Advances in synchronization and superstimulation for OPU/IVEP: optimizing oocyte quantity and quality
Jessica C. L. Motta A , Cameron B. Hayden A , Rodrigo V. Sala B , Pablo J. Ross C and Alvaro García-Guerra A *A
B
C
Abstract
The sustained expansion of in vitro embryo production (IVEP) in cattle necessitates ongoing advancements in IVEP methodologies. The success of IVEP largely depends on the quantity and quality (i.e. developmental competence) of oocytes retrieved after ovum pick-up (OPU). The fundamental role of the ovarian follicle in the development of the oocyte highlights the importance of considering the ovarian physiology during development of OPU/IVEP methodologies. Oocyte quantity is largely influenced by ovarian phenotype determined through antral follicle count (AFC) or circulating anti-Müllerian hormone (AMH). In addition, substantial evidence indicates that follicle size and status can affect OPU/IVEP outcomes such that promoting the presence of medium to large sized dominant-like follicles can enhance oocyte developmental competence and thus embryo production. Ovarian superstimulation, using follicle-stimulating hormone (FSH), allows optimization of follicle development and oocyte developmental competence resulting in greater IVEP efficacy. The present review examines various aspects of FSH administration, providing an update of ovarian superstimulation methodologies and their implementation in OPU/IVEP systems. Additionally, the potential development of targeted ovarian superstimulation approaches that consider the marked physiological differences among individuals with varying AFC/AMH phenotypes is explored with the goal of further enhancing IVEP efficacy.
Keywords: anti-Müllerian hormone, antral follicle count, bovine, cattle, embryo transfer, follicles, follicle-stimulating hormone, in vitro embryo production, oocytes, ovarian superstimulation.
Introduction
The introduction of genomic-assisted selection has revolutionized breeding programs, particularly in dairy cattle, leading to a substantial increase in the rate of genetic gain (García-Ruiz et al. 2016; Wiggans and Carrillo 2022). Assisted reproductive technologies (ART), such as multiple ovulation and embryo transfer (MOET) and particularly in vitro embryo production (IVEP), have played a vital role in the increased rate of genetic gain by providing the opportunity for: (1) greater selection intensity by increasing the number of offspring from elite females; and (2) reduced generation interval by producing offspring from peri- or prepubertal heifers (Schefers and Weigel 2012; Mueller and Van Eenennaam 2022). Accordingly, adoption of embryo technologies has increased dramatically in the last decade (Viana 2023). Concurrently, the embryo transfer (ET) industry has experienced a marked shift from utilization of MOET (−46%) to IVEP (+260%), such that IVEP currently accounts for ~80% of the bovine embryos produced worldwide (Viana 2023). The rapid expansion of IVEP stems, at least in part, to its many advantages, which include: (1) greater embryo production per unit of time; (2) increased efficiency of semen use; (3) ease of incorporation of sex-sorted semen; and (4) the capability to produce embryos from prepubertal, pubertal, and pregnant females, among others (Pontes et al. 2009; Baruselli et al. 2016; Ferré et al. 2020). Furthermore, enhanced understanding of gamete/embryo biology has resulted in improved methodologies and thus contributed to the expansion of IVEP (Hansen 2006; Ferré et al. 2020). The remarkable and sustained growth of IVEP indicates that this method will continue to be, at least for the foreseeable future, the preferred method for embryo production in cattle, underscoring the need for continuous improvement to increase the efficacy (i.e. number of viable embryos per procedure) of IVEP systems.
The success of IVEP systems hinges primarily on the number and quality of cumulus–oocyte complexes (COCs) retrieved during ovum pick-up (OPU) (Baruselli et al. 2016). Developmental competence is the ultimate indicator of oocyte quality and is defined as the capacity of the oocyte to undergo, in a timely manner, the processes required for development into a viable embryo/blastocyst (Luciano and Sirard 2018). Fig. 1 depicts OPU/IVEP data collected from pubertal and pregnant Holstein heifers by our research group at a commercial ET facility (J. C. L. Motta, A. Garcia-Guerra, pers. comm.). Not surprisingly, and in agreement with previous reports (Demetrio et al. 2020), there is large variation in the number and quality (i.e. developmental competence) of oocytes recovered after OPU which in turn leads to large variation in the number of viable embryos produced per OPU procedure. The large variability in IVEP outcomes is detrimental to the predictability, success, and profitability of ET programs; thus, posing a major challenge for the industry. A large number of factors that affect oocyte quantity and/or quality have been identified, including size of the ovarian reserve (Guerreiro et al. 2014), age (Baruselli et al. 2016; Batista et al. 2016), breed (Baruselli et al. 2022), physiological status (Vieira et al. 2014; Baruselli et al. 2016), use and dose of gonadotropins (Hayden et al. 2023), disease (Dickson et al. 2020), reproductive history (Sood et al. 2017), and nutrition (Sales et al. 2015; Dantas et al. 2019). Although of great value, detailed discussion of each of these factors is beyond the scope of the present review. The ovarian follicle is undoubtedly fundamental in the development of the oocyte, underscoring the importance of ovarian physiology on oocyte quantity and quality. Furthermore, follicle development is the target, directly or indirectly, of reproductive management interventions for controlling ovarian function. Thus, the purpose of this review is to summarize and discuss: (1) the contribution of ovarian physiology to oocyte quantity and developmental competence; and (2) the impact of ovarian superstimulation strategies on OPU/IVEP outcomes, with the long-term goal of increasing the efficacy of OPU/IVEP systems.
Distribution of total follicles (a), total number of cumulus–oocyte complex (COC; b), embryo rate (number of viable embryos/viable COC; c) and number of viable embryos (d) after OPU/IVEP in pubertal (11–16 months of age; n = 212) and pregnant (14–25 months of age; 36–104 days of gestation; n = 263) Holstein heifers. All heifers were submitted to ovarian superstimulation before OPU using 160 or 200 mg (280 or 350 IU) of porcine FSH (Folltropin, Vetoquinol USA) distributed in four or six administrations 12 h apart.
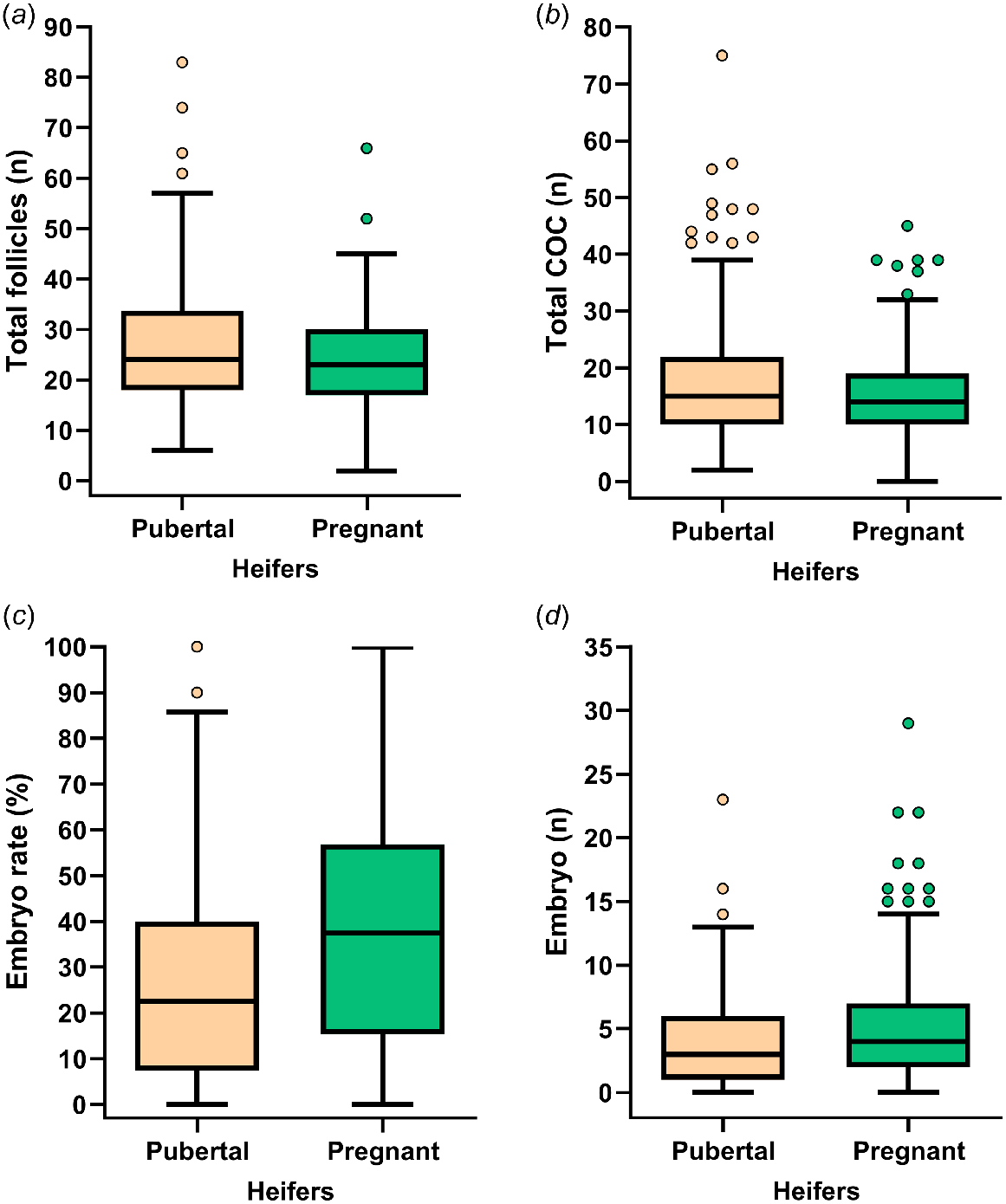
Contributions of ovarian physiology to oocyte quantity and quality
The oocyte is housed within the ovarian follicle for an extended period, during which the follicle experiences profound morphological, cellular, and molecular changes. Furthermore, the presence of a complex bidirectional communication between the oocyte and follicular somatic cells (Knight and Glister 2006; Scaramuzzi et al. 2011; Hsueh et al. 2015) highlights the importance of follicle function on oocyte physiology. Consequently, considerable research efforts have been devoted to elucidating the follicular contributions to oocyte yield and developmental competence, as well as developing management strategies to optimize these contributions.
Follicle size and developmental stage
Follicle development is a precisely regulated process that involves growth and functional maturation of both the somatic cells and oocyte (Scaramuzzi et al. 2011; Monniaux 2016). The marked increase in size of both the follicle (~400-fold) and oocyte (~4-fold) during development from the preantral to the preovulatory stage in cattle (Ginther et al. 1989; Braw-Tal and Yossefi 1997; Hyttel et al. 1997) has led to assessment of the effects of follicle size on oocyte quantity and developmental competence. In addition, follicle size has received special attention because of its potential to serve as a simple and reliable indicator of oocyte quality. Nevertheless, the wave-like pattern of follicular growth coupled with selection of a dominant follicle, characteristic of cattle (García-Guerra et al. 2018), requires that follicle size be considered in the context of follicular wave dynamics.
Follicle size can negatively affect the number of COC collected after OPU (Pieterse et al. 1991; Seneda et al. 2001) due to effects on retrieval efficacy. For instance, in non-stimulated cows, retrieval efficacy was 59%, 55%, and 42% for 3–5 mm, 6–10 mm, and >10 mm follicles, respectively (Pieterse et al. 1991). Similarly, Seneda et al. (2001) reported a ~30% reduction in retrieval efficacy when aspirating follicles >4 mm relative to that of follicles ≤4 mm in non-stimulated cows. The lesser oocyte yield from larger sized follicles has been attributed to the loss of fluid during aspiration owing to the greater follicular fluid viscosity and intrafollicular pressure (Seneda et al. 2001). In contrast, Pieterse et al. (1988) reported numerically greater COC retrieval efficacy in cows stimulated with equine chorionic gonadotropin. This improvement was attributed to the greater number of 5–10 mm follicles, which facilitated ovarian manipulation and follicle puncture. We recently evaluated whether the proportion of medium (6–10 mm) to large (>10 mm) sized follicles after ovarian superstimulation with follicle-stimulating hormone (FSH) affected COC retrieval efficacy in pubertal and pregnant Holstein heifers. Interestingly, there was no association (r = −0.03; P = 0.48) between the proportion of medium to large sized follicles and COC retrieval efficacy (J. C. L. Motta, A. Garcia-Guerra, pers. comm.). It is important to note, however, that needle size and type, vacuum pressure, and technician experience can affect retrieval efficacy (Pieterse et al. 1988; Boni 2012). Consequently, optimization of the retrieval procedure may allow to overcome any potential differences in retrieval efficacy related to follicle size.
The subtle but significant greater number of follicles observed near wave emergence (Pierson and Ginther 1987; Burns et al. 2005), led to the idea that OPU performed after synchronization of follicle wave emergence during the common growth phase could increase oocyte yield. Performing OPU during the common growth phase in lactating Holstein cows resulted in more follicles of ≥5 mm (+142%) and COCs retrieved (+121%) than when performed during dominant follicle regression (Machatkova et al. 2000). Conversely, no differences in COC yield were observed when OPU was performed at different days during the common growth phase. Similarly, Gimenes et al. (2015) observed no differences in number of follicles and COCs retrieved when performing OPU at 1, 3, or 5 days after follicular wave emergence on Bos indicus and Bos taurus heifers. These results taken together suggest that OPU should be performed during the growing or early dominance phase of the follicular wave to optimize oocyte yield. Furthermore, these observations led to the notion that synchronization of follicular wave emergence before OPU was needed. Ongaratto et al. (2015) reported a 1.8-fold increase in the number of follicles aspirated and a 2.5-fold increase in the number of total and viable COCs when OPU was performed 2 days after synchronized follicular wave emergence than at random stages of the follicular wave in Bos taurus and Bos taurus × Bos indicus cows. Conversely, Cavalieri et al. (2018) reported no differences in the number of COCs recovered when OPU was done after synchronized follicular wave emergence or at random stages of the estrous cycle in Bos indicus cows, albeit oocyte yield was numerically greater after synchronization. Performing OPU after synchronization of follicle wave emergence, during the common growth or early dominant phase, may therefore serve as a strategy to increase oocyte yield in non-stimulated donors.
Oocyte developmental competence is acquired when follicles reach 2–3 mm in diameter, coincident with the attainment of terminal oocyte size and reduced transcriptional activity (Pavlok et al. 1992; Fair et al. 1995; Hyttel et al. 1997). A large number of studies published to date have explored the effect of follicle size on oocyte developmental competence, and these are summarized in Table 1. Results from most studies indicate a positive association between follicle size and the percentage of oocytes developing into viable embryos/blastocysts (Pavlok et al. 1992; Lonergan et al. 1994; Blondin et al. 1997; Hagemann et al. 1999; Lequarre et al. 2005; Caixeta et al. 2009; Sarwar et al. 2020a), suggesting that oocyte developmental competence increases as follicle size increases. Nevertheless, most research in this area has utilized abattoir-derived oocytes, which often implies that the developmental stage of a particular follicle is unknown, posing a challenge for result interpretation in the broader context of follicle and oocyte biology. As a result, oocyte developmental competence has also been evaluated in the context of stage of the follicular wave (Hagemann et al. 1999; Machatkova et al. 2000, 2004; Vassena et al. 2003), albeit with contrasting results. For example, results from some studies indicate that developmental competence is greater when COCs are collected during the growing or early dominance phase (Hagemann et al. 1999; Vassena et al. 2003; Machatkova et al. 2004). Accordingly, developmental competence and embryo yield were improved when OPU was performed during the common growth phase after synchronization of follicular wave emergence rather than at a random stage of the estrous cycle in non-stimulated Bos indicus cows (Cavalieri et al. 2018). Conversely, others have reported no effect of stage of follicular wave on oocyte competence (Machatkova et al. 2000; Gimenes et al. 2015). For example, Gimenes et al. (2015) observed no differences in viability, cleavage, or blastocyst rate when COCs were collected at 1, 3, or 5 days after wave emergence in Bos indicus and Bos taurus heifers. The ability to assess the effects of follicular developmental stage is limited, to a certain extent, because COCs are derived from all follicles present at the time of OPU. Therefore, most COCs are retrieved from non-dominant smaller sized follicles precluding proper assessment of the effects of acquisition of dominance on oocyte developmental competence.
Author | Breed A | Category B | Follicle size (mm) | Oocytes (n) | Cleavage (%) | Embryo (%) | |
---|---|---|---|---|---|---|---|
Tan and Lu (1990) | NR | NR | <2 | 257 | 67.7ab | 17.1c | |
2–6 | 954 | 84.6a | 29.8b | ||||
>6 | 65 | 86.2a | 46.2a | ||||
Pavlok et al. (1992) | NR | NR | <2 | 228 | 19.7c | 0.0c | |
2–4 | 930 | 64.3b | 20.9b | ||||
4–8 | 463 | 68.9a | 27.7a | ||||
Lonergan et al. (1994) | NR | Heifer cow | 2–6 | 109 | 90.8 | 34.3b | |
>6 | 98 | 92.9 | 65.9a | ||||
Hagemann et al. (1999) | Holstein | Cow | 3–5 | 767 | – | 35.7D | |
6–8 | 152 | – | 39.5C | ||||
9–12 | 28 | – | 42.9 B | ||||
≥13 | 25 | – | 52.0 A | ||||
Seneda et al. (2001) | Holstein | Cow | ≤4 | 194 | – | 24.7 | |
>4 | 75 | – | 22.5 | ||||
Lequarre et al. (2005) | Holstein | Cow | <4 | – | 80 | 35b | |
4–5 | – | 82 | 45ab | ||||
≥6 | – | 84 | 50a | ||||
Mixed breed | Cow | <4 | – | 72b | 22b | ||
4–5 | – | 84a | 31a | ||||
≥6 | – | 89a | 39a | ||||
Belgian blue | Cow | <4 | – | 53b | 14b | ||
4–5 | – | 54b | 17b | ||||
≥6 | – | 71a | 42a | ||||
Caixeta et al. (2009) | Nelore | Cow | 1–3 | 96 | 59c | 20b | |
3.1–6 | 183 | 73b | 34b | ||||
6.1–8 | 81 | 86a | 62a | ||||
≥8.1 | 107 | 87a | 60a | ||||
Sarwar et al. (2020a) | NR Bos indicus | Cow | <6 | 321 | 47.4b | – | |
≥6 | 310 | 58.1a | – |
Although there is large variation in the reported effects of follicle stage, it is generally agreed that competence is reduced when oocytes are retrieved from follicles in advanced stages of atresia (Merton et al. 2003). Interestingly, the greater developmental rates obtained with oocytes derived from dominant sized follicles in both Bos taurus and Bos indicus cattle (Hagemann et al. 1999; Caixeta et al. 2009) suggest that acquisition of dominance may be associated with improved developmental competence. The acquisition of dominance is characterized by the induction of LH receptors in granulosa cells that confer the dominant follicle the capability to thrive under reduced circulating concentrations of FSH and respond to an ovulatory stimulus (García-Guerra et al. 2018). Furthermore, the decline in FSH support, associated with acquisition of dominance, induces changes within the follicle that include the transition from proliferation to differentiation, as well as increased oocyte chromatin condensation, all of which are important for the acquisition of oocyte developmental competence (Landry and Sirard 2018; Luciano and Sirard 2018). Consequently, manipulation of ovarian function to promote the development of follicles with a dominant or dominant-like phenotype may contribute to optimize oocyte developmental competence and thus IVEP efficacy.
Ovarian phenotype: antral follicle count and anti-Müllerian hormone
The introduction of B-mode ultrasonography allowed for assessment of the size of the antral follicle population (Pierson and Ginther 1984; Sirois and Fortune 1988), commonly termed antral follicle count (AFC). Substantial evidence has been provided to date indicating that AFC is highly repeatable within an individual, however, varies greatly between individuals (Singh et al. 2004; Burns et al. 2005; Ireland et al. 2007). In addition, AFC is markedly greater in Bos indicus females compared to Bos taurus females, which has important implications in the context of IVEP systems (Baldrighi et al. 2014; Batista et al. 2014; Sartori et al. 2016). The discovery that anti-Müllerian hormone (AMH), a dimeric glycoprotein of the transforming growth factor β (TGF-β) superfamily, is produced by granulosa cells of preantral and small antral growing follicles (Vigier et al. 1984; Rico et al. 2009) introduced the possibility of using AMH as a biomarker for AFC. Accordingly, strong positive correlations between circulating AMH and AFC, typically ranging between 0.68 and 0.88, have been reported for both Bos taurus and Bos indicus females (Ireland et al. 2008; Rico et al. 2009; Batista et al. 2014; García-Guerra et al. 2017; Cardoso et al. 2018). Furthermore, AMH concentrations in postpubertal cattle appear to vary minimally during the follicular wave (Ireland et al. 2008), between follicular waves (Rico et al. 2009), and during natural and synchronized estrous (Pfeiffer et al. 2014), suggesting that a single AMH assessment at a random stage of the estrous cycle can be effectively used as a predictor of AFC (Ireland et al. 2011). The large variability observed in AFC and AMH have significant physiological and practical implications as discussed in subsequent sections of the present review.
The distinct low and high AFC/AMH phenotypes in cattle are accompanied by marked physiological differences involving cellular and endocrine changes (Mossa and Ireland 2019). Low AFC/AMH cattle have chronically greater circulating concentrations of FSH (Singh et al. 2004; Burns et al. 2005; Ireland et al. 2007; Mossa et al. 2010a) and LH (Jimenez-Krassel et al. 2009) compared to high AFC/AMH cattle. The chronically heightened FSH does not appear to result from differences in the gonadotropin-secreting capability of the pituitary or its ability to respond to ovarian products (Mossa et al. 2010a). More likely, this difference is due to reduced output of FSH inhibitors by the lesser number of follicles, given that all the follicles within the wave contribute to the suppression of FSH (Gibbons et al. 1997). Interestingly, the low AFC/AMH phenotype in cattle is also associated with a diminished capability of follicular and luteal cells to respond to gonadotropin stimulation (Jimenez-Krassel et al. 2009; Mossa et al. 2010b; Scheetz et al. 2012). Granulosa cells respond to FSH stimulation in a biphasic dose-dependent manner. For example, initial increases in FSH dose result in a linear increase in estradiol secretion, while subsequent increases in FSH dose lead to a linear decrease in estradiol secretion and changes resembling luteinization (Scheetz et al. 2012). Granulosa cells from high AFC/AMH females have a greater amount of FSH receptors and respond more robustly to FSH stimulation compared to those from low AFC/AMH individuals, as evidenced by greater CYP19A1 mRNA abundance (7.8-fold) and estradiol secretion (4.3-fold). More interestingly, maximal CYP19A1 mRNA abundance and estradiol secretion are achieved at a lesser FSH dose (0.1 vs 0.5 ng/mL) in low compared to high AFC/AMH females, respectively (Scheetz et al. 2012). These results suggest that low AFC/AMH females are refractory to gonadotropin stimulation, which may have significant implications for the implementation of ART.
The notion that AFC/AMH phenotype is associated with embryo production potential was first reported by Singh et al. (2004) who observed that high AFC Bos taurus cows presented a greater number of follicles after superstimulation than their low AFC counterparts. Multiple studies in Bos taurus and Bos indicus-taurus, have reported greater superovulatory response and embryo yield in high as compared to low AFC/AMH females during MOET (Ireland et al. 2007; Silva-Santos et al. 2014; Souza et al. 2015; Center et al. 2018). Moreover, moderate to strong correlations were observed between AFC/AMH and superovulatory response, total ova/embryo, and transferable embryos (Rico et al. 2009, 2012; Souza et al. 2015; Center et al. 2018). The association between AFC/AMH and superovulatory response during MOET is consistent with the ability of FSH administration to rescue subordinate follicles, allowing them to achieve ovulatory capacity (Adams et al. 1993; García-Guerra et al. 2015). Interestingly, AMH is not associated with recovery efficacy, percent fertilized ova, or percent transferable embryos during MOET (Souza et al. 2015; Center et al. 2018). Therefore, AFC/AMH determination is a robust method for the selection of donors with superior embryo production potential.
The rapid expansion of IVEP has logically led to assessment of the association between AFC/AMH and IVEP outcomes. As expected, non-stimulated high AFC/AMH females produce a greater number of viable embryos than their low AFC/AMH counterparts due to retrieval of a greater number of COCs, with no apparent detrimental effect on developmental competence, as reported in Bos taurus, Bos indicus, and Bos taurus × Bos indicus females (Ireland et al. 2007; Guerreiro et al. 2014; Silva-Santos et al. 2014; Rosa et al. 2018). In addition, circulating AMH was found to be positively correlated with the number of total follicles aspirated, total COCs retrieved, and number of blastocysts produced after IVEP in non-stimulated Bos indicus and Bos taurus cows and heifers (Guerreiro et al. 2014). Conversely, circulating AMH was not correlated with cleavage or blastocyst rates, suggesting that AMH phenotype is without effect on oocyte developmental competence (Guerreiro et al. 2014). Remarkably, there is an apparent lack of reports on the association between AFC/AMH and IVEP outcomes in FSH-stimulated cattle. Batista et al. (2016) reported positive correlations between AMH, number of COCs retrieved, and embryo yield after laparoscopic OPU in Bos indicus and Bos taurus heifer calves; however, the reported associations included both stimulated and non-stimulated calves.
Our research group recently conducted a retrospective analysis of data collected from FSH-stimulated pubertal (n = 194) and pregnant (n = 255) Holstein heifers at a commercial ET facility (J. C. L. Motta, A. Garcia-Guerra, pers. comm.). Heifers were subjected to follicle ablation, and ovarian superstimulation was initiated 36 h later at the expected time of follicular wave emergence. Ovarian superstimulation consisted of 160 or 200 mg (280 or 350 IU) of porcine FSH (Folltropin, Vetoquinol USA) distributed in four or six administrations, and OPU was performed 40–44 h after the last FSH administration. Circulating AMH concentrations (#AL-114, bovine AMH ELISA, Ansh Labs LLC, Webster, TX, USA) were assessed at the time of follicle ablation, and the association between AMH and various IVEP outcomes was performed using generalized linear mixed models. Greater circulating AMH concentration was associated with greater number of total follicles at OPU (Fig. 2a), total number of COCs retrieved (Fig. 2b), and number of viable embryos (Fig. 2d) in both pubertal and pregnant heifers, in agreement with previous results in non-stimulated cattle. Surprisingly, there was a significant positive association between AMH concentration and the percentage of oocytes that developed into viable embryos suggestive of enhanced oocyte developmental competence (Fig. 2c). Although the association between AMH and oocyte developmental competence should be taken with caution given the limitations of observational studies, it is noteworthy that conflicting results have been reported in the literature. While most studies have reported that AFC/AMH is without effect on developmental competence (Ireland et al. 2007; Guerreiro et al. 2014; Silva-Santos et al. 2014; Rosa et al. 2018), some have suggested that AFC/AMH may have a positive effect (Batista et al. 2016; Santos et al. 2016), underscoring the need for further research in this area. The reported associations between AFC/AMH and embryo production indicate that the substantial individual variability in embryo yield after both MOET and OPU/IVEP can be explained largely by differences in AFC/AMH phenotype among females. Despite the ability of AFC and/or AMH to identify donors with superior embryo production capabilities, the utilization of these criteria for donor selection is limited and largely overshadowed by other attributes such as genetic potential for economically relevant traits. Nevertheless, the observation that both AFC and AMH have moderate heritability ranging from 0.25 to 0.46 (Walsh et al. 2014; Gobikrushanth et al. 2018; Nawaz et al. 2018), indicates the potential for these traits to be incorporated into selection criteria that considers both embryo production potential and economically relevant traits.
Association between circulating AMH and total follicles (a), total number of cumulus–oocyte complex (COC; b), embryo rate (number of viable embryos/viable COC; c) and number of viable embryos (d) after OPU/IVEP in FSH-stimulated pubertal (11–16 months of age; n = 194) and pregnant (14–25 months of age; 36–104 days of gestation; n = 255) Holstein heifers. All heifers were submitted to ovarian superstimulation before OPU using 160 or 200 mg (280 or 350 IU) of porcine FSH (Folltropin, Vetoquinol USA) distributed in four or six administrations 12 h apart. Ovarian superstimulation was initiated 36 h after follicle ablation and OPU was performed 40–44 h after the last FSH administration. Dashed lines indicate 95% confidence intervals.
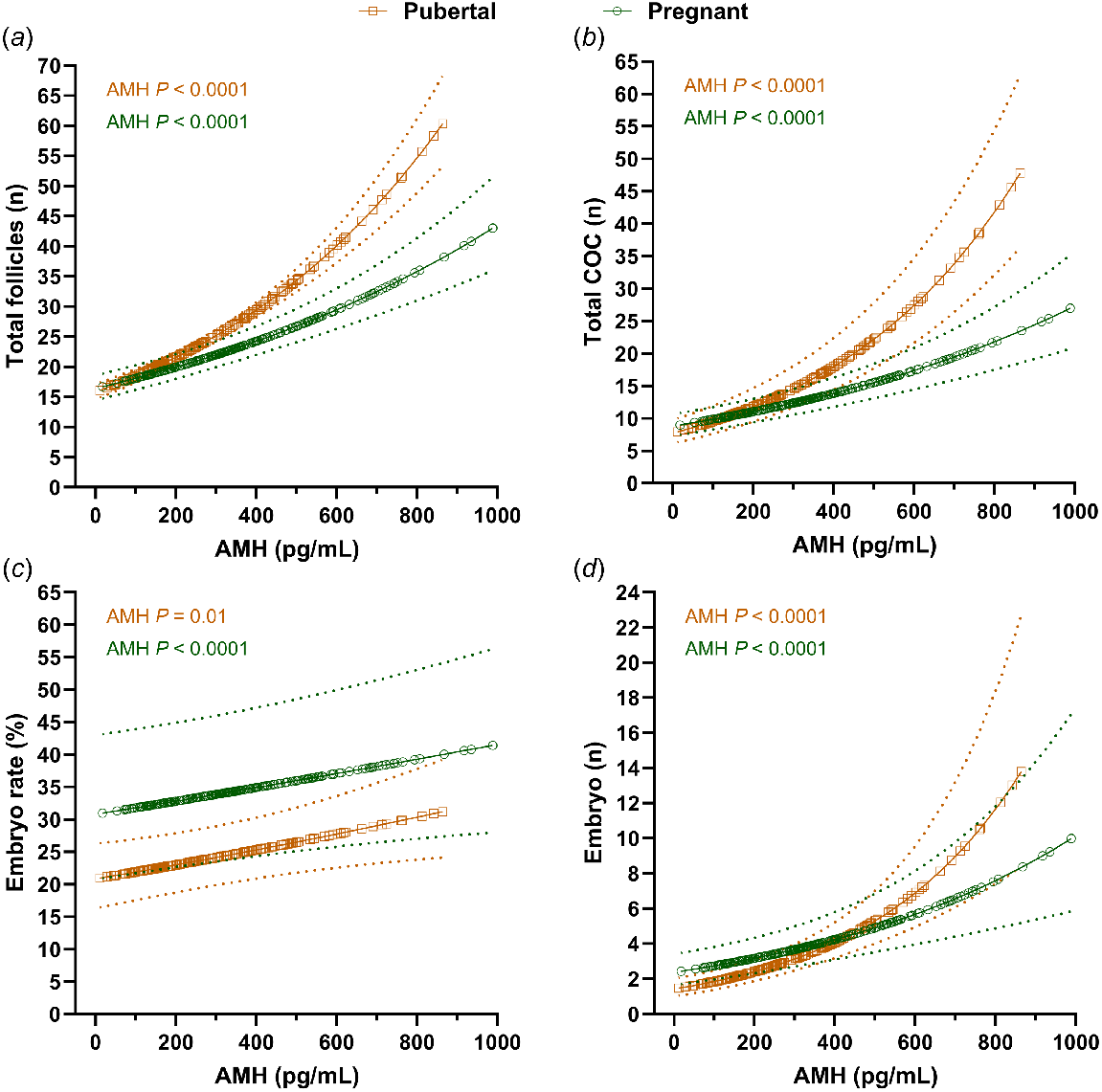
Ovarian superstimulation
The previously discussed effects of follicle size and developmental stage on oocyte developmental competence suggest that collecting oocytes derived from medium to large sized follicles that have acquired a dominant-like phenotype enhances oocyte competence. Follicle selection, however, limits the number of follicles that can acquire these characteristics (García-Guerra et al. 2018). The pivotal role of FSH in follicle selection (Ginther et al. 2000; García-Guerra et al. 2018) coupled with the notion that superstimulation results in follicle rescue, allowing multiple follicles to acquire a dominant phenotype (Adams et al. 1993; García-Guerra et al. 2015), provided a strong rationale for the use of FSH to enhance OPU/IVEP efficacy. Inconsistent results from initial attempts using ovarian superstimulation for IVEP (Lonergan et al. 1994; Blondin et al. 1996) suggested that FSH stimulation may lead to asynchronous development of follicles and oocytes (Sirard 2011). Acquisition of dominance and terminal follicle development is accompanied by a shift from a FSH-dominated milieu to one without it and a dependence on LH support for continuous development (García-Guerra et al. 2018). This shift supported the incorporation of a FSH withdrawal period after ovarian superstimulation and before OPU, known as ‘coasting’, to mimic the decrease in circulating FSH and thus optimize both follicle and oocyte development (Blondin et al. 2002). Incorporating a coasting period of ~40–70 h results in enhanced oocyte developmental competence and therefore has become the industry standard when utilizing FSH stimulation before OPU/IVEP (Sirard et al. 1999; Blondin et al. 2002; Nivet et al. 2012).
Many studies focused on the effect of ovarian superstimulation before OPU/IVEP have been conducted in both Bos taurus and Bos indicus cattle, albeit with inconsistent results. Results from studies performed in postpubertal females and in which embryo yield was reported are summarized in Table 2. Results from most studies indicate a marked advantage of FSH administration in embryo yield, with relative increases ranging from 30% to 380%. A considerable number of studies, however, have found FSH to be without effect on embryo yield. Nevertheless, it is noteworthy that almost all studies in which no effect of FSH administration was observed still reported numerically greater embryo yield for FSH-treated cattle. Although it is not possible to determine the precise reasons for the conflicting results, the large variability in study population and experimental conditions among studies is readily apparent. Of special note are differences observed in breed, age, physiological status, FSH administration (e.g. product, dose, treatment schedule), supplemental treatments (e.g. follicular wave synchronization, progesterone administration), experimental design, and sample size. Interestingly, results from a recent meta-analysis (Sarwar et al. 2020b) indicate that FSH administration before OPU/IVEP, in non-pregnant progestin-primed cattle, increases embryo yield. Not surprisingly, FSH stimulation before OPU/IVEP is widely utilized, particularly in Europe (88%) and North America (69.2%), likely due to the predominance of Bos taurus influenced cattle (Viana 2023).
Breed | Category | Dose | Total follicles | Total COC | Cleavage (%) | Embryo (%) | Embryo (n) | Study | ||
---|---|---|---|---|---|---|---|---|---|---|
No FSH | FSH | |||||||||
Bos taurus | ||||||||||
Beef | Cow | 200 mgA | ↑↑ | ↑↑ | – | – | 0.6 ± 0.8b | 2.1 ± 1.2a | Chaubal et al. (2006) | |
160 mgA | ↑ | = | – | – | 1.2 ± 0.3 | 1.8 ± 0.3 | Ongaratto et al. (2020) | |||
160 mgA | ↑ | ↓ | – | ↑ | 3.2 ± 0.8 | 3.9 ± 0.7 | Sola et al. (2023b) | |||
Cow/Heifer | 17.6 mgB | ↑↑ | ↑↑ | – | ↑ | 0.7 ± 1.2b | 3.4 ± 3.9a | De Roover et al. (2008) | ||
Heifer | 9 mgB | ↑ | = | – | ↑ | 1.0 ± 0.3b | 2.1 ± 0.4a | Goodhand et al. (1999) | ||
Dairy | Cow | 200 mgA | = | = | = | = | 4.1 ± 0.5 | 4.3 ± 0.5 | da Silva et al. (2017) | |
30 mgC | = | = | ↑ | ↑↑ | 4.3 ± 2.9b | 12.8 ± 8.7a | Imai et al. (2007) | |||
240 mgA | – | = | – | = | 2.6 ± 0.5 | 3.0 ± 0.5 | Oliveira et al. (2016) | |||
200 mgA | ↑ | ↑ | ↑ | = | 2.4 ± 0.5 | 3.7 ± 0.7 | Vieira et al. (2016) | |||
200 mgA | = | = | = | ↑ | 1.8 ± 0.4b | 3.0 ± 0.5a | Vieira et al. (2014) | |||
Heiferγ | 160 mgA | ↑ | ↑ | ↑ | ↑ | 2.4 ± 0.3b | 4.0 ± 0.5a | Hayden et al. (2023) | ||
300 mgA | ↑ | ↑ | ↑ | ↑ | 2.4 ± 0.3b | 5.2 ± 0.6a | Hayden et al. (2023) | |||
DP | Heifer | 300 IUD | – | = | = | = | 4.1 ± 0.9 | 4.8 ± 1.1 | Scarlet et al. (2024) | |
Bos indicus | ||||||||||
Dairy | Cow | 140 mgA | – | – | ↑ | – | 2.8b | 5.8a | Elliff et al. (2019) | |
60 mgA | – | = | – | – | 2.3 ± 0.7b | 4.1 ± 0.8a | Cedeño et al. (2023) | |||
60 mgA | – | = | – | – | 2.9 ± 0.4b | 3.9 ± 0.2a | Ocampo et al. (2024) |
Different lowercase superscript letters (a,b) within a row indicate differences between treatments (P ≤ 0.05).
A,B,C,DIndicates FSH product administered (AFolltropin; BOvagen; CAntrin; DPluset). γIndicates pregnant heifers. Symbols represent differences between no FSH vs FSH: no significant difference (=), significant increase for FSH group with a relative change ≤100% (↑), significant increase for FSH group with a relative change >100% (↑↑), significant decrease for FSH group with a relative change >100% (↓), data not reported (−).
DP, dual-purpose.
The reported improvements in IVEP observed after ovarian superstimulation stem primarily from: (1) greater number of follicles and COCs; and/or (2) improved oocyte developmental competence. As expected, administration of FSH before OPU consistently results in a greater number and proportion of medium to large sized follicles, in agreement with the notion that exogenous FSH rescues subordinate follicles (Adams et al. 1993; García-Guerra et al. 2015). Even though ovarian superstimulation does not result in recruitment of new follicles, results from most studies indicate that ovarian superstimulation leads to a greater total number of follicles at OPU (Chaubal et al. 2006; De Roover et al. 2008; Vieira et al. 2016; Ongaratto et al. 2020; Hayden et al. 2023). This somewhat unexpected outcome may stem from improved follicle visualization due to greater follicle size and/or from rescue of very small (<2 mm) antral follicles (Jaiswal et al. 2004; García-Guerra et al. 2015). Increased follicle size after FSH stimulation could reduce COC yield due to the aforementioned negative effect of follicle size on COC retrieval efficacy (Pieterse et al. 1991; Seneda et al. 2001). Although that is certainly the case in some studies (Goodhand et al. 1999; Vieira et al. 2014; Elliff et al. 2019), most indicate that superstimulation does not compromise COC retrieval (Bungartz et al. 1995; De Roover et al. 2008; Vieira et al. 2016; da Silva et al. 2017; Egashira et al. 2019; Elliff et al. 2019; Hayden et al. 2023; Scarlet et al. 2024). Adjustments in the COC retrieval procedure (e.g. needle size, vacuum pressure) and degree of expertise of the technician performing OPU may explain, at least in part, the lack of differences in COC retrieval efficacy (Boni 2012). The increase in the total number of follicles coupled with the lack of an effect of FSH on retrieval efficacy leads to a greater number of COCs available for IVEP, as reported in multiple studies (Chaubal et al. 2006; De Roover et al. 2008; Vieira et al. 2016; Hayden et al. 2023).
The effects of ovarian superstimulation on developmental competence stem from the increase in the number and proportion of medium sized follicles and the role of follicle size and status on oocyte developmental competence. Furthermore, the proportion of medium sized follicles after ovarian superstimulation has been shown to be positively correlated with oocyte developmental competence (Nivet et al. 2012). Accordingly, a substantial number of studies have reported improved cleavage and/or embryo rate after ovarian superstimulation with FSH (Goodhand et al. 1999; Imai et al. 2007; Vieira et al. 2014, 2016; Hayden et al. 2023). The mechanisms underscoring the effect of FSH administration on developmental competence have not been fully elucidated, albeit it is known that FSH administration has profound effects on gene expression of the oocyte (Chu et al. 2012), cumulus (Sugimura et al. 2017), and granulosa cells (Dias et al. 2013a). In addition, the ability of cumulus cells to expand after in vitro maturation (IVM), an indicator of enhanced developmental competence, was greater in COCs obtained after FSH stimulation (Sugimura et al. 2017). Thus, it appears that FSH stimulation allows multiple follicles to acquire a dominant-like phenotype which, coupled with the removal of FSH during coasting, mimics the physiological changes that occur in preparation for ovulation and which are critical for oocyte developmental competence (Landry and Sirard 2018; Sirard 2022). Intriguingly, several reports (Table 2) indicate that FSH administration is without effect on oocyte developmental competence and may reflect culture conditions during IVM that allow adequate oocyte development even when these are derived from follicles at less-than-ideal stages.
Approaches to superstimulation for OPU/IVEP
Initiating FSH administration at the time of follicular wave emergence results in improved superovulatory response and embryo production during MOET (Nasser et al. 1993; Adams et al. 1994; Surjus et al. 2014). Similarly, administration of FSH at follicular wave emergence resulted in a larger number of follicles and COCs at OPU compared to initiating FSH administration in the presence of a dominant follicle in prepubertal Bos taurus heifers (Brogliatti et al. 1997). More recently, our research group evaluated the effect of initiating FSH administration at the time of follicular wave emergence as opposed to random stages of the follicular wave on superstimulatory response and IVEP (Hayden et al. 2022). Although total number of follicles at OPU did not differ, initiation of FSH administration at follicle wave emergence resulted in a greater percentage of medium sized follicles, indicative of a greater superstimulatory response (Table 3). Initiating FSH administration at follicular wave emergence was without effect on the number of COCs retrieved, consistent with the lack of differences in number of total follicles. Conversely, cleavage and embryo rates were markedly greater when FSH administration was initiated at follicular wave emergence, leading to a ~60% increase in the number of embryos produced (Table 3). Interestingly, results from a recent study indicate that administration of FSH at follicular wave emergence results in a large proportion of oocytes with an intermediate degree of chromatin compaction which is associated with enhanced developmental competence (Soares et al. 2020). Thus, initiation of ovarian superstimulation at the onset of the follicular wave leads to a more homogenous group of follicles in terms of size and stage of development which in turn results in retrieval of oocytes with enhanced developmental competence. In contrast, administration of FSH at random stages of the follicular wave is likely to induce growth of follicles that have already entered the plateau or early regressing phase, which may explain the reduced developmental competence considering that developmental competence is short-lived (Luciano and Sirard 2018).
Non-synchronized | Synchronized | ||
---|---|---|---|
N | 32 | 32 | |
Total follicles | 30.6 ± 1.8a | 31.8 ± 1.5a | |
Follicle size distribution (%) | |||
Small follicles (<6 mm) | 41.1 ± 3.7a | 29.6 ± 2.8b | |
Medium follicles (6–10 mm) | 49.9 ± 3.4a | 61.2 ± 2.8b | |
Large follicles (>10 mm) | 8.9 ± 1.4a | 9.2 ± 1.7a | |
COC retrieval | |||
Total COCs | 18.6 ± 1.6a | 20.9 ± 1.7a | |
Viable COCs | 15.7 ± 1.5a | 18.0 ± 1.5a | |
In vitro embryo production | |||
Cleavage rate | 72.8%a | 84.5%b | |
Embryo rate | 33.4%a | 48.2%b | |
Embryos per OPU | 5.5 ± 0.9a | 8.9 ± 1.0b |
Adapted from Hayden et al. (2022).
Different lowercase superscript letters (a,b) within a row indicates differences between treatments (P < 0.05).
Assessment of the adequate FSH dosage administered during superstimulation for MOET indicates a dose-dependent increase in superovulatory response (i.e. number of ovulatory sized follicles or CL), albeit increases beyond 300 or 400 mg (525 or 700 IU) of the commercial porcine FSH Folltropin are without effect (Gonzalez et al. 1990; Souza et al. 2007; Krause et al. 2020). Furthermore, the use of large FSH doses (≥400 mg) during MOET appear to have detrimental effects on oocyte developmental competence, as evidenced by reduced fertilization and embryo development rates (Gonzalez et al. 1990; Souza et al. 2007). The use of FSH before OPU/IVEP, however, presents some major differences which typically include a smaller total dosage (see Table 2). Surprisingly, there is a paucity of research on the effect of FSH dose on OPU/IVEP outcomes. For example, Meintjes et al. (1995) observed that increasing the dose of FSH (FSH-P, Schering-Plough Animal Health) in pregnant beef cows was accompanied by numerical increases in number of follicles and COCs, as well as retrieval efficacy and cleavage rate, albeit these were not significant likely due to the small sample size. Although FSH-P is no longer commercially available, these preliminary results suggested the potential for a dose-dependent effect of FSH.
Our research group recently conducted a series of studies to assess the effect of FSH dose on IVEP in pregnant Holstein heifers using the commercial porcine FSH Folltropin (Hayden et al. 2021, 2023). The number of total follicles, total COCs, and viable COCs, as well as cleavage and embryo development rates increased in a linear dose-dependent manner (Fig. 3), while FSH was without effect on COC retrieval efficacy and COC viability. Interestingly, there was a quadratic effect of FSH dose on the number and proportion of medium and large sized follicles, suggesting the presence of a superstimulatory threshold. Nevertheless, the greater oocyte yield and developmental competence led to a dose-dependent increase in embryo yield (Fig. 3). In addition, the probability of obtaining at least two embryos per OPU procedure increased linearly with FSH dose, such that administration of 300 mg of FSH (525 IU) led to ~90% of donors producing at least two embryos (Hayden et al. 2023). In another study, administration of 300 mg of FSH resulted in a significantly greater number of follicles (24.3 ± 0.9 vs 22.3 ± 0.7), total COCs (14.7 ± 1.0 vs 12.8 ± 0.7), and embryos (3.6 ± 0.7 vs 2.7 ± 0.4) compared to administration of 160 mg (280 IU) of FSH (Hayden et al. 2021). Conversely, Vieira et al. (2016) reported a tendency for fewer viable COCs and fewer embryos with 300 mg as opposed to 200 mg of FSH (350 IU) in Holstein cows; however, a single administration of FSH in a slow releasing formulation was used. These results taken together provide strong evidence for an FSH dose-dependent increase in IVEP efficacy, at least when used in a multiple administration schedule.
Total number of follicles (a), total number of cumulus–oocyte complex (COC; b), embryo rate (number of viable embryos/viable COC; c), and number of viable embryos (d) after OPU/IVEP in pregnant heifers administered 0, 160, or 300 mg (0, 280, or 525 IU) of porcine FSH (Folltropin, Vetoquinol USA). Trt, treatment effect. Adapted from Hayden et al. (2023).
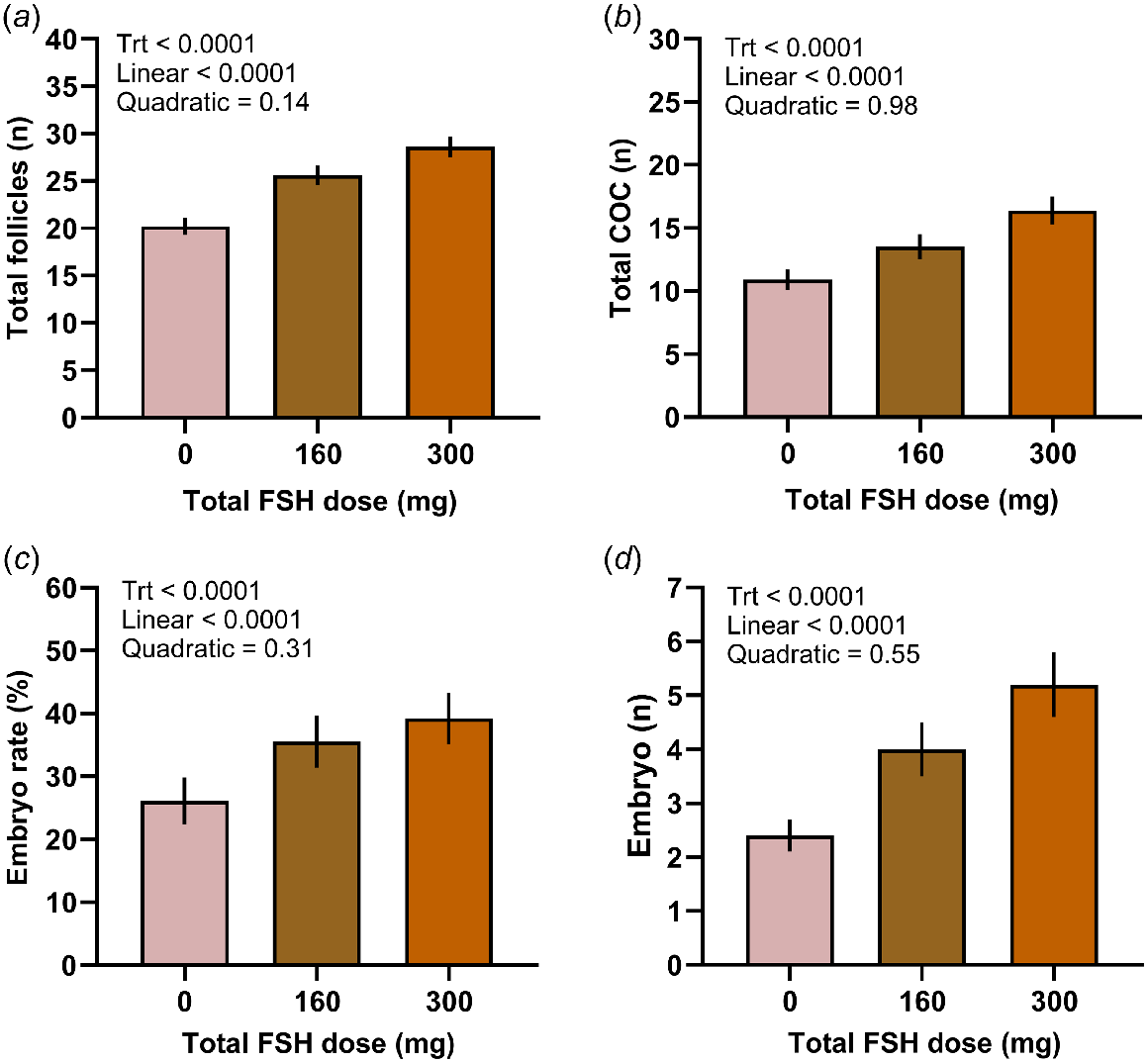
Multiple administrations of FSH, typically at 12-h intervals, are necessary to sustain concentrations required for ovarian superstimulation owing to the short (~5 h) half-life of porcine FSH extracts (Demoustier et al. 1988). Furthermore, administration of FSH has often been performed using a decreasing dose schedule with the intent to replicate the physiological decrease in FSH during the follicular wave and ensure larger amounts of FSH when small, primarily FSH-dependent growing follicles are present. Comparisons of the effects of decreasing and constant dose schedules on superovulatory response and embryo production during MOET, however, have led to conflicting results. While some studies reported similar superstimulatory responses between constant and increasing dose schedules (Bellows et al. 1969; Mapletoft and Murphy 1988), others have reported greater response with the use of a decreasing dose schedule (Chupin and Procureur 1983; Folchini et al. 2022; Sola et al. 2023a). Nevertheless, dose schedule appears to be consistently without effect on embryo production (Chupin and Procureur 1983; Donaldson et al. 1986; Mapletoft and Murphy 1988; Folchini et al. 2022; Sola et al. 2023a).
During OPU/IVEP, superstimulatory treatments are shorter, attainment of ovulatory capacity is not required, and oocyte maturation is performed in vitro, highlighting the need for assessment of FSH dose schedule in the specific context of OPU/IVEP. In this regard, Sirard et al. (1999) observed no differences in the number of follicles or COCs, or oocyte viability following administration of FSH using a constant or decreasing dose schedule. Conversely, embryo yield after a 48-h coasting period was ~4-fold greater when using the constant dose schedule, suggesting enhanced developmental competence. It is noteworthy, however, that the total dose of the commercial porcine FSH Folltropin was greater for the constant (360 mg) than the decreasing (240 mg) dose schedule, which could explain the observed differences in embryo yield. Recently our research group evaluated the effect of dose schedule (decreasing, constant, or increasing) on OPU/IVEP outcomes in pregnant heifers receiving 200 mg (350 IU) of the commercial porcine FSH Folltropin distributed in six administrations (Motta et al. 2022). Despite a tendency for a greater total number of COCs when utilizing the increasing dose schedule, ovarian response, COC retrieval efficacy, COC viability, and developmental competence did not differ. Consequently, embryo production was similar for heifers in the decreasing (6.0 ± 1.0), constant (5.7 ± 0.9), and increasing (6.0 ± 0.7) dose schedules. Thus, the particular choice of dose schedule utilized during FSH administration does not appear to affect OPU/IVEP efficacy, albeit a constant schedule may be preferable to minimize errors during administration.
Superstimulatory treatments for MOET typically involve multiple FSH administrations over 4–5 days (i.e. 8–10 administrations) to maintain appropriate concentrations of FSH to sustain follicle growth and allow multiple follicles to achieve ovulatory capacity (Donaldson 1984; Adams et al. 1993; Bó et al. 1994). Conversely, ovarian superstimulation regimens for OPU/IVEP are typically 2–3 days in duration (i.e. 4–6 FSH administrations), for the reasons previously mentioned. A series of recent experiments compared ovarian response and IVEP after superstimulation using a 2- or 3-day FSH regimen in non-lactating Holstein cows (da Silva et al. 2017) and heifers (Motta et al. 2021). The duration of FSH administration had no effect on the total number of follicles (Fig. 4). Although heifers receiving the 3-day regimen had more large sized follicles, and cows receiving the 2-day regimen tended to have more medium sized follicles, these differences did not affect COC retrieval efficacy, number of COCs retrieved, COC viability, oocyte developmental competence, or embryo production (Fig. 4). Collectively, these data suggest that 2- or 3-day FSH regimens result in similar IVEP efficacy, albeit a 2-day regimen may be preferable to minimize animal handling and associated labor.
Total number of follicles (a), total number of cumulus–oocyte complex (COC; b), COC recovery efficacy (total COC/total follicle; c), viable COC percentage (number of viable COC/total COC; d), embryo rate (number of viable embryos/viable COC; e), and number of embryos (f) after OPU/IVEP in Holstein cows and heifers administered 200 mg (350 IU) of porcine FSH (Folltropin, Vetoquinol USA) distributed in 4 (2-day) or 6 (3-day) administrations. Adapted from da Silva et al. (2017) and Motta et al. (2021).
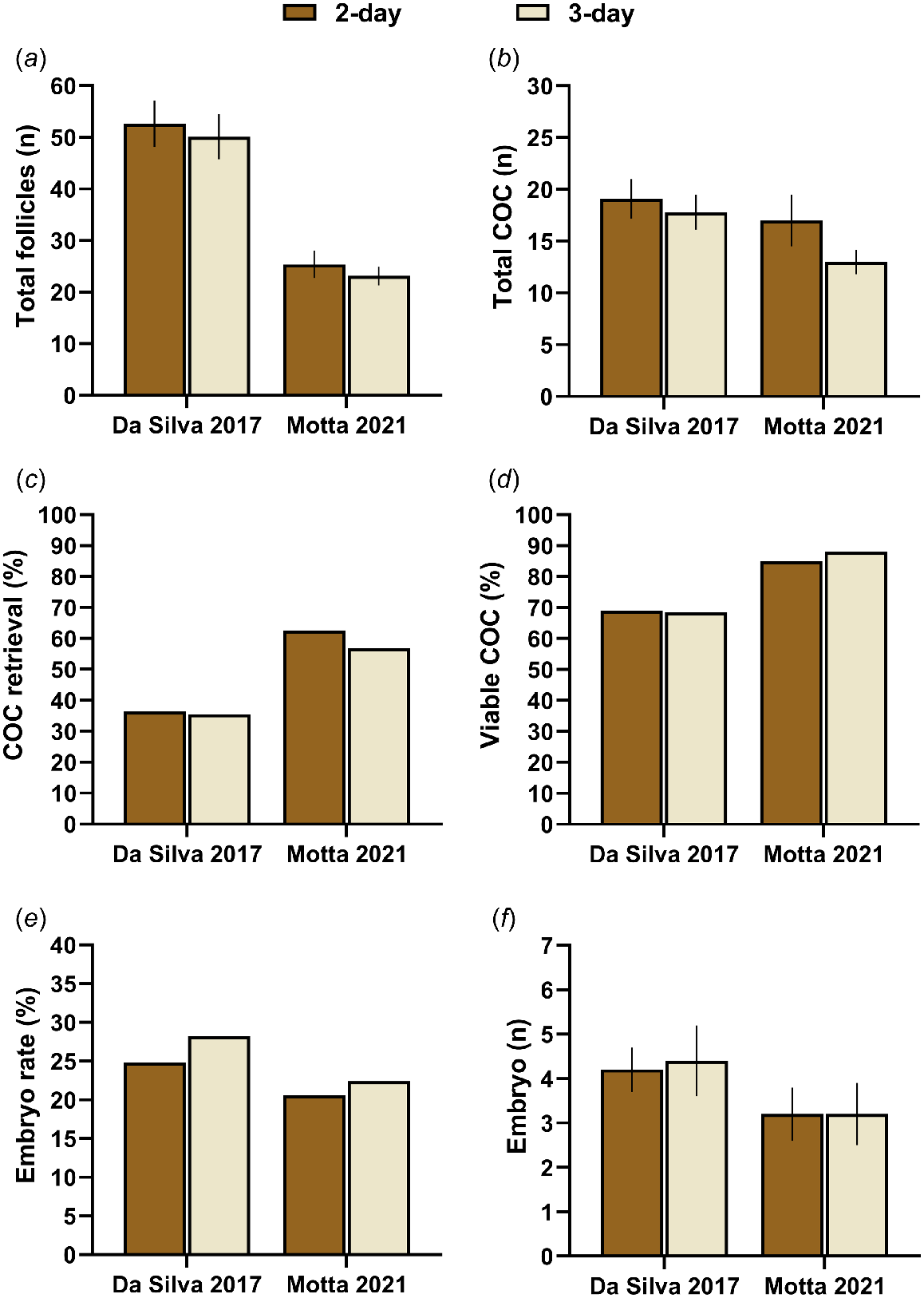
Lengthening the superstimulatory treatment regimen beyond the typical duration has been investigated in the context of MOET as a potential strategy to increase the number of follicles recruited and, thus, superstimulatory response and embryo yield. Even though lengthening the superstimulation regimen from 4 to 7 days did not result in the recruitment of more follicles, it provided sufficient time for small antral follicles (<3 mm) to grow and acquire ovulatory capacity (García-Guerra et al. 2015). Consequently, beef cattle administered the 7-day superstimulatory regimen had more synchronous ovulations, greater superstimulatory response, and numerically greater embryo production compared to cattle submitted to a 4-day FSH treatment regimen (García-Guerra et al. 2012; Dias et al. 2013b; Krause et al. 2020). Furthermore, use of a 7-day FSH regimen with in vivo oocyte maturation in beef heifers resulted in greater superstimulatory response, a tendency for a greater number of COCs retrieved, and a greater proportion of expanded COCs with a mature (MII stage) oocyte (Dias et al. 2013c; Dadarwal et al. 2015). Although embryo development rates were not different, heifers in the 7-day regimen produced 2.5-fold more embryos than those in the 4-day regimen (Dias et al. 2013c). A contentious aspect in evaluating the effect of duration is whether to maintain a constant total FSH dose across different treatment durations, which results in different daily doses, or to maintain a constant daily dose, which leads to different total doses. Interestingly, results from a recent study suggest that the effect of regimen duration is independent of total FSH dose (Krause et al. 2020). Thus, lengthening the superstimulatory treatment regimen, although labor intensive, may provide for the opportunity to improve IVEP. Nevertheless, use of lengthened FSH regimens needs to be validated in the context of commercial IVEP procedures, which typically include in vitro rather than in vivo maturation.
The need for multiple administrations of FSH is a major hurdle for the utilization of ovarian superstimulation in commercial OPU/IVEP systems. As a result, a great deal of effort has been placed on simplified FSH regimens to reduce animal handling. One of the most well-researched strategies is the combination of FSH with biodegradable non-reactive polymers to extend FSH half-life (Bó and Mapletoft 2020). Even though various slow-release formulations have been evaluated, sodium hyaluronate known as hyaluronan, has been the most extensively investigated for both MOET and IVEP. A single administration of FSH in a 2% solution of hyaluronan led to comparable superovulatory response and embryo production as multiple FSH administrations during MOET; however, its use was deemed impractical due to the high viscosity of the solution (Tríbulo et al. 2011). Alternatively, replacement of the traditional 4-day multiple FSH administrations in saline with two administrations of FSH in a 0.5% solution of hyaluronan 48 h apart, resulted in comparable or, at times, greater superovulatory response and embryo production in Bos taurus beef cattle during MOET (Tríbulo et al. 2012; Biancucci et al. 2016). Furthermore, the reduction in the number of FSH administrations was associated with reduced hair cortisol concentrations, suggesting lesser stress (Biancucci et al. 2016).
The relatively short duration of FSH regimens for OPU/IVEP suggest that a single FSH administration in 0.5% hyaluronan might be sufficient. However, circulating concentrations of FSH remain above basal concentrations for a shorter period of time (~32 vs ~55 h) after a single administration of FSH in 0.5% hyaluronan than after a multiple 2-day administration regimen (Vieira et al. 2016). Nevertheless, multiple studies (Fig. 5) have been conducted to determine the efficacy of this strategy relative to the multiple administration regimen in Bos taurus lactating dairy cows (Ongaratto et al. 2010), non-lactating dairy cows (Vieira et al. 2016), beef cows (Ongaratto et al. 2020), cyclic dairy heifers (Demetrio et al. 2021), and pregnant dairy heifers (Sala et al. 2024). Results from most of these studies indicate that a single administration of FSH in 0.5% hyaluronan results in comparable ovarian response, number of COCs retrieved, COC viability, oocyte developmental competence, and embryo production than a multiple FSH administration regimen (Ongaratto et al. 2010; Vieira et al. 2016; Ongaratto et al. 2020; Demetrio et al. 2021). Moreover, a single administration of FSH in 0.5% hyaluronan has been shown to result in greater IVEP efficacy in Bos taurus cattle when compared to a non-stimulated approach in most studies conducted to date (Vieira et al. 2016; Ongaratto et al. 2020; Santos et al. 2021). Conversely, we recently observed reduced embryo production after use of a single FSH administration in 0.5% hyaluronan in pregnant heifers (Sala et al. 2024). Although total number of follicles and developmental competence were not different, a single administration of FSH resulted in a lesser number of COCs retrieved, explaining the lesser embryo yield. The conflicting results obtained by our research group are intriguing and may be due to physiological differences attributed to pregnancy or the implementation of a shorter (~50 vs ~72 h) coasting period for the single administration regimen. However, a recent study reported similar number of follicles, COCs retrieved, and embryo yield after a single FSH administration with a coasting period of 48 or 72 h (Sola et al. 2023b). Collectively, the available evidence suggests that a single administration of FSH in 0.5% hyaluronan is a suitable and simple superstimulation strategy for use during OPU/IVEP.
Total number of follicles (a), total number of cumulus–oocyte complex (COC; b), embryo rate (number of viable embryos/viable COC; c), and number of embryos (d) after OPU/IVEP reported by studies evaluating the effect of delivering porcine FSH (Folltropin, Vetoquinol USA) using multiple administrations diluted in saline (Multiple FSH) or a single administration diluted in 0.5% hyaluronan (Single FSH). Different lowercase superscript letters (a,b) within a study indicate differences between treatment groups (P < 0.05). Adapted from Ongaratto et al. (2010), Vieira et al. (2016), Ongaratto et al. (2020), Demetrio et al. (2021) and Sala et al. (2024).
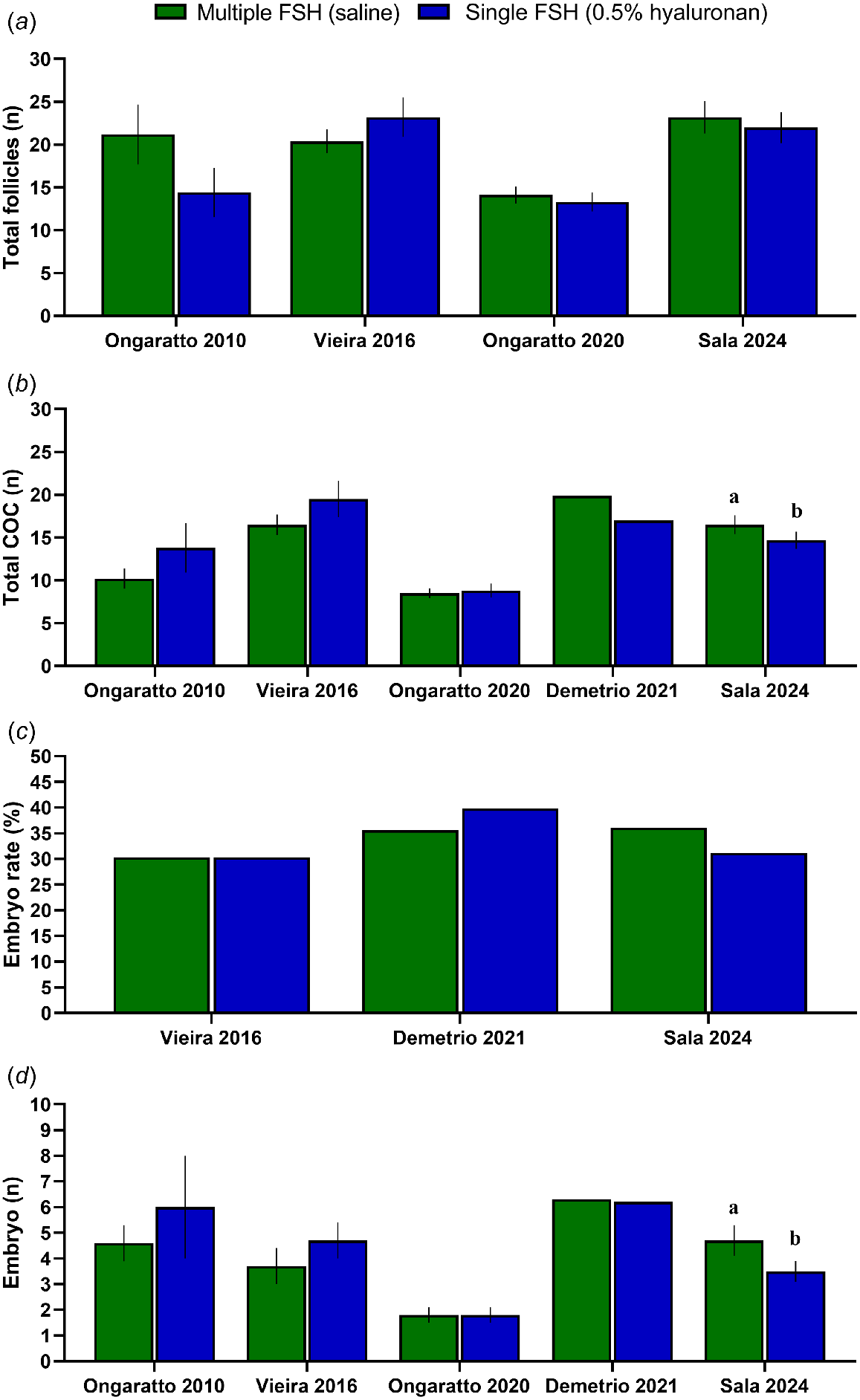
Despite the short half-life of FSH, the continuous search for simplified ovarian superstimulation treatments led also to the evaluation of a single administration of FSH diluted in saline. For example, a single subcutaneous administration of FSH diluted in saline and the traditional multiple FSH administration regimen resulted in a comparable number of follicles, viable COCs, and transferable embryos after OPU/IVEP crossbred cows (Goodhand et al. 2000). The use of a single subcutaneous administration of FSH has been extensively investigated in the context of MOET and found to have variable efficacy (reviewed in Bó and Mapletoft 2020). Such variability was associated with site of administration and body condition score making this strategy less suitable for widespread commercial application. More recently, the use of a single intramuscular administration of FSH diluted in saline has been utilized during OPU/IVEP. Single administration of 60 mg of FSH (Folltropin) diluted in saline after synchronization of follicular wave emergence in Bos indicus dairy cows was found to improve embryo production compared to non-FSH treated cows (Cedeño et al. 2023; Ocampo et al. 2024). The efficacy of this approach, however, appears to be inferior compared to both the traditional multiple administration regimen and the use of a single administration in 0.5% hyaluronan as recently reported by our research group in pregnant heifers (Sala et al. 2024). Total number of follicles was not different; however, use of a single administration of FSH in saline resulted in fewer medium to large sized follicles, fewer COCs, reduced proportion of viable COCs, and reduced oocyte developmental competence, all of which led to fewer embryos (Sala et al. 2024). Although single administration of FSH in saline may offer superior IVEP efficacy compared to non-stimulated approaches, the traditional multiple administration FSH regimen is more efficacious.
The future of ovarian superstimulation: at the crossroads of ovarian phenotype and targeted FSH administration
Substantial evidence, as reviewed herein, supports the utilization of ovarian superstimulation before OPU as a method to enhance the efficacy of IVEP in cattle. As a result, a great deal of effort has been placed in the optimization of FSH-based superstimulation treatment regimens with the goal of enhancing IVEP efficacy and/or simplify their implementation. Individual animal variation in embryo production, however, remains a limitation for embryo production. Although AFC/AMH phenotype largely explains the observed variability, its use for selection of females with enhanced ovarian response and embryo production has been somewhat limited, as previously discussed in the present review. Nevertheless, the marked physiological differences associated with distinct AFC/AMH phenotypes, particularly in terms of follicular responsiveness to gonadotropin stimulation, highlights the potential for AFC/AMH phenotype to be used in the development of targeted ovarian superstimulation treatment regimens with the goal of increasing IVEP efficacy. The notion of targeted ovarian superstimulation treatment regimens is not entirely new, and is often used by practitioners; however, it is typically based on individual animal response to previous superstimulation procedures, which can be influenced by environmental and technical factors (De Roover et al. 2005). Conversely, the use of AMH/AFC phenotype can provide a physiological and more consistent approach for the development of targeted ovarian superstimulation regimens that optimize IVEP in an economically efficient manner. Accordingly, a series of recent studies in ruminants have explored the effect of alterations in FSH treatment regimens for both MOET and OPU/IVEP in the context of specific AFC/AMH phenotypes.
The assessment of differential effects of FSH dose among females with low and high AFC/AMH appears to be a logical starting point, considering that low AFC/AMH individuals have chronically elevated FSH concentrations and reduced responsiveness of granulosa cells to FSH stimulation (Burns et al. 2005; Scheetz et al. 2012). Administration of a total dose of 960 mg (1680 IU) of the commercial porcine FSH Folltropin, as opposed to 320 mg (560 IU), during a traditional 4-day regimen for MOET in low AFC heifers resulted in ovulatory sized follicles with altered endocrine characteristics and diminished ovulatory capacity suggestive of premature luteinization (Karl et al. 2021; Clark et al. 2022a). Although the number of COCs retrieved was not affected, excessive FSH resulted in a greater proportion of follicles containing expanded COCs which resumed meiosis prematurely and responded poorly to IVEP (Karl et al. 2023). Furthermore, transcriptome analysis of oocyte, cumulus, and granulosa cells indicated dysregulation of maturational processes including those involved in cumulus expansion and regulation of meiotic resumption (Clark et al. 2022b; Karl et al. 2023). Collectively, these results indicate that the use of large FSH doses in low AFC/AMH individuals may lead to reduced IVEP efficacy, even though the dosage utilized in these studies was ~3-fold greater than the largest dosage used in current FSH treatment regimens for OPU/IVEP. Recently, our research group evaluated the effect of three different FSH doses (0, 160, or 300 mg) on OPU/IVEP outcomes in high AFC/AMH pregnant heifers (Motta et al. 2023). Preliminary results indicate that total number of follicles, number of COCs, and embryo development rate increase linearly with increasing FSH dose. Consequently, embryo production increased in a dose-dependent manner, in agreement with results obtained from heifers with unknown AFC/AMH phenotype (Hayden et al. 2023). Even though these findings provide support for the use of relatively large doses of FSH in high AFC/AMH donors, two questions remain unanswered: (1) what is the effect of commercially utilized FSH doses on IVEP in low AFC/AMH donors; and (2) what is the effect of larger FSH doses on IVEP in high AFC/AMH donors?
As previously mentioned, lengthening the administration of FSH from 4 to 7 days in beef cows improves superstimulatory response during MOET (García-Guerra et al. 2012; Dias et al. 2013c). This has been attributed to the development of follicles at a growth rate that resembles that observed under non-stimulated conditions and the greater time available for follicles to mature and acquire ovulatory capacity (García-Guerra et al. 2015). The previously discussed differences in granulosa cell responsiveness to FSH stimulation, alongside the detrimental effects of excessively large FSH doses in low AFC/AMH cattle, suggest that reducing the daily dose may be beneficial for low AFC/AMH individuals. Accordingly, lengthening the duration of FSH administration while maintaining the total FSH dose leads to a smaller daily FSH dose. Consequently, our research group recently evaluated the effect of FSH treatment duration on ovarian response and embryo yield during MOET in low and high AMH ewes (Brochado et al. 2024). Although superovulatory response was similar, embryo production increased 3-fold when lengthening the superstimulatory treatment from 3 to 4 days in low but not high AMH ewes. Interestingly, the greater embryo production was the result of increased fertilization and embryo development rates, indicating that extending the duration of the FSH treatment regimen enhances oocyte competence for low AMH ewes (Brochado et al. 2024). Collectively, these data suggest that females with low AFC/AMH could benefit from the use of an extended FSH treatment regimen. Nevertheless, the effects of lengthening the ovarian superstimulation regimen in low or high AFC/AMH females during OPU-IVEP remain to be elucidated.
The development of targeted ovarian superstimulation regimens for OPU/IVEP based on AFC/AMH phenotype certainly holds an inherent appeal. Results obtained thus far are promising and suggest that ovarian superstimulation approaches can be optimized in the context of AFC/AMH phenotype. The ability of AFC/AMH phenotype to inform the selection of a particular FSH treatment regimen has significant practical value, as it can lead to optimization of IVEP outcomes while exercising judicious use of FSH, a costly resource. Nevertheless, widespread application of this approach requires further research to assess the precise effects of various FSH treatment regimens in cattle with differing AFC/AMH phenotype.
Data availability
Data sharing is not applicable as no new data were generated or analyzed during this study.
Declaration of funding
This work was partially funded by the USDA National Institute of Food and Agriculture, Multi-State Hatch project 7004144 (OHO01556-MRF). We extend our gratitude to Vetoquinol USA for the generous donation of Folltropin for some of the studies presented in this manuscript.
Acknowledgements
We thank the personnel at the Ohio Heifer Center (STgenetics, South Charleston, OH, USA) and Volm’s farm (STgenetics, Kewaskum, WI, USA) for assistance with management and animal handling for some of the studies presented in this manuscript.
References
Adams GP, Kot K, Smith CA, Ginther OJ (1993) Selection of a dominant follicle and suppression of follicular growth in heifers. Animal Reproduction Science 30, 259-271.
| Crossref | Google Scholar |
Adams GP, Nasser LF, Bó GA, Garcia A, Del Campo MR, Mapletoft RJ (1994) Superovulatory response of ovarian follicles of Wave 1 versus Wave 2 in heifers. Theriogenology 42, 1103-1113.
| Crossref | Google Scholar | PubMed |
Baldrighi JM, Sá Filho MF, Batista EOS, Lopes RNVR, Visintin JA, Baruselli PS, Assumpção MEOA (2014) Anti-Mullerian hormone concentration and antral ovarian follicle population in Murrah heifers compared to Holstein and Gyr kept under the same management. Reproduction in Domestic Animals 49, 1015-1020.
| Crossref | Google Scholar | PubMed |
Baruselli PS, Batista EOS, Vieira LM, Ferreira RM, Guerreiro BG, Bayeux BM, Sales JNS, Souza AH, Gimenes LU (2016) Factors that interfere with oocyte quality for in vitro production of cattle embryos: effects of different developmental & reproductive stages. Animal Reproduction 13, 264-272.
| Crossref | Google Scholar |
Baruselli PS, Rodrigues CA, Ferreira RM, Sales JNS, Elliff FM, Silva LG, Viziack MP, Factor L, D’Occhio MJ (2022) Impact of oocyte donor age and breed on in vitro embryo production in cattle, and relationship of dairy and beef embryo recipients on pregnancy and the subsequent performance of offspring: a review. Reproduction, Fertility and Development 34, 36-51.
| Crossref | Google Scholar |
Batista EOS, Macedo GG, Sala RV, Ortolan MDDV, Sá Filho MF, Del Valle TA, Jesus EF, Lopes RNVR, Rennó FP, Baruselli PS (2014) Plasma antimüllerian hormone as a predictor of ovarian antral follicular population in Bos indicus (Nelore) and Bos taurus (Holstein) heifers. Reproduction in Domestic Animals 49, 448-452.
| Crossref | Google Scholar | PubMed |
Batista EOS, Guerreiro BM, Freitas BG, Silva JCB, Vieira LM, Ferreira RM, Rezende RG, Basso AC, Lopes RNVR, Rennó FP, Souza AH, Baruselli PS (2016) Plasma anti-Müllerian hormone as a predictive endocrine marker to select Bos taurus (Holstein) and Bos indicus (Nelore) calves for in vitro embryo production. Domestic Animal Endocrinology 54, 1-9.
| Crossref | Google Scholar | PubMed |
Bellows RA, Anderson DC, Short RE (1969) Dose-response relationships in synchronized beef heifers treated with follicle stimulating hormone. Journal of Animal Science 28, 638-644.
| Crossref | Google Scholar | PubMed |
Biancucci A, Sbaragli T, Comin A, Sylla L, Monaci M, Peric T, Stradaioli G (2016) Reducing treatments in cattle superovulation protocols by combining a pituitary extract with a 5% hyaluronan solution: is it able to diminish activation of the hypothalamic pituitary adrenal axis compared to the traditional protocol? Theriogenology 85, 914-921.
| Crossref | Google Scholar | PubMed |
Blondin P, Coenen K, Guilbault LA, Sirard M-A (1996) Superovulation can reduce the developmental competence of bovine embryos. Theriogenology 46, 1191-1203.
| Crossref | Google Scholar | PubMed |
Blondin P, Coenen K, Guilbault LA, Sirard M-A (1997) In vitro production of bovine embryos: developmental competence is acquired before maturation. Theriogenology 47, 1061-1075.
| Crossref | Google Scholar | PubMed |
Blondin P, Bousquet D, Twagiramungu H, Barnes F, Sirard M-A (2002) Manipulation of follicular development to produce developmentally competent bovine oocytes. Biology of Reproduction 66, 38-43.
| Crossref | Google Scholar | PubMed |
Bó GA, Mapletoft RJ (2020) Superstimulation of ovarian follicles in cattle: gonadotropin treatment protocols and FSH profiles. Theriogenology 150, 353-359.
| Crossref | Google Scholar | PubMed |
Bó GA, Hockley DK, Nasser LF, Mapletoft RJ (1994) Superovulatory response to a single subcutaneous injection of Folltropin-V in beef cattle. Theriogenology 42, 963-975.
| Crossref | Google Scholar | PubMed |
Boni R (2012) Ovum pick-up in cattle: a 25 yr retrospective analysis. Animal Reproduction 9, 362-369.
| Google Scholar |
Braw-Tal R, Yossefi S (1997) Studies in vivo and in vitro on the initiation of follicle growth in the bovine ovary. Journal of Reproduction and Fertility 109, 165-171.
| Crossref | Google Scholar | PubMed |
Brochado C, Duran BJ, Kieffer JD, Pinczak A, Menchaca A, Garcia-Guerra A (2024) Reduced embryo yield obtained from superstimulated ewes with low circulating AMH concentration is improved by lengthening the FSH treatment. Theriogenology 218, 79-88.
| Crossref | Google Scholar | PubMed |
Brogliatti GM, Salamone DF, Adams GP (1997) Ovarian follicular wave synchronization and superstimulation in prepubertal calves. Theriogenology 47, 1253-1264.
| Crossref | Google Scholar | PubMed |
Bungartz L, Lucas-Hahn A, Rath D, Niemann H (1995) Collection of oocytes from cattle via follicular aspiration aided by ultrasound with or without gonadotropin pretreatment and in different reproductive stages. Theriogenology 43, 667-675.
| Crossref | Google Scholar | PubMed |
Burns DS, Jimenez-Krassel F, Ireland JLH, Knight PG, Ireland JJ (2005) Numbers of antral follicles during follicular waves in cattle: evidence for high variation among animals, very high repeatability in individuals, and an inverse association with serum follicle-stimulating hormone concentrations. Biology of Reproduction 73, 54-62.
| Crossref | Google Scholar | PubMed |
Caixeta ES, Ripamonte P, Franco MM, Junior JB, Dode MAN (2009) Effect of follicle size on mRNA expression in cumulus cells and oocytes of Bos indicus: an approach to identify marker genes for developmental competence. Reproduction, Fertility and Development 21, 655-664.
| Crossref | Google Scholar | PubMed |
Cardoso CJT, de Oliveira Junior JS, Kischel H, da Silva WAL, da Silva Arruda ED, Souza-Cáceres MB, de Oliveira FAM, Nogueira É, Nogueira GdP, Melo-Sterza FdA (2018) Anti-Müllerian hormone (AMH) as a predictor of antral follicle population in heifers. Animal Reproduction 15, 12-16.
| Crossref | Google Scholar | PubMed |
Cavalieri FLB, Morotti F, Seneda MM, Colombo AHB, Andreazzi MA, Emanuelli IP, Rigolon LP (2018) Improvement of bovine in vitro embryo production by ovarian follicular wave synchronization prior to ovum pick-up. Theriogenology 117, 57-60.
| Crossref | Google Scholar | PubMed |
Cedeño AV, Bernal B, Pinargote L, Ocampo V, Mendoza B, Bó GA (2023) Effect of follicle wave synchronisation and follicle stimulating hormone treatment on in vitro embryo production in Bos indicus (Gyr) donors. Reproduction, Fertility and Development 35, 192.
| Crossref | Google Scholar |
Center K, Dixon D, Looney C, Rorie R (2018) Anti-Mullerian hormone and follicle counts as predictors of superovulatory response and embryo production in beef cattle. Advances in Reproductive Sciences 06, 22-33.
| Crossref | Google Scholar |
Chaubal SA, Molina JA, Ohlrichs CL, Ferre LB, Faber DC, Bols PEJ, Riesen JW, Tian X, Yang X (2006) Comparison of different transvaginal ovum pick-up protocols to optimise oocyte retrieval and embryo production over a 10-week period in cows. Theriogenology 65, 1631-1648.
| Crossref | Google Scholar | PubMed |
Chu T, Dufort I, Sirard M-A (2012) Effect of ovarian stimulation on oocyte gene expression in cattle. Theriogenology 77, 1928-1938.
| Crossref | Google Scholar | PubMed |
Chupin D, Procureur R (1983) Efficiency of pituitary extracts (FSH) for induction of superovulation in cattle. Animal Reproduction Science 6, 11-23.
| Crossref | Google Scholar |
Clark ZL, Karl KR, Ruebel ML, Latham KE, Ireland JJ (2022a) Excessive follicle-stimulating hormone during ovarian stimulation of cattle may induce premature luteinization of most ovulatory-size follicles. Biology of Reproduction 106, 968-978.
| Crossref | Google Scholar | PubMed |
Clark ZL, Ruebel ML, Schall PZ, Karl KR, Ireland JJ, Latham KE (2022b) Follicular hyperstimulation dysgenesis: new explanation for adverse effects of excessive FSH in ovarian stimulation. Endocrinology 163, bqac100.
| Crossref | Google Scholar |
Dadarwal D, Honparkhe M, Dias FCF, Alce T, Lessard C, Singh J (2015) Effect of superstimulation protocols on nuclear maturation and distribution of lipid droplets in bovine oocytes. Reproduction, Fertility and Development 27, 1137-1146.
| Crossref | Google Scholar | PubMed |
Dantas FG, Reese ST, Filho RVO, Carvalho RS, Franco GA, Abbott CR, Payton RR, Edwards JL, Russell JR, Smith JK, Pohler KG (2019) Effect of complexed trace minerals on cumulus-oocyte complex recovery and in vitro embryo production in beef cattle. Journal of Animal Science 97, 1478-1490.
| Crossref | Google Scholar | PubMed |
da Silva JCB, Ferreira RM, Maturana FM, Naves JdR, Santin T, Pugliesi G, Madureira EH (2017) Use of FSH in two different regimens for ovarian superstimulation prior to ovum pick up and in vitro embryo production in Holstein cows. Theriogenology 90, 65-73.
| Crossref | Google Scholar | PubMed |
Demetrio DGB, Benedetti E, Demetrio CGB, Fonseca J, Oliveira M, Magalhaes A, Dos Santos RM (2020) How can we improve embryo production and pregnancy outcomes of Holstein embryos produced in vitro? (12 years of practical results at a California dairy farm). Animal Reproduction 17, e20200053.
| Crossref | Google Scholar | PubMed |
Demetrio DGB, Hasler JF, Oliveira M, Demetrio CGB, Fonseca JC, Santos RM (2021) Comparison of single to multiple injections of follicle-stimulating hormone before ovum pickup in Holstein heifers: oocyte recovery and embryo production. Reproduction, Fertility and Development 33, 180-181.
| Crossref | Google Scholar |
Demoustier MM, Beckers J-F, Van der Zwalmen P, Closset J, Gillard J-L, Ectors F (1988) Determination of porcine plasma follitropin levels during superovulation treatment in cows. Theriogenology 30, 379-386.
| Crossref | Google Scholar | PubMed |
De Roover R, Genicot G, Leonard S, Bols P, Dessy F (2005) Ovum pick up and in vitro embryo production in cows superstimulated with an individually adapted superstimulation protocol. Animal Reproduction Science 86, 13-25.
| Crossref | Google Scholar | PubMed |
De Roover R, Feugang JMN, Bols PEJ, Genicot G, Hanzen C (2008) Effects of ovum pick-up frequency and FSH stimulation: a retrospective study on seven years of beef cattle in vitro embryo production. Reproduction in Domestic Animals 43, 239-245.
| Crossref | Google Scholar | PubMed |
Dias FCF, Khan MIR, Sirard MA, Adams GP, Singh J (2013a) Differential gene expression of granulosa cells after ovarian superstimulation in beef cattle. Reproduction 146, 181-191.
| Crossref | Google Scholar | PubMed |
Dias FCF, Costa E, Adams GP, Mapletoft RJ, Kastelic J, Dochi O, Singh J (2013b) Effect of duration of the growing phase of ovulatory follicles on oocyte competence in superstimulated cattle. Reproduction, Fertility and Development 25, 523-530.
| Crossref | Google Scholar | PubMed |
Dias FCF, Dadarwal D, Adams GP, Mrigank H, Mapletoft RJ, Singh J (2013c) Length of the follicular growing phase and oocyte competence in beef heifers. Theriogenology 79, 1177-1183.e1.
| Crossref | Google Scholar | PubMed |
Dickson MJ, Piersanti RL, Ramirez-Hernandez R, de Oliveira EB, Bishop JV, Hansen TR, Ma Z, Jeong KCC, Santos JEP, Sheldon MI, Block J, Bromfield JJ (2020) Experimentally induced endometritis impairs the developmental capacity of bovine oocytes. Biology of Reproduction 103, 508-520.
| Crossref | Google Scholar | PubMed |
Donaldson LE (1984) Dose of FSH-P as a source of variation in embryo production from superovulated cows. Theriogenology 22, 205-212.
| Crossref | Google Scholar | PubMed |
Donaldson LE, Ward DN, Glenn SD (1986) Use of porcine follicle stimulating hormone after chromatographic purification in superovulation of cattle. Theriogenology 25, 747-757.
| Crossref | Google Scholar |
Egashira J, Ihara Y, Khatun H, Wada Y, Konno T, Tatemoto H, Yamanaka K-I (2019) Efficient in vitro embryo production using in vivo-matured oocytes from superstimulated Japanese Black cows. Journal of Reproduction and Development 65, 183-190.
| Crossref | Google Scholar | PubMed |
Elliff FM, Guimarães EC, Féres LF, Bayeux BM, Colli MHA, Sampaio Baruselli PS (2019) Effect of treatment with follicle-stimulating hormone on in vitro embryo production of Gyr (Bos indicus) calves, pubertal heifers and adult cows. Reproduction, Fertility and Development 31, 191.
| Crossref | Google Scholar |
Fair T, Hyttel P, Greve T (1995) Bovine oocyte diameter in relation to maturational competence and transcriptional activity. Molecular Reproduction and Development 42, 437-442.
| Crossref | Google Scholar | PubMed |
Ferré LB, Kjelland ME, Strøbech LB, Hyttel P, Mermillod P, Ross PJ (2020) Review: Recent advances in bovine in vitro embryo production: reproductive biotechnology history and methods. Animal 14, 991-1004.
| Crossref | Google Scholar | PubMed |
Folchini N, Silva L, Alves R, Consentini C, Silva M, Silva T, Acosta-Galindez J, Drum J, Motta J, Gonzales B, Sartori R (2022) Ovarian response and embryo production of cows superstimulated with different FSH regimens and inseminated with conventional or sex-sorted spermatozoa. Reproduction, Fertility and Development 34, 322.
| Crossref | Google Scholar | PubMed |
García-Guerra A, Tribulo A, Yapura J, Singh J, Mapletoft RJ (2012) Lengthening the superstimulatory treatment protocol increases ovarian response and number of transferable embryos in beef cows. Theriogenology 78, 353-360.
| Crossref | Google Scholar | PubMed |
García-Guerra A, Tribulo A, Yapura J, Adams GP, Singh J, Mapletoft RJ (2015) Lengthened superstimulatory treatment in cattle: evidence for rescue of follicles within a wave rather than continuous recruitment of new follicles. Theriogenology 84, 467-476.
| Crossref | Google Scholar | PubMed |
García-Guerra A, Motta JCL, Melo LF, Kirkpatrick BW, Wiltbank MC (2017) Ovulation rate, antral follicle count, and circulating anti-Müllerian hormone in Trio allele carriers, a novel high fecundity bovine genotype. Theriogenology 101, 81-90.
| Crossref | Google Scholar | PubMed |
García-Guerra A, Wiltbank MC, Battista SE, Kirkpatrick BW, Sartori R (2018) Mechanisms regulating follicle selection in ruminants: lessons learned from multiple ovulation models. Animal Reproduction 15, 660-679.
| Crossref | Google Scholar | PubMed |
García-Ruiz A, Cole JB, VanRaden PM, Wiggans GR, Ruiz-López FJ, Tassell CPV (2016) Changes in genetic selection differentials and generation intervals in US Holstein dairy cattle as a result of genomic selection. Proceedings of the National Academy of Sciences 113, E3995-E4004.
| Crossref | Google Scholar |
Gibbons JR, Wiltbank MC, Ginther OJ (1997) Functional interrelationships between follicles greater than 4 mm and the follicle-stimulating hormone surge in heifers. Biology of Reproduction 57, 1066-1073.
| Crossref | Google Scholar | PubMed |
Gimenes LU, Ferraz ML, Fantinato-Neto P, Chiaratti MR, Mesquita LG, Sá Filho MF, Meirelles FV, Trinca LA, Rennó FP, Watanabe YF, Baruselli PS (2015) The interval between the emergence of pharmacologically synchronized ovarian follicular waves and ovum pickup does not significantly affect in vitro embryo production in Bos indicus, Bos taurus, and Bubalus bubalis. Theriogenology 83, 385-393.
| Crossref | Google Scholar | PubMed |
Ginther OJ, Kastelic JP, Knopf L (1989) Composition and characteristics of follicular waves during the bovine estrous cycle. Animal Reproduction Science 20, 187-200.
| Crossref | Google Scholar |
Ginther OJ, Bergfelt DR, Kulick LJ, Kot K (2000) Selection of the dominant follicle in cattle: role of two-way functional coupling between follicle-stimulating hormone and the follicles. Biology of Reproduction 62, 920-927.
| Crossref | Google Scholar | PubMed |
Gobikrushanth M, Purfield DC, Colazo MG, Butler ST, Wang Z, Ambrose DJ (2018) The relationship between serum anti-Müllerian hormone concentrations and fertility, and genome-wide associations for anti-Müllerian hormone in Holstein cows. Journal of Dairy Science 101, 7563-7574.
| Crossref | Google Scholar | PubMed |
Gonzalez A, Lussier IG, Carruthers TD, Murphy BD, Mapletoft RJ (1990) Superovulation of beef heifers with Folltropin: a new FSH preparation containing reduced LH activity. Theriogenology 33, 519-529.
| Crossref | Google Scholar | PubMed |
Goodhand KL, Watt RG, Staines ME, Hutchinson JSM, Broadbent PJ (1999) In vivo oocyte recovery and in vitro embryo production from bovine donors aspirated at different frequencies or following FSH treatment. Theriogenology 51, 951-961.
| Crossref | Google Scholar | PubMed |
Goodhand KL, Staines ME, Hutchinson JSM, Broadbent PJ (2000) In vivo oocyte recovery and in vitro embryo production from bovine oocyte donors treated with progestagen, oestradiol and FSH. Animal Reproduction Science 63, 145-158.
| Crossref | Google Scholar | PubMed |
Guerreiro BM, Batista EOS, Vieira LM, Sá Filho MF, Rodrigues CA, Castro Netto A, Silveira CRA, Bayeux BM, Dias EAR, Monteiro FM, Accorsi M, Lopes RNVR, Baruselli PS (2014) Plasma anti-mullerian hormone: an endocrine marker for in vitro embryo production from Bos taurus and Bos indicus donors. Domestic Animal Endocrinology 49, 96-104.
| Crossref | Google Scholar | PubMed |
Hagemann LJ, Beaumont SE, Berg M, Donnison MJ, Ledgard A, Peterson AJ, Schurmann A, Tervit HR (1999) Development during single IVP of bovine oocytes from dissected follicles: interactive effects of estrous cycle stage, follicle size and atresia. Molecular Reproduction and Development 53, 451-458.
| Crossref | Google Scholar | PubMed |
Hansen PJ (2006) Realizing the promise of IVF in cattle – an overview. Theriogenology 65, 119-125.
| Crossref | Google Scholar | PubMed |
Hayden C, Sala RV, Pereira DC, Carrenho-Sala LC, Fosado M, Moreno D, Moreno JF, Garcia-Guerra A (2021) Effect of follicle-stimulating hormone dose and circulating progesterone before ovum pick-up and in vitro embryo production in pregnant Holstein heifers. Reproduction, Fertility and Development 33, 143.
| Crossref | Google Scholar |
Hayden CB, Sala RV, Absalón-Medina VA, Motta JCL, Pereira D, Moreno JF, García-Guerra A (2022) Synchronization of follicle wave emergence before ovarian superstimulation with FSH and ovum pickup improves in vitro embryo production in pregnant heifers. Theriogenology 188, 71-78.
| Crossref | Google Scholar | PubMed |
Hayden CB, Sala RV, Pereira DC, Moreno JF, García-Guerra A (2023) Effect of use and dosage of p-follicle-stimulating hormone for ovarian superstimulation before ovum pick-up and in vitro embryo production in pregnant Holstein heifers. Journal of Dairy Science 106, 8110-8121.
| Crossref | Google Scholar |
Hsueh AJW, Kawamura K, Cheng Y, Fauser BCJM (2015) Intraovarian control of early folliculogenesis. Endocrine Reviews 36, 1-24.
| Crossref | Google Scholar | PubMed |
Hyttel P, Fair T, Callesen H, Greve T (1997) Oocyte growth, capacitation and final maturation in cattle. Theriogenology 47, 23-32.
| Crossref | Google Scholar |
Imai K, Inaba Y, Yoshioka H, Aikawa Y, Ohtake M, Suzuki M, Kobayashi S (2007) Effect of follicular wave synchronization and superstimulation on in vitro embryo production. Reproduction, Fertility and Development 20, 182.
| Crossref | Google Scholar |
Ireland JJ, Ward F, Jimenez-Krassel F, Ireland JLH, Smith GW, Lonergan P, Evans ACO (2007) Follicle numbers are highly repeatable within individual animals but are inversely correlated with FSH concentrations and the proportion of good-quality embryos after ovarian stimulation in cattle. Human Reproduction 22, 1687-1695.
| Crossref | Google Scholar | PubMed |
Ireland JLH, Scheetz D, Jimenez-Krassel F, Themmen APN, Ward F, Lonergan P, Smith GW, Perez GI, Evans ACO, Ireland JJ (2008) Antral follicle count reliably predicts number of morphologically healthy oocytes and follicles in ovaries of young adult cattle. Biology of Reproduction 79, 1219-1225.
| Crossref | Google Scholar | PubMed |
Ireland JJ, Smith GW, Scheetz D, Jimenez-Krassel F, Folger JK, Ireland JLH, Mossa F, Lonergan P, Evans ACO (2011) Does size matter in females? An overview of the impact of the high variation in the ovarian reserve on ovarian function and fertility, utility of anti-Müllerian hormone as a diagnostic marker for fertility and causes of variation in the ovarian reserve in cattle. Reproduction, Fertility and Development 23, 1-14.
| Crossref | Google Scholar | PubMed |
Jaiswal RS, Singh J, Adams GP (2004) Developmental pattern of small antral follicles in the bovine ovary. Biology of Reproduction 71, 1244-1251.
| Crossref | Google Scholar | PubMed |
Jimenez-Krassel F, Folger JK, Ireland JLH, Smith GW, Hou X, Davis JS, Lonergan P, Evans ACO, Ireland JJ (2009) Evidence that high variation in ovarian reserves of healthy young adults has a negative impact on the corpus luteum and endometrium during estrous cycles in cattle. Biology of Reproduction 80, 1272-1281.
| Crossref | Google Scholar | PubMed |
Karl KR, Jimenez-Krassel F, Gibbings E, Ireland JLH, Clark ZL, Tempelman RJ, Latham KE, Ireland JJ (2021) Negative impact of high doses of follicle-stimulating hormone during superovulation on the ovulatory follicle function in small ovarian reserve dairy heifers. Biology of Reproduction 104, 695-705.
| Crossref | Google Scholar | PubMed |
Karl KR, Schall PZ, Clark ZL, Ruebel ML, Cibelli J, Tempelman RJ, Latham KE, Ireland JJ (2023) Ovarian stimulation with excessive FSH doses causes cumulus cell and oocyte dysfunction in small ovarian reserve heifers. Molecular Human Reproduction 29, gaad033.
| Crossref | Google Scholar |
Knight PG, Glister C (2006) TGF-β superfamily members and ovarian follicle development. Reproduction 132, 191-206.
| Crossref | Google Scholar | PubMed |
Krause ART, Dias FCF, Adams GP, Mapletoft RJ, Singh J (2020) Effect of dose and duration of FSH treatment on ovarian response in prepubertal calves. Animal Reproduction Science 219, 106471.
| Crossref | Google Scholar | PubMed |
Landry DA, Sirard M-A (2018) Follicle capacitation: a meta-analysis to investigate the transcriptome dynamics following follicle-stimulating hormone decline in bovine granulosa cells. Biology of Reproduction 99, 877-887.
| Crossref | Google Scholar | PubMed |
Lequarre A-S, Vigneron C, Ribaucour F, Holm P, Donnay I, Dalbiès-Tran R, Callesen H, Mermillod P (2005) Influence of antral follicle size on oocyte characteristics and embryo development in the bovine. Theriogenology 63, 841-859.
| Crossref | Google Scholar | PubMed |
Lonergan P, Monaghan P, Rizos D, Boland MP, Gordon I (1994) Effect of follicle size on bovine oocyte quality and developmental competence following maturation, fertilization, and culture in vitro. Molecular Reproduction and Development 37, 48-53.
| Crossref | Google Scholar | PubMed |
Luciano AM, Sirard M-A (2018) Successful in vitro maturation of oocytes: a matter of follicular differentiation. Biology of Reproduction 98, 162-169.
| Crossref | Google Scholar | PubMed |
Machatkova M, Jokesova E, Horky F, Krepelova A (2000) Utilization of the growth phase of the first follicular wave for bovine oocyte collection improves blastocyst production. Theriogenology 54, 543-550.
| Crossref | Google Scholar | PubMed |
Machatkova M, Krausova K, Jokesova E, Tomanek M (2004) Developmental competence of bovine oocytes: effects of follicle size and the phase of follicular wave on in vitro embryo production. Theriogenology 61, 329-335.
| Crossref | Google Scholar | PubMed |
Meintjes M, Bellow MS, Broussard JR, Paul JB, Godke RA (1995) Transvaginal aspiration of oocytes from hormone-treated pregnant beef cattle for in vitro fertilization. Journal of Animal Science 73, 967-974.
| Crossref | Google Scholar | PubMed |
Merton JS, de Roos APW, Mullaart E, de Ruigh L, Kaal L, Vos PLAM, Dieleman SJ (2003) Factors affecting oocyte quality and quantity in commercial application of embryo technologies in the cattle breeding industry. Theriogenology 59, 651-674.
| Crossref | Google Scholar | PubMed |
Monniaux D (2016) Driving folliculogenesis by the oocyte-somatic cell dialog: lessons from genetic models. Theriogenology 86, 41-53.
| Crossref | Google Scholar | PubMed |
Mossa F, Ireland JJ (2019) Physiology and endocrinology symposium: anti-Müllerian hormone: a biomarker for the ovarian reserve, ovarian function, and fertility in dairy cows. Journal of Animal Science 97, 1446-1455.
| Crossref | Google Scholar | PubMed |
Mossa F, Jimenez-Krassel F, Walsh S, Berry DP, Butler ST, Folger J, Smith GW, Ireland JLH, Lonergan P, Ireland JJ, Evans ACO (2010a) Inherent capacity of the pituitary gland to produce gonadotropins is not influenced by the number of ovarian follicles ≥3 mm in diameter in cattle. Reproduction, Fertility and Development 22, 550-557.
| Crossref | Google Scholar | PubMed |
Mossa F, Jimenez-Krassel F, Folger JK, Ireland JLH, Smith GW, Lonergan P, Evans ACO, Ireland JJ (2010b) Evidence that high variation in antral follicle count during follicular waves is linked to alterations in ovarian androgen production in cattle. Reproduction 140, 713-720.
| Crossref | Google Scholar | PubMed |
Motta JCL, Sala RV, Absalón-Medina VA, Fricke VC, Dominguez M, Pereira DC, Hayden C, Canadas ER, Duran BJ, Moreno JF, Garcia-Guerra A (2021) Influence of length of porcine follicle-stimulating hormone (p-FSH) treatment before ovum pickup on ovarian response and in vitro embryo production in Holstein heifers. Reproduction, Fertility and Development 33, 170-171.
| Crossref | Google Scholar |
Motta JCL, Hayden C, Sala RV, Absalon-Medina VA, Fricke VC, Pereira DC, Ross PJ, Moreno JF, Garcia-Guerra A (2022) Effect of pattern of p-FSH treatment prior to ovum pick-up on ovarian response and in vitro embryo production in pregnant Holstein heifers. In ‘Proceedings of the 19th International Congress on Animal Reproduction’. pp. 54–54. (International Congress on Animal Reproduction)
Motta JCL, Sala RV, Hayden CB, Pereira DC, Ross PJ, Moreno JF, Garcia-Guerra A (2023) Ovarian stimulation with FSH increases in vitro embryo production in high AMH heifers in a dose-dependent manner. Animal – Science Proceedings 14, 492.
| Crossref | Google Scholar |
Mueller ML, Van Eenennaam AL (2022) Synergistic power of genomic selection, assisted reproductive technologies, and gene editing to drive genetic improvement of cattle. CABI Agriculture and Bioscience 3, 13.
| Crossref | Google Scholar |
Nasser LF, Adams GP, Bó GA, Mapletoft RJ (1993) Ovarian superstimulatory response relative to follicular wave emergence in heifers. Theriogenology 40, 713-724.
| Crossref | Google Scholar | PubMed |
Nawaz MY, Jimenez-Krassel F, Steibel JP, Lu Y, Baktula A, Vukasinovic N, Neuder L, Ireland JLH, Ireland JJ, Tempelman RJ (2018) Genomic heritability and genome-wide association analysis of anti-Müllerian hormone in Holstein dairy heifers. Journal of Dairy Science 101, 8063-8075.
| Crossref | Google Scholar | PubMed |
Nivet A-L, Bunel A, Labrecque R, Belanger J, Vigneault C, Blondin P, Sirard M-A (2012) FSH withdrawal improves developmental competence of oocytes in the bovine model. Reproduction 143, 165-171.
| Crossref | Google Scholar | PubMed |
Ocampo V, Cedeño AV, Mendoza B, Pinargote L, Romero G, Bó GA (2024) In vitro embryo production in Bos indicus donors super-stimulated with equine chorionic gonadotrophin or FSH prior to ovum pickup. Reproduction, Fertility and Development 36, 207.
| Crossref | Google Scholar |
Oliveira LH, Sanches CP, Seddon AS, Veras MB, Lima FA, Monteiro PLJ, Jr, Wiltbank MC, Sartori R (2016) Short communication: follicle superstimulation before ovum pick-up for in vitro embryo production in Holstein cows. Journal of Dairy Science 99, 9307-9312.
| Crossref | Google Scholar | PubMed |
Ongaratto FL, Tríbulo A, Ramos M, Rodriguez P, Bó GA (2010) Oocyte recovery rates and in vitro blastocyst production in cattle treated with a single injection of Folltropin-V diluted in a slow-release formulation. Reproduction, Fertility and Development 23, 202-203.
| Crossref | Google Scholar |
Ongaratto F, Rodriguez Villamil P, Tribulo A, Bo GA (2015) Effect of follicle wave synchronization and gonadotropin treatments on the number and quality of cumulus-oocyte complex obtained by ultrasound-guided ovum pick-up in beef cattle. Animal Reproduction 12, 876-883.
| Google Scholar |
Ongaratto FL, Cedeño AV, Rodriguez-Villamil P, Tríbulo A, Bó GA (2020) Effect of FSH treatment on cumulus oocyte complex recovery by ovum pick up and in vitro embryo production in beef donor cows. Animal Reproduction Science 214, 106274.
| Crossref | Google Scholar | PubMed |
Pavlok A, Lucas-Hahn A, Niemann H (1992) Fertilization and developmental competence of bovine oocytes derived from different categories of antral follicles. Molecular Reproduction and Development 31, 63-67.
| Crossref | Google Scholar | PubMed |
Pfeiffer KE, Jury LJ, Larson JE (2014) Determination of anti-Müllerian hormone at estrus during a synchronized and a natural bovine estrous cycle. Domestic Animal Endocrinology 46, 58-64.
| Crossref | Google Scholar | PubMed |
Pierson RA, Ginther OJ (1984) Ultrasonography of the bovine ovary. Theriogenology 21, 495-504.
| Crossref | Google Scholar | PubMed |
Pierson RA, Ginther OJ (1987) Follicular populations during the estrous cycle in heifers. I. Influence of day. Animal Reproduction Science 14, 165-176.
| Crossref | Google Scholar |
Pieterse MC, Kappen KA, Kruip TAM, Taverne MAM (1988) Aspiration of bovine oocytes during transvaginal ultrasound scanning of the ovaries. Theriogenology 30, 751-762.
| Crossref | Google Scholar | PubMed |
Pieterse MC, Vos PLAM, Kruip TAM, Wurth YA, van Beneden TH, Willemse AH, Taverne MAM (1991) Transvaginal ultrasound guided follicular aspiration of bovine oocytes. Theriogenology 35, 19-24.
| Crossref | Google Scholar |
Pontes JHF, Nonato-Junior I, Sanches BV, Ereno-Junior JC, Uvo S, Barreiros TRR, Oliveira JA, Hasler JF, Seneda MM (2009) Comparison of embryo yield and pregnancy rate between in vivo and in vitro methods in the same Nelore (Bos indicus) donor cows. Theriogenology 71, 690-697.
| Crossref | Google Scholar | PubMed |
Rico C, Fabre S, Médigue C, Clemente Nd, Clément F, Bontoux M, Touzé J-L, Dupont M, Briant E, Rémy B, Beckers J-F, Monniaux D (2009) Anti-Müllerian hormone is an endocrine marker of ovarian gonadotropin-responsive follicles and can help to predict superovulatory responses in the cow. Biology of Reproduction 80, 50-59.
| Crossref | Google Scholar | PubMed |
Rico C, Drouilhet L, Salvetti P, Dalbiès-Tran R, Jarrier P, Touzé J-L, Pillet E, Ponsart C, Fabre S, Monniaux D (2012) Determination of anti-Müllerian hormone concentrations in blood as a tool to select Holstein donor cows for embryo production: from the laboratory to the farm. Reproduction, Fertility and Development 24, 932-944.
| Crossref | Google Scholar | PubMed |
Rosa CO, Marinho LSR, da Rosa PRA, De Cesaro MP, Lunardelli PA, Silva-Santos KC, Basso AC, Bordignon V, Seneda MM (2018) Molecular characteristics of granulosa and cumulus cells and oocyte competence in Nelore cows with low and high numbers of antral follicles. Reproduction in Domestic Animals 53, 921-929.
| Crossref | Google Scholar | PubMed |
Sala RV, Motta JCL, Absalon-Medina VA, Fricke VC, Crist AE, Reamsnyder T, Ross PJ, Moreno JF, Garcia-Guera A (2024) Assessment of porcine follicle-stimulating hormone delivery mode before ovum pickup and in vitro embryo production in pregnant heifers. Reproduction, Fertility and Development 36, 250.
| Crossref | Google Scholar |
Sales JNS, Iguma LT, Batista RITP, Quintão CCR, Gama MAS, Freitas C, Pereira MM, Camargo LSA, Viana JHM, Souza JC, Baruselli PS (2015) Effects of a high-energy diet on oocyte quality and in vitro embryo production in Bos indicus and Bos taurus cows. Journal of Dairy Science 98, 3086-3099.
| Crossref | Google Scholar | PubMed |
Santos GMGd, Silva-Santos KC, Barreiros TRR, Morotti F, Sanches BV, de Moraes FLZ, Blaschi W, Seneda MM (2016) High numbers of antral follicles are positively associated with in vitro embryo production but not the conception rate for FTAI in Nelore cattle. Animal Reproduction Science 165, 17-21.
| Crossref | Google Scholar |
Santos RM, Oliveira M, Demétrio CGB, Hasler JH, Fonseca JC, Demetrio DGB (2021) Single injection of follicle-stimulating hormone before ovum pickup in lactating Holstein donors: oocyte recovery and embryo production. Reproduction, Fertility and Development 33, 181.
| Crossref | Google Scholar |
Sartori R, Gimenes LU, Monteiro PLJ, Jr, Melo LF, Baruselli PS, Bastos MR (2016) Metabolic and endocrine differences between Bos taurus and Bos indicus females that impact the interaction of nutrition with reproduction. Theriogenology 86, 32-40.
| Crossref | Google Scholar | PubMed |
Sarwar Z, Saad M, Saleem M, Husnain A, Riaz A, Ahmad N (2020a) Effect of follicle size on oocytes recovery rate, quality, and in-vitro developmental competence in Bos indicus cows. Animal Reproduction 17, e20200011.
| Crossref | Google Scholar | PubMed |
Sarwar Z, Sagheer M, Sosa F, Saad M, Hassan M, Husnain A, Arshad U (2020b) Meta-analysis to determine effects of treatment with FSH when there is progestin-priming on in-vitro embryo production using ovum pick-up in Bos taurus cows. Animal Reproduction Science 221, 106590.
| Crossref | Google Scholar | PubMed |
Scaramuzzi RJ, Baird DT, Campbell BK, Driancourt M-A, Dupont J, Fortune JE, Gilchrist RB, Martin GB, McNatty KP, McNeilly AS, Monget P, Monniaux D, Viñoles C, Webb R (2011) Regulation of folliculogenesis and the determination of ovulation rate in ruminants. Reproduction, Fertility and Development 23, 444-467.
| Crossref | Google Scholar | PubMed |
Scarlet D, Serbetci I, Lautner M, Bollwein H (2024) Effects of follicular superstimulation on ovum pickup–in vitro-produced outcome in German Fleckvieh heifers. Reproduction, Fertility and Development 36, 270.
| Crossref | Google Scholar |
Scheetz D, Folger JK, Smith GW, Ireland JJ (2012) Granulosa cells are refractory to FSH action in individuals with a low antral follicle count. Reproduction, Fertility and Development 24, 327-336.
| Crossref | Google Scholar | PubMed |
Schefers JM, Weigel KA (2012) Genomic selection in dairy cattle: Integration of DNA testing into breeding programs. Animal Frontiers 2, 4-9.
| Crossref | Google Scholar |
Seneda MM, Esper CR, Garcia JM, Oliveira JAd, Vantini R (2001) Relationship between follicle size and ultrasound-guided transvaginal oocyte recovery. Animal Reproduction Science 67, 37-43.
| Crossref | Google Scholar | PubMed |
Silva-Santos KC, Santos GMG, Koetz Júnior C, Morotti F, Siloto LS, Marcantonio TN, Urbano MR, Oliveira RL, Lima DCM, Seneda MM (2014) Antral follicle populations and embryo production – in vitro and in vivo – of Bos indicus–taurus donors from weaning to yearling ages. Reproduction in Domestic Animals 49, 228-232.
| Crossref | Google Scholar | PubMed |
Singh J, Dominguez M, Jaiswal R, Adams GP (2004) A simple ultrasound test to predict the superstimulatory response in cattle. Theriogenology 62, 227-243.
| Crossref | Google Scholar | PubMed |
Sirard M-A (2011) Follicle environment and quality of in vitro matured oocytes. Journal of Assisted Reproduction and Genetics 28, 483-488.
| Crossref | Google Scholar | PubMed |
Sirard M-A (2022) The two-step process of ovarian follicular growth and maturation in mammals can be compared to a fruit ripening where quality depends on the second step. Biology of Reproduction 106, 230-234.
| Crossref | Google Scholar |
Sirard M-A, Picard L, Dery M, Coenen K, Blondin P (1999) The time interval between FSH administration and ovarian aspiration influences the development of cattle oocytes. Theriogenology 51, 699-708.
| Crossref | Google Scholar | PubMed |
Sirois J, Fortune JE (1988) Ovarian follicular dynamics during the estrous cycle in heifers monitored by real-time ultrasonography. Biology of Reproduction 39, 308-317.
| Crossref | Google Scholar | PubMed |
Soares ACS, Marques KNG, Bragança LGM, Lodde V, Luciano AM, Buratini J (2020) Synchronization of germinal vesicle maturity improves efficacy of in vitro embryo production in Holstein cows. Theriogenology 154, 53-58.
| Crossref | Google Scholar | PubMed |
Sola J, Carvajal Basto J, Camaño M, Ponte E, Waidelich R, Oviedo JM, Tribulo A, Bo GA (2023a) Superovulatory response and embryo production in Bos indicus and Bos taurus beef donors superstimulated with constant or decreasing doses of FSH. Animal – Science Proceedings 14, 475.
| Crossref | Google Scholar |
Sola J, Alberio V, Carvajal J, Tribulo A, Waidelich R, Oviedo JM, Salamone D, Mapletoft RJ, Bó GA (2023b) The combination of Folltropin and hyaluronan for simplified OPU stimulation in beef cows. Reproduction, Fertility and Development 35, 228-229.
| Crossref | Google Scholar |
Sood P, Zachut M, Dekel I, Dube H, Jacoby S, Moallem U (2017) Preovulatory follicle characteristics and oocyte competence in repeat breeder dairy cows. Journal of Dairy Science 100, 9372-9381.
| Crossref | Google Scholar | PubMed |
Souza AH, Sartori R, Guenther JN, Caraviello D, Monson R, Wiltbank MC (2007) Effect of semen source and dose of FSH on superovulatory response and embryo production in Holstein heifers. Animal Reproduction 4, 70-76.
| Google Scholar |
Souza AH, Carvalho PD, Rozner AE, Vieira LM, Hackbart KS, Bender RW, Dresch AR, Verstegen JP, Shaver RD, Wiltbank MC (2015) Relationship between circulating anti-Müllerian hormone (AMH) and superovulatory response of high-producing dairy cows. Journal of Dairy Science 98, 169-178.
| Crossref | Google Scholar | PubMed |
Sugimura S, Kobayashi N, Okae H, Yamanouchi T, Matsuda H, Kojima T, Yajima A, Hashiyada Y, Kaneda M, Sato K, Imai K, Tanemura K, Arima T, Gilchrist RB (2017) Transcriptomic signature of the follicular somatic compartment surrounding an oocyte with high developmental competence. Scientific Reports 7, 6815.
| Crossref | Google Scholar | PubMed |
Surjus RS, Prata AB, Borsato M, Mattos FCSZ, Martins da Silveira MC, Mourao GB, Pires AV, Wiltbank MC, Sartori R (2014) In vivo embryo production in cows superovulated 1 or 2 days after ovum pick-up. Reproduction, Fertility and Development 26, 527-532.
| Crossref | Google Scholar | PubMed |
Tan SJ, Lu KH (1990) Effects of different oestrous stages of ovaries and sizes of follicles on generation of bovine embryos in vitro. Theriogenology 33, 335.
| Crossref | Google Scholar |
Tríbulo A, Rogan D, Tribulo H, Tribulo R, Alasino RV, Beltramo D, Bianco I, Mapletoft RJ, Bó GA (2011) Superstimulation of ovarian follicular development in beef cattle with a single intramuscular injection of Folltropin-V. Animal Reproduction Science 129, 7-13.
| Crossref | Google Scholar | PubMed |
Tríbulo A, Rogan D, Tríbulo H, Tríbulo R, Mapletoft RJ, Bó GA (2012) Superovulation of beef cattle with a split-single intramuscular administration of Folltropin-V in two concentrations of hyaluronan. Theriogenology 77, 1679-1685.
| Crossref | Google Scholar | PubMed |
Vassena R, Mapletoft RJ, Allodi S, Singh J, Adams GP (2003) Morphology and developmental competence of bovine oocytes relative to follicular status. Theriogenology 60, 923-932.
| Crossref | Google Scholar | PubMed |
Viana JHM (2023) 2022 Statistics of embryo production and transfer in domestic farm animals. Embryo Technology Newsletter 41, 20-38.
| Google Scholar |
Vieira LM, Rodrigues CA, Castro Netto A, Guerreiro BM, Silveira CRA, Moreira RJC, Sá Filho MF, Bó GA, Mapletoft RJ, Baruselli PS (2014) Superstimulation prior to the ovum pick-up to improve in vitro embryo production in lactating and non-lactating Holstein cows. Theriogenology 82, 318-324.
| Crossref | Google Scholar | PubMed |
Vieira LM, Rodrigues CA, Castro Netto A, Guerreiro BM, Silveira CRA, Freitas BG, Bragança LGM, Marques KNG, Sá Filho MF, Bó GA, Mapletoft RJ, Baruselli PS (2016) Efficacy of a single intramuscular injection of porcine FSH in hyaluronan prior to ovum pick-up in Holstein cattle. Theriogenology 85, 877-886.
| Crossref | Google Scholar | PubMed |
Vigier B, Picard J-Y, Tran D, Legeai L, Josso N (1984) Production of anti-Mullerian hormone: another homology between Sertoli and granulosa-cells. Endocrinology 114, 1315-1320.
| Crossref | Google Scholar | PubMed |
Walsh SW, Mossa F, Butler ST, Berry DP, Scheetz D, Jimenez-Krassel F, Tempelman RJ, Carter F, Lonergan P, Evans ACO, Ireland JJ (2014) Heritability and impact of environmental effects during pregnancy on antral follicle count in cattle. Journal of Dairy Science 97, 4503-4511.
| Crossref | Google Scholar | PubMed |
Wiggans GR, Carrillo JA (2022) Genomic selection in United States dairy cattle. Frontiers in Genetics 13, 994466.
| Crossref | Google Scholar |