The societal role of meat: the Dublin Declaration with an Australian perspective
David W. Pethick


A Food Futures Institute, Murdoch University, Murdoch, WA 6150, Australia.
B Queensland Alliance for Agriculture and Food Innovation, The University of Queensland, St Lucia, Qld 4072, Australia.
C School of Agriculture and Food, Faculty of Science, University of Melbourne, Parkville, Vic. 3052, Australia.
D School of Agriculture and Environment, Faculty of Science, The University of Western Australia, 35 Stirling Highway, Crawley, WA 6009, Australia.
E Scibus and The University of Sydney, Camden, NSW 2570, Australia.
Abstract
It is clear that the societal role of meat is being challenged with ideological and simplified logic without substantiation from robust data-driven science. With this background, the international summit titled ‘The societal role of meat – what the science says’ was held in Dublin, Ireland, during October 2022, to provide evidence-based evaluations and the Dublin Declaration was signed by over 1000 scientists. In this paper, we provide a synopsis of the summit and then give context for evaluating the societal role of meat in Australia. The key themes of the summit were the essential roles of meat in (1) diet and health, (2) a sustainable environment and (3) society, economics and culture. Evidence clearly showed the role of meat as a nutrient-dense source of high-quality protein and micronutrients that can be safely consumed by humans. Further, the complementary role of livestock in agricultural systems was highlighted with both plant- and animal-based agriculture reliant on each other to maximise the efficient production of food. Thus, from both an Australian and world perspective, very little food considered to be human-edible is fed to livestock. The role of livestock in rural societies across the world was emphasised to underpin regional and national economies, with particular importance in those countries with developing economies to facilitate growing wealth to ‘step out’ of poverty and provide gender equality. Meat production, particularly from ruminants, is a critical part of Australian primary production and it is concluded that the Dublin Declaration is highly relevant to Australia. Finally, concern regarding future funding and organisation of research and extension is discussed. There is a need to continue funding highly collaborative programs that bring a broad range of disciplines together, in conjunction with undergraduate and postgraduate teaching to underpin the social license to operate for meat and livestock production.
Keywords: circularity, diet, livestock, meat, methane, nutrition, population health, sustainability.
Introduction
Advances in the nutritional sciences, agriculture, animal production and agronomy are based on quantification. The quantitative evaluation of evidence is the core strength of the scientific approach used by these disciplines and has been responsible for major gains in food production efficiency over the past century. However, public debate around complex societal challenges may at times be conducted without a strong quantitative base, often leading to suboptimal outcomes in understanding, legislation, and behaviour change. These concerns have been most prominent in Europe, but also other western countries, with calls from lobby groups and popular press to reduce meat consumption, especially from ruminants, to reduce global warming (BBC News 2022; Oreskes 2022; The Guardian 2022). Also, health professionals have stepped up the pressure to heavily reduce meat consumption with the recent Global Burden of Diseases (GBD 2019, Murray et al. 2020) study, substantially increasing the predicted burden (e.g. premature death or disability) attributable to red meat consumption between the 2017 and 2019 studies (Murray et al. 2020), a finding strongly challenged by a concerned group of scientists (Stanton et al. 2022). Scientific professionals with diverse backgrounds discussed these issues at recent forums of the International Congresses of Meat Science and Technology, where concern was raised that the debate around consumption of meat has been conducted with insufficient quantitative evaluation of the evidence and, hence, scientific rigour. Indeed, these same issues have been raised by eminent scientists over 30 years ago (Blaxter and Webster 1991). However, the view prevails, as articulated by Aiking (2014, p. 486S) ‘Because animal protein production appropriates a huge and disproportionate share of natural resources, it presents a perfect target as an option for significant reduction.’. He goes onto suggest such reductions will also improve food security, equity, health, climate, and biodiversity. We believe that this is a simplistic solution to a series of complex societal issues, for the reasons discussed throughout the paper.
In October 2022, a meeting was convened in Dublin, Ireland titled ‘The societal role of meat’. The meeting provided evidence-based evaluations of the societal role of meat and resulted in the Dublin Declaration (Box 1) signed by 1007 scientists as of 10 May 2023. Key themes of the conference were the roles of meat in (1) diet and health, (2) a sustainable environment and (3) society, economics and culture; a series of journal articles on these themes has been published in Animal Frontiers in 2023, plus the presentations are available at Teagasc (2023). In this paper, we provide a synopsis of the evidence presented at the conference and give an Australian context for evaluating the societal role of meat. Before tackling the primary objective of this paper, we address some of the shortcomings of GBD 2019. The commentary in our paper is not a review but a nuanced perspective on the false dichotomy between plant- and animal-sourced foods as they both have a role in a sustainable food supply (Comerford et al. 2021; Smith et al. 2022a).
Box 1.Dublin Declaration |
The Dublin Declaration of scientists on the societal role of livestock |
Purpose of this declaration |
Livestock systems must progress on the basis of the highest scientific standards. They are too precious to society to become the victim of simplification, reductionism or zealotry. These systems must continue to be embedded in and have broad approval of society. For that, scientists are asked to provide reliable evidence of their nutrition and health benefits, environmental sustainability, socio-cultural and economic values, as well as for solutions for the many improvements that are needed. This declaration aims to give voice to the many scientists around the world who research diligently, honestly and successfully in the various disciplines in order to achieve a balanced view of the future of animal agriculture. |
Challenges for livestock |
Today’s food systems face an unprecedented double challenge. There is a call to increase the availability of livestock derived foods (meat, dairy, eggs) to help satisfy the unmet nutritional needs of an estimated three billion people, for whom nutrient deficiencies contribute to stunting, wasting, anaemia, and other forms of malnutrition. At the same time, some methods and scale of animal production systems present challenges with regards to biodiversity, climate change and nutrient flows, as well as animal health and welfare within a broad One Health approach. With strong population growth concentrated largely among socioeconomically vulnerable and urban populations in the world, and where much of the populace depends on livestock for livelihoods, supply and sustainability challenges grow exponentially and advancing evidence-based solutions becomes ever more urgent. |
Livestock and human health |
Livestock-derived foods provide a variety of essential nutrients and other health-promoting compounds, many of which are lacking in diets globally, even among those populations with higher incomes. Well-resourced individuals may be able to achieve adequate diets while heavily restricting meat, dairy and eggs. However, this approach should not be recommended for general populations, particularly not those with elevated needs, such as young children and adolescents, pregnant and lactating women, women of reproductive age, older adults, and the chronically ill. The highest standards of bio-evolutionary, anthropological, physiological, and epidemiological evidence underscore that the regular consumption of meat, dairy and eggs, as part of a well-balanced diet is advantageous for human beings. |
Livestock and the environment |
Farmed and herded animals are irreplaceable for maintaining a circular flow of materials in agriculture, by recycling in various ways the large amounts of inedible biomass that are generated as by-products during the production of foods for the human diet. Livestock are optimally positioned to convert these materials back into the natural cycle and simultaneously produce high-quality food. Ruminants in particular are also capable of valorising marginal lands that are not suitable for direct human food production. Furthermore, well-managed livestock systems applying agro-ecological principles can generate many other benefits, including carbon sequestration, improved soil health, biodiversity, watershed protection and the provision of important ecosystem services. While the livestock sector faces several important challenges regarding natural resources utilisation and climate change that require action, one-size-fits-all agendas, such as drastic reductions of livestock numbers, could actually incur environmental problems on a large scale. |
Livestock and socio-economics |
For millennia, livestock farming has provided humankind with food, clothing, power, manure, employment and income as well as assets, collateral, insurance and social status. Livestock-derived foods are the most readily available source of high quality proteins and several essential nutrients for the global consumer. Livestock ownership is also the most frequent form of private ownership of assets in the world and forms the basis of rural community financial capital. In some communities, livestock is one of the few assets that women can own, and is an entry point towards gender equality. Advances in animal sciences and related technologies are currently improving livestock performance along all above mentioned dimensions of health, environment and socio-economics faster than at any time in history. |
Outlook for livestock* |
Human civilisation has been built on livestock from initiating the bronze-age more than 5000 years ago towards being the bedrock of food security for modern societies today. Livestock is the millennial-long-proven method to create healthy nutrition and secure livelihoods, a wisdom deeply embedded in cultural values everywhere. Sustainable livestock will also provide solutions for the additional challenge of today, to stay within the safe operating zone of planet Earth’s boundaries, the only Earth we have. |
For scientific evidence please refer to presentation recordings from the 19/20 October 2022 International Summit on the Societal Role of Meat. Evidence has also been published in a Special Issue of Animal Frontiers, volume 13, issue 2, April 2023. |
* The wording of this paragraph is from the Solution Cluster on Sustainable Livestock at the UN Food System Summit 2021. |
Critique of the 2019 Global Burden of Diseases, Injuries, and Risk Factors study
In 2020, an otherwise highly respected research group from the University of Washington, with a 30-year history of contributions to global health metrics, published a questionable cohort study in The Lancet (Murray et al. 2020). This was an updated version of the usually highly regarded, standardised and comprehensive estimates of the Global Burden of Diseases, Injuries, and Risk Factors (GBD), which are used extensively by governments and non-government organisations to set policies and monitor progress over time in regard to policy targets. In the GBD 2019 publication (2019 estimates), Murray et al. (2020) diverged substantially from the previous 2017 estimates (Stanaway et al. 2018). The 2017 GBD analysis attributed 25 000 deaths to diets high in red meat (least important of 15 dietary factors). However, the GBD 2019 publication estimated diets high in red meat to be responsible for 896 000 deaths, an increase of 36-fold, making red meat the fifth leading dietary risk factor for mortality related to a range of chronic diseases (Stanton et al. 2022). One specific conclusion made by the group responsible for GBD 2019 was that the theoretical minimum risk exposure level for red meat was set at 0 g/day, indicating that risk started with even minimal intakes of red meat.
How could the two studies reach such conflicting conclusions? Previous GBD risk-factor analyses used data from published, peer-reviewed, systematic reviews and meta-analyses but the GBD 2019 Risk Factors Collaborators did not identify which peer-reviewed publications were used in their updated conclusions, instead stating there was ‘sufficient evidence supporting the causal relationship of red meat intake with ischaemic heart disease, breast cancer, haemorrhagic stroke, and ischaemic stroke’ (Murray et al. 2020, supplementary material p. S283) and added these outcomes to previously identified relationships with diabetes and colon cancer.
However, this is not in agreement with other recently conducted systematic reviews and meta-analyses (Stanton et al. 2022). In a meta-analysis of cohort studies by Zeraatkar et al. (2019), it was found that there was low- to very low-certainty evidence that diets higher in unprocessed red meat may result in risk of cardiovascular disease, stroke, myocardial infarction, type 2 diabetes, and overall lifetime cancer mortality.
This lack of quantitative evaluation and rigour in science in GBD 2019 was highlighted in presentations at the Dublin summit from Alice Stanton and Bradley Johnston (Johnston et al. 2023), who stressed the need for rigorous approaches to meta-analytical data. The lack of appropriate methods to pooling of data in GBD 2019 represents a failure of editorial method. Stanton et al. (2022) noted the lack of adherence in the article to the basic tenants of quantitative analysis, including a failure to utilise Guidelines for Accurate and Transparent Health Estimates, and the failure to use Preferred Reporting Items for Systematic reviews and Meta-Analyses (Moher et al. 2009).
The result of these failures and others highlighted by Stanton et al. (2022) led those authors to conclude that ‘it would be highly inappropriate and imprudent for the GBD 2019 dietary risk estimates to be used in any national or international policy documents, nor in any regulatory nor legislative decisions’. The most important lesson from the problems with GBD 2019 is that rigorous reviewing standards must be applied to all papers submitted. While meta-analysis of randomised controlled trials is the strongest form of evidence, meta-analysis of observational studies, such as many of those contributing to GBD 2019, is not as robust and must be subject to careful scrutiny.
In addition to inappropriate analysis, undue reliance was placed on dietary studies of western developed countries being representative of humanity in general. When anthropological investigation of human dietary habits and food reliance are conducted across multiple societies, including many in developing countries, the results show a vastly different picture of meat consumption and life expectancy, child development and overall health. A recent analysis of life expectancy across 175 societies, including many in developing countries where food supply and breadth is limited, showed a positive correlation between meat consumption and life expectancy (You et al. 2022).
The GBD 2019 Risk Factors Collaborators also ignored the additional deaths and illness from iron-deficiency anaemia, sarcopenia, and child and maternal malnutrition that would result from zero red meat intake, particularly in developing countries where red meat is a critical aspect of non-diverse and marginal diets.
Of great concern to both human health and the meat industry is the international impact that this misinformation is having. Since publication, GBD 2019 (Murray et al. 2020) has been cited by at least 635 documents, including 351 scientific papers and nine policy documents. The extensive quoting of GBD 2019 risk-factor data in the evidence document of the UK’s National Food Strategy (Stanton et al. 2022) is a significant example of the potential negative impact of misinformation.
Dublin Declaration conference themes
Further details and evidence for the following summaries of the conference themes are available from published conference publications in Animal Frontiers, volume 13, issue 2, 2023. The themes are similar to those examined by Leroy et al. (2022).
The role of meat in diet and health
The first of the evidence-based themes for the Dublin Conference focussed on the role of meat in the diet and health and opened with a talk on the evolution of hominins and the close association with meat eating (Leroy et al. 2023). The introduction of meat eating was initially via scavenging, then hunting and finally by animal domestication. The idea that meat consumption is detrimental to health is at odds with the long history of human and prehuman hominin reliance on animal-sourced food (Mann 2018).
There are numerous highly published fields of investigation used by anthropologists to deduce the evolutionary diet of our evolving hominin ancestors, and they include the following: (1) changes in cranio-dental features; (2) fossil isotopic chemical tracer methods; (3) comparative gut morphology of modern humans and other mammals; (4) the energetic requirements of developing a large ratio of brain to body size; (5) optimal foraging theory; (6) dietary patterns of surviving hunter-gatherer societies; (7) specific diet-related adaptations; (8) fossil evidence of animal butchery (Mann 2000, 2018) and; (9) coevolution of mammalian hosts and their various parasites. Cestodes of the family Taeniidae, for example, are parasites of carnivores, spread by eating meat (Henneberg et al. 1998).
The study of dietary patterns of surviving hunter-gatherer societies also gives us a clear picture of what our pre-agricultural ancestors consumed (Mann 2018), showing that the majority of such societies obtained between 56% and 65% of their subsistence (dietary energy) from animal-sourced foods (Cordain et al. 2000). Palaeobiological studies of the transition from gatherer-hunter-fisher diets to agriculture subsistence have shown nutritional deficiencies, infection, and metabolic perturbations associated with reduced meat consumption and dietary diversity (Chinique de Armas and Pestle 2018).
Essential nutrients and nutrient availability
Humans exhibit a range of specific adaptations demonstrating extensive reliance on animal-sourced foods in the diet (Mann 2018). These include (1) an inefficient ability to elongate plant-rich 18 carbon omega-3 fatty acids into the 20- and 22-carbon polyunsaturated fatty acids (PUFA; Emken et al. 1992), required for structural and functional purposes in humans, (2) decreased ability to synthesise the amino sulfonic acid taurine (found only in animal tissue; Chesney et al. 1998), and (3) haem iron-rich compounds derived only from animal foods are absorbed by humans in preference to ionic forms of iron common in plants. Herbivorous animals cannot absorb these haem complexes and rely on absorption of the ionic form of iron (Bothwell and Charlton 1982).
From a broader perspective, meat is a critical source of high-quality protein and indispensable amino acids (IAA). It is essential to consider not only the amino acid content, but also digestibility of the indispensable amino acids in a food (Leroy et al. 2023). For meat, the digestible indispensable amino acid score (DIAAS; Moughan 2021) is in the range of 0.8–1.4, whereas values for most traditional plant proteins are markedly lower due to limiting IAA or reduced availability (Marinangeli and House 2017). Moughan (2021) showed that more than 100 countries faced inadequate protein supply for their populations after consideration of bioavailability. These were predominantly lower-income countries, and the poorer bioavailability was attributed to low dietary diversity, including minimal access to animal-sourced foods (Leroy et al. 2023).
There are also several key micronutrients of importance for health, and in particular for human brain development, structure and function. These include iron, zinc, vitamin B12 and long-chain omega-3 fatty acids. If not supplemented, these nutrients are either obtained exclusively from animal-sourced foods or are more bioavailable in those foods. It is phytate, the storage form of phosphorus in plants, that binds and reduces the availability of minerals, amino acids and energy; this is well established in monogastric nutrition (Selle et al. 2000; Bryden et al. 2007). Moreover, there is human evidence that plant-based diets decrease bone health (Ma et al. 2021; Falchetti et al. 2022) and increase the incidence of depression (Lee et al. 2021; Jain et al. 2022). In addition, meat contains a range of other B vitamins that can be limiting in micronutrient-poor diets, including thiamine and niacin (Leroy et al. 2023).
The importance of meat in the supply of critical nutrients was reinforced through a presentation on the modelling of the global food system (Smith et al. 2022b). The modelling highlights the value of bioavailability of protein, specific amino acids, iron and zinc, when evaluating the potential to deliver essential nutrients to meet the growing demand for food through to 2050. Smith concluded that meat supply is critical to the delivery of micronutrients to provide adequate diets for humans on a global basis (Leroy et al. 2023).
The role of meat in a sustainable environment
Deeper understanding of the interactions among livestock, the environment and climate are a pre-requisite to achieving sustainable farming and food-production systems. Valuable perspectives on the interactions among livestock, the environment and climate were presented at the meeting. The contribution of pastoral management of ecosystems was explored and the integration with, and importance to society was highlighted. Manzano et al. (2021) estimated that 60% of the terrestrial mass is used by the pastoral industries in over 100 countries. These ‘open ecosystems’ have developed over the past 15 million years in association with extinct megafauna. The current Bovidae are an example of megafauna and are prevalent in these open ecosystems and with current management may have a methane output similar to that before European settlement in North America (Hristov 2012).
Some parts of the world are very dependent on pastoral systems for wellbeing and economy; for example, 88% of agricultural GDP in Mongolia and 60% in Kenya depend on pastoral systems. Manzano et al. (2021) and Leroy et al. (2022) argued for greater recognition of the complexity of evaluating food production systems and for transdisciplinary approaches to address the suitability and societal value of pastoral systems, while supporting the environmental sustainability of the systems.
A consistent theme that emerged from the presentations of Manzano and Rowntree at the Dublin Conference was the need to ensure that the correct metrics are used to evaluate the sustainability of environmental impacts of pastoral systems and animal production (Manzano et al. 2023; see Box 2). For example, the continued use of global warming potential (GWP100) metric where methane has 27–30 times the warming potential of carbon dioxide over 100 years needs to be balanced against GWP*, which recognises that methane has a short half-life of about 12 years. The GWP100 metric overestimates the warming potential of methane when methane emissions are stable or falling and underestimates warming when methane emissions are increasing (Place et al. 2022). Utilisation of the non-human edible biomass by ruminants grazing pastoral systems transforms the biomass into edible human protein and micro-nutrients. This theme was reinforced in the presentation by Wilhelm Windisch who explored the role of grasslands and nutrient circularity in animal agriculture. In central Europe, each kilogram of plant-based food has been estimated to be associated with at least 3–4 kg of non-human edible biomass from best-practice agriculture. Globally, the relative amounts of non-human edible biomass are far higher (Windisch 2021; Thompson et al. 2023). This is important when considering the environmental impacts of plant- and animal-sourced foods. Further, Windisch (2021) and Smith et al. (2022a) noted that that there is little competition between plant-based and animal-based production and argue that they are complementary. It is critical to evaluate the efficiency of livestock production in terms of production of bioavailable essential nutrients for humans. Use of this metric will highlight the limitations of a singular reliance on plant-based diets and the value of meat in the diet.
Box 2.Definitions |
Definitions and efficiency metrics for animal production |
Sustainability: an attribute of a management strategy for a defined area or region. The parameters defining the outcomes of the strategy must be measurable and time bound, with an emphasis on longer time frames (decades or greater). Inherently, a sustainable strategy must address maintenance of productive function, economic viability, biodiversity including phylogenetic diversity, ecosystem function, social acceptability. |
Arable: land that is fit for the purpose of growing crops. |
Biogenic gas: a gas critical for and produced by living organisms. |
Concentrates: feeds lower in fibre, especially fibre >0.5 cm and include grains, by-products of flour and ethanol production, protein meals, pulses and whole cottonseed. |
Global warming potential (GWP100): means of quantifying the strength of different green-house gases (GHG) relative to carbon dioxide. It is derived from estimating the total change in atmospheric energy balance resulting from a pulse emission of the gas, relative to CO2, over a specified time frame (typically 100 years). Methane (CH4) is estimated to have a GWP100 of 27–30. |
GWP*: a metric developed to better reflect the effect of short-lived climate pollutants (SLCP). For GWP*, the time-integral of the rate of change of SLCP emissions over any given time period, or, equivalently, the change in SLCP emission rates between the beginning and end of that period, multiplied by GWPH (H = time period), gives total CO2-e* emissions over that period. |
Greenhouse gas (GHG): a gas that can capture and retain heat from sunlight, thus warming the atmosphere. |
Maintenance: the energetic costs of metabolic service and repair functions that keep an animal alive without retaining energy or being productive. |
Metabolisable energy: energy (ME) obtained from the diet able to be utilised by the animal for maintenance and production; the application of ME implicitly considers losses in production of net energy. The ME accounts for gaseous energy losses in utilisation of digestible energy. |
Efficiency measures |
Gross emissions: total emissions produced. |
Net emissions: emissions produced minus carbon fixed over a defined life-cycle. |
Life-cycle analysis: accounts for all emissions produced and fixed from production to consumption. |
Intensity of production (GHG or methane): amount of livestock product produced (kg live weight, meat or milk) per unit of GHG or methane. |
* Efficiency of production on human-edible intake basis: metabolisable energy for human consumption divided by gross energy consumed of human edible food (J per J). |
*Net protein contribution of meat production: efficiency for converting the human-edible portion of the diet into human-edible protein (meat) × (digestible indispensable amino acid score for meat/digestible indispensable amino acid score of the diet). A score of >1 means more human-edible protein produced than consumed. |
*The latter measures can be extended to all essential nutrients produced and consumed. |
https://www.epa.gov/ghgemissions/understanding-global-warming-potentials |
Water definitions |
Blue water: the water found in rivers, lakes, dams, and aquifers. Blue water is used for irrigation and for industrial and domestic use. |
Green water: the water available in the soil for plant growth and equal to the volume of water lost through evapotranspiration. |
Grey water: water that has been previously used and may contain impurities, including leached nutrients and pesticides following agricultural use. Grey water is usually reused, especially during droughts. |
Virtual water: the volume of water used to make a product and is the sum of the water use throughout the production chain, not just the water in the product. It may contain green, blue, and grey water. |
The role of meat in society, economics and culture
Often meat is considered only as part of the diet and yet it has a much more diverse role (CAST 1999). Whether in an African village or a small town in rural Australia, livestock production can significantly affect economic prosperity. In developing communities, the productivity of local livestock also has implications for health and education.
In examining how global nutrient supplies from food match up to global population requirements, it is evident that meat contributes the majority of the global vitamin B12 supply (DELTA Model®), as well as a quarter of vitamin A (in retinol equivalents), and high proportions of other B vitamins and several minerals such as iron and zinc, despite meat making up only 7% of global food mass and 11% of global food energy, providing further evidence for its nutrient density (Smith et al. 2022b). Importantly, this global picture does not capture regional variation. In the case of unprocessed red meat, the average per capita daily consumption is estimated at just 7 g in southern Asia, 24 g in Sub-Saharan Africa, 36 g in the Middle East and 45 g in northern Africa. Values are much higher in high-income countries, 51 g globally, 68 g in Latin America and the Caribbean, 87 g in Southeast and eastern Asia, and a sizable 114 g in central or eastern Europe and central Asia (Miller et al. 2022). Regions with the lowest intake also show the highest prevalence of malnutrition (Adesogan et al. 2020; Leroy et al. 2023). For instance, resource-poor countries suffer from a high prevalence of juvenile stunting and other forms of malnutrition, in part due to inadequate dietary diversity and a heavy reliance on a single staple (typically cereal grains) for daily energy needs (Ranum et al. 2014; Leroy et al. 2023).
In many countries of Sub-Saharan Africa and southern Asia where meat intake is very low, and malnutrition is high, animal husbandry is a critical aspect of economic survival as well as nutritional necessity, particularly for the young. These populations could benefit from an increased rather than reduced meat intake (Randolph et al. 2007; Adesogan et al. 2020). A prominent feature of this session was the role of meat as a dietary ingredient for children, pregnant and lactating women, women of reproductive age, older adults, and individuals in low- and middle-income countries (Leroy et al. 2023). Animal-sourced foods, such as meat, are the best source of nutrient-rich foods for children in their first 1000 days (a finding supported by the World Health Organization), leading to normal growth and development and compelling benefits on cognitive functions (Balehegn et al. 2022). Thus, global efforts to moderate meat intake for environmental or other reasons should be careful not to restrict its growth in populations where consumption is already low, as this could hinder progress towards reducing malnutrition and thereby not address human suffering and the stifling of economic development (Wong et al. 2017; Balehegn et al. 2019).
In many regions where there is a lack of animal-sourced food in the diet, the population is also exposed to mycotoxins in their food staples (especially maize and peanuts). Chronic exposure to mycotoxins in developing societies have been shown to significantly affect public health by reducing infant growth and development, causing immunosuppression, and increasing the risk of cancer, especially hepatocellular carcinoma (Wu et al. 2014; Wild et al. 2015; Bryden 2019). Reduced access to animal-sourced foods in these societies will further decrease public health as facilities for food storage are often rudimentary, facilitating fungal growth and mycotoxin production in stored grains. Moreover, in developed countries there is increasing pressure to shift from red meat to plant–based meat analogues. This neglects natural toxin contamination, especially by mycotoxins of these plant products (Mihalache et al. 2022a), as has also been found in plant-based milks (Arroyo-Manzanares et al. 2019) Although reducing meat consumption does decrease some diet-related health risks, the overall risk to health is increased by the consumption of plant-based meat substitutes (Mihalache et al. 2022b). In other surveys, it has been also found that populations that exclude animal-sourced foods from their diets have greater exposure to mycotoxins (Leblanc et al. 2005; Penczynski et al. 2022).
The crucial role of livestock in Africa was outlined by Shirley Tarawali from the International Livestock Research Institute (Baltenweck et al. 2020; Tarawali 2022; Ederer et al. 2023). Some 23% of the world’s cattle are in Africa, supplying about 8% of the world’s meat, contrasting this to the USA and Europe where cattle populations total 6–7%, yet supply 15–18% of the world’s meat, clearly showing the low productivity of the African livestock systems. More broadly, in low- to middle-income countries, 1.7 billion people derive some livelihood from livestock and poultry and over half a billion depend on livestock and poultry. Improving livestock productivity and health in these countries is crucial for sustaining incomes, reducing financial risk, growing wealth to ‘step out’ of poverty, gender equality and of course adequate nutrition (Alders and Pym 2009; Wong et al. 2017; de Bruyn et al. 2020). It was concluded that a concerted global effort was needed to support livestock productivity (and so reduce methane intensity) in low- to middle-income countries to improve the standards of living.
The potential for in vitro or cultured meat production was examined by Paul Wood (Wood et al. 2023). The overall process is to culture muscle cells in bioreactors in highly controlled and sterile conditions similar to those used for growing microbes to produce medical and other products (Bonny et al. 2017; Ellies-Oury et al. 2022). It was concluded that the technology was well established and that cultured meat will appear on the market for consumers. Various private investors have claimed that the cultured-meat scenario will have benefits over livestock, citing lower carbon footprints and environmental damage, plus improved animal welfare due to less animal killing. However, the conclusion was that the technology will never be able to scale at the commodity level to represent a real alternative to traditionally raised meat. Moreover, it will never be a solution to providing high-quality nutrition in low- to middle-income countries.
Similar arguments apply to plant proteins processed to resemble animal-based foods and also referred to as meat substitutes, meat analogues, fake meat, and mock meat (Boukid 2021). It is worth noting that the environmental footprint of plant-based meat substitutes determined from life-cycle analyses may be lower than that of feedlot beef, but higher than that of beef produced on pasture (van Vliet et al. 2020).
Global world food flows were examined by Peer Ederer from GOAL Sciences who have developed a modelling platform called ‘Planet Food System Explorer’ (Ederer et al. 2023; GOAL Sciences 2023). The modelling conclusively shows that, on a global basis, both the cost and supply of calories for humans is theoretically enough to meet world demand if there were equitable distribution. However, the supply of bioavailable protein and nutrient-rich foods is more precarious, with over a third of the world population being unable to afford these nutrients. Animal-sourced foods, especially dairy, poultry and eggs, are very cost-competitive on a world stage. Indeed, the cost of bioavailable, high-quality protein from poultry and eggs is cheaper than the same nutrients derived from cereals, meaning that, from a bioavailable-protein perspective, it is better to feed cereals to chickens than to humans! It was also argued that increasing alternate protein supplies especially from grain legumes has proved problematic due to agronomic difficulties in many climatic and geological scenarios, along with the issue of anti-nutritional compounds that legumes contain. In addition, the view that livestock feed grain competes for human food was shown to be flawed, with, for example, only 5% of the world ruminant diet consisting of grains and 86% of the world livestock being fed non-human-edible products (Mottet et al. 2017; Leroy et al. 2022; Ederer et al. 2023). A troubling trend for competition between animal feed versus petroleum production was also highlighted, adding further constraints on world protein production. Finally, it was concluded by Ederer et al. (2023) that depending on what value is chosen for the average protein requirement per person per day, the world protein supply (from all sources) is either adequate, or needs to expand by up to 80%. If this is projected out to 2050, on the basis of population growth estimates, then global protein supply needs to increase by 20–150% over the current levels. These analyses have highlighted the important role of food production from both animals and crops (Ederer et al. 2023).
The ethics of meat consumption were examined by Candace Croney who asked the following question: ‘Is eating meat morally defensible?’ (Croney and Swanson 2023). While the strength of the scientific argument was based on increased global food demand, historical and cultural prominence, nutritional benefits, increased demand in developing and emerging economies and technological developments that distribute benefits, Croney also discussed the perspective that there can be arguments against meat consumption. Ethics questions are more likely to emerge when science is equivocal and ethics questions are more prevalent when there is affluence and choice; however, ‘public opinion’ is diverse. In other words, distinct differences exist among consumer populations and their perceptions and needs. For affluent countries, food is more than sustenance and reflects values and social justice, with many responding to advocacy groups and others seeking to reduce meat consumption (McKendree et al. 2014). Arguments that meat eating does harm include animal suffering and death, has negative impacts on natural resources, ecosystems and local communities, and aspects of worker health and safety and quality of life. However, these arguments have often been unduly simplified, ignored important stakeholders and have poorly framed arguments (de Bruyn et al. 2020; Thompson et al. 2023). Indeed, as discussed by Leroy et al. (2022), crop production, the basis of a diet that excludes animal products, comes with an animal death toll that may be even higher than that from large-animal husbandry and slaughter. There are strong positive arguments for the environmental sustainability of meat production and the application of the least harm principal (Regan 1983; Davis 2003) indicates that animal welfare will be best served by inclusion of meat from large herbivores in the diet, rather than having diets that exclude animal products. In terms of equitable access to food, many do not have the luxury of choice and should have access to meat, as malnutrition is still prevalent among children and females when access to high-quality proteins and micronutrients is limited. The latter theme was explored in depth at the meeting. Croney and Swanson (2023) concluded that eating meat was morally defensible, as have others (de Bruyn et al. 2020).
Societal role of livestock and meat in Australia
Australia has a large land area predominantly suitable for extensive ruminant production but a relatively small population, thus a high proportion of ruminant products are exported. In addition, to the beef, sheep and dairy industries, Australia has very efficient pig and poultry production that includes the pig meat, chicken meat and egg industries. These non-ruminant industries largely cater for the domestic market and have been included in this section to reflect the meat-intake pattern of Australian consumers.
Australian agriculture
Approximately 55% of Australia’s land mass is used for agriculture (325 million ha), of this, approximately 283 million ha, or more than 85%, is native vegetation used for grazing, another 35–41 million ha is improved pasture and only 21–31 million ha is used for crops in any one year (Australian Bureau of Statistics 2016–2017; Australian Bureau of Agricultural and Resource Economics and Sciences 2022a); this reflects the scarcity of arable land in Australia (Fig. 1). The area dominance of grazing native vegetation reflects the importance of the pastoral grazing systems in northern Australia. About 60% of Australia’s beef cattle is in the northern tropics and subtropics. Large areas of land in this region are not suitable for arable agriculture, and thus livestock industries based on the grazing of native pastures remain the only, or one of very few, financially viable industries in these areas. A similar assessment can be made of the southern rangelands.
Areas of agricultural use in Australia as extracted from Snapshot of Australian Agriculture (Australian Bureau of Agricultural and Resource Economics and Sciences 2022a).
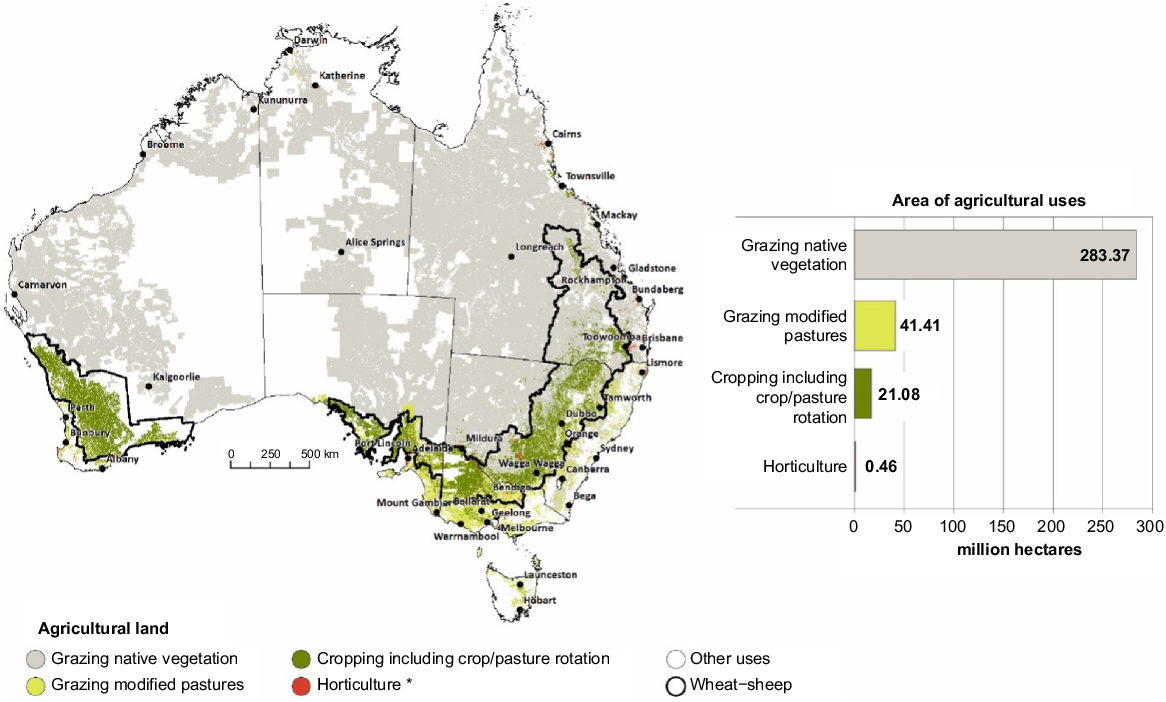
Although much smaller in size, the mixed farming zone is a key driver of food production in Australia. The agro-climatic regions supporting mixed farming range from the winter-rainfall Mediterranean and temperate climates of south-western Western Australia and southern South Australia into the wet temperate regions in Victoria, Tasmania and New South Wales, then up through to the subtropics in southern and central Queensland (Bell et al. 2019; Fig. 1). The zone produces most of Australia’s crops and 40% of the livestock; approximately 83% of the cropping farms include livestock (Bell et al. 2014, 2019).
Meat in the Australian diet
The role of meat in diet and health in Australia is similar to that described earlier for other countries and populations. Australians are among the highest meat eaters in the world, with meat consumption at more than 100 kg per capita for each of the past 60 years (Wong et al. 2015; Whitnall and Pitts 2020). However, overtime, the relative importance of different meats has changed. Beef dominated intake until about 15 years ago and now chicken meat (47.2%) dominates Australian consumption, followed by pork (28.4%), beef and veal (18.9%) and lamb (5.5%) (Australian Bureau of Agricultural and Resource Economics and Sciences 2022a). Australians do have access to a series of books based on the Commonwealth Scientific and Industrial Research Organisation (CSIRO) Total Wellbeing Diet (Noakes and Clifton 2005, 2006). The books, written for Australians, are targeted at consumption of a healthy diet to facilitate weight loss and allow for a moderately high level of animal protein intake (Noakes et al. 2005). The diet is also based on a high intake of fruit and vegetables, low in saturated fat and alcohol and encourages moderate physical activity (Noakes and Clifton 2008). The Level 1 plan suggests 200 g of red meat four times weekly, which is within the Australian Guide to Healthy Eating (NHMRC 2013).
The importance of meat in the Australian diet has been long recognised (Baghurst 1999; Nutrition and Dietetics 2007). The special issue of Nutrition and Dietetics (2007), commissioned by Meat and Livestock Australia contained 20 papers by leading Australian nutrition academics and scientists and covered the nutrient content of meat, the role of red meat in nutrition, in diets at different life stages, and management of chronic disease. As stated in the special issue, the conclusions to the role of red meat in healthy Australian diets’ ‘concur with nutrition statements from the National Heart Foundation of Australia, and have the support of the Dieticians Association of Australia as a useful summary of the contribution of red meat to healthy eating.’
Animal-sourced food and efficiency of production
Costa (2007) and Bell et al. (2011a) provided concise reviews of the challenges and opportunities facing meat production by the Australian livestock industries. Australia is a significant supplier of food, particularly animal protein, to the world. Australia is the fourth largest beef exporter and the world’s largest exporter of sheep and goat meat (Meat & Livestock Australia 2022); beef exports represent 14% of the world market. Of total beef and veal, lamb and mutton produced, 78% is exported to over 100 countries, emerging, developing and developed. The dominant market for beef is Asia, with Japan, South Korea and China being the largest markets. Indonesia was Australia’s largest market for live cattle exports in 2021 (52%), followed by Vietnam (22%) and China (13%). More than 700 000 head of cattle were exported alive in 2021. For sheep meat, the highest sales were to China (30%), followed by the United States (23%) and Malaysia (6%). Live sheep exports in 2019 were more than 550 000, with Kuwait (60%) as the primary destination (Meat & Livestock Australia 2022). These exports both as meat and live animals represents a critical contribution to the intake of high-quality protein and other essential nutrients (particularly essential fats, vitamins and minerals) in many parts of the world. Part of the reason for Australia’s success in growing and exporting meat is efficient systems for conversion of plants to products.
Most of Australia’s cattle are grazed in the rangelands of the monsoonal north, part of the 85% of agricultural land where rainfall is usually too low or erratic to support crops or allows only limited pasture improvement. Without livestock, this zone would produce little or no food at all and the plant resources growing there would not contribute to feeding people inside and outside of the country. The value of grazing is best demonstrated through calculation of net protein contribution (NPC) for a grazing system (Box 2). NPC represents the amount of human-edible protein produced, divided by the amount of human-edible protein consumed by the animal (both produced and consumed, adjusted for amino acid value). In a grazing system that uses a small amount of human-edible protein for feed supplements, an NPC of 1597 has been calculated, that is, 1597 kg human-edible protein produced for every kilogram consumed (Thomas et al. 2021a). It is not a choice between animal and plant protein production, but between animal protein and no protein/food production at all.
In northern Australia, the quality of native pastures is usually low. High rainfall and leaching keep essential nutrients, especially nitrogen, low in the soil profile, and plants are, therefore, usually low in protein. Due to its fragile environment, it has been recognised for many years that it is an area that must be carefully managed (Ferguson 1973). The wet, monsoonal-zone native pastures have high levels of structural carbohydrates, high cellulose and lignin contents, and low digestibility (Minson 1990; Charmley et al. 2023), making it difficult for livestock to obtain sufficient energy for rapid growth. Many of the soils of northern Australia are low in phosphorus, and the plants usually have also low phosphorus content and phosphorus deficiency of cattle is prevalent without supplementation (Dixon et al. 2017; Schatz et al. 2023). Less than 5% of the pastures in these regions have been improved with fertilisers and introduced pasture species. Expenditure on fertiliser for large and medium beef properties in northern Australia has been low to negligible (Lean et al. 2011). The advantages of the extensive pastoral system are the low cost of pasture, while the disadvantage is the great variability in pasture production reflected in a marked variation in growth and development of animals.
The northern pastoral zone is characterised by low-cost pasture and cyclic forage variability. The major constraints to production are under-nutrition, both in amount and quality of feed, costs of management inherent to such extensive properties, and heat stress and parasites and disease (Bell and Sangster 2023). Poppi and McLennan (1995) reviewed major limitations to the beef production system arising from deficiencies in the energy and protein content within the dominant pastures of the northern beef industry and explored the interactions among these major nutrients. These limitations are often expressed in poor reproductive efficiency (see McGowan et al. (2014); this report has since been published in 2023 in a series of papers in Animal Production Science 63 issue 4).
Cyclic weight gain and loss that reflects the annual change in pasture quality and quantity, resulting in a low efficiency of production, is a characteristic of the system. However, Lean et al. (2011) reviewed 40 years of published weight-gain data from northern Australia and identified substantial variations (>1 kg/day difference) in the performance of the cattle on native pastures and further identified substantial (>70% improved weight gain) responses in some cases to supplementation. Bell and Sangster (2023) reviewed the substantial benefits from use of improved agronomic approaches to increase weight gains, including increased adoption and management of Stylosanthes species, Leucaena, Desmanthus cv and other tropically adapted legumes. These findings indicate the potential for the northern beef system to be more productive.
Stocking rates are often extremely low in the pastoral zone, with many hectares being allocated to a single animal (Tothill and Gillies 1992). The latter observation stresses the importance of grazing management under conditions where the energy loss from exercise during grazing or obtaining water will become significant determinants of performance. Bell and Sangster (2023) noted the extreme conditions of the pastoral zone and especially the Northern Forest and the very poor reproductive performance there (McGowan et al. 2014). These factors do lead to low energy-use efficiency (Hunter 2010); however, the key metric that indicates the merit in the pastoral system is that there are very little, to zero, human available nutrients used in this production system to produce beef, unless finished in feedlots, as discussed below.
Most of the agricultural land with modified or improved pastures and crops is part of the mixed farming zone. Most farms retain a mix of crops and livestock because together they are far more efficient in the production of food than is either separately. Crops can benefit the production of meat and wool without compromising the yield of crops for food production. For example, there is a rapidly increasing trend to graze crops in the vegetative stage (Radcliffe et al. 2012). Such crops have a high metabolisable energy (>12 MJ ME/kg DM) and protein content (>20% DM) suitable for pregnant or rapidly growing ruminants (Masters and Thompson 2016) and allow establishment of pastures through deferred grazing (Thomas et al. 2015). Provided grazing ceases before stem elongation, the yield of harvested grain is usually maintained (Harrison et al. 2011). Grazing young crops without loss in grain yield facilitates animal production, with no trade off in the production of food from plants. At the other end of the crop-growth cycle, a single hectare of post-harvest wheat residue provides sufficient metabolisable energy to support one dry sheep equivalent for 50–100 days (Thomas et al. 2021b). If retained, this stubble may decrease subsequent crop yield (Kirkegaard 1995); conversely, loss of stubble through burning and conventional tillage may increase susceptibility to erosion and soil carbon depletion (Chan et al. 2003; Chan and Heenan 2005). The implications of grazing are less clear, with some evidence from overseas that the mixing of stubble residue in soil caused by livestock trampling compensates for the loss of stubble from consumption, with a consequent increase the soil organic carbon (Stavi et al. 2016). Soil compaction due to grazing stubble was once considered a risk to crop production. This risk is no longer supported by the literature (Bell et al. 2011b). Grazing therefore offers another opportunity to convert a crop waste product into food or fibre, with 3–6.5 million ha of crop residue grazed annually (D. T. Thomas, pers. comm.). Finally, livestock offer opportunities to turn failed crops into food (Bell et al. 2009). When the cost of harvesting crops that have been constrained by low rainfall, poor soils or other external factors exceeds the financial return, grazing provides a means of turning a financial loss into an animal product.
Crops do not just provide an option for increasing livestock production, they can also benefit from the interaction, meaning more efficient production of plant-based food. Traditionally, legume pastures have been used in rotation with crops, providing grazing while simultaneously fixing nitrogen, improving soil structure and contributing to the management of weeds, pests and disease (Bell et al. 2019). Other cropping options such as canola have joined the rotations, facilitated by the availability of cheap nitrogen fertilisers; pastures have been displaced and degraded (Howieson et al. 2000). With increasing fertiliser prices, expansion of acid soils and widespread herbicide resistance, there has been a refocus on breeding new pasture options for livestock and crop benefits (HC Norman and DG Masters, Livestock preference and feeding value as key determinants for forage improvement – why not ask the consumer? Animal Production Science, under review).
More than 3.5 million head of cattle were turned off from Australian feedlots in 2022 (Atkinson 2022), representing a 100% increase in numbers over 20 years (Spragg 2018). Many of the 400 feedlots are sited close to the grain-producing regions of Australia, because costs of transport greatly increase the costs of feed and reduce the cost efficiency for those further away from grain-growing regions. Feedlot systems rely on a markedly different efficiency of conversion of feed intake to beef from cattle fed on either northern or southern pastures to be cost-effective. Typically, finishing diets for feedlot cattle contain 80–85% of concentrates, with processed grains, protein meals, molasses, minerals and feed additives and by-products being combined in mixer-wagons with 10–20% of forages, including cereal crop and maize silages, hays and straws, the latter being by-products of grain production. Spragg (2018) reviewed grain use in Australia for the 2017/2018 and 2018/2019 financial years, with wheat and barley being the predominant products (88% of all grain produced). Overall, grain fed to animals was about 30% of national production, with dairy and beef feedlots (48%) and chicken meat and eggs (31%) accounting for nearly 80% of feed grain usage. While it has been argued that feedlot systems are inefficient because the grain use would be better fed to humans, a large proportion of the grain used in feedlots, and indeed animal nutrition in Australia, is at the lower end of quality standards with respect to suitablility for human consumption and often just classified as feed grain, based on cultivar (e.g. sorghum, non-malting barley) and physical characteristics such as rain-damage and suboptimal specifications (Anonymous 2009, 2020, Black et al. 2023). More importantly, there is evidence of an NPC >1 (1.96), even when cattle are finished within a feedlot and fed a cereal-based ration with a significant amount of human-edible protein (Thomas et al. 2021a). The production system, combining grazing and feedlot, results in a net increase in human-edible protein. Further, beef and dairy cattle are fed by-products of ethanol production, human food production and cotton production. These products include dried distillers’ grains, wheat by-products from flour manufacture, including wheat mill run and middlings, canola meal, sunflower meal, and whole cottonseed. Alternate uses for many of these products are limited and the use of these in cattle production lowers the costs of production for ethanol, flour, bread, processed wheat products, cooking oils and cotton.
Cattle for the local market are often fed for approximately 70 days in the feedlot, those for export and premium local markets 100–120 days and those for speciality long-fed markets, particularly Wagyu cattle, 200–230 days or more. In terms of GHG, many feedlot cattle are fed ionophores that reduce methane production (Ranga Niroshan Appuhamy et al. 2013), and the shorter time to slaughter than for growing cattle on pasture has a marked reduction in methane per kilogram of beef (methane intensity). The use of feedlots to finish cattle from the northern pastoral system represents a profound increase in GHG efficiency over traditional finishing at a much older age.
There are 1.4 million dairy cows in Australia and a similar number of young cattle, heifers and calves in the national dairy herd (Australian Bureau of Agricultural and Resource Economics and Sciences 2022b). Milk production per cow has increased from 2848 to 6170 L per annum over the 40 years to 2020. This change has significant effects on GHG emissions intensity as the proportion of methane ascribed to maintenance is reduced. Over this time, the industry has changed from being seasonal and pasture-based (Lean 1987) to less seasonal and with greater reliance on a wide range of fodder crops, feed supplements and concentrate inputs (Rugoho et al. 2017). Victorian farms evaluated for the Dairy Farm Monitor fed 61% of the metabolisable energy consumed as homegrown forages (Anonymous 2021). The forages used are almost exclusively improved and ryegrass species dominant, especially in southern Australia. Legume forages, predominantly clovers and also lucerne, are used. Other grasses include fescues, paspalum, kikuyu and there is increased use of maize silage. Herbs including chicory and plantain are used in mixed swards. Dairy cattle make great use of the by-products noted previously, but also brewers’ grains, vegetable and fruit waste, confectionary, bread and biscuit waste. Spragg (2018) estimated the use of feed grain as 2.63 million tonnes for dairy in Australia. Beef is produced from dairy cattle with a high level of efficiency due to the reduced contribution to maintenance ascribable to the dual purpose of the cattle. Specifically, a beef female is not required to produce the calf. Increased use of sexed semen will allow increased production of beef semen on dairy cattle to produce crossbred cattle; however, Holstein steers are extensively used for beef production in the USA and Australian studies have confirmed that their eating quality matches that of beef breeds (Polkinghorne et al. 2023a).
In Australia, there is little human-edible food used to produce beef and milk. Many of the essential nutrients provided in beef and milk have zero human-edible food inclusion and this is the case for grass-finished beef. Using worst-case scenarios, that is assuming all grain fed was edible by humans, Lean and Moate (2021) found that 0–28% of lifetime nutrient intakes of energy, protein and essential fatty acids were derived from human-edible sources. As shown in previous examples, feeding these small amounts of human-edible foods to animals may leverage a net increase in human-edible protein (Thomas et al. 2021a). Cattle provide an efficient and productive means of turning human-inedible grain and food and ethanol by-products into high-quality foods, thereby reducing the costs of food overall and oxidation and gas generation from these wastes.
The pig and poultry industries have grown exponentially over the past 60 years as local demand for meat (chicken and pork) and eggs has increased, but are small compared with their global counterparts. Each industry in Australia is dominated by a few large companies and the number of farms in each industry has decreased significantly over time. The large companies have adopted a vertically integrated structure and this has facilitated intensification and allowed rapid adoption of new technology. Each industry has sought the best technology from around the world and invested heavily in research and this has resulted in the acquisition of superior genetics, improved nutrition, better disease control, more effective management practices, and improved animal welfare. Together, these have resulted in significant gains in animal performance and feed conversion, boosting overall industry efficiency and productivity.
Australian breeding sow numbers are approximately 265 000, producing 5.2 million pigs annually. Most pigs are sold at bacon weight (100 kg), with a dressed carcass weight of 77 kg, resulting in 420 000 tonnes of pig meat produced annually (Dalgleish and Whitelaw 2021). The major pig production areas are in south-eastern Queensland, southern New South Wales (NSW), northern and western Victoria, south-eastern South Australia, and south-western Western Australia. Farrow to finish is the conventional structure of Australian pig farms and in this system, the farm breeds, farrows, weans and grows out pigs for sale. Production in Australia is largely confined to intensive indoor housing systems, with only 10% of commercial breeding as free range. The Australian production system is focused on the fresh pork market as this product has little competition from the import market of processed meat where 160 000 million tonnes is imported annually, or about 30% of total pig meat use in Australia (Spragg 2018). Currently, 42 000 tonnes are exported from Australia annually (Spragg 2018).
With approximately 600 million broilers producing ~1.2 million tonnes of chicken meat annually, Australia has mirrored the increased global demand for chicken meat (RMCG and BDO EconSearch 2020). Major centres of chicken meat production have developed near major capital cities on the eastern seaboard. Approximately 800 growers produce about 80% of Australia’s meat chickens under contract to the major integrated or processing companies. Contract growers own the farm and provide the management, shedding, equipment, labour, bedding and other inputs to rear chickens from day-old to processing. The processing company provides (and owns) the chickens and provides feed, medication, and technical advice. Australian chicken meat is sold wholly into the domestic market, with some 70% being sold as fresh chicken (RMCG and BDO EconSearch 2020). Supermarkets represent the largest distribution channel, selling 40% of the fresh chicken meat. A very small volume of chicken meat is exported (4%). Chicken meat cannot be imported into Australia, to keep the industry free of major avian diseases. The excellent disease status of Australian poultry is maintained by strict biosecurity measures that exclude importation of poultry meat. This also has the effect of eliminating competition from chicken meat imports.
Australia’s annual egg production is currently 6.6 billion eggs, with a flock size of approximately 21.2 million laying hens (AEL 2022). The industry is dominated by large-scale producers, who sell directly to large retail sellers. As with the chicken meat industry, commercial egg farms are situated close to the major cities, but, in recent years, the industry has re-established near major regional centres. Most of the flocks are found in NSW, followed by Queensland then Victoria, with eggs being produced by birds housed in cages (31.1%), free-range (55.6%), barn (11.3%) or speciality production (2.0%) (AEL 2022). About 85% of eggs are sold into the domestic market, with export being limited by production and transport costs (AEL 2022).
Profitability of the non-ruminant industries is driven by feed efficiency, the cost of feed, genetic improvement, animal/bird health status, and, for the pig industry, competition from imported pig meat. Feed-related expenses comprise 60–70% of total production costs, thus minimisation of feed costs is very important. This is in part achieved by least-cost feed formulation, which allows incorporation into diets of ingredients such as millrun, a co-product of flour milling, oil seed meals that are the residues of vegetable oil manufacture, and feed grade grains or grains that do not meet the criteria for human food manufacturing. For example, in 2021, some 45% of the NSW wheat harvest was downgraded to feed-wheat due to preharvest germination and fungal deterioration (Black et al. 2023). Where grains suitable for human consumption are used, they usually facilitate the conversion of a range of non-human edible by-products and food waste into human-edible protein. In the pig industry, for example, the NPC for an intensive Australian pork supply chain has recently been reported as 3.26, indicating the production of 3.26 times more human-edible protein than is consumed (van Barneveld et al. 2023). The authors are unaware of similar studies for the chicken meat and egg supply chains. Both the pig and poultry industries have become increasingly successful at using co-product meals by incorporating speciality feed additives into diets to improve digestibility with feed enzymes and dietary amino acid balance with supplementary amino acids (Ravindran 2013; Kebreab et al. 2016). These feed additives not only improve feed utilisation but substantially reduce emissions (Kebreab et al. 2016). Moreover, it is often asserted that land used for producing animal feed, especially for cereal grains, could be used to produce human food, such a transition may not be feasible as humans usually require different crops or cultivars that have different agronomic features and require different environmental conditions (Owens and Hansen 2011).
The other major strategy to offset feed costs is to increase production efficiency. For example, the laying rate of hens has increased from about 161 to 320 eggs per year from 1973 to 2016. Number of piglets weaned per litter and number of litters per sow per year have gradually increased, with sows currently producing 23.6 pigs/year (Dalgleish and Whitelaw 2021). The pig industry is less resilient to increases in feed costs than are the chicken meat and egg industries, due competition from imported pig meat. Chicken meat obviously has a significant cost advantage to other meats as the price of chicken has remained relatively constant for many years, reflecting efficiency of feed conversion which has improved from 2.5 in 1975 to 1.6 in 2020 (ACMF 2022). In achieving improvements in productivity the pig, chicken and egg industries do not use hormones or antibiotics as growth promoters.
Livestock and a sustainable environment
All of Australia’s animal industries have strategies to reduce their environmental footprint and research priorities that include climate change and efficient use of resources. Across the red meat industries, Meat and Livestock Australia have asserted that these industries will be carbon neutral by 2030 (Meat & Livestock Australia 2021), with independent research suggesting that Australian sheep production for meat was climate neutral as of 2020 (Ridoutt 2021).
In the extensive pastoral regions, careful management of stocking rates to prevent overgrazing can contribute to the maintenance of biodiversity. There is the opportunity for increased soil carbon sequestration through increased growth of pastures, woody shrubs, weeds and trees, emphasising that the animal and, indeed, all the agricultural industries need to adopt net-emissions metrics (Mayberry et al. 2018). Lam et al. (2013) found that conversion of cropland to grazing increased soil carbon more than did conservation tillage, crop residue retention or nitrogenous fertiliser application over 31–40 years. There is also the opportunity to reduce carbon loss through reduced burning of grasslands and increased carbon sequestration in soil using dung beetles (Mayberry et al. 2018). Altering land use management to increase reforestation, reduce savanna burning practices, reduced deforestation, and, to a lesser extent, increase woody biomass growth were considered likely to have the most substantial effects in mitigating the effects of beef cattle production on GHG emissions in Australia (Mayberry et al. 2018). It also appears likely that such strategies would further enhance the benefits of beef production in maintaining biodiverse habitats. Such management options are consistent with the conclusions of others (Masters et al. 2010; Thompson et al. 2023) that well managed animal systems can convert large quantities of non-edible biomass to high-quality foods, while providing diversity and respecting ecological principles that offer ecosystem services.
The introduction of annual crops and pastures into a landscape previously dominated by perennial plants has led to a level of degradation and dysfunction. Most notable is the prevalence of acid soils, the spread of secondary salinity and erosion of unprotected soils following plant senescence. While overgrazing and annual pastures have contributed to these problems, a well managed livestock component to a mixed farm offers opportunities to address the problems. Salinity results from elevated water tables related to a lack of growing plants for much of the year. There are options to use salt-tolerant perennial plants in saline areas or other perennials in non-saline areas to manage the water table (Masters et al. 2006). Both these options can be used for livestock, but there are not yet any viable perennial crops available for this purpose. The introduction of a mosaic landscape suitable for crops and plants offers a landscape with a function more closely resembling the natural state.
Given that sustainability also includes economic viability to the farm or enterprise, there has been a move towards higher cropping intensity in the mixed farming zone, with some evidence of increased profitability (Kingwell et al. 2020); however, there is also recent economic analysis, based on whole-farm profit of mixed enterprises, indicating that the optimal cropping proportion of the farm is between 40% and 60% (Young et al. 2020), with livestock occupying a significant proportion of the land. Together with the higher whole-farm profitability described by Young et al. (2020), there is strong evidence that a mixture of cropping and livestock reduces the risk of income variability. From a study of six sites within the mixed farming zones across four states, Bell et al. (2021) concluded that the risk-efficient frontier included a mix of crops and livestock at most sites. Significantly, this study assessed the risks when the crop and livestock enterprises were run independently and did not take into account the production synergies (Bell et al. 2021).
The carbon footprint of dairy largely reflects enteric methane production from cattle (70%), by nitrogen use for crop and pasture growth (3.5%), methane from manure (13%) and generation of nitrous oxide from urine (12%; Eckard and Clark 2020). The dairy industry, nationally and internationally, has considerable initiatives towards reducing its environmental footprint (Food and Agriculture Organization of the United Nations and Global Dairy Platform Inc 2019). However, the drive towards increased production efficiency through increased production by increased concentrate use, use of ionophores, fats and oils is the major factor reducing the intensity of GHG production (Eckard and Clark 2020; Lean and Moate 2021). There has been a significant focus on reducing ruminal methane production through use of ionophores, fats and oils, red seaweed, 3-nitrooxypropanol, tannins, essential oils and other interventions (Lean and Moate 2021). The final efficacy and practical choice of products to reduce ruminal methanogenesis as it interacts with production system and diet quality is still under intensive research (Davison et al. 2020). Significant reductions in the GHG footprint are also offered through improved reproductive efficiency, production of beef from dairy cows, increased longevity and nitrogen inhibitors that reduce volatilisation of urinary urea. Initiatives in California have provided evidence that large extensive dairies can become carbon neutral through a combination of co-generation and solar capture (Liu et al. 2021). Application of these techniques should, in combination with increased production efficiency, a focus on animal longevity and further adoption of rumen modification, result in carbon neutrality for many Australian dairies.
The chicken meat, egg, and pig industries have a focus on reducing their environmental footprint in areas such as water management, waste minimisation, re-use and recycling, and reduction of greenhouse-gas output. Larger piggeries use methane gas to generate power for their facilities and sell surplus to the local power grid. It has been shown that the pig (Wiedemann et al. 2016, 2018) and chicken meat (Wiedemann et al. 2017; Copley and Wiedemann 2023) and egg (Copley et al. 2023) industries have had success in reducing their footprints. The largest impact that these industries have on the environment is growing the crops that are used for animal feed. Australia produces about 31.2 million tonnes of grain (wheat, sorghum, barley, oats, maize, lupins, and field peas, excluding canola and cottonseed), of which some 37% is used for domestic purposes (feed grain, flour grain, malting grain and retained seed; Spragg 2018). Of the 13.5 million tonnes of feed grain used by the animal industries, meat chickens (24.1%), laying hens (7.4%), and pigs (12.1%) consume some 5.9 million tonnes (Spragg 2018). The non-ruminant industries have reduced their reliance on imported soybean meal by increasing the concentrations of co-products and other protein meals, especially canola, in diets. There is growing interest in the use of human food wastes (Torok et al. 2022) and insect protein meals (de Souza-Vilela et al. 2019; DiGiacomo et al. 2019) as quantities become commercially available. This reduced reliance on traditional feedstuffs will reduce the environmental footprint of feed. Nevertheless, poultry production was shown to have the least environmental impact of all animal-sourced food, largely due to the efficiency of broiler chickens in converting feed into meat, which reduces the amount of feed and therefore the resources of land and water, required to produce each kilogram of meat (Copley and Wiedemann 2023).
The impact of animal production on freshwater resources is an additional challenge for the sustainability of the animal industries. It is particularly important in Australia, the world’s driest continent. Globally, water is scarce and water-use assessment methods have been developed to measure water use by different commodities, communities and countries (Chapagain and Tickner 2012; Boulay et al. 2021). Calculating the water footprint is complex and varies with region, production system, and the type of water used (e.g. blue, green, grey or virtual; see Box 2). Estimates of the water footprint for animal production includes the water consumed by the animal, water used to grow crops for feed, and water required for meat (product) processing. The major component of water use for animal sourced foods is for crop production, especially irrigation (Ibidhi and Ben Salem 2020). In Australia, where most animal feed, both grains and forages, is produced by dry-land farming, water use is minimal compared with production systems in Europe and the USA, where there is much greater reliance on irrigation. Water-use comparisons of the same commodity produced in different production systems can be made only when there is complete transparency of the values used and their derivation. Recently, there has been a conscious effort internationally to build a consensus on water-use methodology for livestock production systems (Boulay et al. 2021).
Some systems of accounting for water use include rainfall and the rainfall used to produce crops such as grain. Depending on the method used to calculate water use to produce 1 kg beef, estimates range from 3 to 540 L of water by using the life-cycle approach to 10 000–200 000 L of water by using a water-footprint approach (which includes rainfall; Doreau et al. 2012). Moreover, when a detailed assessment was conducted over six different beef growing regions of NSW, very different water footprints were obtained by Ridoutt et al. (2012), demonstrating the importance of local geography and confirming earlier Australian results (Peters et al. 2010). A similar result was also obtained when beef cattle production was compared among different regions of South Africa (Harding et al. 2017). It has also been established that as animal productivity and management improve, water-use efficiency of cattle increases (Klopatek and Oltjen 2022). Chicken meat requires the lowest amount water per kilogram produced, reflecting the efficiency of broilers turning feed into meat (Copley and Wiedemann 2023). Regardless, water use is always higher in the production of animal products than of crop products when expressed per kilogram of product. Nevertheless, when examining water data, beware of generalisations when comparing countries and products, which can be demonstrably misleading.
Across large areas of inland Australia, water is supplied to livestock by artesian bores. There has been considerable waste of water from these free-flowing bores, but recent capping of many of these bores has significantly reduced water losses (Wiedemann et al. 2015). Importantly, the extensive livestock systems in Australia generate products by using rainfall on land that is not suitable for other purposes (Doreau et al. 2012); hence, this valuable water resource is captured and not lost. Should this livestock system be penalised for using rainfall in this instance? Water is a precious resource and the interaction between livestock and water needs should be considered for each production system.
Importance of livestock and meat to Australian society
Livestock make a significant contribution to the Australian economy, employment and farming-system sustainability. The red meat and livestock turnover in 2020–2021 totalled A$67.7 billion (Meat & Livestock Australia 2022). The value of meat exported fluctuates, but is ~A$20–25 billion/year. Meat and livestock (including cattle, sheep, wool, milk, poultry, pigs and other livestock products) represent more than 42% of the value of Australian agricultural production and are the most dominant and growing components of the agricultural economy. Crops account for approximately 34% of the value of agricultural production, indicating that a narrative of diminished importance of livestock production to Australia is misleading. The value for meat and livestock has increased by almost 40% in the past 20 years. The growth in livestock prices reflects, in part, increased demand for protein in developing countries (Australian Bureau of Agricultural and Resource Economics and Sciences 2022a).
The red meat and livestock sector directly employed 191 700 people in 2020–2021, with another 230 000 being indirectly employed by industries servicing the sector (Meat & Livestock Australia 2022). Without livestock, many of the towns and communities, particularly those away from the coast, would cease to be viable; hence, the productive use of forest and rangelands is important to the economy and distribution of population within rural areas. The dairy and non-ruminant meat and egg sectors contribute significantly to the Australian economy, employment and many rural communities, especially on the southern half of the eastern seaboard. The pig industry contributes 36 000 jobs (APL 2020) compared with 4000, 58 000, and 46 000 for the egg (AEL 2022), chicken meat (ACMF 2022), and dairy (Dairy Australia 2022) industries respectively. In the same order, the industries contributed A$5.3, A$0.93, A$7.9 and A$4.7 billion to the Australian economy annually. The dairy industry also exports some 35% of production mainly as value-added products worth about A$3.3 billion (Dairy Australia 2022). All of the dollar figures cited for the different animal industries are farm-gate figures that do not capture the full economic impact. It has been estimated that every dollar of value added in processing of animal-sourced food products supports about A$3.0 of value-added elsewhere in the economy (Deloitte and Access Economics 2021).
Australian animal industries have a social and ethical obligation to deliver demonstrable animal welfare improvements as has been clearly demonstrated (Hemsworth 2018; Salvin et al. 2020; Rivero and Lee 2022; Verdon 2022; Masters et al. 2023). Animal welfare is a key priority for government, industry and research (Barnett and Hemsworth 2009; Hemsworth et al. 2015; Colditz and Hine 2016; Kahn et al. 2017; Tilbrook and Ralph 2017; Colditz 2022). The industries endorse and abide by the relevant Australian Codes of Practice for the Welfare of Animals, and relevant State animal welfare legislation that cover production, transport and slaughter. Nevertheless, Australian producers in all animal industries are under a degree of pressure from animal welfare lobby groups and the public (Bray et al. 2017; Coleman 2018).
Australia is a dry and hot continent and alleviation of heat stress is a welfare issue, as is the long-distance transport of livestock. Mitigating the risk of heat stress in a feedlot is a welfare priority (Johnson 2018). By 2014, community pressure on the feedlot industry and production benefits led to ~80% of feedlots providing shade (Gaughan and Sullivan 2014). In the pig and poultry industries, community pressure associated with confinement of animals has been intense and this has resulted in significant changes. A decade ago only 10% of table eggs were produced free-range, with the remainder being produced by hens housed in cages. Half the table eggs produced in Australia now come from free-range production systems. Although this gives the impression of improved bird welfare, there are concerns regarding choke following pasture consumption and increased exposure to parasites (Bryden et al. 2021; Campbell et al. 2021). It should be remembered that broilers or meat chickens are predominantly housed in large barns and have never been grown in cages. Moreover, some 70% of broilers are farmed under an RSPCA-approved system, including 20% as free-range. The pig industry discontinued the use of dry sow stalls in 2017 and moved to group housing for pregnant sows. The pig industry would like to move away from farrowing crates that prevent piglets being crushed by the sow, but despite ongoing research, no satisfactory alternative has been identified that accounts for the conflicting needs of the sow and her litter (Barnett et al. 2001; Johnson and Marchant-Forde 2009).
There is a general acceptance that improving welfare also improves animal productivity through improved health and reduced medication and mortality (Dawkins 2017), improving the public image of the industries. Moreover, the cost of not maintaining a social licence to operate could be substantial, as it has been estimated that during the period 2015–2030, the potential accumulated loss could amount to A$3.9 billion for the Australian red meat industries (Fernandes et al. 2021). Good stockmanship is a cost-effective way of improving productivity and product quality (Barnett and Hemsworth 2009; Hemsworth 2018). It is an ongoing imperative that all livestock industry personnel are appropriately trained in animal husbandry and stockmanship to ensure animal welfare (Grandin 2018).
The path ahead
It is clear that the societal role of meat is being challenged with ideological and simplified logic, without substantiation from robust data-driven science. The Dublin Declaration is a first step to assemble an evidence-based narrative to underpin the essential role of meat in diet and health, a sustainable environment and society, economics and culture. Meat production, especially from ruminants, is a critical part of Australian agriculture, allowing both food production from non-arable lands plus making huge contributions to the circular economy of all agricultural pursuits. Accordingly, the Dublin Declaration is highly relevant to Australia.
Science alone is not perfect and can have suboptimal outcomes when undertaken within a siloed structure, especially when the biology and issues underpinning the augments are complex. In addition, effective communication is an essential ingredient when presenting the evidence and also for projecting the meat industry as a dynamic supply chain to attract young scientists into these career paths. A report by Polkinghorne et al. (2022) summarised an extensive global survey of meat scientists and industry practitioners and concluded that these broader concerns were deemed of at least equal importance to the traditional core disciplines of meat science and livestock biology.
Given the importance of meat in society, there is a need to urgently examine the best way forward for underpinning scientific funding, industry translation and collaboration in the area. Polkinghorne et al. (2023b) argued that in many countries, resources and staff associated with meat production have declined to critically low levels, threatening both fundamental research and industry support. In Australia, we are increasingly left with pockets of strength in some universities as State and Federal government funded institutions with expertise in meat are in decline. Scientists within the University system are also faced with challenges associated with reduced funding in the face of pressure to train more students, increased administrative load and a lack of base capital to maintain facilities to world standards. Clearly, the way forward is for even more emphasis to support strong multidisciplinary collaboration that will need significant and sustained Industry participation and support.
More recent examples in Australia of interdisciplinary research to tackle industry priorities are the Beef, Sheep, Pork and Poultry Cooperative Research Centres, which have delivered immense benefits to the beef, sheep, pork and poultry sectors of Australia (Keniry 2011; Griffith and Burrow 2015; Rowe et al. 2021). Substantial financial benefits have been quantified in the areas of animal management and welfare, genetic improvement, disease control and meat quality and yield. Importantly, the Cooperative Research Centres also come with a substantive education program, particularly in delivering industry-aligned postgraduate students that have been retained in the sector (Thompson and Rowe 2019). Another example is the Advanced Livestock Measurement technologies project for developing objective measurement of carcase meat yield and eating quality across the beef, lamb and pork industries (Gardner et al. 2021). There have also been efforts to upgrade the wastes of the red meat industry to valuable co-products funded by the Federal government (Ramirez et al. 2021). The benefits have been underpinned by the longevity of the programs (5–15 years), powerful heads of agreement that simplify sharing of intellectual property across institutions and finally embed intense engagement between industry and researchers. These programs have now finished and there is an urgent need for new and even bolder initiatives.
New developments need to bolster existing strengths in meat science and, importantly, expand collaboration to embrace the key themes of the Dublin Declaration. Thus, expertise in meat science, human nutrition, animal production, health and welfare, life-cycle environmental assessment, economics, communication and undergraduate/postgraduate education all need to be blended together in conjunction with industry (Mayberry et al. 2021). There is also the responsibility for scientists who are connected to the societal role of meat to connect outside their core disciplines. One example is the Nutrition Society of Australia, which brings together the disciplines of human and animal nutrition in yearly scientific meetings; unfortunately, the participation by animal science specialists has been dramatically declining in recent years. The final piece of the jig saw is to include mechanisms that strongly encourage international collaboration, which traditionally has been problematic in agriculture outside of programs that support developing countries. Thus, other countries outside one’s own are typically seen as competitors rather than collaborators. The International Meat Research 3G Foundation, a not-for-profit international eating-quality research platform linked to the United Nations Economic Commission, is an example of a mechanism to underpin more collaboration across participating countries (Polkinghorne et al. 2023b). Clearly, the challenges underpinning the Dublin Declaration require urgent global collaboration and solutions.
Finally, there is an ongoing need to challenge false or inadequately substantiated information and to correctly contextualise the societal role of meat, so that the gains to different societies can be realised. There is a need to educate society through rational debate.
Data availability
Data sharing is not applicable as no new data were generated or analysed during this study.
Conflicts of interest
All authors have an active research record in the livestock and/or the meat sectors. WLB and DGM have editorial roles with Animal Production Science. They were both blinded from the peer review process for this paper. The authors have no further conflicts of interest to declare.
References
ACMF (2022) Australian chicken meat federation: facts and figures. Available at https://www.chicken.org.au [Accessed 20 January 2023]
Adesogan AT, Havelaar AH, McKune SL, Eilittä M, Dahl GE (2020) Animal source foods: sustainability problem or malnutrition and sustainability solution? Perspective matters. Global Food Security 25, 100325.
| Crossref | Google Scholar |
AEL (2022) Australian eggs annual report 2022. Available at https://www.australianeggs.org.au. [Accessed 20 January 2023]
Aiking H (2014) Protein production: planet, profit, plus people? The American Journal of Clinical Nutrition 100(Suppl 1), 483S-489S.
| Crossref | Google Scholar |
Alders RG, Pym RAE (2009) Village poultry: still important to millions, eight thousand years after domestication. World’s Poultry Science Journal 65, 181-190.
| Crossref | Google Scholar |
Anonymous (2021) Dairy farm monitor project 2020. (Victorian Department of Primary Industry). Available at https://agriculture.vic.gov.au/about/agriculture-in-victoria/dairy-farm-monitor-project [Accessed 10 May 2023]
Arroyo-Manzanares N, Hamed AM, García-Campaña AM, Gámiz-Gracia L (2019) Plant-based milks: unexplored source of emerging mycotoxins. A proposal for the control of enniatins and beauvericin using UHPLC-MS/MS. Food Additives & Contaminants: Part B 12, 296-302.
| Crossref | Google Scholar |
Atkinson R (2022) Lot feeding brief. Results for the September quarter 2022 feedlot survey. Available at https://www.mla.com.au/globalassets/mla-corporate/prices--markets/documents/trends--analysis/lot-feeding-brief/mla_lot-feeding-brief_nov-2022_111122.pdf [Accessed 11 January 2023]
Australian Bureau of Agricultural and Resource Economics and Sciences (2022a) Snapshot of Australian Agriculture 2022. Available at https://www.agriculture.gov.au/abares/products/insights/snapshot-of-australian-agriculture-2022 [Accessed 1 January 2023]
Australian Bureau of Agricultural and Resource Economics and Sciences (2022b) Agricultural commodities and trade data. Available at https://www.agriculture.gov.au/abares/research-topics/agricultural-outlook/data#agricultural-commodities [Accessed 11 January 2023]
Australian Bureau of Statistics (2016–2017) Land management and farming in Australia. Available at https://www.abs.gov.au/statistics/industry/agriculture/land-management-and-farming-australia/2016-17#cite-window1
Baghurst K (1999) Red meat consumption in Australia: intakes, contributions to nutrient intake and associated dietary patterns. European Journal of Cancer Prevention 8, 185-191.
| Crossref | Google Scholar |
Balehegn M, Mekuriaw Z, Miller L, Mckune S, Adesogan AT (2019) Animal-sourced foods for improved cognitive development. Animal Frontiers 9, 50-57.
| Crossref | Google Scholar |
Balehegn M, Andrade Laborde JE, McKune SL, Adesogan AT (2022) The importance of meat for cognitive development. Meat and Muscle Biology 5, 1-20.
| Crossref | Google Scholar |
Baltenweck I, Enahoro D, Frija A, Tarawali S (2020) Why is production of animal source foods important for economic development in Africa and Asia? Animal Frontiers 10, 22-29.
| Crossref | Google Scholar |
Barnett JL, Hemsworth PH (2009) Welfare monitoring schemes: using research to safeguard welfare of animals on the farm. Journal of Applied Animal Welfare Science 12, 114-131.
| Crossref | Google Scholar |
Barnett JL, Hemsworth PH, Cronin GM, Jongman EC, Hutson GD (2001) A review of the welfare issues for sows and piglets in relation to housing. Australian Journal of Agricultural Research 52, 1-28.
| Crossref | Google Scholar |
BBC News (2022) Climate change: New Zealand’s plan to tax cow and sheep burps. Available at https://www.bbc.com/news/business-61741352. [Accessed 20 April 2023]
Bell A, Sangster N (2023) Research, development and adoption for the north Australian beef cattle breeding industry: an analysis of needs and gaps. Animal Production Science 63, 1-40.
| Crossref | Google Scholar |
Bell LW, Hargreaves JNG, Lawes RA, Robertson MJ (2009) Sacrificial grazing of wheat crops: identifying tactics and opportunities in Western Australia’s grainbelt using simulation approaches. Animal Production Science 49, 797-806.
| Crossref | Google Scholar |
Bell AW, Charmley E, Hunter RA, Archer JA (2011a) The Australasian beef industries – challenges and opportunities in the 21st century. Animal Frontiers 1, 10-19.
| Crossref | Google Scholar |
Bell LW, Kirkegaard JA, Swan A, Hunt JR, Huth NI, Fettell NA (2011b) Impacts of soil damage by grazing livestock on crop productivity. Soil and Tillage Research 113, 19-29.
| Crossref | Google Scholar |
Bell LW, Moore AD, Kirkegaard JA (2014) Evolution in crop–livestock integration systems that improve farm productivity and environmental performance in Australia. European Journal of Agronomy 57, 10-20.
| Crossref | Google Scholar |
Bell LW, Moore AD, Thomas DT (2021) Diversified crop–livestock farms are risk-efficient in the face of price and production variability. Agricultural Systems 189, 103050.
| Crossref | Google Scholar |
Black JL, Tredrea AM, Bird SH, Hughes RJ, Nielsen SG (2023) Effects of germination on the energy value of cereal grains for livestock. Animal Production Science 63, 256-268 (in press).
| Crossref | Google Scholar |
Blaxter KL, Webster AJF (1991) Animal production and food: real problems and paranoia. Animal Science 53, 261-269.
| Crossref | Google Scholar |
Bonny SPF, Gardner GE, Pethick DW, Hocquette J-F (2017) Artificial meat and the future of the meat industry. Animal Production Science 57, 2216-2223.
| Crossref | Google Scholar |
Bothwell TH, Charlton RW (1982) A general approach to the problems of iron deficiency and iron overload in the population at large. Seminars in Haematology 19, 54-67.
| Google Scholar |
Boukid F (2021) Plant-based meat analogues: from niche to mainstream. European Food Research and Technology 247, 297-308.
| Crossref | Google Scholar |
Boulay A-M, Drastig K, Amanullah , Chapagain A, Charlon V, Civit B, DeCamillis C, De Souza M, Hess T, Hoekstra AY, Ibidhi R, Lathuillière MJ, Manzardo A, McAllister T, Morales RA, Motoshita M, Palhares JCP, Pirlo G, Ridoutt B, Russo V, Salmoral G, Singh R, Vanham D, Wiedemann S, Zheng W, Pfister S (2021) Building consensus on water use assessment of livestock production systems and supply chains: outcome and recommendations from the FAO LEAP Partnership. Ecological Indicators 124, 107391.
| Crossref | Google Scholar |
Bray HJ, Buddle EA, Ankeny RA (2017) What are they thinking? Consumer attitudes to meat production in Australia. Animal Production Science 57, 2345-2352.
| Crossref | Google Scholar |
Bryden WL, Li X, Ruhnke I, Zhang D, Shini S (2021) Nutrition, feeding and laying hen welfare. Animal Production Science 61, 893-914.
| Crossref | Google Scholar |
Campbell DLM, Bari MS, Rault J-L (2021) Free-range egg production: its implications for hen welfare. Animal Production Science 61, 848-855.
| Crossref | Google Scholar |
Chan KY, Heenan DP (2005) The effects of stubble burning and tillage on soil carbon sequestration and crop productivity in southeastern Australia. Soil Use and Management 21, 427-431.
| Crossref | Google Scholar |
Chan KY, Heenan DP, So HB (2003) Sequestration of carbon and changes in soil quality under conservation tillage on light-textured soils in Australia: a review. Australian Journal of Experimental Agriculture 43, 325-334.
| Crossref | Google Scholar |
Chapagain AK, Tickner D (2012) Water footprint: help or hindrance? Water Alternatives 5, 563-581.
| Google Scholar |
Charmley E, Thomas D, Bishop-Hurley GJ (2023) Revisiting tropical pasture intake: what has changed in 50 years? Animal Production Science
| Crossref | Google Scholar |
Chinique de Armas Y, Pestle W (2018) Assessing the association between subsistence strategies and the timing of weaning among indigenous archaeological populations of the Caribbean. International Journal of Osteoarchaeology 28, 492-509.
| Crossref | Google Scholar |
Colditz IG (2022) Competence to thrive: resilience as an indicator of positive health and positive welfare in animals. Animal Production Science 62, 1439-1458.
| Crossref | Google Scholar |
Colditz IG, Hine BC (2016) Resilience in farm animals: biology, management, breeding and implications for animal welfare. Animal Production Science 56, 1961-1983.
| Crossref | Google Scholar |
Coleman G (2018) Public animal welfare discussions and outlooks in Australia. Animal Frontiers 8, 14-19.
| Crossref | Google Scholar |
Comerford KB, Miller GD, Reinhardt Kapsak W, Brown KA (2021) The complementary roles for plant-source and animal-source foods in sustainable healthy diets. Nutrients 13, 3469.
| Crossref | Google Scholar |
Copley MA, Wiedemann SG (2023) Environmental impacts of the Australian poultry industry. 1. Chicken meat production. Animal Production Science 63, 489-504.
| Crossref | Google Scholar |
Copley MA, Wiedemann SG, McGahan EJ (2023) Environmental impacts of the Australian poultry industry. 2. egg production. Animal Production Science 63, 505-521 (in press).
| Crossref | Google Scholar |
Cordain L, Miller JB, Eaton SB, Mann N, Holt SHA, Speth JD (2000) Plant-animal subsistence ratios and macronutrient energy estimations in worldwide hunter-gatherer diets. The American Journal of Clinical Nutrition 71, 682-692.
| Crossref | Google Scholar |
Costa ND (2007) Reducing the meat and livestock industry’s environmental footprint. Nutrition & Dietetics 64, S185-S191.
| Crossref | Google Scholar |
Croney C, Swanson J (2023) Is meat eating morally defensible? Contemporary ethical considerations. Animal Frontiers 13, 61-67.
| Crossref | Google Scholar |
Dairy Australia (2022) Dairy Australia; The Australian dairy industry, in focus 2022. Available at https://www.dairyaustralia.com.au [Accessed 25 January 2023]
Davis SL (2003) The least harm principle may require that humans consume a diet containing large herbivores, not a vegan diet. Journal of Agricultural and Environmental Ethics 16, 387-394.
| Crossref | Google Scholar |
Davison TM, Black JL, Moss JF (2020) Red meat: an essential partner to reduce global greenhouse gas emissions. Animal Frontiers 10, 14-21.
| Crossref | Google Scholar |
Dawkins MS (2017) Animal welfare and efficient farming: is conflict inevitable? Animal Production Science 57, 201-208.
| Crossref | Google Scholar |
de Bruyn J, Bagnol B, Chan HH, Grace D, Mitchell MEV, Nunn MJ, Wingett K, Wong JT, Alders RG (2020) The role of animal-source foods in sustainable, ethical, and optimal human diets. In ‘Science, technology, and innovation for sustainable development goals: insights from agriculture, health, environment, and energy’. (Eds AA Adenle, MR Chertow, EHM Moors, DJ Pannell) pp. 344–364. (Oxford University Press: UK)
de Souza-Vilela J, Andrew NR, Ruhnke I (2019) Insect protein in animal nutrition. Animal Production Science 59, 2029-2036.
| Crossref | Google Scholar |
DiGiacomo K, Akit H, Leury BJ (2019) Insects: a novel animal-feed protein source for the Australian market. Animal Production Science 59, 2037-2045.
| Crossref | Google Scholar |
Dixon RM, Kidd LJ, Coates DB, Anderson ST, Benvenutti MA, Fletcher MT, McNeill DM (2017) Utilising mobilisation of body reserves to improve the management of phosphorus nutrition of breeder cows. Animal Production Science 57, 2280-2290.
| Crossref | Google Scholar |
Doreau M, Corson MS, Wiedemann SG (2012) Water use by livestock: a global perspective for a regional issue? Animal Frontiers 2, 9-16.
| Crossref | Google Scholar |
Eckard RJ, Clark H (2020) Potential solutions to the major greenhouse-gas issues facing Australasian dairy farming. Animal Production Science 60, 10-16.
| Crossref | Google Scholar |
Ederer P, Baltenweck I, Blignaut JN, Moretti C, Tarawali S (2023) Affordability of meat for global consumers and the need to sustain investment capacity for livestock farmers. Animal Frontiers 13, 45-60.
| Crossref | Google Scholar |
Ellies-Oury M-P, Chriki S, Hocquette J-F (2022) Should and will ‘cultured meat’ become a reality in our plates? Advances in Food and Nutrition Research 101, 181-212.
| Crossref | Google Scholar |
Emken RA, Adlof RO, Rohwedder WK, Gulley RM (1992) Comparison of linolenic and linoleic acid metabolism in man: influence of dietary linoleic acid. In ‘Essential fatty acids and eicosanoids. Invite papers from the third international congress’. (Eds A Sinclair, R Gibson) pp. 23–35. (American Oil Chemists’ Society)
Falchetti A, Cavati G, Valenti R, Mingiano C, Cosso R, Gennari L, Chiodini I, Merlotti D (2022) The effects of vegetarian diets on bone health: a literature review. Frontiers in Endocrinology 13, 899375.
| Crossref | Google Scholar |
Fernandes JN, Hemsworth PH, Coleman GJ, Tilbrook AJ (2021) Costs and benefits of improving farm animal welfare. Agriculture 11, 104.
| Crossref | Google Scholar |
Gardner GE, Apps R, McColl R, Craigie CR (2021) Objective measurement technologies for transforming the Australian & New Zealand livestock Industries. Meat Science 179, 108556.
| Crossref | Google Scholar |
GOAL Sciences (2023) Planet food system explorer. Available at https://goalsciences.org/planet-food-system-explorer [Accessed 20 October 2022]
Grandin T (2018) Livestock-handling assessments to improve the welfare of cattle, pigs and sheep. Animal Production Science 58, 403-407.
| Crossref | Google Scholar |
Griffith GR, Burrow HM (2015) The value of research: using the impact tool to evaluate realised and anticipated benefits of the cooperative research centre for beef genetic technologies. Animal Production Science 55, 133-144.
| Crossref | Google Scholar |
Harding G, Courtney C, Russo V (2017) When geography matters. A location-adjusted blue water footprint of commercial beef in South Africa. Journal of Cleaner Production 151, 494-508.
| Crossref | Google Scholar |
Harrison MT, Evans JR, Dove H, Moore AD (2011) Dual-purpose cereals: can the relative influences of management and environment on crop recovery and grain yield be dissected? Crop & Pasture Science 62, 930-946.
| Crossref | Google Scholar |
Hemsworth PH (2018) Key determinants of pig welfare: implications of animal management and housing design on livestock welfare. Animal Production Science 58, 1375-1386.
| Crossref | Google Scholar |
Hemsworth PH, Mellor DJ, Cronin GM, Tilbrook AJ (2015) Scientific assessment of animal welfare. New Zealand Veterinary Journal 63, 24-30.
| Crossref | Google Scholar |
Henneberg M, Sarafis V, Mathers K (1998) Human adaptations to meat eating. Human Evolution 13, 229-234.
| Crossref | Google Scholar |
Howieson JG, O’Hara GW, Carr SJ (2000) Changing roles for legumes in Mediterranean agriculture: developments from an Australian perspective. Field Crops Research 65, 107-122.
| Crossref | Google Scholar |
Hristov AN (2012) Historic, pre-European settlement, and present-day contribution of wild ruminants to enteric methane emissions in the United States. Journal of Animal Science 90, 1371-1375.
| Crossref | Google Scholar |
Hunter RA (2010) Hormonal growth promotant use in the Australian beef industry. Animal Production Science 50, 637-659.
| Crossref | Google Scholar |
Ibidhi R, Ben Salem H (2020) Water footprint of livestock products and production systems: a review. Animal Production Science 60, 1369-1380.
| Crossref | Google Scholar |
Jain R, Larsuphrom P, Degremont A, Latunde-Dada GO, Philippou E (2022) Association between vegetarian and vegan diets and depression: a systematic review. Nutrition Bulletin 47, 27-49.
| Crossref | Google Scholar |
Johnson JS (2018) Heat stress: impact on livestock well-being and productivity and mitigation strategies to alleviate the negative effects. Animal Production Science 58, 1404-1413.
| Crossref | Google Scholar |
Johnston B, De Smet S, Leroy F, Mente A, Stanton A (2023) Non-communicable disease risk associated with red and processed meat consumption – magnitude, certainty, and contextuality of risk? Animal Frontiers 13, 19-27.
| Crossref | Google Scholar |
Kahn LP, Johnson IR, Rowe JB, Hogan L, Boshoff J (2017) ASKBILL as a web-based program to enhance sheep well-being and productivity. Animal Production Science 57, 2257-2262.
| Crossref | Google Scholar |
Kebreab E, Liedke A, Caro D, Deimling S, Binder M, Finkbeiner M (2016) Environmental impact of using specialty feed ingredients in swine and poultry production: a life cycle assessment. Journal of Animal Science 94, 2664-2681.
| Crossref | Google Scholar |
Keniry AMJS (2011) Was the cooperative centre (CRC) for an internationally competitive Australian pork industry worth the investment? Manipulating pig production XIII. In ‘Proceedings of the thirteenth biennial conference of the Australasian Pig Science Association’. (Ed. R van Barneveld) pp. 3–8. (Australian Pig Science Association Inc.)
Kingwell R, Islam N, Xayavong V (2020) Farming systems and their business strategies in south-western Australia: a decadal assessment of their profitability. Agricultural Systems 181, 102827.
| Crossref | Google Scholar |
Kirkegaard JA (1995) A review of trends in wheat yield responses to conservation cropping in Australia. Australian Journal of Experimental Agriculture 35, 835-848.
| Crossref | Google Scholar |
Klopatek SC, Oltjen JW (2022) How advances in animal efficiency and management have affected beef cattle’s water intensity in the United States: 1991 compared to 2019. Journal of Animal Science 100, 1-13.
| Crossref | Google Scholar |
Lam SK, Chen D, Mosier AR, Roush R (2013) The potential for carbon sequestration in Australian agricultural soils is technically and economically limited. Scientific Reports 3, 2179.
| Crossref | Google Scholar |
Lean IJ, Moate PJ (2021) Cattle, climate and complexity: food security, quality and sustainability of the Australian cattle industries. Australian Veterinary Journal 99, 293-308.
| Crossref | Google Scholar |
Leblanc J-C, Tard A, Volatier J-L, Verger P (2005) Estimated dietary exposure to principal food mycotoxins from the first French total diet study. Food Additives and Contaminants 22, 652-672.
| Crossref | Google Scholar |
Lee MF, Eather R, Best T (2021) Plant-based dietary quality and depressive symptoms in Australian vegans and vegetarians: a cross-sectional study. BMJ Nutrition, Prevention & Health 4, e000332.
| Crossref | Google Scholar |
Leroy F, Abraini F, Beal T, Dominguez-Salas P, Gregorini P, Manzano P, Rowntree J, van Vliet S (2022) Animal board invited review: animal source foods in healthy, sustainable, and ethical diets – An argument against drastic limitation of livestock in the food system. Animal 16, 100457.
| Crossref | Google Scholar |
Leroy F, Smith NW, Adesogan AT, Beal T, Iannotti L, Moughan PJ, Mann N (2023) The role of meat in the human diet: evolutionary aspects and nutritional value. Animal Frontiers 13, 11-18.
| Crossref | Google Scholar |
Liu S, Proudman J, Mitloehner FM (2021) Rethinking methane from animal agriculture. CABI Agriculture and Bioscience 2, 22.
| Crossref | Google Scholar |
Ma X, Tan H, Hu M, He S, Zou L, Pan H (2021) The impact of plant-based diets on female bone mineral density: evidence based on seventeen studies. Medicine 100, e27480.
| Crossref | Google Scholar |
Mann N (2000) Dietary lean red meat and human evolution. European Journal of Nutrition 39, 71-79.
| Crossref | Google Scholar |
Mann NJ (2018) A brief history of meat in the human diet and current health implications. Meat Science 144, 169-179.
| Crossref | Google Scholar |
Manzano P, Burgas D, Cadahía L, Eronen JT, Fernández-Llamazares Á, Bencherif S, Holand Ø, Seitsonen O, Byambaa B, Fortelius M, Fernández-Giménez ME, Galvin KA, Cabeza M, Stenseth NC (2021) Toward a holistic understanding of pastoralism. One Earth 4, 651-665.
| Crossref | Google Scholar |
Manzano P, Rowntree J, Thompson L, del Prado A, Ederer P, Windisch W, Lee MRF (2023) Challenges for the balanced attribution of livestock’s environmental impacts: the art of conveying simple messages around complex realities. Animal Frontiers 13, 35-44.
| Crossref | Google Scholar |
Marinangeli CPF, House JD (2017) Potential impact of the digestible indispensable amino acid score as a measure of protein quality on dietary regulations and health. Nutrition Reviews 75, 658-667.
| Crossref | Google Scholar |
Masters DG, Thompson AN (2016) Grazing crops: implications for reproducing sheep. Animal Production Science 56, 655-668.
| Crossref | Google Scholar |
Masters D, Edwards N, Sillence M, Avery A, Revell D, Friend M, Sanford P, Saul G, Beverly C, Young J (2006) The role of livestock in the management of dryland salinity. Australian Journal of Experimental Agriculture 46, 733-741.
| Crossref | Google Scholar |
Masters DG, Blache D, Lockwood AL, Maloney SK, Norman HC, Refshauge G, Hancock SN (2023) Shelter and shade for grazing sheep: implications for animal welfare and production and for landscape health. Animal Production Science 63, 623-644.
| Crossref | Google Scholar |
Mayberry D, Hatcher S, Cowley F (2021) New skills, networks and challenges: the changing face of animal production science in Australia. Animal Production Science 61, 201-207.
| Crossref | Google Scholar |
McKendree MGS, Croney CC, Widmar NJO (2014) Effects of demographic factors and information sources on United States consumer perceptions of animal welfare. Journal of Animal Science 92, 3161-3173.
| Crossref | Google Scholar |
Meat & Livestock Australia (2021) Delivering CN30. Available at https://www.mla.com.au/research-and-development/Environment-sustainability/carbon-neutral-2030-rd/delivering-cn30/ [Accessed 11 January 2023]
Mihalache OA, Dellafiora L, Dall’Asta C (2022a) A systematic review of natural toxins occurrence in plant commodities used for plant-based meat alternatives production. Food Research International 158, 111490.
| Crossref | Google Scholar |
Mihalache OA, Dellafiora L, Dall’Asta C (2022b) Assessing the mycotoxin-related health impact of shifting from meat-based diets to soy-based meat analogues in a model scenario based on italian consumption data. Exposure and Health
| Crossref | Google Scholar |
Miller V, Webb P, Cudhea F, et al. (2022) Global dietary quality in 185 countries from 1990 to 2018 show wide differences by nation, age, education, and urbanicity. Nature Food 3, 694-702.
| Crossref | Google Scholar |
Moher D, Liberati A, Tetzlaff J, Altman DG, The PRISMA Group (2009) Preferred reporting items for systematic reviews and meta-analyses: the PRISMA statement. PLoS Medicine 6(7), e1000097.
| Crossref | Google Scholar |
Mottet A, de Haan C, Falcucci A, Tempio G, Opio C, Gerber P (2017) Livestock: on our plates or eating at our table? A new analysis of the feed/food debate. Global Food Security 14, 1-8.
| Crossref | Google Scholar |
Moughan PJ (2021) Population protein intakes and food sustainability indices: the metrics matter. Global Food Security 29, 100548.
| Crossref | Google Scholar |
Murray CJL, Aravkin AY, Zheng P, et al. (2020) Global burden of 87 risk factors in 204 countries and territories, 1990–2019: a systematic analysis for the Global Burden of Disease Study 2019. The Lancet 396, 1223-1249.
| Crossref | Google Scholar |
Noakes M, Clifton P (2008) Health and environmental benefits of the CSIRO total wellbeing diet. Nutrition & Diatetics 65, 232-233.
| Crossref | Google Scholar |
Noakes M, Keogh JB, Foster PR, Clifton PM (2005) Effect of an energy-restricted, high-protein, low-fat diet relative to a conventional high-carbohydrate, low-fat diet on weight loss, body composition, nutritional status, and markers of cardiovascular health in obese women. The American Journal of Clinical Nutrition 81, 1298-1306.
| Crossref | Google Scholar |
Nutrition and Dietetics (2007) The role of red meat in healthy Australian diets. Nutrition and Dietetics 64, s99-s195.
| Google Scholar |
Oreskes N (2022) Eat to save the planet. Scientific American 326(1), 78.
| Google Scholar |
Owens F, Hansen C (2011) Competition between animals and humans for cultivated crops: livestock production and our food supply. In ‘Animal welfare in animal agriculture: husbandry, stewardship, and sustainability in animal production’. (Eds WG Pond, FW Bazer, BE Rollin) pp. 241–262. (CRC Press: Boca Raton, FL, USA)
Penczynski KJ, Cramer B, Dietrich S, Humpf H-U, Abraham K, Weikert C (2022) Mycotoxins in serum and 24-h Urine of vegans and omnivores from the risks and benefits of a vegan diet (RBVD) Study. Molecular Nutrition & Food Research 66, 2100874.
| Crossref | Google Scholar |
Peters GM, Wiedemann SG, Rowley HV, Tucker RW (2010) Accounting for water use in Australian red meat production. The International Journal of Life Cycle Assessment 15, 311-320.
| Crossref | Google Scholar |
Place SE, McCabe CJ, Mitloehner FM (2022) Symposium review: defining a pathway to climate neutrality for US dairy cattle production. Journal of Dairy Science 105, 8558-8568.
| Crossref | Google Scholar |
Polkinghorne R, Philpott J, Cuthbertson H, Wilcock E (2022) Meat science toward 2030: an international forum for the development of strategic objectives. Available at https://www.ampc.com.au/getmedia/c7197619-ac4e-4b6a-9f06-a129d81af91d/220221-FINAL_REPORT_2017_1144_Meat-Science-Toward-2030.pdf?ext=.pdf
Polkinghorne R, Koohmaraie M, Kaster C, Troy D, Rosati A (2023b) Challenges and opportunities for defining the role and value of meat for our global society and economy. Animal Frontiers 13, 75-81.
| Crossref | Google Scholar |
Poppi DP, McLennan SR (1995) Protein and energy utilization by ruminants at pasture. Journal of Animal Science 73, 278-290.
| Crossref | Google Scholar |
Ramirez J, McCabe B, Jensen PD, Speight R, Harrison M, van den Berg L, O’Hara I (2021) Wastes to profit: a circular economy approach to value-addition in livestock industries. Animal Production Science 61, 541-550.
| Crossref | Google Scholar |
Randolph TF, Schelling E, Grace D, Nicholson CF, Leroy JL, Cole DC, Demment MW, Omore A, Zinsstag J, Ruel M (2007) Invited Review: role of livestock in human nutrition and health for poverty reduction in developing countries. Journal of Animal Science 85, 2788-2800.
| Crossref | Google Scholar |
Ranga Niroshan Appuhamy JAD, Strathe AB, Jayasundara S, Wagner-Riddle C, Dijkstra J, France J, Kebreab E (2013) Anti-methanogenic effects of monensin in dairy and beef cattle: a meta-analysis. Journal of Dairy Science 96, 5161-5173.
| Crossref | Google Scholar |
Ranum P, Peña-Rosas JP, Garcia-Casal MN (2014) Global maize production, utilization, and consumption. Annals of the New York Academy of Sciences 1312, 105-112.
| Crossref | Google Scholar |
Ravindran V (2013) Feed enzymes: the science, practice, and metabolic realities. Journal of Applied Poultry Research 22, 628-636.
| Crossref | Google Scholar |
Ridoutt B (2021) Climate neutral livestock production – a radiative forcing-based climate footprint approach. Journal of Cleaner Production 291, 125260.
| Crossref | Google Scholar |
Ridoutt BG, Sanguansri P, Freer M, Harper GS (2012) Water footprint of livestock: comparison of six geographically defined beef production systems. The International Journal of Life Cycle Assessment 17, 165-175.
| Crossref | Google Scholar |
Rivero MJ, Lee MRF (2022) A perspective on animal welfare of grazing ruminants and its relationship with sustainability. Animal Production Science 62, 1739-1748.
| Crossref | Google Scholar |
Rowe JB, van der Werf J, Pethick DW (2021) Keys to innovation in animal science: genomics, big data and collaboration. Animal Production Science 61, 215-219.
| Crossref | Google Scholar |
Rugoho I, Gourley CJP, Hannah MC (2017) Nutritive characteristics, mineral concentrations and dietary cation–anion difference of feeds used within grazing-based dairy farms in Australia. Animal Production Science 57, 858-876.
| Crossref | Google Scholar |
Salvin HE, Lees AM, Cafe LM, Colditz IG, Lee C (2020) Welfare of beef cattle in Australian feedlots: a review of the risks and measures. Animal Production Science 60, 1569-1590.
| Crossref | Google Scholar |
Schatz TJ, McCosker KD, Heeb C (2023) Phosphorus supplementation improves the growth and reproductive performance of female Brahman cattle grazing phosphorus-deficient pastures in the Victoria River District, Northern Territory, Australia. Animal Production Science 63, 544-559 (in press).
| Crossref | Google Scholar |
Selle PH, Ravindran V, Caldwell A, Bryden WL (2000) Phytate and phytase: consequences for protein utilisation. Nutrition Research Reviews 13, 255-278.
| Crossref | Google Scholar |
Smith NW, Fletcher AJ, Hill JP, McNabb WC (2022a) Animal and plant-sourced nutrition: complementary not competitive. Animal Production Science 62, 701-711.
| Crossref | Google Scholar |
Smith NW, Fletcher AJ, Hill JP, McNabb WC (2022b) Achieving sustainable nutrient adequacy globally and locally. Medical Sciences Forum 9, 45.
| Crossref | Google Scholar |
Stanaway J, Afshin A, Gakidou E, et al. (2018) Global, regional, and national comparative risk assessment of 84 behavioural, environmental and occupational, and metabolic risks or clusters of risks for 195 countries and territories, 1990–2017: a systematic analysis for the Global Burden of Disease Study 2017. The Lancet 392, 1923-1994.
| Crossref | Google Scholar |
Stanton AV, Leroy F, Elliott C, Mann N, Wall P, De Smet S (2022) 36-fold higher estimate of deaths attributable to red meat intake in GBD 2019: is this reliable? The Lancet 399, e23-e26.
| Crossref | Google Scholar |
Stavi I, Argaman E, Zaady E (2016) Positive impact of moderate stubble grazing on soil quality and organic carbon pool in dryland wheat agro-pastoral systems. Catena 146, 94-99.
| Crossref | Google Scholar |
Tarawali S (2022) Sustainable livestock opportunities and future food system realities. In ‘The international meat summit: the societal role of meat – what science says’, 19–20 October 2022, Dublin, Ireland. International Livestock Research Institute, Nairobi, Kenya. Available at https://hdl.handle.net/10568/125155
Teagasc (2023) The societal role of meat – what the science says. Available at https://www.teagasc.ie/food/research-and-innovation/research-areas/food-quality-and-sensory-science/meat-technology/international-meat-summit/#:~:text=Meat%20and%20livestock%20contribute%20to,is%20relevant%20in%20modern%20society [Accessed 7 February 2023]
The Guardian (2022) How can the UK reduce meat consumption and cut emissions? Available at https://www.theguardian.com/environment/2022/aug/16/how-can-the-uk-reduce-meat-consumption-and-cut-emissions-aoe [Accessed 13 April 2023]
Thomas DT, Moore AD, Norman HC, Revell CK (2015) Small effects of deferment of annual pastures through grazing spring wheat crops in Western Australia can benefit livestock productivity. Crop & Pasture Science 66, 410-417.
| Crossref | Google Scholar |
Thomas DT, Beletse YG, Dominik S, Lehnert SA (2021a) Net protein contribution and enteric methane production of pasture and grain-finished beef cattle supply chains. Animal 15, 100392.
| Crossref | Google Scholar |
Thomas DT, Toovey AF, Hulm E, Mata G (2021b) The value of stubbles and chaff from grain crops as a source of summer feed for sheep. Animal Production Science 61, 256-264.
| Crossref | Google Scholar |
Thompson L, Rowntree J, Windisch W, Waters SM, Shalloo L, Manzano P (2023) Ecosystem management using livestock: embracing diversity and respecting ecological principles. Animal Frontiers 13, 28-34.
| Crossref | Google Scholar |
Tilbrook AJ, Ralph CR (2017) Neurophysiological assessment of animal welfare. Animal Production Science 57, 2370-2375.
| Crossref | Google Scholar |
Torok VA, Luyckx K, Lapidge S (2022) Human food waste to animal feed: opportunities and challenges. Animal Production Science 62, 1129-1139.
| Crossref | Google Scholar |
van Barneveld R, Hewitt R, D’Souza D (2023) Net protein contribution from an intensive Australian pork supply chain. Animal Production Science
| Crossref | Google Scholar |
van Vliet S, Kronberg SL, Provenza FD (2020) Plant-based meats, human health, and climate change. Frontiers in Sustainable Food Systems 4, 128.
| Crossref | Google Scholar |
Verdon M (2022) A review of factors affecting the welfare of dairy calves in pasture-based production systems. Animal Production Science 62, 1-20.
| Crossref | Google Scholar |
Whitnall T, Pitts N (2020) Meat consumption. Available at https://www.agriculture.gov.au/abares/research-topics/agricultural-outlook/meat-consumption [Accessed 29 March 2023]
Wiedemann SG, Henry BK, McGahan EJ, Grant T, Murphy CM, Niethe G (2015) Resource use and greenhouse gas intensity of Australian beef production: 1981–2010. Agricultural Systems 133, 109-118.
| Crossref | Google Scholar |
Wiedemann SG, McGahan EJ, Murphy CM (2016) Environmental impacts and resource use from Australian pork production assessed using life-cycle assessment. 1. Greenhouse gas emissions. Animal Production Science 56, 1418-1431.
| Crossref | Google Scholar |
Wiedemann SG, McGahan EJ, Murphy CM (2017) Resource use and environmental impacts from Australian chicken meat production. Journal of Cleaner Production 140, 675-684.
| Crossref | Google Scholar |
Wiedemann SG, McGahan EJ, Murphy CM (2018) Environmental impacts and resource use from Australian pork production determined using life cycle assessment. 2. Energy, water and land occupation. Animal Production Science 58, 1153-1163.
| Crossref | Google Scholar |
Windisch W (2021) Can we do without livestock? Journal of Consumer Protection and Food Safety 16, 193-194.
| Crossref | Google Scholar |
Wong L, Selvanathan EA, Selvanathan S (2015) Modelling the meat consumption patterns in Australia. Economic Modelling 49, 1-10.
| Crossref | Google Scholar |
Wong JT, de Bruyn J, Bagnol B, Grieve H, Li M, Pym R, Alders RG (2017) Small-scale poultry and food security in resource-poor settings: a review. Global Food Security 15, 43-52.
| Crossref | Google Scholar |
Wood P, Thorrez L, Hocquette J-F, Troy D, Gagaoua M (2023) ‘Cellular agriculture’: current gaps between facts and claims regarding ‘cell-based meat’. Animal Frontiers 13, 68-74.
| Crossref | Google Scholar |
Wu F, Groopman JD, Pestka JJ (2014) Public health impacts of foodborne mycotoxins. Annual Review of Food Science and Technology 5, 351-372.
| Crossref | Google Scholar |
You W, Henneberg R, Saniotis A, Ge Y, Henneberg M (2022) Total meat intake is associated with life expectancy: a cross-sectional data analysis of 175 contemporary populations. International Journal of General Medicine 15, 1833-1851.
| Crossref | Google Scholar |
Young M, Kingwell R, Young J, Vercoe P (2020) An economic analysis of sheep flock structures for mixed enterprise Australian farm businesses. Australian Journal of Agricultural and Resource Economics 64, 677-699.
| Crossref | Google Scholar |
Zeraatkar D, Han MA, Guyatt GH, Vernooij RWM, El Dib R, Cheung K, Milio K, Zworth M, Bartoszko JJ, Valli C, Rabassa M, Lee Y, Zajac J, Prokop-Dorner A, Lo C, Bala MM, Alonso-Coello P, Hanna SE, Johnston BC (2019) Red and processed meat consumption and risk for all-cause mortality and cardiometabolic outcomes: a systematic review and meta-analysis of cohort studies. Annals of Internal Medicine 171, 703-710.
| Crossref | Google Scholar |