State and trend of surface soil organic carbon stocks and associated nutrients in Tongatapu, Kingdom of Tonga
U. Stockmann A , M. Farrell



A
B
C
D
E
F
G
Abstract
The status and temporal trend in soil organic carbon (SOC) stocks are unknown in Tongatapu, the main island and agriculture hub of the Kingdom of Tonga. This is a critical shortcoming in relation to agricultural sustainability and food security.
Evaluate the soil organic carbon stocks within Tongatapu’s main soil series relative to historic data.
Historical studies were used to establish a SOC baseline for different soil series on Tongatapu. These sites were revisited and sampled in 2018 and the SOC content of the soil samples was determined using a dry combustion analytical method. Changes in the soil nitrogen (N), phosphorus (P), and sulfur (S) nutrient pools were estimated using stoichiometry.
There was a decline in SOC stocks over the last 20–30 years. The mineralisation of soil organic matter, as represented by the decline in SOC stocks, has likely also reduced the pool of N, P, and S. Soils with the greatest SOC stocks (>50 t C ha−1) declined at the highest rates relative to soils with SOC stocks of 20–30 t C ha−1.
Agricultural practices that are employed on Tongatapu have caused the decline of SOC stocks and are threatening sustainable agriculture production.
This study shows that new farming and soil management practices should be employed that build SOC and broader soil functions.
Keywords: atolls, farming systems, Pacific soils, root cropping, soil monitoring, soil organic carbon, sustainability, volcanic islands.
Introduction
Over the last 200 years, Pacific Island Countries and Territories (PICTs) agriculture has become increasingly intensified due to societal and economic drivers. Small-holder agriculture still dominates the PICTs landscape but some cropping systems are now characterised by a reduction in production and fallow periods, increased herbicide and pesticide inputs, and haphazard use of synthetic and organic fertilisers (Antille et al. 2022). Recent assessments of sugar cane and taro production systems have shown the run-down of soil nutrients and associated decline in production (Morrison and Gawander 2016; Sharma 2016a, 2018). The disruption of soil process and function has wider ranging impacts through the modification of nutrient flows. An alarming consequence of the intensification has been the significant reduction of soil organic carbon (SOC) in semi-intensive root cropping systems (Sharma 2018). It is alarming because the degree of mechanisation in these is relatively small compared to the sugar sector, and the soils in these semi-intensive root cropping systems are pedologically young in a stable rainfall environment. Over 22 years of root cropping production soils in Taveuni, Fiji, has resulted in a 58% decline in SOC concentration across all rainfall zones (Fig. 1). It is clear that the effect of soil and plant disturbance caused by agriculture development has changed SOC concentration (Sharma 2018).
Change in SOC concentration (mg C g−1), Taveuni, Fiji. The dry, intermediate, and wet zones represent areas that receive an annual rainfall of 1500–2500, 2000–3500, and 2500–4000 (mm), respectively. The solid line is the 1:1 line, and the dashed line is the regression line (y = 0.42x, R2 = 0.97). Data sourced from Sharma (2016b).
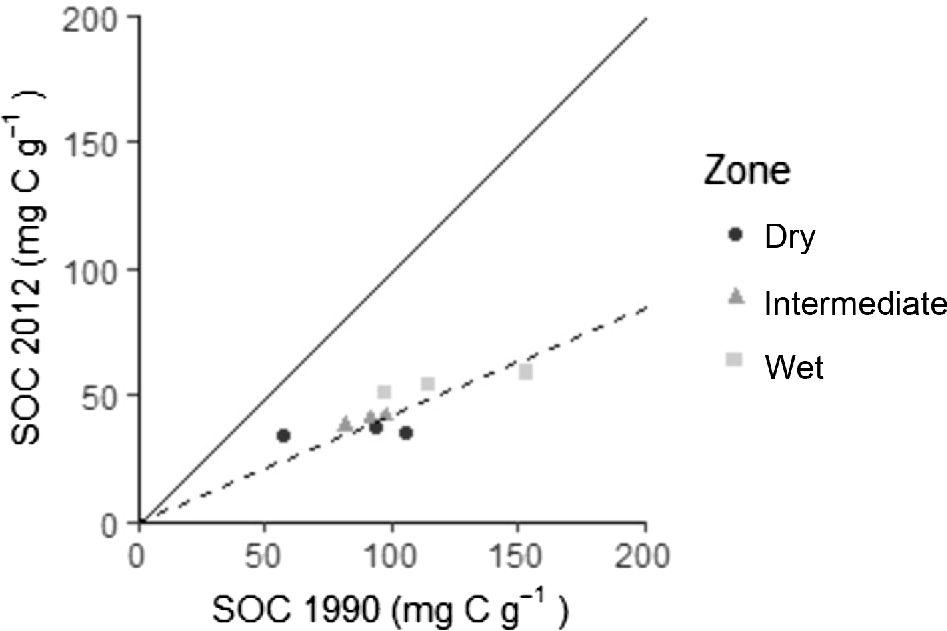
The Kingdom of Tonga, similar to Fiji, has increasingly intensified agricultural production and doubled cropping intensity between 1943 and 1993 (James 1993). This has resulted either in the elimination or shortening of traditional fallow systems which are characterised by opportunistic plant succession until the field is returned to production. Potter (1986) compared different agricultural land uses (fallow to cropping) in the Kingdom of Tonga and found a deterioration of the nutrient and physical status. Organic matter concentration reduced from 10.8% in 15 years of wood fallow to 7.3% in 4 years of grass fallow to 5.5% in a 2-year-old cropping field (Potter 1986). A subsequent study conducted by Manu (2000) in 1997 after the expansion of intensive squash production found a ~50% reduction in soil C concentration between primary forest and intensive cultivation (Fig. 2).
Total soil carbon (%) and soil 13C (%) for primary forest, mixed forest, fallow, and intensive cropping systems in Tongatapu, Kingdom of Tonga (data sourced from Manu 2000). PF is primary forest; MF is mixed forest landuse comprising a tree overstory with either cropping or grazing; I is intensive landuse by mechanised cropping production; F is intensive landuse in a 1–4 years of guinea grass fallow in mechanised cropping production.
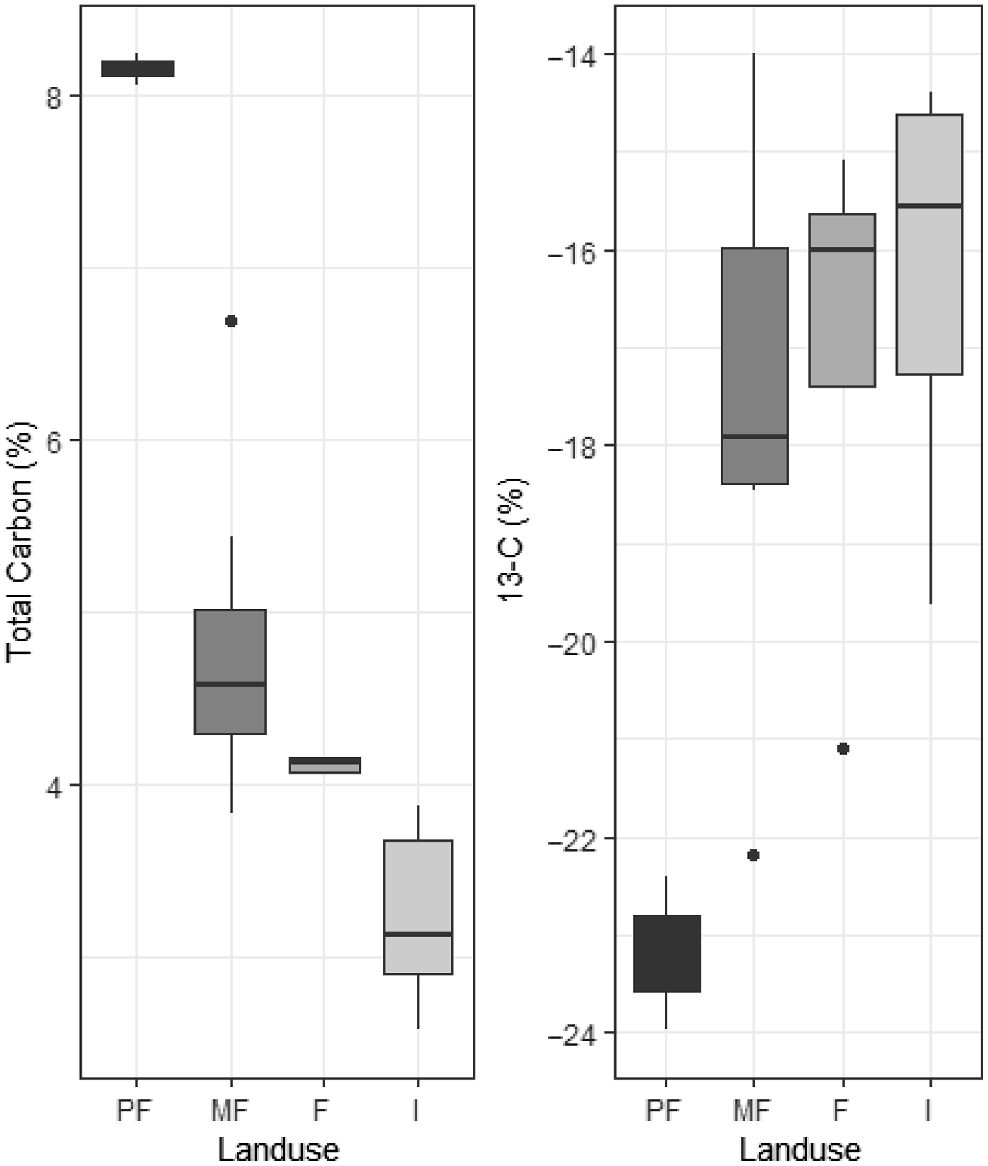
Overall, disturbance caused increased soil organic matter (SOM) mineralisation, as evidenced by the change in the soil 13C (Fig. 2), and readjustment of SOC levels to the new regime/equilibrium (Manu et al. 2014). Manu et al. (2014) concluded that disturbance, especially due to mechanised agriculture and traditional manual cultivation, was the cause of soil C decline relative to the primary forest land use.
The Kingdom of Tonga’s Ministry of Agriculture, Forestry, Fisheries and Food (MAFFF) has identified that as ‘Tonga’s agriculture sector becomes subjected to increasing pressure to feed the Kingdom in the face of climate change and other global changes and pressures, it is essential that the status of its most fundamental agriculture resource [soils] is understood’. The status and temporal trend in SOC stock is unknown in Tongatapu, the main island and agricultural hub of the Kingdom of Tonga. This is a critical shortcoming in relation to agricultural sustainability and food security for the nation as the Kingdom of Tonga seeks to increase production and reduce inputs. Since the Potter (1986) and Manu et al. (2014) studies of the Kingdom’s soils, agriculture has continued to expand cash cropping activities for export. The current work assesses the state and trend of SOC in agricultural production systems and associated nutrients in SOM, and provides insights into future management strategies for sustainable agricultural production on Tongatapu, the Kingdom of Tonga.
Materials and methods
Soils
The Kingdom of Tonga is characterised by three broad island types: low coral islands (<15 m ASL) of the Tongatapu and Ha’apai Island Groups; the raised coral islands, which have been raised by tectonic movement, of the E’ua and Tongatapu islands and the Vava’u Island Groups; and the volcanic islands, such as Hunga Tonga–Hunga Ha’apai. The soils of the Kingdom of Tonga are strongly influenced by volcanism and detailed descriptions and pedogenic interpretation was completed in the 1970s as part of the agricultural intensification research and development program (Orbell 1971, 1977; Gibbs 1976; Cowie 1980; Cowie et al. 1991). The islands of the Kingdom of Tonga consist mainly of low and raised coral limestone overlain by two different layers of andesitic volcanic ash. These deposits were sourced from the western volcanic islands within the Kingdom of Tonga and are composed of layers of fine-grained andesitic volcanic ash, estimated to be 20,000 and 5000 years old. The soils of Tongatapu are differentiated by the thickness of the soil that has developed from the younger ash deposits. Orbell (1977) states that indigenous Tongatapu farming tradition recognises ‘kelefatu soils’ and ‘tou’one soils’ which are soils derived from volcanic ash and those derived from coral sands. The main properties of the soil series that were investigated in this study are briefly described and detailed information can be found in the earlier publications (Cowie et al. 1991). In addition, the distribution of the soil series investigated is shown in Fig. 3.
These are well-drained soils on the western side of Tongatapu with deep deposits of volcanic ash (>1.0 m). They are characterised by a friable clay topsoil, with a few lapilli (spheroid, teardrop, dumbbell, or button-shaped droplets of molten or semi-molten lava) over a compact clay subsoil. These are the young soils and least weathered compared to the soils on the eastern side of the island.
These soils are well drained and developed from a slightly thinner layer of ash overlying an older ash layer 0.6–1.0 m below the ground surface. These soils are like the Fahefa soils but lack lapilli and are more weathered with increasing clay content.
These soils developed on older volcanic ash with a thin surface layer of younger ash. These soils are more weathered than the Fahefa and Vaini soils and are more heavily textured. The soils are characterised by a friable clay topsoil (~0.3 m thick), overlying a sticky clay horizon which varies 0.3–1.7 m in thickness.
These soils are formed from relatively unweathered coral sands and are characterised by sandy textures, and in the older beach ridges have developed dark greyish brown sandy loam A horizons. These soils grade into the Sopu Soil Series which has developed A horizons consisting of a variable mixed peat and finer textured material.
Site selection
There have been several historic soil surveys across Tongatapu, Kingdom of Tonga (Gibbs 1976; Potter 1986; Cowie et al. 1991), which have been used to establish baseline SOC contents for this study. Twenty-three locations were selected to represent four broad soil series found on Tongatapu from the historical soil surveys (Fig. 3). The two historical soil survey missions sampled 23 different sites across the four sampling years: 1967, 1969, 1975 (Gibbs 1976; Cowie et al. 1991), and 1986 (Potter 1986).
The Gibbs (1976) and Cowie et al. (1991) studies were conducted during two separate soil surveys of Tongatapu, and locations were identified to characterise the different Tongatapu soil series. During both studies at each location the soil was sampled with a hand auger or from a soil pit, and samples were collected between 0 and 0.1 m and an approximate sampling location was recorded. Potter (1986) characterised soils within different cropping systems on individual tax allotments, which are the Kingdom of Tonga’s standard land tenure units, and like the other studies at each site, soil was sampled with a hand auger to depth. Potter (1986) sampled five locations within each site and bulked the sampled soil for each 0.1 m depth.
The locations of the historic survey sites were identified from aligning the site sampling descriptions to current tax allotment location maps and satellite image interpretation. While field locations could be identified, the exact sampling positions for the Gibbs (1976), Potter (1986), and Cowie et al. (1991) soil surveys used in this work are approximate, but within the same soil series and land use. Land use was determined by discussions with the landholder or by observation. The land use classification is basic due to the lack of long-term records and may not capture the land use that was employed between the sampling periods.
Carbon stock calculations
The historic SOC (%) data sourced from Gibbs (1976) and Cowie et al. (1991) was determined following the modified Walkley–Black wet oxidation method (Nelson and Sommers 1996). To enable the comparison with contemporary soil carbon measurements, the historic SOC concentrations determined by the modified Walkley–Black method were converted to SOC content equivalent to the dry combustion method using a correction factor of 1.15 (Shamrikova et al. 2022). The Potter (1986) historic dataset reported SOM (%) and this was converted to the dry combustion method (%) using a correction factor of 1.72 (Nelson and Sommers 1996).
The carbon stocks in the top 0.1 m of soil were determined according to the following:
where SOC is the soil organic carbon content dry combustion equivalent (%), ρb is the soil bulk density which is assumed to be 0.90 g cm−3 based on earlier studies (Potter 1986; Cowie et al. 1991), z is soil depth (cm), and c is a conversion constant.
Cowie et al. (1991) measured soil carbon content from a range of sampling depths and often split 0–0.1 m into two sample depths. The carbon stocks in the top 0.1 m of soil were determined from the Cowie et al. (1991) data according to:
where C1 is the carbon stock determined by Eqn 1 from the first depth, 0 > z1 < 10, and C2 is the carbon stock determined by Eqn 1 from the second sample depth z2, where z2 = 10 − z1.
The historic sampling locations (Fig. 3) were relocated and revisited, and then resampled (0–0.1 m) using hand auguring (75 mm) in 2018 at the centre of the field. The SOC content of the samples collected during the 2018 soil survey were determined using the dry combustion Dumas approach (Nelson and Sommers 1996). Soil samples were air-dried and finely ground prior to the measurement of total carbon content using a LECO TruMac instrument. Any sample that had a was pre-treated with sulfurous acid to remove carbonates prior to measurement (Sanderman et al. 2011). SOC concentrations were subsequently reported on an oven-dried basis. SOC stocks in the top 0.1 m were calculated using Eqn 1. The SOC stocks were calculated using the soil bulk density at each site determined by the volumetric method (s = 0.92 g cm−3 ).
Nitrogen, phosphorus, and sulfur stock calculations
The amount of nitrogen (N), phosphorus (P), and sulfur (S) within the mineralised SOM pool was estimated using the established soil C:N:P:S (10,000:833:200:143) ratio (Kirkby et al. 2011, 2013).
Statistical analysis
The R programming language (R Core Team 2021) was used for the statistical analysis and the lme4 package (Bates et al. 2015) was used to perform a linear mixed model (LMM) analysis of the relationship between SOC and time since sampling, land use, and soil series. As fixed effects, the interaction of time since sampling and land use were used in the model. As random effects, the model had intercepts for soil series.
Results
Soil carbon and nutrient stocks
The LMM model that estimated the effect of the interaction between land use type and the sampling intervals on SOC stocks was not significantly different (χ2 = 1.24 (3), P = 0.7) from the simplified model. Visual inspection of residual plots did not reveal any obvious deviations from homoscedasticity or normality. The simplified model estimated the effect of the interaction since time of sampling and showed that SOC has declined (Fig. 4) at a rate of 0.5 t ha−1 year−1 (s.e. ± 0.1) across each soil series. Similarly, the amount of N, P, and S stored in the SOM pool also declined between the sampling periods and between soil series (Table 1).
The decline of soil carbon stocks (t ha−1) of the Fahefa, Lapaha, Nuku’alofa, and Vaini soil series, Tongatapu, Kingdom of Tonga.
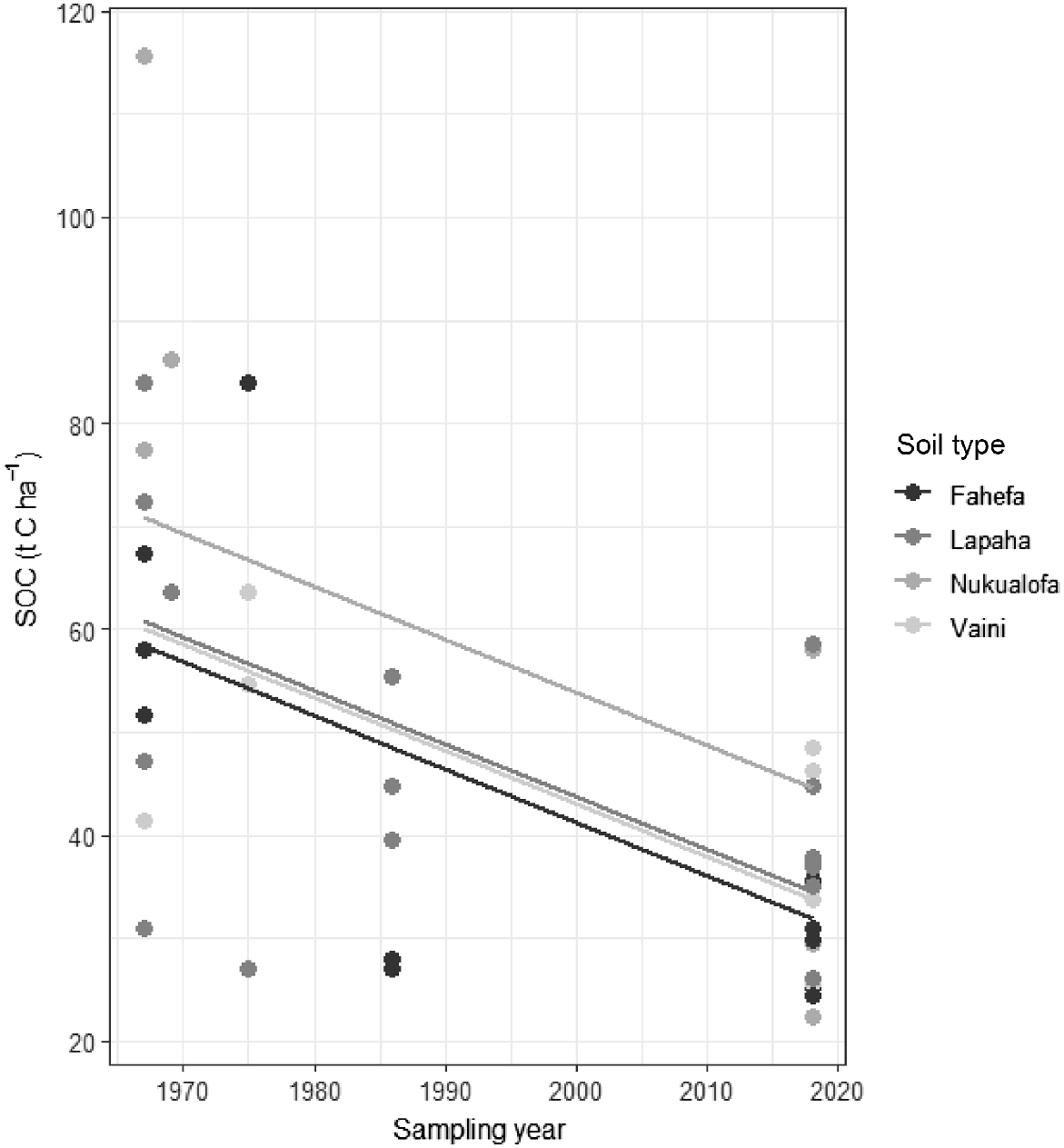
Soil series | Nitrogen (t ha−1) | Phosphorus (t ha−1) | Sulfur (t ha−1) | ||||
---|---|---|---|---|---|---|---|
μ | σ | μ | σ | μ | σ | ||
Fahefa | −2.23 | 0.78 | −0.54 | 0.19 | −0.38 | 0.13 | |
Lapaha | −1.30 | 0.90 | −0.31 | 0.22 | −0.22 | 0.15 | |
Nukua’lofa | −4.96 | 1.63 | −1.19 | 0.39 | −0.85 | 0.28 | |
Vaini | −1.78 | 0.75 | −0.43 | 0.18 | −0.31 | 0.13 | |
Grand average | −2.34 | 0.52 | −0.56 | 0.12 | −0.40 | 0.09 |
There is no relationship between SOC stocks between the historic and 2018 soil sampling period (Fig. 5). At most sampling sites, SOC declined between the historical and the 2018 soil sampling period; however, there are four locations where SOC increased. These locations include intensive cropping on Lapaha and Fahefa soil series (1986–2018), forest and cropping (F) on the Vaini soil series (1967–2018), and grass fallow on the Lapaha soil series (1967–2018).
Discussion
Since the historical soil sampling, all soil series (Fahefa, Lapaha, Nuku’alofa, and Vaini) have experienced declines in SOC stocks (t ha−1). All the soil series sampled during the surveys have been utilised for agriculture and all locations would have been subjected to varying levels of disturbance. This temporal study supports the earlier work of Manu et al. (2014), which found that soil disturbance associated with tillage for the land preparation was a casual factor of SOC decline. The experimental design used in this study indicates that it is not the land use type but soil disturbance that is driving the decline in SOC stocks. Nonetheless, further work should be carried out that enables the monitoring and evaluation of different land use strategies within each soil series on SOC stocks.
In Tongatapu, land preparation and tillage practices across all land uses are centred on disc ploughing, which has been shown elsewhere to cause significant declines in SOC (Freixo et al. 2002). Land clearing and crop residues are also often burnt as part of land preparation and are land management practices known to drive SOC decline. In general, it is evident that the current agricultural management strategy in Tongatapu, Kingdom of Tonga, is causing SOC decline. Stabilisation and rebuilding of SOC may be achieved through minimum tillage, biomass and residue management, incorporation of cover cropping and legumes, and an increased fallow period (Tian et al. 2005), though substantial context dependence exists for each of these practices with regard to their efficacy in reducing declines or indeed building SOC stocks (Chaplot and Smith 2023). Any farming system approach would need to be optimised for cultural, environmental, and economic conditions in the Kingdom of Tonga, as well as being underpinned by a coherent soil policy aimed at sustainable landscape management. Further investigation is required to identify causal effects of the applied farming system management at the four sites that experienced increased SOC stocks between the two-sampling dates in the Vaini, Lapaha, and Fahefa soil series (Fig. 5).
On Tongatapu, unlike Taveuni, Fiji, there was no relationship between the soil carbon contents of the two sampling periods. This may be due to the relatively recent intensification of the Taro production system in Taveuni during the 1990s, as opposed to the longer history of agricultural development in Tongatapu. This began in the Kingdom of Tonga in the late 1940s and evolved to the intensive mechanised agricultural system practiced since the 1980s (James 1993). Overall, SOC in the farming landscapes of Tongatapu has declined by 46% which is similar to the average Australian rate of 51% for agriculturally disturbed lands (Luo et al. 2010). However, this is much greater than the global average of soil carbon decline due to agricultural development (Sanderman et al. 2017) and may reflect the rapid change caused by the recent agricultural development of Tongatapu soils relative to the longer period of global agriculture development.
There has been a potential loss of available nutrients (Table 1) which reduces soil fertility and agriculture productivity in these low input farming systems (Sharma 2016b). The cycling of nutrients in tropical soils and net primary productivity is linked to SOM mineralisation (Tiessen et al. 1994). Current management has potentially reduced soil function in Tongatapu, and farming systems will need to rebuild SOM to provide nutrient storage and fertility. If continued reduction in SOM stock is the future status quo, then fertiliser additions will be needed to underpin farming system productivity and sustainability because of the reduced soil fertility.
Conclusions
Soil organic carbon stocks have declined over the 20–30 years between the historic and 2018 soil sampling periods due to prevailing agricultural management. There is a difference in the SOC stocks in the sampled soil series on Tongatapu, which is likely due to the environmental conditions driving pedogenesis. This study showed that disturbance across all soil types, principally through tillage for agricultural soil management, is driving the change in SOC. In terms of soil function, the enhanced SOM mineralisation rates threaten soil security and the prospect of sustainable landscape management.
Data availability
After publication the dataset will be available via the CSIRO Data Access Portal (DAP) upon request.
Conflicts of interest
Mark Farrell and Senani Karunaratne are Associate Editors for this journal. To mitigate this potential conflict of interest they had no editor-level access to this manuscript during peer review. The authors have no further conflicts of interest to declare.
Declaration of funding
The Crawford Fund provided funding for S. K. and B. S. to travel to the Kingdom of Tonga to conduct the soil sampling mission. ACIAR Grant SMCN/2016/111 provided funding for V. M. and D. M. for field sampling and a CSIRO Agriculture and Food Strategic Investment Project provided funding for the soil laboratory analysis.
Acknowledgements
The authors gratefully acknowledge the landowners who allowed the team access to their land, to sample and remove soil for testing.
References
Antille DL, Field DJ, Halavatau SM, Iramu ET, Macdonald BCT, Singh K, Webb MJ (2022) Regional soil priorities creating partnerships with Australia and New Zealand across the Pacific. Geoderma Regional 29, e00517.
| Crossref | Google Scholar |
Bates D, Mächler M, Bolker B, Walker S (2015) Fitting linear mixed-effects models using lme4. Journal of Statistical Software 67(1), 1-48.
| Crossref | Google Scholar |
Chaplot V, Smith P (2023) Cover crops do not increase soil organic carbon stocks as much as has been claimed: what is the way forward? Global Change Biology 29(22), 6163-6169.
| Crossref | Google Scholar | PubMed |
Cowie JD (1980) Soils from Andesitic Tephra and their variability, Tongatapu, Kingdom of Tonga. Soil Research 18(3), 273-284.
| Crossref | Google Scholar |
Freixo AA, Machado PLOdA, dos Santos HP, Silva CA, Fadigas FdS (2002) Soil organic carbon and fractions of a Rhodic Ferralsol under the influence of tillage and crop rotation systems in southern Brazil. Soil and Tillage Research 64(3-4), 221-230.
| Crossref | Google Scholar |
James K (1993) Cutting the ground from under them? Commercialization, cultivation, and conservation in Tonga. The Contemporary Pacific 5, 225-242.
| Google Scholar |
Kirkby CA, Kirkegaard JA, Richardson AE, Wade LJ, Blanchard C, Batten G (2011) Stable soil organic matter: a comparison of C:N:P:S ratios in Australian and other world soils. Geoderma 163(3-4), 197-208.
| Crossref | Google Scholar |
Kirkby CA, Richardson AE, Wade LJ, Batten GD, Blanchard C, Kirkegaard JA (2013) Carbon-nutrient stoichiometry to increase soil carbon sequestration. Soil Biology and Biochemistry 60, 77-86.
| Crossref | Google Scholar |
Luo Z, Wang E, Sun OJ (2010) Soil carbon change and its responses to agricultural practices in Australian agro-ecosystems: a review and synthesis. Geoderma 155(3-4), 211-223.
| Crossref | Google Scholar |
Manu V, Whitbread A, Blair N, Blair G (2014) Carbon status and structural stability of soils from differing land use systems in the Kingdom of Tonga. Soil Use and Management 30(4), 517-523.
| Crossref | Google Scholar |
Morrison RJ, Gawander JS (2016) Changes in the properties of Fijian Oxisols over 30 years of sugarcane cultivation. Soil Research 54(4), 418-429.
| Crossref | Google Scholar |
Sanderman J, Hengl T, Fiske GJ (2017) Soil carbon debt of 12,000 years of human land use. Proceedings of the National Academy of Sciences 114(36), 9575-9580.
| Crossref | Google Scholar | PubMed |
Shamrikova EV, Kondratenok BM, Tumanova EA, Vanchikova EV, Lapteva EM, Zonova TV, Lu-Lyan-Min EI, Davydova AP, Libohova Z, Suvannang N (2022) Transferability between soil organic matter measurement methods for database harmonization. Geoderma 412, 115547.
| Crossref | Google Scholar |
Sharma AC (2016a) Intensive Taro (Colocasia esculenta) cultivation and soil dynamic on volcanic Anddosols of Taveuni, Fiji. Fiji Agriculture Journal 56(1), 31-36.
| Google Scholar |
Sharma AC (2018) Soil fertility and productivity decline resulting from twenty two years of intensive Taro cultivation in Taveuni, Fiji. Fiji Agriculture Journal 57(1), 50-58.
| Google Scholar |
Tian G, Kang BT, Kolawole GO, Idinoba P, Salako FK (2005) Long-term effects of fallow systems and lengths on crop production and soil fertility maintenance in West Africa. Nutrient Cycling in Agroecosystems 71(2), 139-150.
| Crossref | Google Scholar |
Tiessen H, Cuevas E, Chacon P (1994) The role of soil organic matter in sustaining soil fertility. Nature 371, 783-785.
| Crossref | Google Scholar |