Effect of fire severity and presence of bamboo (Chusquea culeou) on soil chemical properties in Andean Patagonian forests of Argentina
M. Florencia Urretavizcaya A * , M. Melisa Rago A , Marina Caselli A , Fernanda Ríos Campano A , Stefano Gianolini A and Virginia Alonso BA
B
Abstract
Fire is recurrent in Patagonian forests of Argentina, and is frequently associated with the die-off of bamboo (Chusquea culeou). Fire induces changes in soil properties, which may affect vegetation recovery.
This study assesses how fire severity affects soil chemical properties in different Patagonian forests, in the absence and presence of C. culeou.
We collected samples from the upper 5 cm of mineral soil from 44 plots distributed in five forest types affected by low-severity and high-severity fire, and unburned areas. Half of the plots also had C. culeou.
Fire severity affected soil pH, organic matter, electrical conductivity, nitrogen, C:N ratio, and cation exchange capacity. Changes in organic matter and C:N ratio varied among forest type, and variations in pH and sodium were detected among forest types. The presence of C. culeou affected soil pH, C:N ratio, and calcium.
The changes recorded were expected and could have a lasting effect on long-term productivity. Nothofagus pumilio and Nothofagus antarctica, and forests with C. culeou, varied in their soil chemical properties, sometimes regardless of fire.
Considering the heterogeneity of vegetation and soil in postfire environments is vital for predicting possible successional trajectories and defining restoration objectives.
Keywords: Austrocedrus, Chusquea, Nothofagus, Patagonia, post-fire changes, temperate forests, topsoil properties, volcanic soils, wildfire.
Introduction
Fire is a recurrent disturbance in the Andean Patagonian forests of Argentina that has shaped the landscape (Kitzberger and Veblen 1999). In recent decades, wildfires have increased in extension with extreme behaviour (Subsecretaría de Bosques [SSB] et al. 2015; Kitzberger et al. 2022; Rago et al. 2023). Similarly, high-severity wildfires are occurring in large areas of forests worldwide (Silveira et al. 2020; Collins et al. 2021; Higuera and Abatzoglou 2021). This makes the medium- and long-term ecosystem responses after fires difficult to understand, especially in the context of global climate change. For example, some consequences on ecosystems could be the delay or even the inability of forests to recover after large forest fires, since the arrival of seeds of the main forest species could be limited (Stevens-Rumann and Morgan 2019; Urretavizcaya et al. 2022, Mantero et al. 2023). Also, soil properties are critical for the recovery process as these affect ecosystem function after fire disturbance (Coleman et al. 2018; De Deyn and Kooistra 2021). Therefore, studying the impact of these fires on the soil is important in understanding what the natural recovery of these ecosystems would be like after a fire, and what can be done to facilitate ecosystem restoration.
Fire affects both the aboveground and belowground components of the ecosystem. Fire severity describes the magnitude of the disturbance; hence, this reflects the degree of change in ecosystem components (Agee 1993), and integrates both the heat pulse above the ground and the heat pulse transferred downwards into the soil (Neary et al. 2005). The term fire severity provides a description of how fire intensity affects ecosystems (Keeley 2009), which is largely dependent on the nature of the fuel available for burning, and the combustion characteristics that occur when these fuels are burned. Most studies have estimated the severity of fires by focusing on the loss of aboveground and belowground organic matter (Keeley 2009). Included in the broader definition of fire severity is soil burn severity, which is used to differentiate the effects of fire on soil properties from those on vegetation and the total ecosystem health (Parson et al. 2010). Although fire severity is not directly extrapolatable to soil burn severity, the heat transfer from the combustion of biomass and necromass on and in the soil causes some of the most common direct changes in soil (Santín and Doerr 2016).
During a fire event, heating and combustion may drive irreversible transformations of the nutrients stored in fuel. Losses of organic matter occur at temperatures near 100°C, volatile constituents are lost at temperatures up to 200°C, and detrimental distillation destroys 85% of the soil organic matter at temperatures between 200 and 300°C (González-Pérez et al. 2004). At these higher temperatures, chemical properties are affected by the combustion of soil organic matter and the production of pyrogenic organic compounds, as well as an increase in pH (Santín and Doerr 2016). The response of each soil nutrient is inherent to its temperature threshold, which is the temperature where nutrients are volatilised (DeBano 1991; Agee 1993; Neary et al. 2005). Nutrients can be ranked according to their sensitivity to loss from fire. Nitrogen is the most sensitive nutrient with losses at 175–200°C, followed by sulfur at >375°C, potassium at >550°C, phosphorus at 770°C, magnesium at 1107°C, calcium at 1484°C, and manganese at 1962°C (DeBano 1991; Agee 1993; Neary et al. 2005). Reduced organic matter and nitrogen, and increased phosphorus, exchangeable cations (Ca2+, Mg2+, K+, Na+), as well as increased pH and electrical conductivity (EC) are common after a fire event with the magnitude of change related to fire severity (Certini 2005; Neary et al. 2005; Agbeshie et al. 2022).
On the eastern side of the Andes in Patagonia, Argentina, the sharp decrease in precipitation from west to east parallels a dramatic vegetation gradient (Veblen et al. 2008). Between latitudes 41°S and 43°S, extensive stands of Nothofagus dombeyi occur in the area of greatest precipitation. With increasing aridity, the conifer Austrocedrus chilensis appears in pure and mixed stands with N. dombeyi. The sub-alpine Nothofagus pumilio forest occurs in cooler and mesic habitats, while Nothofagus antarctica appears at the bottom of broad valleys (Veblen et al. 2003). In these forests, bamboo (Chusquea culeou) is dominant in the understorey on humid, western sites and becomes less dense as it is replaced by xeric shrubs and small trees on eastern sites. Bamboo can reach 6 m in height and is highly flammable, providing fine fuel vertically that can spread fire from the ground to tree canopies (Blackhall and Raffaele 2019). Shrublands dominated by C. culeou experience more fires than adjacent mesic forests (Mermoz et al. 2005). Chusquea culeou shows great capacity to resprout from its rhizomes following medium to high-severity fire events (Fuentes-Ramirez et al. 2020). Further, it is a semelparous species that synchronously flowers approximately every 60 years at regional scale (Guerreiro 2014). It then dies off, which generates a large amount of biomass as fuel for fires (Veblen et al. 2003; Kitzberger et al. 2016).
Multiple factors contribute to changing fire regimes worldwide including climate change, changes in land use and vegetation structure, and direct human influence on ignition sources (Rogers et al. 2020). In northern Patagonia, the natural fire regime varies depending on the precipitation regime. In general, fire frequency increases as one moves from humid sites in the west to xeric sites in the east (Kitzberger 1994). In addition, human activities and climatic variations are fundamental influences on fire regimes (Veblen et al. 2003). Since 1850, the frequency of fires has increased in stands of N. dombeyi and A. chilensis, coinciding with the increase in the number of Indigenous peoples inhabiting the region and the beginning of European settlement. This frequency of fires peaked in the late 19th century and began to decline thereafter as the Indigenous population declined and the creation of several National Parks in the region prompted a fire suppression policy (Veblen et al. 1999). Current trends show increases in the occurrence of large and severe fires related to strong droughts and warmer summers (Kitzberger et al. 2016).
Most soils in Patagonia are derived from volcanic ash (Pereyra and Bouza 2019), which has high capacity to stabilise soil organic matter, retain phosphorus and water, and buffer pH (La Manna et al. 2020). Globally, our knowledge of the impact of fire on volcanic soils is limited (Neris et al. 2016). In general, fire effects on soil properties recorded in N. pumilio and A. chilensis forests have shown decreased organic matter and nitrogen, and increased pH and EC (Alauzis et al. 2004; Kitzberger et al. 2005; Urretavizcaya 2010; Urretavizcaya et al. 2018). These changes have persisted for at least 4 years in N. pumilio forests (Alauzis et al. 2004), but have not been recorded in the long term in N. pumilio or A. chilensis forests (Urretavizcaya 2010; Urretavizcaya et al. 2018). However, increases, decreases, or no change in extractable phosphorus and exchangeable cations have all been reported (Alauzis et al. 2004; Kitzberger et al. 2005; Urretavizcaya 2010; Urretavizcaya et al. 2018). Further, few studies have evaluated the effect of fire severity on changes in soil properties (Urretavizcaya 2010), and studies on the variation in soil properties associated with the presence of C. culeou are even fewer (Veblen 1982). When C. culeou blooms and then dies over large areas of hundreds of square kilometres, fuel continuity and the risk of fire increases (Veblen et al. 2003). More fires are expected as a result of climate change; therefore, it is important to understand how fire affects soil properties and how natural recovery occurs in order to take appropriate action. This study aimed to assess the effects of fire severity on soil chemical properties in several temperate Patagonian forest types after an extreme fire event in the absence and presence of C. culeou. Results will help us to understand: (1) the type of changes that may impact soil functions; (2) how pre-fire vegetation can influence post-fire conditions and recovery; and (3) what indicators can be used to predict the resilience of temperate forests. This will aid in identifying appropriate management and restoration strategies for ecosystems after fires.
Materials and methods
Study area
The study was carried out in the area affected by the forest fire called ‘Las Horquetas’. The study site is located in the north-west of the Chubut Province, Patagonia, Argentina near the town of Cholila (Fig. 1). The fire was first detected on 16 February 2015, and burned for >1 month over 28,960 ha (Subsecretaría de Bosques [SSB] et al. 2015). The burned area corresponded in extension and altitude to a large part of the Lago Cholila basin, which has a west–east orientation and is located in a mountainous post-glacial landscape of Andean Patagonia (Fig. 1). Forests are distributed throughout the area, from valleys at 300–500 m to mountain areas at 1600 m. The area has a typical temperate Mediterranean climate with cold wet winters, and dry warm summers (Köppen and Geiger 1936). Given the orientation of the valley, there is a marked precipitation gradient from 2400 mm in the west to 800 mm in the east (Bianchi and Cravero 2010), 75% of which falls during the autumn and winter months. The soils are derived from volcanic ashes, with Andisols in areas with the highest humidity and transitional to Mollisols in the driest areas (Pereyra and Bouza 2019; La Manna et al. 2020). Soils formed from volcanic deposits have unique properties that include variable charge, high water retention, high phosphate retention, low bulk density, high friability, highly stable soil aggregates, and excellent tilth (Dahlgren et al. 2004; Pereyra and Bouza 2019).
Burned area (delimited by white lines), with the fire severity classification based on normalised burn ratio (NBR) thresholds from SSB et al. (2015) and circles showing the surveyed plots for each forest type. Study area (point blue) located in Patagonia, Argentina (grey area).
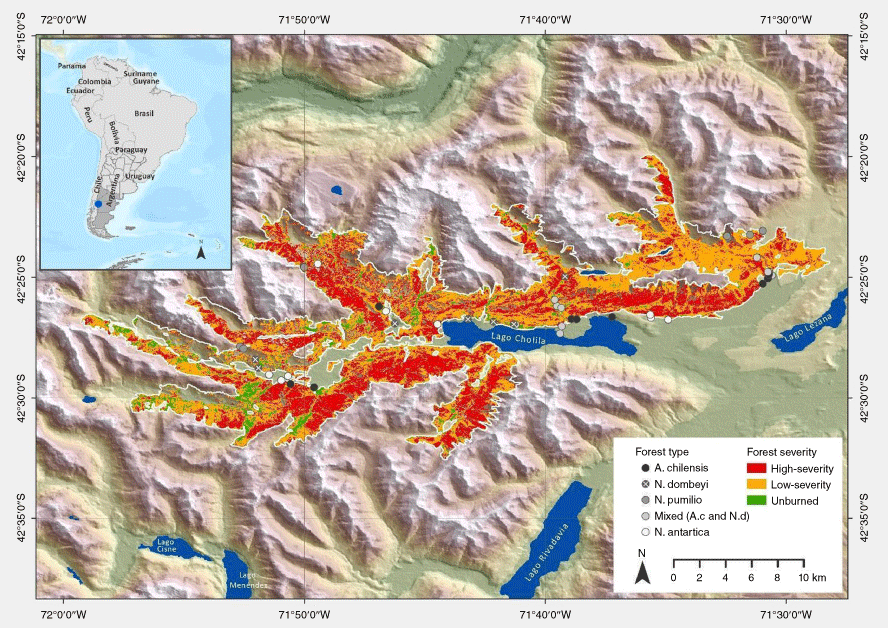
Sampling procedure
We studied the main forest types in this region that were affected by the fire: (1) Nothofagus antarctica (G. Forst.) Oerst.; (2) Nothofagus dombeyi (Mirb.) Oerst.; (3) Nothofagus pumilio (Poepp. & Endl.) Krasser; (4) Austrocedrus chilensis (D.Don) Pic-Serm. & Bizzarri; and (5) mixed A. chilensis–N. dombeyi. In each forest type, we identified burned areas, with high and low fire severity, as well as unburned (control) areas. In both burned and unburned areas, we also identified areas with and without bamboo (Chusquea culeou E. Desv.). Since the burned area was approximately 29,000 ha, the preliminary selection of sampling sites was based on the fire severity classification map elaborated for the Las Horquetas fire (Subsecretaría de Bosques [SSB] et al. 2015). The map was based on a severity index with original values obtained in the office and later calibrated with field surveys in the area of the fire. To estimate the index, the authors used Landsat 8 OLI (USGS) satellite data with a capture date of 4 November 2015, which were previously atmospherically corrected using the ATCOR3 algorithm, and the post-fire Normalised Burn Ratio (NBR). The NBR is a normalised ratio between near infra-red (NIR) band (~785–899 nm, Landsat-8 band # 5) and short wave infra-red 2 (SWIR2) band (~2100–2280 nm, Landsat-8 band # 7). The vegetation of the area, which corresponds to the Andean Patagonian forest, is represented by various dominant native tree species including A. chilensis, N. dombeyi, N. antarctica, N. pumilio, Maytenus boaria Molina, and Fitzroya cupressoides (Molina) I. M. Johnst., as well as conspicuous species of the understorey such as Lomatia hirsuta Diels ex J.F.Macbr., Schinus patagonica (Phil.) Johnst., Diostea juncea (Gill. ET Hook) Miers., Discaria chacaye (G. Don) Tortosa, and Ochetophila trinervis (Gillies ex Hook.) Poepp. ex Endl. In addition to these species, C. culeou occurs in the understorey of most forest types in areas with high mean annual precipitation (Figs. 2, 3). The 2015 fire occurred after the 2013 flowering and seeding of C. culeou and synchronous die-off that covered over hundreds of square kilometres.
Representative images of each fire severity (high-severity, low-severity, unburned) at each forest type (Ac, Austrocedrus chilensis; Nd, Nothofagus dombeyi; Ac–Nd, mixed A. chilensis–N. dombeyi; Np, Nothofagus pumilio; Na, Nothofagus antarctica).
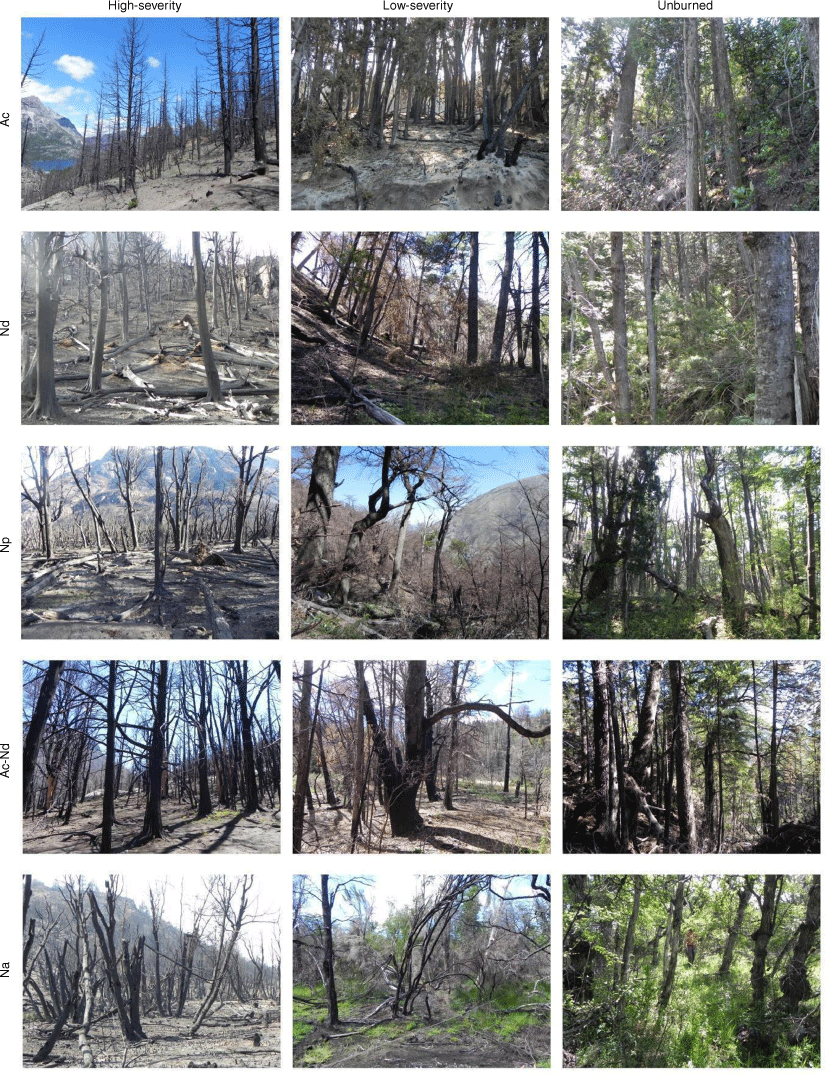
We selected three sampling sites by forest type (n = 5) and fire severity (n = 3), except for N. dombeyi where we only selected two unburned sampling sites; in total, there were 44 sampling sites (Fig. 1; see Supplementary Fig. S1). We ensured that different forests and severities were represented as best as possible in the west–east precipitation gradient, with similar aspect, slope, and pre-fire vegetation coverage. During the first growing season after the fire (November 2015–February 2016), we established a permanent plot of 15 m radius (~700 m2) in each sampling site. This study represents the baseline of a long-term study that is being carried out by periodically measuring the soil and vegetation in the same plots. In each plot, we recorded the fire damage in different strata: litter and duff; herbaceous; shrubs and tree regeneration (0–1 m height); shrubs and low trees (1–7 m); and dominant trees (more than 7 m). We used a scale of 0–5 to record classes of fire severity, adapted from Mutch and Swetnam (1995). These classes were: Class 0, unburned (no evidence of fire); Class 1, light surface fire (no evidence of dead trees by fire); Class 2, mild to moderate severity (some dead seedlings and/or young trees; charred on the bark of living trees); Class 3, moderate severity (most small trees and <50% of intermediate trees dead by fire; charred on the bark of living trees); Class 4, high severity (>50% of intermediate trees dead or damaged; high charring and some crown damage in dominant stratum trees, but <50% dead); and Class 5, very high severity (most intermediate trees dead and >50% canopy trees dead). We considered Class 0 as unburned, Classes 2 and 3 as low-severity, and Classes 4 and 5 as high-severity (Mutch and Swetnam (1995). We recorded the presence of C. culeou in 22 plots, with at least one for each forest type and each fire severity. Most of the plots with C. culeou were located on the western side of the study area (Fig. S1), coinciding with the distribution of this species that decreases in abundance from a humid, temperate climate toward a dry, cold climate (Pearson et al. 1994).
During January and February 2016, we took three composite samples (~630 cm3 each) of the upper 5 cm of mineral soil from each plot; totalling 132 soil samples. We transferred the samples to the CIEFAP Soil Laboratory where they were air dried, sieved on a 2-mm sieve, and analysed the following seven soil properties: (1) current pH, measured with a pH meter at a soil to distilled water ratio of 1:2.5 (Bailey 1943); (2) electrical conductivity (EC), measured with an EC meter at a soil to distilled water ratio of 1:5 (Allison 1980); (3) organic matter, estimated by loss on ignition in a muffle at 430°C for 24 h (Davies 1974); soil organic carbon, estimated from the division of organic matter by the van Bemmelen factor of 1.724 (Tabatabai 1996); (4) total nitrogen, obtained by Kjeldahl method, which consists of wet digestion of the soil sample where the organic nitrogen is converted into ammonium, thereby obtaining the value of the organic nitrogen plus ammonium content (Bremner 1960); (5) available phosphorus, estimated by the Olsen method (Olsen et al. 1954), which consists of the extraction of the labile fraction of phosphorus in the soil with a sodium bicarbonate solution, recommended for slightly acidic soils such as Andisols (Elrashidi 2010); (6) cation exchange capacity (CEC), obtained by a procedure where extractions are carried out with sodium acetate to saturate the cationic exchange complexes with sodium, the excess solution is washed with ethanol, and then ammonium acetate is added to extract the sodium and its concentration is determined photometrically using a flame photometer (Instituto Argentino de Normalización y Certificación [IRAM] and Secretaria de Agricultura Ganadería y Pesca [SAGyP] 2024); and (7) exchangeable bases, carried out with ammonium acetate complexometry to determine calcium and magnesium, and flame photometry to determine sodium and potassium (Schollenberger and Simon 1945).
Data analyses
We assessed the effect of forest type and fire severity on soil properties through linear mixed models (function lme, package nlme (Pinheiro et al. 2023)). We used a factorial design (5 × 3), with the factor forest type (five levels: Ac, A. chilensis; Nd, N. dombeyi; Ac–Nd, mixed A. chilensis–N. dombeyi; Np, N. pumilio; Na, N. antarctica) and the factor fire severity (three levels: unburned; low-severity; high-severity) as fixed effects, and plot (with three subplots each) as a random effect. Response variables were pH, EC, soil organic carbon, total nitrogen, C:N ratio, available phosphorus, calcium, magnesium, potassium, sodium, and CEC. We corroborated the assumptions of normality by Shapiro–Wilk test and homoscedasticity through residual analysis, applied transformations, and modelled heterogeneity when necessary. When significant differences were detected (P < 0.05), we applied LSD-Fisher contrast (function glht, package multcomp (Hothorn et al. 2016)). We carried out the analyses with the R platform (R Core Team 2021).
We assessed the effect of the presence of C. culeou and fire severity on soil properties through linear mixed models. We used a factorial design (2 × 3), with the factor C. culeou (two levels: P, present; A, absent) and the factor fire severity (three levels: unburned; low-severity; high-severity) as fixed effects, and subplots (three per plot) as random effects. Response variables were the soil properties previously mentioned. We performed the analyses in the same way as detailed in the previous section.
Results
Effect of forest type and fire severity on soil properties
Soil pH was significantly different among fire severity (P = 0.006) and forest type (P = 0.008). Sites with high-severity showed higher pH than unburned sites, with a difference in magnitude of 0.6, whereas sites with low-severity had similar pH to high-severity and unburned sites (Fig. 4; Table S1). Average pH of high-severity, low-severity, and unburned sites were 6.9, 6.7, and 6.3, respectively. N. pumilio forests showed a pH significantly lower (6.3) than A. chilensis, N. dombeyi, and the mixed forest (6.9, 6.6, and 7.0), and a value similar to N. antarctica forests (6.4). The latter also had a pH similar to N. dombeyi forests (Fig. 4). EC was also affected by fire severity (P = 0.04). Sites with high-severity showed higher EC than unburned sites, with a difference in magnitude of 0.07 dS m−1, whereas sites with low-severity had similar EC to high-severity and unburned sites (Fig. 4; Table S1).
Mean and s.e. of the soil variables measured by forest type (Ac, Austrocedrus chilensis; Nd, Nothofagus dombeyi; Ac–Nd, mixed A. chilensis–N. dombeyi; Np, Nothofagus pumilio; Na, Nothofagus antarctica) and fire severity (unburned; low-severity; high-severity). Soil was sampled from the upper 5 cm of mineral soil and comprised of three composite samples per plot (630 cm3 each). Means with different letters are significantly different (LSD Fisher P < 0.05). Italics, differences for the interaction between forest type and fire severity; lowercase letters, differences for forest type the main effect forest type; capital letters, differences for the main effect fire severity.
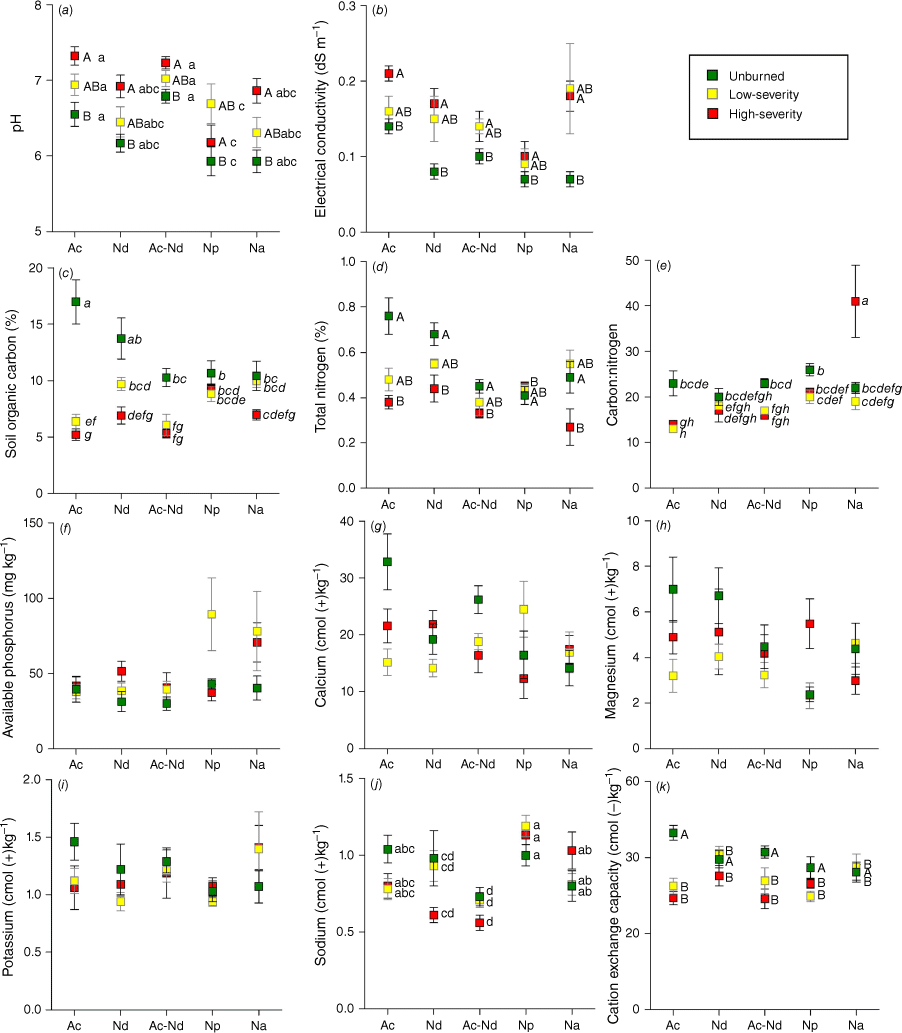
Percentage of soil organic carbon was affected differently by fire severity in the different forest types (interaction forest × severity P = 0.03). In A. chilensis, N. dombeyi, and the mixed forest, sites with high-severity showed significantly lower percentage of soil organic carbon (5.2, 6.9, and 5.3%) than unburned sites (17.0, 13.7, and 10.3%) (Fig. 4; Table S1). In N. pumilio and N. antarctica forests, fire severity did not show a significant effect on the percentage of soil organic carbon (Fig. 4; Table S1). For all forest types, percentage of total nitrogen was significantly lower (P = 0.009) in high-severity sites than unburned sites. The difference in magnitude was on average 0.2%, but it varied from 0.38% in A. chilensis to 0.03% in N. pumilio forests. Sites with low-severity showed intermediate values among high-severity and unburned sites, without significant differences (Fig. 4; Table S1).
C:N ratio was affected differently by fire severity in the different forest types (interaction forest × severity P = 0.002). In A. chilensis and the mixed forest, the ratio was lower in burned (~13.6 and 16.4, considering low-severity and high-severity together) than unburned sites (23.3 and 23.0). In N. dombeyi forests, C:N ratio in burned sites was similar to unburned sites (17.9 on average). N. antarctica forests showed the greatest C:N ratio in sites with high-severity (41.0), compared to those of low-severity and unburned sites (19.3 and 21.7). In N. pumilio forests, C:N ratio in sites with high-severity was similar to sites with low-severity (21.1 and 20.2), but the high-severity did not differ from the unburned (25.8).
For phosphorus concentration, no significant differences were detected associated with fire severity (P = 0.34) or forest type (P = 0.41), although a high variation was observed in the values in N. antarctica and N. pumilio sites (Fig. 4; Table S1). Sodium concentration was significantly different among forest types (P < 0.001), being higher in N. pumilio, followed by N. antarctica and A. chilensis. The latter also showed values similar to those of N. dombeyi and N. dombeyi with those of mixed forest (Fig. 4; Table S1).
For concentrations of potassium, magnesium, and calcium, no significant differences were detected among fire severity (P = 0.86, 0.21, and 0.66, respectively) or forest type (P = 0.65, 0.38, and 0.44, respectively). CEC was affected by fire severity (P = 0.01), showing a similar pattern in all forest types. It was lower in burned sites (32.5 and 34.9 cmol(+) kg−1) for high- and low-severity than unburned sites (40.3 cmol(+) kg−1) (Fig. 4; Table S1).
Effect of C. culeou and fire severity on soil properties
No interaction was found between C. culeou and fire severity for any soil property analysed. Soil pH was lower in sites with C. culeou (P = 0.002) with a difference in magnitude of 0.5 (Fig. 5; Table S2). Although neither the percentage of soil organic carbon nor nitrogen were affected by the presence of C. culeou, C:N ratio was higher in sites with C. culeou (P = 0.03), with a difference in magnitude of 8.7 (Fig. 5; Table S2).
Mean and s.e. of the soil variables measured by the absence (A) or presence (P) of Chusquea culeou and by fire severity (unburned; low-severity; high-severity). Soil was sampled from the upper 5 cm of mineral soil and comprised of three composites per plot (630 cm3 each). Means with different letters are significantly different (LSD Fisher P < 0.05). Italics, differences for the interaction between forest type and fire severity; lowercase letters, differences for the main effect C. culeou; capital letters, differences for the main effect fire severity (interaction between C. culeou and fire severity was not detected).
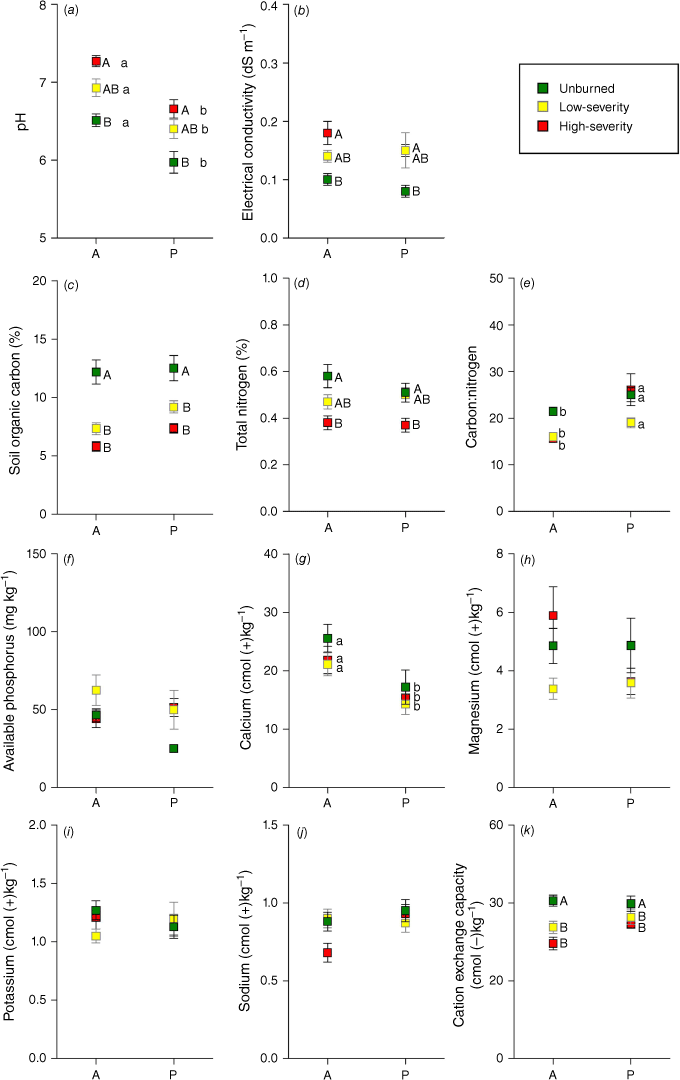
Phosphorus concentration did not show significant effects due to the presence of C. culeou (P = 0.10), although a high variation was observed in unburned sites with and without C. culeou. While calcium concentration decreased significantly (~30%) in forests with C. culeou (P = 0.007), the concentrations of magnesium, potassium, and sodium, as well as CEC did not show significant differences in the presence of C. culeou (Fig. 5; Table S2).
Discussion
In this study, we evaluated the chemical changes that occurred in the soil of temperate forests in Patagonia in relation to fire severity and the presence of C. culeou. Burn severity is a continuous measure of multiple fire effects, including but not limited to overstorey vegetation mortality, soil heating, and fuel consumption (Lentile et al. 2007; Keeley 2009; Luce et al. 2012). We characterised fire severity by reference to the loss and damage of aboveground vegetation, recognising that this severity is not directly extrapolatable as soil burn severity (Parson et al. 2010). In unburned soils of Andean Patagonian forests, we observed values representative of soils derived from volcanic ash such as slightly acidic pH, low EC, high organic matter content, variable levels of nutrients (N, Mg, Ca), low values of available phosphorus due to high anion retention, and high CEC (Pereyra and Bouza 2019; La Manna et al. 2020). Between 9 months and 12 months after a fire, these properties showed variable responses among fire severity, forest type, and the presence of C. culeou. Soil pH differed among the main effects of fire severity, forest type, and the presence of C. culeou. EC differed only among fire severity. Soil organic carbon differences were detected for the interaction between fire severity and forest type. Nitrogen differed only among fire severity. C:N ratio differences responded to the interaction between fire severity and forest type, and also differed in the presence of C. culeou. Sodium differed only among forest type. Calcium differed only with presence of C. culeou. CEC differed only among fire severity. Some of these differences might also be explained by the great edaphic variability associated with the position in the landscape, and also by the different granulometry and composition of the pyroclastic material that varied with the location and age of distinct volcanic eruptions (Pereyra and Bouza 2019; La Manna et al. 2020). In general, the changes observed in our study are those expected after the occurrence of wildfires (Binkley and Fisher 2019; Agbeshie et al. 2022).
Fire can alter the mechanisms that are involved in nutrient availability and the losses and additions of nutrients to the soil. Combustion of organic matter during a fire tends to increase pH because basic cations are released during combustion and deposited on the soil surface, simultaneously increasing EC and diminishing CEC (Dzwonko et al. 2015). However, the pH increase is usually temporary and depends on the original pH of the soil, amount of ash released, chemical composition of the ash, and wetness of the climate. In our study, the forests burned with high-severity showed an increase in soil pH and EC, compared to the unburned forests, whereas sites with C. culeou showed lower values of soil pH, as was also detected in sites with other bamboo species after flowering and death (Takahashi et al. 2007). Changes in soil pH affect the availability of some nutrients due to the combined effects of pH on soil sorption and plant uptake (Barrow and Hartemink 2023). Increased EC after fire is also associated with an increase in base cations, phosphates and carbonates, which can decrease over time due to sorption and precipitation and/or leaching processes, as well as uptake by newly reestablished vegetation (Iglesias et al. 1997; Urretavizcaya 2010). Nothofagus pumilio and N. antarctica forests showed the lowest pH values, regardless of the effects of fire. This is consistent with the results of a comparative study among conifers, broad-leaf evergreen, and deciduous species of Patagonia (Satti et al. 2003).
Fire affects soil organic matter by directly affecting its chemical composition, with a complete loss threshold at 400–500°C (Badía et al. 2014). Therefore, changes in soil organic matter are expected even under a low-severity fire. In our study, the magnitude of changes in soil organic carbon, which may represent around 58% of the organic matter (Tabatabai 1996), were related to the interaction between fire severity and forest type. On average, the loss of soil organic carbon in high-severity compared to unburned forests was around 45% on average, but A. chilensis, N. dombeyi, and the mixed forest showed the greatest losses with 69, 50, and 48%, respectively, while N. pumilio and N. antarctica did not show significant losses. However, total loss of soil organic carbon is likely to be underestimated because we did not assess the effect of fire on the soil organic horizon, but observed that it was completely consumed in high-severity sites and partially in low-severity sites. Burning acts as an instantaneous physical decomposition process that volatilises nutrients from both vegetation and surface organic soil horizons. Furthermore, fire alters the remaining organic materials by heating (Santín and Doerr 2016) and these changes could influence the availability of nutrients (Neris et al. 2016).
Nitrogen is the only soil nutrient that is not supplied to the soil by chemical weathering of parent rock material, and it is the most important nutrient affected by fire (Agbeshie et al. 2022). Despite plants absorbing nitrogen mainly in its ionic forms (ammonium, nitrate, nitrite), organic nitrogen, which represents most of the nitrogen present in soils, acts as a reserve that will be available to plants as the biological activity of the soil and environmental conditions favour its mineralisation. In our study, most of the loss of total nitrogen occurred at high-severity sites, with 26–50% loss compared to unburned sites. Although total nitrogen decreases as organic matter is combusted, available nitrogen usually increases, particularly NH4-N due to the mineralisation of organic N, which is associated with increased soil temperatures, water content, and pH (Caon et al. 2014). Consequently in the short term after a fire, rapid plant growth may occur even in areas of high-severity (Urretavizcaya et al. 2006).
C:N ratio of the soil closely corresponds with C:N ratio in plant debris and the rate of decomposition. In Andean temperate forest soils, C:N ratio is usually high, especially because the deposition of plant debris is high and the decomposition is slow. The native forest species of Patagonia present important differences in the quality of the litter and the rates of potential nitrogen mineralisation (Mazzarino et al. 1998; Satti et al. 2003; La Manna et al. 2020), which can also condition soil chemical fertility (Satti et al. 2007; Diehl et al. 2008). Values of C:N ratio found in our study in unburned sites are in the range of those determined in Horizon A of soils located in mountain flanks originated from volcanic ash (C:N ratio = 20) and pumice (C:N ratio = 27), as well as those originated from volcanic ash and located in valleys plains (C:N ratio = 18), and hills (C:N ratio = 19.4), with a udic humidity regime in the first three and udic-dry in the last (La Manna et al. 2020). This variety of situations of the original material and location in the landscape, as well as the dominant vegetation might explain the differences found in soil C:N ratio among the different unburned forest types. In contrast, changes in C:N ratio are closely associated with the post-fire changes detected in organic matter in the burned areas. Nothofagus antarctica was the only vegetation type that showed higher values of C:N ratio in burned compared to unburned soils. This might indicate that in N. antarctica forests, less nitrogen is available for the growth of post-fire vegetation, probably associated with higher nitrogen loss. Lower microbial activity could also be a factor. Further, we found higher C:N ratio in sites with C. culeou, which could be explained by the input of dead culms that occurs after flowering (Austin and Marchesini 2012). Although a high C:N ratio could indicate a decrease in microbial activity, this might change with time as vegetation recovers. For example, Rivas et al. (2016) found that as C. culeou regenerates after low-severity and mid-severity fires, there is an increase in soil proteins produced by arbuscular mycorrhizal fungi associated with their roots, suggesting the recovery of soil microorganisms.
Fire severity may affect the extractable phosphorus because, during high-severity fires, 50–60% of the total phosphorus could be lost by volatilisation (DeBano 1991; Hosseini et al. 2017). However, we did not find significant differences among fire severity or forest types in our study. This could mean that the threshold temperature for phosphorus combustion, established at 774°C (DeBano 1991), was not reached in the studied sites. When compared with unburned sites, N. antarctica and N. pumilio forests almost doubled the available phosphorus concentration in low-severity sites, and N. antarctica also increased phosphorus in high-severity sites. This result is unexpected since the absence of significant losses of organic matter would indicate that the temperature reached by the soil during the fire was low, thus phosphorus volatilisation would also have been low. However, this might be due to the contribution from the burned plant material. For deciduous Nothofagus species in temperate forests of Patagonia, a higher concentration of organic phosphorus in the soil and in their green leaves has been recorded compared to native conifers of these forests (Satti et al. 2007). Likewise, the application of leaf ashes of Nothofagus species increased available phosphorus in the soil (Escudey et al. 2010) and there are records of a high concentration of phosphorus in the fine detritus of some of these species (Richter and Frangi 1992). While we found no significant differences between sites with and without C. culeou, in unburned forests with C. culeou we observed almost half the concentration of available phosphorus than in unburned forests without C. culeou. Until now, research has focused mainly on the effects of C. culeou on the plant community and few studies have evaluated its effects on the soil. One of these studies found that in a N. dombeyi forest soil phosphorus was significantly lower in the presence of C. culeou (Veblen 1982). Thus, more studies focusing on the effects of C. culeou on post-fire recovery are needed. Additional understanding of the phosphorus cycle would also be useful because although volcanic soils have a high concentration of phosphorus, its availability is low. The tendency of phosphorus to increase after fire in deciduous forests but decrease in the presence of C. culeou suggests that its availability could vary depending on the vegetation type.
We only found differences in sodium among forest types and in calcium between sites with and without C. culeou. Nothofagus pumilio forests showed the highest sodium concentration while the lowest were in N. dombeyi, A. chilensis, and the mixed forest. The temperature threshold of cations is high and as a result, they are not easily volatilised and lost from burned areas (DeBano 1991). This explains the lack of fire effects detected in our study. Ash deposited on the soil surface during a fire may also contain high concentrations of cations and therefore, their availability can increase (Alcañiz et al. 2018). However, nutrients remaining in the ash deposited on the soil surface are vulnerable to post-fire leaching into the soil, or they can also be lost during wind erosion (Kauffman et al. 1993). Although these readily available monovalent and bivalent cations probably do not affect plant growth directly, their amount and composition determine base saturation, which plays an important role in controlling the pH regimes of soils (DeBano et al. 1998). Sites with C. culeou showed lower values of calcium, similar to the results found by Veblen (1982) in an old-growth and unburned N. dombeyi forest. In addition to the nutrients released from organic matter during combustion, a loss of the CEC associated with the organic matter of the mineral soil can also occur. Exchange sites are important for retaining soil cations after fire (Viro 1974). In our study, CEC decreased by around 20% after fire, regardless of forest type. The loss of CEC has been reported by several studies that analysed non-volcanic soils. In general, CEC decreases as soil is exposed to higher temperatures (Soto and Diaz-Fierros 1993; Solomun et al. 2021). Soil heating during a fire can affect CEC by destroying organic compounds and clay materials. This loss of CEC in non-volcanic soils is mainly related to the destruction of organic compounds due to the location of the humus layer at or near the soil surface and the low temperatures needed to destroy organic and humic material. Two studies that evaluated fire effects on andic properties in Patagonia found no significant changes in soil mineralogy (Alauzis et al. 2004; Kitzberger et al. 2005). Thus, similar to non-volcanic soils, the destruction of organic compounds may also explain the reduction of CEC detected in our study.
This study investigated the changes that occur after fire in volcanic soils, which have not been widely studied (Mora et al. 2016; Neris et al. 2016). Our results demonstrated the effects of fire severity and vegetation type on soil properties following a wildfire in Patagonia, similar to other studies done with volcanic soils (Santorufo et al. 2021; Peduto et al. 2022), and soils with phyllosilicate clay mineralogy. Fire effects on soil organic carbon and nutrients vary with pre-fire soil composition, plant community, fire severity, fire history, and other factors (Sanchez et al. 1994; Pardini et al. 2004; Johnson et al. 2007; Mora et al. 2016). Prolific flowering and subsequent die-off of C. culeou produces plant biomass that acts as fine fuel for fires, and its ability to resprout vigorously right from the seedling stage might ensure the persistence of this species in burned forests (Cavallero and Blackhall 2020). More understanding is needed on the chemical composition of the leaves and culms of C. culeou, as well as how they incorporate into soil after decomposition. Local as well as mid-term and long-term studies are required to fill these information gaps and refine our understanding of the relationship among fire, organic carbon, and nutrient dynamics as well as to guide post-fire forest management in the current context of climate change.
Conclusion
The changes observed in our study were expected after the occurrence of wildfires. It is worth noting that fire had no effect on organic C in N. pumilio and N. antarctica forests, but reduced it by nearly half in other forest types. In contrast, soil N was as much as 50% lower in burned soils of all forest types compared with unburned sites.
It was interesting that N. pumilio and N. antarctica forests, both deciduous species, showed different chemical soil properties from other forest types, at times unrelated to the effect of fire (e.g. these soils turned out to be the most acidic). We found that the presence of C. culeou increased soil pH and calcium, and decreased C:N ratio although the presence of C. culeou did not seem to affect the nutritional status of the soil after fire occurrence. These findings coincide in part with the few studies that have evaluated the soil underneath C. culeou in the region, suggesting a relevant role of this species in the functioning of the ecosystem. Thus, more studies focusing on C. culeou and its effects on post-fire recovery would be relevant. This study highlights the importance of considering vegetation and soil heterogeneity in post-fire environments to predict possible successional trajectories and support decision making for ecosystem restoration and management following fire events.
Data availability
The data that support this study are available and will be shared upon reasonable request to the corresponding author.
Conflict of interest
The authors have no competing interest to declare that are relevant to the content of this article.
Declaration of funding
This research was supported by Consejo Nacional de Investigaciones Científicas y Técnicas de Argentina, Project PUE 22920160100128 and Centro de Investigación y Extensión Forestal Andino Patagónico, Project PE7A11703.
Acknowledgements
We are grateful to the technical staff of Secretaría de Bosques de la provincia de Chubut and the landowners for their assistance and permission to carry out the sampling. We thank the anonymous reviewers for comments and suggestions which improved the manuscript.
References
Agbeshie AA, Abugre S, Atta-Darkwa T, Awuah R (2022) A review of the effects of forest fire on soil properties. Journal of Forestry Research 33(5), 1419-1441.
| Crossref | Google Scholar |
Agee J (1993) ‘Fire Ecology in Pacific Northwest Forests.’ (Island Press: Washington, DC, USA) Available at https://digitalcommons.usu.edu/barkbeetles/142/
Alauzis MV, Mazzarino MJ, Raffaele E, Roselli L (2004) Wildfires in NW Patagonia: long-term effects on a Nothofagus forest soil. Forest Ecology and Management 192, 131-142.
| Crossref | Google Scholar |
Alcañiz M, Outeiro L, Francos M, Úbeda X (2018) Effects of prescribed fires on soil properties: a review. Science of The Total Environment 613–614, 944-957.
| Crossref | Google Scholar | PubMed |
Austin AT, Marchesini VA (2012) Gregarious flowering and death of understorey bamboo slow litter decomposition and nitrogen turnover in a southern temperate forest in Patagonia, Argentina. Functional Ecology 26, 265-273.
| Crossref | Google Scholar |
Badía D, Martí C, Aguirre AJ, Aznar JM, González-Pérez JA, De la Rosa JM, León J, Ibarra P, Echeverría T (2014) Wildfire effects on nutrients and organic carbon of a Rendzic Phaeozem in NE Spain: changes at cm-scale topsoil. CATENA 113, 267-275.
| Crossref | Google Scholar |
Barrow NJ, Hartemink Alfred E (2023) The effects of pH on nutrient availability depend on both soils and plants. Plant and Soil 487, 21-37.
| Crossref | Google Scholar |
Bianchi AR, Cravero SAC (2010) ‘Atlas climático digital de la República Argentina.’ (Ediciones INTA: Salta) Available at https://repositorio.inta.gob.ar/handle/20.500.12123/16040 [In Spanish]
Blackhall M, Raffaele E (2019) Flammability of Patagonian invaders and natives: when exotic plant species affect live fine fuel ignitability in wildland-urban interfaces. Landscape and Urban Planning 189, 1-10.
| Crossref | Google Scholar |
Bremner JM (1960) Determination of nitrogen in soil by the Kjeldahl method. The Journal of Agricultural Science 55, 11-33.
| Crossref | Google Scholar |
Caon L, Vallejo VR, Ritsema CJ, Geissen V (2014) Effects of wildfire on soil nutrients in Mediterranean ecosystems. Earth-Science Reviews 139, 47-58.
| Crossref | Google Scholar |
Cavallero L, Blackhall M (2020) Resprouting increases seedling persistence likelihood after fire in a semelparous bamboo species. Acta Oecologica 108, 103623.
| Crossref | Google Scholar |
Certini G (2005) Effects of fire on properties of forest soils: a review. Oecologia 143, 1-10.
| Crossref | Google Scholar | PubMed |
Coleman DC, Callaham MA, Crossley DA (2018) ‘Fundamentals of Soil Ecology.’ (Academic Press: Orlando, FL, USA) 10.1016/c2015-0-04083-7
Collins L, Bradstock RA, Clarke H, Clarke MF, Nolan RH, Penman TD (2021) The 2019/2020 mega- fires exposed Australian ecosystems to an unprecedented extent of high-severity fire. Environmental Research Letters 16, 044029.
| Crossref | Google Scholar |
Dahlgren RA, Saigusa M, Ugolini FC (2004) The nature, properties and management of volcanic soils. Advances in Agronomy 82, 113-182.
| Crossref | Google Scholar |
Davies BE (1974) Loss-on-Ignition as an estimate of soil organic matter. Soil Science Society of America Journal 38, 150-151.
| Crossref | Google Scholar |
DeBano LF (1991) The effects of fire on soil properties. In ‘Proceedings management and productivity of western-Montane. Forest Soils’. (Eds AE Harvey, LF Neuenschwander) pp. 151–155. (US Department of Agriculture, Forest Service, Intermountain Research Station) Available at https://books.google.com.ar/books?hl=es&lr=&id=PGTOhtl2380C&oi=fnd&pg=PA151&dq=DeBano+1991+soil&ots=8loIngNYbX&sig=Y24BHUwlkGJRqqrEmQhFRT6HuqA&redir_esc=y#v=onepage&q=DeBano%201991%20soil&f=false
DeBano LF, Neary DG, Ffolliott PF (1998) ‘Fire Effects on Ecosystems.’ (John Wiley & Sons) Available at https://books.google.com.ar/books?hl=es&lr=&id=cFxtriC2EDkC&oi=fnd&pg=PR16&dq=DeBano+et+al.+1998&ots=lAxq8wRRef&sig=NXmNAIsPWaW5mbQynmwZc8yY4o0&redir_esc=y#v=onepage&q=DeBano%20et%20al.%201998&f=false
De Deyn GB, Kooistra L (2021) The role of soils in habitat creation, maintenance and restoration. Philosophical Transactions of the Royal Society B 376, 20200170.
| Crossref | Google Scholar | PubMed |
Diehl P, Mazzarino MJ, Fontenla S (2008) Plant limiting nutrients in Andean-Patagonian woody species: effects of interannual rainfall variation, soil fertility and mycorrhizal infection. Forest Ecology and Management 255, 2973-2980.
| Crossref | Google Scholar |
Dzwonko Z, Loster S, Gawroński S (2015) Impact of fire severity on soil properties and the development of tree and shrub species in a Scots pine moist forest site in southern Poland. Forest Ecology and Management 342, 56-63.
| Crossref | Google Scholar |
Escudey M, De la Fuente P, Antilén M, Molina M (2010) Effect of ash from forest fires on phosphorus availability, transport, chemical forms, and content in volcanic soils. Environmental Chemistry 7, 103-110.
| Crossref | Google Scholar |
Fuentes-Ramirez A, Salas-Eljatib C, González ME, Urrutia-Estrada J, Arroyo-Vargas P, Santibañez P (2020) Initial response of understorey vegetation and tree regeneration to a mixed-severity fire in old-growth Araucaria–Nothofagus forests. Applied Vegetation Science 23, 210-222.
| Crossref | Google Scholar |
González-Pérez JA, González-Vila FJ, Almendros G, Knicker H (2004) The effect of fire on soil organic matter- a review. Environment International 30, 855-870.
| Crossref | Google Scholar | PubMed |
Guerreiro C (2014) Flowering cycles of woody bamboos native to southern South America. Journal of Plant Research 127, 307-313.
| Crossref | Google Scholar | PubMed |
Higuera PE, Abatzoglou JT (2021) Record- setting climate enabled the extraordinary 2020 fire season in the western United States. Global Change Biology 27(1), 1-2.
| Crossref | Google Scholar | PubMed |
Hosseini M, Rajabi Agereh S, Khaledian Y, Jafarzadeh Zoghalchali H, Brevik EC, Movahedi Naeini SAR (2017) Comparison of multiple statistical techniques to predict soil phosphorus. Applied Soil Ecology 114, 123-131.
| Crossref | Google Scholar |
Iglesias T, Cala V, Gonzalez J (1997) Mineralogical and chemical modifications in soils affected by a forest fire in the Mediterranean area. Science of The Total Environment 204(1), 89-96.
| Crossref | Google Scholar |
Instituto Argentino de Normalización y Certificación [IRAM] Secretaria de Agricultura Ganadería y Pesca [SAGyP] (2024) Norm 29577-2:2024 Calidad del suelo. Determinación de cationes básicos extraíbles y capacidad de intercambio catiónico. Parte 2 - Determinación de la capacidad de intercambio catiónico. Available at https://www.magyp.gob.ar/sitio/areas/samla/normas/ [In Spanish]
Johnson D, Murphy JD, Walker RF, Glass DW, Miller WW (2007) Wildfire effects on forest carbon and nutrient budgets. Ecological Engineering 31, 183-192.
| Crossref | Google Scholar |
Kauffman JB, Sanford RL, Cummings DL, Salcedo IH, Sampaio EVSB (1993) Biomass and nutrient dynamics associated with slash fires in neotropical dry forests. Ecology 74, 140-151.
| Crossref | Google Scholar |
Keeley JE (2009) Fire intensity, fire severity and burn severity: a brief review and suggested usage. International Journal of Wildland Fire 18, 116-126.
| Crossref | Google Scholar |
Kitzberger T (1994) ‘Fire Regime Variation along a Northern Patagonian Forest-Steppe Gradient: Stand and Landscape Response.’ (University of Colorado). Available at https://scholar.google.com/scholar?q=Kitzberger%2C+T.+1994.+Fire+regime+variation+along+a+northern+Patagonian+forest–steppe+gradient%3A+stand+and+landscape+response.+Ph.D.+dissertation%2C+Department+of+Geography%2C+University+of+Colorado%2C+Boulder%2C+Co.#d=gs_cit&t=1705343958116&u=%2Fscholar%3Fq%3Dinfo%3AyOb_rfFT5YcJ%3Ascholar.google.com%2F%26output%3Dcite%26scirp%3D0%26hl%3Des
Kitzberger T, Veblen TT (1999) Fire-induced changes in northern Patagonian landscapes. Landscape Ecology 14, 1-15.
| Crossref | Google Scholar |
Kitzberger T, Raffaele E, Heinemann K, Mazzarino MJ (2005) Effects of fire severity in a north Patagonian subalpine forest. Journal of Vegetation Science 16, 5-12.
| Crossref | Google Scholar |
Kitzberger T, Perry GLW, Paritsis J, Gowda JH, Tepley AJ, Holz A, Veblen TT (2016) Fire–vegetation feedbacks and alternative states: common mechanisms of temperate forest vulnerability to fire in southern South America and New Zealand. New Zealand Journal of Botany 54, 247-272.
| Crossref | Google Scholar |
Kitzberger T, Tiribelli F, Barberá I, Gowda JH, Morales JM, Zalazar L, Paritsis J (2022) Projections of fire probability and ecosystem vulnerability under 21st century climate across a trans-Andean productivity gradient in Patagonia. Science of The Total Environment 839, 156303.
| Crossref | Google Scholar | PubMed |
Lentile LB, Morgan P, Hudak AT, Bobbitt MJ, Lewis SA, Smith AM, Robichaud PR (2007) Post-fire burn severity and vegetation response following eight large wildfires across the western United States. Fire Ecology 3, 91-108.
| Crossref | Google Scholar |
Luce C, Morgan P, Dwire K, Isaak D, Holden Z, Rieman B (2012) ‘Climate change, forests, fire, water, and fish: Building resilient landscapes, streams, and managers’. Gen. Tech. Rep. RMRS-GTR-290. 207 p. (U.S. Department of Agriculture, Forest Service, Rocky Mountain Research Station: Fort Collins, CO)
Mantero G, Morresi D, Negri S, Anselmetto N, Lingua E, Bonifacio E, Garbarino M, Marzano R (2023) Short-term drivers of post-fire forest regeneration in the Western Alps. Fire Ecology 19, 23.
| Crossref | Google Scholar |
Mazzarino MJ, Bertiller MB, Sain C, Satti P, Coronato F (1998) Soil nitrogen dynamics in northeastern Patagonia steppe under different precipitation regimes. Plant and Soil 202, 125-131.
| Crossref | Google Scholar |
Mermoz M, Kitzberger T, Veblen TT (2005) Landscape influences on occurrence and spread of wildfires in Patagonian forests and shrublands. Ecology 86, 2705-2715.
| Crossref | Google Scholar |
Mora JL, Armas‐Herrera CM, Guerra JA, Arbelo CD, Rodríguez‐Rodríguez A, Notario del Pino JS (2016) A comparative study of long‐term effects on fire‐affected volcanic soils in two different ecosystems in the canary islands. Land Degradation & Development 27, 1489-1500.
| Crossref | Google Scholar |
Mutch LS, Swetnam TW (1995) Effects of fire severity and climate on ring-width growth of giant sequoia after fire. In ‘Proceedings symposium on fire in wilderness and park management: past lessons and future opportunities’. Gen. Tech. Rep. INT-GTR-320. (US Department of Agriculture, Forest Service, Ogsen). Available at https://citeseerx.ist.psu.edu/document?repid=rep1&type=pdf&doi=599c13138873d3809c92f34b4b53b3916a3dc439
Neary DG, Ryan KC, DeBano LF (2005) Wildland fire in ecosystems: effects of fire on soils and water. Gen Tech Rep RMRS-GTR-42-Vol4. (US Department of Agriculture, Forest Service, Rocky Mountain Research Station: Ogden, UT) 10.2737/RMRS-GTR-42-V4
Neris J, Santamarta JC, Doerr SH, Prieto F, Agulló-Pérez J, García-Villegas P (2016) Post-fire soil hydrology, water erosion and restoration strategies in Andosols: a review of evidence from the Canary Islands (Spain). iForest-Biogeosciences and Forestry 9, 583-592.
| Crossref | Google Scholar |
Olsen S, Cole CV, Watanabe FS, Dean LA (1954) ‘Estimation of Available Phosphorus in Soils by Extraction with Sodium Bicarbonate.’ (US Department of Agriculture) Available at https://books.google.com/books?hl=en&lr=&id=d-oaM88x5agC&oi=fnd&pg=PA2&ots=z_VhVDgTXF&sig=C8hefL9NShhTUEofgurpTPCgJ2U
Pardini G, Gispert M, Dunjó G (2004) Relative influence of wildfire on soil properties and erosion processes in different Mediterranean environments in NE Spain. Science of The Total Environment 328, 237-246.
| Crossref | Google Scholar | PubMed |
Parson A, Robichaud PR, Lewis SA, Napper C, Clark JT (2010) Field guide for mapping post-fire soil burn severity. General Technical Report of the Rocky Mountain Research Station GTR-243. (US Department of Agriculture, Forest Service, Rocky Mountain Research Station: Ft. Collins, CO, USA) 10.2737/RMRS-GTR-243
Pearson AK, Pearson OP, Gomez IA (1994) Biology of the bamboo Chusquea culeou (Poaceae: Bambusoideae) in southern Argentina. Vegetatio 111, 93-126.
| Crossref | Google Scholar |
Peduto D, Iervolino L, Esposito G, Foresta V, Matano F, Masi R (2022) Clues of wildfire-induced geotechnical changes in volcanic soils affected by post-fire slope instabilities. Bulletin of Engineering Geology and the Environment 81, 454.
| Crossref | Google Scholar |
Pereyra FX, Bouza P (2019) Soils from the Patagonian region. In ‘The Soils of Argentina’. (Ed. G Rubio) pp. 101–121. (World Soils Book Series, Springer) 10.1007/978-3-319-76853-3_7
Pinheiro J, Bates D R Core Team (2023) nlme: Linear and nonlinear mixed effects models. R package version 3.1-164. Available at https://CRAN.R-project.org/package=nlme
Rago MM, Urretavizcaya MF, Morelli P, Mohr Bell D, Guzmán M (2023) Early vegetation recovery following a mid to high-severity fire in the Andean-Patagonian forests. Austral Ecology 49, e13319.
| Crossref | Google Scholar |
Richter LL, Frangi JL (1992) Bases ecológicas para el manejo del bosque de Nothofagus pumilio de Tierra del Fuego. Revista de la Facultad de Agronomía 68, 35-52 [In Spanish with English abstract].
| Google Scholar |
Rivas Y, Canseco MI, Knicker H, Etcheverría P, Godoy R, Matus F, Valenzuela E, Gallardo R (2016) Variación en el contenido de glomalina relacionada a las proteínas del suelo, después de un incendio forestal en un Andisol en bosques de Araucaria araucana del centro-sur de Chile. Bosque (Valdivia) 37, 409-417 [In Spanish].
| Crossref | Google Scholar |
Rogers BM, Balch JK, Goetz SJ, Lehmann CER, Turetsky M (2020) Focus on changing fire regimes: interactions with climate, ecosystems, and society. Environmental Research Letters 15, 030201.
| Crossref | Google Scholar |
Santín C, Doerr SH (2016) Fire effects on soils: the human dimension. Philosophical Transactions of the Royal Society B: Biological Sciences 37, 20150171.
| Crossref | Google Scholar | PubMed |
Santorufo L, Memoli V, Panico SC, Santini G, Barile R, Giarra A, Maisto G (2021) Combined effects of wildfire and vegetation cover type on volcanic soil (functions and properties) in a Mediterranean region: comparison of two soil quality indices. International Journal of Environmental Research and Public Health 18, 5926.
| Crossref | Google Scholar | PubMed |
Satti P, Mazzarino MJ, Gobbi M, Funes F, Roselli L, Fernandez H (2003) Soil N dynamics in relation to leaf litter quality and soil fertility in north-western Patagonian forests. Journal of Ecology 91, 173-181.
| Crossref | Google Scholar |
Satti P, Mazzarino MJ, Roselli L, Crego P (2007) Factors affecting soil P dynamics in temperate volcanic soils of southern Argentina. Geoderma 139, 229-240.
| Crossref | Google Scholar |
Schollenberger CJ, Simon RH (1945) Determination of exchange capacity and exchangeable bases in soil—ammonium acetate method. Soil Science 59, 13-24.
| Crossref | Google Scholar |
Silveira MVF, Petri CA, Broggio IS, Chagas GO, Macul MS, Leite CCSS, et al. (2020) Drivers of fire anomalies in the Brazilian Amazon: lessons learned from the 2019 fire crisis. Land 9, 516.
| Crossref | Google Scholar |
Solomun MK, Ferreira CSS, Eremija S, Tošić R, Lazović N, Češljar G (2021) Long-term* fire effects on vegetation and topsoil properties in beech forests of Manjaca Mountain (western Bosnia and Herzegovina). International Journal of Wildland Fire 30, 269-282.
| Crossref | Google Scholar |
Soto B, Diaz-Fierros F (1993) Interactions between plant ash leachates and soil. International Journal of Wildland Fire 3, 207-216.
| Crossref | Google Scholar |
Stevens-Rumann CS, Morgan P (2019) Tree regeneration following wildfires in the western US: a review. Fire Ecology 15, 15.
| Crossref | Google Scholar |
Takahashi M, Furusawa H, Limtong P, Sunanthapongsuk V, Marod D, Panuthai S (2007) Soil nutrient status after bamboo flowering and death in a seasonal tropical forest in western Thailand. Ecological Research 22, 160-164.
| Crossref | Google Scholar |
Urretavizcaya MF (2010) Propiedades del suelo en bosques quemados de Austrocedrus chilensis en Patagonia, Argentina. Bosque (Valdivia) 31, 140-149 [In Spanish with English abstract].
| Crossref | Google Scholar |
Urretavizcaya MF, Defossé G, Gonda HE (2006) Short-term effects of fire on plant cover and soil conditions in two Austrocedrus chilensis (cypress) forests in Patagonia, Argentina. Annals of Forest Science 63, 63-67.
| Crossref | Google Scholar |
Urretavizcaya MF, Peri PL, Monelos L, Arriola H, Oyharçabal MF, Contardi LT, Muñoz M, Sepúlveda E, Defossé GE (2018) Condiciones de suelo y vegetación en tres bosques quemados de Nothofagus pumilio en Argentina y experiencias para su restauración activa. Ecología Austral 28, 383-399 [In Spanish].
| Crossref | Google Scholar |
Urretavizcaya MF, Albarracín V, Orellana I, Rago MM, López , Bernal P, Monelos L, Peri PL (2022) Composition and spatial variation of germinable seed bank in burned Nothofagus pumilio forests in Patagonia Argentina. Forests 13, 1902.
| Crossref | Google Scholar |
Veblen TT (1982) Growth patterns of Chusquea bamboos in the understory of Chilean Nothofagus forests and their influences in forest dynamics. Bulletin - Torrey Botanical Club 109, 474-487.
| Crossref | Google Scholar |
Veblen TT, Kitzberger T, Villalba R, Donnegan J (1999) Fire history in northern Patagonia: the roles of humans and climatic variation. Ecological Monographs 69(1), 47-67.
| Crossref | Google Scholar |
Veblen TT, Kitzberger T, Raffaele E, Lorenz DC (2003) Fire History and Vegetation Changes in Northern Patagonia, Argentina. In ‘Fire and Climatic Change in Temperate Ecosystems of the Western Americas. Ecological studies’. (Eds TT Veblen, WL Baker, G Montenegro, TW Swetnam) pp. 265–295 (Springer: New York, NY, USA) 10.1007/0-387-21710-X_9.
Veblen TT, Kitzberger T, Raffaele E, Mermoz M, González ME, Sibold JS, Holz A (2008) The historical range of variability of fires in the Andean Patagonian Nothofagus forest region. International Journal of Wildland Fire 17, 724-741.
| Crossref | Google Scholar |