Does the immune stimulant Amplimune® modulate humoral and cytokine responses to commercial bovine respiratory disease vaccines in cattle?
A. L. Alexander






A
B
C
D
Abstract
Feedlot entry can be a period of stress for cattle due to transportation, altered diets and other influences. Stress can suppress host defence mechanisms. Innate immune stimulants, such as mycobacterial cell-wall fractions, attract attention for the primary objective of enhancing non-specific immune resistance of cattle against microbial diseases during periods of stress-induced susceptibility. These stimulants are also recognised for their capacity to modify responses of the adaptive immune system to vaccines.
This study aims to evaluate the potential for mycobacterial cell-wall fractions in Amplimune® to modify adaptive immune responses to the commercial vaccines Rhinogard® (modified live bovine alphaherpesvirus-1 (BoHV-1)) and Bovilis MH + IBR® (inactivated Mannheimia haemolytica and BoHV-1) in yearling cattle during simulated feedlot induction.
Fifty-four mixed-sex Angus yearling cattle were transported for 6 h on Day −1 and on Day 0. The cattle were assigned to the following six treatment groups (n = 9/group): Rhinogard plus 2 mL Amplimune, Rhinogard plus 5 mL Amplimune, Bovilis MH + IBR plus 2 mL Amplimune, Bovilis MH + IBR plus 5 mL Amplimune, Rhinogard plus 5 mL saline, and Bovilis MH + IBR plus 5 mL saline. Blood and nasal secretions were sampled at various time points following treatment and antigen-specific antibody (immunoglobulin G) responses to components of the vaccines were assessed. Interferon-γ production by peripheral blood mononuclear cells in response to BoHV-1, Concanavalin A or media only were assessed.
No adverse clinical reactions were observed to administration of Amplimune and vaccines. A systemic antibody response to vaccination was observed for the Bovilis MH + IBR vaccine. Vaccine-specific antibody and cytokine responses were not modified by Amplimune.
Amplimune can be administered at the same time as Rhinogard or Bovilis MH + IBR vaccines, without undesirable effects on specific immune responses to vaccination.
The primary interest in using Amplimune is to potentiate non-specific immune defences as an alternative to antibiotics for the prevention and/or treatment of microbial diseases such as bovine respiratory disease in production animals. In view of its adjuvant-like activities, administration of Amplimune might also confer beneficial or detrimental effects on antigen-specific responses of the adaptive immune system to contemporaneous vaccination.
Keywords: Amplimune, cattle, enhanced immunity, inactivated vaccine, innate immune stimulant, modified live vaccine, mycobacterial cell wall fractions, respiratory disease.
Introduction
Induction of cattle into a feedlot is an important time that can influence the health and productivity outcomes of the animals undergoing the process (Blakebrough-Hall et al. 2020). Procedures aimed at maintaining or improving the immune health of cattle entering the feedlot include administration of anthelmintics, vaccines and other therapies (Gaughan and Sullivan 2014). Other elements of the transfer of cattle from farm to feedlot include transportation, change in environment, change in feed and increased handling, all of which can cause stress and thereby predispose cattle to bovine respiratory disease (BRD) (Blecha 2000; Cusack et al. 2003; Taylor et al. 2010; Hay et al. 2014). Additional risk factors that can affect the likelihood of feedlot cattle contracting BRD include breed, weight and season in feedlot entry (Hay et al. 2016).
Vaccination status on arrival at the feedlot is not always known, so it is common practice to vaccinate cattle against the pathogens associated with BRD prior to entry (Gaughan and Sullivan 2014). Most vaccines do not confer immediate protection, rather, this can take weeks to develop (Murphy and Weaver 2017), leaving a window for pathogens to trigger disease. However, some vaccine types, such as modified live vaccines (MLV) can induce a rapid innate immune response in addition to the development of protective immunity. For example, vaccinating weaned calves with a MLV containing modified live bovine alphaherpesvirus-1 (BoHV-1) resulted in measurable concentrations of interferon gamma (IFNγ) in serum on Days 7, 14 and 21 post-vaccination and increased concentrations of virus-neutralising antibodies by Day 14 post-vaccination, resulting in no clinical signs and an increased ability to clear viral infection quickly (within 8 days) following experimental challenge with live wild-type BoHV-1 (Chowdhury et al. 2014). Innate immune stimulants administered at the time of vaccination might provide an even broader spectrum of protection than that conferred by specific vaccines alone. For example, fractions of Mycobacterium phlei and liposome-toll-like receptor complexes are being investigated for their ability to improve immune system health, protect against disease and improve growth and production of cattle (Omontese et al. 2020; Wheat et al. 2020).
While they are usually incorporated into the formulation of a vaccine, bystander effects of immune stimulants, administered at a remote site, on antigen-specific vaccine responses are also often evident, especially for mycobacterial products (Zimmermann and Curtis 2018). Mycobacterial preparations used in these studies fall into three classes, namely, whole heat-killed, fractionated heat-killed and live-attenuated (or modified live) mycobacteria. For the first class, Purswani et al. (2011) found that whole heat-killed Mycobacterium indicus pranii increased antibody immunoglobulin G1 (IgG1), IgG2a and IgG2b production against human chorionic gonadotropin vaccine. Regarding the second class, mycolic acids, which make up part of the cell walls of mycobacterium, increased antibody and IFNγ responses to the OVA antigen, which had been concurrently administered in mice (Kubota et al. 2020). Finally, in the third class of mycobacterial preparations, live attenuated mycobacteria such as Mycobacterium bovis (Bacille Calmette Guerin (BCG)), the non-attenuated form of which causes tuberculosis in cattle, can prevent diseases such as tuberculosis and the heterologous yellow fever virus in humans (Arts et al. 2018). However, cattle administered BCG can return false positive results in bovine tuberculosis testing, which is required for monitoring and control of the disease in many countries (Chandran et al. 2019); therefore, researchers are investigating alternatives to BCG for adjuvant-like stimulants in cattle.
To ensure optimal efficacy, it may be necessary to match the immune stimulant to the type of immune response required for protection. For instance, viruses are intracellular pathogens, which typically require a Th1 type response (Ertl 2003). With the well accepted model of BRD development involving a predisposing viral infection (McGill and Sacco 2020), the use of adjuvants that elicit Th1 type responses may be preferred for prevention and treatment of BRD. Previously, subcutaneous injection of M. phlei cell-wall fractions in cattle has been shown to increase the production of the Th1 cytokine tumour necrosis-factor alpha in serum (Alexander et al. 2022), but did not influence interleukin 12 (IL12), another cytokine typically associated with the Th1 response. Nonetheless, contemporaneous administration of innate immune stimulants with commercial vaccines may enhance, or indeed have undesirable effects on the immune response to commercial vaccines.
Amplimune® is an immune stimulant based on the cell-wall fractions of M. phlei, a naturally occurring non-pathogenic mycobacterium (Filion et al. 1999), emulsified in squalane and phosphate buffered solution (PBS). It is commercially available in the USA, Canada and New Zealand (Nosky et al. 2017). In these jurisdictions, it is used for the primary objective of preventing and treating an assortment of livestock diseases via augmentation of non-specific activity of the innate immune system (Griebel 1999; Nosky et al. 2017). For example, the health and clinical signs in dairy calves suffering from enterotoxigenic Escherichia coli infection were significantly improved when calves were administered Amplimune (Romanowski et al. 2017). The safety of Amplimune administered by subcutaneous or intramuscular injection, to cattle slightly younger and lighter than the typical age and weight class entering Australian feedlots was recently established (Alexander et al. 2022). However, the effects of Amplimune on vaccine efficacy, when administered at the same time, but at a different site, as existing vaccine formulations on host immune responses in cattle remains unknown. Therefore, it is hypothesised that contemporaneous administration of Amplimune with commercially available BRD vaccines would enhance adaptive protective immune responses to killed vaccines as compared with MLV, without significant adverse reactions. Hence, the aim of this study was to examine the influence of Amplimune on immune responses of yearling cattle, when administered at the same time, but at a different injection site, as commercial BRD vaccines Rhinogard® (modified live BoHV-1) and Bovilis MH + IBR® (inactivated Mannheimia haemolytica and BoHV-1), exposed to standard feedlot industry procedures.
Materials and methods
Overview
This study was conducted at the CSIRO, F. D. McMaster research station located near Armidale in New South Wales (NSW), Australia. All experimental procedures were pre-approved by the CSIRO, Chiswick Animal Ethics Committee (Animal Research Authority 18/26). Conditions at the time of treatment were designed to mimic those experienced by cattle during initial induction into a commercial feedlot, including exposure to transportation, common animal health treatments and handling through stockyards. Feedlot housing style and feed regime (housed in yards and fed a total mixed ration) were not mimicked. Changes in antigen specific antibody and cytokine parameters were measured to assess responses to both a modified live and an inactivated BRD vaccine in the presence or absence of Amplimune. A detailed timeline of procedures is outlined (Fig. 1).
Animals and experimental design
Fifty-four mixed-sex Angus yearling cattle, (38 heifers and 16 steers) 13 months of age, were enrolled in the study. Cattle were from one contemporary group on the CSIRO Chiswick farm, and thus had the same vaccination background as, and exposure to, potential natural infection. Cattle had not previously been administered Rhinogard, Bovilis MH + IBR, Amplimune, clostridial vaccine or anthelmintic preventative. Cattle had an average liveweight of 222 ± 32 kg. Cattle were grouped on the basis of sex and bodyweight, then randomly allocated to six different treatment groups (n = 9/group). All injections were administered via the subcutaneous route. Bovilis MH + IBR (BOV, Coopers Animal Health, Macquarie Park, NSW, Australia), a vaccine with whole inactivated M. haemolytica and inactivated BoHV-1 components, was applied high on the left side of the neck as per manufacturer’s instructions, Amplimune (an emulsion of 0.5 mg/mL fragmented sections of M. phlei cell wall with nucleic acids conserved onto it, adjuvanted in 2% squalane, final solution delivered in PBS, NovaVive Inc., Napanee, Ontario, Canada) or saline (Sal, Baxter Healthcare Pty Ltd, Old Toongabbie, NSW, Australia) were applied high on the right side of the neck, while Rhinogard (RHINO, Zoetis, West Ryde, NSW, Australia), a vaccine containing attenuated BoHV-1, was administered via intranasal spray as per manufacturer’s instructions.
Cattle received either 2 mL Amplimune and Rhinogard (RHINO2), 5 mL Amplimune and Rhinogard (RHINO5), 5 mL saline and Rhinogard (RHINOSal), 2 mL Amplimune and Bovilis MH + IBR (BOV2), 5 mL Amplimune and Bovilis MH + IBR (BOV5) or 5 mL saline and Bovilis MH + IBR (BOVSal). Cattle were monitored for the development of adverse reactions (lumps and/or lesions) at the injection sites, BRD and signs of general health for 84 days following treatment.
Transport, induction and housing
All cattle were transported by road for 6 h (Day −1) and then yarded overnight with access to lucerne hay and water. The following day (Day 0), animals were again transported by road for 6 h. Following transportation, all cattle were assessed for body condition, respiration rate, rectal temperature and heart rate by a veterinarian to ensure that they were in good health. Cattle received a series of health treatments commonly administered at induction in a commercial feedlot. These included a clostridial vaccination (UltraVac® 7in1, Zoetis, Australia) and anthelmintic treatment (Genesis Ultra, Boehringer Ingleheim, North Ryde, NSW, Australia), administered according to the manufacturers’ recommendations. Experimental treatments as described above, were administered as part of the simulated feedlot induction (Day 0). Cattle were closely monitored by a veterinarian, blinded to treatment groups, for 2 h following treatment for signs of acute adverse reactions. Cattle were drafted out separately immediately post-treatment and kept in separate paddocks for 2 weeks to minimise the risk of BoHV-1 transmission from the groups administered live modified Rhinogard intranasal vaccine to the BOV groups. After the 2 weeks, cattle from both groups were housed in the same paddock for the remainder of the trial. Cattle in the BOV groups were administered a boost vaccine on Day 21 as per the manufacturer’s instructions. Cattle had access to water and pasture ad libitum and were supplemented three times a week with lucerne hay.
Sample collection
Serial blood samples were collected via jugular venepuncture for antigen-specific antibody and cytokine analyses on 0, 14, 21 and 28 days relative to treatment (RHINO groups). To obtain a relevant cross-section of samples in the BOV groups around the boost vaccination of Bovilis MH + IBR, serial blood samples were collected as described above on 0, 14, 21 (prior to booster dose) and 35 days relative to treatment. Blood for serum cytokine concentration analysis was collected into sterile additive free vacutainers (Becton Dickinson, USA), mixed and stored at ambient temperature. In the laboratory, samples were allowed to coagulate at room temperature (RT). Serum was collected from coagulated blood by centrifugation at 700g, for 20 min, RT, and stored in multiple aliquots at −80°C for subsequent antibody assays.
Blood for ex vivo cell culture was collected into sterile EDTA vacutainers (Becton Dickinson USA) from a subset of cattle of both sexes (n = 27) on Day 0 (all groups), Day 42 (RHINO2, n = 5; RHINO5, n = 5; RHINOSal, n = 4) and Day 63 (BOV2, n = 5; BOV5, n = 4; BOVSal, n = 4) and immediately placed on ice. On returning to the laboratory, samples were processed to isolate peripheral blood mononuclear cells (PBMCs) as previously described (Novak et al. 2018). Briefly, blood was chilled on ice, refrigerated when brought to the laboratory for 1 h, then mixed gently and diluted 1:2 with Hank’s balanced salt solution. Diluted blood was layered onto Ficoll-paque (Cytiva, Bio-Strategy, Macquarie Park, Australia) then centrifuged at 400g, 20°C without brake for 45 min. The isolated PBMCs were resuspended in complete culture media (high-glucose Dulbecco’s modified eagle medium (DMEM), 10% fetal calf serum (FCS), 1 × penicillin/streptomycin (P/S), 1 × glutamax; ThermoFisher Scientific, USA) at a rate of 5 × 105 cells/mL.
Nasal secretions were collected from cattle in the RHINO groups on Day 29 for cytokine analysis. Secretions were collected by insertion of sponges (sheep AI sponges; soft grey, 38 mm diameter × 29 mm height, string attached; Animal Health Supplies, Ascot Vale, Vic., Australia) into the medial aspect of one nostril of the animal until the sponge fitted into the nasal curve (approximately to second knuckle of index finger, 40–50 mm) and gently palpated the muzzle. Secretions collected from both nostrils were squeezed through a 50 mL syringe into a 15 mL Falcon tube. Processing involved centrifugation of the raw secretions at 577g for 5 min. The supernatants were then transferred to a new sterile 15 mL tube, volume measured and diluted with an equal volume of PBS containing 0.05% Tween, 1% casein. Three aliquots of the processed secretions were prepared in 1.5 mL tubes and frozen at −20°C for later analysis.
Lymphocyte stimulation
All ex vivo cell cultures were run in duplicate. In total, 5 × 105 cells/mL per well were cultured in 24-well plates (Sarstedt, Germany) and incubated for 24 h at 37°C in a humidified 5% CO2:95% air atmosphere (InCU Safe Sanyo CO2 Incubator, Quantum Scientific). PBMCs in cell culture were stimulated with 1% (v/v) Concanavalin A (ConA) (0.5 μg/μL, Sigma Aldrich, USA) or 0.075% of 1:1000 diluted inactivated BoHV-1 virus (multiplicity of infection = 0.01) or media only as a control. Concanavalin A is a well known mitogen that activates immune cells and is used here as a positive control. Stimulated PBMCs were incubated for 5 days. Supernatant and cells were harvested by scraping and vacuuming from plate wells and aliquoting into four 1.5 mL tubes. Supernatants and cells were immediately frozen and stored at −80°C for further cytokine analysis.
For each blood sample from each animal, isolated PBMCs were stimulated with BoHV-1, ConA or media only. Therefore, at Day 0, there were 81 samples (three culture treatments × 27 animals) to measure production of cytokines in. At Day 42 (RHIN groups), there were 42 samples (three culture treatments × 14 animals) to measure production of cytokines in. At Day 63 (BOV groups), there were 39 samples (three culture treatments × 13 animals) to measure production of cytokines in. Due to time and budget constraints, only supernatant samples from cells cultured on Day 42 and Day 63 were assayed for IFNγ production to evaluate the effect of contemporaneous administration of Amplimune and BRD vaccines on ex vivo responses to viral stimulation.
Enzyme-linked immunosorbent assay
Enzyme-linked immunosorbent assays (ELISAs) were conducted to determine changes in the total Ig antibody response to inactivated M. haemolytica or inactivated or live BoHV-1 in serum. Changes in the concentration of pro-inflammatory cytokine IFNγ in supernatant from cell-culture and nasal-secretion samples collected at various time points post-treatment were determined using bovine-specific ELISA kits. All assays were performed in triplicate. Antibody response to inactivated or live BoHV-1 was measured using a commercial assay, BIO-K 238 Monoscreen AbELISA BoHV-1/indirect, double wells ELISA, according to manufacturer’s instructions (Bio X Diagnostics, Rochefort, Belgium). Antibody response to inactivated M. haemolytica was measured using a commercial assay, BIO-K 139 Monoscreen AbELISA M. haemolytica/indirect, monowell ELISA, according to manufacturer’s instructions (Bio X Diagnostics). These kits used protein G as the conjugate to detection of bovine Ig G isotypes. IFNγ produced by PBMCs and in nasal secretions was measured using a commercial assay, BIO-K 093 QuantELISA Bovine gamma interferon/sandwich, according to the manufacturer’s instructions (Bio X Diagnostics).
Statistical analyses
At the time of the experiment, the stimulant was unregistered for use in Australia, which resulted in limitations on the number of cattle that could be enrolled in the study and their subsequent return to the food chain. Hence, nine animals were selected for each treatment group without conducting sample-size estimations.
Statistical analysis was conducted using Rx64 3.5.0 (R-Core-Team 2018; see https://www.R-project.org/) and packages car (Fox and Weisberg 2019), contrast (O’Callaghan et al. 2020), emmeans (Lenth 2020) and nlme (Pinheiro et al. 2020). For parameters measured over time, repeated measures linear mixed models, fitting animal as a random effect, were used to estimate variance components by using the restricted maximum-likelhood (REML) method. For parameters measured only once, simple linear models were used to estimate variance components by using the REML method. Residuals generated from models were tested for normality by assessing skewness and kurtosis and data were transformed where required to improve normality. Fixed effects assessed in all models included treatment, sex, treatment × sex and bodyweight (at commencement of the trial, Day 0). For repeated measures time and time × treatment were also fitted as fixed effects. Where appropriate, baseline parameter values were fitted as covariates in statistical models. Where fixed effects were clearly not significant (P > 0.1 for single factors or P > 0.2 for interactions) they were removed from the final statistical model. Where significant treatment effects were observed, specific linear contrasts were undertaken to compare Amplimune-treated versus saline-treated (control groups) animals receiving the same vaccine (i.e. Rhinogard or Bovilis MH + IBR). For analysis of ELISA assay data, the plate blank sample was subtracted from the optical-density (OD) samples, then sample ODs were multiplied by the dilution factor and converted to percentages of the positive control sample provided in the kit. For samples measured in the IFNγ kits, sample ODs were converted to arbitrary units (UA) on the basis of the standard curve provided by the kit.
For graphical representation of ELISA results, average ODs with standard error of the mean are displayed, using raw untransformed data. However, the significance of treatments and fixed effects were determined using transformed data, where transformation was required to improve normality.
Results
Results are reported as serum antibody responses to components of vaccines, cytokine responses to ex vivo cell-culture stimulation and cytokine responses in nasal secretions.
Serum antibody responses to vaccination
There was no significant (P = 0.99) effect of treatment group on antibody response to inactivated M. haemolytica, nor was treatment significant over time (P = 0.22). Timing of sample collection was significantly (P < 0.001) associated with antibody responses, suggesting that the vaccine elicited a response regardless of differences among treatment groups (Fig. 2a).
Antibody responses (mean ± s.e.m., optical densities, OD) to (a) inactivated M. haemolytica and (b) inactivated BoHV-1 in Bovilis MH + IBR and (c) live BoHV-1 in Rhinogard vaccines over time in cattle receiving 2 or 5 mL Amplimune, or saline at the same time as the vaccination. Amp, Amplimune. *P < 0.05, **P < 0.01, ***P < 0.001.
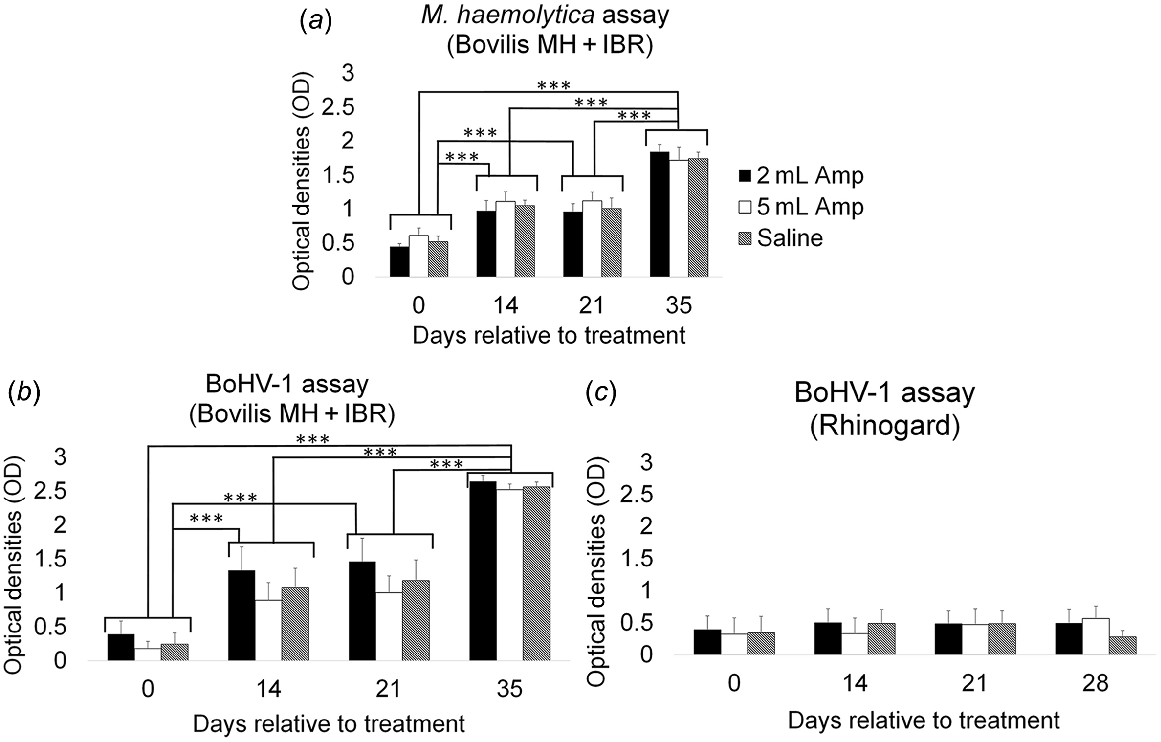
There was no significant (P = 0.94) effect of treatment group on antibody response to inactivated BoHV-1 in the Bovilis MH + IBR vaccine, nor was treatment significant (P = 0.83) over time (Fig. 2b). Timing of sample collection was significantly (P < 0.001) associated with antibody responses, indicating that the vaccine induced a response.
There was no significant effect of treatment group (P = 0.95), or time (P = 0.81) on antibody response to modified live BoHV-1 in the Rhinogard vaccine (Fig. 2c), nor was the effect of treatment significant (P = 0.12) over time.
IFNγ responses by stimulated PBMCs
IFNγ production was detected in 29 of the 39 supernatant samples assayed. There was a significant (P = 0.004) effect of cell stimulation on IFNγ production by PBMCs from cattle that were administered Bovilis MH + IBR (Fig. 3a). IFNγ production was increased by ConA stimulation compared with inactivated BoHV-1 (P < 0.001) or media (P < 0.001), but did not differ between cells stimulated with inactivated BoHV-1 and media alone (P = 0.36). There was no effect of in vivo Amplimune treatment alone on IFNγ production (P = 0.98) and the effect of cell culture stimulation did not differ due to in vivo Amplimune (P = 0.10).
Cytokine IFNγ production (mean ± s.e.m., optical densities, OD) by PBMCs stimulated with inactivated BoHV-1, ConA or Saline. PBMCs were isolated from blood collected from cattle receiving (a) Bovilis MH + IBR or (b) Rhinogard, and either 2 or 5 mL Amplimune, or saline. Amp, Amplimune. BoHV-1, inactivated bovine alphaherpesvirus 1. ConA, Concanavalin A. *P < 0.05, **P < 0.01, ***P < 0.001.
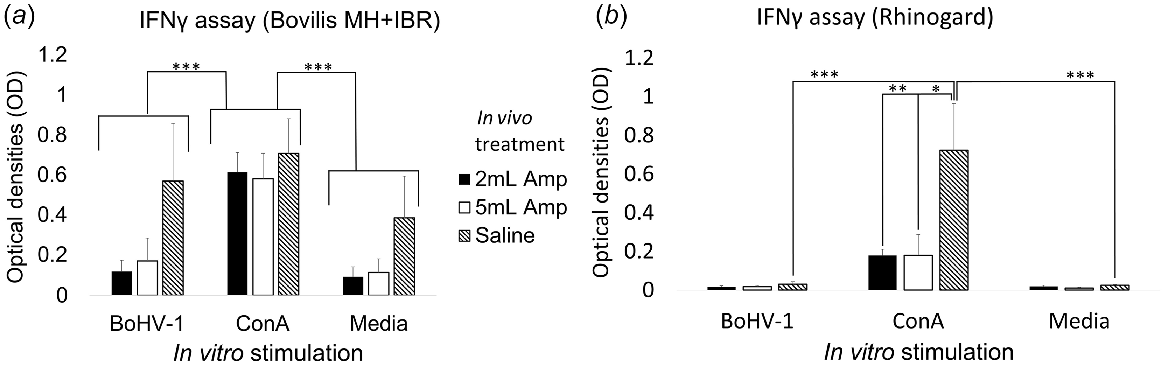
IFNγ production was detected in 12 of the 42 samples assayed. In each case, the responding samples had been stimulated with ConA, indicating that cells were capable of producing IFNγ under appropriate conditions. There was a significant (P < 0.001) effect of cell stimulation on IFNγ production by PBMCs from cattle that were administered Rhinogard, but no effect (P = 0.20) of in vivo Amplimune treatment on its own. However, there was an interaction between in vivo Amplimune treatment and cell stimulation (P < 0.01) (Fig. 3b). IFNγ production was increased by ConA stimulation but the concentration was significantly different between PBMCs from cattle that were administered either 2 mL Amplimune (P = 0.02) or 5 mL Amplimune (P = 0.05) and the saline-treated animals. Within samples from cattle treated with saline only, cells stimulated with ConA produced significantly more IFNγ than did those stimulated with inactivated BoHV-1 (P < 0.001) or media only (P < 0.001). There was no difference in production of IFNγ between cells stimulated with inactivated BoHV-1 and media only (P = 0.10).
Nasal secretions
IFNγ concentrations in nasal-secretion samples were below the limit of detection of the assay (data not shown).
Clinical observations
The rectal temperatures recorded following transportation but before vaccine and Amplimune treatment ranged from 38.5°C to 39.6°C. Temperature was not recorded again. No changes were observed by the veterinarian in any of the clinical parameters (body condition, respiration rate and heart rate) in any cattle following treatments administered in the trial. Small subcutaneous lumps (5–30 mm diameter) at the site of injection with Amplimune were recorded in 39% of the 36 cattle that received this treatment. All lumps had resolved by the conclusion of the trial (Supplementary Table S1, AmplimuneVaccineTrial_LumpTracking). There was no evidence for any associations between Amplimune injection-site lumps and the administered vaccines. One steer and one heifer were treated with Orbenin (Zoetis, Australia) for weepy eyes, 5 days prior to treatment, and 2 weeks post-treatment with Amplimune. There were no signs of any other illness, including BRD, in the 84 days following treatment with Amplimune.
Discussion
We hypothesised that contemporaneous administration of Amplimune (at a paired injection site) with commercial BRD vaccines would have a beneficial, or at least not have a detrimental, effect on antibody and cytokine immune responses to vaccination. Further, we expected the effect to differ between the killed vaccine and the MLV. The effect of contemporaneous administration of two different doses of Amplimune, with two different vaccines, Bovilis MH + IBR, a multi-pathogen inactivated vaccine and Rhinogard, a MLV, were assessed. As expected, serum antibody Ig titres to inactivated M. haemolytica and inactivated BoHV-1 increased following vaccination with Bovilis MH + IBR; however, antibody responses were not affected by treatment with Amplimune. Unexpectedly, serum antibody titres did not increase following vaccination with Rhinogard, although the intranasal route of administration is expected to have implications for serum antibody responses. Serum antibody responses to modified live vaccines, which contain modified live BoHV-1, BVDV and Parainfluenza 3 antigenic components, have displayed significant increases by Day 14 and remain high (depending on boost vaccination status) for up to 196 days post-vaccination (Fulton et al. 1995). It is possible that immune responses to Rhinogard were elicited locally in the nasal and respiratory passages, as Rhinogard is designed to do, and were harder to detect in circulating serum than those measured following an intramuscular injection of modified live vaccines such as those tested by Fulton et al. (1995). Thus, Amplimune neither enhanced nor compromised antibody responses to Bovilis MH + IBR or Rhinogard vaccines.
Ex vivo stimulation of PBMCs, from cattle in the BOV groups, induced production of IFNγ when stimulated by ConA. However, there were no differences in production of IFNγ between PBMCs stimulated with inactivated BoHV-1 and media only. Nor did in vivo administration of Amplimune affect ex vivo production of IFNγ in BOV group animals. There appeared to be a similar response to ConA stimulation of IFNγ production by PBMCs from cattle in the RHINO groups. Interestingly, there was also a significant difference in IFNγ production by PBMCs from cattle in the RHINO groups treated in vivo with Amplimune or saline, with Amplimune having a negative effect on ex vivo IFNγ production. In the current study, it should be noted that only 12 of the 42 supernatant samples contained detectable IFNγ concentrations and those 12 were all stimulated ex vivo with ConA. In addition, PBMCs were cultured with the stimulants ConA, inactivated BoHV-1 and media for five full days in the current study, following the PBMC isolation protocol of Novak et al. (2018). It is possible that different results could have been obtained after a shorter period of stimulation. For example, 3 days of ex vivo challenge with live or heat-inactivated BoHV-1 has been shown to be enough time to measure a significant increase in production of IFNγ by cattle PBMCs (Woolums et al. 2003). Additionally, IFNγ production in supernatant has been measured in sheep abomasal or mesenteric lymph node cells following only 24 h ex vivo challenge with ConA or Haemonchus contortus antigen (Gill et al. 2000).
During a primary infection of the bovine upper respiratory tract, BoHV-1 was found to be a strong trigger for production of IFNγ in nasal secretions (Osman et al. 2017). In the current study, no IFNγ was detected in the nasal-secretion samples; however, IFNγ in nasal-secretion samples was assessed only on Day 29. Unfortunately, supplies were not available for the collection of nasal samples at earlier time points. In comparison, studies on the production of IFNγ in serum from calves following intranasal vaccination with modified live BoHV-1 vaccines has indicated that IFNγ concentrations can be detected 4 days post-vaccination (Hill et al. 2019), and are likely to be low by 28 days following the vaccination (Chowdhury et al. 2014). Additionally, two studies on intranasal challenge with a live strain of BoHV-1 in calves, observed increased production of IFNγ in nasal-secretion samples by Day 3 following infection (Hodgson et al. 2012; Osman et al. 2017). While immune responses are likely to be different between a live challenge and a modified live vaccination, these studies do suggest that IFNγ can be detected in nasal-secretion samples within a week of vaccination or challenge.
Most vaccine adjuvant studies with live attenuated mycobacteria have been conducted with BCG being injected at a remote site (or time) from vaccination (Zimmermann et al. 2019). Complete Freund’s adjuvant is another immune stimulant that has been widely explored for its adjuvanticity (Stils 2005), yet its use is restricted due to the often severe side effects, including lesions, fever, lameness and mortality (Broderson 1989; Haak et al. 1996). A meta-analysis of eight studies in humans has found a beneficial effect of BCG administration on antibody responses to heterologous vaccines in five of those studies and lower antibody responses in one of the studies (Zimmermann and Curtis 2018). Significantly elevated antibody responses have been observed to 4 of the 16 vaccines used in these studies (Zimmermann and Curtis 2018). However, BCG is excluded from use in cattle due to cross-reactivity issues for bovine tuberculosis diagnostic testing. One alternative showed some potential when Hurley et al. (2019) investigated the ability of an immune stimulant based on β-glucan from fungal cell walls to enhance calf responses to a boost vaccination with Bovi-Shield. They found no differences in serum immune responses, but significant differences in mononuclear cell-proliferation response and production of IL4 when the mononuclear cells were subsequently stimulated in vitro with killed BoHV-1, live BVDV-1 or live BVDV-2, suggesting that the immune stimulant had enhanced vaccine antigen recall (Hurley et al. 2019). Nevertheless, many differences occur in the immunomodulatory effects between live and killed bacteria or viruses; hence, the decision to explore the impact of Amplimune and both types of vaccines in the current study. It could be expected that the modified live vaccine would induce a stronger immune response in cattle early in the experiment (Day 14) (Fulton et al. 1995); however, virus-neutralising antibodies and cell-mediated response were not measured in the current trial.
There was a significant increase in serum Ig antibody response to the inactivated Bovilis MH + IBR vaccine over time, but the same was not true for serum Ig antibody response to the live modified Rhinogard vaccine. This is likely to be due to the mode of vaccine delivery affecting the systemic response differently between the two vaccines. Bovilis MH + IBR is administered subcutaneously, where systemic immune defences can respond quickly, while Rhinogard is administered via intranasal spray, where a localised mucosal response is targeted, and it may take longer for the animals to seroconvert. Future studies should aim to measure IFNγ and virus-neutralising antibodies in nasal secretions from an earlier sample collection time point, along with Ig antibodies in serum.
No significant enhancement or suppression of responses to the BRD vaccines used in the current study were observed. The small subcutaneous lumps that appeared following administration of Amplimune, which were likely to be an indication of the cellular response to the stimulant, resolved fully within 3 months. Signs of acute adverse reactions to treatment were absent in the 2 h following treatment. Cattle all appeared in good health by the conclusion of the trial. Results suggest that Amplimune can be administered to cattle at the same time as Rhinogard or Bovilis MH + IBR vaccines without adverse effects on adaptive immune responses to vaccination.
The absence of detectable negative effects on the immune responses to vaccination evaluated in the current study and safety data from prior experiments (Alexander et al. 2022) support the further evaluation of the benefits of Amplimune in either experimental trials that include a pathogen-challenge component or in a commercial feedlot setting. The inclusion of a pathogen-challenge component to future pen trials would enable the indirect assessment of any benefits of Amplimune on the complete immune response to vaccination, rather than the limited number of immune factors measured in the current study. Further, the complex interaction of factors that contribute to the risk of cattle developing BRD in feedlots (Hay et al. 2014, 2016) suggests that it is likely to be difficult to accurately assess the beneficial effects, if any, of Amplimune with respect to this disease in controlled settings. Whereas, under field conditions in a commercial feedlot where multiple factors potentially affect immune function and BRD risk, the benefit of an immune stimulant may be more evident. These benefits could be either direct or indirect, as detected by a reduced BRD incidence or by improvements in production performance respectively (Nosky et al. 2017; de Souza et al. 2018).
Conclusions
The primary interest in using Amplimune is to potentiate non-specific immune defences as an alternative to antibiotics for the prevention and/or treatment of microbial diseases, such as BRD, in production animals. In view of its previously noted adjuvant-like activities (Alexander et al. 2022), administration of Amplimune might also confer beneficial or detrimental effects on antigen-specific responses of the adaptive immune system to contemporaneous vaccination. In the current study, Amplimune, administered at the same time, but at a different anatomical location, as Bovilis MH + IBR or Rhinogard in yearling beef cattle had no detrimental effect on responses to vaccination. Further research should be undertaken to investigate the potential for Amplimune used in conjunction with vaccination to improve health outcomes at the feedlot, both in terms of morbidity and mortality.
Data availability
The data that support this study are available in CSIRO’s Data Access Portal at https://doi.org/10.25919/hty2-hj21 (Alexander et al. 2023).
Declaration of funding
This work was funded by the Commonwealth Scientific and Industrial Research Organisation (CSIRO). Author Annika Alexander was the recipient of the Ian McMaster Bequest scholarship, the Sally Muir Postgraduate Agriculture Award, the QTAC Rural and Regional Enterprise Scholarship and the University of New England DVCR Completion Scholarship.
Acknowledgements
The authors acknowledge Dom Niemeyer, Duncan Elks, Graham Acton, Troy Kalinowski, Jim Lea, Amy Bell and Justin Matthews for their assistance in conducting trial work. We also express gratitude to Nigel Scott for his veterinary expertise in assessing the health of the livestock. We also gratefully acknowledge supply of the stimulant Amplimune by NovaVive Inc.
References
Alexander AL, Doyle E, Ingham AB, Colditz I, McRae G, Alkemade S, Cervantes MP, Hine BC (2022) The innate immune stimulant Amplimune® is safe to administer to young feedlot cattle. Australian Veterinary Journal 100, 261-270.
| Crossref | Google Scholar | PubMed |
Arts RJW, Moorlag SJCFM, Novakovic B, Li Y, Wang S-Y, Oosting M, Kumar V, Xavier RJ, Wijmenga C, Joosten LAB, Reusken CBEM, Benn CS, Aaby P, Koopmans MP, Stunnenberg HG, van Crevel R, Netea MG (2018) BCG vaccination protects against experimental viral infection in humans through the induction of cytokines associated with trained immunity. Cell Host & Microbe 23, 89-100.e5.
| Crossref | Google Scholar | PubMed |
Blakebrough-Hall C, McMeniman JP, González LA (2020) An evaluation of the economic effects of bovine respiratory disease on animal performance, carcass traits, and economic outcomes in feedlot cattle defined using four BRD diagnosis methods. Journal of Animal Science 98, skaa005.
| Crossref | Google Scholar |
Broderson RJ (1989) A retrospective review of lesions associated with the use of Freund’s adjuvant. Laboratory Animal Science 39, 400-405.
| Google Scholar | PubMed |
Chandran A, Williams K, Mendum T, Stewart G, Clark S, Zadi S, Lanni F, McLeod N, Williams A, Villarreal-Ramos B, Vordermeier M, Maroudam V, Prasad A, Bharti N, Banerjee R, Manjari Kasibhatla S, McFadden J (2019) Development of a diagnostic compatible BCG vaccine against bovine tuberculosis. Scientific Reports 9, 17791.
| Crossref | Google Scholar |
Chowdhury SI, Wei H, Weiss M, Pannhorst K, Paulsen DB (2014) A triple gene mutant of BoHV-1 administered intranasally is significantly more efficacious than a BoHV-1 glycoprotein E-deleted virus against a virulent BoHV-1 challenge. Vaccine 32, 4909-4915.
| Crossref | Google Scholar | PubMed |
Cusack PMV, McMeniman N, Lean IJ (2003) The medicine and epidemiology of bovine respiratory disease in feedlots. Australian Veterinary Journal 81, 480-487.
| Crossref | Google Scholar | PubMed |
de Souza KA, Cooke RF, Schubach KM, Brandão AP, Schumaher TF, Prado IN, Marques RS, Bohnert DW (2018) Performance, health and physiological responses of newly weaned feedlot cattle supplemented with feed-grade antibiotics or alternative feed ingredients. Animal 12, 2521-2528.
| Crossref | Google Scholar | PubMed |
Filion MC, Lépicier P, Morales A, Phillips NC (1999) Mycobacterium phlei cell wall complex directly induces apoptosis in human bladder cancer cells. British Journal of Cancer 79, 229-235.
| Crossref | Google Scholar | PubMed |
Fulton RW, Confer AW, Burge LJ, Perino LJ, d’Offay JM, Payton ME, Mock RE (1995) Antibody responses by cattle after vaccination with commercial viral vaccines containing bovine herpesvirus-1, bovine viral diarrhea virus, parainfluenza-3 virus, and bovine respiratory syncytial virus immunogens and subsequent revaccination at day 140. Vaccine 13, 725-733.
| Crossref | Google Scholar | PubMed |
Gill HS, Altmann K, Cross ML, Husband AJ (2000) Induction of T helper 1- and T helper 2-type immune responses during Haemonchus contortus infection in sheep. Immunology 99, 458-463.
| Crossref | Google Scholar | PubMed |
Griebel P (1999) Evaluation of the ability of MCWF immunostimulant to alter blood leucocyte populations in newborn calves. Vaccine and Infectious Disease Organisation Experiment Report for Vetrepharm Research Inc. Available at http://novavive.ca/bovine-research [accessed 3 February 2019]
Haak T, Delverdier M, Amardeilh MF, Oswald IP, Toutain PL (1996) Pathologic study of an experimental canine arthritis induced with complete Freund’s adjuvant. Clinical and Experimental Rheumatology 14, 633-641.
| Google Scholar | PubMed |
Hay KE, Barnes TS, Morton JM, Clements ACA, Mahony TJ (2014) Risk factors for bovine respiratory disease in Australian feedlot cattle: use of a causal diagram-informed approach to estimate effects of animal mixing and movements before feedlot entry. Preventive Veterinary Medicine 117, 160-169.
| Crossref | Google Scholar | PubMed |
Hay KE, Morton JM, Mahony TJ, Clements ACA, Barnes TS (2016) Associations between animal characteristic and environmental risk factors and bovine respiratory disease in Australian feedlot cattle. Preventive Veterinary Medicine 125, 66-74.
| Crossref | Google Scholar | PubMed |
Hill K, Arsic N, Nordstrom S, Griebel PJ (2019) Immune memory induced by intranasal vaccination with a modified-live viral vaccine delivered to colostrum fed neonatal calves. Vaccine 37, 7455-7462.
| Crossref | Google Scholar | PubMed |
Hodgson PD, Aich P, Stookey J, Popowych Y, Potter A, Babiuk L, Griebel PJ (2012) Stress significantly increases mortality following a secondary bacterial respiratory infection. Veterinary Research 43, 21.
| Crossref | Google Scholar | PubMed |
Hurley DJ, Barber CE, Adkins M, Rowson AD, Norton NA, Nickerson SC, Ely LO, Kautz FM, McLean DJ, Chapman JD (2019) An immunomodulatory feed additive enhances in vitro viral vaccine recall antigen responses in dairy heifers. Research in Veterinary Science 127, 11-17.
| Crossref | Google Scholar | PubMed |
Kubota M, Iizasa E, Chuuma Y, Kiyohara H, Hara H, Yoshida H (2020) Adjuvant activity of Mycobacteria-derived mycolic acids. Heliyon 6, e04064.
| Crossref | Google Scholar | PubMed |
McGill JL, Sacco RE (2020) The immunology of bovine respiratory disease: recent advancements. Veterinary Clinics of North America: Food Animal Practice 36(2), 333-348.
| Crossref | Google Scholar |
Nosky BJ, Biwer J, Alkemade S, Prunic B, Milovanovic A, Maletic M, Masic A (2017) Effect of a non-specific immune stimulant (Amplimune™) on the health and production of light feedlot calves. Journal of Dairy, Veterinary & Animal Research 6, 302-306.
| Crossref | Google Scholar |
Novak B, Vatzia E, Springler A, Pierron A, Gerner W, Reisinger N, Hessenberger S, Schatzmayr G, Mayer E (2018) Bovine peripheral blood mononuclear cells are more sensitive to deoxynivalenol than those derived from poultry and swine. Toxins 10, 152.
| Crossref | Google Scholar | PubMed |
Omontese BO, Caixeta LS, Machado VS, Rendahl A, Celestino MLK, Menta PR, Paiva D, Garcia-Munoz A, Masic A (2020) Effects of the administration of a non-specific immune stimulant around transportation on health and performance of Jersey and Jersey-cross heifer calves during the rearing period: randomized clinical trial. Frontiers in Veterinary Science 7, 550202.
| Crossref | Google Scholar |
Osman R, Gonzalez-Cano P, Brownlie R, Griebel PJ (2017) Induction of interferon and interferon-induced antiviral effector genes following a primary bovine herpesvirus-1 (BHV-1) respiratory infection. Journal of General Virology 98, 1831-1842.
| Crossref | Google Scholar | PubMed |
Purswani S, Talwar GP, Vohra R, Pal R, Panda AK, Lohiya NK, Gupta JC (2011) Mycobacterium indicus pranii is a potent immunomodulator for a recombinant vaccine against human chorionic gonadotropin. Journal of Reproductive Immunology 91, 24-30.
| Crossref | Google Scholar | PubMed |
Romanowski R, Culbert R, Alkemade S, Medellin-Peña MJ, Bugarski D, Milovanovic A, Nesic S, Masic A (2017) Mycobacterium cell wall fraction immunostimulant (AMPLIMUNE™) efficacy in the reduction of the severity of ETEC induced diarrhea in neonatal calves. Acta Veterinaria 67, 222-237.
| Crossref | Google Scholar |
Stils HF, Jr. (2005) Adjuvants and antibody production: dispelling the myths associated with Freund’s complete and other adjuvants. ILAR Journal 46, 280-293.
| Crossref | Google Scholar |
Taylor JD, Fulton RW, Lehenbauer TW, Step DL, Confer AW (2010) The epidemiology of bovine respiratory disease: what is the evidence for predisposing factors? The Canadian Veterinary Journal = La Revue Veterinaire Canadienne 51, 1095-1102.
| Google Scholar | PubMed |
Wheat W, Chow L, Rozo V, Herman J, Still Brooks K, Colbath A, Hunter R, Dow S (2020) Non-specific protection from respiratory tract infections in cattle generated by intranasal administration of an innate immune stimulant. PLoS ONE 15, e0235422.
| Crossref | Google Scholar | PubMed |
Woolums AR, Siger L, Johnson S, Gallo G, Conlon J (2003) Rapid onset of protection following vaccination of calves with multivalent vaccines containing modified-live or modified-live and killed BHV-1 is associated with virus-specific interferon gamma production. Vaccine 21, 1158-1164.
| Crossref | Google Scholar | PubMed |
Zimmermann P, Curtis N (2018) The influence of BCG on vaccine responses – a systematic review. Expert Review of Vaccines 17, 547-554.
| Crossref | Google Scholar | PubMed |
Zimmermann P, Donath S, Perrett KP, Messina NL, Ritz N, Netea MG, Flanagan KL, van der Klis FRM, Curtis N (2019) The influence of neonatal Bacille Calmette-Guérin (BCG) immunisation on heterologous vaccine responses in infants. Vaccine 37, 3735-3744.
| Crossref | Google Scholar | PubMed |