Thermal drones are highly effective for detecting elusive Bennett’s tree kangaroos (Dendrolagus bennettianus) in Australia’s tropical rainforests
Emmeline Bernadette Barrett Norris A * and Johan Larson AA
Abstract
Tree kangaroos (Dendrolagus spp.) are poorly studied owing to their elusive arboreal nature, which makes detection difficult using traditional methods such as spotlighting and hand-held thermal cameras. This note documents the first successful detection of Bennett’s tree kangaroos (D. bennettianus) using thermal drones in tropical rainforest at Cape Tribulation, Australia. In less than an hour, six individuals were observed across 17 ha. The method proved minimally invasive and highly effective in rainforest environments despite dense vegetation and high temperatures, demonstrating both the unexpected abundance of this species and the utility of thermal drones for monitoring tropical arboreal fauna.
Keywords: arboreal mammals, population monitoring, remote sensing, RPAS, survey method, thermal infrared, UAV, wildlife conservation.
Introduction
Tropical rainforests are biodiverse yet under-represented in long-term biodiversity monitoring (Collen et al. 2008), partly owing to challenges detecting fauna within dense vegetation. Tree kangaroos (Dendrolagus spp.), rare and elusive marsupials endemic to lowland and montane tropical rainforest in New Guinea and north-eastern Australia, exemplify these difficulties (Flannery et al. 1996). As arboreal folivores, tree kangaroos are vulnerable to deforestation and climate change, which threaten to further restrict their already limited habitat (Kanowski et al. 2001; Krockenberger et al. 2012; Beehler et al. 2021). Alarmingly, 12 of 14 species are listed as threatened (IUCN 2024). Yet, the drivers of abundance and the impacts of climate change on most species remain poorly understood (Kanowski 2021).
The Australian species, Lumholtz’s (D. lumholtzi) and Bennett’s (D. bennettianus) tree kangaroos, are considered secure relative to the New Guinean species because of the protection of most remaining habitat and cessation of subsistence hunting (Newell 1999). But they both remain poorly studied compared with other rainforest mammals (Kanowski 2021; IUCN 2024). More is known about Lumholtz’s tree kangaroo because of its distribution encompassing the closely populated Atherton Tablelands, where logging and agricultural clearing has increased sightings along forest tracks, forest edges, and paddocks (Laurance and Laurance 1996; Kanowski et al. 2003; Shima et al. 2019). Although survey methods using spotlights and hand-held thermal cameras have facilitated detection of Lumholtz’s tree kangaroo (Pocknee et al. 2021; Underwood et al. 2022), these techniques are less effective in the largely intact Bennett’s tree kangaroo habitat where fewer roads, mountainous terrain, denser vegetation, and a taller canopy hinder detectability (Flannery et al. 1996). Consequently, data are lacking for this species (Winter et al. 2019).
Bennett’s tree kangaroo, called tcharibeena by the Kuku Yalanji traditional owners, is confined to 1500–2500 km2 of rainforest between the Daintree River and Cooktown (Fig. 1a; Martin 2005). Its habitat comprises lowland and montane mesophyll vine forests and gallery forests, with young males occasionally dispersing through eucalyptus woodlands (Flannery 1996). Most knowledge about Bennett’s tree kangaroo comes from a single radio-tracking study, which documented its primarily nocturnal activity, daytime roosting in vine-covered emergent tree canopies, and sex-specific differences in home-range size and habitat use (Martin 1996). However, drivers of its distribution and population density remain unresolved because no systematic population surveys have been conducted (Kanowski 2021). Hence, new detection methods are needed for Bennett’s and other tree kangaroo species to enable robust population assessments that can inform appropriate management.
Maps showing (a, b) the location of the Daintree Rainforest Observatory (DRO) adjacent to the Wet Tropics World Heritage Area and Bennett’s tree kangaroo (Dendrolagus bennettianus) extent of occurrence in north-eastern Australia, (c) drone flight paths (dashed lines) over the DRO rainforest with Bennett’s tree kangaroo (BTK) sightings from the 21 and 22 November 2024. Point colours correspond to the drone flight during which they were observed. The home point and canopy crane locations, used for drone launch and remote operation respectively, are also indicated.
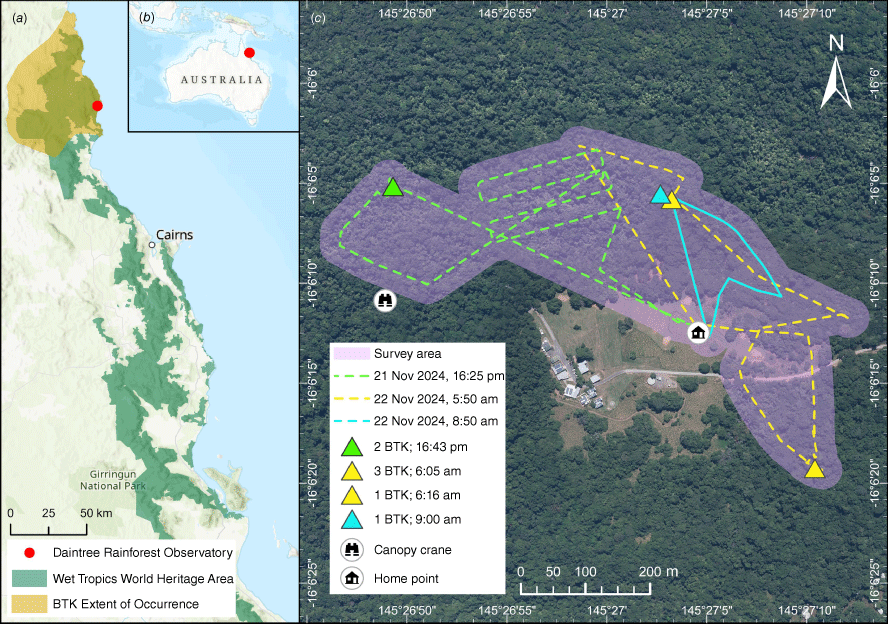
Drone-mounted thermal cameras (hereafter thermal drones) have been shown to be effective for detecting rainforest arboreal mammals, including great apes (Burke et al. 2019a; van Berkel et al. 2023), monkeys (Whitworth et al. 2022; Pinel-Ramos et al. 2024), and sloths (Santos et al. 2023). As large (<14 kg) canopy-dwelling mammals (Flannery 1996), Bennett’s tree kangaroos are obvious candidates for thermal-drone detection (Beranek et al. 2021; Kanowski 2021). To our knowledge, tree kangaroos have not yet been successfully detected using thermal drones. Previous attempts to locate Lumholtz’s tree kangaroos with drones were unsuccessful, possibly owing to lower thermal-camera resolution in earlier drone models (R. Hopkinson, pers. comm. 2024). Improvements in thermal-camera resolution could present drones as a more feasible monitoring option, offering valuable insights into the behaviour, ecology, and population status of Bennett’s tree kangaroo. This paper documents the first successful detection of Bennett’s tree kangaroo by using thermal drones.
Methods
The study was conducted at the Daintree Rainforest Observatory (DRO; −16.104049, 145.449463), in the lowlands of Cape Tribulation, Queensland. The DRO features a 47-m canopy crane providing access to the rainforest canopy (Supplementary Fig. S1). The surrounding forest comprises complex mesophyll vine forest with a dense canopy layer approximately 25 m tall and emergent trees reaching 40 m tall. Canopy lianas and wiry vines are common. The site experiences a tropical climate with a distinct wet season from December to April.
Three exploratory drone flights were conducted on 21 and 22 November 2024 to locate spectacled flying-foxes (Pteropus conspicillatus), and incidental sightings of other species were recorded (James Cook University Animal Research Authority No. A2969). Two drone models were used: a DJI Matrice 350 RTK drone equipped with a DJI Zenmuse H30T high-resolution thermal-camera payload, and a DJI Mavic Enterprise 3T drone with a lower-resolution thermal camera (DJI Technology Co, Shenzhen, China; Supplementary Table S1).
The first flight commenced at 16:25 pm in overcast conditions by using the high-resolution drone, and the second flight was conducted the following morning at 5:50 am in rainy conditions (Table S2). The high-resolution drone was positioned in a nearby clearing (Fig. 1), then launched and operated remotely from the uppermost crane-tower platform (Fig. S1), maintaining visual line of sight (VLOS) over long distances above the rainforest canopy. The third flight commenced at 8:50 am in sunnier conditions by using the low-resolution drone (Table S2), which was launched from the same clearing without the assistance of the canopy crane.
Drones were manually flown 60–70 m above ground level, approximately 20–30 m above the canopy, with cameras angled downward between −10° and −30° to maximise area coverage. Flight speeds varied between 2 and 6 m s−1, with the drones hovering in place to investigate potential fauna detections. Thermal imagery was the primary detection method, supplemented by RGB imagery for species confirmation. Both videos and still images were recorded. All flights adhered to Civil Aviation Safety Authority (CASA) regulations.
Thermal and RGB video footage was reviewed post-flight to confirm detections. Temperature data were extracted from thermal images by using the IRimage-UAV plug-in in Fiji (Schindelin et al. 2012; Pereyra Irujo 2022). Individual sightings, flight paths, and approximate thermal-camera footprints were mapped using GPS metadata (Fig. 1).
Results and discussion
Thermal drones detected six Bennett’s tree kangaroos in under an hour across three flights (Table S2). During the first flight, two tree kangaroos were observed eating mile-a-minute vine (Decalobanthus peltatus) and fire vine (Tetracera daemeliana) in the canopy. The second flight identified three tree kangaroos approximately 800 m from the initial sighting (Fig. 1). Although thermal signatures were clearly visible, dense foliage delayed visual confirmation using the RGB camera (Fig. 2). Another tree kangaroo was detected 400 m away during the second and third flights (Figs 1, S2). The spatial separation and brief time between detections suggest these were six distinct individuals, consistent with home-range estimates of ~10 ha for females with offspring and up to 30 ha for solitary males, whose ranges typically overlap with multiple females (Martin 1996). These findings suggest that Bennett’s tree kangaroos are abundant in lowland rainforest at Cape Tribulation, supporting the idea of their population expansion from mountaintop refugia after traditional hunting practices ceased (Martin 1996; Newell 1999).
Thermal and RGB images of five Bennett’s tree kangaroos (Dendrolagus bennettianus) detected with a high-resolution (1280 × 1024 pixel) drone-mounted thermal camera. Images were captured (a, b) at approximately 16:43 pm on 21 November 2024 and (c, d) at 6:05 am on 22 November 2024 in overcast conditions. Zoom (a, b) and wide-angle (c, d) camera modes were used on both occasions to confirm the species. Paired thermal and RGB images have been cropped to show the same area.
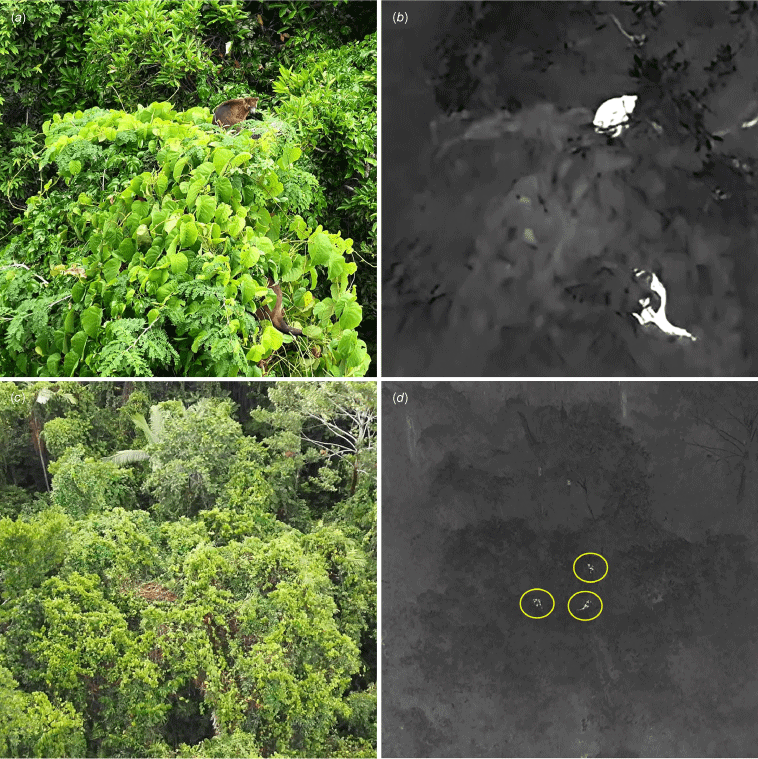
Flights in the early morning and late afternoon under overcast skies enhanced detection, as cooler canopy temperatures (26–30°C) contrasted with tree kangaroo thermal signatures (31–35°C). Rapid heating of canopy foliage under direct sunlight during the third flight increased false positives by causing green ant (Oecophylla smaragdina) nests to appear as hotspots, despite cooler ambient temperatures than during the preceding afternoon’s flight (Table S2). These findings reinforce the importance of selecting appropriate weather conditions for thermal-drone surveys (Burke et al. 2019b), while demonstrating that high temperatures do not preclude using thermal drones to detect rainforest mammals.
Bennett’s tree kangaroos did not exhibit any disturbance response to drones. When threatened, tree kangaroos typically leap to lower branches or the forest floor (Flannery et al. 1996), and we expected to observe this behaviour if the animals were disturbed. One animal paused to look at the drone before resuming foraging behaviour, and no other animals visibly reacted. The non-invasive nature of thermal drones supports their use for long-term monitoring where minimising disturbance is crucial.
Integrating thermal drones with canopy cranes overcomes the challenges of maintaining VLOS during drone-based wildlife surveys in dense rainforests. Although this approach is restricted to areas visible from the crane, it provides a foundation for refining thermal-drone methods to improve spatial coverage in wildlife surveys. In the absence of cranes, alternative strategies, such as operating drones from vantage points or using thermal scopes from crewed aircraft, may be needed to overcome VLOS constraints in tall forests. Concurrent RGB imagery could further enhance surveys by enabling individual identification through unique markings and colouration (Martin 2005), offering demographic insights into age and sex ratios (Kays et al. 2019; Wearn et al. 2023). Future efforts will focus on developing systematic protocols to robustly estimate tree kangaroo population densities by using thermal drones.
Data availability
This research note is based on incidental observations. All relevant data have been included in the supplementary material. Additional raw observation records, images, and metadata can be made available on reasonable request to the corresponding author.
Conflicts of interest
The authors declare that there are no conflicts of interest regarding the publication of this paper.
Declaration of funding
This research did not receive any specific funding. However, funding for the drone used in this study was provided by the National Environmental Science Program (NESP) Resilient Landscapes Hub for an unrelated project on spectacled flying-foxes.
Acknowledgements
We thank Roger Martin for his valuable insights into the ecology and behaviour of Bennett’s tree kangaroos and Richard Hopkinson for generously sharing his expertise on thermal drones. The support and constructive comments of colleagues and reviewers are also gratefully acknowledged.
References
Beranek, C. T., Roff, A., Denholm, B., Howell, L. G., and Witt, R. R. (2021). Trialling a real-time drone detection and validation protocol for the koala (Phascolarctos cinereus). Australian Mammalogy 43(2), 260-264.
| Crossref | Google Scholar |
Burke, C., Rashman, M. F., Longmore, S. N., McAree, O., Glover-Kapfer, P., Ancrenaz, M., and Wich, S. A. (2019a). Successful observation of orangutans in the wild with thermal-equipped drones. Journal of Unmanned Vehicle Systems 7(3), 235-257.
| Crossref | Google Scholar |
Burke, C., Rashman, M., Wich, S., Symons, A., Theron, C., and Longmore, S. (2019b). Optimizing observing strategies for monitoring animals using drone-mounted thermal infrared cameras. International Journal of Remote Sensing 40(2), 439-467.
| Crossref | Google Scholar |
Collen, B., Ram, M., Zamin, T., and McRae, L. (2008). The tropical biodiversity data gap: addressing disparity in global monitoring. Tropical Conservation Science 1(2), 75-88.
| Crossref | Google Scholar |
IUCN (2024). The IUCN Red List of Threatened Species. Version 2024-2. https://www.iucnredlist.org. Accessed on 3 December 2024.
Kanowski, J., Hopkins, M. S., Marsh, H., and Winter, J. W. (2001). Ecological correlates of folivore abundance in north Queensland rainforests. Wildlife Research 28(1), 1-8.
| Crossref | Google Scholar |
Kanowski, J., Winter, J., Simmons, T., Tucker, N., and Rainforest CRC (2003). Conservation strategy for Lumholtz’s tree-kangaroo on the Atherton Tablelands. Ecological Management & Restoration 4, 220-221.
| Google Scholar |
Kays, R., Sheppard, J., McLean, K., Welch, C., Paunescu, C., Wang, V., Kravit, G., and Crofoot, M. (2019). Hot monkey, cold reality: surveying rainforest canopy mammals using drone-mounted thermal infrared sensors. International Journal of Remote Sensing 40(2), 407-419.
| Crossref | Google Scholar |
Krockenberger, A. K., Edwards, W., and Kanowski, J. (2012). The limit to the distribution of a rainforest marsupial folivore is consistent with the thermal intolerance hypothesis. Oecologia 168, 889-899.
| Crossref | Google Scholar | PubMed |
Laurance, W.F., and Laurance, S. G. W. (1996). Responses of five arboreal marsupials to recent selective logging in tropical Australia. Biotropica 28(3), 310-322.
| Crossref | Google Scholar |
Newell, G. R. (1999). Australia’s tree-kangaroos: current issues in their conservation. Biological Conservation 87(1), 1-12.
| Crossref | Google Scholar |
Pereyra Irujo, G. (2022). IRimage: open source software for processing images from infrared thermal cameras. PeerJ Computer Science 8, e977.
| Crossref | Google Scholar | PubMed |
Pinel-Ramos, E. J., Aureli, F., Wich, S., Longmore, S., and Spaan, D. (2024). Evaluating thermal infrared drone flight parameters on spider monkey detection in tropical forests. Sensors 24(17), 5659.
| Crossref | Google Scholar | PubMed |
Pocknee, C.A., Lahoz-Monfort, J. J., Martin, R. W., and Wintle, B. A. (2021). Cost-effectiveness of thermal imaging for monitoring a cryptic arboreal mammal. Wildlife Research 48(7), 625-634.
| Crossref | Google Scholar |
Santos, P. M., Kaizer, M. D., Moreira, D. O., Melo, F. R. D., and Mendes, S. L. (2023). Every flight is a surprise: First records of the southern maned three-toed sloth (Bradypus crinitus: Bradypodidae) through drones. Mammalia 87(3), 223-227.
| Crossref | Google Scholar |
Schindelin, J., Arganda-Carreras, I., Frise, E., Kaynig, V., Longair, M., Pietzsch, T., Preibisch, S., Rueden, C., Saalfeld, S., Schmid, B., Tinevez, J.-Y., White, D. J., Hartenstein, V., Eliceiri, K., Tomancak, P., and Cardona, A. (2012). Fiji: an open-source platform for biological-image analysis. Nature Methods 9(7), 676-682.
| Crossref | Google Scholar | PubMed |
Shima, A. L., Berger, L., and Skerratt, L. F. (2019). Conservation and health of Lumholtz’s tree-kangaroo (Dendrolagus lumholtzi). Australian Mammalogy 41(1), 57-64.
| Crossref | Google Scholar |
Underwood, A. H., Derhè, M. A., and Jacups, S. (2022). Thermal imaging outshines spotlighting for detecting cryptic, nocturnal mammals in tropical rainforests. Wildlife Research 49(6), 491-499.
| Crossref | Google Scholar |
van Berkel, T., Dahms, T., Mbende, M., Loota, J. B., and Jocqué, M. (2023). Detection of Bonobos (Pan paniscus) in tropical rainforest canopies using drone-based thermal imaging: a first step towards accurately estimating population sizes?. International Journal of Primatology 44(2), 240-244.
| Crossref | Google Scholar |
Wearn, O. R., Trinh-Dinh, H., Le, Q. K., and Nguyen, T. D. (2023). UAV-assisted counts of group size facilitate accurate population surveys of the Critically Endangered cao vit gibbon Nomascus nasutus. Oryx 58(2), 183-186.
| Crossref | Google Scholar |
Whitworth, A., Pinto, C., Ortiz, J., Flatt, E., and Silman, M. (2022). Flight speed and time of day heavily influence rainforest canopy wildlife counts from drone-mounted thermal camera surveys. Biodiversity and Conservation 31(13–14), 3179-3195.
| Crossref | Google Scholar |
Winter, J., Burnett, S., and Martin, R. (2019). Dendrolagus bennettianus. The IUCN Red List of Threatened Species 2019: e.T6426A21957127. http://dx.doi.org/10.2305/IUCN.UK.2019-1.RLTS.T6426A21957127.en