Site fidelity trumps disturbance: aerial shooting does not cause surviving fallow deer (Dama dama) to disperse
Andrew J. Bengsen



A
B
C
D
Abstract
Aerial shooting is an important tool for managing the economic and environmental impacts of widespread wild fallow deer populations in eastern Australia and could be crucial for mounting an effective response to an emergency animal disease incursion. However, there is a concern that the disturbance caused by aerial shooting could cause infected animals to disperse, thereby transmitting pathogens to previously uninfected areas.
We sought to describe the nature and extent of spatial behavioural changes in fallow deer exposed to aerial shooting to: (1) assess the risk that aerial shooting poses to disease spread, and (2) better understand how aerial shooting can contribute to routine deer management programs.
We contrasted movement rates, activity range areas, and daily activity patterns of 48 GPS-collared fallow deer before, during and after exposure to aerial shooting at three sites in New South Wales.
No collared deer left its pre-shoot activity range area during shooting or within 30 days after shooting finished. Observed behaviour changes included increased daily and hourly distance travelled by female deer during and after shooting, increased activity range areas for female deer after shooting, and increased nocturnal activity in female and male deer during shooting. However, observed changes were minor, temporary, localised, and variable among sites.
Collared deer showed strong site fidelity despite repeated intense disturbance and substantial population reductions. We found no evidence to support concerns that aerial shooting poses a hazard of disease spread.
Aerial shooting should be retained as a key control tool for managing wild fallow deer populations in Australia, including for reducing disease host population densities in the event of an emergency animal disease incursion.
Keywords: antipredator behaviour, disease management, disturbance, helicopter, movement ecology, population control, predation risk, site fidelity.
Introduction
Wildlife populations around the world are actively managed to alter their abundance or distribution. This often involves direct interference and disturbance from activities such as hunting, capture or translocation. Human disturbance can cause a wide range of behavioural responses in wildlife, including altered movement rates, temporal shifts in peak activity periods, altered space use patterns within activity ranges, and spatial shifts in activity range location (e.g. Tablado and Jenni 2017). Major population reductions and disruptions to social networks can also cause surviving animals to modify their spatial behaviour for some time after the disruption (Ramsey et al. 2002; Williams et al. 2008; Ham et al. 2019). These short- and longer-term behaviour changes can have important implications for wildlife population management (Efford et al. 2000; Latham et al. 2018). Notably, intensive disturbance and population control from humans could contribute to the spread of infectious disease if it increases the probability of infected animals mixing with susceptible uninfected animals (Ham et al. 2019; Mysterud et al. 2020).
High-density deer (Cervidae) populations are often managed to reduce unwanted impacts on conservation assets, agricultural production, wildlife or livestock health, or human safety and amenity (Nugent et al. 2011; Forsyth et al. 2023a). Population management commonly relies on episodic lethal control using shooters on foot or mounted in terrestrial vehicles or helicopters (e.g. Forsyth et al. 2013; Comte et al. 2023). The behaviour of target animals and shooting teams in such operations can be conceptualised using predator–prey frameworks (Hone 1990; Tracey and Fleming 2007). Prey are expected to increase vigilance and defensive behaviour such as fleeing or seeking refuge in safe habitat upon detecting cues associated with the immediate presence of a potential predator (Brown 1999). These predator avoidance behaviours can then trigger further temporal or spatial activity shifts as animals attempt to compensate for foregone activities such as foraging and rest (Lima and Dill 1990). Deer can also shift to more nocturnal activity patterns when exposed to increased hunting pressure, thereby making them less accessible to diurnal hunters (Ikeda et al. 2019). Different population control methods present different forms and intensities of disturbance and are likely to elicit different behavioural responses from deer (Mysterud et al. 2020). Fight, flight, or freeze responses to the presence of predators can also vary between sexes (Stewart et al. 2022) and among habitat types (Bojarska et al. 2024), species (de Boer et al. 2004), and individuals (Ciuti et al. 2012).
In addition to predator avoidance behaviour, deer might also change their movement and landscape use patterns in response to severe population reduction or disruption to herd structure resulting from the removal of animals by shooting. Animals grazing in exposed landscapes and subject to attack by predators that pursue their prey are expected to experience strong pressure to forage in groups (Kie 1999). This may be due to increased foraging efficiency provided by high group-level vigilance (i.e. the ‘many eyes’ effect; Pulliam 1973), the lower individual-level risk of predation when a predator attacks a large group (i.e. the ‘dilution effect’; Turner and Pitcher 1986), or a combination of these and other factors (Roberts 1996). A study of a white-tailed deer (Odocoileus virginianus) population subjected to severe population reduction by shooting proposed that an observed increase in the activity range size of female deer immediately after the control operation was due to social restructuring as deer attempted to establish new foraging groups (Williams et al. 2008). For deer and other ungulates, the importance of establishing or joining new groups is expected to vary depending on the severity of the population reduction (Williams et al. 2008), interactions between sex and season, and the distribution and quality of foraging resources and refuge habitat (Roberts 1996; Kie 1999; Childress and Lung 2003).
Six deer species (fallow deer, Dama dama; red deer, Cervus elaphus; sambar deer, C. unicolor; rusa deer C. timorensis; chital deer, Axis axis; hog deer, A. porcinus) have established wild populations in Australia. Along with other wild ungulates such as feral pigs (Sus scrofa), goats (Capra hircus), and camels (Camelus dromedarius), deer populations could act as wildlife reservoirs for exotic animal diseases such as foot and mouth disease (Davis et al. 2016; Cripps et al. 2019). Given the likely catastrophic impacts of exotic animal disease incursions in Australia (Buetre et al. 2013), and the presence of economically-important diseases in neighbouring countries and trading partners (Mighell and Ward 2021; Susila et al. 2023), there is a pressing need for effective population control methods that can be used to safely manage disease outbreaks in wild ungulate populations in Australia.
Aerial shooting from helicopters can rapidly reduce deer population densities over extensive areas (Bengsen et al. 2023; Cox et al. 2023), as would likely be required in the event of an outbreak of an exotic animal disease in a wild deer population (Animal Health Australia 2023). Alternative population control tools such as ground-based shooting, trapping and fertility control are generally unable to achieve similar results (Merrill et al. 2006; Comte et al. 2023; Forsyth et al. 2023b). However, prolonged aerial shooting from a helicopter presents an intense, repeated disturbance that is likely to induce predator avoidance behaviour in deer (Latham et al. 2018), which could lead to spreading the disease into previously uninfected areas through infected animals (Animal Health Australia 2023). Social reorganisation after intensive population reduction could also lead to wider ranging behaviour in survivors (e.g. Williams et al. 2008) and increased mixing of infected and uninfected animals. Similar behaviour changes observed in badgers (Meles meles) that survived culling for bovine tuberculosis management appear to have increased the risk of disease transmission among badger social groups and between badgers and cattle (Ham et al. 2019).
Fallow deer populations are sympatric with domestic ungulates through large parts of eastern Australia and could serve as reservoirs for infectious diseases that harm livestock production and trade (Davis et al. 2016; Cripps et al. 2019). The short- and long-term behavioural responses of fallow deer to aerial shooting operations are unknown. Fallow deer in Italy increased vigilance behaviour and tended to select foraging areas with wide visibility when subjected to ground-based shooting (Pecorella et al. 2016). However, a study of 20 VHF-collared fallow deer in Tasmania concluded that ground-based shooting of a small number of deer for disease surveillance caused no detectable change in the spatial behaviour of survivors (Statham and Statham 1996). Fallow deer typically flee when approached by helicopters (Bengsen et al. 2021; Hampton et al. 2022), but the expected geographic extent and duration of predator avoidance behaviours are unknown. Consequently, it remains unclear whether sustained aerial shooting of deer could lead to behavioural responses that increase the risk of disease spread.
In this study, we used GPS-collared animals to examine the spatial behaviour of fallow deer immediately before, during, and after four aerial shooting operations at three sites in eastern Australia. Our goal was to assess whether aerial shooting provides a safe method for reducing population density in response to an exotic disease incursion. We estimated the magnitude and duration of changes in the distances travelled by deer during the day, activity range size, and the overlap between pre- and post-shoot activity ranges. We also assessed the extent to which deer: (1) shifted toward greater nocturnal activity patterns, and (2) showed a greater preference for heavily-wooded vegetation (vs open, grassy areas) during the day.
Materials and methods
Study areas and shooting operations
We captured 71 yearling or adult fallow deer at three sites in New South Wales (NSW), Australia, between June 2020 and April 2022 (Fig. 1). All three sites were characterised by a mixture of woodland and open country used for livestock production on undulating to hilly terrain. However, sites varied in topography, altitude, climate, and proportional woodland cover.
Location of three study sites in south-eastern Australia at which 48 GPS-collared fallow deer (Dama dama) were exposed to aerial shooting. There were two aerial shooting operations at A, and one each at B and C.
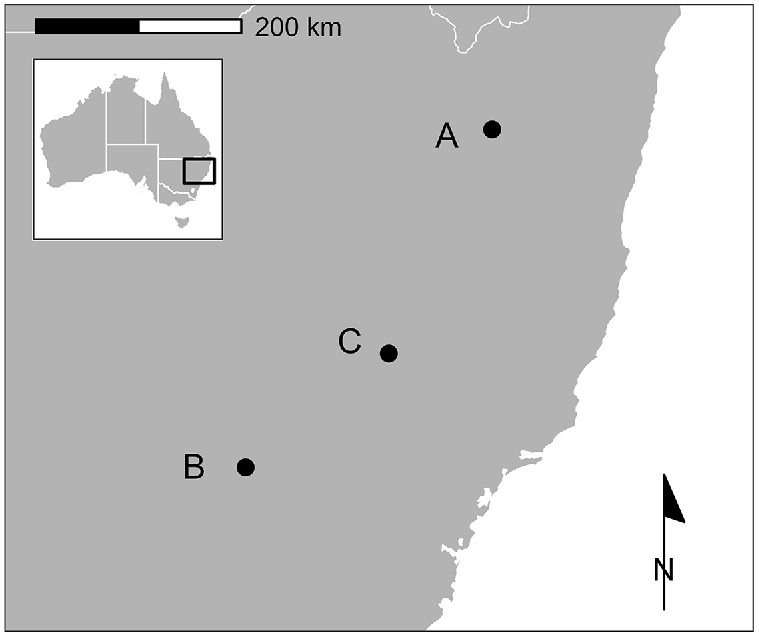
Deer were captured using helicopter net-gunning and fitted with GPS tracking collars programmed to log hourly location fixes (G5-2D, Advanced Telemetry Systems Inc., Isanti MN, USA; LiteTrack Iridium 420, Lotek Wireless Inc., Newmarket ON, Canada). Capture and handling procedures are detailed in Bengsen et al. (2021) and were approved by the Department of Primary Industries’ Orange Animal Ethics Committee (ORA 20-23-003, ORA 21-24-013). Fallow deer were the dominant deer species at each site, but red, chital and rusa deer were also present at some sites in much lesser numbers. Feral pigs were common at all sites but were less abundant than fallow deer. All sites had been subjected to one aerial shooting operation in the preceding 12 months and all collared deer had been pursued by a helicopter during capture and collaring, so no collared animals were naive to harassment by helicopters. Two does captured prior to Operation A2 were presumed to be a mother-daughter pair due to their relative ages and proximity at time of capture, but there was no indication that any other collared deer was a member of a consistent social group with another collared deer. There was a notable female bias in deer exposed to Operations A2 and C (Table 1) because we avoided capturing deer with large antlers that might be prone to injury while netted (Latham et al. 2020), and because some males in the sample died or lost their collars prior to shooting operations. It was not necessary to avoid large males in the sample exposed to Operation B because they were captured in October 2021 after having shed their antlers. All deer were collared between 2 and 10 months prior to shooting operations.
Operation | Date | Area (km2) | Flight time (h) | Deer killed | Deer killed km−2 | Population reduction | Deer collared | GPS fixes | GPS fix success rate (%) | |
---|---|---|---|---|---|---|---|---|---|---|
A1 | September 2020 | 135 | 24 | 778 | 5.7 | 42% A | 3m, 5f | 12,816 | 99.6 | |
A2 | September 2021 | 135 | 19 | 1060 | 7.9 | NA | 4m, 14f | 27,028 | 96.3 | |
B | April 2022 | 282 | 15 | 146 | 0.5 | NA | 7m, 4f | 14,732 | 78.7 | |
C | February 2023 | 491 | 101 | 3228 | 6.6 | 17% B | 2m, 9f | 17,950 | 84.2 |
NA, not available; m, male; f, female
Deer at each site were subjected to ≥1 aerial shooting operation targeting deer and feral pigs. Seven collared deer that were exposed to Operation A2 also provided data during Operation A1. Based on the age of deer at collaring, we expect that most deer exposed to Operation A2 were present during Operation A1. Shooting operations were conducted by NSW Government shooters in accord with the Feral Animal Aerial Shooting Team manual (FAAST 2020) using Bell 206 Jet Ranger or Eurocopter AS350 Squirrel helicopters, as detailed for NSW sites in previous studies (Hampton et al. 2022; Bengsen et al. 2023). Shooting Operations A1, A2, and B used one helicopter shooting team and lasted for 3 days. Operation C used two helicopter shooting teams operating concurrently for 11 days (Table 1). Shooting teams were instructed to avoid shooting collared deer but could shoot other deer in groups containing collared individuals. Helicopter search patterns generally approximated a Lévy walk, characterised by many small steps within geographic patches and fewer long steps between patches (Supplementary material Fig. S1). Shooting teams searched patches intensively for extended periods and revisited patches throughout the course of the operation. Consequently, we expect that individual deer were repeatedly exposed to disturbance and harassment from shooting teams.
Tracking data
For each aerial shooting operation, we examined approximately 2 months of deer tracking data: 30 days before shooting, the duration of the shoot, and 30 days after shooting. This window allowed us to combine enough data for robust before/after analyses of movement behaviour while minimising the bias due to seasonal variability in deer behaviours. However, Operations B and C were close to the expected peak fallow deer breeding season which usually commences around early April (Mulley 2023). We only considered deer that had tracking data for the complete time window (Table 1). Data were screened to remove implausible location fixes, defined as a movement spike with in and out speed of more than 15 km per hour and a turning angle ≤30°. We also removed any location fixes associated with a horizontal dilution of precision (hdop) >3.
Deer space use
We characterised the spatial activity ranges of deer using biased random bridges (Benhamou 2011) which incorporate the spatio-temporal autocorrelation of an animal’s tracking data in calculating their utilisation distribution (UD). We used the movement-based kernel density estimation on the 99% UD (Benhamou and Cornélis 2010) to describe deer activity ranges before the start and after the end of the aerial shooting operations. We estimated the overlap (between 0 and 1) of the post-shooting range with the pre-shooting range, as well as the relative change in range area (i.e. area post-shoot divided by area pre-shoot) as measures of disturbance from the shooting operations. A relative change in range area with 95% confidence intervals including 1 represented no change, whereas values >1 indicated an increase in range area after shooting and values between 0 and 1 represented a decrease in range area after shooting. For both ranging behaviours (i.e. range overlap and change in area), we fitted generalised linear models (GLMs) with sex, site (K = 3) and their interaction as explanatory variables. Due to the structure of the data, we could not include a year effect in the models. We used a beta distribution to model the range overlap (restricted between 0 and 1) and a Gamma distribution for the relative change in range area (positive values). Deer UD’s were calculated using the ‘adehabitat’ package version 0.4.20 (Calenge 2006) and all GLMs fitted with ‘glmmTMB’ version 1.1.5 (Brooks et al. 2017) in R version 4.2.3 (R Core Team 2023).
To describe the effect of intense disturbance on short-term spatial behaviour, we used an intervention analysis to identify structural changes in mean daily step length (mean hourly distance, MHD; Kay et al. 2017) and the daily maximum distance between any two locations (MxD; Kay et al. 2017) for female and male deer at each site before, during and after being exposed to aerial shooting. For step length, we only used steps with location fixes taken one hour apart. We first estimated the expected MHD and MxD for each combination of site, sex and shooting operation by extracting a time series of movement data starting 30 days prior to the shoot and finishing 30 days after the shoot and using a linear mixed effects model specifying day as a fixed effect and animal ID as a random intercept. Models were fitted using the lme4 package (Bates et al. 2015) in R. For each of the 16 resulting time series, we used the Kwiatkowski-Phillips-Schmidt-Shin (KPSS) unit root test to assess trend stationarity. We then identified breakpoints in the time series at which the linear regression coefficient changed from one stable relationship to a new one using the breakpoints function of the strucchange R-package (v.1.5-2 Zeileis et al. 2002). The optimal number and location of breakpoints for each time series were selected from the model with the minimal Bayesian information criterion. Adequacy of range size GLMs and time series models was assessed using standardised residual plots.
Fallow deer diel cycles
Fallow deer are commonly crepuscular, with peaks of feeding activity around sunset and sunrise (Mulley 2023). This is often associated with deer moving between dense cover that provides shelter and refuge during the day, and more open vegetation that provides richer grazing opportunities at night (Borkowski and Pudełko 2007). All aerial shooting activity occurred during daytime, and deer were often driven short distances out of tree cover into more open areas where they could be more easily shot. We expected deer to adjust the spatial and temporal distribution of their ranging behaviour by: (1) shifting to a more nocturnal activity pattern during shooting; and (2) increasing their use of wooded refuge habitat during daylight hours. For each of the four shooting operations, we used the hourly mean step length to characterise diel activity cycles of deer. We fitted a Gaussian generalised additive mixed model (GAMM) with sex (males and females) and period specific splines (before, during and after aerial shoot) to the step length (m) data. We used a cyclic cubic spline for the hour of the day to account for the circular nature of time and included animal ID as a random effect (intercepts and smooth terms).
To estimate the extent to which deer increased their use of woodlands during daylight hours, we modelled the proportion of tree cover across the three sites. We first used the NSW Native Vegetation Extent 5 m Raster (v1.2, Fisher et al. 2016) to create a 5-m grid with presence-absence (1 or 0) of tree cover. We then resampled the data to a 1-ha grid using pixel-averaging (i.e. sum of all 5-m grid cells/400). For each deer GPS location, we extracted the value of the matching grid cell (0 = no tree cover, 1 = 100% tree cover). For each shooting operation, we fitted a Gamma GAMM with sex and period specific splines (before, during and after aerial shoot) to the tree cover data. True zeros were changed to 0.00001, less than 1/400 = 0.0025 (i.e. the lowest empirical tree cover). We used a cyclic cubic spline for the hour of the day to account for the circular nature of time and included animal ID as a random effect (intercepts and smooth terms). Both diel cycle models were fitted using the ‘mgcv’ package (v1.8.41, Wood 2011) in R and visually checked for an absence of pattern in the model residuals and a k-index close to 1.
Results
Space use
Across the three sites, 48 deer (16 males, 32 females) provided useable location data from 30 days before to 30 days after the respective aerial shoot operations (Table 1). Male activity range areas prior to shooting operations (mean = 777.5 ha, 95% CI: 648.3–906.7 ha) were 2.5 times larger than those of females (mean = 318.9 ha, 95% CI: 227.5–410.2 ha). Expected male activity range area remained stable (i.e. confidence intervals including 1) after three of the four aerial shooting operations and increased 2.7 times after Operation C (Fig. 2). Female activity range area increased 1.4 and 1.7 times after operations A2 and B, respectively, and remained stable after operations A1 and C.
Expected mean (and 95% confidence interval) relative change in activity range area (UD 99%) for male and female fallow deer after being exposed to one of four aerial shooting operations. Horizontal dashed line (y = 1) indicates no change, i.e. values above the line show increases in ranging areas and values below the line show decreases in ranging areas after the shoot operations. For operation locations and timings, see Fig. 1 and Table 1, respectively.
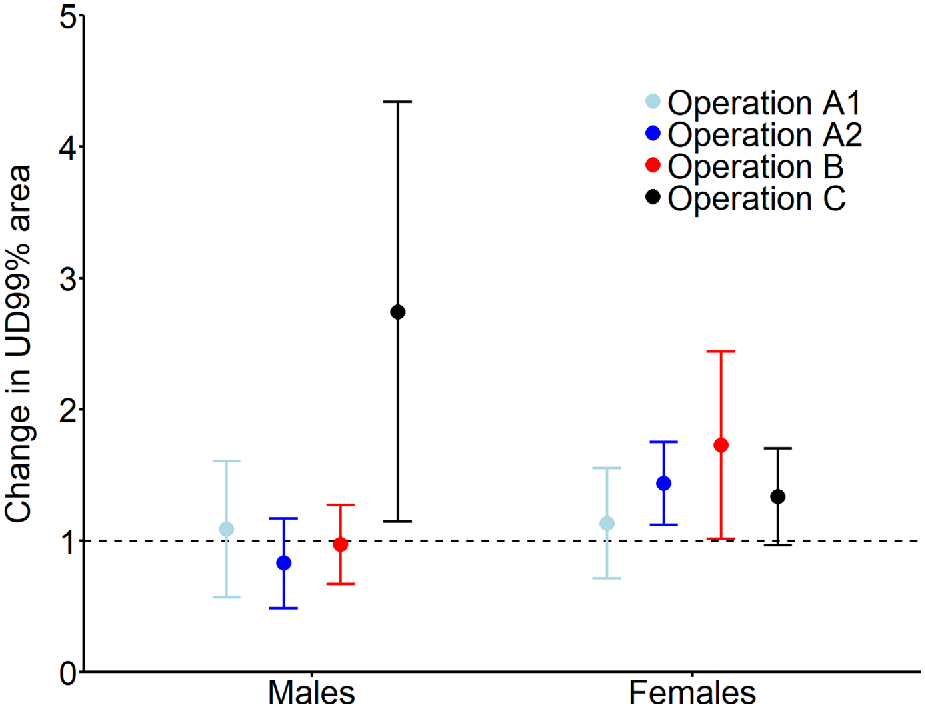
Post-shoot activity ranges of all deer overlapped with their pre-shoot range (mean overlap = 79%, range: 12–99%); no deer abandoned their pre-shoot range. The expected degree of range overlap of males varied among shooting operations (Fig. 3), with the lowest overlap after operation C (mean = 41%, 95% CI: 17–66%) and the highest after operation A1 (89%, 95% CI: 80–97%). Activity ranges of females were more stable than those of males, with a mean overlap above 80% for all operations (Fig. 3).
Expected mean (and 95% confidence interval) overlap of activity range areas of male and female fallow deer subject to one of four aerial shooting operations. The overlap represents the proportion of the activity range post-shoot that is shared with the activity range pre-shoot. For operation locations and timings, see Fig. 1 and Table 1, respectively.
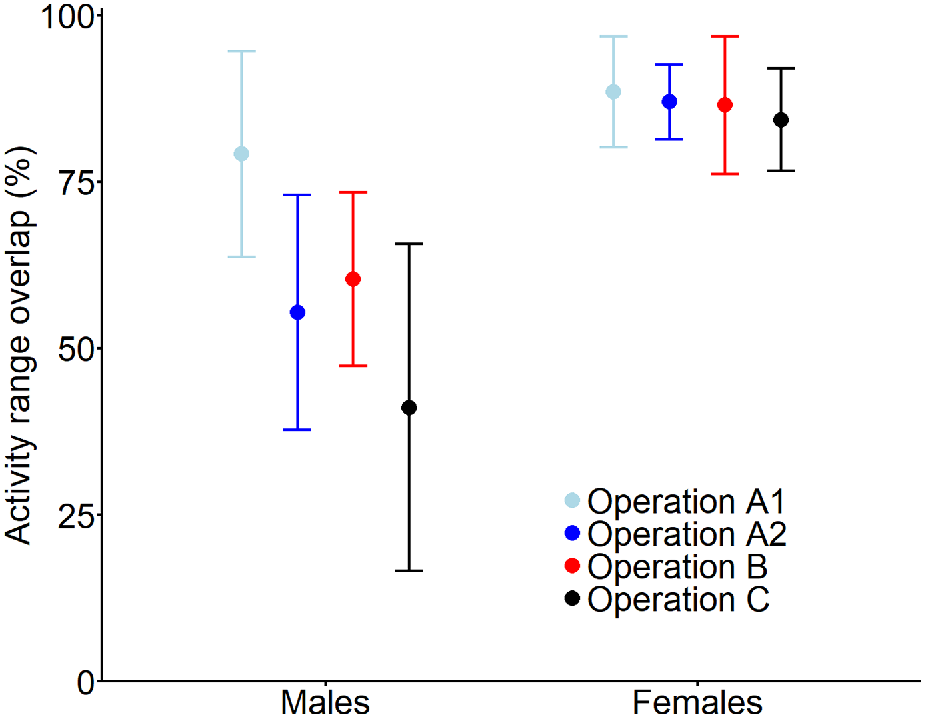
Step length and maximum daily distance
None of the eight MHD time series showed evidence of a unit root (KPSS test statistic < critical value for α = 0.05). Hence, all these time series were assumed to be stationary and suitable for further analysis. The optimal number of breakpoints for each time series ranged from 0 to 3. Breakpoints coincided with the completion of shooting for female deer in Operation A2 and the onset and completion of shooting for female deer in Operation C (Fig. 4). In Operation A2, expected female MHD increased by 40% from 101.5 m (s.e.m. = 6.2 m) to 142.1 m (s.e.m. = 7.0 m) immediately after the conclusion of the shoot and then returned to initial levels (142.1 m, s.e.m. = 6.0 m) after 8 days. In Operation C, expected female MHD increased by 68% from 89.7 m (s.e.m. = 11.0 m) to 150.5 m (s.e.m. = 11.5 m) with the onset of shooting and then decreased to 101.0 m (s.e.m. = 11.0 m) when shooting ceased. Male deer exposed to Operation C also showed increased MHD during the shoot, but the breakpoints in the time series were offset from the start and conclusion of shooting by 1 and 4 days, respectively. Breakpoints did not coincide with the start or finish of shooting for any other combination of sex and site.
Time series of mean hourly distance travelled (MHD) for female (red) and male (blue) fallow deer exposed to one of four aerial shooting operations in eastern Australia. Red and blue ribbons show 95% confidence intervals for each time series. Grey polygons show the timing of shooting operations. Dashed lines represent the fitted values from a model with the optimal number of breakpoints in the time series. For operation locations and timings, see Fig. 1 and Table 1, respectively.
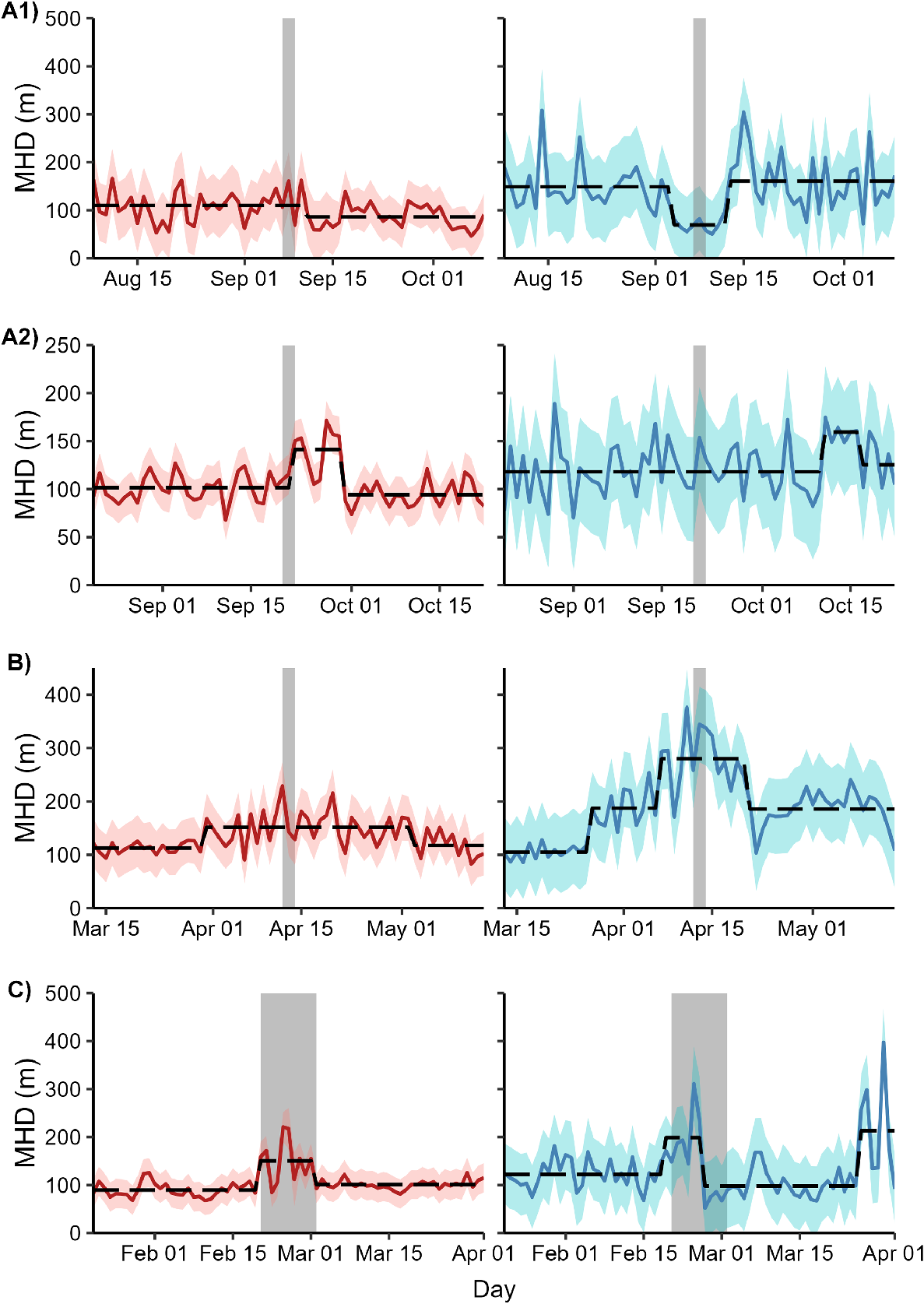
The optimal number of breakpoints for each MxD time series ranged from 0 to 3. For female deer exposed to Operation A2, the expected maximum daily distance between two points increased by 64% from 899 m (s.e.m. = 79.7 m) to 1473 m (s.e.m. = 90.0 m) 1 day after the cessation of the shoot. This increase lasted for 7 days before returning to pre-shoot levels. No breakpoints coincided with the start or completion of shooting for any other combination of sex and site (Fig. 5).
Time series of maximum daily distance between any two locations (MxD) for female (red) and male (blue) fallow deer exposed to one of four aerial shooting operations in eastern Australia. Red and blue polygons show 95% confidence intervals for each time series. Grey polygons show the timing of shooting operations. Dashed lines represent the fitted values from a model with the optimal number of breakpoints in the time series. For operation locations and timings, see Fig. 1 and Table 1, respectively.
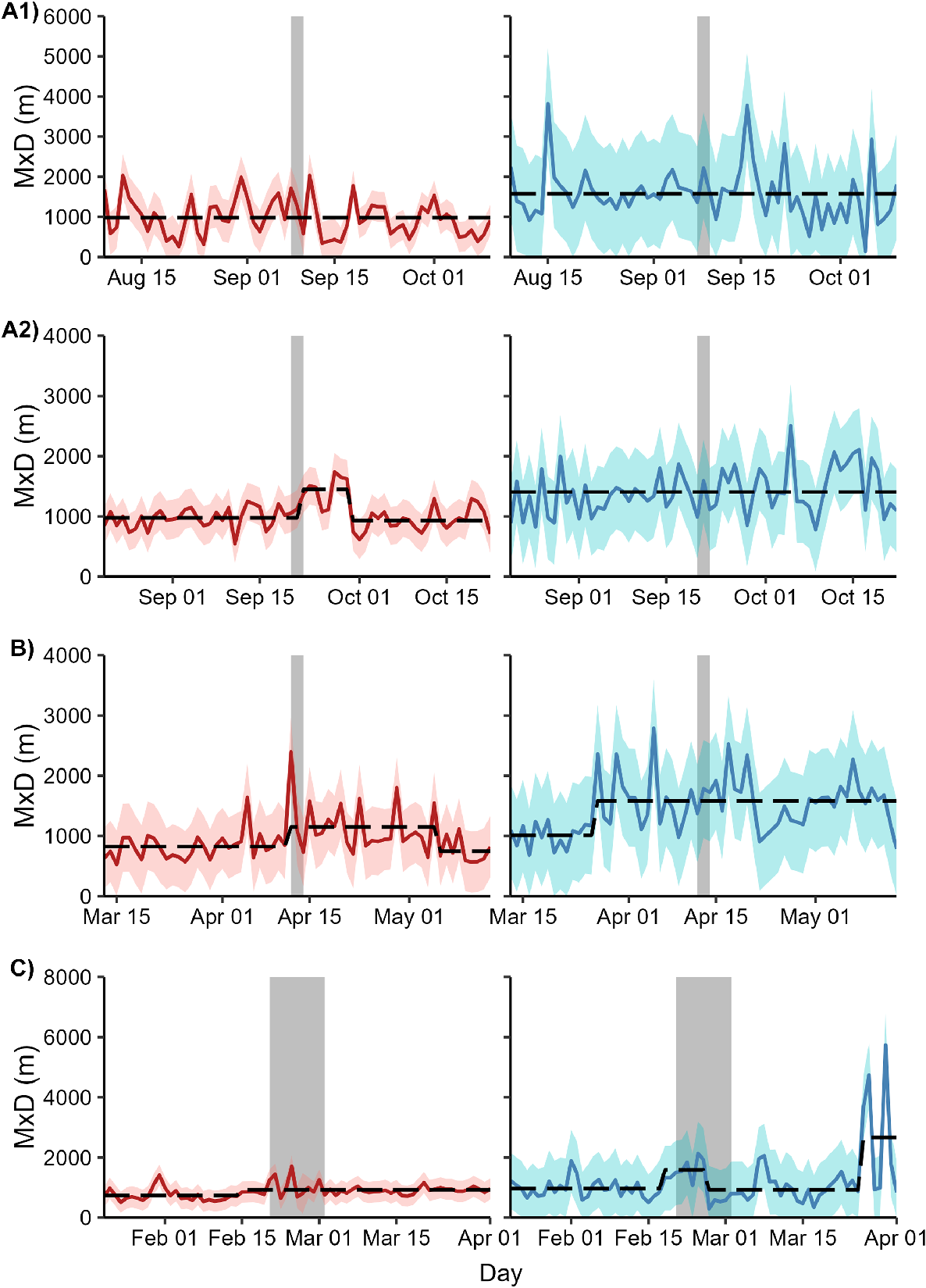
Diel cycle for step length
As expected, both male and female fallow deer showed strong crepuscular activity (i.e. movements concentrated around sunrise and sunset) before the aerial shoot operations on all three sites (Fig. 6). Deer activity patterns showed site-specific disruptions during the shooting operations. During Operation A1, females showed 60% increase in mean step length during daylight with a shift of movements towards dusk, but their night-time movements remained at the same level. Concomitantly, males abandoned their crepuscular activity for a more even activity distribution across night and day resulting in a 20% reduction in night-time activity (Fig. 6). The only change in activity observed during Operation A2 was a 30% increase in night-time movements for females. During Operation B, females maintained their crepuscular pattern and increased their night-time movements by 30%. During that operation, male movements doubled during the daytime (+115%) and night-time (+110%). During Operation C, females increased their movement by 65% during the day and 40% during the night, and males increased their night-time movements by 20%. After all shooting operations, both male and female fallow deer reverted to their crepuscular activity pattern with similar levels of movements as observed before the shooting operations (Fig. 6).
Expected mean (and 95% confidence intervals) diel cycle of movement activity (hourly step length) for female (red) and male (blue) fallow deer exposed to one of four aerial shooting operations in eastern Australia. Vertical dashed lines represent sunrise and sunset. For operation locations and timings, see Fig. 1 and Table 1, respectively.
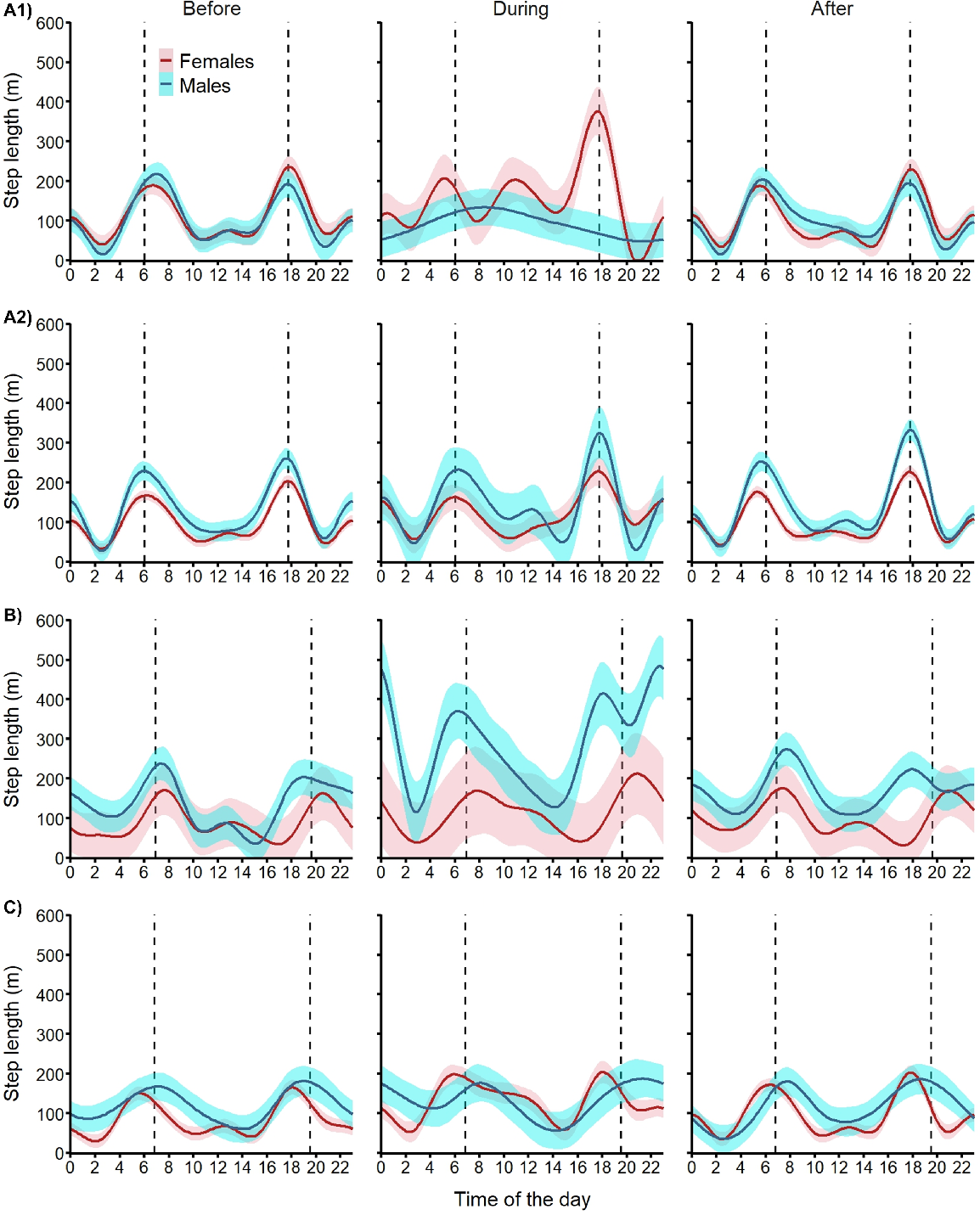
Diel cycle for tree cover
Our models described clear site- and sex-specific patterns of tree cover use by fallow deer before, during and after the aerial shooting operations (Fig. 7). At site A, before Operations A1 and A2, females used 20–30% denser tree cover than males and both sexes used 20–30% denser tree cover during daylight hours than at night. During Operation A1, while males kept the same pattern, females did not increase their use of tree cover during the day. After the shooting operation, both sexes returned to their pre-shoot patterns of tree cover use. Operation A2 had no effect on female use of tree cover (both during and after). In contrast, males used denser tree cover at night during the shooting operation and reverted to the pre-shoot pattern at the end of the shooting operation but used 10% higher tree cover. At site B, males used tree cover more evenly through the day and night and, although there was no change during the shoot, they used more open areas (10–20% lower tree cover) after the operation, especially at night. At this site, females showed a different pattern, spending the second half of the night in dense tree cover and moving to more open areas (<40% tree cover) during daylight and dusk. During shooting at site B, females kept the same pattern but used 20% denser tree cover at night and returned to pre-shoot levels after the operation. At site C, before the shooting operation, males and females showed similar patterns of tree cover use to deer at site A. During the shoot, males showed a more even use of tree cover at similar levels as before the shoot and kept this pattern after the shoot operation but at a 20% higher level of tree cover. Females at site C maintained their use of tree cover during and after the aerial shoot.
Expected mean (and 95% confidence intervals) diel cycle of tree cover use for female (red) and male (blue) fallow deer exposed to one of four aerial shooting operations in eastern Australia. Vertical dashed lines represent sunrise and sunset. For operation locations and timings, see Fig. 1 and Table 1, respectively.
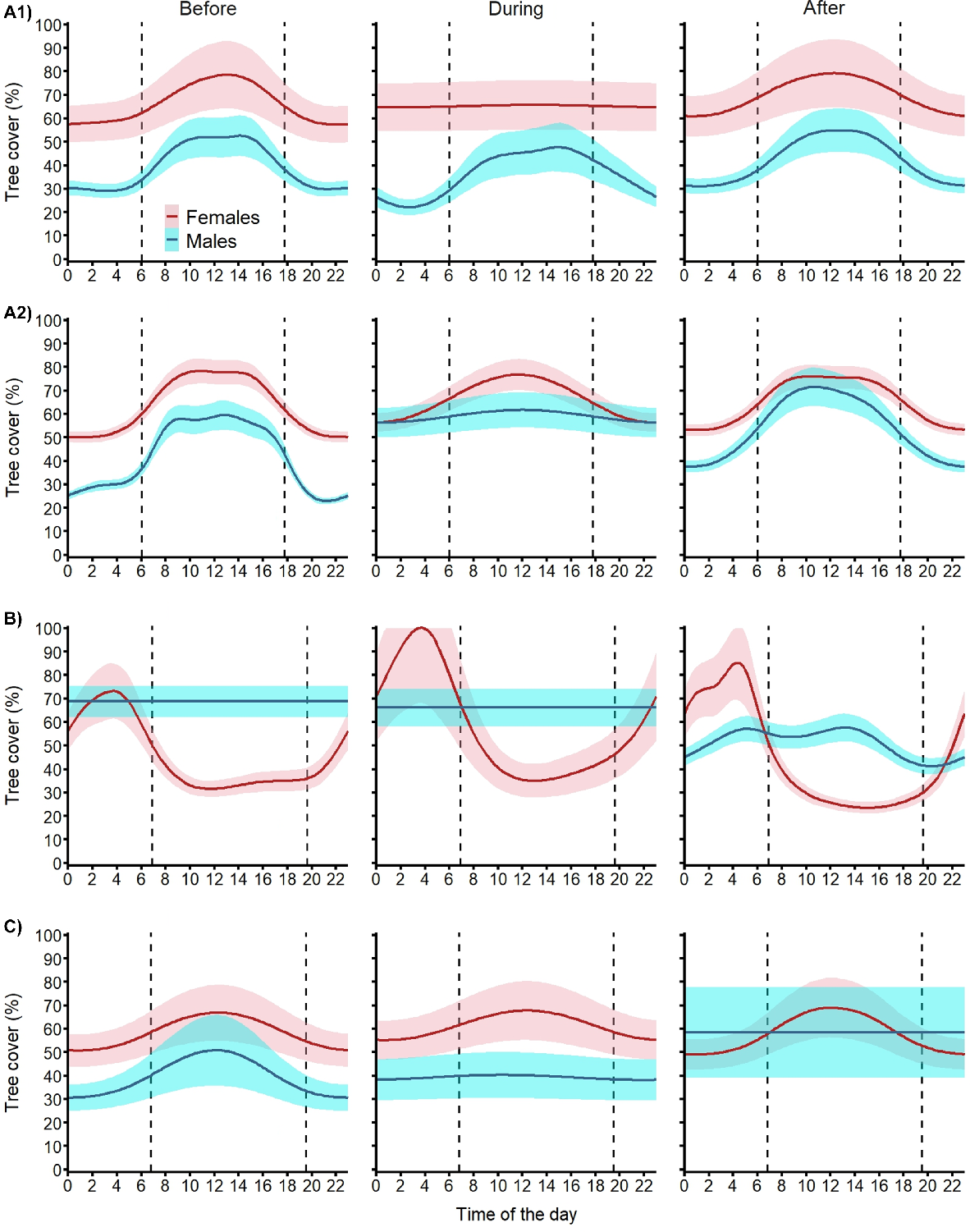
Discussion
The four aerial shooting operations examined in this study represented intense episodic disturbance during daylight hours for between 3 and 11 consecutive days and removed substantial numbers of deer from each population. Female fallow deer increased their activity range areas after two of the four operations and male fallow deer after one operation, but none of the 48 collared deer exposed to aerial shooting dispersed from its pre-shoot activity range into a new area. Females also increased their daily movements during or immediately after two operations, but these changes were short-lived. Both sexes showed temporary, idiosyncratic changes in diurnal and nocturnal movement patterns and in the use of tree cover during shooting operations.
Aerial shooting teams represent an unusual predator and disturbance source for deer. Shooting teams typically aim to maximise the number of deer killed per unit effort over a large area (10s-100s km2). This is achieved by frequently switching between patches of likely deer habitat to maintain a high site-wide kill rate as the number of deer killed per unit effort declines within a patch due to deer fleeing or being killed (Supplementary material). Pursuit and shooting of individual deer constitute an intense and loud disturbance that can last for several minutes (Hampton et al. 2022; Cox et al. 2023). The agility of fallow deer (relative to other species subjected to aerial shooting, Hampton et al. 2022; Bengsen et al. 2023) and the requirement to shoot animals multiple times before moving on to another animal, impose considerable processing time on each animal. Consequently, some deer that occur in large social groups escape (Hampton et al. 2022). These escapees can be expected to associate the distinctive auditory cues of an approaching helicopter with a brief, but high, risk of being killed. Given that all collared deer had experienced pursuit by helicopter during capture, and possibly also during previous shooting operations, no animals in our sample were naive to these cues. Theory and experience suggest that animals should show their strongest anti-predator behaviour when they detect strong cues indicative of brief, high risk predation events (Lima and Bednekoff 1999; Fisher and Stankowich 2018). Persecution by aerial shooting teams should, therefore, provide a powerful prompt for deer to deploy strong anti-predator behaviour, including changes in the ways in which they inhabit and use the landscape. However, the only clear indicators of coarse-scale behaviour change during shooting operations were the apparent disruption of normal crepuscular activity cycles for both sexes during all shooting operations and the increase in mean hourly step length with the onset of shooting for female deer in Operation C.
The increased nocturnal movement rates apparent in our data were consistent with expectations for deer compensating for foregone diurnal feeding and other activities as a result of avoiding or evading a diurnally-active predator. Similar shifts towards greater nocturnal activity have been reported in other deer species exposed to different levels of recreational hunting pressure (e.g. Ikeda et al. 2019; Bonnot et al. 2020). In addition to increased nocturnal activity, the magnitude and variability of diurnal movement rates tended to increase for female deer in Operations A1 and C and male deer in Operation B. This may be due to deer being intermittently pursued by helicopters during the day, as shooting teams sometimes reported pursuing groups that included collared deer. Despite increases in nocturnal or diurnal activity during shooting, only female deer in Operation C showed a clear and consistent increase in hourly step length over the duration of shooting. However, there was no comparable increase in the mean maximum distance between location fixes for these deer. Shooting therefore appears to have caused the nine female deer exposed to Operation C to increase their movement rates during shooting, but not the area over which they moved each day. Only two male collared deer were exposed to this operation, so there were insufficient data to determine whether this behaviour change was restricted to females. Contrary to expectations (e.g. Lone et al. 2015) and the tendency of deer to flee towards tree cover when pursued (Bengsen et al. 2023), neither female nor male deer showed clear indication of increased and persistent use of dense tree cover as predator refuge during daylight hours in any operation.
Some female deer altered their spatial behaviour immediately after shooting. The increased mean hourly step length and mean maximum distance between location fixes evident in female deer for 8 days immediately after Operation A2 were consistent with expectations for a population in which animals temporarily increase their mobility while attempting to join or create new foraging groups (e.g. Williams et al. 2008). Furthermore, the 30-day activity range areas of female deer tended to increase after shooting for two of the four operations, including A2. Group formation and maintenance conveys important benefits for predator avoidance and foraging efficiency in grazing species, such as fallow deer, that use open habitats (Kie 1999). Directly pertinent to the present study, the probability of an individual deer escaping an aerial shooting team tends to increase with group size (Hampton et al. 2022), consistent with the ‘dilution effect’ hypothesis (Turner and Pitcher 1986). Consequently, we expect that deer that have lost group-mates to aerial shooting will experience strong pressure to join or form new groups. Indeed, this phenomenon has been exploited in population control operations for other ungulates by releasing collared ‘Judas’ animals that find new herds and betray their location to managers (Taylor and Katahira 1988). Despite the increases in distance travelled and activity range areas, the spatial overlap of pre- and post-shoot activity ranges of female deer was >80%, indicating strong site fidelity. Spatial behaviour changes in female deer after shooting were therefore localised and did not represent a shift away from previously inhabited areas.
Male deer exposed to Operation C showed a noticeable increase in post-shoot activity range area, but female deer did not. The increase in male range area was consistent with both movement metrics (mean hourly step length and maximum distance between location) suddenly increasing 21 days after the shoot, corresponding with the expected lead up to the rut in early April. A similar increase in both metrics was observed in male deer in late March the previous year, prior to the start of Operation B (Fig. 4). At this time of year, adult male fallow deer often move to lekking stands and attempt to secure exclusive access to female social groups, while subordinate males persistently seek mating opportunities around the fringes of these groups (Mulley 2023). Both these behaviours can be expected to result in increased movement and expansive ranging behaviour. Our findings are similar to those of previous studies in Europe and New Zealand in which activity ranges of male fallow deer increased around the rut (Nugent 1994; Davini et al. 2004). The observed changes in ranging behaviour of males after Operation C are therefore likely to be an artefact of seasonal behaviour rather than a response to aerial shooting. In contrast to a study of fallow deer in Italy (Ciuti et al. 2003), but similar to findings from New Zealand (Nugent 1994), there was no clear indication of an increase in female range area or activity associated with the rut.
The strong site fidelity observed in our study, despite repeated exposure to intense disturbance and the death of conspecifics, is consistent with studies of other deer species exposed to disturbances such as large wildfires, human and non-human predators, and low-level helicopter survey flights (Gehr et al. 2020; Kreling et al. 2021; Dyal et al. 2022). Male deer showed lower pre- and post-shoot range overlap than females, most likely due to the larger areas over which they ranged and the tendency for male ranges to be less stable than those of females (e.g. Nugent 1994). Nonetheless, all male deer maintained at least 12% overlap with their pre-shoot range. Strong site fidelity is common in mammalian herbivores and is expected to confer fitness benefits due to its positive effects on resource acquisition efficiency and predation avoidance (Switzer 1993). Notably, animals should be more prone to predation in unfamiliar environments than in familiar ones due to poorer predator awareness, greater exposure to predators during exploratory behaviour, and poorer knowledge of escape routes and refuge habitats (Metzgab 1967). For example, a study of GPS-collared black-tailed deer (Odocoileus hemionus) found that those deer that had a high probability of leaving their activity range over a given interval experienced a four-fold increase in mortality risk, largely due to increased predation (Forrester et al. 2015). However, game theory suggests that site fidelity may be a maladaptive strategy for prey animals facing a highly efficient predator with spatial memory (Mitchell and Lima 2002), such as an aerial shooting team.
Study limitations
There were several limitations inherent in our sample of collared animals that must be considered when interpreting our results and making recommendations for management. First, all collared deer had previously been exposed to harassment and capture by helicopter, and it is likely that many deer had also been exposed to at least one aerial shooting operation prior to being collared, but our study wasn’t designed to specifically test whether naive deer might have behaved differently. However, as discussed above, we expect that deer that have already been exposed to cues associated with aerial shooting operations should deploy stronger anti-predator behaviour than naive animals. Second, the female sex bias in deer exposed to Operations C and A2 meant that only two and four males, respectively, were exposed to these operations. Future studies using similar methods could reduce sex bias by capturing deer after they have shed antlers and by reducing the interval between capture and exposure to shooting or other treatments. Third, Operation B coincided with the rut, and it is possible that mating behaviour may have obscured some responses to aerial shooting, particularly in males. Fourth, the 60-min interval between location fixes used in our study was too long to identify contacts between individual deer and helicopters, and to characterise immediate avoidance behaviour observed by aerial shooting teams (e.g. Hampton et al. 2022). However, such short-term behaviours are unlikely to cause functionally-important changes in landscape use or disease spread risk unless they accumulate to the point at which they become apparent at coarser time scales that would have been observable in our study. Finally, only deer that were visually assessed to be healthy and in good condition were fitted with collars. Deer that are unwell or in poor condition could behave differently to those studied here, particularly if they are afflicted with a condition that impairs mobility.
Management implications
Contrary to concerns expressed in some wild animal and disease management doctrine (e.g. Animal Health Australia 2023), aerial shooting did not cause any deer to disperse into new areas, and the tendency for deer to become more active at night was not associated with decreased diurnal activity and reduced availability to aerial shooting teams. Inconsistencies in other spatial behaviours between sexes and among operations indicate that some responses of deer to aerial shooting are likely to be context-specific.
Three of the four operations examined were representative of typical practice for population control operations aiming to reduce the impacts of deer on livestock production, using one helicopter shooting team for fewer than 6 consecutive days (e.g. Bengsen et al. 2023). The only clear evidence of behaviour change in these operations was the increased step length in female deer immediately after shooting in one operation, and an increase in activity range area for female deer after two operations. Operation C represented a more intense and sustained disturbance, with two helicopter shooting teams operating concurrently for 11 days. This may be more representative of a disease management operation in which control effort is maintained until a target population density below a disease transmission threshold is reached. Operation C was the only operation in which deer showed a clear and consistent change in spatial behaviour during shooting.
The strong site fidelity evident in collared deer exposed to intense persecution over several days suggests that fallow deer in situations similar to those in our study are unlikely to leave familiar activity range areas if it is not necessary for survival. Consequently, deer that survive initial aerial shooting operations can be expected to remain in situ and be available for follow-up lethal control.
Conclusion
The spatial behavioural changes of GPS-collared fallow deer in response to disturbance and population density reduction from aerial shooting were minor, localised, temporary, and inconsistent at three sites in New South Wales. None of the spatial behaviour changes observed in deer exposed to aerial shooting are likely to increase the risk of deer transmitting pathogens to previously uninfected animals beyond the targeted area. Given the limitations and impracticality of alternative methods for rapidly reducing large wild deer populations, and the minor impact on deer behaviour observed in our study, aerial shooting should be retained as a viable population control tool in case of an emergency animal disease outbreak in wild fallow deer in Australia.
Data availability
The data that support this study cannot be publicly shared due to ethical or privacy reasons and may be shared upon reasonable request to the corresponding author if appropriate.
Declaration of funding
Seed funding for initial collaring operations was provided by NSW DPI’s Game Licencing Unit. Subsequent funding was provided by the NSW State Government’s Special Purpose Pest Management Rate. The 2023 Liverpool Plains shoot (Operation C) was funded by the project ‘Reducing feral deer and pig impacts in NSW hotspots’ which was jointly funded by the NSW Department of Primary Industries and the Australian Government.
Acknowledgements
We thank Andrew Moriarty (NSW DPI) for initial encouragement. Lachie Onslow (Fleet Helicopters) and Stuart Boyd-Law (Pest Animal Control and Training) were instrumental in developing deer capture methods and capturing all animals. Troy Crittle and Pip Taylor (NSW DPI) assisted with animal handling. We thank Mark Tarrant (Northern Tablelands Local Land Services), Clayton Burey (Northern Tablelands Local Land Services), Nigel Boyce (Central West Local Land Services), Rhett Robinson (Central West Local Land Services), Greg Lumber (North West Local Land Services), and private landholders for mediating and providing access to study sites for capture operations and collar recovery. Tarnya Cox, Deane Smith, and two anonymous reviewers provided valuable feedback on earlier versions of this manuscript.
References
Bates D, Mächler M, Bolker B, Walker S (2015) Fitting linear mixed-effects models using lme4. Journal of Statistical Software 67, 1-48.
| Crossref | Google Scholar |
Bengsen AJ, Hampton JO, Comte S, Freney S, Forsyth DM (2021) Evaluation of helicopter net-gunning to capture wild fallow deer (Dama dama). Wildlife Research 48, 722-729.
| Crossref | Google Scholar |
Bengsen AJ, Forsyth DM, Pople A, Brennan M, Amos M, Leeson M, Cox TE, Gray B, Orgill O, Hampton JO, Crittle T, Haebich K (2023) Effectiveness and costs of helicopter-based shooting of deer. Wildlife Research 50, 617-631.
| Crossref | Google Scholar |
Benhamou S (2011) Dynamic approach to space and habitat use based on biased random bridges. PLoS ONE 6, e14592.
| Crossref | Google Scholar |
Benhamou S, Cornélis D (2010) Incorporating movement behavior and barriers to improve kernel home range space use estimates. The Journal of Wildlife Management 74, 1353-1360.
| Crossref | Google Scholar |
Bojarska K, Gerber N, Herzog S, Isselstein J, Meiβner M, Riesch F, Signer J, van Beeck Calkoen S, Zetsche M, Balkenhol N (2024) Red deer responses to drive hunts are related to habitat and landscape characteristics. The Journal of Wildlife Management 88, e22583.
| Crossref | Google Scholar |
Bonnot NC, Couriot O, Berger A, Cagnacci F, Ciuti S, De Groeve JE, Gehr B, Heurich M, Kjellander P, Kröschel M, Morellet N, Sönnichsen L, Hewison AJM (2020) Fear of the dark? Contrasting impacts of humans versus lynx on diel activity of roe deer across Europe. Journal of Animal Ecology 89, 132-145.
| Crossref | Google Scholar | PubMed |
Borkowski J, Pudełko M (2007) Forest habitat use and home-range size in radio-collared fallow deer. Annales Zoologici Fennici 44, 107-114.
| Google Scholar |
Brooks ME, Kristensen K, van Benthem KJ, Magnusson A, Berg CW, Nielsen A, Skaug HJ, Mächler M, Bolker BM (2017) glmmTMB balances speed and flexibility among packages for zero-inflated generalized linear mixed modeling. The R Journal 9, 378-400.
| Crossref | Google Scholar |
Brown JS (1999) Vigilance, patch use and habitat selection: foraging under predation risk. Evolutionary Ecology Research 1, 49-71.
| Google Scholar |
Calenge C (2006) The package “adehabitat” for the R software: a tool for the analysis of space and habitat use by animals. Ecological Modelling 197, 516-519.
| Crossref | Google Scholar |
Childress MJ, Lung MA (2003) Predation risk, gender and the group size effect: does elk vigilance depend upon the behaviour of conspecifics? Animal Behaviour 66, 389-398.
| Crossref | Google Scholar |
Ciuti S, Davini S, Luccarini S, Apollonio M (2003) Variation in home range size of female fallow deer inhabiting a sub-Mediterranean habitat. Revue d’Écologie 58, 381-395.
| Crossref | Google Scholar |
Ciuti S, Muhly TB, Paton DG, McDevitt AD, Musiani M, Boyce MS (2012) Human selection of elk behavioural traits in a landscape of fear. Proceedings of the Royal Society B: Biological Sciences 279, 4407-4416.
| Crossref | Google Scholar |
Comte S, Thomas E, Bengsen AJ, Bennett A, Davis NE, Brown D, Forsyth DM (2023) Cost-effectiveness of volunteer and contract ground-based shooting of sambar deer in Australia. Wildlife Research 50, 642-656.
| Crossref | Google Scholar |
Cox TE, Paine D, O’Dwyer-Hall E, Matthews R, Blumson T, Florance B, Fielder K, Tarran M, Korcz M, Wiebkin A, Hamnett PW, Bradshaw CJA, Page B (2023) Thermal aerial culling for the control of vertebrate pest populations. Scientific Reports 13, 10063.
| Crossref | Google Scholar | PubMed |
Cripps JK, Pacioni C, Scroggie MP, Woolnough AP, Ramsey DSL (2019) Introduced deer and their potential role in disease transmission to livestock in Australia. Mammal Review 49, 60-77.
| Crossref | Google Scholar |
Davini S, Ciuti S, Luccarini S, Apollonio M (2004) Home range patterns of male fallow deer Dama dama in a sub-mediterranean habitat. Acta Theriologica 49, 393-404.
| Crossref | Google Scholar |
Davis NE, Bennett A, Forsyth DM, Bowman DMJS, Lefroy EC, Wood SW, Woolnough AP, West P, Hampton JO, Johnson CN (2016) A systematic review of the impacts and management of introduced deer (family Cervidae) in Australia. Wildlife Research 43, 515-532.
| Crossref | Google Scholar |
de Boer HY, van Breukelen L, Hootsmans MJM, van Wieren SE (2004) Flight distance in roe deer Capreolus capreolus and fallow deer Dama dama as related to hunting and other factors. Wildlife Biology 10, 35-41.
| Crossref | Google Scholar |
Dyal JR, Miller KV, Cherry MJ, D’Angelo GJ (2022) White-tailed deer movement in response to helicopter surveys. Wildlife Society Bulletin 46, e1383.
| Crossref | Google Scholar |
Efford M, Warburton B, Spencer N (2000) Home-range changes by brushtail possums in response to control. Wildlife Research 27, 117-127.
| Crossref | Google Scholar |
Fisher KA, Stankowich T (2018) Antipredator strategies of striped skunks in response to cues of aerial and terrestrial predators. Animal Behaviour 143, 25-34.
| Crossref | Google Scholar |
Fisher A, Day M, Gill T, Roff A, Danaher T, Flood N (2016) Large-area, high-resolution tree cover mapping with multi-temporal SPOT5 imagery, New South Wales, Australia. Remote Sensing 8, 515.
| Crossref | Google Scholar |
Forrester TD, Casady DS, Wittmer HU (2015) Home sweet home: fitness consequences of site familiarity in female black-tailed deer. Behavioral Ecology and Sociobiology 69, 603-612.
| Crossref | Google Scholar |
Forsyth DM, Ramsey DSL, Veltman CJ, Allen RB, Allen WJ, Barker RJ, Jacobson CL, Nicol SJ, Richardson SJ, Todd CR (2013) When deer must die: large uncertainty surrounds changes in deer abundance achieved by helicopter- and ground-based hunting in New Zealand forests. Wildlife Research 40, 447-458.
| Crossref | Google Scholar |
Forsyth DM, Pople A, Nugent G (2023a) Ecology, impacts and management of wild deer in Australia. Wildlife Research 50, i-vii.
| Crossref | Google Scholar |
Gehr B, Bonnot NC, Heurich M, Cagnacci F, Ciuti S, Hewison AJM, Gaillard J-M, Ranc N, Premier J, Vogt K, Hofer E, Ryser A, Vimercati E, Keller L (2020) Stay home, stay safe – site familiarity reduces predation risk in a large herbivore in two contrasting study sites. Journal of Animal Ecology 89, 1329-1339.
| Crossref | Google Scholar | PubMed |
Ham C, Donnelly CA, Astley KL, Jackson SYB, Woodroffe R (2019) Effect of culling on individual badger Meles meles behaviour: potential implications for bovine tuberculosis transmission. Journal of Applied Ecology 56, 2390-2399.
| Crossref | Google Scholar | PubMed |
Hampton JO, Bengsen AJ, Pople A, Brennan M, Leeson M, Forsyth DM (2022) Animal welfare outcomes of helicopter-based shooting of deer in Australia. Wildlife Research 49, 264-273.
| Crossref | Google Scholar |
Hone J (1990) Predator-prey theory and feral pig control, with emphasis on evaluation of shooting from a helicopter. Australian Wildlife Research 17, 123-130.
| Crossref | Google Scholar |
Ikeda T, Takahashi H, Igota H, Matsuura Y, Azumaya M, Yoshida T, Kaji K (2019) Effects of culling intensity on diel and seasonal activity patterns of sika deer (Cervus nippon). Scientific Reports 9, 17205.
| Crossref | Google Scholar | PubMed |
Kay SL, Fischer JW, Monaghan AJ, Beasley JC, Boughton R, Campbell TA, Cooper SM, Ditchkoff SS, Hartley SB, Kilgo JC, Wisely SM, Wyckoff AC, VerCauteren KC, Pepin KM (2017) Quantifying drivers of wild pig movement across multiple spatial and temporal scales. Movement Ecology 5, 14.
| Crossref | Google Scholar | PubMed |
Kie JG (1999) Optimal foraging and risk of predation: effects on behavior and social structure in ungulates. Journal of Mammalogy 80, 1114-1129.
| Crossref | Google Scholar |
Kreling SES, Gaynor KM, McInturff A, Calhoun KL, Brashares JS (2021) Site fidelity and behavioral plasticity regulate an ungulate’s response to extreme disturbance. Ecology and Evolution 11, 15683-15694.
| Crossref | Google Scholar | PubMed |
Latham ADM, Cecilia Latham M, Herries D, Barron M, Cruz J, Anderson DP (2018) Assessing the efficacy of aerial culling of introduced wild deer in New Zealand with analytical decomposition of predation risk. Biological Invasions 20, 251-266.
| Crossref | Google Scholar |
Latham ADM, Davidson B, Warburton B, Yockney I, Hampton JO (2020) Efficacy and animal welfare impacts of novel capture methods for two species of invasive wild mammals in New Zealand. Animals 10, 44.
| Crossref | Google Scholar |
Lima SL, Bednekoff PA (1999) Temporal variation in danger drives antipredator behavior: the predation risk allocation hypothesis. The American Naturalist 153, 649-659.
| Crossref | Google Scholar | PubMed |
Lima SL, Dill LM (1990) Behavioral decisions made under the risk of predation: a review and prospectus. Canadian Journal of Zoology 68, 619-640.
| Crossref | Google Scholar |
Lone K, Loe LE, Meisingset EL, Stamnes I, Mysterud A (2015) An adaptive behavioural response to hunting: surviving male red deer shift habitat at the onset of the hunting season. Animal Behaviour 102, 127-138.
| Crossref | Google Scholar |
Merrill JA, Cooch EG, Curtis PD (2006) Managing an overabundant deer population by sterilization: effects of immigration, stochasticity and the capture process. Journal of Wildlife Management 70, 268-277.
| Crossref | Google Scholar |
Metzgab LH (1967) An experimental comparison of screech owl predation on resident and transient white-footed mice (Peromyscus leucopus). Journal of Mammalogy 48, 387-391.
| Crossref | Google Scholar |
Mighell E, Ward MP (2021) African swine fever spread across Asia, 2018–2019. Transboundary and Emerging Diseases 68, 2722-2732.
| Crossref | Google Scholar | PubMed |
Mitchell WA, Lima SL (2002) Predator-prey shell games: large-scale movement and its implications for decision-making by prey. Oikos 99, 249-259.
| Crossref | Google Scholar |
Mysterud A, Rauset GR, Van Moorter B, Andersen R, Strand O, Rivrud IM (2020) The last moves: the effect of hunting and culling on the risk of disease spread from a population of reindeer. Journal of Applied Ecology 57, 2509-2518.
| Crossref | Google Scholar |
Nugent G (1994) Home range size and its development for fallow deer in the Blue Mountains, New Zealand. Acta Theriologica 39, 159-175.
| Crossref | Google Scholar |
Nugent G, McShea WJ, Parkes J, Woodley S, Waithaka J, Moro J, Gutierrez R, Azorit C, Mendez Guerrero F, Flueck WT, Smith-Flueck JM (2011) Policies and management of overabundant deer (native or exotic) in protected areas. Animal Production Science 51, 384-389.
| Crossref | Google Scholar |
Pecorella I, Ferretti F, Sforzi A, Macchi E (2016) Effects of culling on vigilance behaviour and endogenous stress response of female fallow deer. Wildlife Research 43, 189-196.
| Crossref | Google Scholar |
Pulliam HR (1973) On the advantages of flocking. Journal of Theoretical Biology 38, 419-422.
| Crossref | Google Scholar | PubMed |
Ramsey D, Spencer N, Caley P, Efford M, Hansen K, Lam M, Cooper D (2002) The effects of reducing population density on contact rates between brushtail possums: implications for transmission of bovine tuberculosis. Journal of Applied Ecology 39, 806-818.
| Crossref | Google Scholar |
Roberts G (1996) Why individual vigilance declines as group size increases. Animal Behaviour 51, 1077-1086.
| Crossref | Google Scholar |
Stewart DG, Gulsby WD, Ditchkoff SS, Collier BA (2022) Spatiotemporal patterns of male and female white-tailed deer on a hunted landscape. Ecology and Evolution 12, e9277.
| Crossref | Google Scholar | PubMed |
Susila EB, Daulay RSD, Hidayati DN, Prasetyowati SRB, Wriningati , Andesfha E, Irianingsih SH, Dibia IN, Faisal , Supriyadi A, Yupiana Y, Hidayat MM, Zainuddin N, Wibawa H (2023) Detection and identification of foot-and-mouth disease O/ME-SA/Ind-2001 virus lineage, Indonesia, 2022. Journal of Applied Animal Research 51, 487-494.
| Crossref | Google Scholar |
Switzer PV (1993) Site fidelity in predictable and unpredictable habitats. Evolutionary Ecology 7, 533-555.
| Crossref | Google Scholar |
Tablado Z, Jenni L (2017) Determinants of uncertainty in wildlife responses to human disturbance. Biological Reviews 92, 216-233.
| Crossref | Google Scholar | PubMed |
Taylor D, Katahira L (1988) Radio telemetry as an aid in eradicating remnant feral goats. Wildlife Society Bulletin 16, 297-299.
| Google Scholar |
Tracey JP, Fleming PJS (2007) Behavioural responses of feral goats (Capra hircus) to helicopters. Applied Animal Behaviour Science 108, 114-128.
| Crossref | Google Scholar |
Turner GF, Pitcher TJ (1986) Attack abatement: a model for group protection by combined avoidance and dilution. The American Naturalist 128, 228-240.
| Crossref | Google Scholar |
Williams SC, DeNicola AJ, Ortega IM (2008) Behavioral responses of white-tailed deer subjected to lethal management. Canadian Journal of Zoology 86, 1358-1366.
| Crossref | Google Scholar |
Wood SN (2011) Fast stable restricted maximum likelihood and marginal likelihood estimation of semiparametric generalized linear models. Journal of the Royal Statistical Society Series B: Statistical Methodology 73, 3-36.
| Crossref | Google Scholar |
Zeileis A, Leisch F, Hornik K, Kleiber C (2002) strucchange: an R package for testing for structural change in linear regression models. Journal of Statistical Software 7, 1-38.
| Crossref | Google Scholar |