Sodium alginate and bovine serum albumin co-combined improved the cryopreservation quality of boar sperm through the PI3K-AKT pathway
Jing Wang A , Yongyong Rao A , Chongfan Du A , Ao Wang A , Yanling Wu A , Ruiyi Lin A , Tianfang Xiao A and Weimin Lin
A
Abstract
The occurrence of apoptosis due to transient oxidative damage to spermatozoa presents a significant challenge in semen preservation. At present, the addition of protective agents is the primary method for mitigating this damage and involves the addition of protective agents.
This study aims to elucidate the mechanism of action of cryopreservation and to identify high-quality combinatorial cryopreservation dilutions.
In this study, two protective agents, bovine serum albumin (BSA) and sodium alginate (SA), were combined to evaluate the effectiveness of these novel additives in protecting porcine sperm from damage during cryopreservation. The mechanism of action of the SA and BSA combination was further elucidated at the molecular level, with key proteins being identified through proteomic analysis.
The findings indicated that sperm treated with 2 mg/mL SA and 5 mg/mL BSA exhibited optimal motility parameters, superior functional integrity and the most effective ability to alleviate oxidative stress. Combined with proteomic data, the results suggested that these additives regulate improvements in sperm quality by mediating the activity of the PI3K-AKT pathway.
This study found that the combination of SA and BSA provides an effective protective effect for frozen sperm preservation.
The findings offer theoretical and technical support for the use of composite additive to protect sperm from damage, which is crucial for enhancing the quality of pig semen and improving reproductive outcomes.
Keywords: bovine serum albumin, oxidative stress, PI3K-AKT pathway, pig, proteomics, sodium alginate, sperm cryopreservation, sperm motility.
Introduction
Boar sperm cryopreservation is an effective technology for the long-term preservation of valuable genetic resources (Yánez-Ortiz et al. 2022). However, existing cryopreservation methods often result in the accumulation of free radicals and reactive oxygen species (ROS), which induce oxidative damage to sperm (Lan et al. 2022). Therefore, improving the quality of frozen semen preservation is essential for the broader acceptance and utilization of pig artificial insemination technology. This improvement, in turn, helps decrease the number of boars on farms, which is crucial for the advancement of the modern pig industry.
Current research suggests that the addition of antioxidants and drugs to freezing media can protect sperm from the ROS effects of cryodamage (Mehdipour et al. 2022). The inclusion of antioxidants in sperm cryopreservation has been positively correlated with the freezing tolerance of sperm from boars (Yang et al. 2024), rams (Fang et al. 2022), horses (Peña and Gibb 2022), and cattle (Kumar et al. 2019; Pezo et al. 2021). Bovine serum albumin (BSA), the primary soluble protein component of the mammalian circulatory system, serves numerous physiological functions (Jahanban-esfahlan et al. 2019; Xu et al. 2023). Owing to its wide range of biological properties, BSA plays a significant role in enhancing sperm quality in livestock reproduction, including pigs (Yang et al. 2024), cattle, sheep, chickens (Behnamifar et al. 2021), rats, and rabbits (Rosato and Iaffaldano 2013), as well as in humans (Jahanban-esfahlan et al. 2019). Sulfated alginate (SA) is an acidic polysaccharide with potential roles in regulating intestinal microbiota, as well as exhibiting immunomodulatory and antitumor activities (Shaikh et al. 2023). At the same time, SA has been shown to positively affect the cryopreservation of goat (Liang et al. 2022) and boar sperm (Hu et al. 2014). The antioxidant, cryoprotective, and antimicrobial activities can be enhanced during the cryopreservation of semen from various cattle breeds (Perteghella et al. 2017; Kumar et al. 2019). Given these facts, our hypothesis is to investigate whether thawed porcine sperm can also benefit from the combined supplementation of SA and BSA, and whether this combination is superior to supplementation with either component alone.
On the other hand, the PI3K-AKT pathway is an intracellular signal transduction pathway that promotes metabolism, proliferation, cell survival, growth, and angiogenesis in response to extracellular signals (Yu et al. 2022). This process is mediated by the phosphorylation of serine or threonine residues in a series of downstream substrates (Yu et al. 2022). It has been suggested that AKT phosphorylation following the addition of melatonin is related to membrane integrity, intracellular activity, and caspase-3 activity in cryo-thawed human and sheep sperm. Additionally, it may also interact with MT1 to inhibit the opening of the mitochondrial permeability transition pore (mPTP) (Najafi et al. 2018; Fang et al. 2022). The disruption of the metabolic enrichment of unsaturated fatty acids during the cryopreservation of ram sperm implicates the PI3K-AKT pathway as one of the primary pathways involved (Ofosu et al. 2023). Therefore, in this paper, we speculate that the protective mechanism of combined additives, such as SA and BSA, on sperm cryopreservation may operate through the PI3K-AKT pathway.
In this study, we evaluated the combined effects of addition of various concentrations of SA and BSA on sperm quality during fluid storage, to determine the optimal amount of addition. In addition, we analyzed sperm-quality parameters, antioxidant capacity, and related protein expression to investigate whether the combined effect offers protective benefits to boar sperm by regulating the PI3K-AKT signaling pathway. A combined proteomics analysis focused on the core proteins primarily involved in the regulation of down-regulated proteins. The results of this study provide fundamental knowledge for utilizing the combined action of antioxidants to preserve boar semen and improve sperm quality, and provide evidence that the combined action of SA and BSA protects boar sperm from oxidative damage through the PI3K-AKT pathway.
Materials and methods
Animals
Semen samples were collected from 18 healthy, sexually mature Yorkshire boars, aged 8–10 months, at a commercial breeding farm (Fujian Yongcheng Agriculture and Animal Husbandry Technology Co., China). Each set consisted of six samples, supported by a replicate of the experiment. Additionally, six samples were randomly mixed in pairs to form three sets of parallel experiments. All animal experiments were approved by the Animal Ethics Committee of Fujian Agriculture and Forestry University (PZCASFAFU22001). The reagents required for this experiment are listed in Supplementary Table S1.
Experimental design
Different concentrations of SA (0, 0.5, 1, 2, 4 mg/mL) (Shanghai Yuanye Bio-technology, S11053) were added to the filler to determine the optimal concentration of sodium alginate (SA) on the basis of the combined levels of sperm motility, plasma membrane integrity, acrosome integrity, mitochondrial membrane potential, and antioxidant capacity. Each treatment wass repeated three times. Additionally, the optimal concentration of BSA (0, 1, 3, 5, 7 mg/mL) (Shanghai Yuanye Bio-technology, S12012) was screened using the same procedure. Then, the optimal concentration of the combination was screened by the optimal concentrations of SA and BSA as the center points (Control, S1-B4, S1-B5, S1-B6, S2-B4, S2-B5, S2-B6, S3-B4, S3-B5, S3-B6). Here S1-B4 is defined as SA 1 mg/mL + BSA 4 mg/mL, and the remaining combined concentrations are calculated similarly. Finally, the mechanism of action of the filler was combined from the motility performance, antioxidant performance, protein molecular concentrations, and proteomic analysis of spermatozoa.
Semen collection
Boar semen was collected using the traditional hand-held method (Yánez-Ortiz et al. 2022). A thermostatic semen collection cup maintained at 37°C was utilized to obtain the sample. The sample was then mailed to the laboratory by mail at 17°C for subsequent sperm-quality testing. To eliminate gelatinous proteins, the semen sample was filtered through three layers of gauze. After quality control, the viability of the sperm sample exceeded 80%, and the concentration surpassed 2 × 108 sperm/mL, ensuring adequate support for subsequent experiments.
Cryopreservation and thawing of semen
The Beltsville thawing solution (BTS) was prepared by dissolving 37.0 g of glucose, 1.25 g of EDTA, 6.0 g of sodium citrate, 0.65 g of potassium chloride, 1.25 g of sodium bicarbonate, 0.65 g of penicillin, and 0.6 g of streptomycin sulfate in 1 L of distilled water. Different concentrations of SA or/and BSA were added as experimental additives to the BTS buffer solution to create the final diluent. The final dilution was mixed with egg yolk in a ratio of 4:1 to produce a Freezer Solution I, which was temporarily stored at 17°C. This final dilution had an osmolality of 300–320 mOsm/kg and a pH of 7.2–7.4. To prepare Refrigerant Solution II for temporary storage at 4°C, 6% glycerol and 0.6% SDS were added to Solution I. Once the original semen was cooled to 17°C, the supernatant was removed by centrifugation at 800g for 10 min at 17°C. The semen was then diluted to 3 × 108 sperm/mL by adding Solution I. Subsequently, it was slowly cooled to 4°C and an equal volume of Solution II was added to make its final density 1.5 × 108. Semen is usually filled with a straw, then placed in −140°C for fumigation for 15 min, and then put in liquid nitrogen for long-term storage. To thaw sperm, you need to take the frozen sperm preservation tube in a 50°C water bath for 15 s, add BTS thawing solution at a ratio of 1:1 and incubate at 37°C for 15 min.
Sperm-motility parameters
Our study previously reported a method for measuring sperm-motility parameters by using a computer-assisted sperm analysis system (CASA) (Hamilton IVOS V3, USA) (Lan et al. 2021). The HT-CASA II system can determine the concentration and displacement of spermatozoa by utilizing sequential frames captured at a set acquisition frequency of 60 Hz. Additionally, the first 10 frames are collected to ascertain the motile sperm count. Briefly, 0.2 mL of a diluted semen sample (1 × 106 sperm/mL) is pipetted at 37°C onto a pre-warmed disposable chamber slide (Leja Sperm Counting Slide, Netherlands). Four fields, containing a total of no fewer than 200 spermatozoa, are randomly selected for observation to increase the accuracy of the results. The parameters of CASA are set according to the manufacturer’s instructions, and the system automatically calculates sperm motility capacity and condition.
Plasma membrane integrity, acrosome integrity, and mitochondrial activity
The thawed semen was diluted to a concentration of 8 × 107 cells/mL. The integrity of the sperm plasma membrane was assessed using the sperm tail hypotonic expansion test (HOST). The main step involved preparing a fructose–sodium citrate hypotonic solution, which was then mixed with semen and incubated in a water bath at 37°C for 1 h, followed by observation under light microscope. Second, the integrity of sperm acrosomes was detected by Coomassie Brilliant Blue staining. This involved fixing the samples with 4% formaldehyde, staining with Coomassie Brilliant Blue, and observing them under a ×1000 immersion-oil microscope after natural drying. To evaluate mitochondrial membrane potential, spermatozoa were treated with JC-1 staining solution and incubated at 37°C for 20 min. Following incubation, the supernatant was removed by centrifugation, and the samples were washed twice with 1 × JC-1 staining buffer. The spermatozoa were then re-suspended and measured using a microplate reader. Values were recorded four times with the microplate reader and then normalized.
Determination of redox state
The detection of ROS (reactive oxygen species) was to suspend the sperm in the DCFH-DA probe and incubating it at 37°C for 20 min. Subsequently, the sperm was washed three times with PBS, and the ROS content in the sperm was measured using a fluorescence microplate reader. To detect MDA (malondialdehyde), and MDA detection solution was added to the sperm samples. The mixture was thoroughly combined and heated at 100°C heat for 15 min, then allowed to cool to room temperature in a water bath. After centrifuging the supernatant, the absorbance was measured with a microplate reader at 532 nm to detect the change in MDA content. For the total detection of T-AOC (total antioxidant capacity) measurement, pre-cooled PBS was added to the sperm sample, breaking the sample to release antioxidants. The sample was then centrifuged at 4°C. The T-AOC Working Solution was added to the resulting supernatant liquid. The mixture was allowed to react at room temperature for 6 min before measuring the absorbance at 405 nm with a microplate reader, to determine the T-AOC content. SOD (superoxide dismutase) is detected by first centrifuging the sample to remove the supernatant, followed by ultrasonic disruption. The SOD working solution was then added, mixed, and incubated at 37°C for 20 min. Finally, the absorbance was measured at 450 nm using a microplate reader to detect SOD viability. The reagents required for the above tests are listed in Table S1.
Western blotting
To prepare sperm samples, break them using sonication, Next, lyse the samples on ice with RIPA and PSMF, and then centrifuge the samples at 4°C for 2 min to collect the supernatant. Protein quantification was performed using the BCA Protein Assay Kit. The protein samples were mixed with a protein loading buffer for denaturation at 100°C. The following proteins were separated using SDS-PAGE (20 μg/sample): CAT, SOD1, SOD2, Caspase-3, Caspase-9, BAX, Nrf2, Cytc, GSTA2, NME5, AKT, p-AKT, p-PI3K, PI3K, and GAPDH. The target proteins Were then transferred the target protein to a nitrocellulose (NC) membrane, which was sealed with a 5% skim milk buffer. The membrane was washed three times with Tris-buffered saline containing 1% Tween-20 (TBST). Then, the NC membrane was incubated with the primary antibody overnight at 4°C. After three additional washes, the membrane was incubated with the secondary antibody (Goat anti-Rabbit IgG Secondary Antibody) for 2 h at room temperature. Finally, the blot was then visualized using a LI-COR image analyzer. The antibodies used are listed in Table S2.
Proteome sequencing
Raw data were obtained using the TMT peptide labeling method with the Easy nLC 1200 chromatography system (Thermo Scientific) (Casey et al. 2017). We utilized the Maggi self-authoring platform for quality control of the machine’s raw reads. Differentially expressed proteins were identified on the basis of a false discovery rate (FDR) of less than 0.05 and a fold change of >2. Additionally, we conducted functional annotation and enrichment analyses for the differentially expressed genes in both the blank and treatment groups. The gene ontology (GO) enrichment analysis was conducted using the Goatools software (ver. 0.6.5; Shanghai Majorbio Bio-Pharm Technology Co., Ltd), and the Kyoto Encyclopedia of Genes and Genomes (KEGG) enrichment analysis was performed using Python software. Finally, protein–protein interaction network analysis was performed by comparing the significantly different proteins with the STRING database (ver. 11.5). All of the above analyses were processed using the Majorbio cloud platform (www.majorbio.com).
Statistical analysis
SPSS 26.0 software (IBM, Chicago, IL, USA) was used for statistical analysis. Each measurement was performed at least three times, and the results were reported as the average of these replicates. The results are expressed as mean ± s.e. (s.e.m.). Utilizing a two-tailed Student’s t-test was employed to determine the significance of the comparisons between two groups. Subsequently, a one-way ANOVA was performed to assess total sperm motility, progressive motility, plasma membrane integrity, mitochondrial activity, acrosome integrity, antioxidant capacity, and marker protein expression across different experimental groups. P-values of <0.05 and <0.01 were considered to indicate significant and extremely significant differences respectively.
Results
Effects of SA supplements on sperm-quality parameters, mitochondrial membrane potential, and oxidative stress after cryogenic storage
In this study, alterations in SA concentration were found to lead to significant increases in various sperm parameters, including motility, vitality, curvilinear velocity (VCL), average path velocity (VAP), straight-line velocity (VSL), beat cross-frequency (BCF), average path distance (DAP), curve path distance (DCL), straight path distance (DSL), linearity (LIN), straightness (STR), and head swing proportions (WOB), when compared with the control group (P < 0.05 or P < 0.01). The highest values for motility and vitality were recorded in the group treated with 2 mg/mL of SA (Table 1). Furthermore, the supplementation of SA resulted in significantly higher index values for sperm plasma membrane integrity, mitochondrial motility, and acrosome integrity than in the control group, with the most notable improvements observed in the 2 mg/mL SA group (P < 0.05 or P < 0.01) (Table 1, Supplementary Fig. S1a). Evaluation of sperm oxidative stress indicated elevated concentrations of SOD and T-AOC in the SA supplementation group, in contrast to the control group, whereas the concentrations of ROS and MDA were lower in the SA supplementation group (P < 0.05 or P < 0.01). Notably, the group treated with 2 mg/mL of SA exhibited the most significant changes (Fig. S1a). In summary, the addition of 2 mg/mL of SA as a sole supplement to the filler was deemed the optimal concentration for providing comprehensive protection during frozen sperm storage.
Motion parameter | Raw sperm | 0 mg/mL | 0.5 mg/mL | 1 mg/mL | 2 mg/mL | 4 mg/mL | |
---|---|---|---|---|---|---|---|
Motility (%) | 93.40 ± 0.46A | 22.90 ± 4.694D | 37.47 ± 0.74Ca | 37.43 ± 4.84Ca | 60.27 ± 0.50B | 34.70 ± 1.11Cb | |
Vitality (%) | 90.10 ± 1.04A | 13.67 ± 1.02C | 26.10 ± 2.23Bab | 21.00 ± 5.94Bbc | 30.73 ± 2.45Ba | 18.73 ± 2.46Bcd | |
VCL (μm/s) | 223.40 ± 5.31A | 151.69 ± 3.14B | 146.79 ± 19.63B | 119.48 ± 44.54Bbc | 121.57 ± 7.54Bbc | 105.89 ± 10.58Bc | |
VAP (μm/s) | 119.74 ± 2.44A | 54.19 ± 1.17Cb | 75.79 ± 8.06B | 60.11 ± 20.99BCa | 63.26 ± 3.87BCa | 55.55 ± 7.33Ca | |
VSL (μm/s) | 93.12 ± 3.59A | 44.63 ± 2.83BCa | 60.57 ± 6.479Ba | 47.13 ± 14.44Bab | 48.91 ± 4.26Bb | 43.71 ± 6.48BCa | |
BCF (Hz) | 30.61 ± 0.75C | 26.75 ± 1.69D | 36.84 ± 2.04Aab | 38.84 ± 0.75Aa | 35.67 ± 2.60Aab | 33.83 ± 1.52ABa | |
DAP (μm) | 62.18 ± 3.49A | 27.87 ± 2.31Ca | 40.01 ± 5.08Ba | 35.59 ± 10.23Bab | 31.96 ± 1.25BCa | 28.40 ± 2.93Ca | |
DCL (μm) | 118.02 ± 5.22A | 78.68 ± 0.69Bb | 80.64 ± 1.87Ba | 72.45 ± 21.59Babc | 64.25 ± 1.51Bbc | 56.88 ± 5.71Bc | |
DSL (μm) | 47.48 ± 4.28A | 20.57 ± 2.45Bb | 21.17 ± 3.84Bab | 27.74 ± 6.65Ba | 23.92 ± 2.39Ba | 21.65 ± 2.66Bab | |
LIN (VSL/VCL) (%) | 42.21 ± 1.28A | 34.78 ± 2.47B | 41.26 ± 3.36A | 41.41 ± 3.35A | 40.53 ± 3.05A | 42.13 ± 2.21A | |
STR (VSL/VAP) (%) | 77.31 ± 1.71A | 61.55 ± 1.47B | 62.24 ± 3.45B | 77.22 ± 5.71A | 75.10 ± 2.49A | 77.57 ± 1.42A | |
WOB (VAP/VCL) (%) | 53.95 ± 0.61A | 41.41 ± 1.46B | 51.75 ± 2.11A | 51.41 ± 1.11A | 51.94 ± 2.01A | 52.16 ± 1.89A | |
Plasma membrane (%) | – | 29.86 ± 0.02Cb | 31.93 ± 0.01Ca | 34.14 ± 0.04B | 37.47 ± 0.02A | 28.73 ± 0.01Cd | |
Acrosome integrity (%) | – | 37.67 ± 0.02c | 41.10 ± 0.02bc | 42.82 ± 0.01b | 48.10 ± 0.04A | 41.65 ± 0.01bc |
The values in the table represent the mean ± s.e. (s.e.m.). Within a row, values followed by the same letter are not significantly different. Different lowercase letters indicate a significant (P < 0.05) difference, whereas different uppercase letters indicate an extremely significant (P < 0.01) difference between the values.
VCL, curvilinear velocity; VAP, average path velocity; VSL, straight-line velocity; BCF, beat cross-frequency; DAP, average path distance; DCL, curve path distance; DSL, straight path distance; LIN (VSL/VCL), percentages of linearity; STR (VSL/VAP), straightness; and WOB (VAP/VCL), head swing proportions.
Effects of BSA on sperm-quality parameters, mitochondrial membrane potential, and oxidative stress after cryostorage
This study showed a positive impact of BSA on pig sperm. It demonstrated that varying concentrations of BSA lead to increased motility, vitality, VAP, VSL, BCF, DAP, DSL, LIN, STR, and WOB parameters of sperm when compared with the control group (P < 0.05 or P < 0.01). The group treated with 5 mg/mL of BSA exhibited the highest values for motility, vitality, VSL, and BCF (Table 2). Additionally, the BSA supplementation group showed significantly higher values in sperm plasma membrane integrity, mitochondrial viability, and acrosome integrity than did the control group, with the 5 mg/mL BSA group displaying the most notable results (P < 0.05 or P < 0.01) (Table 2, Fig. S1b). The evaluation of sperm oxidative stress showed that the concentrations of SOD and T-AOC in the BSA supplementation group were significantly higher than those in the control group. Conversely, the concentrations of ROS and MDA were lower in the BSA supplementation group than in the control group (P < 0.05 or P < 0.01). Notably, the 5 mg/mL BSA group exhibited the most significant results (Fig. S1b). In conclusion, the addition of 5 mg/mL of BSA alone to the filler is recommended as the optimal concentration for achieving a comprehensive protective effect during the storage of frozen sperm.
Motion parameter | Raw sperm | 0 mg/mL | 1 mg/mL | 3 mg/mL | 5 mg/mL | 7 mg/mL | |
---|---|---|---|---|---|---|---|
Motility (%) | 93.40 ± 0.46A | 13.67 ± 1.02C | 38.23 ± 6.83Bb | 43.10 ± 1.93Bab | 48.77 ± 3.79Ba | 38.00 ± 4.20Bb | |
Vitality (%) | 90.10 ± 1.04A | 22.90 ± 4.69Cb | 26.20 ± 4.06Ca | 29.33 ± 2.6Ca | 34.70 ± 1.30B | 27.00 ± 4.85Ca | |
VCL (μm/s) | 223.40 ± 5.31A | 151.69 ± 3.14B | 145.13 ± 6.27B | 142.16 ± 12.43B | 146.96 ± 11.03B | 152.79 ± 15.20B | |
VAP (μm/s) | 119.74 ± 2.44A | 54.19 ± 1.17C | 75.29 ± 1.96B | 74.36 ± 7.96B | 76.25 ± 3.49B | 77.47 ± 9.09B | |
VSL (μm/s) | 93.12 ± 3.59A | 44.63 ± 2.83C | 59.36 ± 3.86B | 59.89 ± 6.94B | 61.17 ± 2.18B | 60.70 ± 8.23B | |
BCF (Hz) | 30.61 ± 0.75B | 26.75 ± 1.69C | 36.62 ± 0.96Aab | 37.03 ± 0.72Aab | 38.12 ± 0.72Aa | 35.59 ± 1.75Ab | |
DAP (μm) | 62.18 ± 3.49A | 27.87 ± 2.31C | 38.57 ± 2.50B | 38.62 ± 5.20B | 40.20 ± 3.12B | 42.32 ± 3.99B | |
DCL (μm) | 118.02 ± 5.22A | 78.68 ± 0.69B | 76.97 ± 6.87B | 76.33 ± 9.73B | 79.68 ± 7.57B | 86.33 ± 7.54B | |
DSL (μm) | 47.48 ± 4.28A | 20.57 ± 2.45C | 29.67 ± 2.22B | 30.23 ± 3.96B | 31.63 ± 2.50B | 32.74 ± 3.10B | |
LIN(VSL/VCL) (%) | 42.21 ± 1.28A | 34.78 ± 2.47B | 40.45 ± 3.15A | 41.74 ± 2.85A | 40.73 ± 2.84A | 39.68 ± 2.58A | |
STR(VSL/VAP) (%) | 77.31 ± 1.71A | 61.55 ± 1.47B | 75.14 ± 3.65A | 76.77 + 1.80A | 75.94 ± 3.01A | 75.19 ± 2.11A | |
WOB(VAP/VCL) (%) | 53.95 ± 0.61Aa | 41.41 ± 1.46C | 51.49 ± 1.64Aab | 52.23 ± 2.10Aab | 51.50 ± 1.75Aab | 50.59 ± 1.79AB | |
Plasma membrane (%) | – | 29.86 ± 0.02D | 34.20 ± 0.02Bb | 38.59 ± 0.01Ba | 47.61 ± 0.02A | 32.46 ± 0.04C | |
Acrosome integrity (%) | – | 37.67 ± 0.02E | 60.87 ± 0.03D | 67.43 ± 0.01B | 73.10 ± 0.01A | 63.02 ± 0.06C |
The values in the table represent the mean ± s.e. Within a row, values followed by the same letter are not significantly different. Different lowercase letters indicate a significant (P < 0.05) difference, and different uppercase letters denote an extremely significant (P < 0.01) difference between the values.
The combined effect of SA and BSA on sperm-quality parameters, mitochondrial membrane potential, and oxidative stress after frozen storage in liquid media
The optimal concentrations of BSA and SA, when considered individually, cannot be directly extrapolated to determine the optimal concentration of their combination. Therefore, the combined concentration was screened in proximity to the identified optimal concentrations. On varying the concentrations of BSA and SA, we observed a significant increase in the sperm motility, vitality, and various parameters such as VCL, VAP, VSL, DAP, DCL, and DSL in the combined group compared with the control group (P < 0.05 or P < 0.01) (Table 3). The motility and vitality of the S2-B4 and S2-B5 treatment groups surpassed those of the other combination groups. Notably, the S2-B5 group demonstrated superior performance in VCL, VAP, VSL, and DCL indicators compared with the S2-B4 group (P < 0.05 or P < 0.01) (Table 3). Regarding sperm plasma membrane integrity and acrosome integrity, the index values for the S2-B4 and S2-B5 groups were significantly higher than those of the other groups. Furthermore, the acrosome integrity of the S2-B5 group exceeded that of the S2-B4 group (Table 3). On the basis of these outcomes, the control group, and SA, BSA, S2-B4, and S2-B5 groups were selected for the assessment of mitochondrial activity and oxidative stress. The findings showed that the S2-B5 treatment group was the most effective in mitigating the decline in membrane potential and oxidative stress, induced by frozen sperm preservation (P < 0.05 or P < 0.01) (Table 3, Fig. 1a–e).
Group | Motility (%) | Vitality (%) | VCL (μm/s) | VAP (μm/s) | VSL (μm/s) | BCF (Hz) | DAP (μm) | |
---|---|---|---|---|---|---|---|---|
Control | 22.90 ± 4.69D | 13.67 ± 1.02D | 134.56 ± 26.5Bbc | 67.52 ± 10.87BCab | 51.30 ± 6.16Bbc | 36.75 ± 1.69A | 34.53 ± 3.92B | |
S1-B4 | 41.17 ± 2.18Bbc | 26.97 ± 0.83Ca | 153.08 ± 6.10Bb | 79.27 ± 4.46Ba | 63.18 ± 5.07Aab | 35.59 ± 0.33Aab | 43.46 ± 2.46A | |
S1-B5 | 48.07 ± 2.65Bab | 31.40 ± 2.00Babc | 130.01 ± 7.60Bbc | 70.46 ± 0.84Ca | 57.70 ± 2.04Bb | 35.81 ± 1.39Aab | 36.73 ± 2.77B | |
S1-B6 | 47.27 ± 2.75Bab | 33.03 ± 1.61Babc | 133.19 ± 9.08Bbc | 72.41 ± 2.43BCa | 60.08 ± 1.39Ab | 36.35 ± 1.39A | 34.62 ± 2.48B | |
S2-B4 | 60.90 ± 0.82A | 40.23 ± 2.17A | 148.34 ± 5.23Ba | 78.93 ± 3.49Ba | 60.44 ± 3.86Ab | 36.21 ± 0.68A | 39.64 ± 2.28AB | |
S2-B5 | 59.27 ± 1.67A | 42.93 ± 2.61A | 186.43 ± 3.85A | 93.77 ± 3.55A | 68.25 ± 2.78A | 32.60 ± 0.93B | 44.86 ± 3.43A | |
S2-B6 | 52.40 ± 6.90ABa | 33.77 ± 1.55Ba | 144.39 ± 20.02Bbc | 77.23 ± 10.07Bbc | 63.01 ± 8.42Aab | 35.24 ± 0.61Aab | 39.69 ± 4.95AB | |
S3-B4 | 44.00 ± 1.47Bb | 29.50 ± 1.01Bb | 131.22 ± 7.57Bbc | 71.97 ± 2.07BCa | 59.47 ± 2.74ABa | 37.28 ± 0.68A | 37.04 ± 2.56B | |
S3-B5 | 44.40 ± 5.40Bb | 31.97 ± 3.28Babc | 153.05 ± 10.58Bb | 80.51 ± 3.87Ba | 65.13 ± 3.59Aab | 33.83 ± 0.4bB | 44.65 ± 4.56A | |
S3-B6 | 35.80 ± 3.50C | 24.27 ± 2.00Cab | 121.85 ± 6.87C | 67.86 ± 3.00BCab | 56.83 ± 2.02Bb | 36.47 ± 1.81A | 34.53 ± 1.99B |
Group | DCL (μm) | DSL (μm) | LN (%) | STR (%) | WOB (%) | Plasma membrane (%) | Acrosome integrity (%) | |
---|---|---|---|---|---|---|---|---|
Control | 72.02 ± 11.06Acd | 25.23 ± 2.63BC | 38.11 ± 4.81Ba | 73.21 ± 6.48ABb | 50.08 ± 2.54Bb | 29.86 ± 0.02E | 37.67 ± 0.02E | |
S1-B4 | 86.35 ± 4.40Aab | 34.34 ± 2.63Aa | 40.64 ± 2.40ABa | 76.97 ± 1.96A | 51.16 ± 1.99Ba | 33.26 ± 0.02D | 59.48 ± 0.06Cab | |
S1-B5 | 70.14 ± 4.51Acd | 29.43 ± 3.52Ba | 44.10 ± 3.99Aa | 78.51 ± 3.43A | 54.04 ± 2.41A | 42.46 ± 0.01Bb | 60.19 ± 0.03Ca | |
S1-B6 | 66.49 ± 6.02Acd | 27.77 ± 2.79Bb | 44.52 ± 3.45Aa | 78.74 ± 2.73A | 54.15 ± 2.38A | 43.20 ± 0.03Ba | 62.88 ± 0.04Ca | |
S2-B4 | 77.25 ± 3.48Abc | 29.61 ± 1.98Ba | 40.25 ± 0.75ABa | 73.91 ± 0.61ABb | 52.61 ± 0.61AB | 49.02 ± 0.05A | 64.77 ± 0.02B | |
S2-B5 | 92.18 ± 5.57Aa | 31.53 ± 2.60Aabc | 36.25 ± 0.77C | 70.73 ± 0.99B | 49.82 ± 0.75Bb | 48.67 ± 0.01A | 74.21 ± 0.04A | |
S2-B6 | 76.74 ± 9.53BC | 31.51 ± 4.23Aabc | 42.11 ± 2.66Aab | 78.04 ± 2.60ABa | 52.32 ± 1.78AB | 38.53 ± 0.01Ca | 64.11 ± 0.04B | |
S3-B4 | 69.31 ± 2.24Ca | 29.86 ± 3.32AB | 44.81 ± 3.93Aa | 78.59 ± 3.19ABa | 54.71 ± 2.95A | 36.35 ± 0.01Cd | 53.00 ± 0.03D | |
S3-B5 | 87.55 ± 11.60Aab | 35.60 ± 2.28Aa | 42.32 ± 3.04Aab | 78.87 ± 3.77ABa | 52.25 ± 1.51AB | 36.57 ± 0.01Cd | 55.92 ± 0.05D | |
S3-B6 | 63.72 ± 3.28Cb | 28.60 ± 2.11Ba | 45.74 ± 2.36Aa | 79.95 ± 2.52A | 55.05 ± 1.23A | 28.60 ± 0.01E | 58.71 ± 0.02Cb |
S1-B4 is 1 mg/mL SA + 4 mg/mL BSA, with the remaining combined concentrations calculated similarly. The values in the table represent the mean ± s.e. Within a column, values followed by the same letter are not significantly different. Different lowercase letters indicate a significant (P < 0.05) difference, and different uppercase letters indicate an extremely significant (P < 0.01) difference between the values.
The effect of the combined treatment with SA and BSA on sperm cryopreservation. (a) Thawed-sperm enzyme activity of MDA after the preservation of SA and BSA combined additives. (b) SOD enzyme activity. (c) T-AOC enzyme activity. (d) ROS activity. (e) Mitochondrial membrane potential. (f) Western blot analysis of oxidative stress-related protein markers. (g) Western blot analysis of apoptosis-related protein markers. (h) Western blot analysis of the PI3K-Akt signaling pathway. (i) The ratio of P-PI3K to total PI3K. (j) The ratio of P-AKT to total AKT. Data are expressed as the mean ± s.e. (s.e.m.). Significant (P < 0.05) differences between the groups are indicated by different lowercase letters, and extremely significant (P < 0.01) differences are indicated by uppercase letters. Control, control group; SA, 2 mg/mL SA; BSA, 5 mg/mL BSA; S2-B4, 2 mg/mL SA and 4 mg/mL BSA; S2-B5, 2 mg/mL SA and 5 mg/mL BSA.
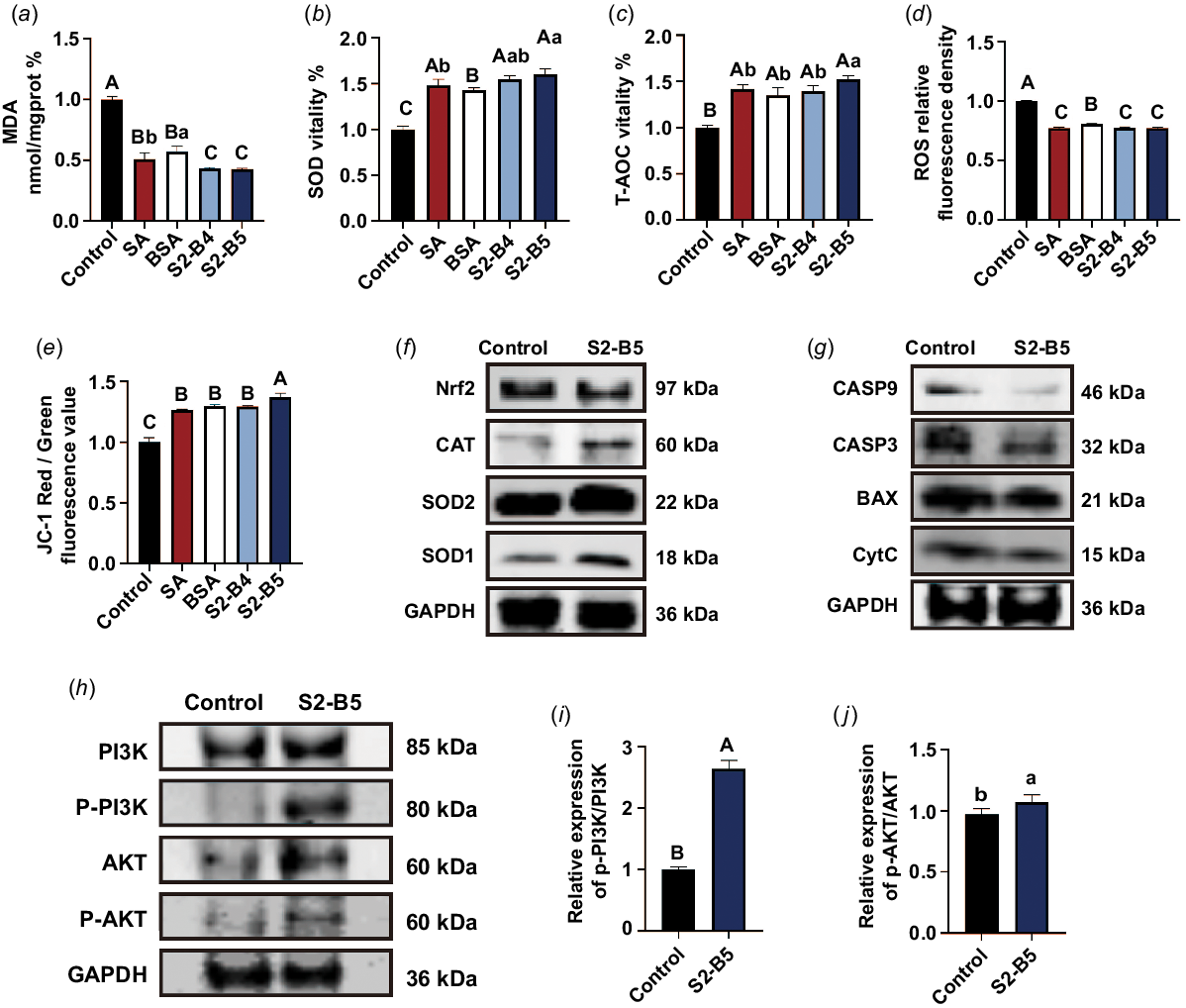
We measured protein concentrations in the S2-B5 and control groups. Among these, the oxidative stress proteins SOD1, SOD2, and CAT demonstrated that the S2-B5 group significantly alleviated the down-regulation of oxidative stress caused by frozen sperm preservation. The Nrf2 protein, a central target in the Nrf2-Keap1 pathway, and its protein concentrations also indicated that the S2-B5 group has alleviated the up-regulation of the protein during frozen sperm preservation (Figs 1f, S1c). The protein concentration of Cytc also indicated that the S2-B5 group could significantly alleviate the up-regulation during frozen sperm preservation. The apoptotic proteins Casp3, Casp9, and BAX indicated that the S2-B5 group inhibited the up-regulation of apoptosis caused by frozen sperm preservation (Figs 1g, S1d). In summary, the combined effect of adding 2 mg/mL SA and 5 mg/mL BSA into the filler resulted in an excellent comprehensive protective effect at both the apparent and protein concentrations during storage. Finally, the activities of AKT, p-AKT, PI3K, and P-PI3K at the end of the preservation period were evaluated using Western blot analysis. The results showed that the combination of SA and BSA significantly alleviated the down-regulation of phosphorylation activity in the PI3K-AKT pathway (P < 0.05 or P < 0.01) (Fig. 1h–j).
Quality control and differential protein analysis in sperm proteomics
Check the identified peptide quality error rate to assess the accuracy of omics data. The distribution of mass error rates was close to zero, with most homologous peptides falling within 5 ppm (Fig. S2a), indicating that the mass accuracy of the data meets the standard requirements. Most peptides typically consist of 8–20 amino acid residues, which is characteristic of trypsin peptides. Therefore, the sample preparation meets the technical standards (Fig. S2b). In addition, the molecular weight of the proteins is mainly distributed between 1 and 150 kDa (Fig. S2c), and the protein coverage is comprehensive (Fig. S2d), meeting the criteria for further proteomics analysis.
The total number of proteins detected in this experiment was 966, including 947 ptoteins shared between the two groups, including three proteins specific to the control group, and 16 proteins specific to the S2-B5 group (Fig. 2a). The criteria for the differential screening analysis of significantly different proteins included a P-value of <0.05 and a fold change of >2. In total, 41 differential proteins were identified in comparison between the S2-B5 and control groups, with 31 proteins being up-regulated and 10 proteins down-regulated (Fig. 2b). In addition, the differential protein enrichment analysis also showed that the two experimental groups statistically categorized the 41 differential proteins into five detailed module branches (Fig. 2c).
Differential protein screening and protein cluster analysis. (a) Presentation of common and endemic proteins among different groups. (b) Differential protein expression between the sample groups. (c) Cluster analysis of protein expression patterns, based on differential protein concentrations, was visualized using heat maps.
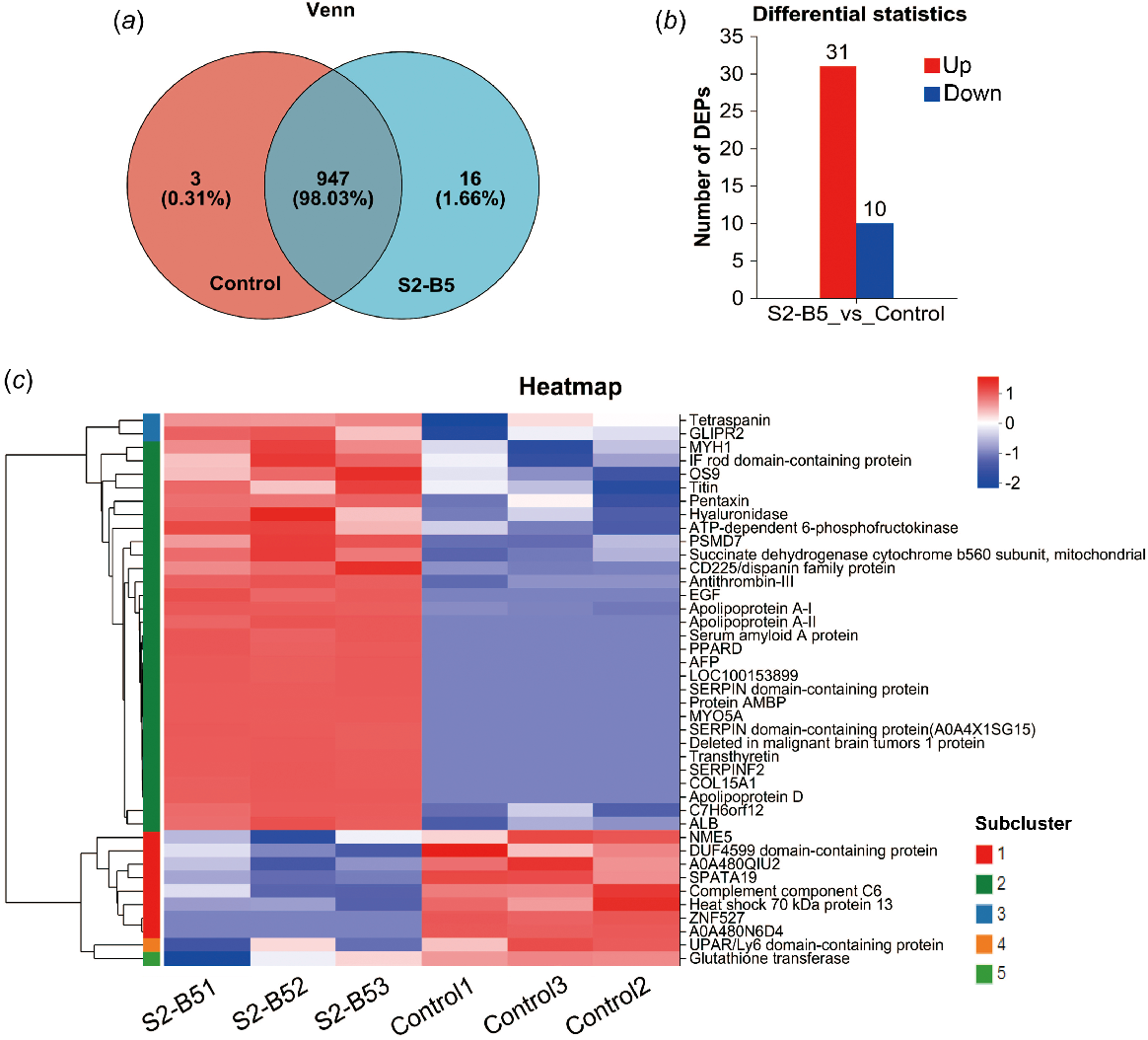
Functional enrichment analysis and interaction network analysis of differential proteins
On the basis of the functional enrichment and protein–protein interaction analyses of GO and KEGG pathways, the characteristics of differentially identified proteins in the S2-B5 group were shown. Molecular function (MF) annotation showed that these proteins are primarily involved in cation binding. Cellular component (CC) annotations predominantly identified intracellular organelles and membrane-bound organelles. The main biological process (BP) annotations included the regulation of cellular processes, regulation of multicellular organismal processes, and negative regulation of biological processes (Fig. S3). The up- and down-regulated proteins were enriched separately. In the enrichment results of up-regulated proteins, the primary enrichment was related to lipid metabolism, whereas the KEGG pathway analysis showed significant enrichment in the AMP-activated protein kinase (AMPK) signaling pathway (Fig. 3a, b). The top five results of down-regulated protein enrichment were spermatid differentiation, sperm mitochondrion organization, positive regulation of cell division, and endoplasmic reticulum organization. The KEGG pathway was mainly enriched in cytochrome P450-substance metabolism (Fig. 3c, e). Finally, an interaction analysis of the differentially expressed proteins was preformed, showing a low degree of linkage among the 41 differential proteins, with NME5 and HSPA13 being identified as the core proteins (Fig. 3d).
Differential protein enrichment analysis, protein interaction, and verification between the S2-B5 group and the control group. (a) The top five GO terms enriched by 31 up-regulated proteins. (b) The 31 up-regulated proteins were enriched in the top five KEGG terms. (c) Five KEGG pathways were identified before enriching 10 down-regulated proteins. (d) Interactions between important proteins. (e) Ten down-regulated proteins were enriched in five KEGG pathways. (f) Validation of the core differential proteins NME5 and GSTA2. (g) Quantification of GSTA2 protein concentrations. (h) Quantification of NME5 protein concentrations. Data are expressed as the mean ± s.e. (s.e.m.). Differences between groups indicated by different lowercase letters are significant (P < 0.05); different uppercase letters indicate an extremely significant (P < 0.01) difference between the values.
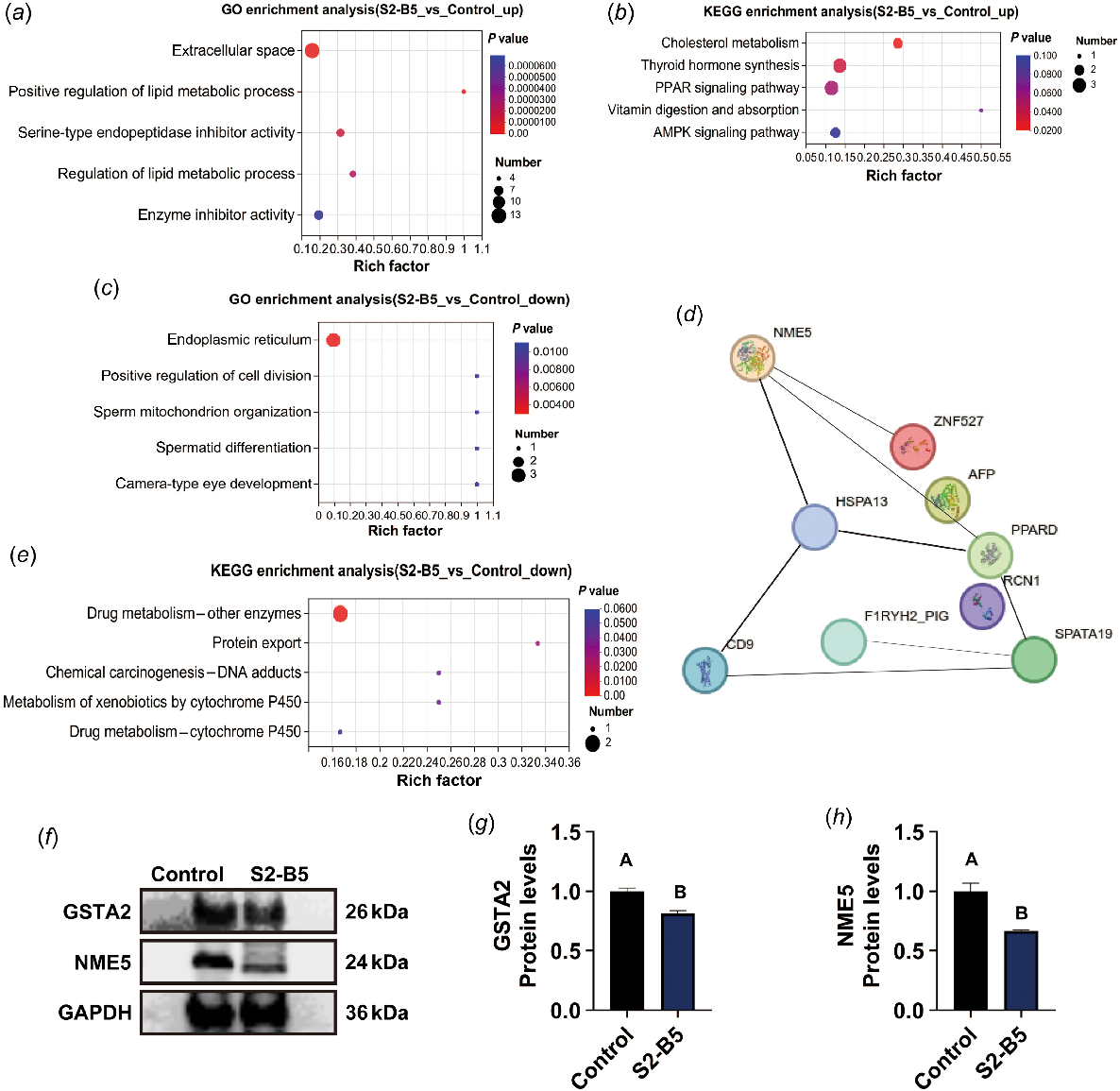
Verification of differential core proteins involved in oxidative stress
This study primarily investigated the protective effect of the interaction between SA and BSA on frozen sperm, with a focus on oxidative stress. Down-regulated protein enrichment was selected for verification, specifically the cell-differentiated oxidative protein GSTA2 and the closely associated key protein NME5. The protein concentrations suggest that the expression of NME5 and GSTA2 in the S2-B5 treatment group is lower than that in the control group (Fig. 3f–h).
Discussion
Fluid preservation of sperm is a common procedure used to maintain male fertility in livestock and domestic animals, improve the quality of sperm preservation, and reduce the expenses associated with large-scale farming (Huene et al. 2022). However, sperm cryopreservation is also associated with the emergence of oxidative stress, which is one of the primary challenges faced by this technique (Lan et al. 2022; Sharma et al. 2023). Accumulating evidence suggests that adding antioxidants to sperm preservation reagents is an important method for enhancing the quality of sperm preservation (Najafi et al. 2018; Pezo et al. 2021; Lan et al. 2022; Liang et al. 2022). In this study, SA and BSA combined filler was used as the research object to investigate their protective effect on sperm damage.
The study confirmed that the optimal concentrations for individual additions are 2 mg/mL of SA and 5 mg/mL of BSA. Previous research has demonstrated that SA positively affects the cryopreservation of Bubalus bubalis and Bos taurus sperm (Perteghella et al. 2017). In addition, an increase in the rate of in vitro fertilization was observed following BSA treatment of frozen and thawed rat sperm (Takeo et al. 2022), aligning with the outcomes of earlier experiments. A limitation of these studies was the absence of consideration for combination therapy. Currently, the addition of fructose and SA to the diluent while storing pig semen at 4°C has led to a notable enhancement in sperm quality (Xin et al. 2019). Another investigation explored the protective effects of l-cysteine and N-acetyl-l-cysteine, used in conjunction with BSA, on the sperm quality of boars during cryogenic liquid storage (Yang et al. 2024). This study lays the foundation for enhancing sperm preservation quality through the use of additives. The experimental results regarding sperm motility, antioxidant capacity, and mitochondrial membrane potential indicated that the combination treatment outperformed the single-treatment group. Additionally, this study has provided molecular-level explanations and validation. The protein concentration assay showed that the antioxidant proteinization in the combined treatment group significantly surpassed that of the control group.
Oxidative stress has been identified as a factor that can trigger apoptosis and autophagy in the male reproductive system (Sharma et al. 2023). The damage caused by cryopreservation-induced oxidative stress on sperm has been extensively studied; yet the precise mechanism of apoptosis remains unclear. In our investigation, we tested the apoptotic proteins and found that the combined additives were able to attenuate the apoptosis induced by oxidative stress resulting from cryopreservation. This finding aligns with previous studies that have demonstrated a trend of decreasing apoptosis rates following the remission of oxidative stress. Additionally, it supports earlier discoveries that oxidative stress causes apoptosis in the male reproductive system. We also use this perspective to elucidate the protective mechanism of the combined additives. The PI3K-AKT pathway, known for its significance in oxidative stress, apoptosis, and cancer treatment, has received limited attention in the context of sperm preservation mechanisms (Liu et al. 2021). Our experiment involved assessing the phosphorylation levels of PI3K and AKT, showing that the combined addition of SA and BSA to sperm cryopreservation fluid effectively counteracted the decrease in phosphorylation. At the same time, omics data have shown the presence of the PI3K-AKT pathway in the preservation of human (Najafi et al. 2018), panda (Ran et al. 2018), sheep (Ran et al. 2018; Fang et al. 2022; Ofosu et al. 2023), porcine (Dai et al. 2019), and bovine sperm (Capra et al. 2017). This is consistent with the results of our molecular tests.
The proteomic analysis identified the crucial proteins NME5 and GSTA2, which are associated with oxidative stress, along with the core proteins NME5 and HSPA13. HSPA13 primarily functions as a cellular response to heat stress, and its protein regulates the cell death response through NF-κB-mediated TNFα signaling (Espinoza et al. 2022). However, further studies are needed to understand its mechanism of action in preserving frozen sperm. The NME5 protein is involved in regulating the oxidative stress-induced intrinsic apoptosis signaling pathway and sperm cell development. Among the related conditions, primary ciliary dyskinesia is the main disease. NME5 overexpression inhibits cell proliferation (Perina et al. 2020), whereas NME5 knockout also positively affects apoptosis and oxidative stress (Li et al. 2012). The data above are similar to the results of our experiments. Additionally, a disease-related frameshift variant, was identified in the NME5 gene. Knockout of Nme5 led to defects in sperm flagella (Anderegg et al. 2019). The differential protein GSTA2 plays a crucial role in cellular detoxification against oxidative stress, carcinogens, and therapeutic drugs. Targeted inhibition of GSTA2 has been shown to suppress the growth and metastasis of HCC cells (Ng et al. 2021). Epigenetic down-regulation of GST1 and GSA2 can inhibit oxidative stress (Wilson et al. 2023). It is postulated that NME5 may have a close relationship with the antioxidant stress of GSTA2. Further research is warranted to explore its protective mechanism in frozen sperm.
Consent to publication
All authors of this article have read the paper and approve for it to be published.
Data availability
The mass spectrometry proteomics data have been deposited to the ProteomeX change Consortium (https://proteomecentral.proteomexchange.org) via the iProX partner repository with the dataset identifier PXD049712.
Conflicts of interest
The authors declare that they have no conflicts of interest in the publication of this article.
Declaration of funding
This project was supported by Natural Science Foundation of Fujian Province (2023J01446), and Fujian Province Young and Middle-Aged Teacher Education Research Project (JAT220059).
Author contributions
J. Wang: software, surveys, formal analysis, writing – original drafts, data management; Y. Rao: conceptualization, methodology, experimental inquiry; A. Wang and C. Du: visualization, survey; Y. Wu: resources, organizing material; R. Lin: writing – review and editing; T. Xiao and W. Lin: funding acquisition, resources, supervision, writing – review and editing.
References
Anderegg L, Im Hof Gut M, Hetzel U, Howerth EW, Leuthard F, Kyöstilä K, Lohi H, Pettitt L, Mellersh C, Minor KM, Mickelson JR, Batcher K, Bannasch D, Jagannathan V, Leeb T (2019) NME5 frameshift variant in Alaskan Malamutes with primary ciliary dyskinesia. PLoS Genetics 15(9), e1008378.
| Crossref | Google Scholar | PubMed |
Behnamifar A, Bernal B, Torres O, Luis-Chincoya H, GGil M, García-Casado P, Rahimi S, Woelders H, Santiago-Moreno J (2021) Research Note: evaluation of two methods for adding cryoprotectant to semen and effects of bovine serum albumin on quality characteristics of cryopreserved rooster spermatozoa. Journal of Poultry Science 100(6), 101093.
| Crossref | Google Scholar |
Capra E, Turri F, Lazzari B, Cremonesi P, Gliozzi TM, Fojadelli I, Stella A, Pizzi F (2017) Small RNA sequencing of cryopreserved semen from single bull revealed altered miRNAs and piRNAs expression between high- and low-motile sperm populations. BMC Genomics 18(1), 14.
| Crossref | Google Scholar | PubMed |
Casey TM, Khan JM, Bringans SD, Koudelka T, Takle PS, Downs RA, Livk A, Syme RA, Tan KC, Lipscombe RJ (2017) Analysis of reproducibility of proteome coverage and quantitation using isobaric mass tags (iTRAQ and TMT). Journal of Proteome Research 16(2), 384-392.
| Crossref | Google Scholar | PubMed |
Dai DH, Qazi IH, Ran MX, Liang K, Zhang Y, Zhang M, Zhou GB, Angel C, Zeng CJ (2019) Exploration of miRNA and mRNA profiles in fresh and frozen-thawed boar sperm by transcriptome and small RNA sequencing. International Journal of Molecular Sciences 20(4), 802.
| Crossref | Google Scholar | PubMed |
Espinoza MF, Nguyen KK, Sycks MM, Lyu Z, Quanrud GM, Montoya MR, Genereux JC (2022) Heat shock protein Hspa13 regulates endoplasmic reticulum and cytosolic proteostasis through modulation of protein translocation. Journal of Biological Chemistry 298(12), 102597.
| Crossref | Google Scholar | PubMed |
Fang Y, Zhao C, Xiang H, Jia G, Zhong R (2022) Melatonin improves cryopreservation of ram sperm by inhibiting mitochondrial permeability transition pore opening. Reproduction in Domestic Animals 55(9), 1240-1249.
| Crossref | Google Scholar |
Hu J, Geng G, Li Q, Sun X, Cao H, Liu Y (2014) Effects of alginate on frozen-thawed boar spermatozoa quality, lipid peroxidation and antioxidant enzymes activities. Animal Reproduction Science 147(3–4), 112-118.
| Crossref | Google Scholar | PubMed |
Huene AL, Koch JC, Arregui L, Liu Y, Nicotra ML, Weis VM, Tiersch TR (2022) Cryopreservation of Hydractinia symbiolongicarpus sperm to support community-based repository development for preservation of genetic resources. Animals 12(19), 2537.
| Crossref | Google Scholar | PubMed |
Jahanban-Esfahlan A, Ostadrahimi A, Jahanban-Esfahlan R, Roufegarinejad L, Tabibiazar M, Amarowicz R (2019) Recent developments in the detection of bovine serum albumin. International Journal of Biological Macromolecules 138, 602-617.
| Crossref | Google Scholar | PubMed |
Kumar P, Pawaria S, Dalal J, Ravesh S, Bharadwaj S, Jerome A, Kumar D, Jan MH, Yadav PS (2019) Sodium alginate potentiates antioxidants, cryoprotection and antibacterial activities of egg yolk extender during semen cryopreservation in buffalo. Animal Reproduction Science 209, 106166.
| Crossref | Google Scholar | PubMed |
Lan Q, Xie Y, Pan J, Chen Q, Xiao T, Fang S (2021) The antibacterial and antioxidant roles of Buckwheat Honey (BH) in liquid preservation of boar semen. Biomed Research International 2021, 5573237.
| Crossref | Google Scholar | PubMed |
Lan Q, Xue L, Cao J, Xie Y, Xiao T, Fang S (2022) Caffeic acid phenethyl ester (CAPE) improves boar sperm quality and antioxidant capacity in liquid preservation (17°C) linked to AMPK activity maintenance. Frontiers in Veterinary Science 9, 904886.
| Crossref | Google Scholar | PubMed |
Li F, Hu G, Jiang Z, Guo J, Wang K, Ouyang K, Wen D, Zhu M, Liang J, Qin X, Zhang L (2012) Identification of NME5 as a contributor to innate resistance to gemcitabine in pancreatic cancer cells. The FEBS Journal 279(7), 1261-1273.
| Crossref | Google Scholar | PubMed |
Liang J, Lv C, Yang H, Zhang Y, Raza SHA, Wu G, Quan G (2022) Ultrastructural modification of ram sperm frozen with cyclohexanediol and trehalose. Biopreservation and Biobanking 20(4), 348-356.
| Crossref | Google Scholar | PubMed |
Liu L, Yan M, Yang R, Qin X, Chen L, Li L, Si J, Li X, Ma K (2021) Adiponectin attenuates lipopolysaccharide-induced apoptosis by regulating the Cx43/PI3K/AKT pathway. Frontiers in Pharmacology 12, 644225.
| Crossref | Google Scholar | PubMed |
Mehdipour M, Daghigh-Kia H, Najafi A, Mehdipour Z, Mohammadi H (2022) Protective effect of rosiglitazone on microscopic and oxidative stress parameters of ram sperm after freeze-thawing. Scientific Reports 12(1), 13981.
| Crossref | Google Scholar | PubMed |
Najafi A, Adutwum E, Yari A, Salehi E, Mikaeili S, Dashtestani F, Abolhassani F, Rashki L, Shiasi S, Asadi E (2018) Melatonin affects membrane integrity, intracellular reactive oxygen species, caspase3 activity and AKT phosphorylation in frozen thawed human sperm. Cell and Tissue Research 372(1), 149-159.
| Crossref | Google Scholar | PubMed |
Ng KT-P, Yeung OW-H, Lam YF, Liu J, Liu H, Pang L, Yang XX, Zhu J, Zhang W, Lau MYH, Qiu WQ, Shiu HC, Lai MK, Lo CM, Man K (2021) Glutathione S-transferase A2 promotes hepatocellular carcinoma recurrence after liver transplantation through modulating reactive oxygen species metabolism. Cell Death Discovery 7(1), 188.
| Crossref | Google Scholar | PubMed |
Ofosu J, Nartey MA, Mo X, Ye J, Zhang Y, Zeng C, Zhang M, Fang Y, Zhou G (2023) Ram sperm cryopreservation disrupts metabolism of unsaturated fatty acids. Theriogenology 204, 8-17.
| Crossref | Google Scholar | PubMed |
Peña FJ, Gibb Z (2022) Oxidative stress and reproductive function: oxidative stress and the long-term storage of horse spermatozoa. Reproduction 164(6), F135-F144.
| Crossref | Google Scholar | PubMed |
Perina D, Korolija M, Mikoč A, Halasz M, Herak Bosnar M, Ćetković H (2020) Characterization of Nme5-Like Gene/Protein from the Red Alga Chondrus Crispus. Marine Drugs 18(1), 13.
| Crossref | Google Scholar | PubMed |
Perteghella S, Gaviraghi A, Cenadelli S, Bornaghi V, Galli A, Crivelli B, Vigani B, Vigo D, Chlapanidas T, Faustini M, Torre ML (2017) Alginate encapsulation preserves the quality and fertilizing ability of Mediterranean Italian water buffalo (Bubalus bubalis) and Holstein Friesian (Bos taurus) spermatozoa after cryopreservation. Journal of Veterinary Science 18(1), 81-88.
| Crossref | Google Scholar | PubMed |
Pezo F, Yeste M, Zambrano F, Uribe P, Risopatrón J, Sánchez R (2021) Antioxidants and their effect on the oxidative/nitrosative stress of frozen-thawed boar sperm. Cryobiology 98, 5-11.
| Crossref | Google Scholar | PubMed |
Ran MX, Li Y, Zhang Y, Liang K, Ren YN, Zhang M, Zhou GB, Zhou YM, Wu K, Wang CD, Huang Y, Luo B, Qazi IH, Zhang HM, Zeng CJ (2018) Transcriptome sequencing reveals the differentially expressed lncRNAs and mRNAs involved in cryoinjuries in frozen-thawed Giant Panda (Ailuropoda melanoleuca) sperm. International Journal of Molecular Sciences 19(10), 3066.
| Crossref | Google Scholar | PubMed |
Rosato MP, Iaffaldano N (2013) Cryopreservation of rabbit semen: comparing the effects of different cryoprotectants, cryoprotectant-free vitrification, and the use of albumin plus osmoprotectants on sperm survival and fertility after standard vapor freezing and vitrification. Theriogenology 79(3), 508-516.
| Crossref | Google Scholar | PubMed |
Shaikh MAJ, Gupta G, Afzal O, Gupta MM, Goyal A, Altamimi ASA, Alzarea SI, Almalki WH, Kazmi I, Negi P, Singh SK, Dua K (2023) Sodium alginate-based drug delivery for diabetes management: a review. International Journal of Biological Macromolecules 236, 123986.
| Crossref | Google Scholar | PubMed |
Sharma P, Kaushal N, Saleth LR, Ghavami S, Dhingra S, Kaur P (2023) Oxidative stress-induced apoptosis and autophagy: balancing the contrary forces in spermatogenesis. Biochimica et Biophysica Acta (BBA) - Molecular Basis of Disease 1869(6), 166742.
| Crossref | Google Scholar | PubMed |
Takeo T, Nakao S, Mikoda N, Yamaga K, Maeda R, Tsuchiyama S, Nakatsukasa E, Nakagata N (2022) Optimized protocols for sperm cryopreservation and in vitro fertilization in the rat. Lab Animal 51(10), 256-274.
| Crossref | Google Scholar | PubMed |
Wilson MM, Danielian PS, Salus G, Ferretti R, Whittaker CA, Lees JA (2023) BMI1 is required for melanocyte stem cell maintenance and hair pigmentation. Pigment Cell & Melanoma Research 36(5), 399-406.
| Crossref | Google Scholar | PubMed |
Xu X, Hu J, Xue H, Hu Y, Liu Y-N, Lin G, Liu L, Xu R-A (2023) Applications of human and bovine serum albumins in biomedical engineering: a review. International Journal of Biological Macromolecules 253(Pt 3), 126914.
| Crossref | Google Scholar | PubMed |
Yánez-Ortiz I, Catalán J, Rodríguez-Gil JE, Miró J, Yeste M (2022) Advances in sperm cryopreservation in farm animals: cattle, horse, pig and sheep. Animal Reproduction Science 246, 106904.
| Crossref | Google Scholar | PubMed |
Yang D, Yu X, Li X, Yu B, Peng H (2024) Protective effects of l-cysteine and N-acetyl-l-cysteine on boar sperm quality during hypothermic liquid storage with bovine serum albumin as a protectant. Theriogenology 216, 185-195.
| Crossref | Google Scholar | PubMed |
Yu L, Wei J, Liu P (2022) Attacking the PI3K/Akt/mTOR signaling pathway for targeted therapeutic treatment in human cancer. Seminars in Cancer Biology 85, 69-94.
| Crossref | Google Scholar | PubMed |