Feral cat predation of the threatened Pilbara leaf-nosed bat – a key threatening process
Jessie Moyses
A
B
C
D
E
F
G
Abstract
Feral cat predation is recognised as a significant threat to Australian wildlife that has contributed to population declines and extinctions. Cat predation of bats worldwide is a growing concern, though there are few studies focusing on Australian species. We consolidated empirical evidence of cat predation on the threatened Pilbara leaf-nosed bat (Rhinonicteris aurantia) in the Pilbara region of Western Australia. Observations in video and camera trap datasets demonstrated repeated predation of R. aurantia by several individual cats at three significant roosts. Between 2020 and 2023, we documented 183–200 bat kills at these sites. We recommend feral cat predation be formally recognised as a key threat to this species. To prevent declines at key colonies that would contribute to a population reduction, ongoing monitoring and proactive control of cats at known R. aurantia roosts should be prioritised. As demonstrated herein, camera trap monitoring is a valid method for quantifying the impact of feral cats at roost sites. Because relatively few individual cats at just a few sites can have a large impact, and most of the larger roost sites of R. aurantia have been discovered, there is potential for amelioration of this threat with multiple established and emerging methods deployed concurrently.
Keywords: Australian wildlife, bat roosts, camera‐trapping, feral cat, invasive species, Pilbara leaf-nosed bat, predation, wildlife management, threatened species, threatening process.
Introduction
Feral cats (Felis catus) are recognised as a significant predation threat to wildlife and have contributed to severe declines and extinctions in native birds, mammals and reptiles across Australia (Lepczyk et al. 2004; Medina et al. 2011; Doherty et al. 2017; Legge et al. 2017; Woolley et al. 2019). Predation by feral cats is recognised as a key threatening process under the Australian Commonwealth Government’s Environment Protection and Biodiversity Conservation Act 1999 (EPBC Act) and threatens the survival of over 100 native species in Australia (Department of Climate Change, Energy, the Environment and Water 2023a). According to the Western Australian Department of Biodiversity, Conservation and Attractions (2023), six mammal, 11 reptile and 22 bird species are threatened by feral cat predation. Feral cats established alongside the colonisation of Australia by Europeans, and by 1900 they were present throughout most of Australia and many offshore islands (Abbott 2002). The exclusion and removal of cats is a major consideration for conservation of small to medium-sized vertebrates on a landscape scale, and especially for threatened species rescue programs that rely on protecting small, vulnerable populations in limited areas such as fenced properties and islands (e.g. Algar et al. 2007, 2010; Moseby et al. 2009; Campbell et al. 2011).
The Pilbara leaf-nosed bat (Rhinonicteris aurantia, Pilbara form; synonym Pilbara Diamond-faced Bat; Armstrong et al. 2016) is listed as Vulnerable under the EPBC Act. It is one of two population isolates of the orange leaf-nosed bat that have divergent morphology and echolocation call frequency characteristics (Armstrong 2002, 2005; Armstrong and Coles 2007) and is distributed throughout the Pilbara region of Western Australia. Its distribution is limited by its poor physiological capacity to manage water and heat balance (Kulzer et al. 1970; Baudinette et al. 2000) and the scarcity of underground roosts with suitably warm and humid roost microclimates (28–32°C and 85–100% relative humidity; Armstrong 2000, 2001) within natural caves and disused underground mines. Suitable natural caves within the ironstone terrain of the Hamersley Range and the margins of the Fortescue Valley coincide with a substantial focus for active mining activities. It also relies on disused gold and copper mines in the eastern Pilbara (around Bamboo Creek, Marble Bar and Nullagine), some of which have disappeared or are at risk of roost collapse (Armstrong 2001; Cramer et al. 2016). The species was listed under the EPBC Act in 2001 following the growth of iron ore mining in the Pilbara, partly because of concerns for encroachment on roost habitat. The Pilbara leaf-nosed bat has since become a significant consideration and research focus in the mining approval process in the Pilbara region (Cramer et al. 2016).
On a global level, studies quantifying or describing the impacts of cats (including domestic, stray and feral cats) on bats are less represented in the literature than other fauna groups and non-volant mammals (Ancillotto et al. 2013; unpubl. data., G. Vázquez-Domínguez., R. A Saldaña-Vázquez, M. V Orduña-Villaseñor and J. Schondube; Welch and Leppanen 2017). However, according to a review by Oedin et al. (2021), 86 bat species have been reported to be preyed upon or threatened by cats, a quarter of which are listed as Threatened or Near Threatened in IUCN Red List assessments. Within Australia, 24 species of bat, including a record of R. aurantia from the Northern Territory, were reported as cat prey (Woolley et al. 2019). The same study also recognised that this number could be an underestimate given recent taxonomic changes and that further research would likely reveal additional records of bat species preyed upon by cats.
Cats prey upon bats in both urban and regional habitats, with impacts on cave-, house- and tree-roosting bat species. Documented examples include the great fruit-eating bat (Artibeus lituratus), pale spear-nosed bat (Phyllostomus discolor) and vampire bat (Desmodus rotundus) in Argentina and Brazil (Delpietro et al. 1994; da Costa-Pinto 2020), central lesser short-tailed bat (Mystacina tuberculata rhyacobia) and long-tailed bat (Chalinolobus tuberculatus) in New Zealand (Scrimgeour et al. 2012; Borkin et al. 2022), as well as several species in Mexico (unpubl. data., G. Vázquez-Domínguez., R. A Saldaña-Vázquez, M. V Orduña-Villaseñor and J. Schondube), the United Kingdom (Khayat et al. 2020), Portugal (Rocha 2015) and Italy (Ancillotto et al. 2013). The ability to fly clearly does not always allow bats to evade cat predation, and bats are generally considered more at risk when in their roosts.
Evidence of cat predation on bats in Australia comes from observations on the eastern blossom bat (Syconycteris australis) and southern bent-winged bat (Miniopterus orianae bassanii) in south-eastern Australia (Phillips et al. 2001; E. van Harten, pers. comm., 2023). Recent publications have recognised feral cats as a threat to other mammal species in the same Pilbara cave habitats as those used by R. aurantia (Rothschild’s rock-wallaby (Petrogale rothschildi), Anderson et al. 2021; and ghost bat (Macroderma gigas), Cramer et al. 2022). The tendency of cats to hunt at cave roosts with low and narrow entrances that are used by M. gigas (Bat Call WA 2021a) also has implications for R. aurantia because the two bat species have been recorded in many of the same roosts. Despite recent (Bat Call WA 2021b) and historical reviews of threats to the species (Cramer et al. 2016; Threatened Species Scientific Committee 2016), none have identified feral cats as posing a potential threat to R. aurantia. Consultant biologists who recorded cat predation of R. aurantia at known bat roosts raised concerns about this threat at the Pilbara leaf-nosed bat conservation workshop in 2022 because of casual observations made throughout several mining project areas.
In this study we consolidate and quantify evidence of feral cat predation on R. aurantia. Our intention was to compile evidence as the basis for: (1) formal recognition of feral cats as a threat to R. aurantia; and (2) highlighting the need for targeted control of cats at and nearby known R. aurantia roosts to reduce the current documented level of impact and the future threat from cat predation. Understanding how feral cat predation impacts Pilbara bat colonies will provide further perspective on the range and level of key threats to their populations that is important within the broader context of other cumulative threats to bats, such as habitat loss and indirect disturbances associated from mining and other human activities.
Methods
To address the lack of published data on the issue of feral cat predation on R. aurantia and to document unpublished records, we conducted an online survey requesting records of cat predation on Australian bat species, particularly R. aurantia and other cave obligate species. The following recipients were included in the request for information: Australasian Bat Society, Inc.; Ecological Consultants Association (chapters in New South Wales, Victoria and Western Australia); various consulting companies in Western Australia (Biologic Environmental Survey Pty Ltd, Biota Environmental Sciences Pty Ltd, Eco Logical Australia Pty Ltd, Ecologia Environment Pty Ltd, Spectrum Ecology Pty Ltd, Stantec Pty Ltd and Terrestrial Ecosystems Pty Ltd); and several mining companies operating in the Pilbara region, including Fortescue Ltd. Of the four respondees, two noted interactions between feral cats and R. aurantia. We collated recorded instances of predation by cats on R. aurantia in the Pilbara, as well as anecdotal findings related to cat presence in the vicinity of bat roosts.
Study site description
Studies conducted by GHD Pty Ltd and Biologic Environmental Survey Pty Ltd in the Pilbara region of Western Australia have produced two empirical datasets of cat predation on R. aurantia at three recognised roost sites. The roosts are located 110 km (Roost 1), 141 km (Roost 2) and 152 km (Roost 3) south of Port Hedland (Fig. 1). All three roosts have been monitored for several years using a combination of visual (infrared camera traps), acoustic (full spectrum ultrasonic recorders) and for two roosts, collection of scat and wings for DNA testing. Thus, the significance of these caves to R. aurantia is well understood. Roost types are categorised according to the definitions in the Conservation Advice for the species (Threatened Species Scientific Committee 2016).
Roost 1 has been identified as a natural ‘permanent diurnal roost (Priority 1)’ and has been subject to an intensive monitoring study conducted by GHD from 2017 to 2023. The three-chambered cave sits near the top of a west-facing cliff of banded iron formation at an elevation of approximately 368 m. The roosting area is located in the rear chamber of the cave that is accessed via passages through chambers 1 and 2. The narrow entrance to the rear roosting chamber is ~50 × 70 cm and is at ground level. The cave is also occupied by the common sheath-tailed bat (Taphozous georgianus), Finlayson’s cave bat (Vespadelus finlaysoni) and, occasionally, by the ghost bat (M. gigas). Cat predation has been recorded at the entrance of the second chamber, which has an opening of ~1.5 m high and 1 m wide.
Roost 2 is also considered a ‘permanent diurnal roost (Priority 1)’. It is positioned on the eastern-facing slope of a hill ~300 m above the surrounding plain. The cave has two chambers. The outer chamber has a low (~0.5 m high, 2 m wide) entrance at ground level. Predation of bats has been recorded at the entrance of this first chamber. The inner chamber is connected by a long (~5 m) but small (50 cm wide × 30 cm high) passage at ground level. R. aurantia roost in the second chamber. The roost is also occupied by T. georgianus and V. finlaysoni.
Roost 3 is also considered to be a ‘permanent diurnal roost (Priority 1)’ and is located at the base of north-western-facing mesa-like escarpment, near the top of small ironstone range. It comprises a single main chamber with five pillars and three vertical ‘pipeholes’ in the ceiling. R. aurantia roost near the entrance of the cave within one of these vertical pipeholes (30 cm × 30 cm) that is ~1.2 m off the ground, and ~5 m from the cave entrance. M. gigas are regularly recorded roosting in another section of the cave, as are T. georgianus and V. finlaysoni.
Survey methods
Video recordings were made at the entrance of Roost 1 with a Uniden Guardian Infrared video camera between 2021 and 2023 for the purpose of understanding bat emergence and visitation. Cats were detected incidentally at the roost entrance over this period. Videos from this camera were reviewed periodically (i.e. not all recordings were analysed). Observation of morphological characteristics (such as bat size and the presence/absence of a nose leaf) and review of ultrasonic recordings allowed for confirmation of bat species in the photographic and video images.
In the case of both Roost 2 and Roost 3, wings of R. aurantia were observed (in February 2022 and June 2020, respectively) outside the roosts during regular monitoring visits, which prompted the deployment of camera traps (Reconyx 2 Covert IR HF2X and Swift Enduro Regular, respectively) to determine the species of predator responsible. Morphological analysis, review of ultrasonic data and DNA sequencing of the wings from Roost 2 allowed for positive identification of the bat prey species. The Reconyx 2 Covert IR camera was set to motion-sensing (high sensitivity), three images per trigger (rapidfire) and one 30 s video per trigger, with no delay between triggers. The Swift Enduro was also set to three images per trigger (high sensitivity).
For both Roost 2 and Roost 3, camera traps were placed at the cave entrances, facing towards or across the entrance, and specifically angled to capture the location where wings had been found. The field of view of camera traps provided good coverage to detect the movement of cat-sized mammals, but the set-up was limited by the terrain (e.g. slope, obstacles such as large rocks) and the size of the cave entrance. The field of view of the camera traps did not cover the full entrance of the cave (nor the inside of the roost), so it is possible that some predation events were not captured during the survey periods, although the location of each camera likely captured the majority of predation events at the roost entrance.
All photographic images from camera traps recorded between March 2022 and January 2023 were inspected to note all visitation to the caves by feral cats. For data collected from Roost 2 and Roost 3, information recorded for each image included categorisation of cat behaviour into ‘stalking’, ‘active hunting with capture (e.g. cat pouncing or swiping prey)’, ‘active hunting without capture’, ‘passive hunting’ (e.g. cat sitting or in lying position but appearing alert and monitoring bat movement), ‘entering cave’, ‘exiting cave’ and ‘feeding’, in addition to the identification of individual cats. The total number of bat predation events from each cat visit was recorded. Some predation events were difficult to confirm due to the distance of the cat from the camera at Roost 3, so a minimum and maximum count of predation events from each cat visit was recorded. Individual cats were identified from their coat pattern, shade and body size.
Genetics-based identification
To further provide an assessment of the level of threat that cats might have on a colony of bats, and thus an appreciation of the threat of feral cats more broadly in the region, we calculated the number of individual R. aurantia within the accumulation of wings collected from Roost 2 in February 2022 using genetic markers. The wings were scattered within a few metres of the entrance to the cave, which is approximately 2 m wide and 0.5 m high. The wings were identified as being from R. aurantia from their forearm lengths (cf. measurements in Armstrong 2002). There were 31 wings in a similar condition that included most of the skin (18 left wings and 13 right wings) collected on one occasion. The timeframe over which the predation of these bats had occurred was not able to be determined, but the condition of the wings was similar, so it could have been from one to several nights within a short period (herein termed a ‘predation event’). Although other predators such as northern quolls (Dasyurus hallucatus) or M. gigas might have been responsible for this accumulation of wings, the observations of cats hunting at the entrance in later months provide more support for cats being responsible.
Genetic markers were used to identify the actual number of individual bats present, given that some pairs of wings could have derived from the same individual. A genotype for each wing was generated using a panel of 149 short fragments of DNA that each had a diagnostic single nucleotide polymorphism present. The markers were developed from an unpublished DArTseq resource (Diversity Arrays Technology, Canberra; K. N. Armstrong, unpubl. data), which was developed for a larger study of local movements of this species in the Iron Bridge mining project area (unpubl. data, C. Grabham, H. North, J. Moyses, E. Westerhuis; unpubl. data, K. N. Armstrong). The panel of markers was amplified via a series of three multiplex PCRs as part of a custom Agena Bioscience MassARRAY System (Ellis and Ong 2017). The resulting genotypes were filtered to include only loci that had a 100% amplification success rate across all 31 samples, which gave a total of 78 loci. A full description of the application of this marker set for estimating colony size and fine-scale movements of R. aurantia is being published separately. Key details for the present study include the calculation of pairwise similarity values among all genotypes using Prevosti Distance in the [R] package poppr (Prevosti 1974; Kamvar et al. 2015). Validation steps as part of the separate population genetic study established that a similarity value of 0.9 was a suitable minimum threshold for accepting that two samples are from the same individual. This was achieved by comparing the range of pairwise similarity values between two or more samples from the same individuals with the range amongst samples from different individuals (details not shown but see Supplementary S1 for an illustration of the pairwise similarity values in the present dataset from the wings).
To give context to the numbers of bats killed at Roost 2, including the number estimated using genetic markers, the colony size was estimated from counts of bats emerging from the roost entrance over an entire night (16 December 2022; unpubl. data, K. N. Armstrong). Colony size at this roost has been shown to be relatively stable (results will be available as part of another study to be published separately). Bats were recorded using a FLIR Boson long-wave infrared thermal video camera (resolution 640 × 512; 60 frames per second; 8.7 mm; 50° field of view) connected to a TEC digital video recorder programmed to record between sunset and sunrise. Counts of flight tracks from Pilbara leaf-nosed bats emerging and entering the cave entrance were made with ThruTracker version 2.0.5 software (Corcoran et al. 2021), as well as a manual count from inspecting the video recording. Correspondence between the two counting methods was 96.7%.
Results
The total number of bat kills observed in video recordings and photographic images at all three focal roosts was 152–169 individuals (Table 1). The direction of bat flight was often difficult to determine, but it appeared bats both leaving and entering the roost were captured. Two individual cats were observed at the entrance to Roost 1 on two consecutive nights (one on 28 April 2021 and one on 29 April 2021). On the first of those nights, the cat (Table 1; Cat A) appeared to capture and feed on one small bat, which was identified as R. aurantia from various characteristics. In addition, at least five ‘active hunting without capture’ events were observed.
Date (night of) | Roost | Cat ID | Number of bats taken | Cumulative no. bats taken each date | Time between visits (nights) | |
---|---|---|---|---|---|---|
29/4/2021 | 1 | Cat A | 1 | 1 | Initial recording | |
03/3/2022 | 2 | Cat B | 7 | 8 | Initial recording | |
16/3/2022 | 2 | Cat B | 23 | 31 | 13 | |
17/3/2022 | 2 | Cat B | 9 | 40 | 1 | |
18/3/2022 | 2 | Cat B | 22 | 62 | 1 | |
19/3/2022 | 2 | Cat B | 15 | 77 | 1 | |
11/12/2022 | 2 | Cat C | 1 | 78 | 227 | |
12/12/2022 | 2 | Cat C | 2 | 80 | 0 | |
09/1/2023 | 2 | Cat C | 0 | 80 | 28 | |
11/1/2023 | 2 | Cat C | 0 | 80 | 1 | |
18/1/2023 | 2 | Cat C | 0 | 80 | 7 | |
23/1/2023 | 2 | Cat C | 0 | 80 | 5 | |
16/2/2023 | 2 | Cat C | 0 | 80 | 24 | |
17/2/2023 | 2 | Cat C | 0 | 80 | 1 | |
15/4/2023 | 2 | Cat C | 7 | 87 | 57 | |
13/5/2023 | 2 | Cat C | 11 | 98 | 29 | |
14/5/2023 | 2 | Cat C | 19 | 117 | 1 | |
19/6/2020 | 3 | Cat D | 3–9 | 3–9 | Initial recording | |
20/6/2020 | 3 | Cat D | 8–13 | 11–22 | 1 | |
23/6/2020 | 3 | Cat D | 6–10 | 17–32 | 3 | |
24/6/2020 | 3 | Cat D | 5–6 | 22–38 | 1 | |
19/7/2020 | 3 | Cat D | 1 | 23–39 | 26 | |
14/8/2020 | 3 | Cat D | 5 | 28–44 | 26 | |
15/8/2020 | 3 | Cat D | 2 | 29–46 | 1 | |
16/8/2020 | 3 | Cat D | 1 | 30–47 | 1 | |
17/8/2020 | 3 | Cat D | 1 | 31–48 | 1 | |
15/9/2020 | 3 | Cat D | 3 | 34–51 | 28 | |
17/9/2020 | 3 | Cat D | 1 | 35–52 | 2 |
At Roost 2, 116 bats were killed by two cats (distinguishable from their coat patterns): 76 by ‘Cat B’ in March 2022 and a total of 40 by ‘Cat C’ between December and May 2023. In most images, the cat was observed to be either ‘stalking’ at the cave entrance (n = 1822 images; 39% of observations) or ‘passive hunting’ (n = 2178 images; 46% of observations), with a further 9% of observations of ‘feeding’, 3% of ‘active hunting without capture’ and 3% of ‘active hunting with capture’ (representations of each cat behaviour in Fig. 2; example images of Cat B and Cat C with R. aurantia in Supplementary S2). The colony size of Roost 2 was estimated at between 2456 and 2534 in December 2022 (unpubl. data, K. N. Armstrong). Therefore, based on observational data, cats removed ~4.7% of individuals within the colony over the period of observations.
Summary of cat behaviour observations from observed photographic images at Roost 2 between March 2022 and May 2023 and Roost 3 between June 2020 and September 2020.
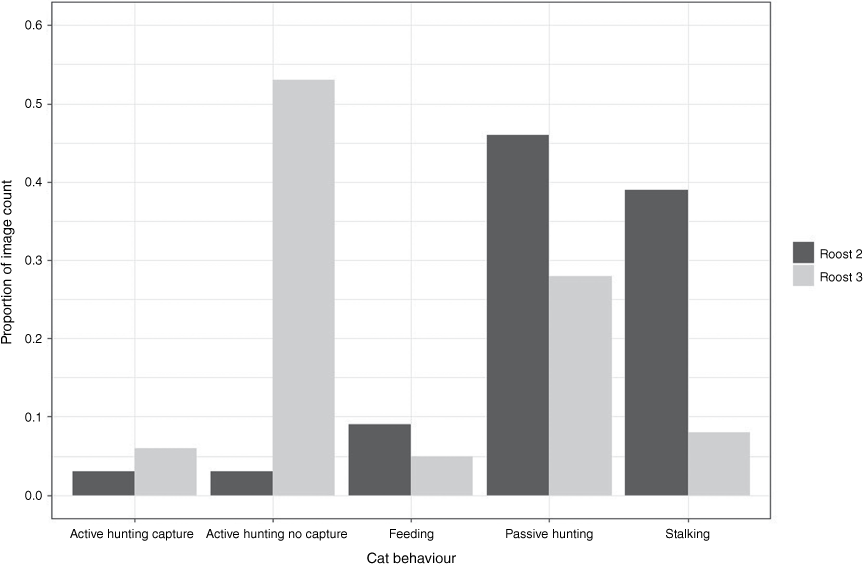
A single adult cat (Roost 3 Cat D) visited Roost 3 on 35 nights between 24 June 2020 and 11 September 2020 (Supplementary S2). The cat successfully captured bats on eight nights, with a total of 33–51 predation events. On six nights between late June to mid-July 2020, three kittens were also seen accompanying Cat D. The kittens made attempts to capture bats on the nights they were present. However, they were not successful until 19 July, when one of the kittens captured one bat. The kittens were not observed again after 19 July. The adult cat observed at Roost 3 (Cat D) displayed very similar hunting behaviour to the two cats observed at Roost 2. It was observed stalking in 53 images (8%), passively hunting in 190 images (28%) or actively hunting – no capture in 365 images (53%), with the remaining time spent largely recorded as actively hunting with capture (44 images, 6%) and feeding (37 images, 5%) (Fig. 2). A roost census using IR video and ultrasonic recording in 2016 indicated that the colony size of R. aurantia likely comprised between 780 and 975 individuals. Therefore, the adult and her kittens potentially impacted 3.4–6.5% of individuals within the colony over 4 months.
In the analysis using the Agena MassARRAY genetic markers to determine the number of individuals among a collection of 31 wings collected at Roost 2 in February 2022, there were 31 unique genotypes, indicating that each wing was from a separate individual. Pairwise similarity values amongst the genotypes ranged from 0.57 to 0.76, much less than the ideal value of 1.0 (and well below the established threshold of 0.9) that would indicate a match of all 78 loci. Thus, there is evidence for a scenario whereby a single cat had captured and consumed at least 31 individual bats over a relatively short period. Given the direct observations of capture described from photographs and videos, the genetics-based determination of the number of individuals killed underestimated the level of predation by cats at least three-fold, given that the total number of bat kills over the observation periods was 152–169 individuals.
Discussion
A relatively small set of observations (comprising 28 days of cat visitation, three roost sites, four individual adult cats and a total kill of between 183 and 200 individual bats) provides a strong basis for concern that feral cats are having, and could continue to have, a significant impact on the Vulnerable-listed Pilbara leaf-nosed bat (R. aurantia). Adding to the significance of these observations, each of the three roosts is recognised as critical habitat for R. aurantia in its Pilbara distribution. The cats exhibited clear targeted hunting behaviour at Roost 2 and Roost 3, which prompts concern for other colonies across the region, highlighting the need for ongoing and suitably designed feral cat management at these and other significant roosts of the species.
R. aurantia are likely to be vulnerable to cat predation because of the accessibility to cats of roosts and roost entrances (e.g. Roost 2’s single, small entrance at ground level), the colonial roosting habit of the bat species and a reliance on specific microclimatic conditions (high temperature and high humidity) that leaves the species with a limited choice of suitable roost sites. As exemplified by Roost 3, R. aurantia often roost in a constricted chamber, which increases the risk of predation if there is only a single narrow opening for their egress. Cats observed in this study at both Roost 2 and Roost 3 were clearly aware of bats flying into and out of the cave entrances and exploited this behaviour, making them a relatively ‘low-effort’ prey item. Furthermore, at Roost 3, a cat also appeared to use the bat colony to train its young. Within Roost 1, R. aurantia roost and breed within a chamber at the rear of the cave that is accessible by a single narrow ground-level opening. Although a cat has not yet been observed to date at this internal entrance or within the roosting chamber, there is certainly potential for a significant number of bats to be captured and killed, should it be discovered by cats.
In addition to the physical characteristics of the roost that were considered an important factor in cat hunting success in the present study, other published studies have demonstrated that the impacts of feral cats can be amplified by increased hunting effectiveness in particular landscapes, such as fire scars (McGregor et al. 2015; Hohnen et al. 2023). Post-fire conditions may have exacerbated the impact of Cat B at Roost 2 (76 observed bat predation events) – a substantial fire had burned surrounding vegetation just 2 months prior to the commencement of monitoring at that roost. Such observations highlight that combinations of factors might multiply both the risk and impacts of a key threat.
The possibility that individual cats specialise on particular prey types contributes further to the level of threat that cats pose to R. aurantia in the Pilbara. The significant number of bats taken from the colonies in this study during the recording periods (total observations: 1 bat at Roost 1; 116 bats at Roost 2; and 35–52 bats at Roost 3) are likely to be a small subset of the actual impact cats have had at these roosts, and may be indicative of the possible scale of predation throughout the region. The effect of prey specialisation by an individual cat has been recorded previously by Scrimgeour et al. (2012), who observed hunting of the New Zealand short-tailed bat (Mystacina tuberculata rhyocobia) at a roost tree until the cat was captured 7 days after its detection. In Italy, Ancillotto et al. (2013) demonstrated that once a roost is found by a cat, targeted hunting can result in a substantial number of mortalities in a short time. They also noted that roosts were easily located because of the broad frequency range of hearing in cats, which encompasses most bat echolocation and social calls (in temperate bat species), as well as the strong smell associated with roosts. In addition, the magnitude of threat that cats pose to bat colonies can vary depending on the characteristics of individual cats, such as their size (e.g. large males) and previous level of experience. Moseby et al. (2015) found that individual cats can have a disproportionate and catastrophic impact that devastates the efforts of reintroduction programs. Therefore, it is imperative to investigate and monitor the relatively small number of roost locations where Pilbara leaf-nosed bats are particularly vulnerable so that cats exhibiting prey specialisation behaviour can be neutralised.
Implications for conservation status assessments
Mentions of introduced species or feral cats are absent from the listed threats to R. aurantia on the Species Profile and Threats Database (SPRAT; Department of Climate Change, Energy, the Environment and Water 2023b). Mention of feral cats is also absent in the current Conservation Advice (Threatened Species Scientific Committee 2016), the Action Plan for Australian Mammals 2012 (Woinarski et al. 2014) and the current IUCN Red List profile for the species (Armstrong et al. 2021). Given the clear and significant threat posed by cats at key diurnal roosts that we present evidence for here, we recommend that feral cats are formally recognised as a key threat to R. aurantia and encourage the scientific community to report on cat predation events so these data can be compiled into the future.
The levels of observed predation of 152–169 individual R. aurantia, and the genetics-based identification of 31 individual R. aurantia from the wings of cat predation at Roost 2 (likely a three-fold underestimate of numbers killed given the direct observations made at other times), is significant because it provides some (albeit limited) empirical evidence in support of calculations of the level of threat posed by feral cats. Given that the colony size at Roost 2 was estimated at between 2456 and 2534 individuals in the period 16–17 December 2022 using thermal video recordings (unpubl. data, K. N. Armstrong), this singular predation event was represented by the 31 wings removed ~1.2% of the colony (and at least another 4.7% removed by two cats between March 2022 and May 2023). If such predation rates are ongoing, it could lead to a significant level of reduction at what is recognised to be important regional colony because of its size and the observations of breeding (unpubl. data, C. Grabham, H. North, J. Moyses, E. Westerhuis). Whether one or two cats could completely remove an entire colony is difficult to say because other associated studies (manuscripts in preparation) have shown movement amongst colonies in the local area, so important colonies such as Roost 2 could function as ‘sinks’, which would provide an ongoing source of new bats for a hunting cat. Further, the risk from cat predation might be exacerbated by the effects of climate change. If the abundance of vertebrate prey of cats is reduced generally because of longer periods of hot and dry conditions, then bat roosts might become more attractive hunting areas for feral cats.
This quantification of loss has implications for the conservation status of R. aurantia, which was assessed as Vulnerable A3c by Woinarski et al. (2014). When this rate of predation is considered in a regional context (unchecked predation levels at multiple roosts), it further supports predictions of future population reduction, and it would contribute to the projected ≥30% decline over 100 years. The contribution of cat predation to the predicted population reduction of this species can now be recognised by adding a subcriterion (A3e; effects of introduced taxa) to the category. The justification for adding a subcriterion to the conservation status category does not require knowledge of population size for criterion A3, but population size is still relevant for understanding the relative contribution of feral cat predation to a projected rate of population reduction. Estimates of population size, and the suggestion of a substantial recent decline because of climatic factors, were presented in a Commonwealth Government review document (Bat Call WA 2021b), but the figures were estimated from bat detector recordings and no validation was presented for peer review. If we make a generous unfounded assumption that the regional population numbers ~20,000 individuals (a value between two estimates given by Bat Call WA 2021b), and that there are several roost sites where cats successfully hunt this species (because of favourable roost entrance shape characteristics), then a total yearly kill of 200 bats would contribute an additional 1% to the annual natural mortality rate – a figure that seems possible (given both observational data in Table 1 and the results from the genetic analysis). With no other contributions to population reduction (and assuming that rates of both recruitment and natural mortality are equivalent within years) and using an exponential decay formula (Supplementary S3), an annual feline-mediated reduction rate of 1% would see the population size reduced by 30% after 36 years, consistent with criterion A3 in the Vulnerable category. With greater amounts of infrastructure being built in the region that are known to attract feral animals such as cats, the risk appears realistic.
If the contribution to this level of population reduction is from relatively few individual predators at relatively few sites, then there appears to be a good prospect for avoiding loss through active and sustained management. There is a range of management options for feral cats in Australia, and although it is beyond the scope of this study to discuss and recommend specific methods and approaches, we do recommend the need for multiple control methods, including specific actions at locations where bats are particularly vulnerable to predation, especially from specialist hunters and their offspring.
Data availability
The data that support this study will be shared upon reasonable request to the corresponding author.
Declaration of funding
This work was supported by funds provided by Fortescue (FMG Iron Bridge (Aust) Pty Ltd).
Acknowledgements
The authors would like to thank Drew Farrar (GHD Pty Ltd) and Jayden O’Brien (Fortescue) for their review that improved the manuscript. We acknowledge Fortescue and Atlas Iron for permitting the use of the data analysed in this manuscript. We are grateful to the Australian Genome Research Facility (AGRF), and particularly Maia Rabinovich (Adelaide node) and Kimberley Rogl and Tamara MacDonald (Brisbane node) for designing the Agena MassARRAY markers, undertaking the DNA extractions and sequencing the genotypes of bat tissue samples.
Author contributions
Craig Grabham and Jessie Moyses conceived the ideas and designed the methods presented. Jessie Moyses, Chris Knuckey and Brighton L. D’Rozario collected and analysed the observational data. Jessie Moyses and Craig Grabham led the writing of the manuscript. Kyle Armstrong performed the genetic analysis, thermal video counts and population reduction calculation, and contributed other aspects to the manuscript.
References
Abbott, I. (2002). Origin and spread of the cat, Felis catus, on mainland Australia, with a discussion of the magnitude of its early impact on native fauna. Wildlife Research 29, 51-74.
| Crossref | Google Scholar |
Algar, D., Angus, G. J., Williams, M. R., and Mellican, A. E. (2007). Influence of bait type, weather and prey abundance on bait uptake by feral cats (Felis catus) on Peron Peninsula, Western Australia. Conservation Science Western Australia 6, 109-149.
| Google Scholar |
Algar, D., Angus, G. J., Brazell, R. I., Gilbert, C., and Withnell, G. B. (2010). Eradication of feral cats on Faure Island, Western Australia. Journal of the Royal Society of Western Australia 93, 133-140.
| Google Scholar |
Ancillotto, L., Serangeli, M. T., and Russo, D. (2013). Curiosity killed the bat: domestic cats as bat predators. Mammalian Biology 78, 369-373.
| Crossref | Google Scholar |
Anderson, H., Richards, B., Ross, W., and Palmer, R. (2021). Feral cat (Felis catus) predation confirmed for Rothschild’s rock-wallaby (Petrogale rothschildi) in the Pilbara. Australian Mammalogy 44, 169-172.
| Crossref | Google Scholar |
Armstrong, K. N. (2000). Roost microclimates of the bat Rhinonicteris aurantius in a limestone cave in Geike Gorge, Western Australia. Australian Mammalogy 22, 69-70.
| Crossref | Google Scholar |
Armstrong, K. N. (2001). The distribution and roost habitat of the orange leaf-nosed bat, Rhinonicteris aurantius, in the Pilbara region of Western Australia. Wildlife Research 28, 95-104.
| Crossref | Google Scholar |
Armstrong, K. N. (2002). Morphometric divergence among populations of Rhinonicteris aurantius (Chiroptera: Hipposideridae) in northern Australia. Australian Journal of Zoology 50, 649-669.
| Crossref | Google Scholar |
Armstrong, K. N. (2005). A description and discussion of the penile morphology of Rhinonicteris aurantius (Gray, 1845) (Microchiroptera: Hipposideridae). Australian Mammalogy 27, 161-167.
| Crossref | Google Scholar |
Armstrong, K. N., and Coles, R. B. (2007). Echolocation call frequency differences between geographic isolates of Rhinonicteris aurantia (Chiroptera: Hipposideridae): implications of nasal chamber size. Journal of Mammalogy 88, 94-104.
| Crossref | Google Scholar |
Armstrong, K. N., Goodman, S. M., Benda, P., and Hand, S. J. (2016). A common name for the bat family Rhinonycteridae—the Trident Bats. Zootaxa 4179, 115-117.
| Crossref | Google Scholar | PubMed |
Armstrong, K. N., Reardon, T. B., Woinarski, J. C. Z., and Burbidge, A. A. (2021). Rhinonicteris aurantia (amended version of 2017 assessment). The IUCN Red List of Threatened Species 2021: e.T19589A209539734. 10.2305/IUCN.UK.2021-3.RLTS.T19589A209539734.en [accessed 9 November 2023].
Bat Call WA (2021a). A review of ghost bat ecology, threats and survey requirements. (Report prepared by R. D. Bullen of Bat Call WA Pty Ltd for the Department of Agriculture, Water and the Environment: Canberra, ACT, Australia.) Available at https://www.dcceew.gov.au/sites/default/files/documents/review-ghostbat-ecology-threats.pdf
Bat Call WA (2021b). A review of Pilbara leaf-nosed bat ecology, threats and survey requirements. (Report prepared by R. D. Bullen of Bat Call WA Pty Ltd for the Department of Agriculture, Water and the Environment: Canberra, Australian Capital Territory, Australia.) Available at https://www.dcceew.gov.au/sites/default/files/documents/review-pilbara-leaf-nosed-bat-ecology-threats.pdf
Baudinette, R. V., Churchill, S. K., Christian, K. A., Nelson, J. E., and Hudson, P. J. (2000). Energy, water balance and the roost microenvironment in three Australian cave-dwelling bats (Microchiroptera). Journal of Comparative Physiology. B, Biochemical, Systemic, and Environmental Physiology 170, 439-446.
| Crossref | Google Scholar | PubMed |
Borkin, K. M., Easton, L., and Bridgman, L. (2022). Bats attacked by companion and feral cats: evidence from indigenous forest and rural landscapes in New Zealand. New Zealand Journal of Zoology 50, 1-8.
| Crossref | Google Scholar |
Corcoran, A. J., Schirmacher, M. R., Black, E., and Hedrick, T. L. (2021). ThruTracker: open-source software for 2-D and 3-D animal video tracking. bioRxiv
| Crossref | Google Scholar |
Cramer, V. A., Armstrong, K. N., Bullen, R. D., Ellis, R., Gibson, L. A., McKenzie, N. L., O’Connell, M., Spate, A., and van Leeuwen, S. (2016). Research priorities for the Pilbara leaf-nosed bat (Rhinonicteris aurantia Pilbara form). Australian Mammalogy 38, 149-157.
| Crossref | Google Scholar |
Cramer, V. A., Armstrong, K. N., Bullen, R. D., Cross, S. L., Gibson, L., Hanrahan, N., Knuckey, C. G., Ottewell, K., Reiffer, S., Ruykys, L., Shaw, R. E., Thavornkanlapachai, R., Thompson, S. A., Wild, S., and van Leeuwen, S. (2022). Research priorities for the ghost bat (Macroderma gigas) in the Pilbara region of Western Australia. Australian Mammalogy 45, 1-12.
| Crossref | Google Scholar |
da Costa-Pinto, A. L. (2020). First record of natural predation on bats by domestic cat in Brazil, with distribution extension for Phyllostomus discolor. Oecologia Australis 24, 242-248.
| Crossref | Google Scholar |
Delpietro, H., Konolsaisen, F., Marchevsky, N., and Russo, G. (1994). Domestic cat predation on vampire bats (Desmodus rotundus) while foraging on goats, pigs, cows and human beings. Applied Animal Behaviour Science 39, 141-150.
| Crossref | Google Scholar |
Department of Biodiversity Conservation and Attractions (2023). Western Australian Feral Cat Strategy. Available at https://www.dbca.wa.gov.au/news/2023/was-new-strategy-crackdown-feral-cats-nation-first [accessed 18 August 2023].
Department of Climate Change, Energy, the Environment and Water (2023a). Feral Cats. Feral cats - DCCEEW. Available at https://www.dcceew.gov.au/environment/invasive-species/feral-animals-australia/feral-cats [accessed 29 June 2023].
Department of Climate Change, Energy, the Environment and Water (2023b). Species Profile and Threats Database Rhinonicteris aurantia (Pilbara form) – Pilbara Leaf-nosed Bat. Available at https://www.environment.gov.au/cgi-bin/sprat/public/publicspecies.pl?taxon_id=82790 [accessed 31 August 2023].
Doherty, T. S., Dickman, C. R., Johnson, C. N., Legge, S. M., Ritchie, E. G., and Woinarski, J. C. Z. (2017). Impacts and management of feral cats Felis catus in Australia. Mammal Review 47, 83-97.
| Crossref | Google Scholar |
Ellis, J. A., and Ong, B. (2017). The MassARRAY ® System for Targeted SNP Genotyping. In ‘Genotyping. Methods in Molecular Biology. Vol 1492’. (Eds S. J. White, S. Cantsilieris.) pp. 77–94. (Humana Press: New York, NY.) 10.1007/978-1-4939-6442-0_5
Hohnen, R., James, A. I., Jennings, P., Murphy, B. P., Berris, K., Legge, S. M., Dickman, C. R., and Woinarski, J. C. Z. (2023). Abundance and detection of feral cats decreases after severe fire on Kangaroo Island, Australia. Austral Ecology 48, 600-615.
| Crossref | Google Scholar |
Kamvar, Z. N., Brooks, J. C., and Grünwald, N. J. (2015). Novel R tools for analysis of genome-wide population genetic data with emphasis on clonality. Frontiers in Genetics 6, 208.
| Crossref | Google Scholar |
Khayat, R. O. S., Grant, R. A., Ryan, H., Melling, L. M., Dougill, G., Killick, D. R., and Shaw, K. J. (2020). Investigating cat predation as the cause of bat wing tears using forensic DNA analysis. Ecology and Evolution 10, 8368-8378.
| Crossref | Google Scholar | PubMed |
Kulzer, E., Nelson, J. E., McKean, J. L., and Moehres, F. P. (1970[Temperature regulation in Australian bats (Microchiroptera)].). Untersuchungen ueber die temperaturregulation Australischer Fledermause (Microchiroptera). Zeitschrift für Vergleichende Physiologie 69, 426-451.
| Crossref | Google Scholar |
Legge, S., Murphy, B. P., McGregor, H., Woinarski, J. C. Z., Augusteyn, J., Ballard, G., Baseler, M., Buckmaster, T., Dickman, C. R., Doherty, T., Edwards, G., Eyre, T., Fancourt, B. A., Ferguson, D., Forsyth, D. M., Geary, W. L., Gentle, M., Gillespie, G., Greenwood, L., Hohnen, R., Hume, S., Johnson, C. N., Maxwell, M., McDonald, P. J., Morris, K., Moseby, K., Newsome, T., Nimmo, D., Paltridge, R., Ramsey, D., Read, J., Rendall, A., Rich, M., Ritchie, E., Rowland, J., Short, J., Stokeld, D., Sutherland, D. R., Wayne, A. F., Woodford, L., and Zewe, F. (2017). Enumerating a continental-scale threat: how many feral cats are in Australia? Biological Conservation 206, 293-303.
| Crossref | Google Scholar |
Lepczyk, C. A., Mertig, A. G., and Liu, J. (2004). Landowners and cat predation across rural-to-urban landscapes. Biological Conservation 115, 191-201.
| Crossref | Google Scholar |
McGregor, H., Legge, S., Jones, M. E., and Johnson, C. N. (2015). Feral cats are better killers in open habitats, revealed by animal-borne video. PLoS One 10, e0133915.
| Crossref | Google Scholar | PubMed |
Medina, F. M., Bonnaud, E., Vidal, E., Tershy, B. R., Zavaleta, E. S., Josh Donlan, C., Keitt, B. S., Le Corre, M., Horwath, S. V., and Nogales, M. (2011). A global review of the impacts of invasive cats on island endangered vertebrates. Global Change Biology 17, 3503-3510.
| Crossref | Google Scholar |
Moseby, K. E., Hill, B. M., and Read, J. L. (2009). Arid recovery–a comparison of reptile and small mammal populations inside and outside a large rabbit, cat and fox‐proof exclosure in arid South Australia. Austral Ecology 34, 156-169.
| Crossref | Google Scholar |
Moseby, K. E., Peacock, D. E., and Read, J. L. (2015). Catastrophic cat predation: a call for predator profiling in wildlife protection programs. Biological Conservation 191, 331-340.
| Crossref | Google Scholar |
Oedin, M., Brescia, F., Millon, A., Murphy, B. P., Palmas, P., Woinarski, J. C. Z., and Vidal, E. (2021). Cats Felis catus as a threat to bats worldwide: a review of the evidence. Mammal Review 51, 323-337.
| Crossref | Google Scholar |
Phillips, S., Coburn, D., and James, R. (2001). An observation of cat predation upon an eastern blossom bat Syconycteris australis. Australian Mammalogy 23, 57-58.
| Crossref | Google Scholar |
Prevosti, A. (1974). La distancia genetica entre poblaciones. Miscellanea Alcobe 68, 109-118.
| Google Scholar |
Rocha, R. (2015). Look what the cat dragged in: Felis silvestris catus as predators of insular bats and instance of predation on the endangered Pipistrellus maderensis. Barbastella 8, 18-21.
| Crossref | Google Scholar |
Scrimgeour, J., Beath, A., and Swanney, M. (2012). Cat predation of short-tailed bats (Mystacina tuberculata rhyocobia) in rangataua forest, Mount Ruapehu, central North Island, New Zealand. New Zealand Journal of Zoology 39, 257-260.
| Crossref | Google Scholar |
Threatened Species Scientific Committee (2016). Conservation Advice Rhinonicteris aurantia (Pilbara form) (Pilbara Leaf-nosed Bat). Available at http://www.environment.gov.au/biodiversity/threatened/species/pubs/82790-conservation-advice-10032016.pdf [accessed 31 August 2023].
Welch, J. N., and Leppanen, C. (2017). The threat of invasive species to bats: a review. Mammal Review 47, 277-290.
| Crossref | Google Scholar |
Woolley, L. A., Geyle, H. M., Murphy, B. P., Legge, S. M., Palmer, R., Dickman, C. R., Augusteyn, J., Comer, S., Doherty, T. S., Eager, C., Edwards, G., Harley, D. K. P., Leiper, I., McDonald, P. J., McGregor, H. W., Moseby, K. E., Myers, C., Read, J. L., Riley, J., Stokeld, D., Turpin, J. M., and Woinarski, J. C. Z. (2019). Introduced cats Felis catus eating a continental fauna: inventory and traits of Australian mammal species killed. Mammal Review 49, 354-368.
| Crossref | Google Scholar |