Market-driven assessment of alternate aquafeed ingredients: seafood waste transformation as a case study
Janet Howieson


A School of Molecular and Life Sciences, Curtin University, Kent Street, Bentley, WA 6102, Australia.
B Department of Primary Industries and Regional Development, Fleet Street, Fremantle, WA 6160, Australia.
Abstract
With the increase in worldwide demand for seafood, the current plateau in production from wild-harvest fisheries has resulted in the rapid growth of the aquaculture sector. Aquaculture relies on quality ingredients such as fishmeal, but cost concerns have led to the investigation of a variety of alternate plant and animal by-products and microbial sources as aquafeed ingredients. Evaluation of alternative aquafeed has traditionally focused on their effects on the growth and immune status of the fish and not always on market-driven assessments of the final edible product. One of the commonly researched groups of alternative ingredients is seafood waste, which, after transformation, has potentially beneficial nutritional characteristics. Transformation, which includes rendering, enzyme hydrolysis and use as a feed source for insects and microbial species, is intended to provide stability and enhance the logistical feasibility of the waste as an aquafeed ingredient. This review discusses transformed fish waste in aquafeeds and describes some of the market and end-user implications (composition, edible safety and quality, sustainability metrics and consumer perceptions) of this approach.
Keywords: circular aquaculture, fight food waste, food processing waste, food science, full utilisation, functional additives, greenhouse gas, insect, seafood waste, single cell protein, sustainable aquaculture, upcycling.
Introduction
The contemporary drive to minimise or transform harvest and processing waste from primary production has been applied to the seafood industry (Venugopal 2021). This paper discusses the use of transformed fish-waste ingredients in aquafeeds and questions the neglect of some of the market and end-user implications (safety, quality, sustainability metrics and consumer perceptions) of including some of these ingredients.
In human nutrition, fish is an important source of protein and lipids, particularly omega-3 polyunsaturated fatty acids (PUFA), vitamins and trace elements. Regular consumption of fish at least once a week is recommended (İbrahim Halilogˇlu et al. 2004; Codabaccus et al. 2013; Han et al. 2018; Quiñones et al. 2021; Xu et al. 2021). The increase in worldwide demand for seafood, and the current plateau in production from wild-harvest fisheries have resulted in the rapid growth of the aquaculture sector. This is now estimated to provide 70% of edible seafood (FAO 2020a).
The aquaculture industry relies on quality aquafeed ingredients. Fishmeal (FM) produced from wild-harvested fish is still considered the best aquafeed protein source due to its favourable nutritional characteristics, excellent palatability and digestibility (FAO 2020b). However, the use of FM and the broadening of the gap between demand and supply has resulted in extensive investigations of protein alternatives to FM in aquafeed (Siddik et al. 2018a; FAO 2020b).
Plant-based raw materials have been investigated and are included in most modern commercial aquafeed (see review by Colombo et al. (2022)). However, the utilisation of conventional plant-based protein for finfish aquaculture, particularly for carnivorous species, faces a number of challenges. These include an imbalanced amino acid profile and antinutritional factors that can affect the growth, feed utilisation, digestibility and overall health of fish that consume them (Francis et al. 2001; Van Vo et al. 2020; Colombo et al. 2022). Further, together with direct competition with human food streams, utilising terrestrial crops in aquafeeds has sustainability implications, including access to freshwater, deforestation and other types of habitat modification, arboreal footprint, pesticide and fertiliser use, and nutrient run-off leading to aquatic pollution (Colombo and Turchini 2021). Various proteins derived from single-cell organisms, grown on the nutrient-rich waste streams, as next-generation protein sources in aquafeed opportunities, have also been investigated as alternate aquafeed ingredients, but are not at a viable scale of production yet (Hua et al. 2019).
Animal by-products (e.g. poultry by-product meal (PBM; Galkanda-Arachchige et al. 2020), insects (see review by Alfiko et al. 2022) and microbial single-cell protein (such as yeast and bacteria; see review by Jannathulla et al. 2021) have also been investigated to replace FM. Although these products are considered a good source of protein, their application in aquafeeds is still constrained by factors including the lack of some essential amino acids, high moisture, indigestible particles, microbial contaminants and the possibility of disease transmission (Siddik et al. 2019; Chaklader et al. 2020a). Aquafeed ingredients formulated from seafood waste are an alternative aquafeed opportunity and are the predominant focus of this review.
Alternate aquafeed ingredients: food and consumer science as a missing research link
Aquafeed studies using alternate ingredients have focused on technical parameters associated with the predicted growth and feed-use efficiency of the target species (such as target weight, and specific growth rate), as well as feed digestibility and palatability. Compositional considerations such as proximate, amino acid and fatty acid composition and the presence of anti-nutritional compounds have been paramount. Studies have also reported on the health and immune status of the fed fish (such as histological, blood biochemistry and gut microbiome results; Chaklader et al. 2021a; Jannathulla et al. 2021; Alfiko et al. 2022; Aragão et al. 2022; Chaklader et al. 2023a). Of equal importance is the consideration of operational/supply parameters for the inclusion of novel ingredients, such as their consistency of quality, cost, transport logistics and scale of production or supply (Hua et al. 2019). More recently, sustainability assessments such as greenhouse-gas (GHG) emissions and circularity have also become a focus (Colombo et al. 2022).
The interdisciplinary approach of measuring edible food safety and the food quality of the aquaculture product (in addition to the above parameters) has often been neglected when experimenting with alternate aquafeed formulations (Chaklader 2021). This oversight is in contrast to other animal production industries where the analyses of feed types in relation to the final edible meat safety and quality are more advanced (Costa et al. 2021).
This lack of focus on food science is surprising, as not only does food science research address the fundamental purpose of aquaculture (to ensure stable and reliable sources of food supply) by evaluating the safety of end products, but it also assists in maintaining or improving the quality of farmed fish, to ensure that products remain competitive in the market (Floros et al. 2010; Calanche et al. 2020; Tacon et al. 2020).
Food science and aquaculture
Compositional analyses of food allow for precise nutritional labelling and can lead to putative health claims. It is therefore noteworthy that the composition of seafood species grown with alternate ingredients is often provided, and has been shown to be affected by diet (Olsen et al. 2004; Mai et al. 2006; Moren et al. 2006; Olsen et al. 2006; El-Rahman and Badrawy 2007; Abdul Kader et al. 2011; Kader and Koshio 2012; Friesen et al. 2013; Gause and Trushenski 2013; Waagbø et al. 2013; Sprague et al. 2015; Emery et al. 2016; Wong et al. 2016; Kim et al. 2019; Monge-Ortiz et al. 2020; Chaklader et al. 2022, 2023b). This alteration of composition, particularly evident in fatty acid content, has consequences for human nutrition (Blondeau et al. 2015; Mensink 2016; Michielsen et al. 2019). Further, this compositional variation will affect a range of food science perspectives, including nutritional messaging and labelling, food safety, quality, and shelf-life (Table 1). Thus, the broader picture of compositional variation should be considered when evaluating novel aquafeed ingredients (Hixson 2014). Lastly, when undertaken, compositional analysis is often completed on the whole fish (including viscera, skin and head) rather than on the edible portion (e.g. fillet), necessitating a secondary analysis relevant to the latter.
Fish species | Alternative feed ingredients (FM replacement %) | Analysed portion | Analysed heavy metal | Outcomes | References | |
---|---|---|---|---|---|---|
Red sea bream (Pagrus major) | Fermented soybean and scallop by-product (0–60%) | Whole fish | Cd, Pb | ASignificant increase in Cd and Pb in fish after fed for 45 days, with Cd significantly higher in the diet and Pb being insignificantly different from test diet | Abdul Kader et al. (2011) | |
Red sea bream (P. major) | Mixture of fish solubles, fermented soybean and squid by-product (0–100%) | Fillet | Cd, Pb | Significant increase in Cd in diet (0.58 mg/kg vs 2.34 mg/kg); no Cd or Pb was detected in final fillet | Kader and Koshio (2012) | |
Atlantic cod (Gadus morhua) | Antartic krill (Euphausia superba; 0–100%) | Fillet | As, Cd, Hg, Pb | Higher Cd concentration (0.61 mg/kg vs 0.19 mg/kg) in test diet than in control diet; no significant differences in final fillets. | Moren et al. (2006) | |
Atlantic salmon (Salmo salar) | Artic krill (Thysanoessa inermis) or amphipod (Themisto libellula; 0–40%) | Fillet | As, Cd, Hg, Pb | Higher Cd (1.4 mg/kg, 12 mg/kg vs 0.19 mg/kg) and Pb (0.22 mg/kg, 0.16 mg/kg vs 0.09 mg/kg) concentration in artic krill and amphipod respectively, than in test diet; no significant differences were found in final fillet | Moren et al. (2006) | |
Japanese seabass (Lateolabrax japanicus) | Squid viscera (6–16%) | Fillet and internal organs | Cd | Up to 27.5 (0.21 mg/kg vs 12.08 mg/kg) times higher in Cd concentration when comparing test diet with control, but no Cd was detected in muscle tissue. Significant increases were found in gill, liver, and kidney | Mai et al. (2006) | |
Nile tilapia (Procambarus clarkia) | Crayfish (Procambarus clarkia), crayfish by-product (0–50%) or treated crayfish (0–100%) | Fillet | Cd, Pb | ASignificant increase in Pb and Cd on fish muscle when fed with 50% crayfish by-product meal (1.60 mg/kg vs 0.40 mg/kg; 0.206 vs 0.141 mg/kg respectively). | El-Rahman and Badrawy (2007) | |
Nile tilapia (P. clarkia) | Tuna by-product (40–70%) | Fillet, internal organs, and whole body | Cd, Hg | ASignificant increase in Hg in muscle portion when comparing 40–70% replacement diet regardless of fish weight. Increase in muscle weight also increased concentration of Hg in fillet. Cd content increased significantly in muscle when fish was over 500 g. Accumulation of Hg and Cd in internal organs increased significantly in 70% replacement, regardless of fish weight. | Kim et al. (2019) | |
Mediterranean yellowtail (Seriola dumerili) | Mixture of corn gluten, krill, meat and bone (33–66%) | Fillet | As, Cd, Hg | Significant reduction of As content compared with FM diet (1.4 mg/kg vs 0.81 mg/kg); insignificant differences in Cd and Hg. | Monge-Ortiz et al. (2020) |
ASignificant increases in heavy metal accumulation were identified in final product.
Safe seafood and quality seafood
In terms of food science, the production of safe food is of primary importance, as it directly affects consumer health (Tritscher et al. 2013; Wong et al. 2016). Food safety can be divided into two components, namely, chemical and microbiological safety. In aquacultured seafood, the microbiological risk is mostly introduced post-harvest (during processing). Therefore, for alternate aquafeed investigations, the chemical safety of the final aquaculture products, specifically heavy metals and persistent organic pollutants (Fernandes et al. 2018; Sheng and Wang 2021), is the main focus. Levels of these compounds in the edible products are variously regulated in seafood safety legislation.
In addition to food safety, the quality of seafood is a complex topic and covers a wide range of end user-driven definitions, including nutritional, microbiological, sensory and physicochemical characteristics (Nielsen et al. 2002). In the field of food science, the quality of fish is often analysed as its perceived ‘eating quality’ and ‘freshness’, which can influence end-user demand, and hence competitiveness of products on the market (Nielsen et al. 2002).
The conventional techniques to determine the quality of seafood can be divided into two types, quantitative human sensory evaluation and instrumental analytical determination. The human sensory analyses include appearance, colour, texture, odour and taste (Nielsen et al. 2002; Wu et al. 2019). The analytical attributes include pH, total volatile base-nitrogen (TVB-N) (protein deterioration) and thiobarbituric acid-reactive substances (TBARS, lipid oxidation; Olafsdóttir et al. 1997; Aro et al. 2003; Grigorakis et al. 2004; Olafsdottir et al. 2004; Iglesias et al. 2009; Yao et al. 2011; Itoh et al. 2013; Cheng et al. 2015; Chung et al. 2021; Chaklader et al. 2022, 2023b). When sensory and instrumental analytes and acceptable limits are combined with microbiological assessment, then the shelf-life of the target products can be estimated. All these quality analyses will affect the consumer experience, from purchase to consumption (Nielsen et al. 2002; Wu et al. 2019).
Consumer science in relation to the perceptions and acceptance of new aquafeed ingredients on purchasing behaviour should also be considered, understood and addressed as part of any multi-disciplinary approach to new aquafeed ingredient investigations (Chaklader 2021; Colombo et al. 2022). It is paramount to ensure that human sensory quality is the same or better than for traditionally cultured fish, as appearance is one of the characteristics used for decision-making by the purchaser (Colombo et al. 2022).
Transformed fish-processing waste is now being considered as an alternate aquafeed ingredient and can partially replace FM, or alternatively be a supplement-fed in conjunction with other plant-, animal-, or microbial-based ingredients. Feeds developed from transformed fish waste have the advantage of delivering essential nutrients (e.g. amino acid and fatty acid, vitamins, macro-minerals and trace elements) that may be lacking in other (non-FM) alternate formulations, as well as improving palatability (Colombo et al. 2022). Depending on inclusion rates, such fish-based ingredients should produce an edible food product with compositional characteristics aligned to those produced from FM (Colombo et al. 2022). Incorporation of fish waste into aquafeeds would also better meet the sustainable development goals aligned to reduced food loss, and thus increase the opportunity for circularity in the aquaculture industry (Colombo et al. 2022; UN 2023; Fig. 1).
Schematic representation of seafood-waste transformation into various next-generation alternative protein sources and sustainable additives for future aquafeed (aquafeed 3.0). This is proposed to overcome several feed constraints generated by conventional aquaculture and modern aquaculture (aquafeed 2.0). The idea was adapted from the study of Colombo et al. (2022) and Venugopal (2022).
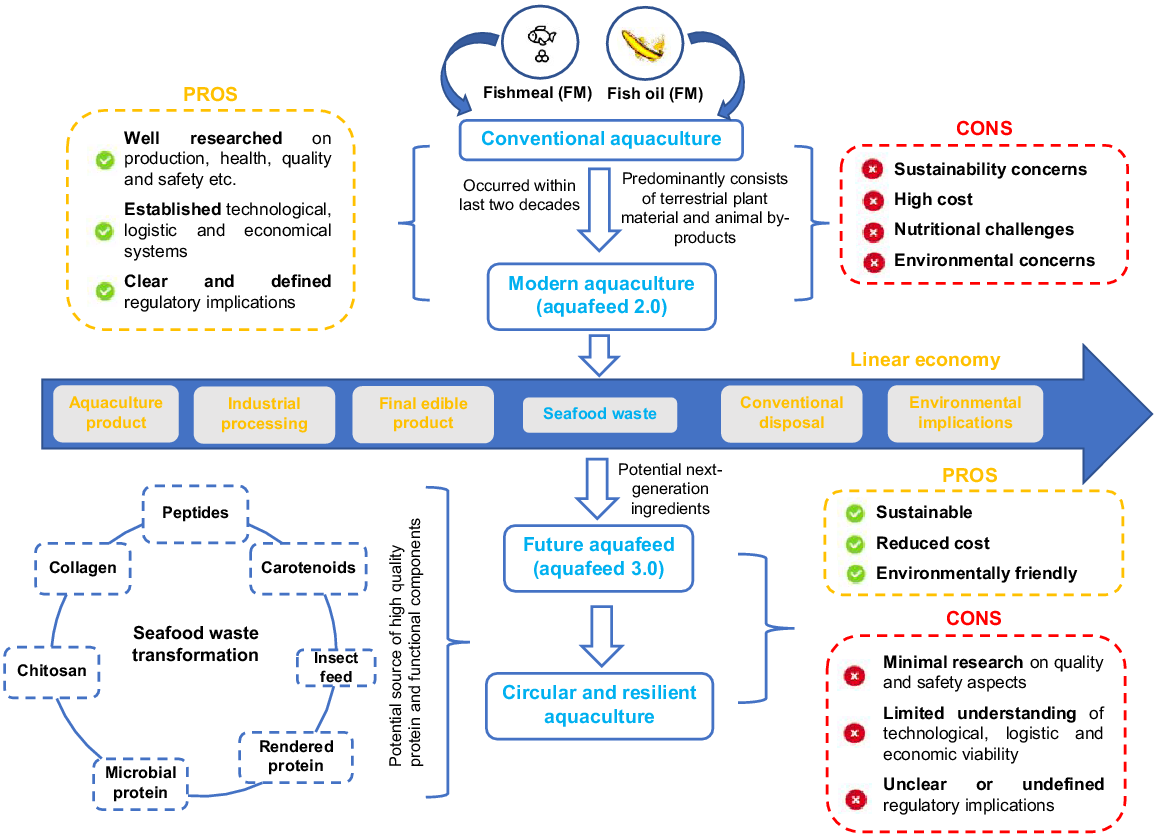
In Australia, it is estimated that between 50 000 (Arcadis 2019) and 100 000 t of waste (Dundas-Smith and Huggan 2006) are produced by seafood industries every year, costing an estimated AUD15 million per annum for disposal (He et al. 2013). An estimated 50 000 t per annum is generated at the manufacturing stage (from whole fish to fillets; Arcadis 2019), as approximately 60% of the fish is discarded during filleting (Chalamaiah et al. 2012). Waste, includes skin, heads, muscle, viscera, liver, and bones. Internationally, it is estimated that ~40% of the total seafood supply is wasted among harvesting, production and processing (Love et al. 2015; Laso et al. 2016) and, in Europe, it is estimated that seafood losses and wastage rates are greater than 30% (FAO 2012). In consideration of fish waste at the harvest stage, the terms ‘bycatch’ and ‘discards’ are applied where the undersized, low-valued or unintentional catch are caught or discarded while fishing for target species. While regulations are not yet implemented in Australia, the EU ‘Landing Obligation (Common Fishery Policy (Regulation EU No. 1380/2013)’ (European Union 2013) now prevents discard from the vessel, and hence profitable use of the bycatch is under greater consideration (Colombo et al. 2022).
Aside from aquafeed ingredients, fish and seafood wastes have been widely researched for a variety of outcomes, including fertiliser, pet food, edible food, nutraceuticals and supplement products (Venugopal 2021; Nag et al. 2022). The main limitation to the effective use of fish waste is predominantly perishability, resulting in reduced product quality and consistency. This is partly due to the disparate locations of the low volumes of fish waste, affecting the ability to reach an economic scale sufficient for processing and transportation. The lack of suitable-scale infrastructure has also been identified as a barrier (Hua et al. 2019). Hence, while there is some work on the addition of untreated fish waste to small-scale aquaculture operations (Bechtel 2007), transforming the perishable raw seafood material into high-quality stable products, and their transport logistics, has been the focus of recent research efforts (Arulkumar et al. 2018; Lerfall et al. 2018; Olatunde and Benjakul 2018a, 2018b; Lambrianidi et al. 2019; Zhaleh et al. 2019; Baptista et al. 2020; Castro et al. 2020; Shokri et al. 2020; Ucar et al. 2020; Hoque et al. 2022).
This article will focus on three types of transformational processes for fish waste, and opportunities for the extraction of specific supplemental compounds. We will, first, briefly consider the use of these ingredients in aquaculture nutrition, with reference to the growth and immune status of the fed fish. Where data are available, we will discuss the impact of the alternate feed ingredients on food safety and the quality of the final edible product. Sustainability and circularity considerations will follow as well as a discussion of limitations and barriers to widespread commercial implementation.
Rendering refers to the drying of seafood by-products. Rendered by-product usually has lower protein and higher ash concentration than does high-quality FM derived from whole fish, which contains 66–74% crude protein, 8–11% crude lipids, and <12% ash (Hua et al. 2019). In comparison, white fishmeal produced from rendered by-products contains 60–67% crude protein, 7–11% crude lipids, and 21–23% ash, and tuna fishmeal produced from rendered by-products contains 57–60% crude protein, 8–14% fat, and 12–21% ash (Goddard et al. 2008; Hernández et al. 2014; Jeon et al. 2014; Kim et al. 2014; Ween et al. 2017; Hua et al. 2019). The lower protein and higher ash content in by-product FM are not unexpected, as the nutrient composition differs among whole fish, fillets, and other parts of the body (viscera, heads, skin, bones, and blood). The different proportions of variously rendered by-products that are added to fishmeal will therefore also contribute to the nutrient variability of the feed (Hua et al. 2019)
Nonetheless, rendered by-products are currently added to aquafeed as a partial replacement for FM, without apparent compromise. For example, growth was maintained at replacement rates of 15.8–21.4% in spotted rose snapper (Lutjanus guttatus), and up to 30% in olive flounder (Paralichthys olivaceus) at a dietary inclusion rate of 21%. For Korean rockfish (Sebastes schlegeli), 75% of FM could be substituted by tuna by-product meal at a dietary inclusion rate of 58.1%, without compromising growth and feed utilisation (see summary by Hua et al. (2019)).
The process of hydrolysis converts fish waste to fish protein hydrolysate (FPH) in liquid or dried form. There are several hydrolysis methods including chemical hydrolysis (acid and alkaline hydrolysis), autolysis, bacterial fermentation and enzymatic hydrolysis (Siddik et al. 2021). Enzymatic hydrolysis is widely implemented to produce precise hydrolysates that retain the nutritive value of the source protein (Zamora-Sillero et al. 2018). Enzyme hydrolysis targets specific peptide bonds and amino acids. It produces a consistent-quality product, while excluding any residual organic solvents or toxic chemicals in the end-products (Najafian and Babji 2012). Product stability in storage and transport can also be optimised (Siddik et al. 2021).
An extensive review by Siddik et al. (2021) summarised enzyme hydrolysis from fish-processing waste in a wide range of seafood species and by-product raw materials, including skin, heads, muscle, viscera, liver and bones. FPH products were reported to be a good source of protein, peptides, and amino acids. The process of hydrolysis results in the breakdown of larger protein molecules into smaller, more bioactive compounds, and hence FPH has been reported to possess desirable functional and bioactive peptides. A moderate inclusion of FPH in aquafeeds has the potential to improve growth, feed utilisation, immune response and disease resistance of a wide range of fish (see review by Siddik et al. (2021)).
Our laboratory conducted several studies to convert Australian seafood-production waste, including tuna (Thunnus maccoyii) and kingfish (Seriola lalandi), to FPH, so as to assess their subsequent utilisation in aquafeed. We reported that the replacement of FM with >20% tuna hydrolysates negatively affected the welfare of barramundi (Lates calcarifer). The hydrolysates were rich in free amino acids, and reported to act as anti-nutritional factors (Siddik et al. 2018b). However, a 56-day feeding study that supplemented 5–20% of tuna hydrolysates against a reference diet (FM-based) indicated that 5% and 10% supplementation improved the growth of barramundi, improved haematology indicators, gut mucosal barrier function, immune response and disease resistance against Streptococcus iniae (Siddik et al. 2018a). The beneficial effects of tuna hydrolysates shown by this study motivated us to supplement a low-quality protein ingredient (poultry by-product meal) with 5% and 10% tuna and kingfish hydrolysates (Chaklader et al. 2020b). We found that these hydrolysates when added to poultry-by product meal could completely replace FM, with a significant improvement in feed utilisation, growth, mucosal barrier function and immunity in barramundi, compared with an FM-only diet. Importantly, supplementation with 10% tuna hydrolysate enhanced the gut microbial diversity, along with a positive influence on beneficial bacteria (Siddik et al. 2020).
Similarly, several protein hydrolysates from seafood waste have recently been tested on other aquaculture species (Chaklader et al. 2020b). One study found that supplementation of plant protein with 10% whole blue whiting (Micromesistius poutassou) hydrolysate allowed higher inclusion of plant protein into the diet of Atlantic salmon (Salmo salar) and increased the growth rate relative to controls (Egerton et al. 2020). The authors also found an improved essential amino acid bioavailability in the blood of the same dietary groups. In a further study, a fishmeal-based diet top-coated with 2% tuna hydrolysate improved the digestibility, immune responses and disease resistance of red sea bream (Pagrus major) and olive flounder (P. olivaceus) against Edwardsiella tarda (Khosravi et al. 2015).
Although a number of different insect species have been investigated for aquafeed ingredients (see review by Alfiko et al. (2022), black soldier fly (Hermetia Illuscens) larvae (BSFL) is considered the most promising owing to its amino acid composition (Hua et al. 2019)). This insect species possesses the capacity to valorise low-value organic waste or by-products into highly nutritious biomass while requiring less arable land and water consumption, as well as reduced CO2 production (Henry et al. 2015). The final biomass contains high protein and lipid but this may vary depending on the substrate and processing method (Wang et al. 2019).
A number of studies have investigated BSFL in aquafeed across a range of fish species and inclusion rates. However, in most of these studies, the growth on BSFL substrates was not well articulated. The main disadvantage of BSFL incorporated directly into aquafeed is the presence of high amounts of saturated fatty acids and a negligible amount of some essential polyunsaturated fatty acids (Alfiko et al. 2022). In our work, we initially demonstrated that the fatty acid profile of the BSFL could be manipulated using fish waste from carp as the growth substrate (Tilley et al. 2019). In our following studies, BSFL meal grown on 70% carp waste and 30% agriculture waste was mixed with poultry by-product meal (PBM). Results indicated that adding 10–30% BSFL meal to PBM could replace FM completely in the barramundi diet (Chaklader et al. 2019; Chaklader et al. 2020c; Chaklader et al. 2021a, 2021b). We reported that by feeding BSFL on fish waste the lipid profile of the ensuing larval meal was optimised for aquafeed (Tilley et al. 2019). Some defatting further improved the value of BSFL meal in aquafeed formulations.
There are several single-celled organisms, including marine protists such as Aurantiochytrium, Schizochytrium and Thraustochytrium; methanotrophic bacteria such as Methylobacterium and Methylococcus; chemotrophic proteobacteria such as Clostridium and Baccillus; yeasts such as Candida, Cyberlindnera, Kluyveromyces, Rhodotorula, Saccharomyces and Wickerhamomyces that have been tested for use as aquafeed single-cell ingredients (Colombo et al. 2022). Many of these studies have identified the potential to cultivate these microorganisms in seafood- and aquaculture-processing wastes. Even though the industry is still in its infancy, 20 major producers of microalgae and cyanobacteria, and 16 major producers of protists, yeasts and bacteria have already been identified by a recent industry report (Krishfield et al. 2019). A recent review comprehensively illustrated the potential of using single-cell ingredients as next-generation sources of protein and/or lipid in fish and shrimp aquafeeds (Colombo et al. 2022).
Microalgae such as Chlorella spp., Spirulina spp., Dunaliella spp., diatoms, and cyanobacteria, are promising agents for the bioconversion of seafood waste. Their digestive actions allow the degradation of organic contents, unused food, and excretory products, together with the removal of CO2, NH3-N, CO2, and H2S, thereby ameliorating environmental pollution (Puyol et al. 2017; Gifuni et al. 2019). The algal biomass contains high protein (60%) and oil (75% with high n-3 PUFA contents) and is also a good source of polysaccharides, minerals, and pigments including chlorophylls, carotenoids, and phycobiliproteins (Stengel and Connan 2015; Venugopal 2021). These nutrient and functional molecules have motivated the use of microalgae as a source of bioactive peptides, animal feeds, food additives, and as probiotics in aquaculture, as illustrated in the reviews of Venugopal (2021). Further, several aerobic, anaerobic, or facultative microbes could be used to detach food components by various microbial fermentation techniques including solid-state, liquid-state, or submerged-state fermentation, environmentally friendly, safe, and cost-effective techniques (Nag et al. 2022). Small food components derived from fermentation or fermented product could be used as functional ingredients in aquafeed formulation (see the review of Nag et al. (2022)).
Chemical contaminants that can accumulate in fish via feed include a wide range of persistent toxic substances (PTS) such as inorganic heavy metals, and persistent organic pollutants (POPs) (Karl et al. 2003; Lundebye et al. 2004; Tritscher et al. 2013; Hixson 2014; Wong et al. 2016). In fish fed with alternative non-plant-based ingredients, the majority of the published research has focused on four main elements, namely, cadmium (Cd), lead (Pb), arsenic (As) and mercury (Hg) (Table 1).
In a summary of studies involving the use of seafood by-products originating from either scallops, crayfish or tuna as a FM replacement, approximately 38% identified heavy metal contamination in the final fish products (El-Rahman and Badrawy 2007; Abdul Kader et al. 2011; Kim et al. 2019). Drilling down further into these data showed that accumulation was related to the portions analysed and the fish species. In one of the heavy metal studies that identified a significant increase in the Cd and Pb content, the authors suggested that the results were most likely to be due to the inclusion of waste internal organs in the analysis (Abdul Kader et al. 2011). It is well known that heavy metal accumulation from feed mostly occurs in the viscera of the fish, especially in the liver and kidney, while in comparison, the muscle portion of the fish is unaffected (Berntssen et al. 2000; Mai et al. 2006; Abdul Kader et al. 2011; Paschoalini and Bazzoli 2021). In the study conducted by Mai et al. (2006), despite a 27.5 times higher Cd concentration in the test diet than in the control (12.08 mg/kg, 0.21 mg/kg respectively), no Cd increase was detected in the fillet.
However, in another study, Nile tilapia (Oreochromis niloticus) accumulated heavy metals in the fillets. It is uncertain what caused this anomaly, since various factors including biological and water parameters could all affect the susceptibility of fish towards metal accumulation (Jezierska and Witeska 2006; Ali and Khan 2018; Paschoalini and Bazzoli 2021).
A feature of the academic literatures on aquaculture feed studies, where rendered by-products and FPH have been examined as an alternate ingredient, is the paucity of information on the food safety and quality of the edible product. However, we do note that a broader examination of various animal by-products included in feed (e.g. PBM; blood and bone meal) indicated no detrimental impacts on final fillet quality (Williams et al. 2003; Chaklader et al. 2021c) (Table 2).
Fish species | Alternative feed ingredients (FM replacement %) | Type of methods | Investigated parameters | Outcomes | References | |
---|---|---|---|---|---|---|
Mediterranean yellowtail (S. dumerili) | Mixture of corn gluten, krill, meat and bone (33–66%) | Analytical and sensory evaluation | Analytical: colour, moisture, pH, texture | ASignificant changes in colour, especially increase brightness ASignificant reduction in adhesiveness, chewiness, gumminess, and hardness | Monge-Ortiz et al. (2020) | |
Sensory: appearance, odour, colour, texture taste | ASignificant in marine aroma, whiteness and water retention were detected by sensory panel | |||||
Japanese seabass (Lateolabrax japonicus) | Mixture of poultry by-product meal, meat and bone meal, spray-dried blood meal and hydrolysed feather meal in 40:35:20:5 ratio (20–80%) | Analytical and sensory evaluation | Analytical: texture | ASignificant increase in hardness, chewiness, cohesiveness, and reduction in adhesiveness on fish fed with test diet | Hu et al. (2013) | |
Sensory: appearance, odour, colour, texture, taste, overall preference | AConsumer significantly favour fish fed with control diet | |||||
Barramundi (Lates calcarifer) | Mixture of poultry by-product meal and black soldier fly larvae (Hermetia illucens) (100% in 85:15, 80:20, 75:25) | Analytical and sensory evaluation | Analytical: drip loss, colour, texture, structural changes, Ph, lipid oxidation | ASignificant increase in brightness and yellowness in fish fed with test diet ATest diet suppressed rancidity of fish during storage | Chaklader et al. (2022) | |
Sensory: quality index evaluation, appearance, odour, texture, taste, overall preference | AConsumer significantly prefered fish fed with test diets | |||||
Barramundi (L. calcarifer) | Mixture of poultry by-product meal and defatted or full-fat black soldier full larva (Hermetia illucens) (100% in 70:30) | Analytical and sensory evaluation | Analytical: drip loss, colour, texture, structural changes, Ph, lipid oxidation | ADefatted diet improved the texture profile of fish ASignificant increase in brightness in fish fed with test diet AIncrease fish resistance to degradation during storage | Chaklader et al. (2023a) | |
Sensory: quality index evaluation, appearance, odour, texture, taste, overall preference | AConsumer significantly prefer fish fed with test diets AIncrease fish resistance to degradation during storage | |||||
Atlantic salmon (S. salar) | Black soldier fly larvae (25–100%) | Sensory evaluation | Sensory: taste, texture | No significant differences were identified | Lock et al. (2016) | |
Atlantic salmon (S. salar) | Black soldier fly larvae (0–100%) | Sensory evaluation | Sensory: appearance, odour, colour, texture, taste | ASignificant increase in rancid odour and off-odour of fish fed with test diet ASignificant reduction in colour intensity of cooked salmon in fish fed with test diet ASignificant softer in raw salmon, significant harder in cooked salmon in fish fed with test diet | Belghit et al. (2019) | |
Rainbow Trout (O. mykiss) | Black soldier fly larvae (25–50%) | Sensory evaluation | Sensory: differences test | No significant differences were identified | Sealey et al. (2011) | |
Rainbow Trout (O. mykiss) | Mixture of poultry by-product, blood and feather meal (0–100% in 27:5:5, 25:3:3) | Sensory evaluation | Sensory: preference, odour, taste, texture | ASignificant increase in grassy flavour and softness of fish ASignificant reduction in fish aroma | Craft et al. (2016) | |
Rainbow Trout (O. mykiss) | Black soldier fly larvae (25–50%) | Analytical and sensory evaluation | Analytical: texture | No differences in shear force | Borgogno et al. (2017) | |
Sensory: description, colour, odour, texture, taste | ASignificant reduction in overall aroma and increase in overall flavour ASignificant improvement in texture, including juiciness and tenderness | |||||
Gilthead sea bream (Sparus aurata) | Blood or haemoglobin meal (0–10%) | Analytical and sensory evaluation | Analytical: colour | No differences in colour | Martínez-Llorens et al. (2008) | |
Sensory: differences test | ASignificant differences were detected in all test diets beside 5% blood meal | |||||
Pacú (Piaractus mesopotamicus) | Bovine plasma protein concentrate (0–100%) | Analytical and sensory evaluation | Analytical: texture, colour | ASignificant increase in hardness and chewiness | Pavón et al. (2018) | |
Sensory: colour, odour, taste, texture | ASignificant increase oily mouthfeel, flavour intensity, foreign flavour, firmness and chewiness | |||||
Hybrid striped seabass (white bass Morone chrysops × striped bass M. saxatilis) | Poultry by-product meal (~6%) | Analytical and sensory evaluation | Analytical: colour | No differences in colour | Turek et al. (2020) | |
Sensory: differences test | No differences compare with control diet | |||||
Atlantic salmon (S. salar) | Poultry by-product oil (80%) or mixture of 1:1 tallow and poultry by-product oil (80%) | Sensory evaluation | Sensory: preference, taste | No differences between both diets | Mai et al. (2006) | |
Brown trout (Salmo trutta L.) | Poultry fat or pork lard (0–100%) | Sensory evaluation | Sensory: odour, colour, texture, taste | No differences between both diets | Turchini et al. (2003) |
ASignificant changes in physicochemical attributes were identified in final products.
In a promising outcome, BSFL meal was shown to improve final fillet quality in rainbow trout (Oncorhynchus mykiss; Borgogno et al. 2017) and barramundi (L. calcarifer; Chaklader et al. 2022, 2023b). In these three studies, an improvement in flavour and texture, especially juiciness, was reported. As well, a reduction in colour intensity was observed in fish fed with BSFL (Belghit et al. 2019; Chaklader et al. 2022, 2023b). While a lighter colour is preferred in white-fleshed fish such as barramundi, it might not be preferable in coloured-flesh fish such as salmon (Alfnes et al. 2006; Chaklader et al. 2022, 2023b). This study also showed improved sensory quality and lipid oxidation in the BSFL-fed fillets in an 8-day shelf-life experiment. It is noteworthy that in the studies by Chaklader et al. (2022, 2023b), BSFL was produced partially on fish waste; however, the food source was not clear in other studies.
These reports underpin the acceptance of seafood waste as a substrate to enrich PUFA in BSFL (one of the limiting factors in BSFL meal in aquadiets). In the contradictory results reported by Belghit et al. (2019), the inclusion of BSFL caused a significant increase in undesirable flavours. This may have been due to the growth substrate (which was not clearly articulated). Further studies are needed to produce BSFL by using seafood waste and other food waste as substrates, with their subsequent utilisation in aquafeed in a commercially relevant trial to confirm the potential of BSFL to improve the final product quality in aquaculture production.
Functional ingredients extracted from fish waste and added to aquafeed can result in an extension to shelf-life (Fig. 1). A recent review examined the potential of FPHs as edible coatings to preserve food (Tkaczewska 2020). Protein hydrolysates embedded into edible packaging effectively inhibited pathogenic microorganisms and lipid oxidation in fish products (Tkaczewska 2020). As noted previously, there is a paucity of data on the shelf-life and quality of aquacultured fillets produced with FPH supplementation. It might also be possible that FPH addition to aquafeed results in the assimilation of bioactive peptides not only to enhance growth performance and immune status in the target species but also as a means to improve product quality.
Carotenoids such as astaxanthin (commonly used in salmon aquaculture) can be obtained from the discards of crab, salmon, and prawn processing (Nag et al. 2022). Carotenoids are biomacromolecules possessing antioxidant potential associated with lipid peroxidation (Nag et al. 2022). The contemporary assimilation of carotenoids via an aquaculture diet may also play a role in elevating the shelf-life of aquaculture products (Nag et al. 2022). Similarly, chitin is present in discarded components of seafood and may be partially deacetylated by enzymatic hydrolysis to chitosan (Pati et al. 2020; Pati et al. 2021). In alkaline conditions, or under the influence of a chitin deacetylase, it exhibits antimicrobial, antioxidant, and antiviral properties (Li et al. 2010). This has motivated researchers to examine chitosan as a coating to extend the shelf-life of fish products (and other animal products), as detailed in the review of Kumar et al. (2020) and Socaciu et al. (2018). Chitosan supplementation as an antioxidant, growth promoter and immunostimulant in aquatic animals has been reviewed by Abdel-Ghany and Salem (2020); however, the effect on aquaculture product quality is unknown.
Contemporary markets also assess sustainability credentials. In assessing GHG emissions associated with fish waste use in aquaculture, there is a separation required between
the GHG emissions associated with current outcomes for waste production in the seafood supply chain, and
the GHG emissions associated with the conversion of seafood waste to a specific aquafeed ingredient.
There is also a growing impetus to understand the GHG emission variation for the final edible product, including the impact of different feed formulations. This last separation is not covered in this review as it has been the subject of multiple other reviews and reports (Hua 2021; Ruiz-Salmón et al. 2021; Blueshift Consulting 2022; Ziegler et al. 2022).
In the context of sustainability within seafood supply chains, there are some advantages to utilising fish waste (Murali et al. 2021). GHG emissions (carbon dioxide equivalent (CO2 eq) from two Western Australian finfish supply chains, from harvest to retail outlet, were assessed using streamlined life-cycle methodology and cleaner production strategies (Denham et al. 2016). Electricity consumption contributed to the highest GHG emissions within the supply chains, followed by leakage of refrigeration gas and landfill disposal of unused fish portions (calculated as 62.5% wastage by weight). By isolating this waste during processing and developing compost using the methods of López-Mosquera et al. (2011), a reduction in the GHG of 5.8% and 1.2% was measured from the regional and city supply chains respectively.
A recent audit of GHG emissions in the Australian fishing and aquaculture sectors found that 45% of the emission profile was derived from feed/bait, transport, and processing-related costs (termed Scope 3 emissions), followed by 31% from Scope 1 emissions (fuel and fugitive emissions from refrigerant gases etc.; Blueshift Consulting 2022). The study did not specifically address the effect of interventions to reduce or re-use processing waste but did consider the impact of the aligned landfill disposal of these materials.
Efficient utilisation of wastes and by-products could also decrease the volume of waste generation and correspondingly the energy and water consumed by their treatment (Tomczak-Wandzel et al. 2015). Kurniasih et al. (2018) reported that the minimisation and conversion of waste generated in the seafood industry could potentially result in a saving of 27.2% of clean water.
There has been little work on GHG emissions from novel aquafeed ingredients generated on fish waste, in the context of their transformation to aquafeed components. However, feed manufacturers, in particular the larger companies such as Skretting and BioMar, are now taking significant steps in advanced carbon accounting methodologies for the raw materials used in their feeds (Blueshift Consulting 2022). Maiolo et al. (2020) reported that only a small number of studies dealt with an evaluation of feed components on an individual basis, including alternative ingredients for salmon aquafeed formulations (Pelletier and Tyedmers 2007); FM and fish oil production in Peru (Fréon et al. 2017); several aquafeed ingredients commonly used in Indonesia (Henriksson et al. 2017); and alternative meal and fat (or oil) sources (Silva et al. 2018). In this study, Maiolo et al. (2020) found that insect meal had a similar impact to PBM on GHG emissions, and was more efficient than were microalgae and macroalgae as potential ingredients.
Developing next-generation feed supplements for aquafeed ingredients via a circular economy (Fig. 1) that does not deplete natural resources, and may have positive impacts to reduce the environmental footprint, is the opportunity awaiting future sustainable and resilient aquaculture (Chaklader et al. 2021a). Food loss and waste associated with the seafood industry, and the knock-on effect of triggering an increased fishing effort to meet increasing market demand, perpetuates the linear economy in seafood production (Ruiz-Salmón et al. 2021). Aquafeed ingredient formulation from fish waste is, therefore, an opportunity to close the loop in the seafood industry, through the valorisation of waste streams, and subsequent utilisation in other industries, while eventually being fed back to the original industry (de la Caba et al. 2019).
The limitations to the future use of seafood waste in the development of alternate aquafeed ingredients, particularly in an Australian context, are described below.
Worldwide, there are logistical barriers to accumulating the minimum quantities required for fish-waste transformation to be economically viable (Hua et al. 2019). In Australia, local processing is often of insufficient volume for cost efficiency, multiple species are harvested and Australian labour costs are high (Cunningham et al. 2022). Large volumes of waste are needed for viable fish-waste transformation, and the transport of perishable material from disparate locations results in quality and consistency issues in the final product (Cunningham et al. 2022). High electricity costs are also a barrier to storing sufficient products to increase scale. Upfront capital costs, and investment costs without appropriate incentives, make alterations to fish-waste utilisation in these production systems difficult, especially for small businesses (Cunningham et al. 2022). Nonetheless, there are some examples of such transformation, in particular the enzyme hydrolysis of tuna waste in Port Lincoln and its subsequent application in horticulture and aquaculture (Howieson et al. 2017).
In regard to waste transformation via insects, while it is estimated that insect-meal production was ~10 000 t in Australia in 2020, and will rise to 0.5 million t by 2030, these volumes are still much lower than the present production levels of protein feeds and co-products (~5 million t of FM used/year; Alfiko et al. 2022). This industry will require significant investment, research, and development to mature into a viable, competitive commodity. To minimise the existing high production costs, more research into automation processes is required. While production remains low, the price will continue to be a barrier to the wider adoption of insect proteins generally, and in aquaculture specifically (Alfiko et al. 2022).
Despite regulatory changes relating to animal by-products and insect transformations, biosecurity concerns remain a barrier to reprocessing organic waste and circularity. For example, proteins from the waste of one species cannot be used to feed the same species, to avoid cannibalism, but can be fed to other species. There are also other biosecurity barriers; feed for salmon in Australia is not allowed to include waste from other fish industries such as tuna from Thailand (Cunningham et al. 2022). The regionality of raw materials and transport to a central hub is also problematic as different States and Territories have different transport and border regulations.
Since insect species are able to convert biowastes into protein sources, sanitation measures for the safe use of substrate must be developed to ensure that insect meals are free of diseases and undesirable elements. The use of insect meals as a replacement for FM in aquafeeds requires the ongoing development of legal frameworks and legislation, as well as the improvement of risk-assessment procedures (Alfiko et al. 2022).
The need to examine edible portions of aquacultured fish for heavy metal contamination resulting from aquafeed ingredients grown on viscera has been previously explained (Table 1). Another food-safety issue to be considered is the persistent organic pollutants (POPs), which include a wide range of compounds such as dioxins, polychlorinated biphenyls (PCBs) and polybrominated diphenyl ethers (PBDEs) (Berntssen et al. 2000; Hixson 2014; Sprague et al. 2015). In fact, the consumption of fish has been regarded as one of the major dietary exposure routes for POPs (Sprague et al. 2015). The risk of bioaccumulation of heavy metal and POPs is a barrier to commercialisation of novel feed ingredients. Hence, where the viscera of fish might be valorised, the safety of the final edible product should not be overlooked.
The gap in knowledge of the impact of alternate rendered by-products and FPH on edible product quality and shelf-life has been discussed. Similarly, it is also necessary to research the impact of feeding aquaculture species with different sources such as insect meals on the safety, quality, and societal acceptance of those seafood (Alfiko et al. 2022). Positive consumer perception of by-products may increase their viability (Hua et al. 2019).
Future considerations, opportunities and directions
We contend that end-user/market-driven considerations of the final edible product when trialling new aquafeed ingredients are often neglected. Specifically, to fully investigate the opportunities for use of fish waste in aquafeed ingredients, and to better assess some of the market/end-user implications (safety, quality, sustainability and perceptions), the following areas of market-driven investigation are recommended:
A better understanding of the impact of different aquafeed ingredients on food science characteristics, including compositional analysis of the edible component, food safety, sensory and biochemical assessment, when compared with traditional feed formulations, and shelf-life.
A better understanding of the importance of consumer perception of aquacultured products fed on alternate ingredients.
A better understanding of the sustainability assessments of individual aquafeed ingredients used in feed, and the impact of reduction of food waste in the seafood supply-chain life-cycle.
Economic and logistical feasibility assessments and initiatives to overcome commercialisation barriers associated with scale, transport and infrastructure (Fig. 2).
A number of multidisciplinary and holistic stages, as illustrated in Fig. 2, will need to be developed and implemented to increase circularity in the seafood value chains.
The potential areas that need to be implemented for increased uptake of seafood circularity in seafood value chains, adapted from the study of Cooney et al. (2023).
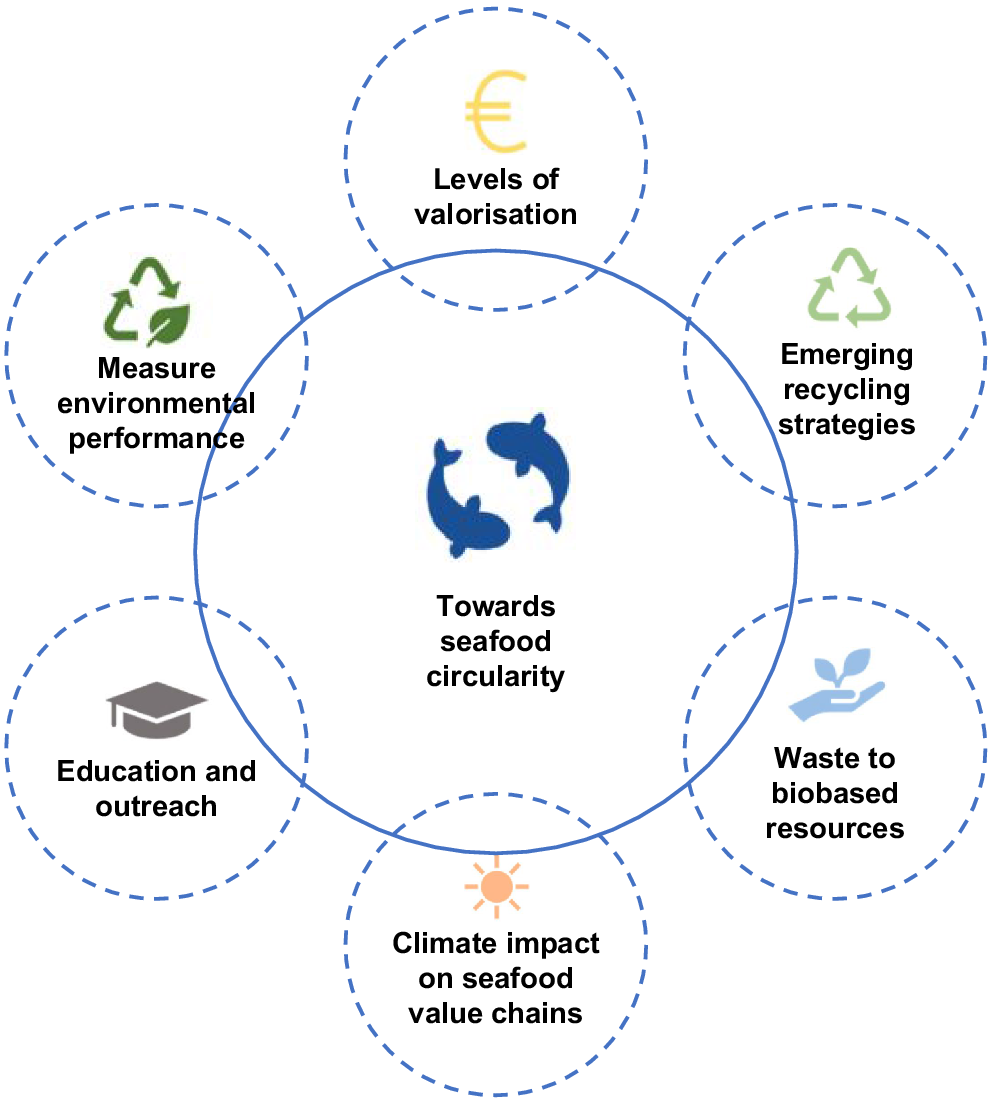
Data availability
The authors support transparency and reproducibility of research, to access information used in this review, email reaz.chaklader@dpird.wa.gov.au.
Declaration of funding
This study was supported by the Research Training Program (RTP) Stipend Scholarship, funded by Australian Government to Md Reaz Chaklader (No. 19061054-Curtin), FRDC 2016.180 (Assessment of options for Virus affected carp) and FRDC 2013/711.40 (New options for Seafood Processing Waste Seafood).
References
Abdel-Ghany HM, Salem ME-S (2020) Effects of dietary chitosan supplementation on farmed fish; a review. Reviews in Aquaculture 12, 438-452.
| Crossref | Google Scholar |
Abdul Kader M, Koshio S, Ishikawa M, Yokoyama S, Bulbul M, Honda Y, Mamauag RE, Laining A (2011) Growth, nutrient utilization, oxidative condition, and element composition of juvenile red sea bream Pagrus major fed with fermented soybean meal and scallop by-product blend as fishmeal replacement. Fisheries Science 77, 119-128.
| Crossref | Google Scholar |
Alfiko Y, Xie D, Astuti RT, Wong J, Wang L (2022) Insects as a feed ingredient for fish culture: status and trends. Aquaculture and Fisheries 7, 166-178.
| Crossref | Google Scholar |
Alfnes F, Guttormsen AG, Steine G, Kolstad K (2006) Consumers’ willingness to pay for the color of salmon: a choice experiment with real economic incentives. American Journal of Agricultural Economics 88, 1050-1061.
| Crossref | Google Scholar |
Ali H, Khan E (2018) Bioaccumulation of non-essential hazardous heavy metals and metalloids in freshwater fish. Risk to human health. Environmental Chemistry Letters 16, 903-917.
| Crossref | Google Scholar |
Aragão C, Gonçalves AT, Costas B, Azeredo R, Xavier MJ, Engrola S (2022) Alternative proteins for fish diets: implications beyond growth. Animals 12, 1211.
| Crossref | Google Scholar |
Aro T, Tahvonen R, Koskinen L, Kallio H (2003) Volatile compounds of Baltic herring analysed by dynamic headspace sampling–gas chromatography–mass spectrometry. European Food Research and Technology 216, 483-488.
| Crossref | Google Scholar |
Arulkumar A, Paramasivam S, Miranda JM (2018) Combined effect of icing medium and red alga Gracilaria verrucosa on shelf life extension of Indian Mackerel (Rastrelliger kanagurta). Food Bioprocess and Technology 11, 1911-1922.
| Crossref | Google Scholar |
Baptista RC, Horita CN, Sant’Ana AS (2020) Natural products with preservative properties for enhancing the microbiological safety and extending the shelf-life of seafood: a review. Food Research International 127, 108762.
| Crossref | Google Scholar |
Belghit I, Liland NS, Gjesdal P, Biancarosa I, Menchetti E, Li Y, Waagbø R, Krogdahl Å, Lock E-J (2019) Black soldier fly larvae meal can replace fish meal in diets of sea-water phase Atlantic salmon (Salmo salar). Aquaculture 503, 609-619.
| Crossref | Google Scholar |
Berntssen MHG, Lundebye A-K, Hamre K (2000) Tissue lipid peroxidative responses in Atlantic salmon (Salmo salar L.) parr fed high levels of dietary copper and cadmium. Fish Physiology and Biochemistry 23, 35-48.
| Crossref | Google Scholar |
Blondeau N, Lipsky RH, Bourourou M, Duncan MW, Gorelick PB, Marini AM (2015) Alpha-linolenic acid: an omega-3 fatty acid with neuroprotective properties – ready for use in the stroke clinic? BioMed Research International 2015, 1-8.
| Crossref | Google Scholar |
Borgogno M, Dinnella C, Iaconisi V, Fusi R, Scarpaleggia C, Schiavone A, Monteleone E, Gasco L, Parisi G (2017) Inclusion of Hermetia illucens larvae meal on rainbow trout (Oncorhynchus mykiss) feed: effect on sensory profile according to static and dynamic evaluations. Journal of the Science of Food and Agriculture 97, 3402-3411.
| Crossref | Google Scholar |
Calanche JB, Beltrán JA, Hernández Arias AJ (2020) Aquaculture and sensometrics: the need to evaluate sensory attributes and the consumers’ preferences. Reviews in Aquaculture 12, 805-821.
| Crossref | Google Scholar |
Castro M, Parraga K, Alonso J, Cobar J, Watts E (2020) Black drum (Pogonias cromis) shelf life comparing three packaging technologies. Journal of Aquatic Food Product Technology 29, 925-934.
| Crossref | Google Scholar |
Chaklader MR, Siddik MAB, Fotedar R, Howieson J (2019) Insect larvae, Hermetia illucens in poultry by-product meal for barramundi, Lates calcarifer modulates histomorphology, immunity and resistance to Vibrio harveyi. Scientific Reports 9, 16703.
| Crossref | Google Scholar |
Chaklader MR, Siddik MAB, Fotedar R (2020a) Total replacement of fishmeal with poultry by-product meal affected the growth, muscle quality, histological structure, antioxidant capacity and immune response of juvenile barramundi, Lates calcarifer. PLoS ONE 15, e0242079.
| Crossref | Google Scholar |
Chaklader MR, Fotedar R, Howieson J, Siddik MAB, Foysal MJ (2020b) The ameliorative effects of various fish protein hydrolysates in poultry by-product meal based diets on muscle quality, serum biochemistry and immunity in juvenile barramundi, Lates calcarifer. Fish & Shellfish Immunology 104, 567-578.
| Crossref | Google Scholar |
Chaklader MR, Howieson J, Fotedar R, Siddik MAB (2020c) Supplementation of Hermetia illucens larvae in poultry by-product meal-based barramundi, Lates calcarifer diets improves adipocyte cell size, skin barrier functions, and immune responses. Frontiers in Nutrition 7, 61358.
| Crossref | Google Scholar |
Chaklader MR, Howieson J, Foysal MJ, Fotedar R (2021a) Transformation of fish waste protein to Hermetia illucens protein improves the efficacy of poultry by-products in the culture of juvenile barramundi, Lates calcarifer. Science of The Total Environment 796, 149045.
| Crossref | Google Scholar |
Chaklader MR, Howieson J, Fotedar R (2021b) Growth, hepatic health, mucosal barrier status and immunity of juvenile barramundi, Lates calcarifer fed poultry by-product meal supplemented with full-fat or defatted Hermetia illucens larval meal. Aquaculture 543, 737026.
| Crossref | Google Scholar |
Chaklader MR, Howieson J, Siddik MAB, Foysal MJ, Fotedar R (2021c) Supplementation of tuna hydrolysate and insect larvae improves fishmeal replacement efficacy of poultry by-product in Lates calcarifer (Bloch, 1790) juveniles. Scientific Reports 11, 4997.
| Crossref | Google Scholar |
Chaklader MR, Chung WH, Howieson J, Fotedar R (2022) A combination of Hermetia illucens reared on fish waste and poultry by-product meal improves sensory and physicochemical quality of farmed Barramundi filets. Frontiers in Nutrition 8, 788064.
| Crossref | Google Scholar |
Chaklader MR, Howieson J, Foysal MJ, Hanif MA, Abdel-Latif HMR, Fotedar R (2023a) Fish waste to sustainable additives: fish protein hydrolysates alleviate intestinal dysbiosis and muscle atrophy induced by poultry by-product meal in Lates calcarifer juvenile. Frontiers in Nutrition 10, 424.
| Crossref | Google Scholar |
Chaklader MR, Chung WH, Howieson J, Fotedar R (2023b) A mixture of full-fat and defatted Hermetia illucens larvae and poultry by-products as sustainable protein sources improved fillet quality traits in farmed Barramundi, Lates calcarifer. Foods 12, 362.
| Crossref | Google Scholar |
Chalamaiah M, Dinesh Kumar B, Hemalatha R, Jyothirmayi T (2012) Fish protein hydrolysates: proximate composition, amino acid composition, antioxidant activities and applications: a review. Food Chemistry 135, 3020-3038.
| Crossref | Google Scholar |
Cheng J-H, Sun D-W, Zeng X-A, Liu D (2015) Recent advances in methods and techniques for freshness quality determination and evaluation of fish and fish fillets: a review. Critical Reviews in Food Science and Nutrition 55, 1012-1225.
| Crossref | Google Scholar |
Chung WH, Howieson J, Chaklader MDR (2021) The ameliorative effects of low-temperature pasteurization on physicochemical and microbiological quality of raw Akoya pearl oyster (Pinctada fucata). Food Control 129, 108241.
| Crossref | Google Scholar |
Codabaccus MB, Ng W-K, Nichols PD, Carter CG (2013) Restoration of EPA and DHA in rainbow trout (Oncorhynchus mykiss) using a finishing fish oil diet at two different water temperatures. Food Chemistry 141, 236-244.
| Crossref | Google Scholar |
Colombo SM, Turchini GM (2021) ‘Aquafeed 3.0’: creating a more resilient aquaculture industry with a circular bioeconomy framework. Reviews in Aquaculture 13, 1156-1158.
| Crossref | Google Scholar |
Colombo SM, Roy K, Mraz J, Wan AHL, Davies SJ, Tibbetts SM, Øverland M, Francis DS, Rocker MM, Gasco L, Spencer E, Metian M, Trushenski JT, Turchini GM (2022) Towards achieving circularity and sustainability in feeds for farmed blue foods. Reviews in Aquaculture 2022, 1-27.
| Crossref | Google Scholar |
Cooney R, Sousa DB, Fernández-Ríos A, Mellett S, Rowan N, Morse AP, Hayes M, Laso J, Regueiro L, Wan AHL (2023) A circular economy framework for seafood waste valorisation to meet challenges and opportunities for intensive production and sustainability. Journal of Cleaner Production 392, 136283.
| Crossref | Google Scholar |
Costa M, Cardoso C, Afonso C, Bandarra NM, Prates JAM (2021) Current knowledge and future perspectives of the use of seaweeds for livestock production and meat quality: a systematic review. Journal of Animal Physiology and Animal Nutrition 105, 1075-1102.
| Crossref | Google Scholar |
Craft CD, Ross C, Sealey WM, Gaylord TG, Barrows FT, Fornshell G, Myrick CA (2016) Growth, proximate composition, and sensory characteristics of rainbow trout Oncorhynchus mykiss consuming alternative proteins. Aquaculture 459, 223-231.
| Crossref | Google Scholar |
de la Caba K, Guerrero P, Trung TS, Cruz-Romero M, Kerry JP, Fluhr J, Maurer M, Kruijssen F, Albalat A, Bunting S, Burt S, Little D, Newton R (2019) From seafood waste to active seafood packaging: an emerging opportunity of the circular economy. Journal of Cleaner Production 208, 86-98.
| Crossref | Google Scholar |
Denham FC, Biswas WK, Solah VA, Howieson JR (2016) Greenhouse gas emissions from a Western Australian finfish supply chain. Journal of Cleaner Production 112, 2079-2087.
| Crossref | Google Scholar |
Egerton S, Wan A, Murphy K, Collins F, Ahern G, Sugrue I, Busca K, Egan F, Muller N, Whooley J, McGinnity P, Culloty S, Ross RP, Stanton C (2020) Replacing fishmeal with plant protein in Atlantic salmon (Salmo salar) diets by supplementation with fish protein hydrolysate. Scientific Reports 10, 4194.
| Crossref | Google Scholar |
El-Rahman AA, Badrawy N (2007) Evaluation of using crayfish (Procambarus clarkii) as partial or complete replacement of fish meal protein in rearing the Nile tilapia (Oreochromis Niloticus) fry. Egyptian Journal of Aquatic Biology and Fisheries 11, 31-39.
| Crossref | Google Scholar |
Emery JA, Smullen R, Keast RSJ, Turchini GM (2016) Viability of tallow inclusion in Atlantic salmon diet, as assessed by an on-farm grow out trial. Aquaculture 451, 289-297.
| Crossref | Google Scholar |
European Union (2013) No 1380/2013 of the European Parliament and of the Council of 11 December 2013 on the Common Fisheries Policy, amending Council Regulations (EC) No 1954/2003 and (EC) No 1224/2009 and repealing Council Regulations (EC) No 2371/2002 and (EC) No 639/2004 and Council Decision 2004/585/EC. Official Journal of European Union 354, 22-61.
| Google Scholar |
Fernandes DVGS, Castro VS, Neto AdC, Figueiredo EEdS (2018) Salmonella spp. in the fish production chain: a review. Ciência Rural 48, e20180141.
| Crossref | Google Scholar |
Floros JD, Newsome R, Fisher W, Barbosa-Canovas GV, Chen H, Dunne CP, German JB, Hall RL, Heldman DR, Karwe MV, Knabel SJ, Labuza TP, Lund DB, Newell-McGloughlin M, Robinson JL, Sebranek JG, Shewfelt RL, Tracy WF, Weaver CM, Ziegler GR (2010) Feeding the world today and tomorrow: the importance of food science and technology an IFT scientific review. Comprehensive Reviews in Food Science and Food Safety 9, 572-599.
| Crossref | Google Scholar |
Francis G, Makkar HPS, Becker K (2001) Antinutritional factors present in plant-derived alternate fish feed ingredients and their effects in fish. Aquaculture 199, 197-227.
| Crossref | Google Scholar |
Friesen E, Balfry SK, Skura BJ, Ikonomou M, Higgs DA (2013) Evaluation of poultry fat and blends of poultry fat with cold-pressed flaxseed oil as supplemental dietary lipid sources for juvenile sablefish (Anoplopoma fimbria). Aquaculture Research 44, 300-316.
| Crossref | Google Scholar |
Fréon P, Durand H, Avadí A, Huaranca S, Orozco Moreyra R (2017) Life cycle assessment of three Peruvian fishmeal plants: toward a cleaner production. Journal of Cleaner Production 145, 50-63.
| Crossref | Google Scholar |
Galkanda-Arachchige HSC, Wilson AE, Davis DA (2020) Success of fishmeal replacement through poultry by-product meal in aquaculture feed formulations: a meta-analysis. Reviews in Aquaculture 12, 1624-1636.
| Crossref | Google Scholar |
Gause BR, Trushenski JT (2013) Sparing fish oil with beef tallow in feeds for rainbow trout: effects of inclusion rates and finishing on production performance and tissue fatty acid composition. North American Journal of Aquaculture 75, 495-511.
| Crossref | Google Scholar |
Gifuni I, Pollio A, Safi C, Marzocchella A, Olivieri G (2019) Current bottlenecks and challenges of the microalgal biorefinery. Trends in Biotechnology 37, 242-252.
| Crossref | Google Scholar |
Goddard S, Al-Shagaa G, Ali A (2008) Fisheries by-catch and processing waste meals as ingredients in diets for Nile tilapia, Oreochromis niloticus. Aquaculture Research 39, 518-525.
| Crossref | Google Scholar |
Grigorakis K, Alexis M, Gialamas I, Nikolopoulou D (2004) Sensory, microbiological, and chemical spoilage of cultured common sea bass (Dicentrarchus labrax) stored in ice: a seasonal differentiation. European Food Research and Technology 219, 584-587.
| Crossref | Google Scholar |
Han D, Shan X, Zhang W, Chen Y, Wang Q, Li Z, Zhang G, Xu P, Li J, Xie S, Mai K, Tang Q, De Silva SS (2018) A revisit to fishmeal usage and associated consequences in Chinese aquaculture. Reviews in Aquaculture 10, 493-507.
| Crossref | Google Scholar |
He S, Franco C, Zhang W (2013) Functions, applications and production of protein hydrolysates from fish processing co-products (FPCP). Food Research International 50, 289-297.
| Crossref | Google Scholar |
Henriksson PJG, Mohan CV, Phillips MJ (2017) Evaluation of different aquaculture feed ingredients in Indonesia using life cycle assessment. Indonesian Journal of Life Cycle Assessment and Sustainability 1, 13-21.
| Google Scholar |
Henry M, Gasco L, Piccolo G, Fountoulaki E (2015) Review on the use of insects in the diet of farmed fish: past and future. Animal Feed Science and Technology 203, 1-22.
| Crossref | Google Scholar |
Hernández C, Hardy RW, Contreras-Rojas D, López-Molina B, González-Rodríguez B, Domínguez-Jimenez P (2014) Evaluation of tuna by-product meal as a protein source in feeds for juvenile spotted rose snapper Lutjanus guttatus. Aquaculture Nutrition 20, 574-582.
| Crossref | Google Scholar |
Hixson SM (2014) Fish nutrition and current issues in aquaculture: the balance in providing safe and nutritious seafood, in an environmentally sustainable manner. Journal of Acquaculture Research & Development 5, 234.
| Crossref | Google Scholar |
Hoque MZ, Akhter N, Chowdhury MSR (2022) Consumers’ preferences for the traceability information of seafood safety. Foods 11, 1675.
| Crossref | Google Scholar |
Hu L, Yun B, Xue M, Wang J, Wu X, Zheng Y, Han F (2013) Effects of fish meal quality and fish meal substitution by animal protein blend on growth performance, flesh quality and liver histology of Japanese seabass (Lateolabrax japonicus). Aquaculture 372–375, 52-61.
| Crossref | Google Scholar |
Hua K (2021) A meta-analysis of the effects of replacing fish meals with insect meals on growth performance of fish. Aquaculture 530, 735732.
| Crossref | Google Scholar |
Hua K, Cobcroft JM, Cole A, Condon K, Jerry DR, Mangott A, Praeger C, Vucko MJ, Zeng C, Zenger K, Strugnell JM (2019) The future of aquatic protein: implications for protein sources in aquaculture diets. One Earth 1, 316-329.
| Crossref | Google Scholar |
Iglesias J, Medina I, Bianchi F, Careri M, Mangia A, Musci M (2009) Study of the volatile compounds useful for the characterisation of fresh and frozen-thawed cultured gilthead sea bream fish by solid-phase microextraction gas chromatography–mass spectrometry. Food Chemistry 115, 1473-1478.
| Crossref | Google Scholar |
Itoh D, Koyachi E, Yokokawa M, Murata Y, Murata M, Suzuki H (2013) Microdevice for on-site fish freshness checking based on K-value measurement. Analytical Chemistry 85, 10962-10968.
| Crossref | Google Scholar |
İbrahim Halilogˇlu H, Bayır A, Necdet Sirkeciogˇlu A, Mevlüt Aras N, Atamanalp M (2004) Comparison of fatty acid composition in some tissues of rainbow trout (Oncorhynchus mykiss) living in seawater and freshwater. Food Chemistry 86, 55-59.
| Crossref | Google Scholar |
Jannathulla R, Sravanthi O, Moomeen S, Gopikrishna G, Dayal JS (2021) Microbial products in terms of isolates, whole-cell biomass, and live organisms as aquafeed ingredients: production, nutritional values, and market potential – a review. Aquaculture International 29, 623-650.
| Crossref | Google Scholar |
Jeon GH, Kim HS, Myung SH, Cho SH (2014) The effect of the dietary substitution of fishmeal with tuna by-product meal on growth, body composition, plasma chemistry and amino acid profiles of juvenile Korean rockfish (Sebastes schlegeli). Aquaculture nutrition 20, 753-761.
| Crossref | Google Scholar |
Kader MA, Koshio S (2012) Effect of composite mixture of seafood by-products and soybean proteins in replacement of fishmeal on the performance of red sea bream, Pagrus major. Aquaculture 368–369, 95-102.
| Crossref | Google Scholar |
Karl H, Kuhlmann H, Ruoff U (2003) Transfer of PCDDs and PCDFs into the edible parts of farmed rainbow trout, Oncorhynchus mykiss (Walbaum), via feed. Aquaculture Research 34, 1009-1014.
| Crossref | Google Scholar |
Khosravi S, Bui HTD, Rahimnejad S, Herault M, Fournier V, Kim S-S, Jeong J-B, Lee K-J (2015) Dietary supplementation of marine protein hydrolysates in fish-meal based diets for red sea bream (Pagrus major) and olive flounder (Paralichthys olivaceus). Aquaculture 435, 371-376.
| Crossref | Google Scholar |
Kim HS, Jung W-G, Myung SH, Cho SH, Kim DS (2014) Substitution effects of fishmeal with tuna byproduct meal in the diet on growth, body composition, plasma chemistry and amino acid profiles of juvenile olive flounder (Paralichthys olivaceus). Aquaculture 431, 92-98.
| Crossref | Google Scholar |
Kim K, Park Y, Je H-W, Seong M, Damusaru JH, Kim S, Jung J-Y, Bai SC (2019) Tuna byproducts as a fish-meal in tilapia aquaculture. Ecotoxicology and Environmental Safety 172, 364-372.
| Crossref | Google Scholar |
Krishfield L, Olson S, Haslun J (2019) Future fish feed: forecasting alternative aqua feed ingredients. Lux Research, Boston, USA. Available at https://members.luxresearchinc.com/research/report/33270
Kumar S, Mukherjee A, Dutta J (2020) Chitosan based nanocomposite films and coatings: emerging antimicrobial food packaging alternatives. Trends in Food Science & Technology 97, 196-209.
| Crossref | Google Scholar |
Kurniasih SD, Soesilo TEB, Soemantojo RW (2018) Water resources management at the fish processing industry using waste minimization approach. E3S Web of Conferences 74, 06004.
| Crossref | Google Scholar |
Lambrianidi L, Savvaidis IN, Tsiraki MI, El-Obeid T (2019) Chitosan and oregano oil treatments, individually or in combination, used to increase the shelf life of vacuum-packaged, refrigerated european eel (Anguilla anguilla) fillets. Journal of Food Protection 82, 1369-1376.
| Crossref | Google Scholar |
Laso J, Margallo M, Celaya J, Fullana P, Bala A, Gazulla C, Irabien A, Aldaco R (2016) Waste management under a life cycle approach as a tool for a circular economy in the canned anchovy industry. Waste Management & Research: The Journal for a Sustainable Circular Economy 34, 724-733.
| Crossref | Google Scholar |
Lerfall J, Jakobsen AN, Skipnes D, Waldenstrøm L, Hoel S, Rotabakk BT (2018) Comparative evaluation on the quality and shelf life of Atlantic Salmon (Salmo salar L.) filets using microwave and conventional pasteurization in combination with novel packaging methods. Journal of Food Science 83, 3099-3109.
| Crossref | Google Scholar |
Li R, Guo Z, Jiang P (2010) Synthesis, characterization, and antifungal activity of novel quaternary chitosan derivatives. Carbohydrate Research 345, 1896-1900.
| Crossref | Google Scholar |
Lock ER, Arsiwalla T, Waagbø R (2016) Insect larvae meal as an alternative source of nutrients in the diet of Atlantic salmon (Salmo salar) postsmolt. Aquaculture Nutrition 22, 1202-1213.
| Crossref | Google Scholar |
Love DC, Fry JP, Milli MC, Neff RA (2015) Wasted seafood in the United States: quantifying loss from production to consumption and moving toward solutions. Global Environmental Change 35, 116-124.
| Crossref | Google Scholar |
Lundebye A-K, Berntssen MHG, Lie O, Ritchie G, Isosaari P, Kiviranta H, Vartiainen T (2004) Dietary uptake of dioxins (PCDD/PCDFs) and dioxin-like PCBs in Atlantic salmon (Salmo salar). Aquaculture Nutrition 10, 199-207.
| Crossref | Google Scholar |
López-Mosquera ME, Fernández-Lema E, Villares R, Corral R, Alonso B, Blanco C (2011) Composting fish waste and seaweed to produce a fertilizer for use in organic agriculture. Procedia Environmental Sciences 9, 113-117.
| Crossref | Google Scholar |
Mai K, Li H, Ai Q, Duan Q, Xu W, Zhang C, Zhang L, Tan B, Liufu Z (2006) Effects of dietary squid viscera meal on growth and cadmium accumulation in tissues of Japanese seabass, Lateolabrax japonicus (Cuvier 1828). Aquaculture Research 37, 1063-1069.
| Crossref | Google Scholar |
Maiolo S, Parisi G, Biondi N, Lunelli F, Tibaldi E, Pastres R (2020) Fishmeal partial substitution within aquafeed formulations: life cycle assessment of four alternative protein sources. The International Journal of Life Cycle Assessment 25, 1455-1471.
| Crossref | Google Scholar |
Martínez-Llorens S, Vidal AT, Moñino AV, Gómez Ader J, Torres MP, Cerdá MJ (2008) Blood and haemoglobin meal as protein sources in diets for gilthead sea bream (Sparus aurata): effects on growth, nutritive efficiency and fillet sensory differences. Aquaculture Research 39, 1028-1037.
| Crossref | Google Scholar |
Michielsen CCJR, Hangelbroek RWJ, Feskens EJM, Afman LA (2019) Disentangling the effects of monounsaturated fatty acids from other components of a Mediterranean diet on serum metabolite profiles: a randomized fully controlled dietary intervention in healthy subjects at risk of the metabolic syndrome. Molecular Nutrition & Food Research 63, 1801095.
| Crossref | Google Scholar |
Monge-Ortiz R, Martínez-Llorens S, Lemos-Neto MJ, Falcó-Giaccaglia SL, Pagán MJ, Godoy-Olmos S, Jover-Cerdá M, Tomás-Vidal A (2020) Growth, sensory and chemical characterization of Mediterranean yellowtail (Seriola dumerili) fed diets with partial replacement of fish meal by other protein sources. Aquaculture Reports 18, 100466.
| Crossref | Google Scholar |
Moren M, Suontama J, Hemre G-I, Karlsen Ø, Olsen RE, Mundheim H, Julshamn K (2006) Element concentrations in meals from krill and amphipods, – possible alternative protein sources in complete diets for farmed fish. Aquaculture 261, 174-181.
| Crossref | Google Scholar |
Murali S, Krishnan VS, Amulya PR, Alfiya PV, Delfiya DSA, Samuel MP (2021) Energy and water consumption pattern in seafood processing industries and its optimization methodologies. Cleaner Engineering and Technology 4, 100242.
| Crossref | Google Scholar |
Nag M, Lahiri D, Dey A, Sarkar T, Pati S, Joshi S, Bunawan H, Mohammed A, Edinur HA, Ghosh S, Ray RR (2022) Seafood discards: a potent source of enzymes and biomacromolecules with nutritional and nutraceutical significance. Frontiers in Nutrition 9, 879929.
| Crossref | Google Scholar |
Najafian L, Babji AS (2012) A review of fish-derived antioxidant and antimicrobial peptides: their production, assessment, and applications. Peptides 33, 178-185.
| Crossref | Google Scholar |
Nielsen J, Hyldig G, Larsen E (2002) ‘Eating quality’ of fish – a review. Journal of Aquatic Food Product Technology 11, 125-141.
| Crossref | Google Scholar |
Olafsdottir G, Nesvadba P, Di Natale C, Careche M, Oehlenschläger J, Tryggvadóttir SV, Schubring R, Kroeger M, Heia K, Esaiassen M, Macagnano A, Jørgensen BM (2004) Multisensor for fish quality determination. Trends in Food Science & Technology 15, 86-93.
| Crossref | Google Scholar |
Olafsdóttir G, Martinsdóttir E, Oehlenschläger J, Dalgaard P, Jensen B, Undeland I, Mackie IM, Henehan G, Nielsen J, Nilsen H (1997) Methods to evaluate fish freshness in research and industry. Trends in Food Science & Technology 8, 258-265.
| Crossref | Google Scholar |
Olatunde OO, Benjakul S (2018a) Natural preservatives for extending the shelf-life of seafood: a revisit. Comprehensive Reviews in Food Science and Food Safety 17, 1595-1612.
| Crossref | Google Scholar |
Olatunde OO, Benjakul S (2018b) Nonthermal processes for shelf-life extension of seafoods: a revisit. Comprehensive Reviews in Food Science and Food Safety 17, 892-904.
| Crossref | Google Scholar |
Olsen RE, Henderson RJ, Sountama J, Hemre G-I, Ringø E, Melle W, Tocher DR (2004) Atlantic salmon, Salmo salar, utilizes wax ester-rich oil from Calanus finmarchicus effectively. Aquaculture 240, 433-449.
| Crossref | Google Scholar |
Olsen RE, Suontama J, Langmyhr E, Mundheim H, RingØ E, Melle W, Malde MK, Hemre G-I (2006) The replacement of fish meal with Antarctic krill, Euphausia superba in diets for Atlantic salmon, Salmo salar. Aquaculture Nutrition 12, 280-290.
| Crossref | Google Scholar |
Paschoalini AL, Bazzoli N (2021) Heavy metals affecting Neotropical freshwater fish: a review of the last 10 years of research. Aquatic Toxicology 237, 105906.
| Crossref | Google Scholar |
Pati S, Chatterji A, Dash BP, Raveen Nelson B, Sarkar T, Shahimi S, Atan Edinur H, Binti Abd Manan TS, Jena P, Mohanta YK, Acharya D (2020) Structural characterization and antioxidant potential of chitosan by γ-irradiation from the carapace of horseshoe crab. Polymers 12, 2361.
| Crossref | Google Scholar |
Pati S, Sarkar T, Sheikh HI, Bharadwaj KK, Mohapatra PK, Chatterji A, Dash BP, Edinur HA, Nelson BR (2021) γ-Irradiated chitosan from Carcinoscorpius rotundicauda (Latreille, 1802) improves the shelf life of refrigerated aquatic products. Frontiers in Marine Science 8, 664961.
| Crossref | Google Scholar |
Pavón Y, Cian RE, Campos Soldini MA, Hernández DR, Sánchez S, Drago SR (2018) Sensory and instrumental textural changes in fillets from Pacú (Piaractus mesopotamicus) fed different diets. Journal of Texture Studies 49, 646-652.
| Crossref | Google Scholar |
Pelletier N, Tyedmers P (2007) Feeding farmed salmon: Is organic better? Aquaculture 272, 399-416.
| Crossref | Google Scholar |
Puyol D, Batstone DJ, Hülsen T, Astals S, Peces M, Krömer JO (2017) Resource recovery from wastewater by biological technologies: opportunities, challenges, and prospects. Frontiers in Microbiology 7, 2106.
| Crossref | Google Scholar |
Quiñones J, Díaz R, Dantagnan P, Hernández A, Valdes M, Lorenzo JM, Cancino D, Sepúlveda N, Farías JG (2021) Dietary inclusion of Durvillaea antarctica meal and rapeseed (Brassica napus) oil on growth, feed utilization and fillet quality of rainbow trout (Oncorhynchus mykiss). Aquaculture 530, 735882.
| Crossref | Google Scholar |
Ruiz-Salmón I, Laso J, Margallo M, Villanueva-Rey P, Rodríguez E, Quinteiro P, Dias AC, Almeida C, Nunes ML, Marques A, Cortés A, Moreira MT, Feijoo G, Loubet P, Sonnemann G, Morse AP, Cooney R, Clifford E, Regueiro L, Méndez D, Anglada C, Noirot C, Rowan N, Vázquez-Rowe I, Aldaco R (2021) Life cycle assessment of fish and seafood processed products – a review of methodologies and new challenges. Science of the Total Environment 761, 144094.
| Crossref | Google Scholar |
Sealey WM, Gaylord TG, Barrows FT, Tomberlin JK, McGuire MA, Ross C, St-Hilaire S (2011) Sensory analysis of rainbow trout, Oncorhynchus mykiss, fed enriched black soldier fly prepupae, Hermetia illucens. Journal of the World Aquaculture Society 42, 34-45.
| Crossref | Google Scholar |
Sheng L, Wang L (2021) The microbial safety of fish and fish products: recent advances in understanding its significance, contamination sources, and control strategies. Comprehensive Reviews in Food Science and Food Safety 20, 738-786.
| Crossref | Google Scholar |
Shokri S, Parastouei K, Taghdir M, Abbaszadeh S (2020) Application an edible active coating based on chitosan – Ferulago angulata essential oil nanoemulsion to shelf life extension of Rainbow trout fillets stored at 4°C. International Journal of Biological Macromolecules 153, 846-854.
| Crossref | Google Scholar |
Siddik MAB, Howieson J, Partridge GJ, Fotedar R, Gholipourkanani H (2018a) Dietary tuna hydrolysate modulates growth performance, immune response, intestinal morphology and resistance to Streptococcus iniae in juvenile barramundi, Lates calcarifer. Scientific Reports 8, 15942.
| Crossref | Google Scholar |
Siddik MAB, Howieson J, Ilham I, Fotedar R (2018b) Growth, biochemical response and liver health of juvenile barramundi (Lates calcarifer) fed fermented and non-fermented tuna hydrolysate as fishmeal protein replacement ingredients. PeerJ 6, e4870.
| Crossref | Google Scholar |
Siddik MAB, Chungu P, Fotedar R, Howieson J (2019) Bioprocessed poultry by-product meals on growth, gut health and fatty acid synthesis of juvenile barramundi, Lates calcarifer (Bloch). PLoS ONE 14, e0215025.
| Crossref | Google Scholar |
Siddik MAB, Chaklader MR, Foysal MJ, Howieson J, Fotedar R, Gupta SK (2020) Influence of fish protein hydrolysate produced from industrial residues on antioxidant activity, cytokine expression and gut microbial communities in juvenile barramundi Lates calcarifer. Fish and Shellfish Immunology 97, 465-473.
| Crossref | Google Scholar |
Siddik MAB, Howieson J, Fotedar R, Partridge GJ (2021) Enzymatic fish protein hydrolysates in finfish aquaculture: a review. Reviews in Aquaculture 13, 406-430.
| Crossref | Google Scholar |
Silva CB, Valente LMP, Matos E, Brandão M, Neto B (2018) Life cycle assessment of aquafeed ingredients. The International Journal of Life Cycle Assessment 23, 995-1017.
| Crossref | Google Scholar |
Socaciu M-I, Semeniuc CA, Vodnar DC (2018) Edible films and coatings for fresh fish packaging: focus on quality changes and shelf-life extension. Coatings 8, 366.
| Crossref | Google Scholar |
Sprague M, Walton J, Campbell PJ, Strachan F, Dick JR, Bell JG (2015) Replacement of fish oil with a DHA-rich algal meal derived from Schizochytrium sp. on the fatty acid and persistent organic pollutant levels in diets and flesh of Atlantic salmon (Salmo salar, L.) post-smolts. Food Chemistry 185, 413-421.
| Crossref | Google Scholar |
Tacon AGJ, Lemos D, Metian M (2020) Fish for health: improved nutritional quality of cultured fish for human consumption. Reviews in Fisheries Science & Aquaculture 28, 449-458.
| Crossref | Google Scholar |
Tilley A, Colquhoun E, O’Keefe E, Nash S, McDonald D, Evans T, Gillespie G, Hardwick D, Beavis S, Francina C, Wheat DML, Howieson J (2019) Utilisation of carp biomass final report. Fisheries Research and development corporation (FRDC), Subiaco, WA, Australia. Available at https://nla.gov.au/nla.obj-2141053594/view
Tkaczewska J (2020) Peptides and protein hydrolysates as food preservatives and bioactive components of edible films and coatings – a review. Trends in Food Science & Technology 106, 298-311.
| Crossref | Google Scholar |
Tritscher A, Miyagishima K, Nishida C, Branca F (2013) Ensuring food safety and nutrition security to protect consumer health: 50 years of the Codex alimentarius commission. Bulletin of the World Health Organization 91, 468.
| Crossref | Google Scholar |
Turchini GM, Mentasti T, Frøyland L, Orban E, Caprino F, Moretti VM, Valfré F (2003) Effects of alternative dietary lipid sources on performance, tissue chemical composition, mitochondrial fatty acid oxidation capabilities and sensory characteristics in brown trout (Salmo trutta L.). Aquaculture 225, 251-267.
| Crossref | Google Scholar |
Turek J, Sampels S, Khalili Tilami S, Červený D, Kolářová J, Randák T, Mráz J, Másílko J, Steinbach C, Burkina V, Kozák P, Žlábek V (2020) Insects in the feed of rainbow trout, oncorhynchus mykiss (Actinopterygii, salmonidae): effect on growth, fatty acid composition, and sensory attributes. Acta Ichthyologica et Piscatoria 50, 171-181.
| Crossref | Google Scholar |
Ucar Y, Özogul Y, Özogul F, Durmuş M, Köşker AR (2020) Effect of nisin on the shelf life of sea bass (Dicentrarchus labrax L.) fillets stored at chilled temperature (4 ± 2°C). Aquaculture International 28, 851-863.
| Crossref | Google Scholar |
UN (2023) Goal 13: take urgent action to combat climate change and its impacts. Available at https://www.un.org/sustainabledevelopment/climate-change/ [Verified 7 January 2023]
Van Vo B, Siddik MAB, Fotedar R, Chaklader MR, Hanif MA, Foysal MJ, Nguyen HQ (2020) Progressive replacement of fishmeal by raw and enzyme-treated alga, Spirulina platensis influences growth, intestinal micromorphology and stress response in juvenile barramundi, Lates calcarifer. Aquaculture 529, 735741.
| Crossref | Google Scholar |
Venugopal V (2021) Valorization of seafood processing discards: bioconversion and bio-refinery approaches. Frontiers in Sustainable Food Systems 5, 611835.
| Crossref | Google Scholar |
Venugopal V (2022) Green processing of seafood waste biomass towards blue economy. Current Research in Environmental Sustainability 4, 100164.
| Crossref | Google Scholar |
Waagbø R, Berntssen MHG, Danielsen T, Helberg H, Kleppa AL, Berg Lea T, Rosenlund G, Tvenning L, Susort S, Vikeså V, Breck O (2013) Feeding Atlantic salmon diets with plant ingredients during the seawater phase – a full-scale net production of marine protein with focus on biological performance, welfare, product quality and safety. Aquaculture Nutrition 19, 598-618.
| Crossref | Google Scholar |
Wang G, Peng K, Hu J, Yi C, Chen X, Wu H, Huang Y (2019) Evaluation of defatted black soldier fly (Hermetia illucens L.) larvae meal as an alternative protein ingredient for juvenile Japanese seabass (Lateolabrax japonicus) diets. Aquaculture 507, 144-154.
| Crossref | Google Scholar |
Ween O, Stangeland JK, Fylling TS, Aas GH (2017) Nutritional and functional properties of fishmeal produced from fresh by-products of cod (Gadus morhua L.) and saithe (Pollachius virens). Heliyon 3, e00343.
| Crossref | Google Scholar |
Williams KC, Paterson BD, Barlow CG, Ford A, Roberts R (2003) Potential of meat meal to replace fish meal in extruded dry diets for barramundi, Lates calcarifer (Bloch). II. Organoleptic characteristics and fatty acid composition. Aquaculture Research 34, 33-42.
| Crossref | Google Scholar |
Wong M-H, Mo W-Y, Choi W-M, Cheng Z, Man Y-B (2016) Recycle food wastes into high quality fish feeds for safe and quality fish production. Environmental Pollution 219, 631-638.
| Crossref | Google Scholar |
Wu L, Pu H, Sun D-W (2019) Novel techniques for evaluating freshness quality attributes of fish: a review of recent developments. Trends in Food Science & Technology 83, 259-273.
| Crossref | Google Scholar |
Xu H, Bi Q, Liao Z, Sun B, Jia L, Wei Y, Liang M (2021) Long-term alternate feeding between fish oil-and terrestrially sourced oil-based diets mitigated the adverse effects of terrestrially sourced oils on turbot fillet quality. Aquaculture 531, 735974.
| Crossref | Google Scholar |
Yao L, Luo Y, Sun Y, Shen H (2011) Establishment of kinetic models based on electrical conductivity and freshness indictors for the forecasting of crucian carp (Carassius carassius) freshness. Journal of Food Engineering 107, 147-151.
| Crossref | Google Scholar |
Zamora-Sillero J, Gharsallaoui A, Prentice C (2018) Peptides from fish by-product protein hydrolysates and its functional properties: an overview. Marine Biotechnology 20, 118-130.
| Crossref | Google Scholar |
Zhaleh S, Shahbazi Y, Shavisi N (2019) Shelf-life enhancement in fresh and frozen rainbow trout fillets by the employment of a novel active coating design. Journal of Food Science 84, 3691-3699.
| Crossref | Google Scholar |
Ziegler F, Jafarzadeh S, Hognes ES, Winther U (2022) Greenhouse gas emissions of Norwegian seafoods: From comprehensive to simplified assessment. Journal of Industrial Ecology 26, 1908-1919.
| Crossref | Google Scholar |