A comparison of field and genetic methods for identifying feral cat predation events during wildlife translocations
Ned L. Ryan-Schofield
A
B
C
Abstract
Translocation programs are commonly undertaken to conserve threatened species, but loss of animals to predation after release remains a significant challenge. Feral cats (Felis catus) are a major predator of many native Australian mammals and a significant contributor to translocation failure in this group. However, identifying cat predation as the cause of mortality in the field is challenging and can hinder or delay the implementation of appropriate management actions. We compared field and genetic data collected from 92 cat predation events that occurred during native mammal translocations in South Australia. Our study found that necropsy and genetic evidence obtained through DNA analysis were the most reliable methods for identifying feral cat predation. Field evidence such as cat tracks, scats or collar bite marks were recorded infrequently and inconsistently. Relying solely on field evidence will significantly underestimate predation by feral cats on translocated species and even DNA evidence was found to underestimate impact. We demonstrated the value of intensively monitoring translocated animals post-release so as to facilitate prompt collection of multiple lines of evidence such as necropsy, field evidence and reliable DNA samples. An absence of field evidence should not be used to discount cat predation as a potential cause of mortality in translocation programs.
Keywords: autopsy, bettong, bilby, caching, carcass, genetics, possum, quoll, reintroduction.
Introduction
Feral cats (Felis catus) are one of the most harmful and widespread of invasive mammalian predators (Doherty et al. 2016). In Australia, cats are considered a significant threat to Australian mammals and have been implicated in approximately two-thirds of Australian mammal extinctions (Woinarski et al. 2015; Radford et al. 2018). Cats continue to hinder recovery efforts of many threatened mammals, and have been responsible for the failure of multiple translocation programs (Short et al. 1992; Christensen and Burrows 1995; Priddel and Wheeler 2004; Hardman et al. 2016; Short 2016). The impact and management of feral cats on native mammal populations has been a topic of considerable research interest in Australia for the past 40 years (reviewed by Doherty et al. 2017 and Woinarski et al. 2019).
Cats locate prey primarily by using visual and auditory cues (Kitchener et al. 2010). Their retinas mostly comprise rod photoreceptor cells, which are more effective in low light than are cone cells (Gunter 1951; Hughes 1985). Cats also have one of the broadest hearing ranges among mammals (from 48 Hz up to 85 kHz), advantageous for localising sounds (Heffner and Heffner 1985), and so detecting prey. Like most felids, feral cats are ambush predators, employing either a ‘sit-and-wait’ strategy at heavily used sites such as burrow entrances, ready to pounce on emerging prey (Judge et al. 2012; Moseby and McGregor 2022), or locating and then slowly stalking unaware prey (Fitzgerald 2000) before making a short rush or pounce (Turner and Meister 1988). Cats may then pin their prey using their forelimbs and, after correctly positioning them, dispatch prey by delivering a bite to the back of the neck to sever the spinal cord (Adamec 1976; James et al. 2002; Fancourt 2015).
Direct evidence of impacts by feral cats has been recorded from failed translocation attempts through the deaths of radio-tracked individuals (Moseby et al. 2011; Hardman et al. 2016; Short 2016). However, difficulties in determining the cause of death on the basis of prey remains can hinder accurate assessments of cat impacts and can lead researchers to apportion deaths to other causes. Accurately identifying the cause of death at the kill site (see methods in Hardman et al. 2016; Leahy et al. 2016) is difficult. Locating radio-tracked animals promptly after death can be challenging, and delays can reduce the probability of finding predator sign, and allows time for scavenging by other species, potentially confounding evidence. Carcasses can also be dismembered during consumption and moved, leaving only the tracking device. Necropsies and recent advances in DNA testing of carcass remains have assisted researchers in identifying feral cats as a key predator in translocations (Marlow et al. 2015; Moseby et al. 2015) but these methods are costly and/or require substantial expertise, and are usually conducted using only freshly collected carcasses or transmitters. Understanding the diagnostic features of kill sites and prey carcasses may assist in correctly identifying cat predation in translocation programs. Removal of reliance on DNA would save both time and money, enabling problem predators to be quickly targeted for removal, thus benefiting translocated populations.
To better characterise any defining diagnostic features of feral cat predation events, we present data on DNA- or necropsy-confirmed cat predation events, and other deaths strongly suspected to be cat predation, by using data from translocations of four native mammals. We investigate carcass remains, caching events, predator sign, and habitat and location features of carcass sites. Our results will assist in determining whether field evidence can accurately predict cat predation events and provide insight into how cats hunt mammalian prey in arid and semi-arid Australia.
Methods
Study sites and species
Data for this study were collected between 2014 and 2019 from two sites in South Australia. The Ikara-Flinders Ranges National Park (IFRNP) is a 93,812 ha unfenced semi-arid conservation reserve 400 km north of Adelaide (31.5286°S, 138.6036°E). The elevation of the ranges produces higher rainfall (444 mm/year) than in the surrounding arid plains, and a variety of habitats are present including river red gum (Eucalyptus camaldulensis) woodlands and creeklines, mixed native pine (Callitris glaucophylla) and gum coolibah (E. intertexta) woodlands, shrubland (Acacia and Allocasuarina spp.), and mallee (Eucalyptus spp.) (Brandle 2001; Moseby et al. 2020). Introduced red foxes (Vulpes vulpes) have been successfully controlled since the mid-1990s (Brandle et al. 2018), and detection rates on remote cameras averaged 0.005% during the study (Moseby et al. 2020). Both western quolls (Dasyurus geoffroii) and brushtail possums (Trichosurus vulpecula) were locally extinct, and were translocated to the park between 2014 and 2018 (Moseby et al. 2020, 2021). Western quolls, hereafter quolls, are a nationally threatened carnivorous marsupial. Quolls are nocturnal, sheltering during the day in hollow logs, burrows and under rock crevices. Brushtail possums, hereafter possums, are a nocturnal, semi-arboreal marsupial that shelters predominantly in tree hollows.
The second study site, Arid Recovery (AR), is a 123 km2 conservation reserve in South Australia’s arid zone (30.37144°S, 136.90409°E), north-west of IFRNP, made up of six paddocks surrounded by a predator-exclusion fence (Moseby and Read 2006). The climate is arid with hot dry summers and mild winters. The long-term average annual rainfall is 144.5 mm (Records from Olympic Dam Aerodrome, www.bom.gov.au/climate/data, accessed March 2022). The habitat is dominated by longitudinal dunes separated by interdunal clay swales, with a small number of minor ephemeral creek lines. Dunes support sparse Acacia and Dodonaea shrubland, whereas swales are covered in low (~50 cm) chenopod (Maireana spp. and Atriplex spp.) shrubs. Burrowing bettongs (Bettongia lesueur) and greater bilbies (Macrotis lagotis) were translocated in 2000 and 2001 respectively (Moseby and O’Donnell 2003; Moseby et al. 2011). Burrowing bettongs, hereafter bettongs, are nocturnal burrowing potoroids, that live communally in warrens (Sander et al. 1997), whereas greater bilbies, hereafter bilbies, are solitary, fossorial nocturnal bandicoots. In 2015, both species were translocated to one of the experimental paddocks, the ‘Predator Paddock’, where feral cats were present at low densities (0.11–0.41/km2), but foxes were excluded (Bannister et al. 2021). Bilbies from both the feral-free and cat-exposed Predator Paddock were then translocated to a second experimental paddock, the 37 km2 ‘Release Paddock’, in 2017 (Ross et al. 2019). Bettongs were released using the same method into the Release Paddock in both 2017 and 2018 (Bannister et al. 2021).
Translocations
In total, 93 quolls were translocated to the IFRNP from 2014 to 2016, of which 86 were radio-collared. An additional 17 subadults were collared in the IFRNP in December 2014 (further details of post-release monitoring are given in Moseby et al. 2021).
In 2015 and 2016, 148 possums were translocated to the IFRNP. Sixty-eight were fitted with VHF or GPS radiocollars. A further 26 juveniles were fitted with lightweight radiocollars on their first capture after exiting the pouch (further details of the release and post-release monitoring are given in Bannister et al. 2019, and Moseby et al. 2020).
In November 2017, 39 burrowing bettongs fitted with VHF or GPS radiocollars were translocated from the feral-free paddocks (n = 20) and cat-exposed (n = 19) Predator Paddock of the Arid Recovery Reserve to the Release Paddock. In November 2018, 50 bettongs (feral-free n = 25, cat-exposed n = 25) from the same source populations were again translocated to the Release Paddock (further details are given in Bannister et al. 2021).
In June 2017, 42 bilbies were captured in the feral-free (n = 21) and cat-exposed (n = 21) paddocks by using spotlights and handheld nylon fishing nets as per Moseby et al. (2012), and had VHF transmitters attached to shaved areas on the tail. The bilbies were then translocated to the Release Paddock and were tracked daily for up to 40 days (further details are given in Ross et al. 2019).
Data collection
All transmitters were equipped with a mortality mode that activated after 10 h of inactivity. Any transmitters detected in mortality triggered an immediate ground search to locate the carcass. Once found, the transmitter site was searched for any predator sign such as tracks or scats, and any carcass remains. We also noted whether the carcass appeared to have been moved and/or scavenged by birds of prey. DNA swab samples were taken from radiocollars and carcass puncture wounds or saliva marks on the fur by using a cotton swab dipped in Tissue Digest (DXT; QIAGEN), before being stored in the freezer.
Samples from 11 Quolls released in 2014 were sent to the Wildlife Genetics Lab (Institute for Applied Ecology, University of Canberra, ACT, Australia). Species identification was conducted using universal mtDNA primers CB-J-10612 and CB-N-10920 targeting a highly conserved region of the cytochrome b gene. Detailed methods for extraction, amplification, and sequencing are given in Moseby et al. (2015).
Swabs from all other reintroduced animals were sent to Helix Molecular Solutions (Perth, WA, Australia). All samples were extracted using the QIAamp Fast DNA Stool Mini kit (QIAGEN, Hilden, Germany), as per manufacturers protocols. All samples were tested for potential predator DNA by using the species identification primers and protocols developed by Berry and Sarre (2007). This real-time PCR approach tests for the presence of fox, cat, dog (Canis familiaris) and quoll (Dasyurus sp.) DNA. Each 25 µL reaction consisted of 12.5 µL iTaq Universal SYBR green supermix (Bio-Rad), 0.4 µM of each primer and 5 µL of DNA and was run on a QuantStudio 3 Real-Time PCR system (Thermo Fisher Scientific). A positive amplification had to meet limits of detection and cycling thresholds.
After being swabbed, carcasses were placed into sealed plastic bags and stored in the freezer. If IFRNP animal carcasses were fresh and transport was available, they were sent for necropsy by experienced wildlife veterinarians at the Adelaide Zoo (Zoos SA). During necropsy, veterinarians examined animal hydration, muscle mass and fat stores, looking externally and internally for signs of trauma. Where signs of haemorrhage and muscle damage were evident, size, spacing, and location of puncture wounds and/or lacerations, if present, were used to determine whether cat predation was likely. Stomach contents were also examined for evidence of meat baits that may have caused 1080 poisoning. Histopathology was not conducted because all carcasses were slightly to heavily autolysed.
Deaths were recorded as either confirmed cat predation (using necropsy, direct observation of cats on fresh carcasses or by DNA analysis of carcass swabs), strongly suspected cat predation (no DNA swab or necropsy but other evidence such as bite marks on collars similar to those recorded in confirmed cat predation cases), or other causes.
Photographs were taken of the carcass in situ, or the carcass was collected and photographs were taken in a laboratory after the carcass was frozen. The state of the carcass (whole and uneaten; partially consumed, some flesh eaten but body parts still joined by skin/spine; partially consumed, dismembered and dispersed across kill site, not joined; dismembered, hindquarters with tail found uneaten; dismembered, only front of snout/jaw found, brain eaten; almost completely consumed, bones picked clean; likely totally consumed, no remains found, VHF transmitter only; carcass whole, only pouch and viscera eaten), and the major body parts of the animal that appeared to have been consumed were noted (back, forelegs, hindlegs, neck, organs). Differences between the feeding habits of cats were compared among species by using individual Fisher’s exact tests.
The microhabitat at the carcass site was also recorded (in open, under vegetation, under rocks, in logs), and compared among prey species by using individual Fisher’s exact tests.
For confirmed and suspected cat predation events of bettongs, quolls, and possums, the macrohabitat type at the carcass site was recorded (AR = dune, dune edge, creek line, swale, sandplain, IFRNP = Callitris woodland, eucalypt woodland, mallee woodland, mixed woodland, E. camaldulensis creek line). No macrohabitat data were recorded for bilbies; so, this species was excluded from further analysis.
The macrohabitat type of all known prey shelter sites was recovered from radiotracking records. To determine whether carcasses were found in habitats similar to those used by prey for shelter sites, we compared the proportion of different carcass macrohabitats with the proportion of diurnal shelter site macrohabitats by using individual Fisher’s exact tests.
The GPS location of the remains of each carcass was recorded, and radiotracking records used to determine the distance from the carcass location to the closest known shelter site of that prey individual.
We compared the distance of carcasses from closest known shelter site to the frequency distribution of prey movement distances from shelter sites by obtaining GPS data from a sample of 11 bettongs and 12 possums tracked during the project. Data were filtered to dilution of precision (DOP) of <5, and any data in the first 3 weeks after animals were released were excluded because home ranges were still being established. For each GPS fix, the distance to the closest recorded den site for that individual was calculated. These data were compared with the frequency of carcass location distances from shelter sites to determine whether cats were killing prey at shelter sites, or during normal foraging behaviour. Kolmogorov–Smirnov tests were used to test for differences between the empirical cumulative distribution function (ECDF) of the distance of GPS fixes to an individual’s closest known den site and the ECDF of the distances from closest known den sites to the locations where carcasses were recovered.
All statistical analyses were conducted in R (ver. 4.3.2; www.r-project.org, accessed March 2024).
Results
Evidence of predation
During the translocations of 389 individuals of four species at two locations between 2014 and 2019, most cat predation events were confirmed using DNA evidence obtained by swabbing radiocollars and wounds found on fresh carcasses (71 of 74, 96% of all confirmed cat kills). Six animals were confirmed killed by cats by using necropsy by qualified veterinarians, but five of these also returned positive DNA results. Cats were sighted at three freshly killed carcasses, with cat DNA being detected on only one carcass. Of the 74 animals confirmed by these three methods, nearly half (27, or 44% of animals carrying collars) had bitemarks on their radiocollars (bilbies were excluded because they carried tail transmitters). As such, we concluded that a further 18 animals were strongly suspected of being killed by cats despite the lack of genetic or other conclusive evidence, owing to the presence of similar bite marks. Because bitemarks are often used to diagnose cat predation (e.g. James et al. 2002), we included these 18 animals in further analysis. The presence of field sign at the carcass sites (cat tracks or scats) was not a reliable indicator of cat predation and was recorded for only 18 of the 92 animals (Table 1). Only samples from the first 11 quolls that died in the IFRNP in 2014 were assessed using universal mtDNA primers. No other species were identified from these swabs, as detailed in Moseby et al. (2015).
Item | Bettong | Bilby | Possum | Quoll | |
---|---|---|---|---|---|
Confirmed cat predation | 42 | 13 | 9 | 10 | |
DNA evidence | 42 | 13 | 7 | 9 | |
Necropsy | 0 | 0 | 3 A | 3 B | |
Cat sighted on carcass | 0 | 0 | 2 C | 1 | |
Bitemarks on collar/carcass | 18 | N/A D | 3 | 6 | |
Cat tracks/scat at carcass location | 9 | 5 | 0 | 0 | |
Suspected cat predation (bitemarks only) | 5 | N/A D | 3 | 10 | |
Bitemarks on collar/carcass | 5 | N/A D | 3 | 10 | |
Cat tracks/scat at carcass location | 1 | 0 | 0 | 3 | |
Total sample of animals | 47 | 13 | 12 | 20 |
Feeding habits of cats
Between 6.4% and 30% of carcasses were found intact and uneaten (Table 2, e.g. Fig. 1a), but this was not significantly different among prey species (P = 0.0853 Fisher’s exact test, hereafter omitted). All three uneaten bettongs were found down a warren or at a warren entrance, potentially representing animals that escaped a predation event but later expired.
Item | Bettong (n) | Bilby (n) | Possum (n) | Quoll (n) | |
---|---|---|---|---|---|
Carcass characteristics | |||||
Carcass whole and uneaten | 3 | 4 | 2 | 2 | |
Carcass partially consumed, some flesh eaten but body parts still joined by skin/spine | 24 | 2 | 2 | 3 | |
Carcass partially consumed, dismembered and dispersed across kill site (not joined) | 4 | 1 | 2 | 4 | |
Carcass dismembered, hindquarters with tail found uneaten | 3 | 5 | 1 | 0 | |
Carcass dismembered, only front of snout/jaw found (brain eaten) | 0 | 1 | 3 | 4 | |
Carcass almost completely consumed, bones picked clean | 3 | 0 | 0 | 0 | |
Carcass likely totally consumed (no remains found, VHF transmitter only) | 10 | 0 | 2 | 6 | |
Carcass whole, only pouch and viscera eaten | 0 | 0 | 0 | 1 | |
Pulled fur at kill site | 7 | 4 | 1 | 6 | |
Scavenging | |||||
Carcass moved/scavenged by bird of prey | 7 | 0 | 1 | 0 | |
Caching | |||||
Sticks/leaves partially covering carcass | 11 | 2 | 0 | 0 | |
Down burrow | 0 | 0 | 0 | 1 | |
In hollow log/base of E. camaldulensis | 0 | 0 | 3 | 0 | |
Animals confirmed/suspected as killed by cats | 47 | 13 | 12 | 20 |
Examples of some characteristic features of feral cat predation. (a) Dead possum found unconsumed, (b) burrowing bettong carcass mostly eaten but remains still joined by skin and spine, (c) western quoll almost entirely ingested apart from snout/jaws, and (d) bilby hindlegs and snout uneaten by feral cat.
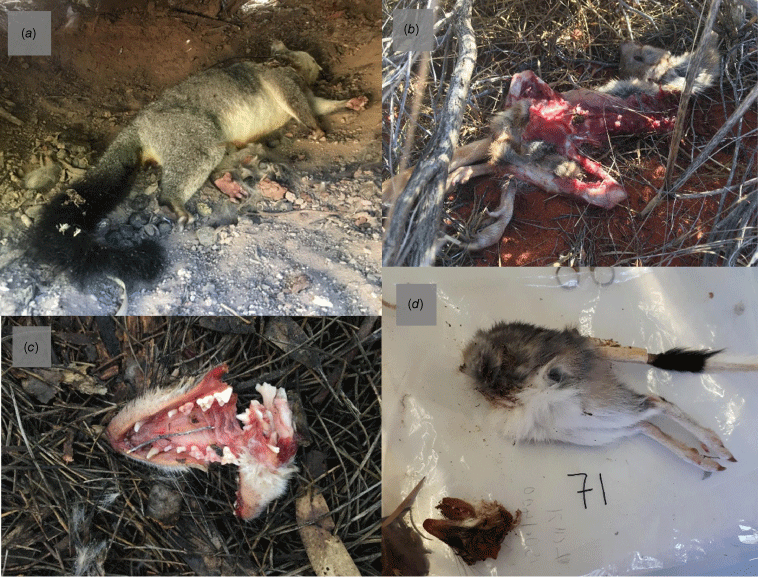
Carcasses were frequently recovered with the flesh from the limbs and abdomen partially or completely removed, but the carcass still articulated, with the head and legs/tail remaining, joined by the skin or spine rather than separated into pieces (Fig. 1b). All species were eaten in this manner (Table 2), but it was significantly more common for bettong predation events (P = 0.0004, OR = 5.55, CI = 1.94–17.8). Cats were significantly more likely to leave almost nothing, but the snout or front of the skull (e.g. Fig. 1c) of quolls, bilbies, and possums relative to bettongs (P = 0. 0023, OR = 0, CI = 0.00–0.50). The hindlegs of bilbies were also disarticulated and left uneaten (e.g. Fig. 1d) significantly more frequently than those of other species (P = 0.0024, OR = 11.16, CI = 1.98–69.2). Furthermore, the recovery of body parts of prey was not guaranteed. In 19.5% of predation events, no remains were found, and only the collar was recovered, although this did not differ significantly (P = 0.1574) among species.
Although feeding sign differed in some respects among species, generally all major body parts appeared to be eaten with similar frequency (Fig. 2). No significant preference by cats for any part of the carcass was found for bettongs (P = 0.9807), bilbies (P = 0.1665), possums (P = 1), or quolls (P = 0.7915).
Microhabitat of carcass locations
To investigate the microhabitats where carcasses were found, any scavenged animals and those with missing data were filtered from the dataset, leaving 40 bettongs, 8 bilbies, 10 possums, and 20 quolls. No differences were found in the frequency that carcasses were located out in the open (P = 0.5313), or beneath vegetation (P = 0.0966) (Fig. 3). Three possums were also found in hollow logs and five quoll carcasses were located in rocky crevices or beneath overhangs, whereas five bettongs were found at warren entrances, and one quoll was found down a warren.
Proportion of carcasses of burrowing bettongs (n = 40), greater bilbies (n = 8), brushtail possums (n = 10), and western quoll (n = 20) known to be killed by feral cats found in different microhabitats at carcass locations. Beneath vegetation includes under bushes, shrubs, or larger trees. Animals suspected of being scavenged or with missing data were removed from analysis.
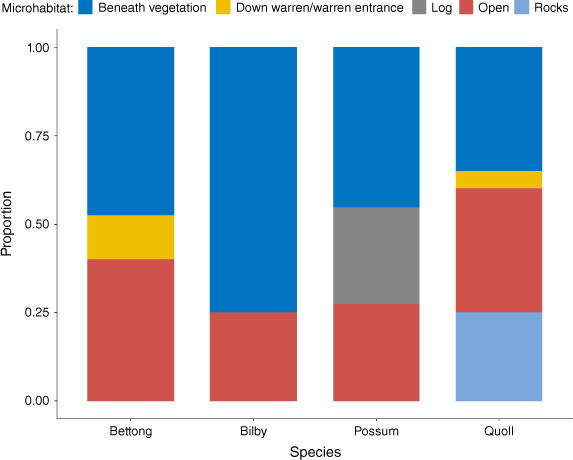
For bettongs and bilbies, the vegetation microhabitat at the kill site was commonly small shrubs, both alive and dead, such as Dodonaea viscosa, Acacia ligulata, or Acacia tetragonophylla. They were also commonly recovered beneath piles of dead mulga branches on the ground (Acacia aneura). Possums and quolls were more regularly located beneath larger trees in IFRNP (e.g. Callitris glaucophylla, Eucalyptus camaldulensis, Eucalyptus intertexta).
Macrohabitat of kill sites compared with diurnal shelter sites
Macrohabitat was recorded at 786 diurnal shelter sites of 45 bettongs (mean number of records per individual = 6.6, s.d. = 4.3), 12 possums (mean records = 35.8, s.d. = 40.9) and 16 quolls (mean records 4.1, s.d. = 3.9) confirmed or suspected of being killed by feral cats. Macrohabitat data from carcass locations were also available for 47 bettongs, 10 possums, and 10 quolls. No macrohabitat data were recorded for bilbies; so, this species was excluded from further analysis.
We found significant differences between the macrohabitat type at carcass locations and the habitat type at shelter sites used by prey species (Fig. 4). The majority of the bettongs’ known den sites were in warrens on dune edges (175, 58%); however, significantly fewer carcasses (8, 17%) were recovered from this habitat type than from other habitats (P = <0.0001, OR = 0.146, CI = 0.057–0.331). Bettong carcasses were more likely to be found in a variety of other habitats less frequently used for diurnal shelter sites, such as sandplains (P = 0.0002, OR = 34.8, CI = 3.77–1665.9) or swales (P = <0.0001, OR = 6.43, CI = 2.53–16.11). There was no difference in the frequency of carcass occurrence in either dry creek lines (P = 0.184), or on dunes (P = 0.6003), from the frequency of den sites in these habitats.
Macrohabitats of kill sites and all known den sites for burrowing bettongs (n = 46), brushtail possums (n = 10), and western quoll (n = 10) known to be killed by feral cats at both field sites, Arid Recovery (AR) and Ikara-Flinders Ranges National Park (IFRNP).
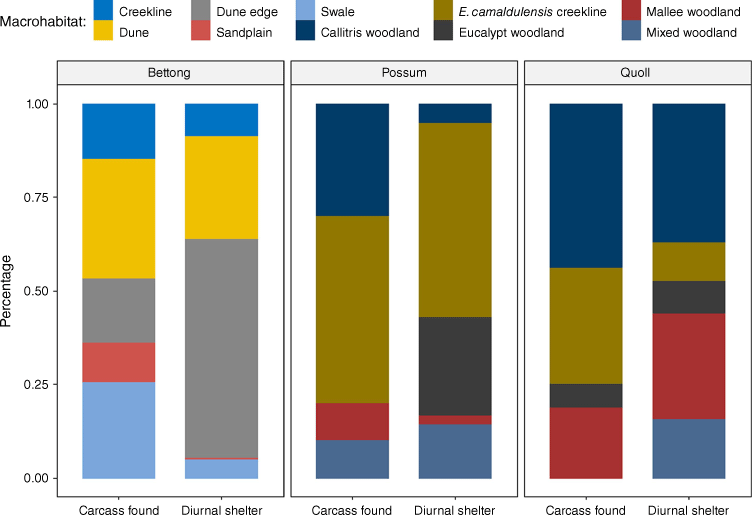
Similarly, 30% of possum carcasses were found in Callitris woodlands, despite only 5.1% of diurnal shelter sites being recorded in this habitat (P = 0.0149, OR = 7.86, CI = 1.23–37.5), while 36% of quolls were found dead in E. camaldulensis creek lines, while only sheltering there 10% of the time (Fig. 4), although this was not quite statistically significant (P = 0.055), possibly due to small sample size.
Distance animals were found from diurnal shelter sites
Carcasses were generally not found at known diurnal shelter sites. Of the 92 carcasses of animals killed by cats that were recovered, 76 had location data for both kill sites and diurnal shelter sites.
Only 13% (2 bettongs, 1 bilby, 6 possums, and 1 quoll) were found dead less than 50 m from a known shelter site. Two possums and one bettong were found dead at a diurnal shelter site where they were previously recorded. The bettong was found alive but later expired, likely indicating a predation escapee. The average distance from bettong and quoll carcasses to their closest known den site (903 m and 1741 m respectively), was greater than the average diameter of the species’ home ranges, in contrast to possums (132 m) and bilbies (269 m) where the average carcass distance was similar to their home range diameter, at least in females (Table 3).
Species | Mean (m) | s.d. (m) | Median (m) | IQR (m) | Home range average linear D (m) A | n | |
---|---|---|---|---|---|---|---|
Bettong | 903 | 1338 | 403 | 840 | 192.14–211.1 B | 45 | |
Bilby | 269 | 191 | 298 | 300 | f = 270, m = 1131.6 C | 7 | |
Possum | 132 | 282 | 26.9 | 73.5 | f = 165.5, m = 242.01 D | 11 | |
Quoll | 1741 | 1943 | 930 | 1877 | f = 558.5, m = 1880.7 E | 13 |
Home range estimates derived from studies conducted at Arid Recovery and Ikara-Flinders Ranges National Park. IQR, interquartile range.
When GPS data were used to compare foraging distances from known shelter sites with carcass distances from shelter site, we found that bettong carcasses were more likely to be located at the edges of their foraging distribution, unlike possums, which were more likely to be found dead close to their den sites. The empirical cumulative distribution function (ECDF) of the distance from bettong carcass locations to their nearest known den site lies below the ECDF of distance from all GPS points to nearest den sites (D− = 0.2915, P = 0.0006, asymptotic two-sample Kolmogorov–Smirnov test, hereafter KST), indicating that bettong carcasses were more often found towards the perimeter of the normal bettong foraging distribution (i.e. towards the edge of their home range). Conversely, the ECDF of distances from possum carcasses to nearest known den sites lies above the ECDF of distance from all GPS points to nearest den sites (D+ = 0.4364, P = 0.0158, KST), indicating that carcasses were more frequently found in areas closer to their den sites than was expected from the time they spent foraging in these locations.
Discussion
Loss of animals to predation is a common challenge in many translocation programs, especially in Australia (Moseby et al. 2011). Owing to the remoteness of many study sites and the delay in receiving DNA swab results, confirming cat predation by using field evidence would assist in mounting a rapid management response. However, our study found that necropsy and genetic evidence obtained through DNA analysis were the most reliable methods for identifying predation. Field evidence such as cat tracks or scats, collar bite marks, carcass remains or location were recorded inconsistently and/or varied widely. Although not a rapid field assessment method, DNA evidence has been used successfully to identify the cause of death in radiotracking survival studies in Australia (Glen et al. 2010; Marlow et al. 2015; Moseby et al. 2015) and overseas (Wengert et al. 2014; Forrester and Wittmer 2019; Peelle et al. 2019; Ganz et al. 2023). DNA evidence can be easily acquired in the field by swabbing any visible wounds or collar bite marks and storing for later analysis. However, genetic evidence is not infallible, and carcass swabs may not always detect cat DNA owing to operators introducing contamination, insufficient saliva deposition during the kill, total consumption of carcasses and/or degradation of DNA over time. For example, of the three predated animals where cats were physically seen feeding on carcasses in this study, cat DNA was detected only on one animal, suggesting that DNA results are also likely to underestimate the impact of cat predation. A key limitation of this method is the need to locate and sample the carcass while fresh because DNA from saliva degrades quickly over time in the environment (Burrows et al. 2017). Further research should be conducted on the optimum method of obtaining DNA samples from carcasses of various ages considering the relatively fast degradation of DNA, and intensive monitoring is required to determine whether cat predation is the cause of post-release mortalities.
Previous studies have relied on field evidence such as cat tracks at carcasses, decapitation of carcasses, and/or removal of flesh from the skin to diagnose cat predation, and so an absence of this evidence has led to conclusions that cats were not responsible for predation events (Gibson et al. 1994; Christensen and Burrows 1995; Short and Turner 2000; Hardman et al. 2016). For instance, Short and Turner (2000) did not attribute the cause of death to cats if a carcass was recovered from a warren, or if an intact carcass was recovered from under a dense bush with no signs of struggle or broken limbs. However, our data demonstrated that feral cats do not always consume prey, and frequently do not leave visible signs of predation. Similarly, McGregor et al. (2015) found that feral cats did not consume 28% of the prey they killed. Other predator species such as dingoes (Canis familiaris), black bears (Ursus americanus), bobcats (Lynx rufus), mountain lion (Puma concolor), coyotes (Canis latrans), and wolves (C. lupus) can also leave little field evidence, with predation confirmed only through necropsy, stomach analysis or DNA evidence (Moseby et al. 2011; Ganz et al. 2023).
More than half of carcasses were recovered from under vegetation, but we found no evidence of dragging of carcasses by feral cats to shelter sites, despite this behaviour being recorded in other studies (Gibson et al. 1994). Although carcasses may have been carried without leaving field sign, Moseby and McGregor (2022) found that cats at both of our study sites (AR and IFRNP) spent significantly more time at microsites with high vegetation cover to increase their chances of affecting an ambush. This suggests that prey found under vegetation in our study may have been killed and consumed in close proximity. Additionally, 38% of bettongs, possums, and quolls were eaten in the open, away from any vegetation, suggesting that most animals were eaten at or close to the kill site.
We found 17 instances of caching carcasses, including all four reintroduced species. Caching has been widely described in larger felids such as leopards (Panthera pardus) (De Ruiter and Berger 2001), European lynx (Lynx lynx) (Červený and Okarma 2002), and mountain lion (Akenson et al. 2003), but only more recently in smaller felids such as sand cats (Felis margarita) (Brighten and Burnside 2019), black-footed cats (Felis nigripes) (Sliwa 1994), and European wildcat (Felis silvestris) (Ruiz-Villar et al. 2020; Krofel et al. 2021). Caching by feral cats has been documented (Gibson et al. 1994), but is more commonly reported in foxes (Saunders et al. 1999; Tobajas and Díaz‐Ruiz 2022). Caching is thought to occur when prey items are too big to be eaten in a single day (Bischoff-Mattson and Mattson 2009), but in our study prey were commonly less than 1.5 kg.
We found almost no evidence that cats caught or killed prey down burrows or inside shelter sites. Our results suggest that cats hunted prey when they were active at night and caught bettongs, quolls and bilbies away from the immediate vicinity of their shelter sites. In addition, cats frequently killed prey in habitats not used as diurnal shelter sites. This suggests that cats were either not employing a sit and wait strategy immediately outside prey shelter sites, or prey species were warier as they left or entered shelter sites and were thus harder to catch. Social prey species such as Barbary ground squirrels (Atlantoxerus getulus) increase their collective high-quality vigilance as group size increases (van der Marel et al. 2019). Bettongs are also a social species and build complex warren systems that house an average of four to five individuals (Moseby et al. 2018). Bettongs use alarm calls in response to external stimuli to alert conspecifics prior to fleeing (Trenwith et al. 2023). Moseby and McGregor (2022) found that cats learn and focus their hunting behaviour in areas with strong prey cues such as multi-entrance bettong warrens, which is likely to increase their chances of encountering prey at higher densities. Despite the likely increased hunting by cats at shelter sites, bettong carcasses were found more often away from warrens at the edges of their foraging distribution. These results could indicate that group vigilance of bettongs at warrens moderates cat hunting success, and bettongs are more vulnerable when they are more likely to be alone or in smaller groups on the edge of their foraging distribution. Quoll and bilby carcasses were also predominantly found away from den sites; however, they are solitary and move shelter sites frequently, rather than living communally. This strategy may make it difficult for cats to locate their shelter sites, resulting in reduced predation at these locations. In contrast, possums do not live communally (Rouco et al. 2018), and were found dead closer to their shelter sites than was expected relative to their foraging distribution. Male possums scent mark (Hynes 1999), and possums are easily visible when climbing trees to enter and leave hollows; so, cats may learn the location of possum shelter sites and focus their hunting in this vicinity. Without group vigilance to reduce predation likelihood, cats may be more successful when hunting at possum shelter sites.
Limitations
Although we thoroughly searched the transmitter site for remains, 19.5% of the time, only the transmitter was found. Cats are known to decapitate prey (Gibson et al. 1994; Christensen and Burrows 1995); therefore, some prey remains could have been undetected, and either discarded at a distance from the collar site or cached as was found in 17 other animals. Our results suggest that feral cats do cache, and this may hinder identification of the cause and location of death if the radiotransmitter is detached from the carcass.
Foxes, which are a significant threat to Australian mammals (Radford et al. 2018), were absent from our field sites, and so we could not compare field and genetic methods for identifying fox predation events. Future research should address this knowledge gap.
Eight carcasses were thought to have been scavenged and/or moved by birds of prey, primarily wedge-tailed eagles (Aquila audax), and bettong carcasses. This could be an underestimate, as scavenging by eagles was not tested using DNA as part of this study. More extensive movement or scavenging of carcasses could have hidden or masked any patterns of consumption by feral cats.
Conclusions
Identifying predation and the species responsible is a critical aspect of translocation programs. Finding cached remains should not be used to discount cat predation as both cats and foxes cache carcasses. Our study highlighted that necropsy and genetic evidence are the most reliable methods for identifying feral cat predation, whereas field evidence such as tracks or scats were recorded infrequently. Our data demonstrated that feral cats often do not consume all the mammals they kill, and frequently do not leave visible signs of predation. Relying solely on field evidence will significantly underestimate the impact of feral cats on translocated species and even DNA evidence alone may underestimate impact. We urge conservation practitioners to intensively monitor post-release, use necropsy and DNA evidence to determine the cause of death, and consider cat predation as a possible cause of death in translocation programs, even when field evidence is absent.
Data availability
Data used in this paper is available at https://github.com/nedschofield/carcass_characteristics.
Declaration of funding
Data collection for this study was supported by ARC Linkage Grants (LP130100173 and LP160100270), FAME, the Foundation for Australia’s Most Endangered species, with other resourcing from the South Australian Department for Environment and Water, the Holsworth Wildlife Research Endowment, Nature Foundation South Australia, and the Field Naturalists Society of South Australia (Lirabenda Endowment Fund), with extensive inkind support provided by Arid Recovery. Arid Recovery is a conservation and research organisation supported by BHP Billiton, The University of Adelaide, The SA Department for Environment and Water, and Bush Heritage Australia. N. L. Ryan-Schofield and H. L. Bannister acknowledge the support they received through the provision of University of Adelaide Research Scholarships.
Acknowledgements
Ecological Horizons Pty Ltd environmental consultancy coordinated the possum and quoll translocation programs. We thank R. West, K. Tuft, L. Falls, P. Hodgens, A. Rogers, M. Jensen, F. Bernhardt, H. McGregor, M. Young, N. Beerkens, Z. Richardson J. Crompton, J. Ross, D. Williams, E. Griffith, R. Shepherd, M. Hyatt, T. Mooney, T. Moyle, P. Mitchell, M. Henderson, S. Dorries, K. Smith, M. Le Pla, B. Philp, H. Crisp, N. Riessen, M. Farrelly, D. Roshier, K. Bellchambers, and W. Boardman for their assistance on the projects from which we acquired data for this study. We acknowledge the Kokatha and Adnyamathanha peoples on whose unceded lands these projects took place.
Author contributions
All authors conceived the ideas and designed methodology; KEM, HLB and AR collected the data; NLRS analysed the data; NLRS led writing of the manuscript; and all authors contributed critically to the drafts and gave final approval.
References
Adamec, R. E. (1976). The interaction of hunger and preying in the domestic cat (Felis catus): an adaptive hierarchy? Behavioral Biology 18, 263-272.
| Crossref | Google Scholar | PubMed |
Bannister, H. L., Hodgens, P., and Moseby, K. E. (2019). Offspring sex and maternal effects influence the development and natal dispersal of an arboreal marsupial. Journal of Mammalogy 100, 423-434.
| Crossref | Google Scholar |
Bannister, H. L., Letnic, M., Blumstein, D. T., and Moseby, K. E. (2021). Individual traits influence survival of a reintroduced marsupial only at low predator densities. Animal Conservation 24, 904-913.
| Crossref | Google Scholar |
Berry, O., and Sarre, S. D. (2007). Gel‐free species identification using melt‐curve analysis. Molecular Ecology Notes 7, 1-4.
| Crossref | Google Scholar |
Bischoff-Mattson, Z., and Mattson, D. (2009). Effects of simulated mountain lion caching on decomposition of ungulate carcasses. Western North American Naturalist 69, 343-350.
| Crossref | Google Scholar |
Brandle, R., Mooney, T., De Preu, N., Garnett, S., Latch, P., Lindenmayer, D., and Woinarski, J. (2018) Broadscale feral predator and herbivore control for yellow-footed rock-wallabies Petrogale xanthopus ssp. xanthopus: improved resilience for plants and animals. Bounceback. In ‘Recovering Australian Threatened Species: a Book of Hope’. (Eds S Garnett, P Latch, D Lindenmayer, J Woinarski.) pp. 135–146. (CSIRO Publishing: Melbourne, Vic, Australia.)
Brighten, A. L., and Burnside, R. J. (2019). Insights into the feeding ecology of and threats to Sand Cat Felis margarita Loche, 1858 (Mammalia: Carnivora: Felidae) in the Kyzylkum Desert, Uzbekistan. Journal of Threatened Taxa 11, 13492-13496.
| Crossref | Google Scholar |
Burrows, A. M., Ristow, P. G., and D’Amato, M. E. (2017). Preservation of DNA from saliva samples in suboptimal conditions. Forensic Science International: Genetics Supplement Series 6, E80-E81.
| Google Scholar |
Červený, J., and Okarma, H. (2002). Caching prey in trees by Eurasian lynx. Acta Theriologica 47, 505-508.
| Crossref | Google Scholar |
De Ruiter, D. J., and Berger, L. R. (2001). Leopard (Panthera pardus Linneaus) cave caching related to anti‐theft behaviour in the John Nash Nature Reserve, South Africa. African Journal of Ecology 39, 396-398.
| Crossref | Google Scholar |
Doherty, T. S., Glen, A. S., Nimmo, D. G., Ritchie, E. G., and Dickman, C. R. (2016). Invasive predators and global biodiversity loss. Proceedings of the National Academy of Sciences 113, 11261-11265.
| Crossref | Google Scholar | PubMed |
Doherty, T. S., Dickman, C. R., Johnson, C. N., Legge, S. M., Ritchie, E. G., and Woinarski, J. C. (2017). Impacts and management of feral cats Felis catus in Australia. Mammal Review 47, 83-97.
| Crossref | Google Scholar |
Fancourt, B. A. (2015). Making a killing: photographic evidence of predation of a Tasmanian pademelon (Thylogale billardierii) by a feral cat (Felis catus). Australian Mammalogy 37, 120-124.
| Crossref | Google Scholar |
Finlayson, G. R., and Moseby, K. E. (2004). Managing confined populations: the influence of density on the home range and habitat use of reintroduced burrowing bettongs (Bettongia lesueur). Wildlife Research 31, 457.
| Crossref | Google Scholar |
Forrester, T. D., and Wittmer, H. U. (2019). Predator identity and forage availability affect predation risk of juvenile black-tailed deer. Wildlife Biology 2019, 1-12.
| Crossref | Google Scholar |
Ganz, T. R., DeVivo, M. T., Reese, E. M., and Prugh, L. R. (2023). Wildlife whodunnit: forensic identification of predators to inform wildlife management and conservation. Wildlife Society Bulletin 47, e1386.
| Crossref | Google Scholar |
Gibson, D., Lundie-Jenkins, G., Langford, D., Cole, J., and Johnson, K. (1994). Predation by feral cats, Felis catus, on the rufous hare-wallaby, Lagorchestes hirsutus, in the Tanami Desert. Australian Mammalogy 17, 103-107.
| Crossref | Google Scholar |
Glen, A. S., Berry, O., Sutherland, D. R., Garretson, S., Robinson, T., and De Tores, P. J. (2010). Forensic DNA confirms intraguild killing of a chuditch (Dasyurus geoffroii) by a feral cat (Felis catus). Conservation Genetics 11, 1099-1101.
| Crossref | Google Scholar |
Gunter, R. (1951). The absolute threshold for vision in the cat. The Journal of Physiology 114, 8-15.
| Crossref | Google Scholar | PubMed |
Hardman, B., Moro, D., and Calver, M. (2016). Direct evidence implicates feral cat predation as the primary cause of failure of a mammal reintroduction programme. Ecological Management & Restoration 17, 152-158.
| Crossref | Google Scholar |
Heffner, R. S., and Heffner, H. E. (1985). Hearing range of the domestic cat. Hearing Research 19, 85-88.
| Crossref | Google Scholar | PubMed |
Hughes, A. (1985). New perspectives in retinal organisation. Progress in Retinal Research 4, 243-313.
| Crossref | Google Scholar |
James, H., Acharya, A., Taylor, J., and Freak, M. (2002). A case of bitten bettongs. The Journal of Forensic Odonto-Stomatology 20, 10-12.
| Google Scholar | PubMed |
Judge, S., Lippert, J. S., Misajon, K., Hu, D., and Hess, S. C. (2012). Videographic evidence of endangered species depredation by feral cat. Pacific Conservation Biology 18, 293-296.
| Crossref | Google Scholar |
Krofel, M., Južnič, D., and Allen, M. L. (2021). Scavenging and carcass caching behavior by European wildcat (Felis silvestris). Ecological Research 36, 556-561.
| Crossref | Google Scholar |
Leahy, L., Legge, S. M., Tuft, K., McGregor, H. W., Barmuta, L. A., Jones, M. E, and Johnson, C. N. (2016). Amplified predation after fire suppresses rodent populations in Australia’s tropical savannas. Wildlife Research 42, 705-716.
| Crossref | Google Scholar |
Marlow, N. J., Thomas, N. D., Williams, A. A., Macmahon, B., Lawson, J., Hitchen, Y., Angus, J., and Berry, O. (2015). Cats (Felis catus) are more abundant and are the dominant predator of woylies (Bettongia penicillata) after sustained fox (Vulpes vulpes) control. Australian Journal of Zoology 63, 18-27.
| Crossref | Google Scholar |
McGregor, H., Legge, S., Jones, M. E., and Johnson, C. N. (2015). Feral cats are better killers in open habitats, revealed by animal-borne video. PLoS One 10, e0133915.
| Crossref | Google Scholar | PubMed |
Moseby, K., and McGregor, H. (2022). Feral cats use fine scale prey cues and microhabitat patches of dense vegetation when hunting prey in arid Australia. Global Ecology and Conservation 35, e02093.
| Crossref | Google Scholar |
Moseby, K., and O’Donnell, E. (2003). Reintroduction of the greater bilby, Macrotis lagotis (Reid) (Marsupialia: Thylacomyidae), to northern South Australia: survival, ecology and notes on reintroduction protocols. Wildlife Research 30, 15-27.
| Crossref | Google Scholar |
Moseby, K., and Read, J. (2006). The efficacy of feral cat, fox and rabbit exclusion fence designs for threatened species protection. Biological Conservation 127, 429-437.
| Crossref | Google Scholar |
Moseby, K., Read, J., Paton, D., Copley, P., Hill, B., and Crisp, H. (2011). Predation determines the outcome of 10 reintroduction attempts in arid South Australia. Biological Conservation 144, 2863-2872.
| Crossref | Google Scholar |
Moseby, K. E., Cameron, A., and Crisp, H. A. (2012). Can predator avoidance training improve reintroduction outcomes for the greater bilby in arid Australia? Animal behaviour 83, 1011-1021.
| Crossref | Google Scholar |
Moseby, K., Peacock, D., and Read, J. (2015). Catastrophic cat predation: a call for predator profiling in wildlife protection programs. Biological Conservation 191, 331-340.
| Crossref | Google Scholar |
Moseby, K. E., Blumstein, D. T., Letnic, M., and West, R. (2018). Choice or opportunity: are post-release social groupings influenced by familiarity or reintroduction protocols? Oryx 54, 215-221.
| Crossref | Google Scholar |
Moseby, K. E., Brandle, R., Hodgens, P., and Bannister, H. L. (2020). Can reintroductions to degraded habitat succeed? A test using the common brushtail possum. Austral Ecology 45, 675-690.
| Crossref | Google Scholar |
Moseby, K., Hodgens, P., Peacock, D., Mooney, P., Brandle, R., Lynch, C., West, R., Young, C., Bannister, H., and Copley, P. (2021). Intensive monitoring, the key to identifying cat predation as a major threat to native carnivore (Dasyurus geoffroii) reintroduction. Biodiversity and Conservation 30, 1547-1571.
| Crossref | Google Scholar |
Peelle, L. E., Wirsing, A. J., Pilgrim, K. L., and Schwartz, M. K. (2019). Identifying predators from saliva at kill sites with limited remains. Wildlife Society Bulletin 43, 546-557.
| Crossref | Google Scholar |
Priddel, D., and Wheeler, R. (2004). An experimental translocation of brush-tailed bettongs (Bettongia penicillata) to western New South Wales. Wildlife Research 31, 421-432.
| Crossref | Google Scholar |
Radford, J. Q., Woinarski, J. C., Legge, S., Baseler, M., Bentley, J., Burbidge, A. A., Bode, M., Copley, P., Dexter, N., and Dickman, C. R. (2018). Degrees of population-level susceptibility of Australian terrestrial non-volant mammal species to predation by the introduced red fox (Vulpes vulpes) and feral cat (Felis catus). Wildlife Research 45, 645-657.
| Crossref | Google Scholar |
Ross, A. K., Letnic, M., Blumstein, D. T., and Moseby, K. E. (2019). Reversing the effects of evolutionary prey naiveté through controlled predator exposure. Journal of Applied Ecology 56, 1761-1769.
| Crossref | Google Scholar |
Rouco, C., Jewell, C., Richardson, K., French, N., Buddle, B., and Tompkins, D. (2018). Brushtail possum (Trichosurus vulpecula) social interactions and their implications for bovine tuberculosis epidemiology. Behaviour 155, 621-637.
| Crossref | Google Scholar |
Ruiz-Villar, H., López-Bao, J. V., and Palomares, F. (2020). A small cat saving food for later: caching behavior in the European wildcat (Felis silvestris silvestris). European Journal of Wildlife Research 66, 76.
| Crossref | Google Scholar |
Sander, U., Short, J., and Turner, B. (1997). Social organisation and warren use of the burrowing bettong, Bettongia lesueur (Macropodoidea: Potoroidae). Wildlife Research 24, 143-157.
| Crossref | Google Scholar |
Saunders, G., Kay, B., and McLeod, L. (1999). Caching of baits by foxes (Vulpes vulpes) on agricultural lands. Wildlife Research 26, 335-340.
| Crossref | Google Scholar |
Short, J. (2016). Predation by feral cats key to the failure of a long-term reintroduction of the western barred bandicoot (Perameles bougainville). Wildlife Research 43, 38-50.
| Crossref | Google Scholar |
Short, J., and Turner, B. (2000). Reintroduction of the burrowing bettong Bettongia lesueur (Marsupialia: Potoroidae) to mainland Australia. Biological Conservation 96, 185-196.
| Crossref | Google Scholar |
Short, J., Bradshaw, S., Giles, J., Prince, R., and Wilson, G. R. (1992). Reintroduction of macropods (Marsupialia: Macropodoidea) in Australia – a review. Biological Conservation 62, 189-204.
| Crossref | Google Scholar |
Sliwa, A. (1994). Diet and feeding behaviour of the black-footed cat (Felis nigripes Burchell, 1824) in the Kimberley region, South Africa. Der Zoologische Garten 64, 83-96.
| Google Scholar |
Tobajas, J., and Díaz-Ruiz, F. (2022). Fishing behavior in the red fox: opportunistic‐caching behavior or surplus killing? Ecology 103, 3814.
| Crossref | Google Scholar | PubMed |
Trenwith, B., Armstrong, K. N., Van Der Weyde, L. K., and Moseby, K. E. (2023). The acoustic repertoire of the burrowing bettong (Bettongia lesueur). Australian Mammalogy 45, 264-274.
| Crossref | Google Scholar |
van der Marel, A., López-Darias, M., and Waterman, J. M. (2019). Group-enhanced predator detection and quality of vigilance in a social ground squirrel. Animal behaviour 151, 43-52.
| Crossref | Google Scholar |
Wengert, G. M., Gabriel, M. W., Matthews, S. M., Higley, J. M., Sweitzer, R. A., Thompson, C. M., Purcell, K. L., Barrett, R. H., Woods, L. W., Green, R. E., Keller, S. M., Gaffney, P. M., Jones, M., and Sacks, B. N. (2014). Using DNA to describe and quantify interspecific killing of fishers in California. The Journal of Wildlife Management 78, 603-611.
| Crossref | Google Scholar |
Woinarski, J. C., Burbidge, A. A., and Harrison, P. L. (2015). Ongoing unraveling of a continental fauna: decline and extinction of Australian mammals since European settlement. Proceedings of the National Academy of Sciences 112, 4531-4540.
| Crossref | Google Scholar | PubMed |