Incubation temperature affects growth and efficacy of white-rot fungi to improve the nutritive value of rice straw
T. T. Hai






A
B
C
D
Abstract
A great body of evidence is available on the in vitro efficacy of white-rot fungi (WRF) to degrade lignin in fibre-rich biomass (e.g. wheat straw, wood chips and rice straw (RS)) and improve the biomass’ nutritive value for ruminants.
Determining the impact of incubation temperature of three WRF to improve the nutritional value of rice straw.
Growth of Ceriporiopsis subvermispora, Lentinula edodes and Pleurotus eryngii on RS for 26 days at the following six temperature regimes: continuous at 24°C, 30°C, 35°C and 40°C, and 3 days at 35°C and 40°C, with subsequent days at 24°C. In a follow-up experiment, improvement in fermentability in buffered rumen fluid of RS treated by the three WRF at 24°C and 30°C for up to 8 weeks was investigated.
All three fungi grew at temperatures up to 35°C, with no growth observed at 40°C, with C. subvermispora being more temperature sensitive. There were significant differences in cellulose, hemicellulose and lignin degradation of RS at 24°C and 30°C, with C. subvermispora degrading 69% and 90% of the hemicellulose and lignin respectively at 30°C, greater than at 24°C (55% and 80% respectively). For L. edodes, there were significant differences in cellulose degradation between 24°C and 30°C, with 12% more degradation at 30°C, but not for hemicellulose and lignin. In vitro gas production showed no significant differences between the two incubation temperatures for either of the two fungi. Pleurotus eryngii treatment did not show any improvement in terms of in vitro gas production.
Treatment of RS with L. edodes and C. subvermispora, but not P. eryngii, is robust and temperature changes will not have a major impact on their efficacy as long as the temperature remains below 30°C.
Temperature during the incubation of WRF with rice straw needs to be below 30°C for this biotechnology to be applied in practice.
Keywords: animal nutrition, beef cattle, dairy cows, digestibility, microbiology.
Introduction
Rice is the largest cereal crop in Southeast Asia, and rice grain production coincides with the production of high amounts of straw (Van Soest 2006). Rice straw contains a high fibre content originating from cell walls and is commonly used in ruminant nutrition in these countries. However, a major practical drawback for use is the presence of the high lignocellulose content, which hinders degradability of the cell-wall fraction by the rumen microflora, thereby not allowing the valuable (hemi)cellulose to be fully utilised (Arora and Sharma 2009). Various methods (i.e. physical, chemical, biological) have been developed that can improve the degradation of the fibre content of lignified biomass such as rice straw (Sarnklong et al. 2010). However, many of these treatments cannot be utilised by smallholder farmers because of economic and practical reasons (Sarnklong et al. 2010).
The use of white-rot fungi (WRF) such as Ceriporiopsis subvermispora, Lentinula edodes, and Pleurotus eryngii to upgrade highly lignified biomass, such as rice straw, has recently gained interest because the use of WRF is environmentally friendly, safe and inexpensive (Tian et al. 2012; Tuyen et al. 2012; van Kuijk et al. 2015a). A solid body of evidence is available predominantly on the in vitro efficacy of WRF in degrading lignin and improving the nutritional value of lignified biomass (Tuyen et al. 2012; van Kuijk et al. 2016; Mao et al. 2018; Zhao et al. 2020). A number of variables remains to be investigated before this biotechnology can be applied under practical conditions in the tropics. One of these variables involves the combination of temperature and duration of the incubation of WRF with rice straw. An incubation temperature of 24°C is commonly used to study the in vitro efficacy of WRF to degrade lignin and improve the nutritional value. In comparison, ambient temperatures in, for example, Vietnam fluctuate between 26°C and 35°C, with a mean daily temperature of ~30°C (Nguyen et al. 2012). Temperature is considered as one of the most important factors that affect the growth of fungi (Schubert et al. 2010) and their enzyme activities (Mfombep et al. 2013). To the authors’ knowledge, there are currently no published data available on the impact of incubating highly lignified biomass with WRF at temperatures of 30°C or higher to improve the nutritive value of rice straw.
The aim of the current contribution was to determine the impact of incubation temperature of three WRF to improve the nutritional value of rice straw. First, the sensitivity in terms of growth rate of C. subvermispora, L. edodes and P. eryngii to commonly encountered temperatures (24–40°C) in Southeast Asia was investigated. Next, the fermentability of rice straw incubated with the three WRF species at either 24°C or 30°C for up to 8 weeks in buffered rumen fluid was investigated, using an in vitro gas production (IVGP) technique.
Materials and methods
Preparation of fungal strains and spawn
Three WRF species (C. subvermispora (MES 13094), L. edodes (MES 02121), and P. eryngii (MES 13049)) maintained in the liquid nitrogen collection of Plant Breeding, Wageningen, The Netherlands, were used. Fungal species were propagated on malt extract agar (MEA, malt extract, 20.0 g/L; KH2PO4, 0.5 g/L; MgSO4·7H2O, 0.5 g/L; Ca(NO3)2·4H2O, 0.5 g/L) with a pH of 5.4 at 24°C, until mycelia colonised most of the agar surface. Spawn was prepared by adding a piece (1.5 × 2.0 cm) of colonised agar to sterilised sorghum grains, and incubated in a container (O95/60 + OD95, Sac O2, Deinze, Belgium) at 24°C until all grains were colonised by the mycelium. The spawn was kept at 4°C until further use.
Substrate preparation, fungal inoculation and sampling
To determine the impact of temperature on the colonisation speed of the WRF, rice straw variety ‘Ha Phat 3’ from Vietnam was collected, transported to Wageningen University and ground to pass a 1 mm screen by using a cross-beater mill (Peppink, Deventer, the Netherlands). Then, the ground straw was soaked in tap water for 1 h at room temperature before the water was drained by applying manual pressure to remove excess water. The final dry-matter (DM) content was ~19%. The hydrated straw was subsequently used to manually fill glass tubes (Ø 22 × L 150 mm) open at one side, which were autoclaved at 121°C for 1 h before being cooled to room temperature. Tubes were positioned vertically in six racks, with each rack containing nine tubes. A piece (1 × 1 mm) of malt extract agar from each WRF was placed on top of the rice straw of three tubes per rack before a lose-fitting cap was fitted. A permanent marker was used to mark the top edge of the substrate where the spawn was located, before two vertical lines opposite each other were drawn from the top to the bottom of the tube for measurement of mycelium growth. The tubes in six racks were incubated in four temperature-controlled water baths (water level was below the rack) at the following six different temperature regimes: continuous at 24°C, 30°C, 35°C, and 40°C, 35°C for 3 days, and subsequently 24°C and 40°C for 3 days, and subsequently 24°C. Every Tuesday and Friday of each week, the colony front was marked between the two lines and the distance to the top was measured using each line. At Day 26, the mycelium of one of the strains reached the bottom of the tube, whereafter the experiment was terminated.
In a follow-up experiment, rice straw (Khang Dan 18 cultivar) was obtained from farmers located in central Vietnam, transported to Wageningen University and chopped into particles of approximately 3–5 cm by using a cutting machine at Unifarm (Wageningen, the Netherlands). Thereafter, the rice straw was soaked in tap water for 3 days at room temperature. The water was drained from the wet straw by using a perforated strainer for 5 h, which resulted in a final dry-matter content of 26.2% of the wetted rice straw. The moistened straw was used to fill micropropagation containers (TP1200 + TPD1200, Sac O2, Deinze, Belgium) before all filled containers were autoclaved at 121°C for 1 h and allowed to cool overnight in an aseptic room at room temperature. The weights of empty container, container with wetted straw before and after autoclaving and after spawn inoculation were recorded to determine the weight change at each step. The autoclaved rice straw was aseptically inoculated with spawn at 5% fresh spawn weight to autoclaved rice straw fresh weight. Containers (triplicates for each time point) with inoculated rice straw were incubated at either 24°C or 30°C for 8 weeks in two dedicated climate-controlled chambers. Three containers inoculated with each fungus from each climate-controlled room were collected at Weeks 0, 4, 6, 7, and 8 of incubation. The contents of each container were weighed, freeze-dried, and milled to pass a 1 mm sieve by sing a Wiley mill (100AN, Peppink, Olst, The Netherlands), before being stored at room temperature for chemical analysis and in vitro gas production.
In vitro gas production
Gas production of the WRF-treated rice straw samples was determined for 72 h during incubation in buffered rumen fluid, using fully automated gas-recording equipment (Cone et al. 1996). Samples of each substrate were freeze-dried and ground over a 1 mm sieve by using a Wiley mill (100AN, Peppink, Olst, The Netherlands). An accurately weighed amount (~0.5 g DM) of each sample was transferred into 250 mL fermentation bottles (Schott, Mainz, Germany), with each substrate and blanks (rumen fluid without sample) run in duplicate. Approximately 250 mL rumen fluid was collected from the front ventral, middle ventral and cranial dorsal sac of each of three non-lactating, rumen-cannulated donor cows provided only hay ad libitum. Rumen fluid from each cow was collected before the morning feeding in pre-warmed insulated flasks flushed with CO2. Then, rumen fluid was filtered through two layers of cheese cloth and subsequently mixed (1:2, v/v) with an anaerobic buffer-mineral solution (Cone et al. 1996) under continuous flushing with CO2. Prior to inoculation, fermentation bottles were placed in a shaking water bath and kept at 39°C and pre-flushed with CO2. The fermentation bottles containing fungal-treated rice straw samples were then filled with 60 mL of buffered rumen fluid and connected to the fully automated gas-recording equipment (Cone et al. 1996).
Chemical analysis
Dry matter was determined by drying at 103°C to a constant weight (ISO6496 1999) and ash content after combustion at 550°C for 3 h in a muffle furnace (ISO5984 2002). Nitrogen was determined by means of the Kjeldahl method (ISO5983 2005) and crude protein (CP) was calculated as N × 6.25. Neutral detergent fibre (NDF) was determined using heat-stable amylase according to the standard procedures of Van Soest et al. (1991), with acid detergent fibre (ADF) and acid detergent lignin (ADL) being determined as described by Van Soest et al. (1991), by using an automatic Ankom fibre analyser (A2000I; ANKOM Technology, Macedon, NY, USA). The NDF and ADF contents are expressed exclusive of residual ash. Cellulose was calculated as the difference between ADF and ADL and hemicellulose as the difference between NDF and ADF. Loss of DM was calculated on the basis of the weight change of the content of a container between the beginning and each time point (4, 6, 7 and 8 weeks) of the incubation. The pH of the dried and ground samples was measured using a pH meter (Metrohm, Herisau, Switzerland) after adding 30 mL demineralised water to 1 g of sample and mixing for 30 min at 24 radians per second in a shaking water bath.
Statistical analyses
Linear regression analysis with zero intercept was conducted on the growth data of each individual tube, with growth (mm) as the dependent variable and time (days) as the independent variable. The slopes of the regression lines were subjected to ANOVA by using the general linear model procedure in SPSS, as follows:
where Yij = response variable, μ = overall mean, Ti = incubation temperature (i = 6; 24, 30, 35, 40, 35/24 or 40/24°C), Sj = strains (j = 3; C. subvermispora, L. edodes, or P. eryngii), (T × S)ij = interaction term between incubation temperature and strains, and eij = residual error.
All data from the follow-up experiment were subjected to ANOVA by using the same general linear model where Yij = response variable, μ = overall mean, Ti = incubation temperature (i = 2; 24 or 30°C), Sj = Wj = week (j = 5; 0, 4, 6, 7, or 8) and (T × W)ij = interaction term between incubation temperature and time. Differences among treatment means were compared using Tukey’s statement for multiple comparisons. The level of statistical significance was declared at P < 0.05. Prior to statistical analysis, duplicate in vitro values were averaged.
Results
Growth of WRF incubated at different temperatures
At 40°C, no growth of any of the fungal species was observed. Except for C. subvermispora at 35°C, all growth rates showed a linear pattern. In general, C. subvermispora grew faster than did L. edodes and P. eryngii (P < 0.001), with L. edodes growing, on average, 11.4% slower than P. eryngii (P = 0.009). Ceriporiopsis subvermispora grew 3.3 ± 0.21 and 4.0 ± 0.16 mm/day at 24°C and 30°C respectively, whereas the growth rate was 2.3 ± 0.27 mm/day during the first 8 days at 35°C, with the subsequent 16 days yielding 1.5 mm growth. Pleurotus eryngii mycelium reached a growth rate of 2.1 ± 0.06 and 2.5 ± 0.07 mm/day at 24°C and 30°C respectively, and grew 1.1 ± 0.16 mm/day at 35°C (Fig. 1). After three incubation days at 35°C and the subsequent 23 days at 24°C, P. eryngii and L. edodes had a growth rate of 1.8 ± 0.26 and 1.4 ± 0.10 mm/day respectively. Lentinula edodes, in contrast, did not show any difference in growth rate when incubated between 24°C and 35°C.
Average growth rate of Ceriporiopsis subvermispora (□), Lentinula edodes (), and Pleurotus eryngii (■) incubated on rice straw for 26 days at six temperature regimes (24°C, 30°C, 35°C, 40°C, 35°C/24°C, and 40°C/24°C). No growth was observed for 40°C and 40°C/24°C. Error bars indicate the standard deviation. Means with different letters differ significantly (P < 0.05).
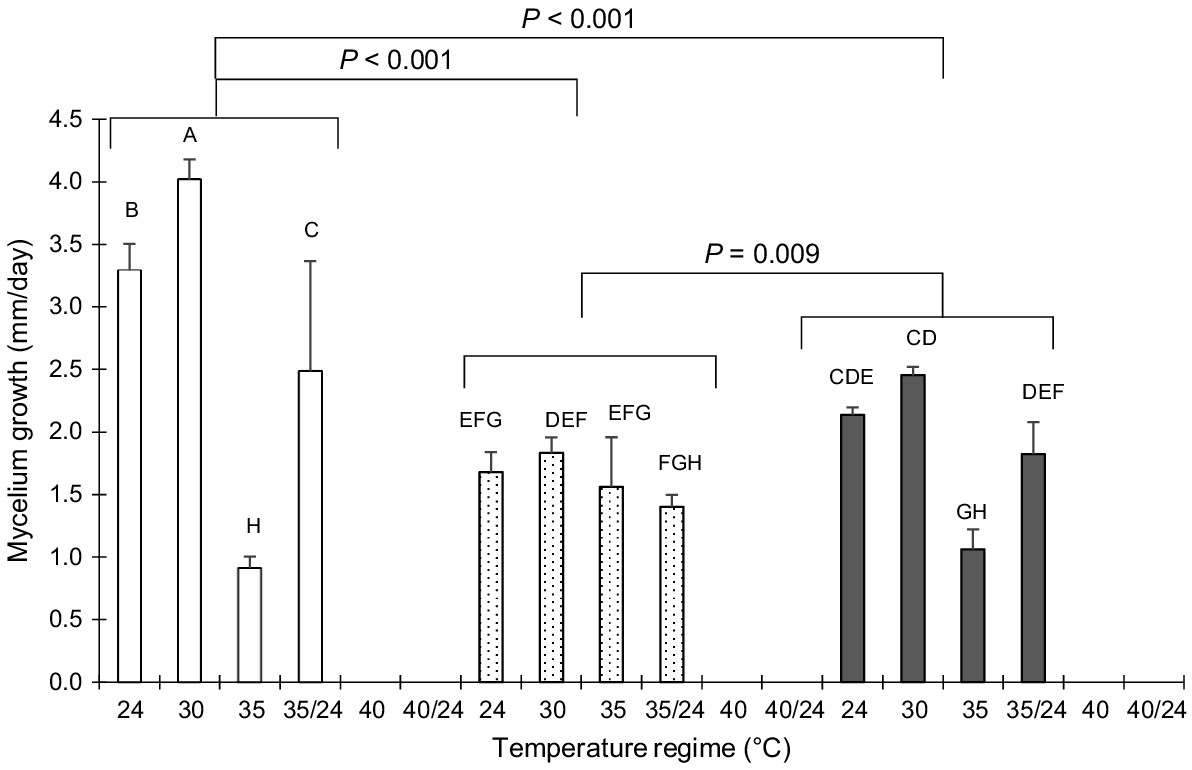
pH changes of WRF-treated rice straw over 8 weeks
There was an effect of strain, temperature, time and their interaction on pH of treated rice straw (P < 0.001). Ceriporiopsis subvermispora and L. edodes decreased the pH over time, whereas P. eryngii increased the pH of the substrate (P < 0.001). The pH was also affected by the incubation temperature, with incubation at 24°C decreasing the pH more than that at 30°C (P < 0.001). Ceriporiopsis subvermispora significantly decreased the pH of rice straw from 4.9 ± 0.08 to 4.0 ± 0.01 when incubated at 24°C and to 4.4 ± 0.03 when incubated at 30°C, with the major decrease occurring during the first 4 weeks of incubation. At both temperatures, no differences were detected from Week 4 to Week 8 of the incubation. A similar trend was observed for L. edodes, with the pH of the treated straw decreasing when incubated at both temperatures. At 24°C, the lowest pH was detected at Week 4 (4.5 ± 0.28), whereafter it significantly increased from Week 6 to Week 8. There were no significant (P = 0.065) differences in the pH of treated rice straw when incubating L. edodes from Week 6 to Week 8 at both temperatures. Pleurotus eryngii significantly increased the pH of treated rice straw for both temperatures.
IVGP and compositional changes of fungal treated rice straw
Tables 1, 2, and 3 show the change in the IVGP and chemical composition of the rice straw during the fungal treatment at 24°C, and 30°C for C. subvermispora, L. edodes and P. eryngii. There was a significant (P = 0.976) effect of incubation time but not the incubation temperature on the IVGP of rice straw treated with WRF. Ceriporiopsis subvermispora significantly (P = 0.015) increased the IVGP of treated rice straw after 7 weeks of incubation. Gas production also increased at Week 6 (281 and 272 mL/g organic matter (OM)) and Week 8 (270 and 280 mL/g OM) of incubation compared with Week 0 (254 mL/g OM), but this difference was not significant (P > 0.05). For L. edodes, a similar trend was observed, with a significant effect of incubation time but not of temperature. The IVGP significantly decreased at Week 4 before being increased from Week 6 to Week 8. The average improvement in IVGP was 12.8% after 6 weeks of incubation at both temperatures (P = 0.059). Pleurotus eryngii also showed a significant (P = 0.011) change in IVGP, but unlike for the other fungi, this was a decrease.
Temperature (°C) | Time (week) | IVGP (mL/g OM) | Content (g/kg dry matter) | Absolute amount (g) | |||||||||
---|---|---|---|---|---|---|---|---|---|---|---|---|---|
OM | CP | Cell | Hcell | ADL | OM | CP | Cell | Hcell | ADL | ||||
Control | 0 | 254 | 912a | 60.5 | 356 | 289 | 44.1 | 20.2 | 1.3 | 7.9 | 6.4a | 1.0 | |
24°C | 4 | 248 | 900b | 66.8 | 371 | 219 | 27.9 | 18.6 | 1.4 | 7.6 | 4.5b | 0.6 | |
6 | 281 | 895bc | 66.2 | 379 | 186 | 17.3 | 17.4 | 1.3 | 7.4 | 3.6c | 0.3 | ||
7 | 297 | 892bcd | 70.3 | 383 | 165 | 16.1 | 17.2 | 1.4 | 7.4 | 3.2cd | 0.3 | ||
8 | 270 | 890cd | 68.1 | 397 | 157 | 9.9 | 16.6 | 1.3 | 7.4 | 2.9cd | 0.2 | ||
30°C | 4 | 268 | 895bc | 73.0 | 373 | 183 | 22.4 | 17.5 | 1.4 | 7.3 | 3.6c | 0.4 | |
6 | 272 | 886de | 78.4 | 388 | 139 | 11.9 | 16.7 | 1.5 | 7.3 | 2.6de | 0.2 | ||
7 | 276 | 887cde | 75.6 | 396 | 149 | 11.1 | 15.5 | 1.3 | 6.9 | 2.6de | 0.2 | ||
8 | 280 | 880e | 78.5 | 406 | 119 | 6.8 | 14.6 | 1.3 | 6.7 | 2.0e | 0.1 | ||
RMSE | 9.3 | 2.0 | 2.64 | 8.1 | 16.0 | 3.51 | 0.48 | 0.08 | 0.41 | 0.27 | 0.08 | ||
P-value | |||||||||||||
Temperature | 0.976 | <0.001 | <0.001 | 0.042 | <0.001 | 0.007 | 0.002 | 0.106 | 0.055 | <0.001 | 0.004 | ||
Time | 0.015 | <0.001 | <0.001 | <0.001 | <0.001 | <0.001 | <0.001 | 0.164 | 0.021 | <0.001 | <0.001 | ||
Temperature × time | 0.288 | 0.033 | 0.210 | 0.661 | 0.117 | 0.622 | 0.288 | 0.176 | 0.581 | 0.020 | 0.537 |
a,b,c Values within a column and within a temperature followed by different letters differ significantly (P < 0.05).
IVGP, in vitro gas production; OM, organic matter; CP, crude protein; cell, cellulose; Hcell, hemicellulose; ADL, acid detergent lignin; RMSE, root mean square error.
Temperature (°C) | Time (week) | IVGP (mL/g OM) | Content (g/kg dry matter) | Absolute amount (g) | |||||||||
---|---|---|---|---|---|---|---|---|---|---|---|---|---|
OM | CP | Cell | Hcell | ADL | OM | CP | Cell | Hcell | ADL | ||||
Control | 0 | 254 | 914 | 59.6d | 372 | 284 | 45.1 | 20.5 | 1.3 | 8.3 | 6.4 | 1.0 | |
24°C | 4 | 238 | 901 | 68.8bc | 396 | 199 | 28.2 | 17.9 | 1.4 | 7.9 | 4.0 | 0. 6 | |
6 | 292 | 895 | 70.0abc | 405 | 181 | 15.3 | 17.0 | 1.3 | 7.7 | 3.4 | 0.3 | ||
7 | 286 | 894 | 68.8bc | 417 | 163 | 13.4 | 16.4 | 1.3 | 7.6 | 3.0 | 0.3 | ||
8 | 284 | 894 | 72.4ab | 415 | 161 | 13.7 | 16.2 | 1.3 | 7.5 | 2.9 | 0.3 | ||
30°C | 4 | 248 | 902 | 64.8cd | 392 | 201 | 32.9 | 16.8 | 1.2 | 7.3 | 3.7 | 0.6 | |
6 | 281 | 893 | 72.9ab | 385 | 176 | 16.9 | 15.9 | 1.3 | 6.9 | 3.2 | 0.3 | ||
7 | 277 | 891 | 75.7a | 387 | 157 | 20.6 | 14.8 | 1.3 | 6.5 | 2.6 | 0.3 | ||
8 | 258 | 889 | 75.3ab | 398 | 146 | 11.9 | 14.5 | 1.2 | 6.5 | 2.4 | 0.2 | ||
RMSE | 11.2 | 1.0 | 2.28 | 11.7 | 12.0 | 3.39 | 0.63 | 0.09 | 0.52 | 0.42 | 0.08 | ||
P-value | |||||||||||||
Temperature | 0.321 | 0.092 | 0.049 | 0.004 | 0.313 | 0.071 | 0.012 | 0.099 | 0.001 | 0.082 | 0.458 | ||
Time | 0.004 | <0.001 | <0.001 | 0.001 | <0.001 | <0.001 | <0.001 | 0.566 | 0.002 | <0.001 | <0.001 | ||
Temperature × time | 0.584 | 0.393 | 0.008 | 0.207 | 0.788 | 0.188 | 0.680 | 0.484 | 0.337 | 0.860 | 0.588 |
a,b,c Values within a column and within temperature followed by different letters differ significantly (P < 0.05).
IVGP, in vitro gas production; OM, organic matter; CP, crude protein; cell, cellulose; Hcell, hemicellulose; ADL, acid detergent lignin; RMSE, root mean square error.
Temperature (°C) | Time (week) | IVGP (mL/g OM) | Content (g/kg dry matter) | Absolute amount (g) | |||||||||
---|---|---|---|---|---|---|---|---|---|---|---|---|---|
OM | CP | Cell | Hcell | ADL | OM | CP | Cell | Hcell | ADL | ||||
Control | 0 | 258 | 912 | 62.4 | 372 | 264a | 49.6 | 19.8 | 1.4 | 8.1 | 5.7 | 1.1 | |
24°C | 4 | 178 | 904 | 64.9 | 397 | 264a | 47.2 | 19.1 | 1.4 | 8.4 | 5.6 | 1.0 | |
6 | 220 | 904 | 64.3 | 408 | 237cd | 37.9 | 18.7 | 1.3 | 8.4 | 4.9 | 0.8 | ||
7 | 236 | 902 | 64.4 | 395 | 224d | 37.1 | 18.4 | 1.3 | 8.0 | 4.6 | 0.8 | ||
8 | 237 | 904 | 66.0 | 402 | 235cd | 29.0 | 17.8 | 1.3 | 8.0 | 4.6 | 0.6 | ||
30°C | 4 | 231 | 904 | 66.7 | 412 | 258ab | 34.5 | 18.5 | 1.4 | 8.4 | 5.3 | 0.7 | |
6 | 227 | 901 | 65.6 | 414 | 262a | 34.6 | 18.4 | 1.3 | 8.5 | 5.4 | 0.7 | ||
7 | 243 | 898 | 68.4 | 406 | 246abc | 28.0 | 16.5 | 1.3 | 7.5 | 4.5 | 0.5 | ||
8 | 219 | 899 | 67.4 | 414 | 243bc | 27.4 | 16.7 | 1.3 | 7.7 | 4.5 | 0.5 | ||
RMSE | 13.5 | 1.0 | 1.99 | 8.6 | 6.6 | 5.98 | 0.50 | 0.06 | 0.37 | 0.30 | 0.12 | ||
P-value | |||||||||||||
Temperature | 0.253 | 0.020 | 0.028 | 0.012 | 0.001 | 0.024 | 0.023 | 0.426 | 0.264 | 0.957 | 0.007 | ||
Time | 0.011 | <0.001 | 0.009 | <0.001 | <0.001 | <0.001 | <0.001 | 0.097 | 0.010 | <0.001 | <0.001 | ||
Temperature × time | 0.149 | 0.350 | 0.534 | 0.613 | 0.002 | 0.337 | 0.402 | 0.884 | 0.586 | 0.308 | 0.237 |
a,b,c Values within a column and within temperature followed by different letters differ significantly (P < 0.05).
IVGP, in vitro gas production; OM, organic matter; CP, crude protein; cell, cellulose; Hcell, hemicellulose; ADL, acid detergent lignin; RMSE, root mean square error.
In rice straw treated with C. subvermispora, only the organic matter (OM) content and the absolute amount of hemicellulose (Table 1) were significantly affected by an interaction between incubation time and temperature (P = 0.033 and P = 0.02 respectively). The chemical composition of rice straw changed significantly, with a continuous decrease in OM, ADL and hemicellulose concentration. The absolute amounts of lignin and hemicellulose were decreased by 80% and 55% at 24°C compared with 90% and 69% at 30°C respectively. In contrast, the cellulose amount did not change when incubated at different temperatures. The absolute amount of CP did not change after 8 weeks at either temperature.
When rice straw was treated with L. edodes, there was an interaction only between incubation temperature and time on CP content (P = 0.008), but not in terms of the absolute amount. There was a significant impact of temperature on OM and cellulose loss. Organic matter and cellulose loss of 21% and 10%, compared with 27% and 22% when incubating at 24°C and 30°C respectively, were observed. On average, 58% and 78% of hemicellulose and lignin contents were degraded by L. edodes after 8 weeks of incubation at both temperatures respectively, with no difference being detected from Week 6 to Week 8.
Pleurotus eryngii showed a significant (P = 0.007) effect of incubation temperature on lignin degradation, with 42% and 45% of the lignin amount being degraded at 24°C and 30°C respectively. In general, P. eryngii degraded 13%, 6%, 3% and 20% in terms of absolute amount of OM, CP, cellulose and hemicellulose respectively, during incubation time.
Discussion
Temperature significantly affeccted the growth of the three WRF as well as the composition of substrate and its nutritive value. All three fungi showed the highest growth rate at 30°C (Fig. 1), although the difference with 24°C was significant only for C. subvermispora. The latter fungus performed poorly at 35°C, showing an initial growth rate of 2.3 mm/day for the first 8 days, but after that time failed to grow (1.5 mm over 16 days). The lack of growth of C. subvermispora at 35°C shows a relatively weak high-temperature resistance of this strain compared with the other two strains used here. It is also possible that C. subvermispora could not absorb nutrients from the rice straw at higher temperatures, and only grew only during the first 8 days owing to the nutrients provided by the spawn, which after being exhausted resulted in a lack of growth. Pleurotus eryngii was also affected by the higher temperatures because the growth rate significantly decreased at 35°C, unlike L. edodes, which showed a consistent growth from 24°C to 35°C. At 40°C, no growth of any of the three fungi was observed. At this temperature, all three WRF appeared to be permanently inactivated as for 3 days at 40°C, no growth was observed when the fungi were, thereafter, incubated at 24°C. Inactivation was not the case when the fungi were grown first for 3 days at 35°C and then at 24°C (Fig. 1). The lack of growth at higher temperatures was unlikely, owing to low moisture levels because all fungi grew under high-humidity conditions in water-filled water baths fitted with a cover. The data regarding temperature are in line with the results for 12 wood-rotting basidiomycete fungi where the optimum growth rate was reported to be between 20°C and 30°C, with none of the fungi being able to grow at 40°C (Boddy 1983). Only 5 of the 12 strains could grow at 35°C. Magan (2008) reported that, in temperate regions, wood-decaying fungi have their optimum temperature for growth between 25°C and 30°C. The WRF used in the present study all originated from temperate regions (C. subvermispora and P. eryngii from the USA, and L. edodes from Japan).
As the WRF grew well at both 24°C and 30°C, these temperatures were selected to determine the effect of incubation temperature on pH changes, IVPG, and chemical composition of treated rice straw. A decrease in pH of the rice straw occurred with C. subvermispora and L. edodes after 8 weeks of growth (Fig. 2). Similar results were reported by Nayan et al. (2018) who incubated 12 strains of C. subvermispora, 10 of L. edodes, and 10 of P. eryngii on autoclaved wheat straw for 7 weeks. On average, the C. subvermispora and L. edodes strains decreased the pH from 4.8 to 3.3 and 3.9 respectively. Although there was an increase in the first 3 weeks, none of the P. eryngii strains increased the pH at Week 7 like the strain used in the current research. Nayan et al. (2018) did report an increased pH of two L. edodes strains at 7 weeks. There appears to be a large variation in terms of pH changes, probably depending on the substrate used by different strains. The P. eryngii strain (MES 13049) used here was not investigated by Nayan et al. (2018). White-rot fungi produce a myriad of organic acids during incubation (Mäkelä et al. 2002; Kwak et al. 2016; Mao et al. 2021). The latter authors found that the majority of the organic acids were produced during the first 4 weeks of incubation, leading to the greatest decrease in pH (Mao et al. 2021). The P. eryngii strain used in the current work increased the pH of the autoclaved rice straw from 5.5 to 6.1 at 8 weeks, which may indicate that this strain did not or produce limited amounts of organic acids during the incubation period.
pH of Ceriporiopsis subvermispora (CS), Lentinula edodes (LE), and Pleurotus eryngii (PE) incubated on rice straw for 8 weeks at 24°C and 30°C. Error bars indicate the standard deviation. Means with different letters differed significantly over time (P < 0.05).
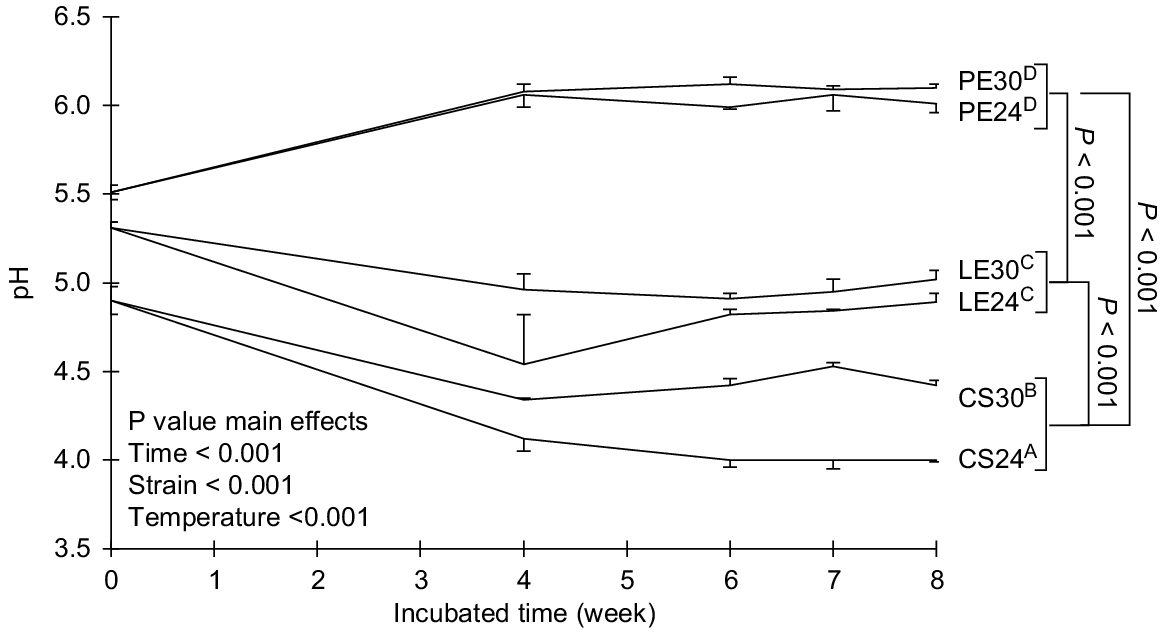
The impact of temperature on the growth of WRF is important from a practical point of view because ambient temperatures fluctuate. Ultimately, however, it is the extent of their improvement in the nutritive value of highly lignified biomass that is key for sustainability of animal production. In vitro gas production was used to measure the efficiency of the WRF in improving the fermentability of treated rice straw by rumen microorganisms. Both C. subvermispora and L. edodes significantly improved the IVGP of rice straw, depending on incubation time, but not temperature. The time to reach the highest IVGP differed by 1 week between C. subvermispora and L. edodes (7 vs 6 respectively). Improvement was, on average, 12.2% and 12.8% respectively, compared with Week 0. In contrast, P. eryngii did not improve, but, in fact, reduced the quality of rice straw. The improvement of the nutritional value of substrate by WRF has been reported by several researchers. Tuyen et al. (2013) showed that rice straw quality improved by incubation with the same strain of C. subvermispora as in the present study, but with a different strain of L. edodes and P. eryngii during 6 weeks of incubation. Besides rice straw, C. subvermispora and L. edodes have been found to improve the quality of wheat straw (Mao et al. 2018; Nayan et al. 2018), wood chips (van Kuijk et al. 2016, 2017), oil palm fronts and sugarcane bagasse (Tuyen et al. 2013), and miscanthus (van Kuijk et al. 2015b; Sonnenberg et al. 2016). In contrast, van Kuijk et al. (2015b) reported a decrease in IVGP of rice straw by a different strains of L. edodes. There are several factors that can explain differences among studies. First, each fungus and strain can perform differently, as Nayan et al. (2018) clearly showed that delignification of wheat straw and, as such the IVGP, is affected by fungal species and strain. The current research used the commercial P. eryngii strain (MES 13049) and indicated that this strain may not be a good strain to treat rice straw. Nayan et al. (2019) also showed that wheat straw cultivars affect the ability of fungal species to delignify the substrate. In this respect, van Kuijk et al. (2015b) used rice straw from Indonesia, which appeared to be of a higher quality than the rice straw used in the current research obtained from Vietnam because a higher IVGP at Week 0 was found by van Kuijk et al. (2015b) than in the current study (275 vs 254 mL/g OM respectively), although all processing and analytical techniques were similar. Therefore, it is important to determine the impact of different rice straw varieties as well as different fungal strains within species on fungal growth and substrate nutritional value.
To better understand the role of temperature on the fungal delignification system, chemical analyses of treated rice straw were conducted. As expected, the CP amount (Tables 1–3) did not change in all treatments at both temperatures. Organic matter loss was observed and it increased with a longer incubation time and a higher incubation temperature. The degradation of carbohydrates to CO2 and H2O during incubation resulted in a loss of OM but not of N. This loss in OM is unwanted but the increase in nutritive value of the treated rice straw is considered more important. The higher temperature appears to increase the production or activity of lignocellulose degradation enzyme systems in all fungi. Ceriporiopsis subvermispora degraded more hemicellulose and lignin at 30°C than 24°C (Table 1). The absolute amount of cellulose showed a similar trend, with 15% loss at 30°C compared with 6% loss at 24°C; however, this difference was not significant. Temperature had a different effect on L. edodes, affecting only the absolute amount of cellulose degradation, whereas P. eryngii degraded more lignin at the higher temperatures, although only 50% of the total lignin that was degraded after 8 weeks, compared with 85% and 75% by C. subvermispora and L. edodes respectively. According to van Kuijk et al. (2016), a higher efficiency in lignin degradation can be linked to higher IVGP. However, in the present study, it was found that L. edodes and C. subvermispora degraded more cellulose and hemicellulose respectively, at 30°C. This may explain why no improvement in IVGP was observed with these strains at 30°C, despite lignin degradation being higher at this temperature. The poor lignin degradation of P. eryngii is in line with the decrease in IVGP of this strain.
Conclusions
Temperature significantly affects the growth of Ceriporiopsis subvermispora, Lentinula edodes, and Pleurotus eryngii on rice straw and their capacity to improve the quality as animal feed. Ceriporiopsis subvermispora was the most sensitive to temperature changes, whereas L. edodes showed a stable growth up to 35°C. More hemicellulose and lignin were degraded by C. subvermispora at 30°C, with L. edodes using more cellulose at this temperature. Unlike P. eryngii, C. subvermispora and L. edodes showed an overall high potential to improve the nutritive value of rice straw up to 30°C.
Data availability
The data that support this study will be shared upon reasonable request to the corresponding author.
Conflicts of interest
We certify that there are no conflicts of interest with any financial organisation regarding the material discussed in the paper.
Declaration of funding
This research was funded by the Victam Foundation and Wageningen University and Research.
Acknowledgements
This study was completed at the Plant Breeding and Animal Nutrition Group (Wageningen University and Research, Wageningen, The Netherlands. Authors are grateful for the financial support of Victam Foundation through the University Fund Wageningen, a scholarship from Wageningen University and Research. The authors thank Dr NQ Co, C Zheng, P Hendrickx and M Visser for their input to this study.
References
Arora DS, Sharma RK (2009) Enhancement in in vitro digestibility of wheat straw obtained from different geographical regions during solid state fermentation by white rot fungi. BioResources 4, 909-920.
| Crossref | Google Scholar |
Boddy L (1983) Effect of temperature and water potential on growth rate of wood-rotting basidiomycetes. Transactions of the British Mycological Society 80, 141-149.
| Crossref | Google Scholar |
Cone JW, van Gelder AH, Visscher GJW, Oudshoorn L (1996) Influence of rumen fluid and substrate concentration on fermentation kinetics measured with a fully automated time related gas production apparatus. Animal Feed Science and Technology 61, 113-128.
| Crossref | Google Scholar |
Kwak A-M, Lee I-K, Lee S-Y, Yun B-S, Kang H-W (2016) Oxalic acid from Lentinula edodes culture filtrate: antimicrobial activity on phytopathogenic bacteria and qualitative and quantitative analyses. Mycobiology 44, 338-342.
| Crossref | Google Scholar | PubMed |
Mäkelä M, Galkin S, Hatakka A, Lundell T (2002) Production of organic acids and oxalate decarboxylase in lignin-degrading white rot fungi. Enzyme and Microbial Technology 30, 542-549.
| Crossref | Google Scholar |
Mao L, Sonnenberg ASM, Hendriks WH, Cone JW (2018) Preservation of Ceriporiopsis subvermispora and Lentinula edodes treated wheat straw under anaerobic conditions. Journal of the Science of Food and Agriculture 98, 1232-1239.
| Crossref | Google Scholar | PubMed |
Mao L, van Arkel J, Hendriks WH, Cone JW, de Vos RCH, Sonnenberg ASM (2021) Assessing the nutritional quality of fungal treated wheat straw: compounds formed after treatment with Ceriporiopsis subvermispora and Lentinula edodes. Animal Feed Science and Technology 276, 114924.
| Crossref | Google Scholar |
Mfombep PM, Senwo ZN, Isikhuemhen OS (2013) Enzymatic activities and kinetic properties of β-glucosidase from selected white rot fungi. Advances in Biological Chemistry 3, 198-207.
| Crossref | Google Scholar |
Nayan N, Sonnenberg ASM, Hendriks WH, Cone JW (2018) Screening of white-rot fungi for bioprocessing of wheat straw into ruminant feed. Journal of Applied Microbiology 125, 468-479.
| Crossref | Google Scholar | PubMed |
Nayan N, van Erven G, Kabel MA, Sonnenberg ASM, Hendriks WH, Cone JW (2019) Improving ruminal digestibility of various wheat straw types by white-rot fungi. Journal of the Science of Food and Agriculture 99, 957-965.
| Crossref | Google Scholar | PubMed |
Nguyen AT, Singh MK, Reiter S (2012) An adaptive thermal comfort model for hot humid South-East Asia. Building and Environment 56, 291-300.
| Crossref | Google Scholar |
Sarnklong C, Cone JW, Pellikaan W, Hendriks WH (2010) Utilization of rice straw and different treatments to improve its feed value for ruminants: a review. Asian–Australasian Journal of Animal Sciences 23, 680-692.
| Crossref | Google Scholar |
Schubert M, Mourad S, Schwarze F (2010) Radial basis function neural networks for modeling growth rates of the basidiomycetes Physisporinus vitreus and Neolentinus lepideus. Applied Microbiology and Biotechnology 85, 703-712.
| Crossref | Google Scholar | PubMed |
Tian X-f, Fang Z, Guo F (2012) Impact and prospective of fungal pre-treatment of lignocellulosic biomass for enzymatic hydrolysis. Biofuels, Bioproducts and Biorefining 6, 335-350.
| Crossref | Google Scholar |
Tuyen VD, Cone JW, Baars JJP, Sonnenberg ASM, Hendriks WH (2012) Fungal strain and incubation period affect chemical composition and nutrient availability of wheat straw for rumen fermentation. Bioresource Technology 111, 336-342.
| Crossref | Google Scholar | PubMed |
Tuyen DV, Phuong HN, Cone JW, Baars JJP, Sonnenberg ASM, Hendriks WH (2013) Effect of fungal treatments of fibrous agricultural by-products on chemical composition and in vitro rumen fermentation and methane production. Bioresource Technology 129, 256-263.
| Crossref | Google Scholar | PubMed |
van Kuijk SJA, Sonnenberg ASM, Baars JJP, Hendriks WH, Cone JW (2015a) Fungal treated lignocellulosic biomass as ruminant feed ingredient: a review. Biotechnology Advances 33, 191-202.
| Crossref | Google Scholar | PubMed |
van Kuijk SJA, Sonnenberg ASM, Baars JJP, Hendriks WH, Cone JW (2015b) Fungal treatment of lignocellulosic biomass: importance of fungal species, colonization and time on chemical composition and in vitro rumen degradability. Animal Feed Science and Technology 209, 40-50.
| Crossref | Google Scholar |
van Kuijk SJA, del Río JC, Rencoret J, Gutiérrez A, Sonnenberg ASM, Baars JJP, Hendriks WH, Cone JW (2016) Selective ligninolysis of wheat straw and wood chips by the white-rot fungus Lentinula edodes and its influence on in vitro rumen degradability. Journal of Animal Science and Biotechnology 7, 55.
| Crossref | Google Scholar |
van Kuijk SJA, Sonnenberg ASM, Baars JJP, Hendriks WH, del Río JC, Rencoret J, Gutiérrez A, de Ruijter NCA, Cone JW (2017) Chemical changes and increased degradability of wheat straw and oak wood chips treated with the white rot fungi Ceriporiopsis subvermispora and Lentinula edodes. Biomass and Bioenergy 105, 381-391.
| Crossref | Google Scholar |
Van Soest PJ (2006) Rice straw, the role of silica and treatments to improve quality. Animal Feed Science and Technology 130, 137-171.
| Crossref | Google Scholar |
Van Soest PJ, Robertson JB, Lewis BA (1991) Methods for dietary fiber, neutral detergent fiber, and nonstarch polysaccharides in relation to animal nutrition. Journal of Dairy Science 74, 3583-3597.
| Crossref | Google Scholar | PubMed |
Zhao X, Wang F, Fang Y, Zhou D, Wang S, Wu D, Wang L, Zhong R (2020) High-potency white-rot fungal strains and duration of fermentation to optimize corn straw as ruminant feed. Bioresource Technology 312, 123512.
| Crossref | Google Scholar | PubMed |