature conservation value.
We have, at least, two cases in which lag periods for the effects of urbanisation have been determined. O’Shea and Kirkpatrick (2000) observed a three decade lag period after adjacent house building in the displacement of most native plants by non-natives in a coastal reserve in Hobart, Tasmania, Australia, and du Toit et al. (2016) observed a two to four decade lag period between urbanisation and the development of synthetic vegetation in woody grassland at Potchefstroom, South Africa. While no data were given on the time of urban envelopment, ongoing changes in species composition, including more non-natives, were recorded over a decade in urban grassland remnants in Melbourne, Victoria, Australia, at the same time that rural remnants suffered little change (Zeeman et al. 2017). Thus, it seems that any examination of potential stability of synthetic vegetation in relation to nature conservation values should wait at least 40 years from urbanisation of its matrix.
One longterm record of vegetation change in an urban reserve enveloped well before monitoring is from Kings Park, Perth, Western Australia, which was declared in 1871 and compared between 1939 and 1999 (Crosti et al. 2007). These 60 years saw dramatic changes in tree density, tree species composition, understorey type and cover of non-native grasses in a period of much management change, which together were interpreted as negative in their nature conservation impact (Crosti et al. 2007). Yet, in the Sandringham coastal reserve in Melbourne, Victoria, although non-native species richness increased between 1971 and 2013, non-native cover decreased and native richness increased, a putatively positive nature conservation outcome attributed to intensive weeding and planting (Ivey-Law and Kirkpatrick 2015). This observation period started several decades after suburbanisation (Kirkpatrick 1974, 1975).
The aim of the present paper is to examine the degree to which the nature conservation values of the vegetation of the Queens Domain (Domain), nipaluna (Hobart), lutruwita (Tasmania), Australia, a 19th century urban reserve, have been maintained between 1974 and 2022/23 in a period of climate change and changes in vegetation management. We endeavour to determine whether there is a management pathway to stability in conservation values that is implied by our results. We focus on changes in native species frequencies, the richness of native species, and the density of native tree stems by species and size.
Methods
Study area
The Domain is situated adjacent to the Hobart CBD, the inner suburbs of Hobart and the Derwent Estuary (Fig. 1). Climatic means are somewhat meaningless, except for global comparison, as temperatures in Hobart have increased exponentially since the mid-20th century and rainfall has declined markedly in a context of high inter- and intra-annual variability (Fig. 2). However, the average annual rainfall was 559 mm between 1974 and 2022, mean monthly rainfall in the same period varied from a mean of 34 mm in the driest month (February) to 61 mm in the wettest month (August), the mean daily maximum temperature was 17.5°C, with the mean for the warmest month (January) averaging 22.4°C and the coldest month (July) averaging 12.4°C. The mean daily minimum temperature was 8.9°C, with the coldest month (July) averaging 4.9°C and the warmest (January) averaging 12.8°C.
Variation in (a) rainfall (mm) and (b) temperature (°C) from 1964 to 2022 on the Queens Domain, with the survey years highlighted. Source: Bureau of Meteorology (bom.gov.au/tas/climate/data station 094030, accessed December 2022).
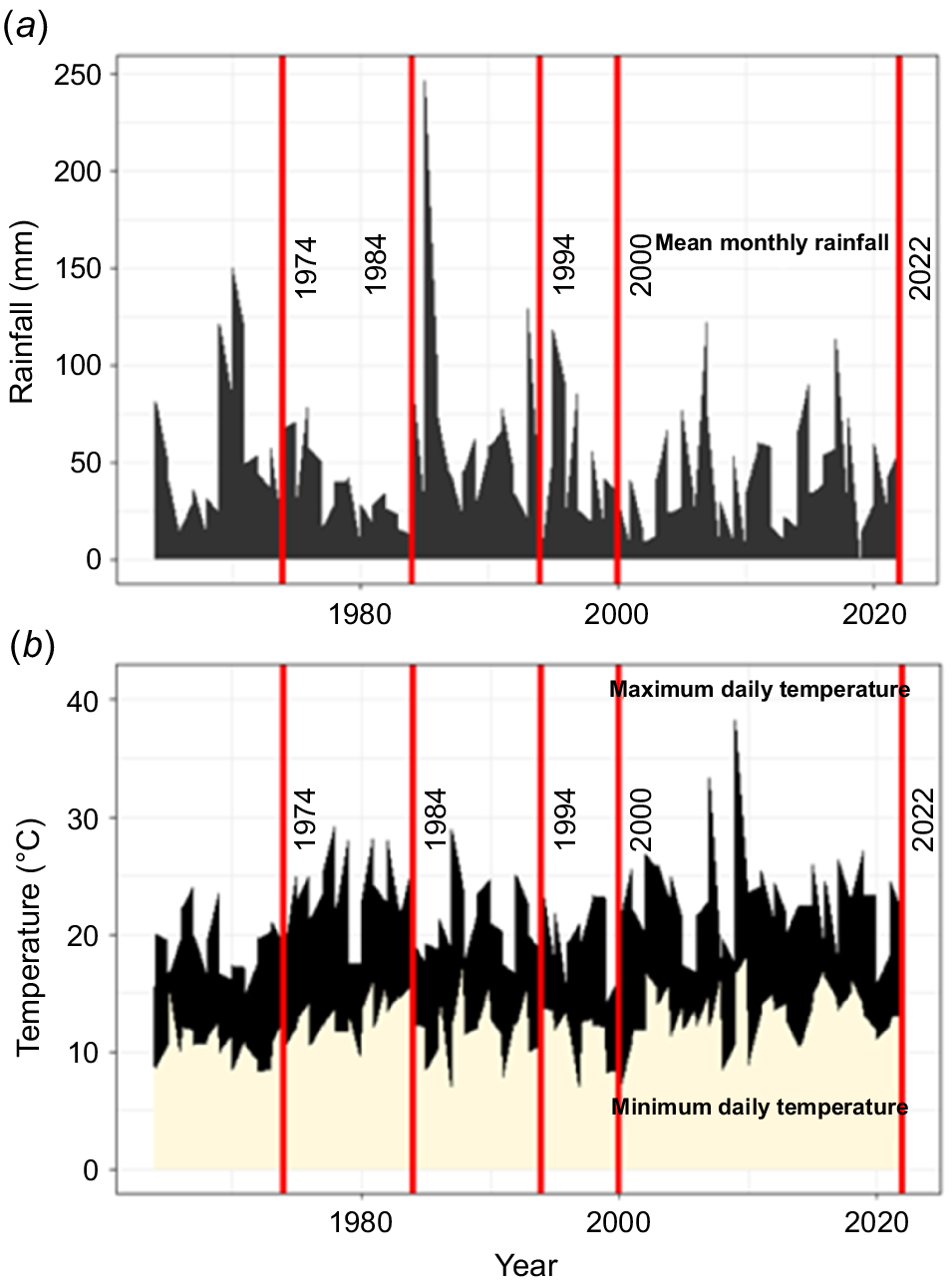
A large part of the Domain is covered by vegetation dominated by native species (Fig. 1). The major dominants are eucalypts (Eucalyptus), sheoaks (Allocasuarina) and tussock grasses in the genera Themeda and Poa (see vegetation map in Kirkpatrick (2004); vascular plant nomenclature follows de Salas and Baker (2023)) The substrate is almost entirely Jurassic dolerite, on which there have formed black prairie soils (dermosols). In the latter part of the 20th century, the Domain was inhabited by native possums (Trichosurus vulpecula (Kerr)) and Pseudocheirus peregrinus (Winge)). There were no signs or sightings of macropods and small digging marsupials during sampling between 1974 and 1994. By 2022, the Domain had been occupied by the Bennett’s wallaby (Notamacropus rufogriseus (Desmarest)), the pademelon (Thylogale billardierii (Desmarest)), the brown bandicoot (Isoodon obesulus (Shaw)) and the nationally endangered eastern barred bandicoot (Parameles gunnii (Gray)), with all being observed during sampling, as were widespread scats and diggings. This putative return to the remnant was possibly as a result of dog control in suburbia, as many people used to let their dogs roam freely at all times of day and night even in the late 20th century, a practice that has now ceased (J. B. Kirkpatrick, pers. obs.).
Many of the nature conservation values most dependent on the Domain are those associated with the nationally listed Tasmanian Themeda triandra tussock grassland. To be regarded as being in good condition, this grassland is required to have an intertussock native herb layer, as well as less than 10% tree projective foliage cover. The Domain has populations of many herbs that are listed as rare or threatened. Some occur in grasslands, many in the zone of root suppression beneath woodland and exotic trees and others in wetlands and sheoak thickets (Kirkpatrick 2004).
The Domain was grazed by domestic stock between the British invasion of 1803 and the mid-20th century. In the same period there was much illegal harvesting of trees. Trees and understoreys became less dense (Sorensen and Kirkpatrick 2021). The release from stock grazing and wood hooking in the second half of the 20th century resulted in woody thickening and a consequent increase in fire incidence (Kirkpatrick 1986, 2004; Sorensen and Kirkpatrick 2021). The Hobart City Council currently manages the vegetation of the Domain by mowing parts of it, planned burning other parts, thinning sheoaks where they have formed thickets and by widespread weeding of non-natives, particularly woody non-natives. Recently, the managers have also attempted to reintroduce native herb species, initially in cages, to parts of the Domain from which they had disappeared, but this gardening activity is so far experimental.
Data collection
In 1974, one of us (JBK) used a random number table to locate 75 quadrats within the vegetation dominated by native species on the Domain. Sixty-nine of these quadrats avoided being developed for recreation or roads by the last sampling in 2022/23 (Fig. 1). Approximate relocation in 1984, 1994 and 2000 was achieved by navigating using an aerial photograph on which all trees were visible with pinholes for each quadrat location. In 2000, photographs were taken of each quadrat and a Garmin GPS, accurate to 10 m, was used to obtain locations. The geocoordinates and photographs facilitated relocation in 2022.
The quadrats were 1 × 15 m, laid parallel to the contours. Observable vascular plant species above or in the quadrat were recorded in December. The senior author was an observer at all times. The top right-hand corner of the quadrat was used as the point in point-centred-quarter sampling (Mueller-Dombois and Ellenberg 1974) of the nearest tree in each quarter. Quarters were oriented with the quadrat. The circumference at breast height (1.3 m) and species of each tree were recorded, along with distance from the central point. The senior author was an observer at all times.
The slope at each point was measured using a clinometer and the aspect was measured using a compass, and later converted into a scale representing topographically controlled moistness (1 = north-west (the driest aspect); 2 = north and west; 3 = north-east and south-west; 4 = east and south; 5 = south-east (the moistest aspect)). Equinoxial solar radiation was calculated using the formula of Nunez (1983). Altitude was obtained from topographic maps. Whether the site was mown or not was recorded at each time. Previously published fire mapping (Kirkpatrick 1986, 2004) and the fire history layer in ListMap were used to determine the number of fires in the previous decade, fire incidence in the previous 2 years and the number of years since the last fire for each quadrat.
Data analysis
Minitab21.4. (Minitab, LLC, 2023, https://www.minitab.com) was used for all analyses. Chi-squared test was used to determine the significance of relationships among class variables. Year of sampling was related to the following: fire in the past 2 years; stems per hectare of species and tree-size classes; and the presence/absence of individual taxa. Mowing presence/absence was related to the presence/absence of individual taxa, as was fire in the past 2 years. One way ANOVA with Tukey’s test was used to determine the significance of relationships between class and continuous variables. Year of sampling was related to number of fires in the past decade, years since the last fire, and species richness variables. Linear models were created in the ‘general linear model’ routine to explain richness variables. The variable with the weakest explanatory power was successively removed until only significant variables remained. The predictor variables included in the modelling process were as follows: year; mown (yes/no), burned in the past 2 years (yes/no); years since fire; number of fires in the past decade; equinoxial radiation (MJ m−2 day−1); aspect (1–5); slope (°); and altitude (m). Year of sampling was treated as a random variable, whereas all others were fixed. The normality of the residuals was examined to determine the suitability of the models.
Results
Fire history
The number of fires increased between 1974 and 2000. The average number of fires in the previous 10 years to sampling in 1974 was 0.6, leaping to 2.06 in 2022 (Table 1). There was much greater differentiation between years in the incidence of fires in the 2 years previous to sampling (χ2 = 51.8, d.f. = 4, P < 0.001), with a range from zero in 2001 to approximately half of the plots in 1984 (Table 1). The mean number of years since the last fire was greater in 2022 than in 1974 and 1984. The apparent contradiction between this result and the high decadal fire frequency for 2022 is resolved by the increasing spread of values between 1974 and 2022 (Fig. 3).
Variable | 1974 | 1984 | 1994 | 2000 | 2022 | Significance | |
---|---|---|---|---|---|---|---|
Mean number of fires in past decade | 0.54a | 0.8b | 0.51b | 0.54b | 2.06b | *** | |
% of plots burnt in last 2 years prior to survey | 8.70 | 50.72 | 30.43 | 0.00 | 33.33 | *** (C) | |
Mean time since last fire (years) | 7.75b | 7.83b | 11.94ab | 10.83ab | 13.42a | ** |
Values within a row followed by the same letter are not statisitically signficantly different (at P = 0.05).
Significance results (bold type) are for one-way ANOVA and chi-squared test (C). ***P < 0.001, **P < 0.01.
Changes in tree structure and dominance
The total number of native stems per hectare increased steadily between 1974, when it was 98, and 2023, when it was 254 (Table 2). Allocasuarina verticillata was the strongest proportionate increaser in density, followed by Bursaria spinosa, Acacia mearnsii and Eucalyptus viminalis. Eucalyptus globulus and E. pulchella did not significantly vary in density through time (Table 2). The density of stems <10 cm in diameter increased until 2000 and then decreased between 2000 and 2023 because plant growth, rather than plant death, resulted in individuals being reclassified into the 10–30 cm diameter class (Table 2). The density of stems 10–30 cm in diameter increased steadily over the period, whereas the density of those larger than 30 cm increased markedly only between 2000 and 2023 (Table 2).
Species/size category | 1974 | 1984 | 1994 | 2000 | 2023 | Significance | |
---|---|---|---|---|---|---|---|
Eucalyptus globulus | 3 | 3 | 3 | 8 | 7 | n.s. | |
Eucalyptus pulchella | 2 | 8 | 6 | 8 | 11 | n.s. | |
Eucalyptus viminalis | 27 | 31 | 24 | 52 | 51 | *** | |
Allocasuarina verticillata | 24 | 45 | 30 | 61 | 104 | *** | |
Acacia mearnsii | 12 | 14 | 17 | 30 | 24 | * | |
Bursaria spinosa | 9 | 10 | 12 | 26 | 32 | *** | |
Stems <10 cm in diameter | 33 | 60 | 55 | 115 | 82 | *** | |
Stems 10–30 cm in diameter | 35 | 42 | 52 | 63 | 113 | *** | |
Stems >30 cm in diameter | 30 | 21 | 36 | 37 | 59 | *** | |
Stems total | 98 | 123 | 143 | 215 | 254 | *** |
n.s., P > 0.05; *P < 0.05; ***P < 0.001 (chi-squared test).
Changes in species richness
Native species richness was higher than for 1974 in the period 1984–2000 (Table 3). There was no variation in the percentage of native species among years (Table 3). In the linear model for native species richness, it was depressed by mowing and was higher in moist, sheltered situations, on steeper slopes and at high altitudes. In the linear model for percentage of native species, the value was depressed by mowing and time elapsed since the last fire and higher in places burned in the previous 2 years, on steep slopes and at higher altitude (Table 4).
Variable | 1974 | 1984 | 1994 | 2000 | 2022 | Significance | |
---|---|---|---|---|---|---|---|
Native species richness | 14b | 17.36a | 16.64a | 16.8a | 15.88ab | ** | |
Native richness (%) | 77.44 | 72.64 | 74.87 | 76.36 | 76.38 | n.s. | |
Non-native species richness | 4.07b | 6.25a | 5.49a | 5.04ab | 5.04ab | *** | |
Native tree richness | 0.91c | 1.01c | 1.83b | 1.84b | 2.58a | *** |
Values within a row followed by the same letter are not signficantly different (at P = 0.05; Tukey’s test).
n.s., P > 0.05; **P < 0.01; ***P < 0.001 (ANOVA).
Variable | Year | Not mown | Fires 10 years | Time since fire | Not burn 2 years | Equinox radiation | Slope (°) | Aspect | Altitude (m) | R2 | |
---|---|---|---|---|---|---|---|---|---|---|---|
Native species richness | *** | *** | n.s. | n.s. | n.s. | -** | ** | *** | *** | 29.95 | |
Native richness (%) | n.s. | ** | n.s. | -** | -** | n.s. | *** | n.s. | * | 16.22 | |
Non-native richness | *** | -* | n.s. | ** | n.s. | -** | -** | n.s. | n.s. | 17.64 | |
Native tree richness | *** | *** | -* | -** | n.s. | n.s. | ** | ** | n.s. | 35.38 |
n.s., P > 0.05; *P < 0.05; **P ≤ 0.01; ***P < 0.001, -, negative relationship.
Non-native species richness was higher in 1984 and 1994 than in 1974 (Table 3). Mowing, time since fire, gentle slopes and moister topographic positions all resulted in higher values for non-native species richness (Table 4).
Native tree species richness increased through the period of observation (Table 3). It was negatively affected by mowing and positively affected by high fire frequencies at both the decade and 2-year time scales. It was also higher on steeper slopes and in moist topographic situations (Table 4).
Changes at the species level
Allocasuarina verticillata was the only significantly varying consistent increaser of the 67 most frequent taxa (Table 5). High frequency of A. verticillata was associated with dry and steep sites at higher altitudes, frequent fire and a lack of mowing (Table 6). The native perennial herbs Acaena echinata and Leptorhynchos squamatus and the non-native shrubby weed Chrysanthemoides monilifera were the only significantly varying consistent decreasers (Table 5). Acaena echinata was associated with moist aspects and higher altitudes and decreased with time elapsed since the last fire (Table 6). Leptorhynchos squamatus was associated with gentle slopes and moister situations and was favoured by mowing and infrequent fire (Table 6). Chrysanthemoides monilifera was associated with steep slopes and a lack of mowing (Table 6).
Taxon | 1974 | 1984 | 1994 | 2000 | 2022 | Significance | |
---|---|---|---|---|---|---|---|
Annual herbs | |||||||
ACirsium vulgare | 0.0 | 5.8 | 2.9 | 1.4 | 21.7 | *** | |
APetrorhagia nanteuilii | 2.9 | 26.1 | 26.1 | 47.8 | 29.0 | *** | |
ASonchus spp. | 5.8 | 37.7 | 4.3 | 4.3 | 18.8 | *** | |
ACentaurium erythraea | 7.2 | 18.8 | 33.3 | 17.4 | 46.4 | *** | |
AVicia spp. | 11.6 | 17.4 | 13.0 | 4.3 | 10.1 | n.s. | |
Senecio spp. | 37.7 | 53.6 | 33.3 | 42.0 | 58.0 | * | |
Perennial herbs | |||||||
ALysimachia arvensis | 2.9 | 13.0 | 1.4 | 1.4 | 11.6 | ** | |
AMedicago spp. | 2.9 | 21.7 | 2.9 | 0.0 | 0.0 | *** | |
ATrifolium spp. | 4.3 | 2.9 | 13.0 | 14.5 | 11.6 | n.s. | |
Leptorhynchos nitidulus | 7.2 | 10.1 | 4.3 | 17.4 | 13.0 | n.s. | |
BLinum spp. | 13.0 | 36.2 | 17.4 | 11.6 | 23.2 | ** | |
Ranunculus lappaceus | 11.6 | 10.1 | 2.9 | 10.1 | 8.7 | n.s. | |
Oxalis perennans | 44.9 | 40.6 | 17.4 | 14.5 | 47.8 | *** | |
Wahlenbergia spp. | 11.6 | 15.9 | 4.3 | 11.6 | 11.6 | n.s. | |
AHypochaeris radicata | 27.5 | 60.9 | 31.9 | 15.9 | 23.2 | *** | |
Hackelia suaveolens | 0.0 | 17.4 | 13.0 | 11.6 | 20.3 | *** | |
Chrysocephalum apiculatum | 7.2 | 11.6 | 20.3 | 11.6 | 2.9 | * | |
AUrospermum dalechampii | 53.6 | 60.9 | 59.4 | 53.6 | 47.8 | n.s. | |
Gonocarpus tetragynus | 18.8 | 18.8 | 14.5 | 23.2 | 11.6 | n.s. | |
ASanguisorba minor | 11.6 | 23.2 | 20.3 | 13.0 | 4.3 | * | |
APlantago lanceolata | 78.3 | 79.7 | 87.0 | 85.5 | 63.8 | ** | |
Plantago varia | 58.0 | 33.3 | 20.3 | 30.4 | 27.5 | *** | |
Goodenia lanata | 11.6 | 11.6 | 4.3 | 2.9 | 4.3 | n.s. | |
Geranium potentilloides | 24.6 | 33.3 | 14.5 | 15.9 | 10.1 | ** | |
Acaena echinata | 73.9 | 63.8 | 59.4 | 58.0 | 40.6 | ** | |
Leptorhynchos squamatus | 29.0 | 23.2 | 23.2 | 18.8 | 5.8 | * | |
Annual grasses | |||||||
AAira spp. | 31.9 | 55.1 | 43.5 | 53.6 | 52.2 | * | |
ABriza spp. | 30.4 | 34.8 | 14.5 | 29.0 | 33.3 | n.s. | |
AVulpia spp. | 14.5 | 20.3 | 18.8 | 11.6 | 15.9 | n.s. | |
Perennial grasses | |||||||
Microlaena stipoides | 1.4 | 27.5 | 5.8 | 18.8 | 39.1 | *** | |
ADactylis glomerata | 13.0 | 14.5 | 31.9 | 23.2 | 27.5 | * | |
AHolcus lanatus | 8.7 | 2.9 | 5.8 | 5.8 | 8.7 | n.s. | |
Anthosachne scabra | 53.6 | 89.9 | 82.6 | 85.5 | 71.0 | *** | |
Austrostipa spp. | 73.9 | 94.2 | 94.2 | 95.7 | 91.3 | *** | |
Deyeuxia quadriseta | 0.0 | 21.7 | 31.9 | 17.4 | 18.8 | *** | |
Poa rodwayi | 88.4 | 85.5 | 85.5 | 89.9 | 94.2 | n.s. | |
Rytidosperma spp. | 94.2 | 84.1 | 94.2 | 98.6 | 81.2 | ** | |
Themeda triandra | 91.3 | 94.2 | 92.8 | 95.7 | 78.3 | ** | |
Dichelachne spp. | 76.8 | 71.0 | 75.4 | 63.8 | 63.8 | *** | |
Lachnagrostis aemula | 58.0 | 62.3 | 71.0 | 42.0 | 33.3 | *** | |
Pentapogon quadrifidus | 14.5 | 21.7 | 10.1 | 10.1 | 1.4 | ** | |
ALolium perenne | 17.4 | 10.1 | 10.1 | 7.2 | 4.3 | n.s. | |
Graminoids | |||||||
Schoenus apogon | 1.4 | 47.8 | 44.9 | 31.9 | 29.0 | *** | |
Lomandra longifolia | 18.8 | 24.6 | 30.4 | 27.5 | 31.9 | n.s. | |
Dianella spp. | 23.2 | 34.8 | 44.9 | 52.2 | 33.3 | ** | |
Lepidosperma laterale | 21.7 | 26.1 | 34.8 | 37.7 | 24.6 | n.s. | |
Lepidosperma gunnii | 24.6 | 29.0 | 44.9 | 34.8 | 24.6 | * | |
Carex breviculmis | 68.1 | 71.0 | 62.3 | 76.8 | 33.3 | *** | |
Shrubs | |||||||
Styphelia humifusa | 10.1 | 14.5 | 23.2 | 20.3 | 33.3 | *** | |
Olearia ramulosa | 8.7 | 14.5 | 23.2 | 23.2 | 17.4 | n.s. | |
Lissanthe strigosa | 8.7 | 14.5 | 18.8 | 15.9 | 15.9 | n.s. | |
Bossiaea prostrata | 53.6 | 46.4 | 59.4 | 72.5 | 52.2 | * | |
ARosa rubiginosa | 10.1 | 13.0 | 15.9 | 15.9 | 7.2 | n.s. | |
AAcacia pycnantha | 5.8 | 8.7 | 8.7 | 10.1 | 1.4 | n.s. | |
Pimelea humilis | 44.9 | 40.6 | 39.1 | 34.8 | 34.8 | n.s. | |
Hibbertia hirsuta | 11.6 | 10.1 | 7.2 | 7.2 | 7.2 | n.s. | |
AChrysanthemoides monilifera | 15.9 | 13.0 | 7.2 | 4.3 | 2.9 | * | |
Trees | |||||||
Eucalyptus pulchella | 2.9 | 1.4 | 7.2 | 5.8 | 14.5 | * | |
Allocasuarina verticillata | 8.7 | 26.1 | 47.8 | 47.8 | 72.5 | *** | |
Eucalyptus globulus | 1.4 | 4.3 | 7.2 | 7.2 | 10.1 | n.s. | |
Acacia mearnsii | 11.6 | 8.7 | 14.5 | 11.6 | 39.1 | *** | |
Eucalyptus viminalis | 14.5 | 13.0 | 39.1 | 30.4 | 39.1 | *** | |
Dodonaea viscosa | 7.2 | 5.8 | 5.8 | 7.2 | 17.4 | n.s. | |
Bursaria spinosa | 37.7 | 31.9 | 47.8 | 60.9 | 50.7 | ** | |
Geophytes | |||||||
Thelymitra spp. | 1.4 | 17.4 | 15.9 | 11.6 | 2.9 | ** | |
Arthropodium milleflorum | 30.4 | 24.6 | 30.4 | 39.1 | 26.1 | n.s. | |
ARomulea longifolia | 36.2 | 59.4 | 30.4 | 14.5 | 10.1 | *** |
Taxa are ordered from greatest to smallest change in frequency from 1974 to 2022 within each life-form class. Bold values are the highest frequencies for a taxon. Underlined values exhibit a continuous increase or decrease across the years. Significance of change in time was determined by chi-squared analysis. *P < 0.05, **P < 0.01, ***P < 0.001.
n.s., not significant.
Taxon | Mown status | Number of fires in the decade previous to surveying | Time since fire | Burnt status (2 years prior to surveying) | Equinoxial radiation | Slope (°) | Aspect | Altitude (m) | |
---|---|---|---|---|---|---|---|---|---|
Annual herbs | |||||||||
ACentaurium erythraea | n.s. | n.s. | n.s. | n.s. | ***– | n.s. | ***+ | ***+ | |
ACirsium vulgare | **– | ***+ | n.s. | *+ | **+ | n.s. | *– | **– | |
APetrorhagia nanteuilii | ***– | ***+ | ***– | ***+ | ***+ | n.s. | ***– | n.s. | |
Senecio spp. | ***– | **+ | ***– | **+ | **– | n.s. | n.s. | **+ | |
ASonchus spp. | ***– | **+ | *– | ***+ | n.s. | n.s. | ***- | n.s. | |
AVicia spp. | **+ | *– | **+ | n.s. | *- | **– | ***+ | n.s. | |
Perennial herbs | |||||||||
Acaena echinata | n.s. | n.s. | *– | n.s. | n.s. | n.s. | ***+ | **+ | |
Chrysocephalum apiculatum | n.s. | *– | n.s. | *– | **+ | n.s. | n.s. | ***– | |
Geranium potentilloides | n.s. | n.s. | n.s. | n.s. | n.s. | n.s. | *+ | *+ | |
Gonocarpus tetragynus | n.s. | n.s. | n.s. | n.s. | ***– | **+ | ***+ | **+ | |
Goodenia lanata | n.s. | n.s. | **– | *+ | n.s. | n.s. | n.s. | n.s. | |
Hackelia suaveolens | n.s. | n.s. | n.s. | n.s. | n.s. | n.s. | n.s. | n.s. | |
AHypochaeris radicata | ***+ | **– | **+ | n.s. | n.s. | n.s. | **+ | n.s. | |
Leptorhynchos nitidulus | n.s. | n.s. | *– | n.s. | n.s. | n.s. | n.s. | n.s. | |
Leptorhynchos squamatus | ***+ | ***– | n.s. | *– | *– | **– | ***+ | n.s. | |
BLinum spp. | *– | *+ | n.s. | **+ | *– | n.s. | ***– | n.s. | |
ALysimachia arvensis | n.s. | ***+ | n.s. | *+ | n.s. | n.s. | n.s. | n.s. | |
AMedicago spp. | ***+ | **– | *+ | n.s. | n.s. | **– | *+ | n.s. | |
Oxalis perennans | n.s. | n.s. | n.s. | n.s. | **– | n.s. | n.s. | n.s. | |
APlantago lanceolata | ***+ | ***– | **+ | n.s. | *– | *– | ***+ | n.s. | |
Plantago varia | n.s. | *– | n.s. | **– | *– | n.s. | ***+ | n.s. | |
Ranunculus lappaceus | n.s. | n.s. | n.s. | n.s. | ***– | ***+ | ***+ | **+ | |
ASanguisorba minor | n.s. | n.s. | n.s. | n.s. | n.s. | n.s. | **- | n.s. | |
ATrifolium spp. | ***+ | n.s. | *+ | n.s. | n.s. | ***– | ***+ | n.s. | |
AUrospermum dalechampii | *+ | *– | *+ | n.s. | ***+ | n.s. | n.s. | ***– | |
Wahlenbergia spp. | n.s. | n.s. | n.s. | n.s. | *– | n.s. | n.s. | **+ | |
Annual grasses | |||||||||
AAira spp. | ***+ | n.s. | n.s. | n.s. | n.s. | ***– | *+ | n.s. | |
ABriza spp. | n.s. | *+ | n.s. | n.s. | ***– | n.s. | *+ | ***+ | |
AVulpia spp. | ***+ | n.s. | n.s. | *– | *– | ***– | ***+ | n.s. | |
Perennial grasses | |||||||||
Anthosachne scabra | n.s. | n.s. | n.s. | n.s. | n.s. | n.s. | n.s. | n.s. | |
Austrostipa spp. | n.s. | *+ | n.s. | n.s. | n.s. | *+ | n.s. | **+ | |
ADactylis glomerata | ***+ | n.s. | ***+ | n.s. | *– | **– | n.s. | n.s. | |
Deyeuxia quadriseta | **– | n.s. | n.s. | n.s. | ***- | **+ | n.s. | ***+ | |
Dichelachne spp. | *– | **+ | ***– | **+ | n.s. | n.s. | n.s. | ***+ | |
AHolcus lanatus | n.s. | n.s. | ***+ | n.s. | n.s. | ***– | **+ | *- | |
Lachnagrostis aemula | n.s. | **– | *– | n.s. | n.s. | n.s. | ***+ | *+ | |
ALolium perenne | ***+ | ***– | ***+ | **– | *– | **– | ***+ | *– | |
Microlaena stipoides | *– | ***+ | n.s. | **+ | **– | n.s. | n.s. | *+ | |
Pentapogon quadrifidus | **+ | n.s. | n.s. | n.s. | n.s. | n.s. | n.s. | n.s. | |
Poa rodwayi | n.s. | n.s. | n.s. | *– | n.s. | n.s. | ***+ | n.s. | |
Rytidosperma spp. | n.s. | n.s. | n.s. | n.s. | n.s. | n.s. | n.s. | n.s. | |
Themeda triandra | *+ | n.s. | n.s. | n.s. | n.s. | n.s. | n.s. | n.s. | |
Graminoids | |||||||||
Carex breviculmis | n.s. | ***– | n.s. | n.s. | n.s. | n.s. | **+ | ||
Dianella spp. | ***– | n.s. | n.s. | n.s. | n.s. | ***+ | n.s. | ||
Lepidosperma gunnii | n.s. | n.s. | *– | n.s. | n.s. | n.s. | n.s. | **+ | |
Lepidosperma laterale | ***- | n.s. | **– | n.s. | *+ | ***+ | ***– | *– | |
Lomandra longifolia | ***– | n.s. | n.s. | n.s. | ***– | ***+ | n.s. | n.s. | |
Schoenus apogon | n.s. | n.s. | *+ | n.s. | ***– | n.s. | ***+ | *+ | |
Shrubs | |||||||||
AAcacia pycnantha | n.s. | n.s. | *– | n.s. | ***+ | n.s. | *– | n.s. | |
Bossiaea prostrata | n.s. | n.s. | **– | n.s. | n.s. | n.s. | *+ | ***+ | |
AChrysanthemoides monilifera | *– | n.s. | n.s. | n.s. | n.s. | **+ | n.s. | n.s. | |
Hibbertia hirsuta | n.s. | n.s. | n.s. | n.s. | ***– | n.s. | ***+ | ***+ | |
Lissanthe strigosa | n.s. | n.s. | n.s. | n.s. | ***– | n.s. | ***+ | **+ | |
Olearia ramulosa | ***– | n.s. | n.s. | n.s. | ***– | ***+ | **+ | ***+ | |
Pimelea humilis | n.s. | n.s. | n.s. | n.s. | n.s. | **+ | n.s. | n.s. | |
ARosa rubiginosa | n.s. | **– | *+ | n.s. | ***– | n.s. | n.s. | n.s. | |
Styphelia humifusa | n.s. | *+ | n.s. | n.s. | ***– | **+ | n.s. | ***+ | |
Trees | |||||||||
Acacia mearnsii | **– | **+ | n.s. | n.s. | n.s. | n.s. | n.s. | n.s. | |
Allocasuarina verticillata | ***– | ***+ | ***– | ***+ | **+ | ***+ | ***– | *+ | |
Bursaria spinosa | **– | n.s. | n.s. | n.s. | n.s. | ***+ | *+ | n.s. | |
Dodonaea viscosa | **– | **+ | *– | n.s. | n.s. | n.s. | n.s. | n.s. | |
Eucalyptus globulus | n.s. | n.s. | n.s. | n.s. | ***– | n.s. | ***+ | n.s. | |
Eucalyptus pulchella | *– | n.s. | n.s. | n.s. | ***– | n.s. | ***+ | *+ | |
Eucalyptus viminalis | n.s. | n.s. | n.s. | n.s. | n.s. | n.s. | **+ | **+ | |
Geophytes | |||||||||
Arthropodium milleflorum | n.s. | n.s. | n.s. | n.s. | ***– | *+ | ***+ | ***+ | |
ARomulea longifolia | ***+ | n.s. | n.s. | n.s. | n.s. | ***– | n.s. | n.s. | |
Thelymitra spp. | n.s. | n.s. | n.s. | n.s. | n.s. | n.s. | n.s. | n.s. |
In total, 24 (36%) of the 67 taxa with sufficient data for chi-squared analysis did not change in frequency between years (Table 5). The number of significantly varying taxa with peak values in each year was not different between years (χ2 = 5.26, d.f. = 4, P > 0.05), although there was a range from 5 in 1974 to 13 in 1984.
Most of the more common taxa responded to mowing, with the numbers approximately equal between those negatively and positively affected (Table 6). Most, but not all, native species were reduced by mowing, the reverse of non-native species. A similar pattern was observed for fire, which tended to favour more natives than non-natives (Table 6). Most taxa were concentrated in the moister areas as indicated by aspect and solar radiation incidence.
At the reserve scale, many of the rare and threatened native species that occurred on the Domain have become rarer or apparently locally extinct between 2000 and 2022. We did not relocate any populations of Lepidium hyssopifolium or Eryngium ovinum. Vittadinia cuneata has been reduced from three populations to one population. Vittadinia muelleri has been reduced to one population from a much larger number in 2000. Carex tasmanica has continued to decline, as has Scleranthus fasciculatus. Lepidium pseudotasmanicum does not appear to have changed. In contrast, Hydrocotyle laxiflora, which is found only on the Domain in Tasmania, has increased its number of populations where felled Allocasuarina verticillata has been burned.
Discussion
Stability and conservation?
There were very few instances of consistent increases or decreases for the full 1974–2022 period in the variables we examined. A large proportion of the steady increases related to A. verticillata, which increased in stems per hectare, in frequency in quadrats, in stems of 10–30 cm diameter per hectare, and in total stems, indicating a dramatic change towards closed-forest and bigger individuals over much of the study area. The only other tree species that steadily increased in its stem density was Bursaria spinosa, a species that has also occupied the understorey in Cumberland Plain Woodland where fire is infrequent (Watson et al. 2009; Watson and Morris 2020). Both trees resprout after fire from their bases and are eaten by native animals (Hazeldine and Kirkpatrick 2015). Rabbits can also eliminate A. verticillata seedlings and resprouts (Cooke 1988), but the low numbers of rabbits on the Domain have not evinced such an effect. The combination of fire with either subsequent intense grazing by stock and/or natives (Kirkpatrick et al. 2007) or mechanical stem thinning (Kirkpatrick and Jenkinson 2022) appears to be necessary in eastern Tasmania to prevent woody thickening. The increase of A. verticillata and B. spinosa on the Domain occurred after stock grazing was removed (Sorensen and Kirkpatrick 2021) and before a population of macropods re-established in numbers commensurate with similar environments outside the city.
A very recent mechanical thinning program run by the Hobart City Council (Jeffery and Smith 2019) has been effective in reversing thickening where implemented (Kirkpatrick and Jenkinson 2022), but not in reversing thickening back to its 2000 levels on the Domain as a whole. In the absence of mowing or thinning, the succession that is taking place is towards a closed-forest largely consisting of A. verticillata, except in the topographically moistest areas, where Dodonaea viscosa appears likely to become the dominant in the type of closed-forest sometimes labelled as ‘dry rainforest’ (Pollard 2006), as it has done in a long unburned quarry reserve (Fig. 4). The understorey dominance of these small tree species is often followed by dieback of the overstorey eucalypts (Kirkpatrick and Marks 1985; Fig. 4).
Vegetation of fenced quarry on western slopes of the Domain (bottom right-hand (SE) corner 94/55 5253704N, 526342E), an area unburned for at least 60 years. Dodonaea viscosa closed-forest is bottle green. Allocasuarina verticillata closed-forest is greyish-green. The dead upper branches of dead eucalypts are visible throughout.
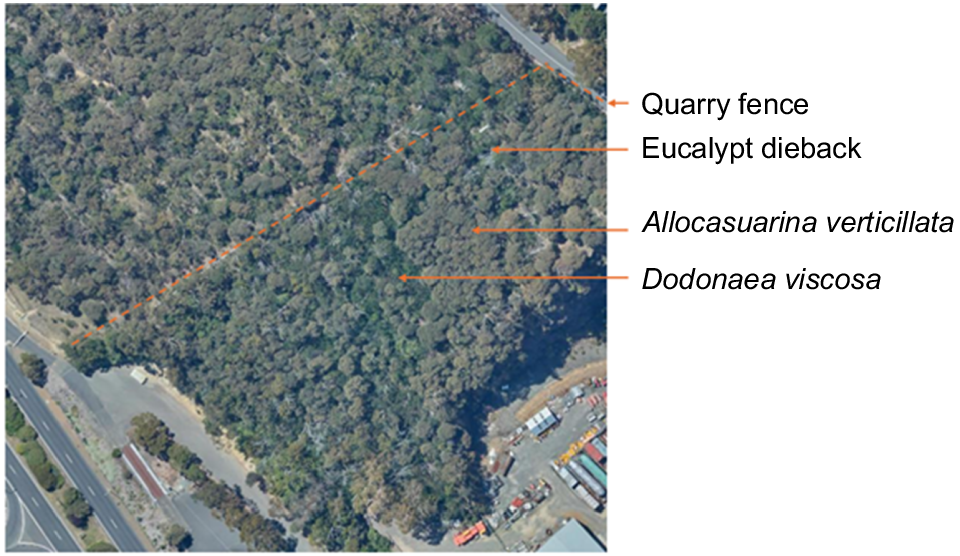
Given that both D. viscosa and A. verticillata will regenerate in small tree-fall gaps when stands are mature (Kirkpatrick 1995; Pollard 2006), they fit one of the major criteria for rainforest, namely, self-replacement without exogenous disturbance (Bowman 2008). Thus, if this transition to closed-forest were to be allowed, the vegetation would become much more stable, but probably also much more native-species poor, given that A. verticillata excludes most ground-stratum species when in its thicket phase. The same phenomenon of understorey species depletion occurs with rainforest invasion in Queensland (Baker et al. 2020) and Leptospermum scoparium invasion of grassy woodland in the Grampians, Victoria (Price and Morgan 2008). Understorey thickets of A. verticillata are also associated with dieback of eucalypts, particularly in drought conditions (Kirkpatrick 1997). Eucalypts cannot regenerate in dry rainforest gaps (Pollard 2006).
The three variables that steadily decreased in magnitude between 1974 and 2022 were all species frequencies. Two of the species, Leptorhynchos squamatus and Acaena echinata, are common lowland grassy woodland inter-tussock herbs in Tasmania (Kirkpatrick et al. 1988). They do not survive the dense shade of Allocasuarina thickets, along with the four other perennial herbs and six perennial grasses, with their lowest frequencies occurring in 2022. The only significantly varying perennial grass that had its highest frequency in 2022 was Microlaena stipoides, which grows well in shade (Magcalemacandog and Whalley 1991). The decrease in frequency of Themeda triandra between 2000 and 2022 may have implications for non-native species invasion, as T. triandra takes up the nitrates that many non-natives thrive on (Prober and Lunt 2009). However, there are few of the non-native species that prosper with shade and intense root competition.
The third steadily decreasing species was a woody weed Chrysanthemoides monilifera, which once seemed to threaten the native understorey vegetation of the Domain (Paterson and Volframs 1976; Kirkpatrick 1986). Its numbers have been reduced by extensive weeding and more frequent burning. The recent occupation of the Domain by the pademelons and wallabies that had been absent for many decades could also have contributed to the decline of C. monilifera, because they selectively browse its leaves and small stems (Scurr et al. 2008).
Overall, there is no indication in the data that non-native species as a whole were increasing or decreasing between 1974 and 2022. Variation in non-native richness relates to recent fire history and recent climatic conditions, with non-natives seemingly favoured by longer time periods after fire as was also observed by Watson and Morris (2020) in Cumberland Plain Woodland. The observation time with the highest non-native richness was 1984 after a long drought. Non-natives also appear to have been promoted by mowing, but have attained constancy in the mown areas, which in themselves have largely been constant in location. The observations of Kirkpatrick (1986) and Fensham (1989) suggest that non-natives can co-exist in Tasmanian grassy woodland without affecting the native plants.
Management implications
Our analyses suggested that the Domain supports potentially stable synthetic vegetation sensuBridgewater (1988). The major way of realising this stability is to stabilise the abundance of small trees, particularly Allocasuarina verticillata, as declines in native herbs and grasses appear consequent on their thickening. The Hobart City Council is well on the way to stabilising the small trees with a combination of thinning and burning (Kirkpatrick and Jenkinson 2022).
Continuation of active control of woody weeds, such as Chrysanthemoides monilifera, Acacia pycnantha, Rosa rubiginosa, Cotoneaster spp. and Ulex europaeus, will also be necessary to achieve stability, as they have all demonstrated the capability to dominate the understorey on parts or all of the Domain. Urospermum dalechampii, an introduced herbaceous daisy with a fleshy root stock, has been subjected to herbiciding since 2021. Its widespread distribution on the drier parts of the Domain has not changed statistically since 1974, suggesting that it may not be as appropriate a target as the invasive non-native shrubs.
Broad floristic and structural stability may be achieved by managing the density of Allocasuarina verticillata in a variety of ways. However, the decline in listed and uncommon native herbs may require specific actions to maintain rare environmental conditions or to provide a specific set of disturbances where disseminules are still available. The unanticipated increase in Hydrocotyle laxiflora as a result of the Allocasuarina verticillata thinning program provides an educational example of unexpected outcomes.
Conclusions
We conclude that the vegetation of the Domain has been as dynamic in its floristic and structural characteristics as that of Kings Park (Crosti et al. 2007), but that this dynamism is largely attributable to the explosion in the abundance of Allocasuarina verticillata which, in turn, has reduced native perennial herbs and native perennial grasses. These changes have not shifted the proportionate richness of native and non-native plants at the quadrat scale, indicating stability in nativeness in the remnant as a whole.
This and previous work (Kirkpatrick and Jenkinson 2022) has indicated that there is a feasible, and largely adopted, management pathway to stabilisation of the abundance of A. verticillata.
Data availability
The data that support this study are available in the article and accompanying online supplementary material. Further data are available on request to the senior author.
References
Aronson MFJ, La Sorte FA, Nilon CH, Katti M, Goddard MA, Lepczyk CA, Warren PS, Williams NSG, Cilliers S, Clarkson B, Dobbs C, Dolan R, Hedblom M, Klotz S, Kooijmans JL, Kuhn I, MacGregor-Fors I, McDonnell M, Mortberg U, Pysek P, Siebert S, Sushinsky J, Werner P, Winter M (2014) A global analysis of the impacts of urbanization on bird and plant diversity reveals key anthropogenic drivers. Proceedings of the Royal Society B: Biological Sciences 281, 20133330.
| Crossref | Google Scholar |
Baker AG, Catterall C, Benkendorff K, Fensham RJ (2020) Rainforest expansion reduces understorey plant diversity and density in open forest of eastern Australia. Austral Ecology 45, 557-571.
| Crossref | Google Scholar |
Bridgewater PB (1988) Synthetic plant communities: problems in definition and management. Flora 180, 139-144.
| Crossref | Google Scholar |
Bridgewater PB, Backshall DJ (1981) Dynamics of some western Australian ligneous formations with special reference to the invasion of exotic species. Vegetatio 46, 141-148.
| Crossref | Google Scholar |
Cooke BD (1988) The effects of rabbit grazing on regeneration of sheoaks, Allocasuarina verticilliata and saltwater ti-trees, Melaleuca halmaturorum, in the Coorong National Park, South Australia. Australian Journal of Ecology 13, 11-20.
| Crossref | Google Scholar |
Crosti R, Dixon KW, Ladd PC, Yates CJ (2007) Changes in the structure and species dominance in vegetation over 60 years in an urban bushland remnant. Pacific Conservation Biology 13, 158-170.
| Crossref | Google Scholar |
de Salas MF, Baker ML (2023) A census of the vascular plants of Tasmania, including Macquarie Island. Tasmanian Herbarium, Tasmanian Museum and Art Gallery, Hobart, Tas, Australia. Available at https://flora.tmag.tas.gov.au/resources/census/
du Toit MJ, Kotze DJ, Cilliers SS (2016) Landscape history, time lags and drivers of change: urban natural grassland remnants in Potchefstroom, South Africa. Landscape Ecology 31, 2133-2150.
| Crossref | Google Scholar |
Fensham RJ (1989) The pre-European vegetation of the Midlands, Tasmania: a floristic and historical analysis of vegetation patterns. Journal of Biogeography 16, 29-45.
| Crossref | Google Scholar |
Florgård C (2007) Preserved and remnant natural vegetation in cities: a geographically divided field of research. Landscape Research 32, 79-94.
| Crossref | Google Scholar |
Hahs AK, McDonnell MJ, McCarthy MA, Vesk PA, Corlett RT, Norton BA, Clemants SE, Duncan RP, Thompson K, Schwartz MW, Williams NSG (2009) A global synthesis of plant extinction rates in urban areas. Ecology Letters 12, 1165-1173.
| Crossref | Google Scholar | PubMed |
Hazeldine A, Kirkpatrick JB (2015) Practical and theoretical implications of a browsing cascade in Tasmanian forest and woodland. Australian Journal of Botany 63, 435-443.
| Crossref | Google Scholar |
Hobbs RJ, Arico S, Aronson J, Baron JS, Bridgewater P, Cramer VA, Epstein PR, Ewel JJ, Klink CA, Lugo AE, Norton D, Ojima D, Richardson DM, Sanderson EW, Valladares F, Vilà M, Zamora R, Zobel M (2006) Novel ecosystems: theoretical and management aspects of the new ecological world order. Global Ecology and Biogeography 15, 1-7.
| Crossref | Google Scholar |
Ives CD, Lentini PE, Threlfall CG, Ikin K, Shanahan DF, Garrard GE, Bekessy SA, Fuller RA, Mumaw L, Rayner L, Rowe R, Valentine LE, Kendal D (2016) Cities are hotspots for threatened species. Global Ecology and Biogeography 25, 117-126.
| Crossref | Google Scholar |
Ivey-Law M, Kirkpatrick JB (2015) Gardening the wild: change in the flora and vegetation of a suburban coastal reserve 1911–2013. Geographical Research 53, 121-133.
| Crossref | Google Scholar |
Kendal D, Zeeman BJ, Ikin K, Lunt ID, McDonnell MJ, Farrar A, Pearce LM, Morgan JW (2017) The importance of small urban reserves for plant conservation. Biological Conservation 213, 146-153.
| Crossref | Google Scholar |
Kirkpatrick JB (1974) Plant invasion and extinction in a suburban coastal reserve. Australian Geographical Studies 12, 107-118.
| Crossref | Google Scholar |
Kirkpatrick JB (1975) Vegetation change in a suburban coastal reserve. Australian Geographical Studies 13, 137-153.
| Crossref | Google Scholar |
Kirkpatrick JB (1986) The viability of bush in cities: 10 years of change in an urban grassy woodland. Australian Journal of Botany 34, 691-708.
| Crossref | Google Scholar |
Kirkpatrick JB (1995) The vegetation of Dover and Erith Islands, Kent Group, Bass Strait. Papers and Proceedings of the Royal Society of Tasmania 129, 25-33.
| Crossref | Google Scholar |
Kirkpatrick JB (2004) Vegetation change in an urban grassy woodland 1974–2000. Australian Journal of Botany 52, 597-608.
| Crossref | Google Scholar |
Kirkpatrick JB, Gilfedder L (1995) Maintaining integrity compared with maintaining rare and threatened taxa in remnant bushland in subhumid Tasmania. Biological Conservation 74, 1-8.
| Crossref | Google Scholar |
Kirkpatrick JB, Jenkinson I (2022) Effects of increasing fire frequency on conservation values in Eucalyptus grassy woodland in the process of invasion by Allocasuarina verticillata. Fire 5, 31.
| Crossref | Google Scholar |
Kirkpatrick JB, Marks F (1985) Observations on drought damage to some native plant species in eucalypt forests and woodlands near Hobart, Tasmania. Papers and Proceedings of the Royal Society of Tasmania 119, 15-21.
| Crossref | Google Scholar |
Magcalemacandog DB, Whalley RDB (1991) Distribution of Microlaena stipoides and its association with introduced perennial grasses in a permanent pasture on the Northern Tablelands of New South Wales. Australian Journal of Botany 39, 295-303.
| Crossref | Google Scholar |
Miller JR, Hobbs RJ (2002) Conservation where people live and work. Conservation Biology 16, 330-337.
| Crossref | Google Scholar |
Nunez M (1983) Estimation of solar radiation received on slopes in Tasmania. Papers and Proceedings of The Royal Society of Tasmania 117, 153-159.
| Crossref | Google Scholar |
O’Shea EM, Kirkpatrick JB (2000) The impact of suburbanization on remnant coastal vegetation in Hobart, Tasmania. Applied Vegetation Science 3, 243-252.
| Crossref | Google Scholar |
Price JN, Morgan JW (2008) Woody plant encroachment reduces species richness of herb-rich woodlands in southern Australia. Austral Ecology 33, 278-289.
| Crossref | Google Scholar |
Prober SM, Lunt ID (2009) Restoration of Themeda australis swards suppresses soil nitrate and enhances ecological resistance to invasion by exotic annuals. Biological Invasions 11, 171-181.
| Crossref | Google Scholar |
Rohwer Y, Marris E (2021) Ecosystem integrity is neither real nor valuable. Conservation Science and Practice 3, e411.
| Crossref | Google Scholar |
Scurr G, Kirkpatrick JB, Daniels GD, McQuillan PB (2008) Biotic resistance to Chrysanthemoides monilifera ssp. monilifera in Tasmania. Austral Ecology 33, 941-950.
| Crossref | Google Scholar |
Sorensen ER, Kirkpatrick JB (2021) Vegetation change in an urban grassy woodland since the early nineteenth century. Papers and Proceedings of The Royal Society of Tasmania 155, 37-54.
| Crossref | Google Scholar |
Watson PJ, Morris EC (2020) Effects of fire frequency and microhabitat on the ground layer in a grassy woodland. Australian Journal of Botany 68, 425-438.
| Crossref | Google Scholar |
Watson PJ, Bradstock RA, Morris EC (2009) Fire frequency influences composition and structure of the shrub layer in an Australian subcoastal temperate grassy woodland. Austral Ecology 34, 218-232.
| Crossref | Google Scholar |
Zeeman BJ, McDonnell MJ, Kendal D, Morgan JW (2017) Biotic homogenization in an increasingly urbanized temperate grassland ecosystem. Journal of Vegetation Science 28, 550-561.
| Crossref | Google Scholar |