Forage value of vegetative leaf and stem biomass fractions of selected grasses indigenous to African rangelands
Kevin Z. Mganga
A Department of Agricultural Sciences, South Eastern Kenya University, Kitui, Kenya.
B Department of Forest Sciences, University of Helsinki, Latokartanokaari 7, Helsinki, Finland.
C MetaMeta Research, Postelstraat 2, 5211 EA ’s-Hertogenbosch, The Netherlands.
D Corresponding author. Email: kzowe@yahoo.com; kmganga@seku.ac.ke
Animal Production Science 61(14) 1476-1483 https://doi.org/10.1071/AN19597
Submitted: 16 October 2019 Accepted: 12 April 2021 Published: 25 May 2021
Journal Compilation © CSIRO 2021 Open Access CC BY
Abstract
Context: Rangeland grasses native to Africa constitute the main diet for free-ranging livestock and wild herbivores. Leaf:stem ratio is a key characteristic used for assessing quality of forages. However, studies to determine the allocation of biomass to leaves and stems as well as chemical components and nutritive value, especially of grasses in African rangelands, are rare.
Aim: This study was conducted to establish biomass allocation and chemical and mineral components in leaf and stem fractions of three grasses, Eragrostis superba, Enteropogon macrostachyus and Cenchrus ciliaris, all indigenous to African rangelands.
Methods: Plant height, plant densities, plant tiller densities and biomass yields were estimated at the elongation stage, before inflorescence. Chemical and mineral components were determined from biomass harvested at the vegetative phase for all three grass species. Dry matter, ash content, organic matter, crude protein, neutral detergent fibre, acid detergent fibre, acid detergent lignin, and calcium, phosphorus and potassium contents were determined.
Key results: Enteropogon macrostachyus displayed significantly greater plant and tiller densities and plant height than the other two species. Leaf and stem biomass fractions varied significantly (P < 0.05) among grasses. Leaf:stem ratio of E. superba was double that of E. macrostachyus and C. ciliaris. Crude protein and organic matter yields and net energy for lactation were highest (P < 0.05) in E. superba leaf biomass, as was Ca content.
Conclusions: Eragrostis superba demonstrated greater potential as a forage species for ruminant animal production than E. macrostachyus and C. ciliaris.
Implications: Eragrostis superba is a key forage species that warrants promotion in pasture establishment programs in its native environments.
Keywords: semiarid, dryland, reseeding, ruminants, nutrition, pastoralists, African foxtail grass, buffel grass, Maasai love grass, bush rye grass.
Introduction
In Africa, arid and semi-arid rangelands cover ~41% of the total land mass (Vohland and Barry 2009). These rangeland environments provide a rich source of forage to support different livestock production systems. Nomadic and transhumant systems characterised by mobility and flexibility for best utilisation of the patchy forage resources and unpredictable climatic conditions are important livelihood strategies in African rangelands. In Africa, pastoral communities inhabiting rangelands derive most of their livelihoods from grazing livestock in natural pastures. Indigenous grasses such as Cenchrus ciliaris L. (African foxtail grass or buffel grass), Eragrostis superba Peyr (Maasai love grass), Enteropogon macrostachyus (Hochst. ex A.Rich.) Munro ex Benth (bush rye grass) (Mganga et al. 2015), Chloris roxburghiana Schult. (horsetail grass) (Mnene et al. 2005) and Themeda triandra Forssk. (red oat grass) (Snyman et al. 2013) constitute important and reliable sources of forage for free foraging livestock herds. These grasses are adaptable to harsh climatic conditions.
Cenchrus ciliaris has capacity to withstand heavy grazing and a deep, stabilising root system, and it responds quickly to rainfall events (Marshall et al. 2012). Herbage produced by E. superba, E. macrostachyus and C. roxburghiana is of good grazing value and palatable to cattle, sheep and goats (Mnene et al. 2005). Themeda triandra is often described as keystone grass forage species in Africa, playing a critical role in supporting grazing herbivores and thus vital to livestock production (Snyman et al. 2013). Despite their significant contribution to pastoral livelihoods, there is a dearth of information related to how these indigenous African grasses compare with respect to allocation of biomass to the leaf and stem fractions and the forage value of these individual biomass portions to ruminants.
Studies over the last three decades that have determined the chemical components have aggregated leaf and stem biomass fractions (Abate et al. 1981; Kabuga and Darko 1993; Koech et al. 2016). This approach conceals significant information related to the contribution of the separate biomass portions (Poorter et al. 2012) because: (i) biomass allocation to the leaf and stem fractions of terrestrial plants is not fixed and may vary over time, across environments and among species; and (ii) leaf:stem ratio plays a significant role in ruminant diet selection and forage value determination. Stritzler et al. (1996) attempted to establish the chemical components of leaf and stem biomass fractions for semi-arid warm-season forage grass species in Argentina; however, the forage value of leaf and stem fractions was not compared statistically. Furthermore, Terry and Tilley (1964), using leaf and stem fractions of temperate grasses, determined only the dry matter digestibility and not the chemical components. Koech et al. (2016) established the quality of hay from six tropical rangeland grasses (i.e. C. roxburghiana, E. superba, E. macrostachyus, C. ciliaris, Chloris gayana Kunth and Sorghum sudanense (Piper) Stapf), but like many previous studies, forage analysis was an aggregation of leaf and stem biomass. Studies determining and comparing allocation of biomass to leaf and stem portions and nutritive value of these separate biomass fractions in grasses forages, especially those adapted to African rangeland environments, are limited. Lack of such critical knowledge and information has partly contributed to low livestock productivity in sub-Saharan Africa (SSA). This partly explains why livestock performances in SSA have decreased in the last three decades, corresponding to regional per capita meat and milk production, relative to developed countries indicators, of ~13% and 8%, respectively (Cardoso 2012). Therefore, considerable effort is needed to enhance livestock productivity in this region, including various components such as livestock nutrition and feed resource.
In the present study, leaf and stem fractions of E. superba, E. macrostachyus and C. ciliaris were used to quantify biomass allocation, chemical and mineral components. These grasses were selected based on their contribution to livestock production in African rangelands, evolved adaptive mechanisms for survival, and multipurpose uses to pastoral communities, notably as a source of income through the sale of seed and baled hay, thatching material for houses and granaries, and soil conservation (Mganga et al. 2015). The objectives of the study were to determine and compare aboveground biomass allocation and chemical and mineral components in the leaf and stem biomass fractions of the selected forage grasses. We hypothesised that (i) allocation of biomass in the leaf and stem fractions would be comparable in the three forage grass species, and (ii) chemical rather than mineral components in the leaf and stem fractions would be significantly different in the three grasses in their vegetative phase.
Materials and methods
Morpho-ecological characteristics and forage biomass fractionation
Pure stands of E. superba, E. macrostachyus and C. ciliaris were established from seed (seeding rate 5 kg/ha) in early November 2017 at the South Eastern Kenya University (SEKU) research farm (1.31701, 1°19′1.02317″S; 37.7543, 37°45′26.75293″E (own GPS data)), located in a typical semi-arid rangeland in Kenya. The rainfall pattern in the area is bimodal, with the long rains in March–May and short rains in October–December. Total annual rainfall ranges between 300 and 800 mm and monthly temperatures ranges between 14°C and 34°C, with a mean of 24°C (Schmitt et al. 2019). Rainfall and temperature during the study fell within these ranges (Fig. 1). Basic site characteristics include: soil texture 6% sand, 31% silt, 22% clay; nitrogen (N) 0.08%; carbon 0.8%; phosphorus (P) 165 mg/kg.
![]() |
The experimental layout consisted of five blocks, with an area of 150 m2 (15 m by 10 m), each divided into three subplots of 50 m2 (5 m by 10 m). Each grass species was established without fertilisation in one subplot per block. Grass seeds were sown by hand along ox-ploughed micro-catchments and covered with a thin layer of soil.
Morpho-ecological characteristics and biomass yield measurements were estimated at the recommended harvesting stage corresponding to the phenological stage of development of the selected grasses (i.e. elongation stage, before inflorescence). Plant densities (no. of plants/m2) and average tiller densities per plant species were estimated by using six quadrats, each 0.25 m2 (0.5 m by 0.5 m) within each plot (Cox 1990). Plant height was determined manually by using a ruler (2 m length). The ruler was placed vertically on the ground and the height of the top leaf was estimated to the nearest centimetre.
Forage yields (dry matter (DM) basis) were determined from fresh aboveground biomass. The quadrat sampling technique (Crocker and Tiver 1948) was used to estimate biomass yields. Briefly, fresh biomass of the grass species was clipped during the vegetative phase at a stubble height of 2 cm within a quadrat (0.25 m2). Biomass used for each grass species was obtained from 15 quadrats (i.e. three quadrats per subplot for each species; n = 15). Freshly harvested biomass was placed in labelled brown paper bags and oven-dried at 60°C for 48 h to estimate DM yields. Fresh weight was not taken before drying. Stem and leaf (i.e. leaf blade and leaf sheath) biomass fractions were then manually separated to determine the leaf:stem ratios. Thereafter, dried leaf and stem biomass for each quadrat were ground separately using a mixer mill (MM 200; Retsch, Haan, Germany) and stored before chemical and mineral component analyses.
Forage laboratory analyses
Standard laboratory protocols were followed to establish the chemical components of the harvested forage. Dry matter was estimated after oven-drying at 60°C for 24 h. Ash content was determined after manual combustion in a muffle furnace at 650°C for 24 h (Henken et al. 1986). Organic matter (OM) content was calculated as the difference between DM and ash (i.e. OM = DM – ash content). Nitrogen content (crude protein (CP) = N × 6.25) was determined by the conventional Kjeldahl method. Additionally, in order to determine which of the established grass species presented the most protein, we calculated the CP yield (i.e. kg CP/ha) derived from the already established CP content and DM yields (kg DM/ha). This was done because it is possible for a grass forage species with high CP content to have a low net CP yield due to low DM yield. Similarly, another species may have low CP content but compensate for net CP yield through greater DM yields.
Neutral detergent fibre (NDF) was assayed without heat-stable amylase and expressed inclusive of residual ash (Mertens 2002). Acid detergent fibre (ADF) was expressed inclusive of residual ash (Latimer 2016). We used the NDF and ADF values obtained to calculate the relative feed value (RFV) as displayed in the equations below adapted from Kuehn et al. (1999):


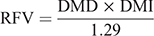
Other studies (e.g. Mwendia et al. 2017; Savadogo et al. 2009) have also computed RFV to assess forage quality of grasses native to Africa. Acid detergent lignin (ADL) was determined by solubilisation of cellulose with sulfuric acid (Robertson and van Soest 1981). The obtained ADF values were used to calculate net energy for lactation (NEl) content as described by Coppock et al. (1981).
The wet ash method was used to prepare samples to determine contents of calcium (Ca; by atomic absorption spectrometry), P (by UV–visible spectroscopy), and K (by flame emission spectroscopy) (Pflaum and Howick 1956). According to Khaled et al. (2006), the main chemical criteria that determine the forage value for ruminants are the concentrations of NDF, ADL, CP, plant-digestible OM and minerals. Calcium, P and K were selected because they are the three most abundant mineral elements in livestock.
Statistical and data analyses
Statistical analyses were performed by using the software STATISTICA 10.0 (StatSoft, Tulsa, OK, USA). One-way ANOVA was used to test for significant differences among the forage grasses. Tukey’s honestly significant difference post hoc test was used to separate significant differences between treatments at P = 0.05 significance level. All displayed results represent arithmetic means (± standard error) of replicates per species. Each replicate was derived from plant biomass in each sampled quadrat.
Results
Enteropogon macrostachyus exhibited significantly higher (P < 0.05) plant density (18 plants/m2) and tiller density (38 tillers/plant) than E. superba (10 plants/m2, 19 tillers/plant) and C. ciliaris (12 plants/m2, 17 tillers/plant) (Table 1). Similarly, at the vegetative stage, E. macrostachyus plants were significantly taller (1.1 m) than E. superba (76 cm), which was taller than C. ciliaris (46 cm) (Table 1).
Leaf (i.e. leaf blade and leaf sheath) and stem biomass fractions of total DM varied among the grasses. Significant differences were mainly observed in leaves. Eragrostis superba (2200 ± 489 kg DM/ha) had significantly higher (P < 0.05) leaf biomass than C. ciliaris (1167 ± 263 kg DM/ha) and E. macrostachyus (1133 ± 265 kg DM/ha). However, stem biomass was not significantly different (P > 0.05) among species: range 1500 DM/ha (E. macrostachyus) to 1367 DM/ha (C. ciliaris) (Table 1). Leaf biomass fraction of aboveground biomass for E. superba (0.61) was significantly higher (P < 0.05) than of C. ciliaris (0.46) and E. macrostachyus (0.43); consequently, the leaf:stem ratio of E. superba (1.57) was significantly different (P < 0.05) from, and two times higher than, that of C. ciliaris (0.85) and E. macrostachyus (0.76) (Table 1).
Dry matter content in biomass was not significantly different among the grasses or between leaf and stem components (Table 2). However, ash, OM, CP, NDF, ADF and ADL contents differed significantly (P < 0.05) between the leaf and stem biomass fractions of the grasses, but not among the grasses (Table 2). Net energy for lactation was significantly higher (P < 0.05) in leaf than stem biomass in all grass species, and highest in E. superba leaf; CP yield was higher in leaf than stem biomass of E. superba and C. ciliaris and was also highest (P < 0.05) in E. superba (Table 3). RFV was also significantly higher (P < 0.05) in leaf than stem biomass fractions, and was comparable in all of the grass species (Table 3). OM yield was higher in leaf than stem biomass of E. superba, whereas the reverse occurred in the other two species; OM yield was highest in E. superba leaf.
Mineral (Ca, P and K) contents did not differ significantly (P > 0.05) between leaf and stem biomass fractions in C. ciliaris (Tables 2). Leaf and stem biomass fractions in E. superba also displayed comparable P and K contents. Calcium content was significantly (P < 0.05) different between the leaf and stem biomass in E. superba and, to a lesser extent, E. macrostachyus, and was highest in E. superba leaves (2.1 g/kg DM). Phosphorus and K contents were significantly higher (P < 0.05) in stem than in leaf biomass in E. macrostachyus (Table 2).
Discussion
Greater plant height (of up to 1.1 m) and plant and tiller densities of E. macrostachyus than of E. superba and C. ciliaris is mainly attributed to its faster seed germination and establishment. This species is known to establish easily, grow very quickly and mature early (Mganga et al. 2015). These morpho-ecological characteristics of E. macrostachyus show its competitive advantage and strength particularly for light interception. During the same growing season, C. ciliaris and E. superba exhibited much shorter culms, and lower plant and tiller densities. Shorter culms of C. ciliaris (0.5 m) are attributed to its slow-growing nature, which is also a coping mechanism against drought, similar to other tropical forages such as E. superba. The measured height is within the average range (0.2–1.5 cm tall) for C. ciliaris culms as reviewed by Marshall et al. (2012). Tinoco-Ojanguren et al. (2016) showed that C. ciliaris exhibits seed dormancy that often hinders successful germination and subsequent establishment. The dormancy mechanism of C. ciliaris lies both within the caryopsis and in the associated structures of the fascicle (Mganga et al. 2015). Similarly, low germination rates of E. superba, which contributed to significantly lower plant densities, have been attributed to involvement of some physiological inhibition mechanism (Krichen et al. 2014) whose effects are partially nullified by the presence of florets. These mechanisms in C. ciliaris and E. superba probably indicate drought-avoidance dormancy syndrome.
Leaf biomass fraction and leaf:stem ratio were higher in E. superba than in E. macrostachyus and C. ciliaris. These results confirm that biomass allocation to different morphological components of terrestrial plants is not fixed and may vary among herbaceous species including grasses (Poorter et al. 2012). A meta-analysis to investigate the effect of nutrient availability on biomass allocation patterns in 27 species of herbaceous plants including grasses (Poa pratensis, Lolium perenne, Bromus inermis) also demonstrated variability in leaf and stem biomass allocation (Müller et al. 2000). Ratio of leaf to stem biomass fractions in tropical forage grasses is of greater significance considering its contribution to diet selection, forage quality and intake by ruminants. Higher mean voluntary intake of leaf than of stem biomass has been demonstrated in tropical grass forages C. ciliaris cv. Biloela, C. ciliaris cv. Gayndah, Panicum coloratum cv. Bambatsi and C. gayana, associated with a shorter retention time of dry matter in the reticulorumen (Mero and Udén 1998). Relative proportions of the different morphological components (leaf blades and stems) have an essential role in controlling the chemical composition of tropical forage grasses. Considering the proportions of the leaf and stem fractions of the three grasses, it is envisaged that E. superba will demonstrate higher voluntary intake indices than E. macrostachyus and C. ciliaris.
Biomass allocation between the leaves and stems also has a significant influence on plant growth and development (Poorter and Nagel 2000). Leafy biomass is a strong driver of the capacity of plants to take up light and CO2. Greater leaf biomass fraction and higher leaf:stem ratio in E. superba strongly suggest its competitive advantage over E. macrostachyus and C. ciliaris in intercepting light for photosynthesis, and its greater leaf fraction strongly indicates its potential for carbon sequestration by capturing and reducing CO2 levels in the atmosphere. Furthermore, higher leaf:stem ratio in E. superba demonstrates its adaptation to nutrient-poor soils in rangeland environments. According to Yan et al. (2016), plants adapted to nutrient limitation allocate less biomass to stems in arid–hot grasslands. Interestingly, the stem biomass fraction did not show significant differences among the three grasses. This suggests that under the prevailing environmental conditions, the grasses allocated a comparable amount of biomass to the stems to provide mechanical support and a hydraulic pathway. Understanding such patterns in biomass allocation is of fundamental importance to agricultural practice and implementation (Poorter et al. 2012).
Differences in the chemical components and RFV in the stem and leaf biomass fractions were not significant between the grass species. These findings conform with previous studies that showed no significant differences in the chemical components (e.g. CP, Ash, ADL, ADF and NDF) of the aggregated aboveground biomass of the same pasture species (Kabuga and Darko 1993; Koech et al. 2016). Aggregate CP of 50 g/kg DM in all three grasses analysed in this study demonstrates that they have the required content for maintenance levels of CP for ruminants (50 g/kg DM) (Boutton et al. 1988) and therefore provide good source of forage for free-grazing herbivores in rangeland environments in Africa. Our findings compare well with those of Ramírez et al. (2004), who reported a CP content of 90 g/kg DM in C. ciliaris and introduced species in arid and semi-arid environments in Mexico. However, these values are lower than the reported CP content of other tropical grass forages, notably C. gayana (121 g/kg DM) and Pennisetum clandestinum (146 g/kg DM) (Tran et al. 2009). On the other hand, E. superba, E. macrostachyus and C. ciliaris had significantly higher DM content (>900 g/kg DM) than C. gayana (390 g/kg DM) (Abate et al. 1981). Low DM content in C. gayana is a characteristic of both mature and immature forages adapted to a humid climate compared with the three grasses adapted to arid and semi-arid climatic conditions in Africa. Conversion of CP content to yield (kg CP/ha) demonstrated that E. superba presented more protein than E. macrostachyus and C. ciliaris. This clearly demonstrates its superior forage value over the other two species. The range of the chemical components ash (40–90 g/kg DM), NDF (650–860 g/kg DM), ADF (400–590 g/kg DM) and ADL (50–190 g/kg DM) found in C. ciliaris and E. superba by Kabuga and Darko (1993) compare well with those found in the leaf and stem biomass fractions of the selected grasses in this study. Additionally, NDF content of the three grass species studied here ranged between ~700 and 800 g/kg DM. This is comparable to other tropical forage grasses C. gayana (693 g/kg DM) and P. clandestinum (681 g/kg DM) (Tran et al. 2009).
Forage value in leafy biomass was significantly higher than in stem biomass in each of the grass species. Significant differences in the chemical component content and RFV between leaf and stem biomass fractions of the selected forage grasses indigenous to African rangelands are probably attributed to the metabolic role of the leaf and structural function of stems. Generally, leaf blades are more digestible, richer in CP and poorer in cell-wall constituents than stems; thus an increasing or decreasing forage value depends on the proportion of plant parts (Delagarde et al. 2000). Our results are consistent with other studies demonstrating that leaf blades have approximately twice as much CP as stems (Ferri 2011). NDF content, an estimate of the cell-wall concentration, is negatively linked to digestibility and intake potential of forages. Leaf biomass fractions had lower NDF concentration than stems in all three grass species. High digestibility of leaf compared with stem fractions has been established in temperate grass species (Terry and Tilley 1964). Leafy biomass is usually retained in the rumen for a shorter period than stems because of faster rates of NDF digestion and higher rates of passage (Delagarde et al. 2000). Tropical grass forage species with high leafy biomass (e.g. E. superba) are more nutritious and will be consumed and digested more readily than those with a higher stem biomass proportion, such as E. macrostachyus and C. ciliaris.
Consequently, higher leaf:stem ratio in E. superba than in E. macrostachyus and C. ciliaris demonstrates its greater potential value for livestock production. Pastoral communities in African rangelands (e.g. Pokot and Il Chamus in Kenya) have identified E. superba as a key forage source for free-ranging livestock. This is attributed mainly to its role in increased milk production and fattening (Wasonga et al. 2003). Pastoral communities in Kenya practising reseeding to replenish depleted natural pastures have also demonstrated a preference for E. superba because of its high nutritional value for ruminants (Mganga et al. 2015). Pastoral Maasai of East Africa have observed that free-grazing livestock tend to select pasture patches dominated by E. superba. This observation conforms with previous studies showing leaf biomass fraction to be the best predictor of bite mass and instantaneous intake rate across different phenological stages of a grasses (Baumont et al. 2000).
Mean concentration of Ca in E. superba (1.5 g/kg DM), E. macrostachyus (1.2 g/kg DM) and C. ciliaris (1 g/kg DM) was much less than 6 g/kg DM recommended for livestock (Juknevičius and Sabiene 2007; NRC 2000; Erickson and Kalscheur 2020). Calcium requirements in growing, gestating and lactating beef cattle are up to 8, 3 and 6 g/kg DM, respectively (Greene 2000). Calcium content of the grasses was also less than in other tropical forage grasses Pennisetum purpureum (36 g/kg DM) and P. maximum (7.4 g/kg DM) reported in tropical Africa (Kambashi et al. 2014). Inadequate Ca content suggests that livestock grazing pastures dominated by these grasses are likely to suffer Ca deficiency. Consequently, Ca supplementation (e.g. mineral licks) is recommended when these grasses constitute the largest portion of the basal diet.
Phosphorus content of 3.5 g/kg DM has been considered optimum for livestock nutrition (Juknevičius and Sabiene 2007; NRC 2000). On average, lactating dairy cows require 3.2–4.2 g P/kg DM in their diet and calves 3.0–4.2 g/kg DM (Erickson and Kalscheur 2020) and beef cattle 2.0–4.0 g/kg DM (Greene 2000). Phosphorus concentration in all of the selected grasses (5 g/kg DM) was much higher than in P. purpureum (1.2 g/kg DM) and P. maximum (2.1 g/kg DM) (Kambashi et al. 2014). Natural fertilisation through manure deposition by grazers contributes significantly to increased available P in open pastures in African rangelands. This translates to high P in plant biomass. Higher P content than the critical range suggests that livestock can obtain sufficient P from all three grasses, thus not limiting production in both beef and dairy enterprises.
In addition to Ca and P, ruminants have a high K requirement to perform numerous body functions, growth and muscle development. Average K content of the analysed grasses was 5–6 g/kg DM. This is lower than the 7.5 g/kg DM recorded for E. superba in Kruger National Park, South Africa (Ben-Shahar and Coe 1992). Critical K levels for lactating dairy cows and calves are 15 and 5 g/kg DM, respectively (Erickson and Kalscheur 2020). On the other hand, critical K contents 6–8 g/kg DM have been established for beef cattle, for growing and fattening of steers and heifers (Greene 2000). Consequently, our results suggest that E. superba, E. macrostachyus and C. ciliaris are more suitable for the beef enterprise and growing and fattening of steers and heifers. Other tropical forage grasses with higher K content, such as P. purpureum (33.6 g/kg DM) and P. maximum (23.8 g/kg DM) (Kambashi et al. 2014), are best suited for dairy production.
Plants allocate more nutrients to leaf biomass to support growth and use only nutrients stored in stems to satisfy the needs of leaves in limited conditions. However, our results demonstrate a uniform distribution of the acquired nutrients to the more metabolically active tissues (i.e. leaves) and less active structural tissues (stems). This allocation pattern suggests that there was less demand for these nutrients in the leaf tissues during the vegetative phase to trigger their translocation from the stem tissues. Furthermore, accumulation of nutrients in stem tissues indicates a possible strategy to store nutrients for a later time when the demand is intensified (e.g. during flowering and seed production). This probably explains higher P and K content in stem than leaf biomass in E. macrostachyus. Unlike E. macrostachyus and C. ciliaris, E. superba demonstrated considerably higher Ca content in leaf than stem biomass. Delivery and allocation of Ca to biomass fractions is linked to transpiration rate. Lower transpiration rates result in lower Ca content of plant tissue (Gilliham et al. 2011). Accumulation of Ca in E. superba leaves suggests its higher transpiration rate than E. macrostachyus and C. ciliaris.
Conclusions
Indigenous grasses E. superba, E. macrostachyus and C. ciliaris are key sources of forage for free-ranging livestock in African rangeland environments. These forage species demonstrated different morpho-ecological characteristics and patterns of biomass allocation and forage quality in the leaf and stem fractions. Eragrostis superba allocated significantly more biomass to the leaf than the stem fraction, translating to two times higher leaf:stem ratio than E. macrostachyus and C. ciliaris. Furthermore, forage value (chemical and mineral components) was largely greater in leaf than stem biomass fractions in all of the selected grasses. The outcomes demonstrate that E. superba is a superior forage species to E. macrostachyus and C. ciliaris. These observed results relate well to indigenous technical knowledge among pastoral communities in African rangelands who have identified E. superba as an important forage species for pastoral livestock production systems.
Conflicts of interest
The authors declare no conflicts of interest.
Acknowledgement
This work was supported by the Netherlands Organisation for Scientific Research and Science (NWO-WOTRO) for Global Development under the Food and Business Applied Research Fund (ARF) (grant no. 3350). The authors dedicate this manuscript to the late Professor Nashon K. R. Musimba, who passed on during the implementation of this work under the ‘Rainwater harvesting from roads for indigenous pasture production and improved rural livelihoods in Kenya’ (ROFIP) project. Additional information of the ROFIP project can be found here https://knowledge4food.net/research-project/arf3-kenya-rofip/
References
Abate A, Kayongo-Male H, Karue CN (1981) Dry matter, protein, energy and fibre intake by dairy heifer grazing a Rhodes grass (Chloris gayana) pasture. Animal Feed Science and Technology 6, 15–26.| Dry matter, protein, energy and fibre intake by dairy heifer grazing a Rhodes grass (Chloris gayana) pasture.Crossref | GoogleScholarGoogle Scholar |
Baumont R, Prache S, Meuret M, Morand-Fehr P (2000) How forage characteristics influence behaviour and intake in small ruminants: a review. Livestock Production Science 64, 15–28.
| How forage characteristics influence behaviour and intake in small ruminants: a review.Crossref | GoogleScholarGoogle Scholar |
Ben-Shahar R, Coe MJ (1992) The relationships between soil factors, grass nutrients and the foraging behaviour of wildebeest and zebra. Oecologia 90, 422–428.
| The relationships between soil factors, grass nutrients and the foraging behaviour of wildebeest and zebra.Crossref | GoogleScholarGoogle Scholar | 28313531PubMed |
Boutton TW, Tieszen LL, Imbamba SK (1988) Seasonal changes in the nutrient content of East African grassland vegetation. African Journal of Ecology 26, 103–115.
| Seasonal changes in the nutrient content of East African grassland vegetation.Crossref | GoogleScholarGoogle Scholar |
Cardoso LA (2012) Environmental and economic impacts of livestock productivity increase in sub-Saharan Africa. Tropical Animal Health and Production 44, 1879–1884.
| Environmental and economic impacts of livestock productivity increase in sub-Saharan Africa.Crossref | GoogleScholarGoogle Scholar | 22528537PubMed |
Coppock CE, Woelfel CG, Belyea RL (1981) Forage and feed testing programs — problems and opportunities. Journal of Dairy Science 64, 1625–1633.
| Forage and feed testing programs — problems and opportunities.Crossref | GoogleScholarGoogle Scholar |
Cox G (1990) ‘Laboratory manual of general ecology.’ 6th edn. (William C. Brown: Dubuque, IA, USA)
Crocker RL, Tiver NS (1948) Survey methods in grassland ecology. Grass and Forage Science 3, 1–26.
| Survey methods in grassland ecology.Crossref | GoogleScholarGoogle Scholar |
Delagarde R, Peyraud JL, Delaby L, Faverdin P (2000) Vertical distribution of biomass, chemical composition and pepsin-cellulase digestibility in a perennial rye grass sward: interaction with month of year, regrowth age and time of day. Animal Feed Science and Technology 84, 49–68.
| Vertical distribution of biomass, chemical composition and pepsin-cellulase digestibility in a perennial rye grass sward: interaction with month of year, regrowth age and time of day.Crossref | GoogleScholarGoogle Scholar |
Erickson PS, Kalscheur KF (2020) Nutrition and feeding of dairy cattle. In ‘Animal agriculture: sustainability, challenges and innovations’. (Ed. W Bazer, GC Lamb, G Wu) pp. 157–180. (Elsevier: Netherlands)
Ferri CM (2011) The seasonal and inter-annual patterns of biomass accumulation and crude protein in kleingrass (Panicum coloratum) in the semiarid Pampean region of Argentina. Ciencia e Investigación Agraria 38, 191–198.
| The seasonal and inter-annual patterns of biomass accumulation and crude protein in kleingrass (Panicum coloratum) in the semiarid Pampean region of Argentina.Crossref | GoogleScholarGoogle Scholar |
Gilliham M, Dayod M, Hocking BJ, Xu B, Conn SJ, Kaiser BN, Leigh RA, Tyerman SD (2011) Calcium delivery and storage in plant leaves: exploring the link with water flow. The Journal of Experimental Biology 62, 2233–2250.
| Calcium delivery and storage in plant leaves: exploring the link with water flow.Crossref | GoogleScholarGoogle Scholar |
Greene LW (2000) Designing mineral supplementation of forage programs for beef cattle. Journal of Animal Science 77, 1–9.
| Designing mineral supplementation of forage programs for beef cattle.Crossref | GoogleScholarGoogle Scholar |
Henken AM, Lucas H, Tijssen PAT, Machiels MAM (1986) A comparison between methods used to determine the energy content of feed, fish and faeces samples. Aquaculture 58, 195–201.
| A comparison between methods used to determine the energy content of feed, fish and faeces samples.Crossref | GoogleScholarGoogle Scholar |
Juknevičius S, Sabiene N (2007) The content of mineral elements in some grasses and legumes. Ekologija (Lietuvos Mokslu Akademija) 53, 44–52.
Kabuga JD, Darko CA (1993) In sacco degradation of dry matter and nitrogen in oven dried and fresh tropical grasses and some relationships to in vitro dry matter digestibility. Animal Feed Science and Technology 40, 191–205.
| In sacco degradation of dry matter and nitrogen in oven dried and fresh tropical grasses and some relationships to in vitro dry matter digestibility.Crossref | GoogleScholarGoogle Scholar |
Kambashi B, Picron P, Boudry C, Théwis A, Kiatoko H, Bindelle J (2014) Nutritive value of tropical forage plants fed to pigs in the Western provinces of the Democratic Republic of the Congo. Animal Feed Science and Technology 191, 47–56.
| Nutritive value of tropical forage plants fed to pigs in the Western provinces of the Democratic Republic of the Congo.Crossref | GoogleScholarGoogle Scholar |
Al Haj Khaled R, Duru M, Decruyenaere V, Jouany C, Cruz P (2006) Using leaf traits to rank native grasses according to their nutritive value. Rangeland Ecology and Management 59, 648–654.
| Using leaf traits to rank native grasses according to their nutritive value.Crossref | GoogleScholarGoogle Scholar |
Koech OK, Kinuthia RN, Karuku GN, Mureithi SM, Wanjogu R (2016) Field curing methods and storage duration affect the quality of hay from six rangeland grass species in Kenya. Ecological Processes 5, 3
| Field curing methods and storage duration affect the quality of hay from six rangeland grass species in Kenya.Crossref | GoogleScholarGoogle Scholar |
Krichen K, Mariem HB, Chaieb M (2014) Ecophysiological requirement on seed germination of a Mediterranean perennial grass (Stipa tenacissima L.) under controlled temperatures and water stress. South African Journal of Botany 94, 210–217.
| Ecophysiological requirement on seed germination of a Mediterranean perennial grass (Stipa tenacissima L.) under controlled temperatures and water stress.Crossref | GoogleScholarGoogle Scholar |
Kuehn CS, Jung HG, Linn JG, Martin NP (1999) Characteristics of alfalfa hay quality grades based on the relative feed value index. Journal of Production Agriculture 12, 681–684.
| Characteristics of alfalfa hay quality grades based on the relative feed value index.Crossref | GoogleScholarGoogle Scholar |
Latimer GW (2016) ‘Official methods of analysis of AOAC International.’ 20th edn. (AOAC International: Rockville, MD, USA)
Marshall VM, Lewis MM, Ostendorf B (2012) Buffel grass (Cenchrus ciliaris) as an invader and threat to biodiversity in arid environments: a review. Journal of Arid Environments 78, 1–12.
| Buffel grass (Cenchrus ciliaris) as an invader and threat to biodiversity in arid environments: a review.Crossref | GoogleScholarGoogle Scholar |
Mero RN, Udén P (1998) Promising tropical grasses and legumes as feed resources in Central Tanzania III: Effect of feeding level on digestibility and voluntary intake of four grasses by sheep. Animal Feed Science and Technology 70, 79–95.
| Promising tropical grasses and legumes as feed resources in Central Tanzania III: Effect of feeding level on digestibility and voluntary intake of four grasses by sheep.Crossref | GoogleScholarGoogle Scholar |
Mertens DR (2002) Gravimetric determination of amylase-treated neutral detergent fibre in feeds with refluxing beakers or crucibles: collaborative study. Journal of AOAC International 85, 1217–1240.
Mganga KZ, Musimba NKR, Nyariki DM, Nyangito MM, Mwang’ombe AW (2015) The choice of grass species to combat desertification in semi-arid rangelands is greatly influenced by their forage value for livestock. Grass and Forage Science 70, 161–167.
| The choice of grass species to combat desertification in semi-arid rangelands is greatly influenced by their forage value for livestock.Crossref | GoogleScholarGoogle Scholar |
Mnene WN, Hanson J, Ekaya WN, Kinyamario JI, Mweki P, Lall G, Stuth JW, Jamnadass RH (2005) Genetic variation between ecotypic populations of Chloris roxburghiana grass detected through RAPD analysis. African Journal of Range & Forage Science 22, 107–115.
| Genetic variation between ecotypic populations of Chloris roxburghiana grass detected through RAPD analysis.Crossref | GoogleScholarGoogle Scholar |
Müller I, Schmid B, Weiner J (2000) The effect of nutrient availability on biomass allocation patterns in 27 species of herbaceous plants. Perspectives in Plant Ecology, Evolution and Systematics 3, 115–127.
| The effect of nutrient availability on biomass allocation patterns in 27 species of herbaceous plants.Crossref | GoogleScholarGoogle Scholar |
Mwendia SW, Yunusa IAM, Sindel BM, Whalley RDB, Kariuki IW (2017) Assessment of Napier grass accessions in lowland and highland tropical environments in East Africa: productivity and forage quality. Experimental Agriculture 53, 27–43.
| Assessment of Napier grass accessions in lowland and highland tropical environments in East Africa: productivity and forage quality.Crossref | GoogleScholarGoogle Scholar |
NRC (National Research Council) (2000) ‘Nutrient requirements of beef cattle.’ 7th edn. (National Academy Press: Washington, DC). Available at http://www.nap.edu/books/0309069343/html
Pflaum RT, Howick LC (1956) Spectrophotometric determination of potassium with sodium tetraphenylborate. Analytical Chemistry 28, 1542–1544.
| Spectrophotometric determination of potassium with sodium tetraphenylborate.Crossref | GoogleScholarGoogle Scholar |
Poorter H, Nagel O (2000) The role of biomass allocation in the growth response of plants to different levels of light, CO2, nutrients and water: a quantitative review. Australian Journal of Plant Physiology 27, 595–607.
| The role of biomass allocation in the growth response of plants to different levels of light, CO2, nutrients and water: a quantitative review.Crossref | GoogleScholarGoogle Scholar |
Poorter H, Niklas KJ, Reich PB, Oleksyn J, Poot P, Mommer L (2012) Biomass allocation to leaves, stems and roots: meta-analyses of interspecific variation and environmental control. New Phytologist 193, 30–50.
| Biomass allocation to leaves, stems and roots: meta-analyses of interspecific variation and environmental control.Crossref | GoogleScholarGoogle Scholar |
Ramírez RG, Haenlein GFW, García-Castillo CG, Núñez-González MA (2004) Protein, lignin and mineral contents and in situ dry matter digestibility of native Mexican grasses consumed by range goats. Small Ruminant Research 52, 261–269.
| Protein, lignin and mineral contents and in situ dry matter digestibility of native Mexican grasses consumed by range goats.Crossref | GoogleScholarGoogle Scholar |
Robertson JB, van Soest PJ (1981) The detergent system of analysis. In ‘The analysis of dietary fibre in food’. (Eds WPT James, O Theander) pp. 123–158. (Marcel Dekker: New York)
Savadogo P, Tigabu M, Sawadogo L, Odén PC (2009) Herbaceous phytomass and nutrient concentrations of four grass species in Sudanian savanna woodland subjected to recurrent early fire. African Journal of Ecology 47, 699–710.
| Herbaceous phytomass and nutrient concentrations of four grass species in Sudanian savanna woodland subjected to recurrent early fire.Crossref | GoogleScholarGoogle Scholar |
Schmitt CB, Kisangau D, Matheka KW (2019) Tree diversity in a human modified riparian forest landscape in semi-arid Kenya. Forest Ecology and Management 433, 645–655.
| Tree diversity in a human modified riparian forest landscape in semi-arid Kenya.Crossref | GoogleScholarGoogle Scholar |
Snyman HA, Ingram LJ, Kirkman KP (2013) Themeda triandra: a keystone grass species. African Journal of Range & Forage Science 30, 99–125.
| Themeda triandra: a keystone grass species.Crossref | GoogleScholarGoogle Scholar |
Stritzler NP, Pagella JH, Jouve VV, Ferri CM (1996) Semi-arid warm-season grass yield and nutritive value in Argentina. Journal of Range Management 49, 121–125.
| Semi-arid warm-season grass yield and nutritive value in Argentina.Crossref | GoogleScholarGoogle Scholar |
Terry RA, Tilley JMA (1964) The digestibility of the leaves and stems of perennial rye grass, cocksfoot, Timothy, tall fescue, lucerne and sainfoin, as measured by an in vitro procedure. Grass and Forage Science 19, 363–372.
| The digestibility of the leaves and stems of perennial rye grass, cocksfoot, Timothy, tall fescue, lucerne and sainfoin, as measured by an in vitro procedure.Crossref | GoogleScholarGoogle Scholar |
Tinoco-Ojanguren C, Reyes-Ortega I, Sánchez-Coronado ME, Molina-Freaner F, Orozco-Segovia A (2016) Germination of an invasive Cenchrus ciliaris L. (buffel grass) population of the Sonaran Desert under various environmental conditions. South African Journal of Botany 104, 112–117.
| Germination of an invasive Cenchrus ciliaris L. (buffel grass) population of the Sonaran Desert under various environmental conditions.Crossref | GoogleScholarGoogle Scholar |
Tran H, Salgado P, Lecomte P (2009) Species, climate and fertilizer effects on grass fibre and protein in tropical environments. The Journal of Agricultural Science 147, 555–568.
| Species, climate and fertilizer effects on grass fibre and protein in tropical environments.Crossref | GoogleScholarGoogle Scholar |
Vohland K, Barry B (2009) A review of in situ rainwater harvesting (RWH) practices modifying landscape functions in African drylands. Agriculture, Ecosystems & Environment 131, 119–127.
| A review of in situ rainwater harvesting (RWH) practices modifying landscape functions in African drylands.Crossref | GoogleScholarGoogle Scholar |
Wasonga VO, Ngugi RK, Kitalyi A (2003) Traditional range condition and trend assessment: lessons from Pokot and Il Chamus pastoralists of Kenya. The Anthropologist 5, 79–87.
| Traditional range condition and trend assessment: lessons from Pokot and Il Chamus pastoralists of Kenya.Crossref | GoogleScholarGoogle Scholar |
Yan B, Ji Z, Fan B, Wang X, He G, Shi L, Liu G (2016) Plants adapted to nutrient limitation allocate less biomass into stems in a arid-hot grassland. New Phytologist 211, 1232–1240.
| Plants adapted to nutrient limitation allocate less biomass into stems in a arid-hot grassland.Crossref | GoogleScholarGoogle Scholar |