Resurfacing of underground peat fire: smouldering transition to flaming wildfire on litter surface
Yichao Zhang A B , Yang Shu B , Yunzhu Qin


A
B
C
Abstract
Smouldering wildfires in peatlands are one of the largest and longest-lasting fire phenomena on Earth, but it is unclear whether such underground peat fires can resurface to the ground and ignite a flame on the litter layer.
This work conducted a laboratory experiment by putting a 5-cm thick litter layer (banyan tree leaves with a density of 27–53 kg/m3) onto a 10-cm thick peat sample (moisture content of 10–100%).
Tests confirmed that a smouldering peat fire, ignited at the bottom, can propagate upwards and resurface to ignite a flaming wildfire on the surface litter layer. The propensity of litter to be flaming ignited decreased with increasing peat moisture content and litter layer density. We found the threshold of such surface flaming as a function of temperature and temperature increase rate at the interface between peat and litter. Finally, large field experiments successfully reproduced and validated the laboratory observations.
This work reveals an important wildfire ignition phenomenon that has received little attention but may cause new spot fires, accelerate fire progression and exacerbate its hazards.
Keywords: flaming ignition, hot spot, litter layer, peat fire, re-emerging wildfire, smouldering to flaming transition, upward peat fire, wildland fire.
Introduction
Peat is a type of carbon-rich organic soil that accumulates a considerable amount of incompletely decomposed vegetation residues under acidic, anaerobic and close to water-saturated conditions (Hugron et al. 2013). Peatlands are essential ecosystems and carbon sinks globally, supporting biological diversity for a wide variety of wildlife habitats (Page et al. 2011; Lin et al. 2020), and storing nearly one-third (~600 Gt) of the terrestrial organic carbon on the planet (Freeman et al. 2001; Page et al. 2011). However, peatlands are threatened by wildfires driven by anthropogenic and natural reasons (Turetsky et al. 2015; Rein and Huang 2021; Santoso et al. 2022; Cui et al. 2023). For example, in Malaysia, land clearance activities such as slash and burn practised by the local community are the main contributors to peat fire spread to forest reserves, leading to cross-border haze events and many health issues for nearby residents. As environmental conditions become more favourable for wildfires, peatlands have suffered from their worst wildfire seasons and longest-lasting burning durations (Witze 2020; Lin et al. 2021a). Over the past few decades, more frequent wildfires in peatlands have emerged as a global concern owing to their potential to cause significant air pollution, accelerate terrestrial organic carbon loss and exacerbate the effects of climate change (Page et al. 2002; Mack et al. 2011; Rein and Huang 2021; Qin et al. 2022b).
Peat fire is dominated by smouldering, a slow, low-temperature and flameless burning process, different from regular flaming wildfire in its chemistry, heat and mass transport processes, and time scales (Ohlemiller 1986; Rein 2013; Lin et al. 2019, 2020). In general, the upper peat layer is most vulnerable to ambient conditions and often experiences a high burn severity during wildfires (Wilkinson et al. 2020). Once ignited from the top surface, smouldering peat fires can spread both laterally and vertically with limited oxygen supply or high moisture conditions to expand the burning zone (Prat-Guitart et al. 2016; Lin et al. 2020; Qin et al. 2022a), supporting one of the most predominant and persistent fire phenomena on Earth (Rein 2014; Rein and Huang 2021). These fires may survive underground for months and years despite extreme weather changes (e.g. violent rainy weather) or human interventions (e.g. building a firebreak) (Ramadhan et al. 2017; Lin et al. 2020, 2021b; Santoso et al. 2021), and firefighting operations are usually ineffective, which may result in significant economic losses and pose a severe threat to the safety of firefighters (Garg et al. 2023b).
Even when near-surface smouldering fires are extinguished, hibernating underground hot spots may still survive in the deep soil layer (Lukenbach et al. 2015; Qin et al. 2022b), as schematically illustrated in Fig. 1. These hidden underground smouldering fires (i.e. hot spots) can sustain themselves at a low temperature and propagate in a creeping manner. Recent laboratory studies have shown that peat fires can last for weeks with a limited oxygen supply (Qin et al. 2022a, 2022b), so they are extremely difficult to detect by human patrols and satellite imaging. When the dry and hot season arrives, the topsoil layer may gradually become dry and warm, and the deep smouldering hot spots may start to spread upwards and resurface to the ground (Fig. 1). Currently, this ‘holdover’ and ‘re-emerging’ fire phenomenon has been observed in peatlands of different regions (McCarty et al. 2020; Scholten et al. 2021), and has also been successfully reproduced in laboratory-scale experiments (Huang and Rein 2019; Qin et al. 2022b; Shan et al. 2023; Yin et al. 2023). However, whether a resurfacing smouldering fire can ignite a flame on the litter layer, leading to a flaming wildfire, requires more fundamental research.
Schematic diagram of a hibernating underground peat fire that may spread upwards and resurface to trigger a flaming wildfire.
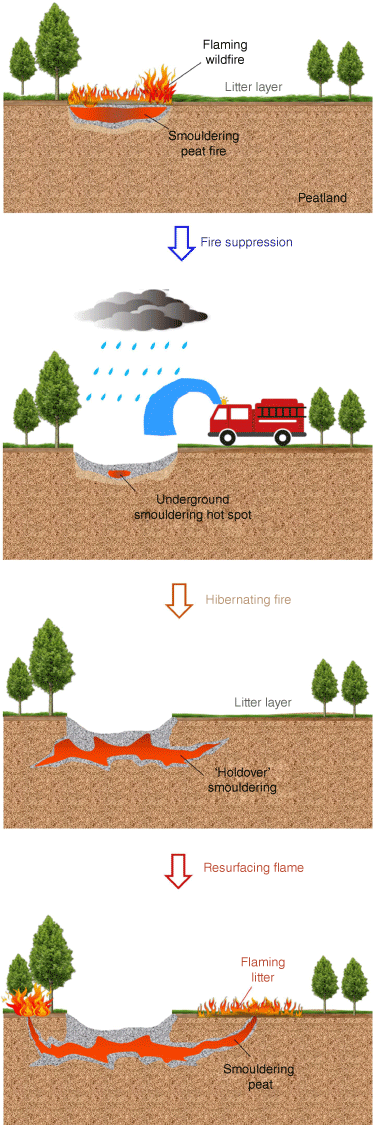
Smouldering and flaming are both important combustion phenomena in wildfires, and one can transition to the other under specific conditions (Santoso et al. 2019). The smouldering-to-flaming (StF) transition represents an abrupt initiation of gas-phase burning preceded by smouldering, accelerating fire spread and heat release, as well as increasing the corresponding fire hazards (Santoso et al. 2019). When the resurfacing smouldering front arrives at the ground surface, it can dry and heat the accumulated litter. Eventually, the litter layer will start to smoulder, and then, because of its highly porous structure, the smouldering of the litter layer may transition to flaming with the wind (i.e. a sufficient oxygen supply), igniting a new flaming wildfire, as illustrated in Fig. 1. This temporally and spatially unpredictable process poses a challenge in wildfire prevention, detection and mitigation, and requires in-depth scientific investigation.
This work aims to explore whether a hibernating underground peat fire can resurface from underground and then ignite a flame on the surface litter layer through laboratory-scale experiments. Replicated tests were conducted by putting a litter layer of banyan tree leaves with different densities on a smouldering peat column of different moisture contents. Probabilities and thresholds of flaming ignition of the litter layer by the resurfacing smouldering hot spots were quantified. Finally, large-scale outdoor experiments were performed to reproduce and validate the laboratory tests.
Experimental methods
Fuel samples and lab test setup
Moss peat from Estonia (Fig. 2) was used in this work owing to its uniform density, particle size and organic content, ensuring good repeatability of the experiments. Before tests, the peat samples were oven-dried at 90°C for at least 48 h. When the oven-dried peat was exposed to air, it can rapidly absorb ambient moisture and achieve a new equilibrium moisture content (MCp) of ~10%. In order to achieve other desired moisture contents, the oven-dried samples were further mixed with water and stored in sealed boxes for at least 1 week for homogenisation, as in our previous work (Huang et al. 2016; Lin et al. 2019). The dry bulk density of peat (ρp) was measured to be ~145 kg/m3, a value that was kept for samples of different moisture contents.
Leaves of banyan trees were chosen as a representative litter layer in the experiment (see Fig. 2). Before tests, litter samples were oven-dried at 90°C for 48 h and reached an equilibrium moisture content (MCl) of 10% when exposed to ambient conditions. The natural dry bulk density (ρl) of leaves was measured to be ~27 kg/m3, and the relative uncertainty was within 5%.
Fig. 2 shows the schematic diagram of the experimental set-up, which consisted mainly of a reactor, an array of thermocouples, a visual camera and a coil igniter. The open-top reactor, with an inner cross-section area of 15 × 15 cm2 and a total height of 15 cm, was made of 1-cm thick insulation ceramic boards. The outer surfaces of the reactor were further sealed with aluminium foil to prevent air leakage and reduce environmental heat loss. To monitor the underground smouldering peat fire and the transition to flaming, six thermocouples with a bead diameter of 1 mm were inserted from the side insulation wall into the central axis at intervals of 3 cm (see Fig. 2). Specifically, the interface between litter and peat layers was set to ‘0 cm’, ‘+3 cm’ was in the upper litter layer, and ‘−3 cm’, ‘−6 cm’, ‘−9 cm’, and ‘−12 cm’ were in the lower peat layer. Similar set-ups have also been widely used for past laboratory-scale fire experiments (Benscoter et al. 2011; Christensen et al. 2019; Huang and Rein 2019; Lin et al. 2021a; Qin et al. 2022b).
Laboratory test procedure
The reactor had a 5-cm thick upper layer of litter and a 10-cm thick lower layer of peat (see Fig. 2). Two groups of experiments were designed for two control parameters, the moisture content of the peat layer and the bulk density of the litter layer:
The moisture content of the moss peat layer (MCp) was increased from 10% (air-dried) to 25, 50, 70 and 100%, with an absolute uncertainty of ~5%;
The bulk density of the litter layer was increased from 27 to 36, 40, 44 and 53 kg/m3, with an uncertainty of ~1 kg/m3. At the same time, the moisture content of the moss peat layer was kept at 10%.
Because the litter layer would be dried by the hot emissions from the smouldering peat layer, the moisture content of the upper litter latter was kept at air-dried conditions (i.e. MCl ≈ 10%) to simplify the problem. In addition to the thermocouple array, a visual camera was also installed above the samples to record the experimental phenomena.
To initiate upward peat fire spread, a 10-cm long coil heater was placed directly above the bottom of the ceramic board, and the ignition protocol was set as 100 W for 20 min. This ignition protocol was proved to be sufficient to start a robust smouldering peat fire (Huang and Rein 2019). Afterwards, the ignited sample was allowed to burn and propagate freely to observe whether the underground smouldering peat could ignite the litter layer and trigger a flame. During laboratory experiments, the ambient temperature was 25 ± 2°C, the relative humidity was 50 ± 5% and the ambient pressure was 101.3 kPa (1 atm). To ensure test repeatability, for each scenario, at least five repeating tests were conducted.
Field tests
To better demonstrate the existence of resurfacing flames from an underground peat fire, we further conducted a group of larger-scale outdoor experiments in wildlands in the Inner Mongolia region. Specifically, we dug a large fire test area of 1.00 × 1.00 m on clay ground with a depth of 15 cm and then filled it with the same type of moss peat (lower soil layer) and banyan leaves (upper litter layer). Their thicknesses and arrangement were kept the same as for the small-scale laboratory experiments, that is, a 10-cm thick air-dried peat layer covered by a 5-cm thick air-dried litter layer of 27 kg/m3.
To mimic a real underground peat fire spot, only a small region of peat soil at one corner was heated to smouldering initially. The corner ignition region had dimensions of 10 cm (length) × 10 cm (width) × 15 cm (depth). Instead of using a coil heater, some pre-ignited peat samples were placed into this region to act as a heat and ignition source to initiate an underground smouldering hot layer. Afterwards, this ignition region was refilled with peat and litter layers, just like the laboratory test. The experimental process was recorded with a visual camera from above. During the tests, the ambient temperature was ~20°C, the relative humidity was ~40%, and the low environmental wind velocity was below 3 m/s.
Results and discussion
Fire phenomena
Fig. 3a shows an example where the smouldering front successfully propagated upwards and ignited the litter to sustain a flame; a more detailed burning process can be found in Supplementary Video S1. This specific case used air-dried moss peat of 10% moisture content and air-dried leaves of 27 kg/m3. During the experiment, several stages could be observed:
Once the peat sample was ignited from the bottom, the smouldering fire started to spread upward (evidenced by the thermocouple reading; see Supplementary Fig. S1). However, no smoke could be observed because smoke particles were absorbed by the peat soil (Huang and Rein 2019; Qin et al. 2022b).
When the smouldering front arrived at the interface between the peat and litter (see 119 min in Fig. 3a), the litter layer started to smoulder as it was heated by the smouldering peat, generating a visible smoke plume. The litter layer also collapsed locally owing to the burning and deformation of leaves.
Straight after that, a portion of the litter surface turned black (or charred), and suddenly, a flame was ignited at 121 min, which grew quickly and became self-sustaining.
The litter layer was mostly burnt out within 2 min, and then the flame extinguished. After that, the smouldering fire continued propagating downward for several hours to consume the charred peat, where a process of surface regression could be seen (Huang and Rein 2019).
Snapshots of fire phenomena: (a) resurfacing with flame; and (b) no flaming ignition from the smouldering peat fire, where air-dried moss peat of 10% MC and leaves of 27 kg/m3 density were used.
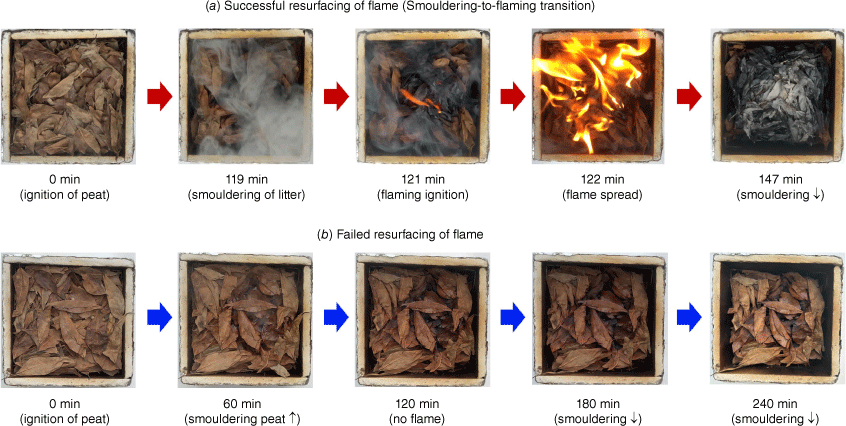
Therefore, we demonstrate that the resurfacing of underground peat fire can ignite litter to sustain a flaming wildfire. Such a resurfacing phenomenon may start new flaming wildfires, which are largely unexpected and whose ignition source is difficult to identify.
Smouldering-to-flaming can be regarded as a rapid initiation of homogeneous gas-phase ignition (i.e. flaming) induced by a heterogeneous solid surface reaction (i.e. smouldering), which is predominantly controlled by the oxygen supply and heat loss (Santoso et al. 2019; Garg et al. 2023a). In this transition process, a robust smouldering reaction with a higher temperature (i.e. glowing) and propagation rate is necessary to provide sufficient heat to accelerate pyrolysis and ignite the fuel–air mixture (Torero et al. 2020). As the smouldering front gradually propagated upwards, its temperature or intensity increased owing to a better oxygen supply (Huang and Rein 2019; Qin et al. 2022b). When the smouldering front arrived at the interface between the peat and litter, the litter layer was ignited by smouldering hot spots and started to pyrolyse and smoulder. Meanwhile, the smouldering peat continuously provided heat to the litter layer, which may compensate for heat losses to the surroundings. As a result, the reaction inside the litter layer became stronger, leading to increasing reaction intensity and temperatures favouring the occurrence of the StF transition. Eventually, with the excess pyrolysates and heating from the smouldering front, a gas-phase flaming ignition occurred, seen as an Stf transition or resurfacing flame.
In contrast, Fig. 3b shows an example where the smouldering peat fire successfully propagated upward to the surface but no flame was triggered in the litter layer (see Supplementary video S2), where the same air-dried moss peat and leaves had been selected. After the peat was ignited at the bottom, the smouldering fire front also gradually propagated upwards to the surface. The litter layer was also heated and pyrolysed, but the observed smoke plume was much weaker, and no flaming ignition was observed. Finally, the peat layer was mostly consumed, evidenced by the depressed surface and the ash layer below the litter layer. However, the litter layer showed little change, except for some shrinkage and colour change.
Note that different fire phenomena can be observed even under the same ignition and fuel conditions. One of the possible reasons is that the properties of peat soils and litters may vary with time, location and depth, with significant variability and uncertainty. Meanwhile, the stacking orientation (e.g. the direction of litter) may also affect the heat and mass transfer processes. Because of the large uncertainty in such a complex fire event, the Stf transition (or the resurfacing flame) triggered by the resurfacing underground peat fire is essentially a probabilistic event. Thus, it is critical to conduct several replicated tests and analyse the detailed fire processes for each test. In terms of the time to flaming, it takes a long time (100–600 min) for the transition from the ignition of the smouldering peat layer to the appearance of flame in the litter layer (see Supplementary Fig. S2). In general, such a transition time increases as the peat moisture content increases, because of slower upward smouldering spread in the peat layer and a longer heat time to the litter layer.
Effect of moisture content (peat) and bulk density (leaves)
Fig. 4 plots the probability of flaming ignition on the litter layer by an underground smouldering peat fire as a function of (a) peat moisture content, and (b) litter bulk density. With a decrease in both the moisture content of the peat layer and the bulk density of the litter layer, the probability of such process increases. For example, as the moisture content of the peat layer decreases from 70 to 10%, the probability of flaming ignition in the dry litter increases from 40% to ~80% (Fig. 4a). Similarly, as the dry bulk density of the litter layer decreases from 44 to 27 kg/m3, the flaming ignition probability increases from ~20% to ~80% (Fig. 4b). By defining the 50% probability as the characteristic value, we can find a maximum peat moisture of 60% and maximum litter bulk density of 38 kg/m3 as the critical conditions for resurfacing flaming. In short, a loosely packed litter layer on drier peatland is prone to trigger a new flaming wildfire when there is an underground smouldering peat fire.
Probability of flaming ignition of the litter layer under different conditions: (a) different moisture contents of peat layer; and (b) different bulk densities of litter layer.
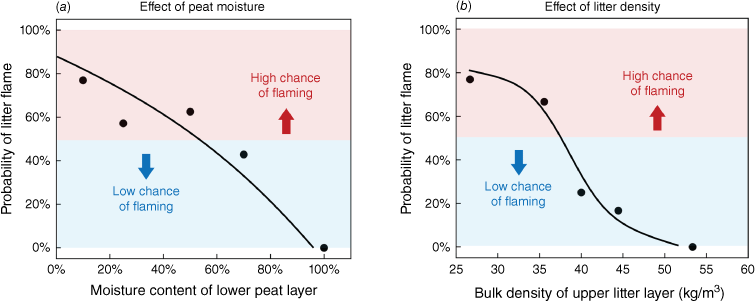
When the peat layer has a higher moisture content, extra heat is required to evaporate the water. Then, heating from the smouldering peat fire becomes insufficient to ignite a flame on the litter layer. As a result, the probability of a resurfacing flame decreases as the peat moisture increases (Fig. 4a). However, as the density of the litter layer increases, its porosity decreases accordingly, so there is less oxygen supply to the litter layer to help trigger a flame. This is why the flaming ignition probability decreases as the bulk density of the litter layer increases (Fig. 4b).
Flaming threshold of the litter layer
Fig. 5 shows the time evolution of the temperatures and the temperature increase rates (i.e. the time derivative of temperature) when the smouldering peat fire reaches the litter layer. For a better comparison, only the temperature curves of the interface (0 cm), litter layer (3 cm above) and peat layer (3 cm below) are shown here. More examples of temperature profiles can be found in the Supplemental materials.
Temperatures (upper) and temperature increase rates (lower) for (a) a resurfacing flame, and (b) no flaming ignition, where time zero is the moment when the coil igniter is turned on, and the yellow shadowed region represents the flaming period of the litter layer.
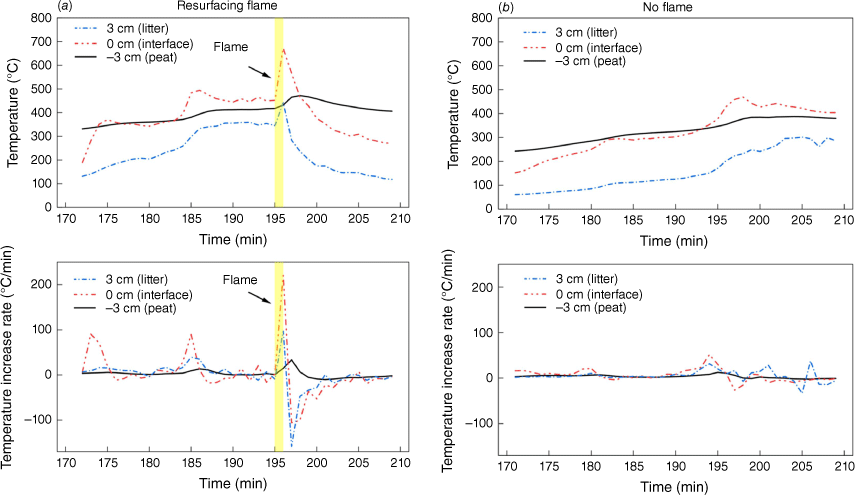
As shown in Fig. 5a, before the occurrence of flame, the surface temperature of the peat layer was fairly stable at ~450°C. Afterwards, a sudden increase to ~700°C with an average temperature increase rate of ~100°C/min was observed along with flaming ignition of the litter layer. Comparatively, for the case of no flaming ignition (Fig. 5b), although the temperature of the peat layer surface also increased to ~500°C, no flaming ignition or clear sudden temperature increase could be seen, and the maximum temperature increase rate was below 50°C/min.
Fig. 6 summarises the characteristic temperatures at the top surface of the peat layer and the corresponding temperature increase rates under different test conditions. For the no-flame conditions, the characteristic temperature is the peak temperature recorded for the whole process, whereas for flaming conditions, the characteristic temperature is the temperature recorded when the flame was triggered. The blue hollow markers represent no flaming ignition, whereas the red solid markers represent flaming ignition. Note that the temperature recorded is just the value of a single point; thus, it may not be the real highest temperature of the peat layer surface.
The relationship between the peak interface temperature (Tin (°C)) and the peak temperature increase rate β (°C/min) of all test cases. The solid red symbols represent test cases of flaming, and the hollow blue symbols represent cases of no flaming.
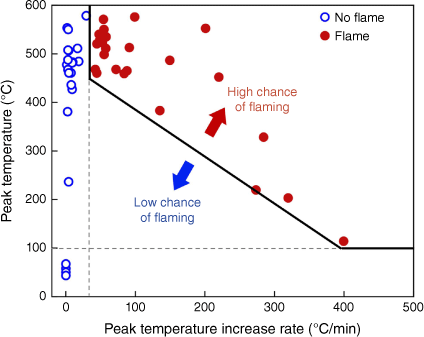
Firstly, when the recorded peak temperature at the interface between peat and litter layers is smaller than 100°C, or the corresponding peak temperature increase rate is smaller than 30°C/min, no flaming ignition of the litter layer is achieved. Above these two boundaries, the required (peak) interface temperature (Tin (°C)) is inversely proportional to the (peak) temperature increase rate (β (°C/min)). Then, an empirical criterion for resurfacing flame as a function of Tin (°C) and β (°C/min) is:
which can be used to explain all the laboratory experimental data.
Mechanism of smouldering-to-flaming transition
Fundamentally, the StF transition is an ignition of a premixed fuel–air mixture inside the pores of the litter layer, either piloted by a hot spot or auto-ignited inside a hot boundary. Here, we use Semenov’s classical 0-D auto-ignition theory to explain the observed critical conditions for the observed StF transition (Babrauskas 2003). As illustrated in Fig. 7, we choose a control volume for the hot fuel–air mixture inside pores, which releases heat via oxidation reactions while being cooled down by the surrounding leaves and soil.
Schematic diagram of the energy balance in the auto-ignition of a hot premixed fuel–air mixture inside the porous litter layer (see text for definitions of terms).
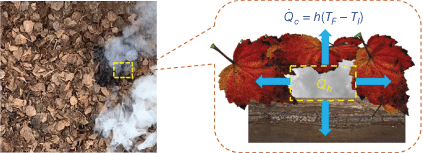
The energy balance equation of a flammable mixture is:
where mg and cν are the mass and specific heat capacity of the flammable gas mixture inside the porous litter layer, Q̇h and Q̇c are the heating and cooling rates of the gas mixture, is the volumetric reaction rate, V is the volume of the control volume inside the porous litter layer, ϕ is the porosity of the litter layer, ΔH is the heat of combustion of the gas mixture, h is the convective cooling coefficient of the gas mixture to the litter particles, S is the total surface area of the surroundings, TF is the temperature of the flammable gas mixture and Tl is the temperature of the surrounding litter particles.
To achieve auto-ignition of a flame, the heating rate should be larger than the cooling rate as:
By rearranging Eqn 3, the minimum temperature of the litter particles to achieve Stf transition can be approximated as:
This demonstrates the existence of a critical temperature of the litter layer to achieve StF transition. Moreover, as the porosity of the litter layer increases (or the bulk density decreases), the estimated minimum temperature of the litter particles to achieve a resurfacing flame decreases. All of these agree with the experimental observations shown in Fig. 4b.
Larger-scale demonstration
Scaling up the laboratory fire test to a larger field fire test is critical to understand the real wildfire process. Fig. 8 shows snapshots of some key fire phenomena from the experiment, and the original video can be found in Supplementary material Video S3. Initially, there was no significant change on the surface of the litter layer. Neither the spreading process nor smoke from the smouldering peat at the bottom could be seen. After ~15 min, some white smoke was observed, demonstrating that the self-sustained smouldering fire front was gradually propagating upwards towards the litter layer. Afterwards, some small areas on the surface turned black and charred, and a larger smoke plume could be observed. Then, a small flame suddenly appeared on the surface 1 min later, and started to propagate over the entire fuel bed.
Snapshots of some key fire phenomena of the experiment; the original video can be found in Supplementary material Video S3.
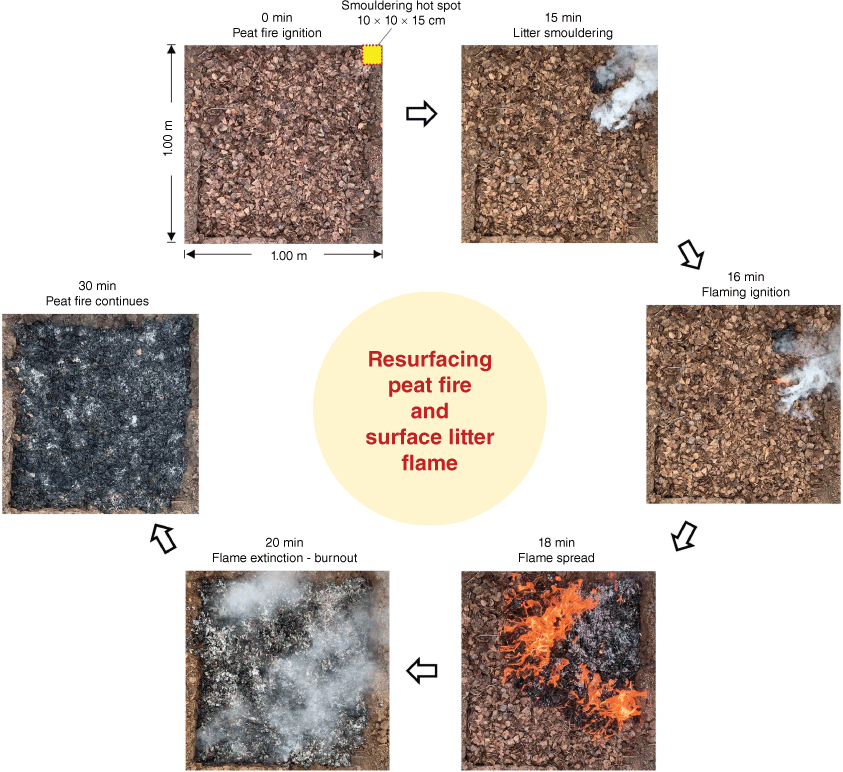
After flaming burning for 2–3 min, the flame extinguished, but the residue continued smouldering until consumption of the entire fuel bed. The whole process in the large-scale demonstration reproduced the phenomena observed in the small-scale laboratory tests (as shown in Fig. 3a). Note that the whole flaming ignition process occurred in 16 min, which was much faster than the small-scale laboratory tests (>1 h). There are three possible reasons: (1) there is environmental wind (although small) to promote the Stf transition; (2) the underground smouldering peat fire in the field is less restrained and can spread in different directions to grow faster; and (3) the smouldering of a larger litter pile can heat each other and retain heat to reduce the overall environmental cooling. The large-scale tests provide more compelling evidence that underground smouldering fires can propagate upward to the surface and ignite a flaming fire on the litter layer. In future, more and large-scale resurfacing field tests under different environmental conditions (e.g. wind and humidity) will be conducted to quantify the critical conditions and transition times of resurfacing flame in wildland fires.
Conclusions
In this work, we conducted bench-scale experiments to explore whether a hibernating underground peat fire can resurface above the ground and then ignite litter to sustain a flaming wildfire. We found that an underground smouldering peat fire could propagate upwards to the litter layer on the peatland surface. After hours, a flaming wildfire could be initiated on the litter layer without external wind, thus accelerating wildfire progression and increasing fire hazards.
Then, we quantified the effect of the moisture content of the peat layer and bulk density of the litter layer on the probability of smouldering-to-flaming transition. As the moisture content of the lower peat layer or the bulk density of the upper litter layer increases, the propensity of flaming ignition of the litter layer decreases. By defining the 50% probability as the characteristic value, we found a critical peat moisture of 60% and critical litter bulk density of 38 kg/m3 separate high and low chances of flaming ignition. A flaming ignition threshold as a relationship of temperature and temperature increase rate at the peat layer surface was also determined.
Then, a heat transfer analysis based on classical auto-ignition theory was applied to explain the critical litter layer temperature and the influence of litter porosity and density. Finally, we performed a large-scale outdoor experiment to reproduce a resurfacing flame in a much shorter transition time, which successfully demonstrated the existence of this unique smouldering-to-flaming wildfire in real fire scenarios. In our future work, we will conduct more field tests and develop a numerical model to deepen our understanding and predict this particular wildfire phenomenon.
Data availability
The data that support this study will be shared upon reasonable request to corresponding authors.
Conflicts of interest
The authors declare that they have no conflicts of interest. Dr Xinyan Huang is an Associate Editor of the Journal. To mitigate this potential conflict of interest, he was blinded from the peer review process and was not involved at any stage in the editing of this manuscript.
Declaration of funding
This work was funded by the Inner Mongolia Science and Technology Department (No. 2021CG0002), National Natural Science Foundation of China (NSFC No. 52322610), and Hong Kong Research Grants Council GRF Scheme (No. 15221523).
Author contributions
Yichao Zhang: investigation, writing of original draft, formal analysis, resources; Yang Shu: investigation, supervision, resources; Yunzhu Qin: investigation, resources; Yuying Chen: formal analysis, resources; Shaorun Lin: methodology, conceptualisation, formal analysis, writing review and editing; Xinyan Huang: supervision, formal analysis, writing review andediting, funding acquisition; Mei Zhou: supervision, resources.
References
Babrauskas V (2003) ‘Ignition Handbook.’ (Fire Science Publishers/Society of Fire Protection Engineers: Issaquah, WA, USA) 10.1023/B:FIRE.0000026981.83829.a5
Benscoter BW, Thompson DK, Waddington JM, Flannigan MD, Wotton BM, de Groot WJ, Turetsky MR (2011) Interactive effects of vegetation, soil moisture and bulk density on depth of burning of thick organic soils. International Journal of Wildland Fire 20, 418-429.
| Crossref | Google Scholar |
Cui W, Hu Y, Rein G (2023) Experimental study of the ignition conditions for self-sustained smouldering in peat. Proceedings of the Combustion Institute 39, 4125-4133.
| Crossref | Google Scholar |
Freeman C, Evans CD, Monteith DT, Reynolds B, Fenner N (2001) Export of organic carbon from peat soils. Nature 412, 785.
| Crossref | Google Scholar | PubMed |
Garg P, Shan I, Lin S, Gollner M, Fernandez-Pello C (2023a) Limiting conditions of smoldering-to-flaming transition of cellulose powder. Fire Safety Journal 141, 103936.
| Crossref | Google Scholar |
Garg P, Wang S, Oakes JM, Bellini C, Gollner MJ (2023b) The effectiveness of filter material for respiratory protection worn by wildland firefighters. Fire Safety Journal 139, 103811.
| Crossref | Google Scholar |
Huang X, Rein G (2019) Upward-and-downward spread of smoldering peat fire. Proceedings of the Combustion Institute 37, 4025-4033.
| Crossref | Google Scholar |
Huang X, Restuccia F, Gramola M, Rein G (2016) Experimental study of the formation and collapse of an overhang in the lateral spread of smouldering peat fires. Combustion and Flame 168, 393-402.
| Crossref | Google Scholar |
Lin S, Sun P, Huang X (2019) Can peat soil support a flaming wildfire? International Journal of Wildland Fire 28, 601-613.
| Crossref | Google Scholar |
Lin S, Cheung YK, Xiao Y, Huang X (2020) Can rain suppress smoldering peat fire? Science of the Total Environment 727, 138468.
| Crossref | Google Scholar |
Lin S, Liu Y, Huang X (2021a) Climate-induced Arctic–boreal peatland fire and carbon loss in the 21st century. Science of the Total Environment 796, 148924.
| Crossref | Google Scholar |
Lin S, Liu Y, Huang X (2021b) How to build a firebreak to stop smouldering peat fire: insights from a laboratory-scale study. International Journal of Wildland Fire 30, 454-461.
| Crossref | Google Scholar |
Lukenbach MC, Hokanson KJ, Moore PA, Devito KJ, Kettridge N, Thompson DK, Wotton BM, Petrone RM, Waddington JM (2015) Hydrological controls on deep burning in a northern forested peatland. Hydrological Processes 29, 4114-4124.
| Crossref | Google Scholar |
Mack MC, Bret-Harte MS, Hollingsworth TN, Jandt RR, Schuur EAG, Shaver GR, Verbyla DL (2011) Carbon loss from an unprecedented Arctic tundra wildfire. Nature 475, 489-92.
| Crossref | Google Scholar | PubMed |
McCarty JL, Smith TEL, Turetsky MR (2020) Arctic fires re-emerging. Nature Geoscience 13, 658-660.
| Crossref | Google Scholar |
Ohlemiller TJ (1986) Smoldering combustion, National Institute of Standard and Technology, Gaithersburg, MD, USA. 10.6028/NBS.IR.85‐3294
Page SE, Siegert F, Rieley JO, Boehm H-DV, Jaya A, Limin S (2002) The amount of carbon released from peat and forest fires in Indonesia during 1997. Nature 420, 61-5.
| Crossref | Google Scholar | PubMed |
Page SE, Rieley JO, Banks CJ (2011) Global and regional importance of the tropical peatland carbon pool. Global Change Biology 17, 798-818.
| Crossref | Google Scholar |
Prat-Guitart N, Rein G, Hadden RM, Belcher CM, Yearsley JM (2016) Propagation probability and spread rates of self-sustained smouldering fires under controlled moisture content and bulk density conditions. International Journal Of Wildland Fire 25, 456-465.
| Crossref | Google Scholar |
Qin Y, Chen Y, Lin S, Huang X (2022a) Limiting oxygen concentration and supply rate of smoldering propagation. Combustion and Flame 245, 112380.
| Crossref | Google Scholar |
Qin Y, Musa DNS, Lin S, Huang X (2022b) Deep peat fire persistently smouldering for weeks: a laboratory demonstration. International Journal of Wildland Fire 32, 86-98.
| Crossref | Google Scholar |
Ramadhan ML, Palamba P, Imran FA, Kosasih EA, Nugroho YS (2017) Experimental study of the effect of water spray on the spread of smoldering in Indonesian peat fires. Fire Safety Journal 91, 671-679.
| Crossref | Google Scholar |
Rein G (2013) Smouldering Fires and Natural Fuels. In ‘Fire Phenomena in the Earth System’. (Ed. CM Belcher) pp. 15–34. (John Wiley & Sons, Ltd.: New York, NY, USA) 10.1002/9781118529539.ch2
Rein G (2014) Smoldering Combustion. In ‘SFPE Handbook of Fire Protection Engineering’. (Eds MJ Hurley, D Gottuk, JR Hall, et al.) pp. 581–603. (Springer: New York, NY, USA) 10.1007/978-1-4939-2565-0_19
Rein G, Huang X (2021) Smouldering wildfires in peatlands, forests and the arctic: challenges and perspectives. Current Opinion in Environmental Science & Health 24, 100296.
| Crossref | Google Scholar | PubMed |
Santoso MA, Christensen EG, Yang J, Rein G (2019) Review of the transition from smouldering to flaming combustion in wildfires. Frontiers in Mechanical Engineering 5, 49.
| Crossref | Google Scholar |
Santoso MA, Cui W, Amin HMF, Christensen EG, Nugroho YS, Rein G (2021) Laboratory study on the suppression of smouldering peat wildfires: effects of flow rate and wetting agent. International Journal of Wildland Fire 30, 378-390.
| Crossref | Google Scholar |
Santoso MA, Christensen EG, Amin HMF, Palamba P, Hu Y, Purnomo DMJ, Cui W, Pamitran A, Richter F, Smith TEL, Nugroho YS, Rein G (2022) GAMBUT field experiment of peatland wildfires in Sumatra: from ignition to spread and suppression. International Journal of Wildland Fire 31, 949-966.
| Crossref | Google Scholar |
Scholten RC, Jandt R, Miller EA, Rogers BM, Veraverbeke S (2021) Overwintering fires in boreal forests. Nature 593, 399-404.
| Crossref | Google Scholar | PubMed |
Shan Y, Chen X, Yin S, Cao L, Tang S, Yu B, Cui C (2023) Study on the limit of moisture content of the sub-surface fires converted to the surface fires in the boreal forests of China. Fire 6, 364.
| Crossref | Google Scholar |
Torero JL, Gerhard JI, Martins MF, Zanoni MAB, Rashwan TL, Brown JK (2020) Processes defining smouldering combustion: integrated review and synthesis. Progress in Energy and Combustion Science 81, 100869.
| Crossref | Google Scholar |
Turetsky MR, Benscoter B, Page S, Rein G, Van Der Werf GR, Watts A (2015) Global vulnerability of peatlands to fire and carbon loss. Nature Geoscience 8, 11-14.
| Crossref | Google Scholar |
Wilkinson SL, Tekatch AM, Markle CE, Moore PA, Waddington JM (2020) Shallow peat is most vulnerable to high peat burn severity during wildfire. Environmental Research Letters 15, 104032.
| Crossref | Google Scholar |
Witze A (2020) The Arctic is burning like never before — and that’s bad news for climate change. Nature 585, 336-337.
| Crossref | Google Scholar | PubMed |
Yin S, Shan Y, Tang S, Douglas G, Yu B, Cui C, Cao L (2023) Study on the limit of moisture content of smoldering humus during sub-surface fires in the boreal forests of China. Forests 14, 252.
| Crossref | Google Scholar |