Hydrology and runoff water quality from three improved pastures compared with virgin brigalow (Acacia harpophylla) woodland over 8 years in semiarid Australia
Amanda Elledge
A Department of Environment and Science, PO Box 413, Rockhampton, Qld 4700, Australia.
The Rangeland Journal 44(3) 177-192 https://doi.org/10.1071/RJ22042
Submitted: 20 July 2022 Accepted: 18 November 2022 Published: 8 December 2022
© 2022 The Author(s) (or their employer(s)). Published by CSIRO Publishing on behalf of the Australian Rangeland Society. This is an open access article distributed under the Creative Commons Attribution-NonCommercial-NoDerivatives 4.0 International License (CC BY-NC-ND)
Abstract
The Fitzroy Basin in central Queensland has the largest cattle herd of any natural resource management region in Australia, and legumes have been widely used to boost fertility of rundown soil and improve cattle liveweight gains. However, there is a paucity of information on the effect of leguminous pastures on hydrology and water quality. This study investigated runoff water quality over eight hydrological years from virgin brigalow (Acacia harpophylla) woodland and three improved pastures, namely, buffel grass (Pennisetum ciliare), butterfly pea (Clitoria ternatea) and leucaena (Leucaena leucocephala). Runoff event mean concentrations and loads of total and dissolved nitrogen, phosphorus, and carbon in addition to total suspended solids are reported. Brigalow woodland had the greatest loss of sediment and nitrogen attributed to the inherently fertile Vertosols (clay soil), but the low occurrence and amount of runoff meant that it had a low risk to water quality. Despite a similar number of runoff events from the improved pastures, leucaena pasture had less total runoff and a lower maximum peak runoff rate in addition to lower nitrogen and carbon in runoff. Total suspended solids and carbon in runoff were greater from grass pasture than from the leguminous pastures, whereas nitrogen and phosphorus were greatest from the butterfly pea pasture, especially in the first 2 years post-planting. Greater exports of phosphorus from the improved pastures were concerning, given the potential for downstream impacts.
Keywords: carbon, Clitoria ternatea, forest, Leucaena leucocephala, nitrogen, Pennisetum ciliare, phosphorus, sediment.
Introduction
Declining soil fertility is a major threat to productivity of cereal cropping and grass pastures in the central Queensland region of the Brigalow Belt bioregion, Australia, because of continuous cropping, grazing or mixed farming, with minimal nutrient inputs from fertiliser or legumes (Armstrong et al. 1997; Collins and Grundy 2005). Nitrogen fixation can be achieved using either short-term (3–5 year) ley pastures, such as butterfly pea, or long-term (>25 years) permanent pastures, such as leucaena (Collins and Grundy 2005; Bowen and Chudleigh 2017).
Despite support for legume inclusion into central Queensland grazing systems (Bowen et al. 2018; Buck et al. 2019), there are no quantitative studies on the effect of leguminous pastures on runoff water quality in Australia. This is of particular concern in the Fitzroy Basin, because it is Queensland’s largest coastal catchment that drains directly into the Great Barrier Reef. Thus, data on the impact of improved pastures to runoff water quality are needed to refine models, such as those used by the Paddock to Reef Integrated Monitoring, Modelling and Reporting Program (Department of Environment and Science 2019).
This study investigated runoff water quality from improved pastures and native vegetation over eight hydrological years. The research (1) compared both butterfly pea and leucaena leguminous pastures with a grass pasture, (2) compared these improved pastures with virgin brigalow woodland representative of the landscape in its pre-European condition and (3) reported on both event mean concentrations (EMCs) and loads of total and dissolved nitrogen, phosphorus, and carbon, in addition to total suspended solids for all land uses.
Materials and methods
Site description
This study reports on a subset of catchments over a discrete period of time from the long-term Brigalow Catchment Study, which is located near Theodore in central Queensland, Australia (Fig. 1). It was established in 1965, to quantify the impact of land development for agriculture on hydrology, productivity, and resource condition (Cowie et al. 2007). The study site represents the Brigalow Belt bioregion, which covers approximately 36.7 million hectares from Townsville in northern Queensland to Dubbo in central-western New South Wales (Thornton et al. 2007). Native vegetation was dominated by brigalow (Acacia harpophylla), either in a monoculture or in association with other species, such as belah (Casuarina cristata) and Dawson River blackbutt (Eucalyptus cambageana; Johnson 2004). Slope averages 2.5% (range 1.8–3.5%), and soils are an association of Vertosols, Dermosols, and Sodosols (Cowie et al. 2007). These soil types are representative of 67% of the Fitzroy Basin under grazing, including 28% Vertosols, 28% Sodosols, and 11% Dermosols (Roots 2016). Surface-soil fertility of the study site has been described in Thornton and Shrestha (2021). The climate is semiarid to subtropical, with a mean annual hydrological year (October 1965–September 2017) rainfall of 650 mm.
![]() |
Catchment treatments
Unreplicated catchments were monitored over eight hydrological years (2010–2017) to compare rainfall, runoff, and water quality from the following four different land uses (Fig. 2):
Virgin brigalow woodland (16.8 ha) which was an uncleared control representative of the Brigalow Belt bioregion in its pre-European condition.
Buffel grass (Pennisetum ciliare ‘Biloela’) pasture (12.7 ha) that was cleared and developed from 1982 to 1983, and subsequently grazed without fertiliser or supplement feeding (Radford et al. 2007).
Butterfly pea (Clitoria ternatea cv. ‘Milgarra’) and Rhodes grass (Chloris gayana cv. ‘Callide’) pasture (11.7 ha) that was cleared and developed from 1982 to 1983, and then primarily cropped with either wheat or sorghum. A butterfly pea ley pasture was planted in 2010 to improve soil fertility, as no fertiliser had been used and fertility decline was evident to the point where it was limiting grain production (Radford et al. 2007). Rhodes grass was not planted but established from naturalised stands in waterways, along roadsides, and in adjacent paddocks.
Leucaena (Leucaena leucocephala cv. Cunningham) and buffel grass pasture (23.3 ha). On the basis of aerial photography, this catchment was cleared and developed sometime between 1965 and 1969. It had a prior history of improved pasture before cropping with either sorghum or barley between 1988 and 1991 (Thornton and Elledge 2021). This was a common management practice following clearing and initial pasture development to control brigalow regrowth (Johnson 1968). The catchment was cultivated and planted to buffel grass in 1991, and leucaena was planted into the established pasture on 8 m hedgerows in 1998.
![]() |
Photographic comparison of the catchments is provided in Fig. 3. Vertosols and Dermosols (clay soils) cover approximately 70% of the brigalow woodland and butterfly pea pasture, 58% of the grass pasture, and 82% of the leucaena pasture. Sodosols cover the remaining area of each catchment. Further details on experimental design and land-use history have been documented elsewhere (Cowie et al. 2007; Radford et al. 2007; Thornton et al. 2007; Thornton and Elledge 2021).
During this study, all three pastures were conservatively grazed. Stocking rates were based on pasture biomass and have been converted to adult equivalents (considered to be a 450 kg non-lactating animal) per hectare per year (AE/ha.year) to account for differences in cattle size and grazing duration. Grass pasture had a mean stocking rate of 0.19 AE/ha.year (range 0.13–0.26 AE/ha.year) and was destocked over the 2012 and 2013 hydrological years. Butterfly pea remained destocked until March 2011 to allow pasture establishment, and mean stocking rate was 0.12 AE/ha.year (range 0.06–0.16 AE/ha.year) from 2014 to 2016. This catchment was destocked over the 2012, 2013 and 2017 hydrological years due to low pasture biomass. Mean stocking rate in the leucaena pasture was 0.35 AE/ha.year (range 0.09–0.47 AE/ha.year), with grazing in all years.
Hydrology
Rainfall and runoff were monitored over eight hydrological years from 1 October 2010 to 30 September 2017. Rainfall was measured using a 0.5 mm tipping bucket rain gauge (Fig. 2). Each catchment was instrumented to measure runoff using a 1.2 m HL flume with a 3.9 × 6.1 m approach box. Water heights through the flume were recorded using a pressure transducer with a mechanical float-recorder backup. Stage heights were converted to discharge using a rating table (Brakenseik et al. 1979), and peak runoff rate was calculated on an event basis from instantaneous peak height. A runoff event commenced when stage height exceeded zero and finished when it returned to zero. Further details on calculating total runoff and peak runoff rates are documented elsewhere (Thornton et al. 2007; Thornton and Yu 2016).
Flow data were missed for four runoff events from the leucaena pasture, and total runoff was estimated using HowLeaky (Ghahramani 2021). Two events were estimated to have no flow (2011 and 2012) and a third only 1 mm flow (2012), but there were five and four other events with measured flows in these 2 years respectively. The fourth event missed due to equipment failure was estimated to have 47 mm of flow (2014), and there were three other events with measured flow in that year. A HowLeaky model specific to the leucaena pasture was developed based on the original grass pasture model (Thornton et al. 2007), but with a lower curve number, reduced drainage rates in the top 0.3 m of the soil profile, and a lower green cover percentage from May to December. Peak runoff rates for these four events were estimated using the multiple regression approach described by Thornton and Yu (2016), but also based on a regression model developed specifically for the leucaena pasture (Eqn 1). Peak runoff rate (Qp) was not normally distributed, so a log transformation was performed to allow statistical testing (log(Qp + 1)). Model coefficients that were statistically significant (P < 0.05), both individually and in a multiple parameter model, were log-transformed total runoff (log(Qtot + 1)), total rainfall (Ptot), storm energy, also known as rainfall kinetic energy (Etot), and peak rainfall intensity over a 4-h interval (I4h). Storm erosivity, which is a product of storm energy and peak rainfall intensity over a 30-min interval (EI30), was significantly correlated with Qp in a single- but not multiple-parameter model. Peak rainfall intensity (I) over 6-, 10-, 15-, 20- and 30-min intervals was not significantly correlated with Qp in a single-parameter model, whereas 1-, 2-, 3-, 6-, 12-, 18- and 24-h peak intensities were correlated with Qp. Antecedent rainfall (A) at 1, 2, 3, 5, 10, 20 and 30 days had no significant correlation with Qp in a single-parameter model.
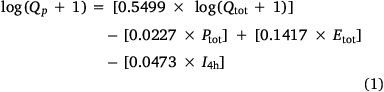

Water quality
Discrete water-quality samples were obtained using an auto-sampler located at the flume of each catchment. Auto-samplers were programmed to sample every 0.1 m change in absolute stage height. If there were insufficient water-quality samples to characterise an event from auto-samplers, samples from rising-stage samplers taken at approximately 0.1 m increments between 0.05 and 0.55 m stage height were used. Laboratory analyses of runoff samples were undertaken by Queensland Health Forensic and Scientific Services (Table 1).
![]() |
Event-based water-quality loads were calculated by dividing the hydrograph into sampling intervals, multiplying the discharge in each interval by the sample concentration, and summing the resulting loads from all intervals. The intervals were defined as the start of flow to the mid-point of Sample 1 and Sample 2, the midpoint of Sample 1 and Sample 2 to the mid-point of Sample 2 and Sample 3, and so on. Where samples were collected only on the rising limb of the hydrograph, the event peak was the end of the sampling interval for the last discrete sample, and the mean concentration of the discrete samples applied to flow from the event peak to the event end. For each pollutant within each catchment, an event mean concentration (EMC) was calculated on an event basis by dividing observed total load by observed total flow. A mean annual EMC was then calculated by averaging event-based EMCs within each year, and a site EMC was calculated by averaging the mean annual EMCs from 2010 to 2017. A comparison of four methods for calculating a site EMC is provided in the Supplementary Material file S1 for this paper (available online), which demonstrates the validity of this approach.
Where missing water-quality data occurred, load estimations were obtained by multiplying the site EMC for the catchment by the observed flow for the event. Cumulative load for each year was calculated as the sum of observed and estimated loads. Mean annual load for each catchment was then calculated by dividing the cumulative load for all years by the number of full hydrological years data (n = 8), whether runoff occurred or not.
The proportion of dissolved fractions determined the dominant pathway of pollutant losses in runoff. For example, if total dissolved phosphorus was above 60% of total phosphorus, it was transported primarily in a dissolved phase, and if it was below 40%, it was transported primarily in a particulate phase. Between 40% and 60%, it was considered to have no dominant loss pathway. This method was applied to EMCs for nitrogen, phosphorus and carbon parameters, because EMCs were based on observed data only, whereas loads were based on both observed and estimated data.
Results
Hydrology
Rainfall
This study captured the wettest (1009 mm in 2011) and driest (272 mm in 2017) years on record for the Brigalow Catchment Study, with total annual rainfall exceeding the long-term mean of 650 mm (October 1965–September 2017) in the first 5 years (Fig. 4).
![]() |
Runoff
Runoff was greatest from all catchments in the wettest year (2011), and no runoff occurred from any catchment in the driest year (2017), nor from brigalow woodland in 2012 or 2016 (Table 2). Over the eight hydrological years, runoff was greatest from grass and butterfly pea pastures and smallest from brigalow woodland (Fig. 5, Table 2). Brigalow woodland had less than half the runoff events of the improved pastures.
![]() |
![]() |
In 2012, rainfall exceeded the 80th percentile of long-term records, but there was no runoff from brigalow woodland and only small runoff events from the pasture catchments (Fig. 4, Table 2). Furthermore, despite minimal difference in total annual rainfall between 2013 and 2014, total annual runoff from 2013 was 2.6 times greater than that in 2014 from leucaena pasture, 2.1 times greater from grass pasture, 1.8 times greater from butterfly pea pasture, and 1.6 times greater from brigalow woodland.
In contrast to mean annual runoff, the average and maximum peak runoff rates from brigalow woodland were similar to grass pasture (Table 3). Butterfly pea and leucaena pastures had, on average, 79% of the average peak runoff rate of grass pasture. However, despite a similar average peak runoff rate from both leguminous pastures, maximum peak runoff rate from butterfly pea was greater than from leucaena.
![]() |
Water quality
Total suspended solids
EMCs of total suspended solids were greater from brigalow woodland than from improved pastures (Table 4). Similarly, cumulative load was greatest from brigalow woodland and smallest from leguminous pastures (Fig. 6). Mean annual load of total suspended solids was, on average, 1.3 times greater from brigalow woodland than from the improved pastures (Table 5).
![]() |
![]() |
![]() |
There were 13 events where runoff occurred from both brigalow woodland and grass pasture. Total runoff from grass pasture (593 mm) was double that from brigalow woodland (290 mm), whereas it had only 50% of the mean EMC of total suspended solids (202 and 409 mg/L respectively). This resulted in similar mean loads (103 and 100 kg/ha respectively).
Nitrogen
EMCs of both total and dissolved nitrogen fractions were greater from brigalow woodland than from improved pastures (Table 4). Similarly, cumulative loads of total nitrogen and total dissolved nitrogen were greatest from brigalow woodland and smallest from leucaena pasture (Fig. 7). Mean annual load of total nitrogen was 1.5 times greater from brigalow woodland than from improved pastures, whereas total dissolved nitrogen from brigalow woodland was 1.4 times greater than that from grass and butterfly pea pastures and 2.7 times greater than that from leucaena pasture (Table 5). Dissolved inorganic nitrogen showed a different trend, with loads being equally highest from brigalow woodland and butterfly pea pasture, which were, on average, 2.9 times greater than were the loads from grass and leucaena pastures.
![]() |
Dissolved fractions dominated total nitrogen loss from brigalow woodland, grass pasture and butterfly pea pasture, whereas leucaena pasture had substantial contributions of both dissolved and particulate fractions. There was no clear loss pathway for dissolved nitrogen fractions from brigalow woodland or leucaena pasture. However, dissolved fractions from butterfly pea pasture were primarily lost in an inorganic form and conversely from grass pasture in an organic form. Dissolved inorganic nitrogen primarily comprised oxidised nitrogen from all catchments (mean 93%; range 85–97%).
Phosphorus
In contrast to total suspended solids and nitrogen, EMCs of total and dissolved phosphorus fractions were greatest from butterfly pea pasture (Table 4). Butterfly pea pasture also had the greatest cumulative loads, whereas brigalow woodland had the smallest (Fig. 8). Mean annual loads of all phosphorus parameters from butterfly pea pasture were double those from grass and leucaena pastures (Table 5). Butterfly pea pasture had approximately twice the total phosphorus load of brigalow woodland, but considerably greater loads of both total dissolved phosphorus and dissolved inorganic phosphorus.
![]() |
Dissolved fractions dominated total phosphorus loss from improved pastures, whereas particulate fractions were dominant from brigalow woodland. Dissolved phosphorus fractions from all catchments were primarily lost in an inorganic form (mean 81%; range 74–87%).
Carbon
EMCs of both total and dissolved carbon fractions were greatest from brigalow woodland (Table 4). In contrast, cumulative loads of all carbon parameters were greatest from grass pasture and smallest from leucaena pasture (Fig. 9). Although the mean annual load of total carbon from brigalow woodland was only 90% of that from grass pasture, total organic carbon and dissolved organic carbon were similar (Table 5). Mean annual load of total and organic carbon fractions were 1.6 times greater from grass than from leguminous pastures. The dominant pathway of total carbon loss from all catchments was in an organic form (mean 68%; range 60–78%), which was dominated by dissolved fractions (mean 74%; range 68–86%).
![]() |
Discussion
Hydrology
This study captured the wettest and driest years on record for the Brigalow Catchment Study, which indicates that it provided the minimum and maximum boundaries for flow and water quality. Global warming is forecast to result in larger rainfall events separated by longer dry periods (Knapp et al. 2008), which highlights the importance of long-term studies. Improved pastures had more than double the number of runoff events and, on average, 1.5 times more total runoff than did brigalow woodland. This supports earlier data from the long-term study, which found that clearing brigalow woodland for grazing doubled total runoff and peak runoff rates (Thornton et al. 2007; Thornton and Yu 2016), and other international literature reporting greater total runoff and peak runoff rates from pasture than forest (Ogden et al. 2013).
Greater runoff from improved pastures compared with brigalow woodland can be partly explained by grazing. That is, cattle trampling reduces ground cover and compacts soil, which decreases infiltration rates and moisture-holding capacity, and subsequently increases runoff (Trimble and Mendel 1995). Furthermore, brigalow trees have been found to maintain a dry soil profile up to 7.2 m depth, despite a potential plant-available water capacity of about 640 mm between 1.2 and 7.2 m (Silburn et al. 2009). This soil water deficit, combined with the ability of brigalow to rapidly utilise available soil water, is likely to be a key driver of the lower frequency and magnitude of runoff compared with improved pastures.
Leucaena pasture has root depths and water use similar to those of native woodlands (Shelton and Dalzell 2007), and has been used in agroforestry systems to reduce runoff and erosion (Narain et al. 1997). This study found a similar trend, and despite an average peak runoff rate similar to that of butterfly pea, leucaena had only 59% of the maximum peak runoff rate. Several reasons explain these fewer intense runoff events. First, a reduction in runoff under leucaena has been attributed to increased canopy interception of rainfall (Narain et al. 1998). Second, leucaena can produce more roots when planted with grass than as a monoculture (Liu et al. 2018). Last, Shelton and Dalzell (2007) reported leucaena roots at depths of 5–6 m, compared with only 2 m for buffel grass, suggesting greater water utilisation.
Another explanation for the greater total runoff and maximum peak runoff rate from butterfly pea pasture than from leucaena pasture was that leucaena was planted into an established pasture in 1998, whereas butterfly pea was planted only at the start of this study in 2010. Fine-root biomass of leucaena increases with age and there could be a steady state in mature stands after an initial rapid increase (Guo et al. 2012). Thus, hydrological differences may be partly biased by time since soil disturbance at planting and pasture age, which affects both above- and below-ground biomass.
In contrast, total runoff and maximum peak runoff rate from the grass and butterfly pea pastures were similar, despite the grass pasture being planted in 1982. This suggests that there should have been greater root biomass under the grass pasture, resulting in greater soil water use and less runoff. However, this outcome was not observed, indicating that age as a surrogate for root biomass and soil water use may be an oversimplification. The ability of each pasture species to extract soil water at the lower limit of plant-available water capacity is also a driver of soil profile hydrology. Limited soil moisture monitoring at the study site indicated that butterfly pea pasture consistently maintained a drier soil profile than did both brigalow woodland and grass pasture (data not presented). Thus, additional infiltration would have been required under butterfly pea pasture for saturation excess runoff, irrespective of root biomass (Dunne and Black 1970). A dry soil profile suggests an abundance of macropores that would have increased the initial abstraction prior to runoff, via either infiltration excess or saturation excess processes (Allen et al. 2005; Ringrose-Voase and Nadelko 2013). Given the low soil permeability and the lack of a permanent shallow standing-water table (Silburn et al. 2009), increased initial abstraction prior to infiltration excess runoff is more likely to be the key driver of soil water dynamics under butterfly pea pasture. Other studies across northern Australia have also observed infiltration excess runoff (Bonell and Williams 1987; Koci et al. 2020).
An exception to the belief that more rainfall leads to more runoff was that no runoff was recorded from brigalow woodland and only small runoff events occurred from the improved pastures in 2012, despite rainfall that exceeded the 80th percentile of long-term records. This may be explained by the effect of prior-year rainfall on current-year above-ground net primary production (Oesterheld et al. 2001; Sims and Colloff 2012; Dudney et al. 2017; Gong et al. 2020). This above-average rainfall in 2010 and 2011 would likely have increased pasture biomass in 2012 (Dudney et al. 2017), resulting in a greater use of available water and less runoff (Thornton and Elledge 2013). Similarly, despite minimal difference in total annual rainfall between 2013 and 2014, total annual runoff was greater from all catchments in 2013. This is probably due to the same phenomenon, but to a lesser extent due to less extreme rainfall in the preceding 2 years. That is, above-ground net primary production fluctuations are buffered if more productive years alternate with less productive years, and are amplified if extended wet or dry sequences occur (Oesterheld et al. 2001).
Water quality
Total suspended solids
Mean annual EMC of total suspended solids from the grass pasture was within the range reported for runoff water quality from Australian grazing studies on both improved and native pastures dominated (>90%) by a single land use (Bartley et al. 2012). In that review, Bartley et al. (2012) found that EMCs of total suspended solids were lower from forests than from improved pasture, and those from both of these land uses were lower than from native pastures. In contrast, the site EMC of total suspended solids from brigalow woodland in this study was at least double that from the improved pastures, and it also had the greatest load of total suspended solids, despite having only 67% (range 63–73%) of the total runoff.
When runoff occurred from both brigalow woodland and grass pasture (13 events), grass pasture had double the total runoff, but 50% of the total suspended solids EMC, resulting in similar mean loads. The varying magnitude and direction of pollutant concentrations and loads in runoff can be attributed to dilution. This phenomenon explains how larger runoff events, whether from above-average rainfall or a treatment effect, result in lower EMCs, whereas smaller runoff events result in higher EMCs (El-Sadek et al. 2005; Thornton and Elledge 2019). These observations explain the low total runoff and high EMC of total suspended solids reported from brigalow woodland.
The improved pastures exported similar EMCs and loads of total suspended solids, although values were overall greater from grass pasture. This can be attributed to the conservative grazing strategy used to maintain above 80% ground cover and 1000 kg/ha of available pasture (Thornton et al. 2007; Thornton and Elledge 2013). One exception to high ground cover in this study was when the long-term cropping catchment was converted to a grazed ley pasture of butterfly pea. A substantial increase in the cumulative load of total suspended solids was observed during establishment (Fig. 6), notably over the first 2 years post-planting.
Nitrogen
Mean annual EMC of total nitrogen from the grass pasture was within the range reported for improved and native pastures dominated (>90%) by a single land use (Bartley et al. 2012). Conversely, the EMC for dissolved inorganic nitrogen was within the range for improved pastures, but greatly exceeded that for native pastures. This can be partly explained by a more relaxed criteria for the inclusion of studies that reported dissolved inorganic nitrogen.
Mean annual EMCs of total and dissolved inorganic nitrogen from brigalow woodland also greatly exceeded that for forest in the review by Bartley et al. (2012). This reflects the inherently high soil fertility of brigalow lands compared with the rangeland, savanna and woodland landscapes from which comparable data were available. Furthermore, modelling of long-term water-quality data indicated that brigalow woodland had greater concentrations and loads of nitrogen (total and dissolved) than did conservatively grazed pasture (Elledge and Thornton 2017). This contrasts with studies elsewhere that noted higher loads from pasture than forest (Quinn and Stroud 2002; Vink et al. 2007; Udawatta et al. 2011).
Elledge and Thornton (2017) found that dissolved inorganic nitrogen accounted for only 5% of total nitrogen from grass pasture, and assumed that the majority was exported in a particulate phase. However, runoff samples were not analysed for total dissolved nitrogen until 2012 and data from this study accounted for 65% of total nitrogen, indicating that particulate nitrogen from the grass pasture contributed much less than was originally assumed. Furthermore, dissolved inorganic nitrogen accounted only for 37% of total dissolved nitrogen from grass pasture. In contrast, the legume species (including brigalow woodland) had higher contributions of dissolved inorganic nitrogen, accounting for 52% (range 48–74%) of total dissolved nitrogen. Packett (2017) found that rainfall contributed to 37% of dissolved inorganic nitrogen loads in Fitzroy Basin rivers, suggesting that the small export from grass pasture could be from rainfall rather than cattle. Whereas, the greater dissolved inorganic nitrogen from catchments with leguminous trees and grasses can be attributed to nitrogen fixation.
There was a particularly high load of dissolved inorganic nitrogen from butterfly pea pasture in December 2010, which can be attributed to fallow management and tillage prior to planting, which would have increased soil nitrogen mineralisation. However, the newly planted butterfly pea pasture was potentially insufficiently established to utilise the mineral nitrogen, which was then subject to loss via runoff or as leachate (Sigua et al. 2010). In contrast, the lower loads of dissolved inorganic nitrogen from the grass and leucaena pastures may be explained by pasture rundown, with mineral nitrogen being immobilised, rather than a net loss from the system (Robertson et al. 1997; Lawrence et al. 2014; Conrad et al. 2018).
Phosphorus
Mean annual EMC of total phosphorus from the grass pasture was within the range reported for both improved and native pastures within Australia, whereas the EMC for dissolved inorganic phosphorus was within the range for improved pastures, but greatly exceeded that for native pastures (Bartley et al. 2012). Similar to nitrogen, these result reflect the inherently high soil fertility of brigalow lands. This is supported by bicarbonate-extractable phosphorus data from surface soil (0–10 cm) in the grass pasture, which was at a moderate level before land development and had stabilised to similar levels about 15 years following clearing and development (Thornton and Shrestha 2021). This is in contrast to the low, deficient (very low) and acute (extremely low) status given to 72% of central and north-eastern Queensland grazing lands (Ahern et al. 1994) and the deficient and acute status given to 68% of northern Australian soils (McCosker and Winks 1994). Given that plant-available (dissolved) phosphorus in the soil surface is related to concentrations of phosphorus in runoff (Sharpley 1995; Haan et al. 2006; McDowell et al. 2011), greater losses of total and dissolved phosphorus from improved pastures is of concern because of the possibility of downstream eutrophication (Bol et al. 2018).
Butterfly pea pasture had the greatest EMCs and loads for both total and dissolved phosphorus fractions in runoff, being particularly evident in the 2 years post-planting. Despite low ground cover, 84% of total phosphorus was lost as total dissolved phosphorus. Total and dissolved phosphorus from the grass and leucaena pastures were similar. The lower EMCs and loads of these pastures compared with butterfly pea may be attributed to their establishment before this study and their observed greater basal plant cover. At least 90% cover has been recommended to manage phosphorus loss (Hart and Cornish 2012).
Carbon
Carbon in runoff from the long-term study was analysed only from 2010, and a paucity of literature on carbon loss through erosion and runoff was highlighted by Nachimuthu and Hulugalle (2016). Nonetheless, a comparison of EMCs from grass pasture with other grazing trials indicated that the results were within a similar range, typically being below 40 mg/L for total organic carbon (Schepers and Francis 1982; Nelson et al. 1996; Endale et al. 2011) and below 25 mg/L for dissolved organic carbon (Nelson et al. 1996; Dhillon and Inamdar 2013).
Surface-soil sampling (0–10 cm) in 2014 and 2018 provided mean soil organic carbon values of 1.59% from grass pasture and 1.12% from butterfly pea pasture (Thornton and Shrestha 2021; The State of Queensland 2022). Similarly, runoff from butterfly pea pasture had 69% of the total suspended solids concentration and 70% of the total organic carbon concentration compared with grass pasture. This supports a relationship between concentrations of carbon in soil, sediment in runoff and carbon in runoff (Doetterl et al. 2016). However, less carbon exported in runoff from butterfly pea pasture than from grass pasture can be attributed to land-use and management history rather than pasture type. Butterfly pea was planted as a grazed ley pasture in 2010 to arrest soil fertility rundown (Thornton and Shrestha 2021). Low cover during establishment resulted in 86% of the total suspended solid load and 59% of the total organic carbon load exported in runoff during the first 2 years when rainfall was some of the highest on record. This suggests that the increased loads of pollutants in runoff were associated with both increased erosion and higher erosive forces (Nadeu et al. 2011; Martínez-Mena et al. 2012; Stacy et al. 2015; Koiter et al. 2017). In comparison, brigalow woodland and the two established pastures had, on average, 54% of the total suspended solid load and 48% of the total organic carbon load in these 2 years.
Data from 2014–2018 surface-soil samplings also found similar mean soil organic carbon values from the grass (1.59%) and leucaena pastures (1.39%; Thornton and Shrestha 2021; The State of Queensland 2022). Although leucaena had 87% of the soil organic carbon and 80% of the total suspended solids concentration in runoff, it had only 54% of the total organic carbon concentration. The longer grazing history plus cropping prior to the planting of leucaena is likely to explain the lower soil and runoff organic carbon concentrations.
Conclusions
Within the Fitzroy Basin, leucaena and butterfly pea pastures were reported to be more productive and profitable than were perennial grass pastures such as buffel grass (Bowen et al. 2018). However, environmental outcomes are rarely incorporated into such assessments because of the difficulty of quantifying their value (Dasgupta 2008; Moravek et al. 2017). This is of concern, given the extensive area of the Fitzroy Basin that has undergone land-clearing and land-use change. Improved pastures had, on average, 1.5 times more total runoff and more than double the number of runoff events compared with brigalow woodland. Although brigalow woodland had the greatest EMCs and loads of total suspended solids and nitrogen in runoff, the low occurrence of runoff from this land use means that it has an overall low risk to water quality. The established leucaena pasture also had a low risk to water quality, whereas butterfly pea pasture had the highest exports of nitrogen and phosphorus, particularly during the first 2 years post-planting. Risk to runoff water quality from improved pastures was reduced by good pasture management. However, greater exports of total and dissolved fractions of phosphorus from improved pastures compared with brigalow woodland were identified as a concern due to the possibility of downstream eutrophication.
Supplementary material
Supplementary material is available online.
Data availability
Data that support this study will be shared upon reasonable request to the corresponding author.
Conflicts of interest
The authors declare no conflicts of interest.
Declaration of funding
This research was jointly funded by the Queensland Department of Resources (formerly the Department of Natural Resources, Mines and Energy) and the Reef Rescue Research and Development Program (Project RRRD009) of the Australian Government’s Caring for our Country initiative.
Acknowledgements
The authors thank past and present staff from multiple Queensland Government agencies that have contributed to the long-term Brigalow Catchment Study. We are also grateful to our industry collaborator Elrose Brahman Stud, and, in particular, Walter and Leicha Gleeson from Brigalow Station for their assistance with on-ground cattle operations. Comments on an earlier draft were provided by David Freebairn (formerly of the Department of Environment and Resource Management) and Daren Harmel (United States Department of Agriculture, Agricultural Research Service).
References
Ahern CR, Shields PG, Enderlin NG, Baker DE (1994) ‘The soil fertility of central and north-east Queensland grazing lands.’ (Department of Primary Industries: Qld, Australia)Allen, PM, Harmel, RD, Arnold, J, Plant, B, Yelderman, J, and King, K (2005). Field data and flow system response in clay (vertisol) shale terrain, north central Texas, USA. Hydrological Processes 19, 2719–2736.
| Field data and flow system response in clay (vertisol) shale terrain, north central Texas, USA.Crossref | GoogleScholarGoogle Scholar |
Armstrong, RD, Walsh, K, McCosker, KJ, Millar, GR, Probert, ME, and Johnson, S (1997). Improved nitrogen supply to cereals in central Queensland following short legume leys. Australian Journal of Experimental Agriculture 37, 359–368.
| Improved nitrogen supply to cereals in central Queensland following short legume leys.Crossref | GoogleScholarGoogle Scholar |
Bartley, R, Speirs, WJ, Ellis, TW, and Waters, DK (2012). A review of sediment and nutrient concentration data from Australia for use in catchment water quality models. Marine Pollution Bulletin 65, 101–116.
| A review of sediment and nutrient concentration data from Australia for use in catchment water quality models.Crossref | GoogleScholarGoogle Scholar |
Bol, R, Gruau, G, Mellander, P-E, Dupas, R, Bechmann, M, Skarbøvik, E, Bieroza, M, Djodjic, F, Glendell, M, Jordan, P, Van der Grift, B, Rode, M, Smolders, E, Verbeeck, M, Gu, S, Klumpp, E, Pohle, I, Fresne, M, and Gascuel-Odoux, C (2018). Challenges of reducing phosphorus based water eutrophication in the agricultural landscapes of northwest Europe. Frontiers in Marine Science 5, 276.
| Challenges of reducing phosphorus based water eutrophication in the agricultural landscapes of northwest Europe.Crossref | GoogleScholarGoogle Scholar |
Bonell M, Williams J (1987) Infiltration and redistribution of overland flow and sediment on a low relief landscape of semi-arid, tropical Queensland. In ‘Forest Hydrology and Watershed Management. Publication No. 167’. (Eds RH Swanson, PY Bernier, PD Woodward) pp. 199–211. (International Association of Hydrological Sciences (IAHS): Vancouver, Canada)
Bowen MK, Chudleigh F (2017) ‘Productivity and profitability of a range of alternative steer growth paths resulting from manipulating the pasture feed base in central Queensland: a modelling approach.’ (Department of Agriculture and Fisheries: Qld, Australia)
Bowen, MK, Chudleigh, F, Buck, S, and Hopkins, K (2018). Productivity and profitability of forage options for beef production in the subtropics of northern Australia. Animal Production Science 58, 332–342.
| Productivity and profitability of forage options for beef production in the subtropics of northern Australia.Crossref | GoogleScholarGoogle Scholar |
Brakenseik DL, Osborn HB, Rawls WJ (1979) ‘Field manual for research in agricultural hydrology. Agriculture handbook No. 224.’ pp. 550. (United States Department of Agriculture: Washington, USA)
Buck, S, Rolfe, J, Lemin, C, and English, B (2019). Adoption, profitability and future of leucaena feeding systems in Australia. Tropical Grasslands-Forrajes Tropicales 7, 303–314.
| Adoption, profitability and future of leucaena feeding systems in Australia.Crossref | GoogleScholarGoogle Scholar |
Collins R, Grundy T (2005) ‘The butterfly pea book: a guide to establishing and managing butterfly pea pastures in central Queensland.’ (Department of Primary Industries and Fisheries: Brisbane, Qld, Australia)
Conrad, KA, Dalal, RC, Allen, DE, Fujinuma, R, and Menzies, NW (2018). Free light fraction carbon and nitrogen, a physically uncomplexed soil organic matter distribution within subtropical grass and leucaena–grass pastures. Soil Research 56, 820–828.
| Free light fraction carbon and nitrogen, a physically uncomplexed soil organic matter distribution within subtropical grass and leucaena–grass pastures.Crossref | GoogleScholarGoogle Scholar |
Cowie, BA, Thornton, CM, and Radford, BJ (2007). The Brigalow Catchment Study: I. Overview of a 40-year study of the effects of land clearing in the brigalow bioregion of Australia. Australian Journal of Soil Research 45, 479–495.
| The Brigalow Catchment Study: I. Overview of a 40-year study of the effects of land clearing in the brigalow bioregion of Australia.Crossref | GoogleScholarGoogle Scholar |
Dasgupta, P (2008). Creative accounting. Nature 456, 44.
| Creative accounting.Crossref | GoogleScholarGoogle Scholar |
Department of Environment and Science (2019) Reef 2050 water quality improvement plan: Fitzroy. Available at www.reefplan.qld.gov.au/reef-regions/fitzroy [accessed 4 November 2020]
Dhillon, GS, and Inamdar, S (2013). Extreme storms and changes in particulate and dissolved organic carbon in runoff: entering uncharted waters? Geophysical Research Letters 40, 1322–1327.
| Extreme storms and changes in particulate and dissolved organic carbon in runoff: entering uncharted waters?Crossref | GoogleScholarGoogle Scholar |
Doetterl, S, Berhe, AA, Nadeu, E, Wang, Z, Sommer, M, and Fiener, P (2016). Erosion, deposition and soil carbon: A review of process-level controls, experimental tools and models to address C cycling in dynamic landscapes. Earth-Science Reviews 154, 102–122.
| Erosion, deposition and soil carbon: A review of process-level controls, experimental tools and models to address C cycling in dynamic landscapes.Crossref | GoogleScholarGoogle Scholar |
Dudney, J, Hallett, LM, Larios, L, Farrer, EC, Spotswood, EN, Stein, C, and Suding, KN (2017). Lagging behind: Have we overlooked previous-year rainfall effects in annual grasslands? Journal of Ecology 105, 484–495.
| Lagging behind: Have we overlooked previous-year rainfall effects in annual grasslands?Crossref | GoogleScholarGoogle Scholar |
Dunne, T, and Black, RD (1970). Partial area contributions to storm runoff in a small New England watershed. Water Resources Research 6, 1296–1311.
| Partial area contributions to storm runoff in a small New England watershed.Crossref | GoogleScholarGoogle Scholar |
El-Sadek A, Radwan M, Abdel-Gawad S (2005) Analysis of load versus concentration as water quality measures. In ‘Proceedings of the 9th International Water Technology Conference’, Egypt. pp. 1281–1292. (International Water Technology Association)
Elledge, A, and Thornton, C (2017). Effect of changing land use from virgin brigalow (Acacia harpophylla) woodland to a crop or pasture system on sediment, nitrogen and phosphorus in runoff over 25 years in subtropical Australia. Agriculture, Ecosystems & Environment 239, 119–131.
| Effect of changing land use from virgin brigalow (Acacia harpophylla) woodland to a crop or pasture system on sediment, nitrogen and phosphorus in runoff over 25 years in subtropical Australia.Crossref | GoogleScholarGoogle Scholar |
Endale, DM, Fisher, DS, Owens, LB, Jenkins, MB, Schomberg, HH, Tebes-Stevens, CL, and Bonta, JV (2011). Runoff water quality during drought in a zero-order Georgia Piedmont pasture: nitrogen and total organic carbon. Journal of Environmental Quality 40, 969–979.
| Runoff water quality during drought in a zero-order Georgia Piedmont pasture: nitrogen and total organic carbon.Crossref | GoogleScholarGoogle Scholar |
Ghahramani A (2021) HowLeaky: Open source water modelling environment to investigate impacts on a range of land uses, soils, management practices and climates. Available at https://howleaky.com/ [accessed 12 August 2021]
Gong, YH, Zhao, DM, Ke, WB, Fang, C, Pei, JY, Sun, GJ, and Ye, JS (2020). Legacy effects of precipitation amount and frequency on the aboveground plant biomass of a semi-arid grassland. Science of the Total Environment 705, 135899.
| Legacy effects of precipitation amount and frequency on the aboveground plant biomass of a semi-arid grassland.Crossref | GoogleScholarGoogle Scholar |
Guo, T, He, B, Jiang, X, Ma, Y, Yong, W, Xiang, M, Chen, Y, and Tang, C (2012). Effect of Leucaena leucocephala on soil organic carbon conservation on slope in the purple soil area. Acta Ecologica Sinica 32, 190–197.
| Effect of Leucaena leucocephala on soil organic carbon conservation on slope in the purple soil area.Crossref | GoogleScholarGoogle Scholar |
Haan, MM, Russell, JR, Powers, WJ, Kovar, JL, and Benning, JL (2006). Grazing management effects on sediment and phosphorus in surface runoff. Rangeland Ecology & Management 59, 607–615.
| Grazing management effects on sediment and phosphorus in surface runoff.Crossref | GoogleScholarGoogle Scholar |
Hart, MR, and Cornish, PS (2012). Available soil phosphorus, phosphorus buffering and soil cover determine most variation in phosphorus concentration in runoff from pastoral sites. Nutrient Cycling in Agroecosystems 93, 227–244.
| Available soil phosphorus, phosphorus buffering and soil cover determine most variation in phosphorus concentration in runoff from pastoral sites.Crossref | GoogleScholarGoogle Scholar |
Johnson, RW (1968). Brigalow clearing and the control of regrowth. Tropical Grasslands 2, 115–118.
Johnson RW (2004) Vegetation survey of the Brigalow Research Station, Theodore, Queensland. In ‘Proceedings of the Royal Society of Queensland’. pp. 39–61. (The Royal Society of Queensland: Brisbane)
Knapp, AK, Beier, C, Briske, DD, Classen, AT, Luo, Y, Reichstein, M, Smith, MD, Smith, SD, Bell, JE, Fay, PA, Heisler, JL, Leavitt, SW, Sherry, R, Smith, B, and Weng, E (2008). Consequences of more extreme precipitation regimes for terrestrial ecosystems. BioScience 58, 811–821.
| Consequences of more extreme precipitation regimes for terrestrial ecosystems.Crossref | GoogleScholarGoogle Scholar |
Koci, J, Sidle, RC, Kinsey-Henderson, AE, Bartley, R, Wilkinson, SN, Hawdon, AA, Jarihani, B, Roth, CH, and Hogarth, L (2020). Effect of reduced grazing pressure on sediment and nutrient yields in savanna rangeland streams draining to the Great Barrier Reef. Journal of Hydrology 582, 124520.
| Effect of reduced grazing pressure on sediment and nutrient yields in savanna rangeland streams draining to the Great Barrier Reef.Crossref | GoogleScholarGoogle Scholar |
Koiter, AJ, Owens, PN, Petticrew, EL, and Lobb, DA (2017). The role of soil surface properties on the particle size and carbon selectivity of interrill erosion in agricultural landscapes. Catena 153, 194–206.
| The role of soil surface properties on the particle size and carbon selectivity of interrill erosion in agricultural landscapes.Crossref | GoogleScholarGoogle Scholar |
Lawrence D, Buck S, Johnson B, Peck G (2014) Fertilising for yield and quality in sown grass pastures and forage crops: final report (Project B.NBP.0768). Meat and Livestock Australia, Sydney, NSW, Australia.
Liu, F, Gao, C, Chen, M, and Li, K (2018). Above- and below-ground biomass relationships of Leucaena leucocephala (Lam.) de Wit in different plant stands. PLoS One 13, e0207059.
| Above- and below-ground biomass relationships of Leucaena leucocephala (Lam.) de Wit in different plant stands.Crossref | GoogleScholarGoogle Scholar |
Martínez-Mena, M, López, J, Almagro, M, Albaladejo, J, Castillo, V, Ortiz, R, and Boix-Fayos, C (2012). Organic carbon enrichment in sediments: Effects of rainfall characteristics under different land uses in a Mediterranean area. CATENA 94, 36–42.
| Organic carbon enrichment in sediments: Effects of rainfall characteristics under different land uses in a Mediterranean area.Crossref | GoogleScholarGoogle Scholar |
McCosker T, Winks L (1994) Phosphorus nutrition of beef cattle in northern Australia (Report QI94012). Department of Primary industries, Brisbane, Qld, Australia.
McDowell, RW, Sharpley, AN, Crush, JR, and Simmons, T (2011). Phosphorus in pasture plants: potential implications for phosphorus loss in surface runoff. Plant and Soil 345, 23–35.
| Phosphorus in pasture plants: potential implications for phosphorus loss in surface runoff.Crossref | GoogleScholarGoogle Scholar |
Moravek T, Schrobback P, East M, Star M, Rust S (2017) Understanding the economics of grazing management practices and systems for improving water quality run-off from grazing lands in the Burdekin and Fitzroy Catchments. Reef Plan Action 4: Gap Analysis Report 2016. Department of Agriculture and Fisheries, Queensland, Australia.
Nachimuthu, G, and Hulugalle, N (2016). On-farm gains and losses of soil organic carbon in terrestrial hydrological pathways: a review of empirical research. International Soil and Water Conservation Research 4, 245–259.
| On-farm gains and losses of soil organic carbon in terrestrial hydrological pathways: a review of empirical research.Crossref | GoogleScholarGoogle Scholar |
Nadeu, E, de Vente, J, Martínez-Mena, M, and Boix-Fayos, C (2011). Exploring particle size distribution and organic carbon pools mobilized by different erosion processes at the catchment scale. Journal of Soils and Sediments 11, 667–678.
| Exploring particle size distribution and organic carbon pools mobilized by different erosion processes at the catchment scale.Crossref | GoogleScholarGoogle Scholar |
Narain, P, Singh, RK, Sindhwal, NS, and Joshie, P (1997). Agroforestry for soil and water conservation in the western Himalayan Valley Region of India 1. Runoff, soil and nutrient losses. Agroforestry Systems 39, 175–189.
| Agroforestry for soil and water conservation in the western Himalayan Valley Region of India 1. Runoff, soil and nutrient losses.Crossref | GoogleScholarGoogle Scholar |
Narain, P, Singh, RK, Sindhwal, NS, and Joshie, P (1998). Water balance and water use efficiency of different land uses in western Himalayan valley region. Agricultural Water Management 37, 225–240.
| Water balance and water use efficiency of different land uses in western Himalayan valley region.Crossref | GoogleScholarGoogle Scholar |
Nelson, PN, Cotsaris, E, and Oades, JM (1996). Nitrogen, phosphorus, and organic carbon in streams draining two grazed catchments. Journal of Environmental Quality 25, 1221–1229.
| Nitrogen, phosphorus, and organic carbon in streams draining two grazed catchments.Crossref | GoogleScholarGoogle Scholar |
Oesterheld, M, Loreti, J, Semmartin, M, and Sala, OE (2001). Inter-annual variation in primary production of a semi-arid grassland related to previous-year production. Journal of Vegetation Science 12, 137–142.
| Inter-annual variation in primary production of a semi-arid grassland related to previous-year production.Crossref | GoogleScholarGoogle Scholar |
Ogden, FL, Crouch, TD, Stallard, RF, and Hall, JS (2013). Effect of land cover and use on dry season river runoff, runoff efficiency, and peak storm runoff in the seasonal tropics of central Panama. Water Resources Research 49, 8443–8462.
| Effect of land cover and use on dry season river runoff, runoff efficiency, and peak storm runoff in the seasonal tropics of central Panama.Crossref | GoogleScholarGoogle Scholar |
Packett, R (2017). Rainfall contributes ~30% of the dissolved inorganic nitrogen exported from a southern Great Barrier Reef river basin. Marine Pollution Bulletin 121, 16–31.
| Rainfall contributes ~30% of the dissolved inorganic nitrogen exported from a southern Great Barrier Reef river basin.Crossref | GoogleScholarGoogle Scholar |
Quinn, JM, and Stroud, MJ (2002). Water quality and sediment and nutrient export from New Zealand hill‐land catchments of contrasting land use. New Zealand Journal of Marine and Freshwater Research 36, 409–429.
| Water quality and sediment and nutrient export from New Zealand hill‐land catchments of contrasting land use.Crossref | GoogleScholarGoogle Scholar |
Radford, BJ, Thornton, CM, Cowie, BA, and Stephens, ML (2007). The Brigalow Catchment Study: III. Productivity changes on brigalow land cleared for long-term cropping and for grazing. Australian Journal of Soil Research 45, 512–523.
| The Brigalow Catchment Study: III. Productivity changes on brigalow land cleared for long-term cropping and for grazing.Crossref | GoogleScholarGoogle Scholar |
Ringrose-Voase, AJ, and Nadelko, AJ (2013). Deep drainage in a Grey Vertosol under furrow-irrigated cotton. Crop & Pasture Science 64, 1155–1170.
| Deep drainage in a Grey Vertosol under furrow-irrigated cotton.Crossref | GoogleScholarGoogle Scholar |
Robertson, FA, Myers, RJK, and Saffigna, PG (1997). Nitrogen cycling in brigalow clay soils under pasture and cropping. Australian Journal of Soil Research 35, 1323–1339.
| Nitrogen cycling in brigalow clay soils under pasture and cropping.Crossref | GoogleScholarGoogle Scholar |
Roots K (2016) ‘Land area under various soil orders extracted for grazing in the Fitzroy Basin using layers sourced from the Queensland Government’s Spatial Information Resource (SIR) database: ‘SLR.ASRIS_ASR_L4_2M_RESULTS_V’ Australian Soil Resource Information System (ASRIS) Level 4 (1:2,000,000 scale) Australian Soil Classifications replaced with sections of ‘SLR.ASRIS_ASR_L5_250K_RESULTS_V’ Level 5 (1:250,000 scale) where available; ‘RSC.QLD_LANDUSE_CURRENT_X’ land use map which is a product of the ‘Queensland Land Use Mapping Program (QLUMP)’; and ‘P2R_56_sub_basins’ shapefile provided by C. Dougall (Paddock to Reef modeller, Department of Natural Resources and Mines) dissolved to the Fitzroy Basin using ‘PROP.QLD_NRMREGBDY_100K’ natural resource management boundaries. Developed using ArcGIS version 10.3.’ (Department of Natural Resources and Mines: Rockhampton, Qld, Australia)
Schepers, JS, and Francis, DD (1982). Chemical water quality of runoff from grazing land in Nebraska: I. Influence of grazing livestock. Journal of Environmental Quality 11, 351–354.
| Chemical water quality of runoff from grazing land in Nebraska: I. Influence of grazing livestock.Crossref | GoogleScholarGoogle Scholar |
Sharpley A (1995) Fate and transport of nutrients: phosphorus. Working paper no. 8. Available at www.nrcs.usda.gov [accessed 2 June 2020]
Shelton, M, and Dalzell, S (2007). Production, economic and environmental benefits of leucaena pastures. Tropical Grasslands 41, 174–190.
Sigua, GC, Hubbard, RK, Coleman, SW, and Williams, M (2010). Nitrogen in soils, plants, surface water and shallow groundwater in a bahiagrass pasture of Southern Florida, USA. Nutrient Cycling in Agroecosystems 86, 175–187.
| Nitrogen in soils, plants, surface water and shallow groundwater in a bahiagrass pasture of Southern Florida, USA.Crossref | GoogleScholarGoogle Scholar |
Silburn, DM, Cowie, BA, and Thornton, CM (2009). The Brigalow Catchment Study revisited: effects of land development on deep drainage determined from non-steady chloride profiles. Journal of Hydrology 373, 487–498.
| The Brigalow Catchment Study revisited: effects of land development on deep drainage determined from non-steady chloride profiles.Crossref | GoogleScholarGoogle Scholar |
Sims, NC, and Colloff, MJ (2012). Remote sensing of vegetation responses to flooding of a semi-arid floodplain: implications for monitoring ecological effects of environmental flows. Ecological Indicators 18, 387–391.
| Remote sensing of vegetation responses to flooding of a semi-arid floodplain: implications for monitoring ecological effects of environmental flows.Crossref | GoogleScholarGoogle Scholar |
Stacy, EM, Hart, SC, Hunsaker, CT, Johnson, DW, and Berhe, AA (2015). Soil carbon and nitrogen erosion in forested catchments: implications for erosion-induced terrestrial carbon sequestration. Biogeosciences 12, 4861–4874.
| Soil carbon and nitrogen erosion in forested catchments: implications for erosion-induced terrestrial carbon sequestration.Crossref | GoogleScholarGoogle Scholar |
The State of Queensland (2022) Soil and Land Information (SALI): Soil Chemistry Application Programming Interface (API). Available at www.data.qld.gov.au/dataset/sali-soil-chemistry-api [accessed 29 March 2022].
Thornton CM, Elledge AE (2013) Runoff nitrogen, phosphorus and sediment generation rates from pasture legumes: an enhancement to reef catchment modelling (Project RRRD009). Report to the Reef Rescue Water Quality Research and Development Program. Reef and Rainforest Research Centre Ltd, Cairns, Qld, Australia.
Thornton CM, Elledge AE (2019) Agricultural land management practices and water quality in the Fitzroy Basin: technical report for the 2015 to 2019 hydrological years. Addendum to ‘Paddock scale water quality monitoring of grazing management practices in the Fitzroy Basin: technical report on the effect of grazing pressure on water quality for the 2015 to 2018 hydrological years’. Report to the Queensland Reef Water Quality Program. Department of Natural Resources, Mines and Energy, Rockhampton, Qld, Australia.
Thornton, CM, and Elledge, AE (2021). Heavy grazing of buffel grass pasture in the Brigalow Belt bioregion of Queensland, Australia, more than tripled runoff and exports of total suspended solids compared to conservative grazing. Marine Pollution Bulletin 171, 112704.
| Heavy grazing of buffel grass pasture in the Brigalow Belt bioregion of Queensland, Australia, more than tripled runoff and exports of total suspended solids compared to conservative grazing.Crossref | GoogleScholarGoogle Scholar |
Thornton, CM, and Shrestha, K (2021). The Brigalow Catchment Study: V. Clearing and burning brigalow (Acacia harpophylla) in Queensland, Australia, temporarily increases surface soil fertility prior to nutrient decline under cropping or grazing. Soil Research 59, 146–169.
| The Brigalow Catchment Study: V. Clearing and burning brigalow (Acacia harpophylla) in Queensland, Australia, temporarily increases surface soil fertility prior to nutrient decline under cropping or grazing.Crossref | GoogleScholarGoogle Scholar |
Thornton, CM, and Yu, B (2016). The Brigalow Catchment Study: IV. Clearing brigalow (Acacia harpophylla) for cropping or grazing increases peak runoff rate. Soil Research 54, 749–759.
| The Brigalow Catchment Study: IV. Clearing brigalow (Acacia harpophylla) for cropping or grazing increases peak runoff rate.Crossref | GoogleScholarGoogle Scholar |
Thornton, CM, Cowie, BA, Freebairn, DM, and Playford, CL (2007). The Brigalow Catchment Study: II. Clearing brigalow (Acacia harpophylla) for cropping or pasture increases runoff. Australian Journal of Soil Research 45, 496–511.
| The Brigalow Catchment Study: II. Clearing brigalow (Acacia harpophylla) for cropping or pasture increases runoff.Crossref | GoogleScholarGoogle Scholar |
Trimble, SW, and Mendel, AC (1995). The cow as a geomorphic agent: a critical review. Geomorphology 13, 233–253.
| The cow as a geomorphic agent: a critical review.Crossref | GoogleScholarGoogle Scholar |
Udawatta, RP, Henderson, GS, Jones, JR, and Hammer, D (2011). Phosphorus and nitrogen losses in relation to forest, pasture and row-crop land use and precipitation distribution in the midwest usa. Journal of Water Science 24, 269–281.
| Phosphorus and nitrogen losses in relation to forest, pasture and row-crop land use and precipitation distribution in the midwest usa.Crossref | GoogleScholarGoogle Scholar |
Vink, S, Ford, PW, Bormans, M, Kelly, C, and Turley, C (2007). Contrasting nutrient exports from a forested and an agricultural catchment in south-eastern Australia. Biogeochemistry 84, 247–264.
| Contrasting nutrient exports from a forested and an agricultural catchment in south-eastern Australia.Crossref | GoogleScholarGoogle Scholar |