Comparison of suckling and artificial rearing on calf growth and milk requirements in pastoral dairy systems
Racheal H. Bryant

A
B
C
Abstract
Dairy calf-rearing practises have the potential to influence profitability through milk requirements at rearing, and affect lifetime productivity and market access from an animal-welfare perspective.
The aim of this research was to compare calf growth and milk requirements by using conventional artificial rearing (AR) and restricted milk allocation with AR and high milk allocation or suckled calves.
Forty-five Friesian × Jersey calves were allocated to be either AR on 6 L/calf.day, (ARC) or 12 L/calf.day, (ARH), or suckled with dams in a cow–calf contact system using partial contact (15 h/day, CCC). AR calves were fed, and intake measured, using an automatic feeder, whereas CCC calves had access to their dams between 1500 hours and 0600 hours. Calves were transitioned off milk, once they reached at least 75 kg liveweight (LW), by gradually reducing their milk allocation (AR) or reducing access to their dam (CCC).
Milk consumption for ARH was greater than for ARC calves (382 vs 450 L/calf, P < 0.05), whereas for CCC calves milk-yield difference between dams for the control and suckling groups during the suckling period and over the full season was 706 and 1048 L/cow respectively. There was no difference in weaning weight of calves (87 ± 1.7 kg LW), but, owing to differences in pre-weaning growth rate, age to weaning was youngest (P < 0.05) for CCC (55 days), followed by ARH (62 days) and ARC (73 days). Respective growth rates between birth and weaning (P < 0.001) for CCC, ARH and ARC calves were 0.955, 0.873 and 0.755 ± 0.028 kg/day. Although there was a growth check among CCC calves during the weaning period, there were no post-weaning differences in growth rate among the groups.
Increasing milk allowance had the benefit of improving calf pre-weaning growth rate, giving the option of weaning calves either earlier or when heavier. However, milk yield losses under suckling systems may be too high to warrant the elevated pre-weaning growth of calves.
The costs–benefits of high milk allocation or suckling systems need to be ascertained over the lifetime of the animals to assess long-term survival and productivity outcomes.
Keywords: health, immunity, live weight, milk flow rate, milk yield, natural rearing, nursing, separation, vaccine response.
Introduction
Consumers are becoming increasingly aware of the environmental and animal-welfare impact on their food (Hampton et al. 2020). Livestock farmers are experiencing more regulations and rules relating to animal husbandry practices. For instance, in New Zealand, public scrutiny of historic bobby calf practices elicited regulatory response to improve bobby calf welfare (Government NZ 2016). However, interest in calf-rearing practices remains, with concerns regarding the ethics of conventional practices of separating calves from cows at birth (Placzek et al. 2021). Research into delayed separation systems, widely referred to as suckling or extended cow–calf contact systems (CCC), has shown numerous benefits associated with improved social behaviour and increased liveweight gain of suckled calves (Meagher et al. 2019; Bertelsen and Jensen 2023a). However, CCC systems have also demonstrated reductions in milk yield during the suckling period (de Passillé et al. 2008; Mac et al. 2023; Ospina Rios et al. 2023), although the impact of suckling on milk yield varies widely with the system. Ospina Rios et al. (2023) recently demonstrated that implementing a partial contact system in pasture-based dairy resulted in an 8% reduction in milk yield. The milk yield reductions and associated management complexity of suckling systems could present an adoption barrier, particularly under pastoral conditions as little research exists. In addition, the ethical decision to keep calves with their dams may be replaced with the conundrum of having to separate them later in life, after strong bonds have been formed.
In many pastoral-based dairy systems, heifer replacement costs are managed through restricting milk feeding of artificially reared (AR) calves, which commonly sees calf milk allocations of 10–15% of birth weight (Muir et al. 2002). These restricted milk-feeding systems can promote rapid rumen development (McCoard et al. 2019) for high post-weaning growth rates but at the expense of lower pre-weaning growth rates (Khan et al. 2022). Emerging evidence suggests that there is a positive relationship between pre-weaning growth rate and first-lactation milk yield (Soberon et al. 2012; Margerison et al. 2013). The proposed mechanism is thought to be via increased deposition of secretory cells in mammary tissue (Soberon and Van Amburgh 2017; McCoard et al. 2019). However, long-term studies on the impact of early life nutrition are still limited, particularly in pasture-based dairy systems, which have inherently lower dry-matter intakes post-weaning than total mixed rations in housed systems overseas (Kolver and Muller 1998).
After feeding and labour, the health cost of calf-rearing between birth and weaning contributes to ~10% of total costs (Boulton et al. 2017). In housed conditions, health outcomes for suckling calves have shown to be inferior compared with calves removed from their dams at birth (Wenker et al. 2022), owing to the increased cross-contamination of excreta from dams under confinement. Although these issues are expected to be of lesser risk in a pastoral setting, more information is needed to understand the health and immune response of pre-weaning management for heifers that are predominantly reared outdoors.
The lack of information on the impact of calf-rearing in pastoral systems warrants greater understanding of both the short- and long-term outcomes. This knowledge is particularly important as consumers increasingly demand animal feed products that put welfare at the forefront of food production. The aim of this research was to compare opportunities for enhanced calf welfare through increased milk feeding either artificially or through contact with their dam, and consider the farm-system implications for pasture-based dairying.
Materials and methods
The research was conducted at the Ashley Dene Research and Development Station (ADRDS), Canterbury, New Zealand (latitude −43.647, longitude 172.347) between July 2023 and May 2024. The study was conducted with the permission of the Lincoln University Animal Ethics Committee (AEC #2023-33). The source population of mixed-aged, Friesian × Jersey bred cows at ADRDS (n = 470) is managed under a seasonal spring-calving pasture-based dairy operation, milking twice daily between July and May. The current study is a parallel group design with two cow comparisons where cows had their calves removed at birth (Control, n = 15) or where cows continued to suckle their calves for 8 weeks in a cow–calf contact (Suckling, n = 15). The parallel group consisted of three calf treatments, including (1) calves of the Control cows that were artificially reared in a restricted milk-feeding regime (18% of birth weight, ARC), (2) calves in the cow–calf contact treatment (CCC), and (3) an additional artificial rearing group offering high milk allocation (36% of birth weight, ARH).
From 24 July 2023, enrolment of 30 cows and 45 heifer calves commenced until either the target population size was reached (15 animals per treatment) or enrolment period ended (18 days). Restricted randomisation was used to allocate dams and calves into treatments from the ADRDS herd. The eligibility criteria for inclusion were as follows: calf gender (female only), dam age (3 years or older as calves from primiparous that are not bred for replacement), availability (cows not involved in other research), and maternal behaviour (CCC calves were observed suckling). Care was taken to balance calves and dams for breeding worth (BW) and age where possible, and a summary is presented in Tables 1 and 2.
Item | ARC | ARH | CCC | s.e.m. | P-value | |
---|---|---|---|---|---|---|
Breeding worth | 252 | 267 | 266 | 12.9 | 0.66 | |
Parts Friesian | 11.3 | 11.1 | 11.2 | 0.49 | 0.98 | |
Parts Jersey | 4.7 | 4.8 | 4.7 | 0.49 | 0.98 | |
Pre-weaning | ||||||
Liveweight at birth (kg) | 33.3 | 34.6 | 33.6 | 1.09 | 0.68 | |
Serum protein Days 1–2 (mg/dL) | 58.4 | 64.5 | 70.7 | 4.06 | 0.10 | |
Milk intake average (L/day) | 5.47 | 8.16 | – | 0.229 | <0.001 | |
Milk intake peak (L/day) | 5.81 | 9.11 | – | 0.252 | <0.001 | |
Total milk (L) | 382 | 450 | – | 20.3 | 0.025 | |
Total milk powder (kg) | 25.4 | 31.0 | – | 1.13 | 0.002 | |
Calf milk replacer as % of milk intake | 44.4 | 43.9 | – | 1.1 | 0.76 | |
Growth rate Days 0–42 (g/day) | 700b | 911a | 978a | 27.1 | <0.001 | |
Serum beta hydroxybutyrate (BHB) Days 40–50 (μmol/L) | 168 | 138 | 184 | 10.5 | 0.21 | |
Weaning | ||||||
Growth rate Days 43–90 (g/day) | 905a | 825b | 617c | 27.0 | <0.001 | |
Age at weaning (day) | 73a | 62b | 55c | 2.0 | <0.001 | |
Liveweight at weaning (kg) | 88.2 | 87.8 | 85.4 | 1.68 | 0.47 | |
Serum BHB Days 44–70 (μmol/L) | 250 | 205 | 251 | 33.0 | 0.40 | |
Post-weaning | ||||||
Liveweight 3 months (kg) | 105 | 112 | 107 | 2.7 | 0.21 | |
Growth rate 3–6 months (g/day) | 629 | 665 | 634 | 23.4 | 0.49 | |
Growth rate 6–9 months (g/day) | 338 | 367 | 400 | 20.2 | 0.10 | |
Liveweight 9 months (kg) | 186 | 198 | 194 | 4.5 | 0.16 | |
Serum BHB Days 80–100 (μmol/L) | 389 | 370 | 386 | 26.3 | 0.71 |
Values within a row followed by different letters are significantly different (at P = 0.05).
Item | Control | Suckling | s.e.m. | P-value | |
---|---|---|---|---|---|
Breeding worth | 178 | 195 | 13.8 | 0.38 | |
Production worth | 216 | 213 | 43.4 | 0.96 | |
Liveweight at calving (kg) | 503 | 488 | 16.9 | 0.54 | |
Age (years) | 5.1 | 4.9 | 0.54 | 0.86 | |
Pre-separation (Days 4–65) | |||||
Milk yield (kg/day) | 24.8 | 11.7 | 0.99 | <0.001 | |
Fat (%) | 4.84 | 3.64 | 0.207 | <0.001 | |
Protein (%) | 3.74 | 3.92 | 0.078 | 0.11 | |
Lactose (%) | 5.09 | 4.83 | 0.042 | <0.001 | |
Somatic cell count (SCC; cells/mL) | 23,918 | 38,854 | 1248.3 | 0.13 | |
Milksolids (kg/day) | 2.14 | 0.88 | 0.085 | <0.001 | |
Flow rate amA (L/min) | 2.69 | 2.25 | 0.147 | 0.04 | |
Flow rate pm (L/min) | 2.01 | 1.66 | 0.110 | 0.03 | |
Rumination time (min/day) | 463 | 467 | 14.4 | 0.82 | |
Eating time (min/day) | 423 | 488 | 22.3 | 0.05 | |
Total milk yield (kg/cow) | 1341 | 635 | 60.3 | <0.001 | |
Total milksolids (kg MS/cow) | 116 | 51 | 4.5 | <0.001 | |
Post-separation (day 66–284) | |||||
Milk yield (kg/day) | 19.9 | 17.8 | 0.865 | 0.099 | |
Fat % | 5.64 | 5.69 | 0.240 | 0.88 | |
Protein % | 4.22 | 4.35 | 0.093 | 0.33 | |
Lactose % | 4.96 | 4.90 | 0.041 | 0.24 | |
SCC (cells/mL) | 31,125 | 62,250 | 1366.0 | 0.12 | |
Milk solids (kg/day) | 1.91 | 1.70 | 0.007 | 0.03 | |
Flow rate am (L/min) | 2.57 | 2.49 | 0.147 | 0.71 | |
Flow rate pm (L/min) | 1.90 | 1.76 | 0.082 | 0.25 | |
Rumination time (min/day) | 483 | 481 | 11.7 | 0.92 | |
Eating time (min/day) | 447 | 441 | 24.0 | 0.87 | |
Total milk yield (kg) | 4147 | 3805 | 262 | 0.20 | |
Total milksolids (kg) | 391 | 359 | 12.5 | 0.09 | |
Full season | |||||
Days in milk | 267 | 272 | 2.2 | 0.15 | |
Milk yield (kg/cow.year) | 5488 | 4440 | 230 | 0.003 | |
Milksolids yield (kg MS/cow.year) | 507 | 410 | 16.1 | <0.001 |
Management
All calves were collected from the paddock and taken to the rearing facility within 8–20 h of birth, where they were weighed, tube-fed 2 L of first-milking colostrum and their navels were disinfected with iodine. Calves were collected once daily or more frequently in poor weather. Calves in the CCC treatment were returned to their dams, whereas both ARC and ARH calves remained in the rearing facility and, following two consecutive feeds of first-milking colostrum, were trained to suckle from an automatic feeder (Lely Calm, Smart™, Lely, Canterbury, New Zealand). AR calves received whole-herd bulk colostrum (milkings 2–4) and whole milk until 15 August, when they were transitioned to a 50:50 diet of whole milk and calf milk replacer (CMR, Ancalf, Farm Source NZ) combined with water at a rate of 150 g/L. To allow a minimum of two feeds per day to meet their total allocation, the maximum meal entitlement from the feeder was set at 3 and 5 L per meal for ARC and ARH respectively. All AR calves were run as a single mob in a three-walled rearing facility with natural ventilation and woodchip bedding. ARC and ARH calves had ad lib access to fresh water, meal, baleage and fresh pasture through a door in the rearing facility. Calves in the CCC treatment were kept in a 3 ha pasture area surrounded by calf-proof fencing. CCC calves also had access to shelter via a mobile shed, straw bedding, meal, baleage and fresh water. CCC calves were able to access their shelter and meal below a single wire fence, which excluded access by their dams.
Throughout the study, Control cows were milked twice daily at 0600 hours and 1500 hours, whereas Suckling cows were initially milked once daily at 1500 hours and, after 6 weeks, were milked twice daily. Following the afternoon milking, Suckling cows rejoined their calves where they remained together for ~15 h between 1600 hours and 0700 hours. In the morning, Suckling cows were separated from their calves and grazed pasture and pasture baleage supplement with the Control cows. When control and suckling cows were separated, permanently and temporarily, from calves, there was no fenceline contact, although visual contact was possible across a distance ranging from 50 to 100 m. All cows were herded together for milking in the afternoon, after which the Control cows were drafted to a fresh pasture allocation adjacent to the Suckling cows and calves. All cows received a total daily allowance of 18 kg DM/cow.day above 4.5 cm (approximately 1500 kg DM/ha) by increasing the allocation area each week and reducing the supplement. Pasture allocation in terms of area per cow per day, pre-graze mass and supplement were carefully managed to ensure that both groups received similar metabolisable energy each day.
Calves were weaned off milk between 21 September and 26 October when they had reached 80 kg LW. To achieve a minimum target weaning weight of 80 kg, all calves were weighed weekly from Week 6 and any calf weighing 75 kg or more was transitioned off milk over 7–10 days. Calves were transitioned off milk by either reducing the milk allocation from the automatic feeder (ARC and ARH) or by reducing access to their dam (CCC). At 42 days of age, calves in the ARH treatment were automatically transitioned from 12 to 6 L by reducing allocation by 0.6 L per day. To reduce dam access for suckling calves, the suckling period was switched from a day separation (15 h night access) to a night separation (8 h day access) soon after the commencement of twice daily milking of suckled cows on 12 September. Over the next 7 days, the duration of contact between dam and calf was reduced to a single 10 min suckling bout after the morning milking. Suckling calves received their last milk feed from dams on 21 September and any CCC calves below 80 kg LW (n = 6) were trained onto the Lely feeder to continue receiving milk until weaning. After weaning, calves continued to receive approximately 0.5 kg/calf.day meal for a further 4 weeks. Calves were transported to a support block on 24 November for grazing with replacement heifers from the rest of the farm (n = 150).
Cows were mated using artificial insemination between 14 October and 24 December with the aid of AfiCollar™ monitoring for detection of oestrus behaviour (Afimilk Agricultural Cooperative Ltd, Kibbutz Afikim, Israel). Dry-off procedures were implemented from March 2024, whereby cows were dried off on the basis of low body condition score (BCS), low milk yield and predicted calving date the following season.
Health
Calves were monitored daily and any animals observed with bacterial or viral scours were isolated and provided with alternate feeds of electrolyte (Novolyte™, NZ AgBiz, Hamilton, New Zealand) and milk until health was restored. Naval infections were treated with antibiotic (5 mL Bivatop® 200 LA, Boehringer, Ingelheim). An outbreak of rotovirus occurred in the adjacent non-experimental rearing facility when experimental calves were approximately 4 weeks old. As a preventative for scours, milk offered to ARC and ARH calves was dosed with 10 g/calf.day of RotogenCombo (Vetpak, Te Awamutu, New Zealand) for 5 days. Disbudding was contracted to veterinarians, when the calves were between 3 and 5 weeks, who used sedation, local anaesthetic and pain relief during the hot iron procedure. At 6 weeks of the experiment (40–55 days of age), all calves received their first vaccination, with a second booster vaccination given 4 weeks later. The vaccination Ultravac 7in1 (Zoetis, Parsippany-Troy Hills, NJ, USA) contained ultrafiltered toxoids for Clostridium perfringens Type D, Clostridium tetani, Clostridium novyi Type B, Clostridium septicum, and formol cultures for Clostridium chauvoei, Leptospira borgpeterseni serovar hardjo, and Leptospira interrogans serovar pomona. All calves received oral drench (Corporal® Alleva Animal Health Ltd, Auckland, New Zealand) at 5 mL/calf, for internal parasites at 18 weeks prior to transfer to support-block area.
The dams had previously been vaccinated for bovine viral diarrhoea virus (BVD) and leptospirosis, with the most recent vaccination occurring during late gestation in July 2023. Throughout the study, cows were monitored for injury or infection. Any clinical mastitis was sampled for bacterial identification and treated with appropriate antibiotics. Any Suckling cow requiring antibiotic treatment during the pre-separation period remained in their herds and their milk was collected into a bucket when machine harvested.
Measurements
Milk yield and milk flow rates were determined daily using in-shed flow meters (AfiFarm, Afimilk Agricultural Cooperative, Kibbutz Afikim, Israel). Representative subsamples of milk from a morning (Control cows) and afternoon (Control and Suckling cows) milking were collected monthly into 50 mL pottles containing a preservative and sent to MilkTestNZ (Horotiu, New Zealand) where samples were analysed for fat (%), protein (%), lactose (%), and somatic cell count (SCC, 000 cells/mL) by using mid-infrared spectrophotometry (MIR, CombiFoss, Foss Electric, Denmark). Grazing and rumination times were captured using the AfiFarm monitoring system. Liveweight was determined daily at each milking as cows exited the milking parlour across a calibrated automatic walk-over scale (Waikato Milking Systems, Hamilton, New Zealand). BCS (1–10 scale; Roche et al. (2009)) was determined monthly. The teats of Control and Suckling cows were inspected and scored for damage at the beginning and end of the suckling period.
To compare growth, calf liveweight was recorded at birth, 3, 6, and 9 months of age. During weaning (Weeks 6–12), calves were weighed weekly. Calf milk consumption by AR calves was captured by Lely software. We were unable to measure milk consumption of CCC calves, rather the impact of suckling on machine-harvested milk was determined as the average difference between the milk volumes of the Control and Suckling cow groups. Intake of meal and pasture was not measured because of group-housing conditions. Scouring events and health treatments were recorded throughout the study.
Blood was sampled via jugular venepuncture on the following four occasions: birth (1–2 days), pre-weaning (41–57 days), during weaning (46–71 days) and post-weaning (83–99 days). All blood was collected into 10 mL red-top vacutainers and allowed to clot prior to centrifuging at 3000g for 15 min at 4°C. Sera were extracted and stored at −20°C for further analysis at the Lincoln University analytical laboratory. Serum concentrations of total protein and beta hydroxybutyrate (BHB) were measured using the RX Daytona analyser by using commercial kits (Randox Laboratories, Crumlin, UK).
Vaccination response was determined using sera collected immediately prior to vaccination (19 September) and 2 weeks after the booster vaccination (31 October). Antibody responses against clostridial antigens were evaluated using an ELISA for the detection of antigen-specific immunoglobulin G (IgG). Antigen working solution was prepared by washing the Ultravac 7:1 vaccine (Zoetis) in 10 mM phosphate-buffered saline (PBS) and resuspending cells in PBS supplemented with casein at 10 g/L (Sigma, St Louis, MO, USA) to an optical density of 0.08, then diluting 1:50 in carbonate–bicarbonate buffer (Sigma). Microtitre plates (Maxisorb 96-well flat-bottomed; Nunc, Roskilde, Denmark) were coated with antigen working solution at 50 μL/well and incubated overnight at 4°C. Plates were then washed six times with PBS supplemented with Tween 20 (PBST20; Sigma) at 0.5 mL/L. Sera samples diluted in PBST20 supplemented with 10 g/L casein (PBST20C; Sigma) to achieve a two-fold dilution series (1:400–1:409,600 for samples from calves and 1:4000–1:4,096,000 for samples from dams) were added at 100 μL/well, along with a no serum control of PBST20C only. Plates were incubated at room temperature for 1 h and then washed six times with PBST20. Detection antibody (rabbit anti-bovine IgG (H&L):HRP [AbD Serotec AHP855P], Bio-Rad, Hercules, CA, USA; diluted 1:2000 in PBST20C) was added at 50 μL/well, incubated at room temperature for at least 1 h, and then washed six times with PBST20. Finally, 50 μL/well of 3,3′,5,5′-tetramethylbenzidine (TMB substrate, Becton Dickinson, Franklin Lakes, NJ, USA) was added and incubated at room temperature until optimal colour development had occurred (15–30 min). The reaction was stopped by addition of 50 μL/well of 0.5 M H2SO4 and absorbance was measured within 10 min by using a plate reader (VERSAmax™ microplate reader; Molecular Devices Corporation, Sunnyvale, CA, USA) at a wavelength of 450 nm.
Statistical analyses
The data from one dam in the Suckling cow data set were removed because of her having reoccurring mastitis. The data from one calf in the ARH calf data set were removed because of the poor health leading to euthanasia. Means for calf and dam variables were compared using Genstat (v. 22, VSN International) general linear-model procedure. Separate analyses were conducted for data pre- and post-weaning or separation using treatment group as the fixed term and animal as the random term.
The linear mixed-models procedure for left censored data was used to compare means for pre-weaning serum BHB concentrations with a lower detection limit of 100 (μmol/L). Vaccine efficacy for calf sera was analysed follow loge transformation of data and the means among the three treatment groups for pre-, post-, and their difference, were compared using the ANOVA function of Genstat.
Repeated-measures analysis was conducted for weekly milk yield, milk flow rate, behaviour and fortnightly milk composition data using treatment and time and their interaction as the fixed term and cow as the random term. Repeated measures for post-separation milk variables included data collected between 1 October 2023 and 1 March 2024, after which point cows were progressively dried off. SCC from herd test data was transformed to somatic cell score (SCS, log2(SCC/1000) prior to analysis of variance. Back-transformed values are presented.
Results
Calves
Average calf birth weight was 33.8 kg (±1.09 kg), resulting in milk allocations of 18% and 36% of initial liveweight for the ARC and ARH groups respectively. At peak allocation, ARH calves consumed 76% of their allocated 12 L of milk, whereas ARC calves consumed 97% of their 6 L entitlement. ARH calves consumed 18% more milk than did the ARC calves (Table 1), which resulted in increased pre-weaning growth rates of approximately 200 g/day. Milk consumption by CCC calves could not be measured, but pre-weaning growth rates were similar to those of calves in the ARH treatment. During the weaning period (Days 43–90), growth rates were highest for ARC, followed by ARH, and lowest for CCC. During the first 5 weeks after separation, 9 of the 15 calves in CCC either did not gain (n = 6) or lost (n = 3) weight. One calf in the ARC group lost weight and one calf from ARH did not gain weight during weaning.
The number of unrewarded feeder visits for ARC calves was considerably greater than that of ARH calves, as depicted in Fig. 1. During the first 3 weeks, ARC calves visited the feeder an average of 22 times per day without feeding, compared with just 3.5 unrewarded visits for ARH calves. The highest unrewarded visits occurred for the ARC treatment between 7 and 10 days of age (32 ± 7.4 unrewarded visits/calf.day) and 50 days of age for ARH (21 ± 3.8 unrewarded visits/calf.day). There was no difference between group treatment means for serum BHB concentrations prior to, during or after weaning. However, during the weaning phase, the range in serum BHB concentrations was 169–388, 114–307 and 102–584 mmol/L for ARC, ARH and CCC respectively.
Unrewarded visits to the feeder pre-weaning, based on a milk allocation of either 6 L/day (solid symbols), or 12 L/day (open symbols). The shaded area represents the weaning period. Error bars represent the standard errors of the mean.
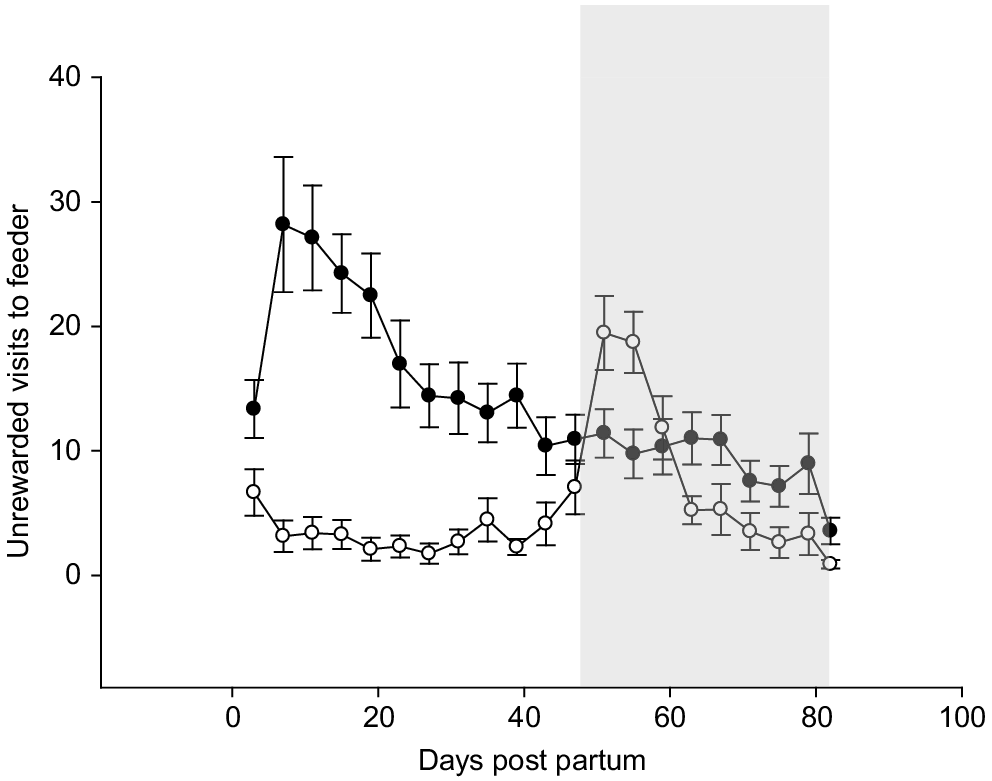
Liveweight at weaning (87.1 ± 1.68 kg) was similar for all treatments but age at weaning was lower for the calves offered high milk allowance (Table 1). Consequently, between birth and weaning, the average daily gain for ARC, ARH and CCC was 0.755, 0.873 and 0.955 kg/day respectively (P < 0.001). Post-weaning growth parameters were similar for all treatments and, by 9 months of age, liveweight was similar for all treatments at 192 ± 4.5 kg (P = 0.17, Fig. 2).
As an indication of passive immunity transfer, serum total protein for ARC, ARH and CCC was 58.4, 64.5, 70.7 ± 4.06 mg/dL respectively (P = 0.10). In total, 8 of the 44 calves sampled had a serum total protein lower the optimal threshold of 50 mg/dL for passive immunity transfer (3/15, 4/14 and 1/15 for ARC, ARH and CCC respectively). Despite testing positive for rotovirus, the ARC and ARH calves showed no signs of scours during the rotovirus outbreak. Calves in the CCC group tested negative for rotovirus. There was evidence of nutritional scours in the majority of CCC calves, although electrolyte treatment was provided to only one calf in the CCC group because others showed no signs of ill thrift. In the ARH group, three calves were observed with temporary abomasal bloating during the milk powder transition period and one with nutritional scours. No scours were observed among ARC calves.
Prior to vaccination, the mean antibody titres were relatively high 1:4716 ± 1448 (±s.e.), although not statistically different among the treatments (P > 0.10, Fig. 3). After vaccination, antibody titres were greater for CCC than ARC calves (14,689 vs 7691 ± 3163, P < 0.05); however, because of the high proportion of calves that either had no change or lower antibody titres in their post- compared with pre-vaccination titres, there was no treatment difference in terms of response.
Vaccine-specific antibody pre-vaccination (black bars) and post-vaccination (grey bars) titre in dairy calves with varying pre-weaning milk allocation of either 6 L/day (ARC), 12 L/day (ARH) or cow–calf contact for 15 h/day (CCC). Titre was defined as the first dilution step at which the optical density was less than for the no-serum control. Error bars represent the standard errors of the mean.
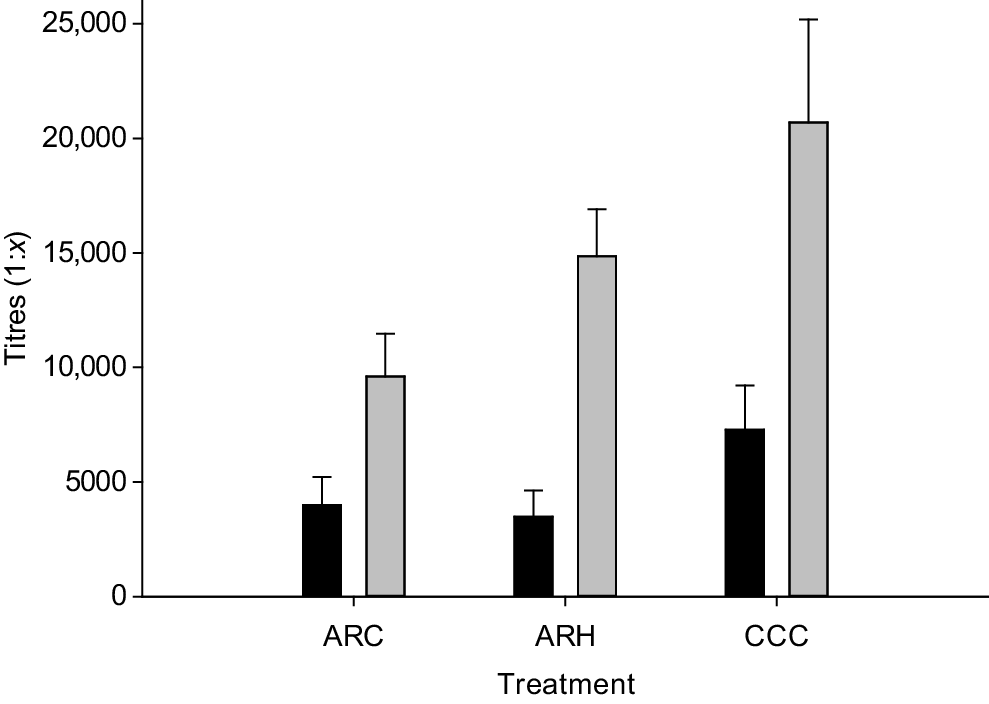
Cows
During the suckling period, the machine milk yield for Suckling cows was reduced by 54% compared with Control cows (P < 0.001, Fig. 4, Table 2). During the post-separation period, milk yield was 11% lower for Suckling cows; however, this was not statistically different from Control cows (P = 0.10). Across the entire lactation, the Suckling cows produced 19% less milk than did the Control cows (P < 0.01, Table 2).
Daily average milk yield for cows in a conventional early calf separation (solid symbols) or cow–calf contact rearing (open symbols). The shaded area is the weaning period. Error bars represent standard errors of the mean.
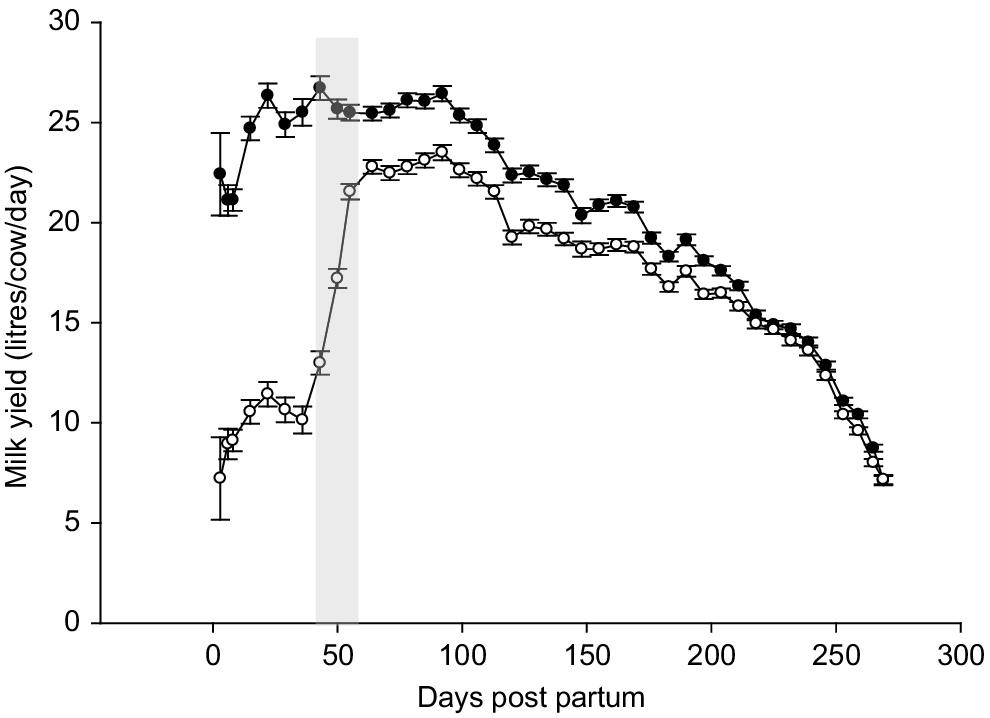
During the suckling period, both milk fat and lactose concentrations were lower in the milk of Suckling cows than of the Control cows (P < 0.05, Table 2), although milk protein remained similar between the two treatments. Suckling cows were milked once daily for the majority of the suckling period, and milk flow rate during this time was lower than either in the morning or afternoon milking for the Control cows. Even when the morning milking was introduced for Suckling cows during the weaning phase, the milk flow rates from Suckling cows remained lower than those from Control cows. Milk flow rates were similar after separation. Following separation, there was no difference in milk composition between treatments, but the trend for a lower milk yield from the Suckling cows resulted in lower daily milksolids (MS) (P < 0.05).
There was no difference in average liveweight (457 ± 11.1 kg, P = 0.48) among treatments or between pre- and post-separation periods. Although there was no difference among the treatments for BCS during the pre-weaning period (average 4.2 ± 0.065; P = 0.45), BCS was greater for the Suckling than in Control cows for the remainder of lactation (4.3 vs 4.6 ± 0.061, P = 0.014). There was no difference among treatments for the number of days from calving to first mating (82 ± 2.6 days). Pregnancy scanning following mating showed 100% of the Suckling cows in calf (14 of 14 inseminated) and 80% in calf for the Control cows (13 of 15 inseminated).
From a health perspective, three cows from ARC and two cows from CCC were treated for mastitis. One of the CCC cows was removed from the trial because of Staphylococcus aureus bacteria infection, which was likely to be due to historical infection and unrelated to the treatment. SCC was not statistically different among the treatments in either the suckling or post-separation period (Table 2). By the end of the suckling period, the majority of cows showed minor abrasions on one or more teats; however, there was no difference among treatment groups.
Discussion
Calves
This research has highlighted the large influence of calf-rearing regime on calf growth pre- and post-weaning. Our results are consistent with previously published research demonstrating the positive relationship between milk intake and pre-weaning growth rate during the first 6 weeks of life (Jasper and Weary 2002; Khan et al. 2022). All calves continued to gain weight during weaning, which occurred between 7 and 12 weeks of age, indicating general suitability of calves for weaning. Typically, it has been shown that at high milk allowance, motivation to consume solid feed is reduced (de Passillé et al. 2011, Fig. 1), potentially delaying rumen development. Markers such as serum BHB, have been used as an indicator of solid feed intake and rumen development (Deelen et al. 2016; Suarez-Mena et al. 2017), although fluctuations in circulating BHB concentrations relative to meals make it difficult to provide a specific threshold BHB concentration for weaning (Suarez-Mena et al. 2017). In the current study, there was large variation in BHB concentrations during weaning, particularly among CCC calves, and the large proportion of CCC calves not gaining weight post-weaning indicates that a liveweight target of 80 kg may not a suitable threshold for weaning suckling calves at less than 8 weeks of age.
A combination of stressors, including removal of both milk and dam and recent vaccination, are all likely to have contributed to reductions in growth rate of CCC calves post-weaning. The CCC calves were separated from their dam within days of their first vaccination and previous research has shown that this reduces feed intake (Suarez-Mena et al. 2017). Moreover, the general stress of separation from their dam has been widely reported to affect routine behaviours and growth of suckling calves (Meagher et al. 2019; Wenker et al. 2021). To minimise the impact of weaning for the CCC calves, we had adopted a partial contact system to acclimatise calves to frequent separation and used a transitional approach, by reducing access time to the dam (CCC). We anticipated that CCC calves would start consuming solid feed earlier than would AR calves, owing to the social facilitation of the dams, which has previously been shown to stimulate solid-feed intake (Costa et al. 2016; Broucek et al. 2020; Bertelsen and Jensen 2023b). When evaluating average weaning growth rate across the entire weaning period of up to 43 and 90 days of age, the gain of ~600 g/day was lower for CCC calves than in the AR treatments. However, following weaning there was no difference in growth rates among any of the treatments, suggesting that the weaning disturbance for CCC calves in the current study was not prolonged. Bertelsen and Jensen (2023a) compared weaning methods of suckled calves and observed that although using a transitional weaning, as opposed to abrupt weaning, lessened the difference between dam contact and control, it did not eliminate the negative impact of dam separation. Ospina Rios et al. (2023) used weaning flaps on the nose of calves to avoid the dual stress of removing the source of milk and removal of the dam, which may have improved post-weaning outcomes for those calves. More information on the short- and long-term effects of weaning methods are warranted.
The lack of pre-weaning treatment effect on post-weaning growth rates supports the findings of previous studies (Khan et al. 2022). Once combined as a single mob with remaining heifers from the wider farm, there was no evidence to indicate carry-over advantages in attaining nutrients in a pastoral grazing setting. In seasonal calving dairy systems, the timing of weaning in October is followed by a period of declining energy content of grasses as they commence their flowering phase. The target growth rate for the calves in this study was at least 600 g/day, which was not achieved during the period representing mid-summer to autumn (6 and 9 months of age) when low pasture growth rates resulted in a large proportion of the diet being offered as low-energy baleage supplement. The results presented in this study highlight the challenges associated with pastoral dairying and the influence of the farm system on feed availability and nutritional management of young stock pre- and post-weaning.
There were no major health issues during the trial, even though the research farm calf-rearing facility tested positive for rotovirus. Calves in the ARH group tended to be more prone to abomasal bloating, but usually in instances of user error, with the calf feeder running low on whole milk and increasing the proportion of milk replacer. The faecal colour and consistency from CCC calves often displayed nutritional scours, but calves in this treatment looked bright and alert throughout the study.
The relatively high antibody titres observed in calf serum at the pre-vaccination stage are likely to have attributed to a combination of maternal antibodies, transferred through colostrum immediately after birth, and exposure to pathogens in the environment, leading to a natural immune response and the development of specific antibodies. Older mothers or those previously exposed to specific pathogens may increase maternal interference and reduce vaccine response of their calves (Reddout et al. 2024). Additionally, vaccination against leptospirosis during pregnancy could have generated high concentrations of specific antibodies targeted to this pathogen, which were then transferred to the calves through colostrum (Saso and Kampmann 2020).
With respect to calf vaccine response, there was no difference among treatment groups for the change in antibody titres, which is consistent with Khan et al. (2022), who reported no effect of high and low milk allowance on antibody response after booster vaccination. The elevated antibodies measured for CCC calves are likely to be associated with the recruitment criteria of calves into the CCC group. Any calf that was not detected suckling was transferred to an artificial rearing treatment, which may have biased the CCC group as evidenced by the near statistically greater serum total protein concentrations. Although the vaccine did prompt an immune response, as evidenced by the incremental rise in antibody titres, the high pre-vaccination antibody concentration may have affected the immunogenic response to the vaccination. Understanding the source and nature of these antibodies is crucial for tailoring vaccination strategies, to ensure optimal immune protection for young animals.
Cows
The amount of milk associated with each calf-rearing system, pre-weaning, was 382, 450 and 706 kg/calf for ARC, ARH and CCC respectively (Table 2). Given the similarity in pre-weaning growth rate of suckling and ARH calves, we would hypothesise that these groups consumed similar quantities of milk. Therefore, the difference of 282 kg between artificial rearing and suckling is likely to be associated with a physiological response to suckling versus machine harvesting. Previous research has consistently demonstrated a reduction in harvested milk pre-weaning when compared with control groups, which had calves removed within 24 h (Bar-Peled et al. 1995; Mac et al. 2023; Ospina Rios et al. 2023; Sørby et al. 2024). The reduction in machine milk yield is reported to be due to both the impact of milk consumed by the calf and changes in milk let down associated with lower oxytocin release during milking by suckled cows (Bar-Peled et al. 1995; de Passillé and Rushen 2006).
In the current study, carry-over effects of suckling post-weaning resulted in a full lactation milk yield reduction of 1048 L/cow compared with the control cows. Although the number of studies looking at full lactation milk yield following suckling are limited, those that have looked at carry-over effects of suckling have reported variable results, showing no effect on post-separation milk yield (de Passillé et al. 2008; Ospina Rios et al. 2023; Sørby et al. 2024), or reduced milk yield during the post-separation period (Bar-Peled et al. 1995; Barth 2020; Johanssen et al. 2024). In previous studies, the negative carry-over effect of suckling is more pronounced when the duration of suckling is extended beyond the peak milk phase (Barth 2020; Sørby et al. 2024), or when milk harvesting either by calf or machine is incomplete (Bar-Peled et al. 1995). The timing of separation in the current study was around peak milk production (based on the Control group, Fig. 4) and suckling did not extend beyond this period. A more likely explanation for the carry-over low milk yield post-separation is due to incomplete milking and accumulation of residual milk in the udder. Bar-Peled et al. (1995) investigated the effect of milking frequency with and without suckling and concluded that the physiological disruption associated with weaning resulted in incomplete milk ejection at milking. In the present study, cows were removed from their calves at approximately 0700 hours and were machine milked 7–8 h later from 1400 hours. It is likely that residual milk in the mammary gland, associated with incomplete milking, had reduced secretory activity to the extent that apoptosis had occurred, resulting in the reduced milk secretion after separation (Stelwagen et al. 2013; Deacon et al. 2023).
Milk composition during the suckling period showed reduced milk fat concentrations, which is consistent with previous findings (Wenker et al. 2022; Ospina Rios et al. 2023). As with milk yield, the reduction in milk fat content is thought to be associated with variation in oxytocin and milk ejection between suckling and machine milking. Suckling has shown to elicit a stronger oxytocin and milk-ejection response than does machine milking (Bar-Peled et al. 1995). A lower oxytocin response from suckled cows under machine milking (Bar-Peled et al. 1995; Tančin et al. 1995) will elicit a weakened myoepithelial cell contraction, which is insufficient to press large milk fat molecules from the alveoli. This effect might have been more pronounced in the current study because of the long period of daily contact (15 h) leading to partially filled alveoli and less pressure during milk ejection (Bruckmaier and Hilger 2001). Furthermore, Rico et al. (2014) demonstrated that the ejection of milk fatty acids is not constant through the milking process, with lower milk fat concentrations in the foremilk than in the hind milk. The effect of incomplete milking on milk composition also explains the lower lactose concentration, which has been explained by loss of integrity of tight junctions between mammary epithelial cells (Stelwagen et al. 2013; Deacon et al. 2023).
One of the concerns expressed by farmers for the adoption of suckling systems is mastitis (Neave et al. 2022). In this study, the suckling treatment did not display increased risk of mastitis. The effect of suckling systems on udder health has been assessed in other research, and our findings here are consistent with previous conclusions that showed no detriment to udder health (Grøndahl et al. 2007; Fröberg et al. 2008; Wenker et al. 2022). Further, teat damage as a result of suckling was not evident in the current study, likely owing to the partial contact system adopted and early separation. The lower milk yield of the suckled cows than conventional cows was the likely reason for increased BCS, which, in the longer term, may provide enduring benefits in the following lactation season.
Farm-system implications
This study was designed for assessing the feasibility of a suckling system in pastoral systems. The majority of research on cow–calf contact has been conducted in non-seasonal, predominantly housed systems (Meagher et al. 2019), where herds are small (<100 cows) and workload is distributed evenly throughout the year. In seasonal dairy systems, block-calving in late winter to match feed demand with pasture growth drives high workload demand and welfare stressors associated with large numbers of transition cows, adverse weather, calf-rearing and staff-management-under-fatigue conditions. Adopting a suckling system does not necessarily remove the need for artificial rearing, depending on management of non-replacement and mis-mothered calves. However, the present research noted a change in labour requirement because additional staff were required for the herding/daily separation of cows and calves, but less labour was required for rearing.
Adoption of a suckling regime allows both the dam and her calf to express natural behaviours. Although not quantified in the present study, it was observed that hygiene appeared improved among the suckling group (CCC), owing to regular grooming by dams. Compared with AR calves, which would often have faecal debris attached to their coats, the level of cleanliness in suckled calves was always high. Hygiene becomes important when environmental conditions deteriorate to the extent that animal health is compromised. In seasonal block-calving systems, the risk of hygiene deterioration is a concern because there is high traffic between people and animals around feeding stations. Although the rotovirus outbreak recorded in the current study did not lead to visible impacts on artificially reared calves, it is worth noting that CCC calves were not affected by the outbreak.
Another aspect of natural-behaviour expression was the absence of apparent hunger among calves with high milk allowance (CCC and ARH). Calves in the ARC group displayed greater hunger levels than did ARH calves at an early age (Fig. 1), and it is likely that this drove motivation towards solid-feed consumption. Previous research has shown that although calves on restricted milk intakes are able to compensate reductions in milk energy through increased solid-feed intake, there is a delay of at least 3 weeks before adaptation to solid feed (de Passillé et al. 2011; Khan et al. 2022), which prevents full energy compensation compared with high-milk diets. A high-milk feeding regime, whether in an artificial or suckling system, provides immediate benefits in terms of growth rates and satiety of calves. From an economic perspective, calf rearers reluctant to increase total milk allocation to calves might consider adopting a feeding strategy that supplies greater milk allocation during the first 3–4 weeks of life and prolonged transition from a higher allocation, rather than a constant milk allocation from birth. Under this cost-neutral accelerated and step-down approach, calves are likely to experience less hunger while still meeting growth rate targets. Considering the early recruitment of heifer replacements during calving, this accelerated step-down feeding approach may be more economic because the high-milk feeding period would more likely coincide with the period of colostrum availability, which is generally not sold to the processor.
For the CCC system, allowing calves to suckle naturally is expected to negate concerns around hunger; however, the cost to suckling, as observed in the present study, is the large reduction in machine-harvested milk yield. For a pasture-based dairy system, such as used in New Zealand, where milk payment is based on milksolid production (MS, milk fat + protein), the observed 19% reduction in harvested MS is likely to be a considerable barrier to adoption. Even taking into consideration the use of whole milk for rearing in conventional practices, the difference in saleable MS compared with either ARC and ARH was a 13.5% and 12.4% reduction respectively, by adopting CCC. Given that other studies have shown smaller reductions in milk loss (Ospina Rios et al. 2023), it is feasible to suggest that improvements in our understanding of suckling systems will enable design of systems that can minimise the impact suckling has on milk yield. More challenging will be the perception of difficulties associated with suckling systems. In a survey of New Zealand farmers, Neave et al. (2022) reported that most farmers were most concerned about animal welfare, housing, separation stress and system complexity when asked to consider a cow–calf contact system.
The suckling system adopted in this research was designed to create an approach that could be scalable to a commercial level. Because the major challenge is the labour intensity of herding cows and newborn calves for milking, a pasture-based suckling system would benefit from having areas near the milking parlour reserved for fresh cows and calves, to minimise herding pressure. Use of portable shelter solutions (such as hay bales, shade cloth, or moveable sheds) and plantings can lessen the impact of inclement weather. Managing separation stress may present some challenges but approaches that can manage nutritional dependence and bonding separation, as with nose flaps for weaning, may smooth the transition for cow and calf. What becomes apparent is the many trade-offs associated with suckling systems and more research is required to mitigate some of the short-term impacts such as low milk yield and weaning stress, while exploring the longer-term benefits such as improved heifer performance during her lifetime and greater market access to improve revenue and profitability.
Conclusions
In this study, a cow–calf contact system resulted in milk yield losses in excess of calf requirements for high growth rate, although benefits of suckling included increased BCS. Compared with artificially reared calves on a restricted milk regime, suckling calves had a greater pre-weaning growth rate, but the present results demonstrated that similar calf growth outcomes can be achieved through artificial rearing by using a high milk allocation. Future research is required to determine strategies that can overcome milk yield losses, and to evaluate the long-term effects of rapid pre-weaning growth rate on life-time performance.
Conflicts of interest
Alison Hodgkinson and Sally-Anne Turner are employees of the funder of this research. There are no further conflicts of interest.
Acknowledgements
The authors gratefully acknowledge the support of the farm staff at ADRDS who willingly supported the project. We also thank Lely for technical support.
References
Bar-Peled U, Maltz E, Bruckental I, et al. (1995) Relationship between frequent milking or suckling in early lactation and milk production of high producing dairy cows. Journal of Dairy Science 78, 2726-2736.
| Crossref | Google Scholar | PubMed |
Barth K (2020) Effects of suckling on milk yield and milk composition of dairy cows in cow–calf contact systems. Journal of Dairy Research 87, 133-137.
| Crossref | Google Scholar | PubMed |
Bertelsen M, Jensen MB (2023a) Behavior of calves reared with half-day contact with their dams. Journal of Dairy Science 106, 9613-9629.
| Crossref | Google Scholar | PubMed |
Bertelsen M, Jensen MB (2023b) Comparing weaning methods in dairy calves with different dam contact levels. Journal of Dairy Science 106, 9598-9612.
| Crossref | Google Scholar | PubMed |
Boulton AC, Rushton J, Wathes DC (2017) An empirical analysis of the cost of rearing dairy heifers from birth to first calving and the time taken to repay these costs. Animal 11, 1372-1380.
| Crossref | Google Scholar | PubMed |
Broucek J, Uhrincat M, Kisac P, Hanus A (2020) Effect of different rearing during the milk-feeding period on growth of dairy calves. Agriculture 10, 346.
| Crossref | Google Scholar |
Bruckmaier RM, Hilger M (2001) Milk ejection in dairy cows at different degrees of udder filling. Journal of Dairy Research 68, 369-376.
| Crossref | Google Scholar | PubMed |
Costa JHC, von Keyserlingk MAG, Weary DM (2016) Invited review: effects of group housing of dairy calves on behavior, cognition, performance, and health. Journal of Dairy Science 99, 2453-2467.
| Crossref | Google Scholar | PubMed |
Deacon A-M, Blouin R, Thibault C, Lacasse P (2023) Mechanism underlying the modulation of milk production by incomplete milking. Journal of Dairy Science 106, 783-791.
| Crossref | Google Scholar | PubMed |
Deelen SM, Leslie KE, Steele MA, Eckert E, Brown HE, DeVries TJ (2016) Validation of a calf-side β-hydroxybutyrate test and its utility for estimation of starter intake in dairy calves around weaning. Journal of Dairy Science 99, 7624-7633.
| Crossref | Google Scholar | PubMed |
de Passillé AMB, Rushen J (2006) Calves’ behaviour during nursing is affected by feeding motivation and milk availability. Applied Animal Behaviour Science 101, 264-275.
| Crossref | Google Scholar |
de Passillé AM, Marnet P-G, Lapierre H, Rushen J (2008) Effects of twice-daily nursing on milk ejection and milk yield during nursing and milking in dairy cows. Journal of Dairy Science 91, 1416-1422.
| Crossref | Google Scholar |
de Passillé AM, Borderas TF, Rushen J (2011) Weaning age of calves fed a high milk allowance by automated feeders: effects on feed, water, and energy intake, behavioral signs of hunger, and weight gains. Journal of Dairy Science 94, 1401-1408.
| Crossref | Google Scholar |
Fröberg S, Gratte E, Svennersten-Sjaunja K, Olsson I, Berg C, Orihuela A, Galina CS, Garcia B, Lidfors L (2008) Effect of suckling (‘restricted suckling’) on dairy cows’ udder health and milk let-down and their calves’ weight gain, feed intake and behaviour. Applied Animal Behaviour Science 113, 1-14.
| Crossref | Google Scholar |
Government NZ (2016) Animal Welfare (calves) regulations 2016. Available at https://www.legislation.govt.nz/regulation/public/2016/0170/17.0/DLM6905430.html
Grøndahl AM, Skancke EM, Mejdell CM, Jansen JH (2007) Growth rate, health and welfare in a dairy herd with natural suckling until 6–8 weeks of age: a case report. Acta Veterinaria Scandinavica 49, 16.
| Crossref | Google Scholar |
Hampton JO, Jones B, McGreevy PD (2020) Social license and animal welfare: developments from the past decade in Australia. Animals 10, 2237.
| Crossref | Google Scholar |
Jasper J, Weary DM (2002) Effects of ad libitum milk intake on dairy calves. Journal of Dairy Science 85, 3054-3058.
| Crossref | Google Scholar | PubMed |
Johanssen JRE, Adler S, Johnsen JF, Sørheim K, Bøe KE (2024) Performance in dairy cows and calves with or without cow–calf contact on pasture. Livestock Science 285, 105502.
| Crossref | Google Scholar |
Khan MA, Heiser A, Maclean PH, Leath SR, Lowe KA, Molenaar AJ (2022) Growth performance, antibody response, and mammary gland development in New Zealand dairy replacement bovine heifers fed low or high amounts of unpasteurized whole milk. Journal of Animal Science 100, skac219.
| Crossref | Google Scholar |
Kolver ES, Muller LD (1998) Performance and nutrient intake of high producing Holstein cows consuming pasture or a total mixed ration. Journal of Dairy Science 81, 1403-1411.
| Crossref | Google Scholar | PubMed |
Mac SE, Lomax S, Clark CEF (2023) Dairy cow and calf behavior and productivity when maintained together on a pasture-based system. Animal Bioscience 36, 322-332.
| Crossref | Google Scholar | PubMed |
Margerison JK, Robarts ADJ, Reynolds GW (2013) The effect of increasing the nutrient and amino acid concentration of milk diets on dairy heifer individual feed intake, growth, development, and lactation performance. Journal of Dairy Science 96, 6539-6549.
| Crossref | Google Scholar | PubMed |
McCoard S, Heiser A, Lowe K, Molenaar A, MacLean P, Johnstone P, Leath S, Hoskin SO, Khan MA (2019) Effect of weaning age on growth, mammary gland development, and immune function in Holstein Friesian calves fed conserved alfalfa (FiberStart). Journal of Dairy Science 102, 6076-6087.
| Crossref | Google Scholar | PubMed |
Meagher RK, Beaver A, Weary DM, von Keyserlingk MAG (2019) Invited review: A systematic review of the effects of prolonged cow–calf contact on behavior, welfare, and productivity. Journal of Dairy Science 102, 5765-5783.
| Crossref | Google Scholar | PubMed |
Muir PD, Fugle CJ, Ormond AWA (2002) Calf rearing using a once-a-day milk feeding system: current best practice. Proceedings of the New Zealand Grassland Association 64, 21-24.
| Crossref | Google Scholar |
Neave HW, Sumner CL, Henwood RJT, Zobel G, Saunders K, Thoday H, Watson T, Webster JR (2022) Dairy farmers’ perspectives on providing cow–calf contact in the pasture-based systems of New Zealand. Journal of Dairy Science 105, 453-467.
| Crossref | Google Scholar | PubMed |
Ospina Rios SL, Lee C, Andrewartha SJ, Verdon M (2023) A pilot study on the feasibility of an extended suckling system for pasture-based dairies. Animals 13, 2571.
| Crossref | Google Scholar | PubMed |
Placzek M, Christoph-Schulz I, Barth K (2021) Public attitude towards cow–calf separation and other common practices of calf rearing in dairy farming – a review. Organic Agriculture 11, 41-50.
| Crossref | Google Scholar |
Reddout C, Chase CCL, Beck P, Salak-Johnson JL (2024) Dam age differentially affects immune response of her calf to bovine respiratory disease vaccination. Agriculture 14, 68.
| Crossref | Google Scholar |
Rico DE, Marshall ER, Choi J, Kaylegian KE, Dechow CD, Harvatine KJ (2014) Within-milking variation in milk composition and fatty acid profile of Holstein dairy cows. Journal of Dairy Science 97, 4259-4268.
| Crossref | Google Scholar | PubMed |
Roche JR, Friggens NC, Kay JK, Fisher MW, Stafford KJ, Berry DP (2009) Invited review: Body condition score and its association with dairy cow productivity, health, and welfare. Journal of Dairy Science 92, 5769-5801.
| Crossref | Google Scholar | PubMed |
Saso A, Kampmann B (2020) Maternal immunization: nature meets nurture. Frontiers in Microbiology 11, 1499.
| Crossref | Google Scholar |
Soberon F, Van Amburgh ME (2017) Effects of preweaning nutrient intake in the developing mammary parenchymal tissue. Journal of Dairy Science 100, 4996-5004.
| Crossref | Google Scholar | PubMed |
Soberon F, Raffrenato E, Everett RW, Van Amburgh ME (2012) Preweaning milk replacer intake and effects on long-term productivity of dairy calves. Journal of Dairy Science 95, 783-793.
| Crossref | Google Scholar | PubMed |
Sørby J, Holmøy IH, Nødtvedt A, Ferneborg S, Johnsen JF (2024) Comparing the effects of contact duration on cow and calf performance beyond separation – a prospective cohort study. Acta Veterinaria Scandinavica 66, 21.
| Crossref | Google Scholar |
Stelwagen K, Phyn CVC, Davis SR, Guinard-Flament J, Pomies D, Roche JR, Kay JK (2013) Invited review: reduced milking frequency: milk production and management implications. Journal of Dairy Science 96, 3401-3413.
| Crossref | Google Scholar | PubMed |
Suarez-Mena FX, Hu W, Dennis TS, Hill TM, Schlotterbeck RL (2017) β-Hydroxybutyrate (BHB) and glucose concentrations in the blood of dairy calves as influenced by age, vaccination stress, weaning, and starter intake including evaluation of BHB and glucose markers of starter intake. Journal of Dairy Science 100, 2614-2624.
| Crossref | Google Scholar |
Tančin V, Harcek L, Brouček J, Uhrinčať M, Mihina Š (1995) Effect of suckling during early lactation and changeover to machine milking on plasma oxytocin and cortisol levels and milking characteristics in Holstein cows. Journal of Dairy Research 62, 249-256.
| Crossref | Google Scholar | PubMed |
Wenker ML, van Reenen CG, de Oliveira D, McCrea K, Verwer CM, Bokkers EAM (2021) Calf-directed affiliative behaviour of dairy cows in two types of cow–calf contact systems. Applied Animal Behaviour Science 243, 105461.
| Crossref | Google Scholar |
Wenker ML, Verwer CM, Bokkers EAM, Te Beest DE, Gort G, De Oliveira D, Koets A, Bruckmaier RM, Gross JJ, van Reenen CG (2022) Effect of type of cow–calf contact on health, blood parameters, and performance of dairy cows and calves. Frontiers in Veterinary Science 9, 855086.
| Crossref | Google Scholar |