A codeveloped management tool to determine harvest limits of introduced mud crabs, Scylla serrata (Forskål, 1775), within a Native Hawaiian fishpond
Kaleonani K. C. Hurley A E , Maia Sosa Kapur B , Margaret Siple C , Keli‘iahonui Kotubetey D , A. Hi‘ilei Kawelo D and Robert J. Toonen AA Hawai‘i Institute of Marine Biology, University of Hawai‘i at Mānoa, 46-007 Lilipuna Road, Kāne‘ohe, HI 96744, USA.
B School of Aquatic and Fisheries Sciences, University of Washington, 1122 NE Boat Street, Seattle, WA 98105, USA.
C Department of Ecology, Evolution and Marine Biology, University of California, Santa Barbara, CA 93106, USA.
D Paepae o He‘eia, Kāne‘ohe, HI 96744, USA.
E Corresponding author. Email: hurleyk@hawaii.edu
Pacific Conservation Biology 27(4) 418-431 https://doi.org/10.1071/PC20023
Submitted: 25 February 2020 Accepted: 29 September 2020 Published: 30 October 2020
Journal Compilation © CSIRO 2021 Open Access CC BY-NC-ND
Abstract
Hawaiian fishponds, or loko i‘a, are ancient aquaculture systems and models of sustainable aquatic resource management from traditional Native Hawaiian harvest practices. Of the 488 fishponds documented in ancient Hawai‘i, only 38 are currently actively managed. Building on Indigenous and local knowledge, fishponds are being adapted to current community needs. Functional fishponds perpetuate culture, improve food security, enhance ecosystem services and transform conservation biology through Indigenous perspectives. Here we examine how Indigenous practices effectively maintain sustainable harvest of an introduced, but economically important, crab species, the mud crab, Scylla serrata (Forskål 1775). The State of Hawai‘i has only a size limit, and no bag limits, for this species. With Indigenous management in a Hawaiian fishpond, limits are set and enforced in response to fluctuations in catch. We used a mark–recapture experiment to parameterise a size-structured population model, which we used to investigate the impact of changing harvest rates. Throughout the study period of 2017–20, the mean number of crabs per harvest was 28 individuals (s.d. = 7) and average catch per unit effort was 0.20 crabs per trap-hour (s.d. = 0.054). During winter, catch per unit effort was lower but mean crab size was larger than during summer. Model simulations indicated that current Indigenous practices are less likely to cause a decline in population growth rate than a strict size limit. Using information from Indigenous harvest practices and the mark–recapture study, we codeveloped a versatile crab population model that can be tailored to changing management objectives.
Keywords: aquaculture, conservation biology, crab fishery, food security, Hawaiian fishponds, mark–recapture, mud crab, non-native species, Scylla serrata, size-based model.
Introduction
Global concerns regarding sustainability and food security have led in recent years to increased interest in Indigenous knowledge systems for solutions (Howitt 2002; Johnson et al. 2016; Price and Toonen 2017). Ancient island societies throughout Oceania typically relied on restricted access to resources to sustain their populations (Kirch and Hunt 1997; Anderson 2009). Kānaka maoli, Native Hawaiians, had a system of nested land divisions for resource management, referred to in some literature as the ahupua‘a system, that simultaneously fulfilled social and spiritual well-being as well as providing sustainable food production for people without access to resources outside of the islands (Kamakau 1976; Kurashima et al. 2018; Winter et al. 2018, 2020). Hawaiian fishponds, or loko i‘a, are one of the most important technological advances of ancient aquaculture systems, and they provide a model of sustainable aquatic resource management based on long-term experience from traditional Native Hawaiian harvest practices (Kikuchi 1976). A survey of commercial fisheries in 1901 estimated that 350 fishponds had been in operation in pre-European-contact Hawai‘i (around the year 1778), but only 99 remained in production at the publication of the survey (Cobb 1901; Cobb 1905). Approximately 2 million pounds of fish (primarily mullet and milkfish) were estimated to be the annual combined output from fishponds before Western contact. This production was part of a management strategy that provided food security to support a population of ~1 million people, nearly equivalent to present day, without any external food subsidy (Stannard 1989; Dye 1994; Kurashima et al. 2019). Over the past century, the last of the fishponds in active production fell to disrepair as colonial efforts to disassemble the ahupua‘a system of management and redistribute land resulted in many fishponds being filled in for coastal housing developments, and upstream land developments that made the upkeep of remaining fishponds more difficult (Kikuchi 1976; Farber 1997). By the 1970s, fewer than 100 fishponds remained functional, with a combined annual fish output estimated at ~9072 kg (Apple and Kikuchi 1975; DHM, Inc 1989; Keala et al. 2007). Obstacles to restoration and upkeep include the introductions of many terrestrial and aquatic species (MacCaughey 1917; Moulton and Pimm 1986; Eldredge and Carlton 2002), as well as increases in coastal development, sedimentation, eutrophication and heavy metal contamination (Keala et al. 2007).
Following the loss of fishpond functionality, reclamation efforts beginning in the 1970s have resulted in the rejuvenation of 38 fishponds across the State. In addition, fishponds are being adapted to modern human population needs because functional fishponds contribute to improved food security. Modern Hawai‘i residents are almost entirely dependent on receiving resources from outside of the State, with ~90% of food being imported (USA DOC 2012). However, the value of fishponds extends far beyond food security. Fishponds also contribute to hazard resilience by offering protection from coastal storms, support the revitalisation of Indigenous cultural heritage, and provide invaluable tools for education about healthy coastal ecosystems, climate change impacts on the environment, and sustainable coastal development through a process of ecomimicry (Winter et al. 2020).
The addition of modern research techniques to established Indigenous and local knowledge (ILK) may provide for open and informed decision making about the best strategies to manage sustainable harvest of resources given increasing interest in locally grown and harvested food (Pascua et al. 2017; Winter et al. 2020). To better understand the potential for increased production and food security, we first need to establish a baseline for current activity and determine levels of harvest that are sustainable. He‘eia Fishpond, located on the windward coast of O‘ahu, Hawai‘i, is a loko i‘a in active restoration and serves as a model for Native Hawaiian marine resource management (Fig. 1a).
![]() |
One of the most reliable fishery resources at He‘eia Fishpond, and the focus of this study, is the mud crab, Scylla serrata (Forskål 1775). Scylla serrata is found across the Indo-West Pacific in estuarine habitats and is often associated with coastal shallows and mangroves (Gopurenko et al. 1999; Williams and Primavera 2001). Although S. serrata is called a mud or mangrove crab throughout its range, in the Hawaiian Islands it is known by the common name of Samoan crab due to the origin of introduction. From 1926 to 1935, approximately 100 individuals of S. serrata gathered from Sāmoa were intentionally introduced across the islands of O‘ahu and Hawai‘i to start a commercial crabbing industry (Brock 1960). As early as 1936, S. serrata was reported to cause damage to coastal fishpond environments, including the destruction of seawalls by burrowing beneath them (Anon. 1936). In 1940, S. serrata was seen throughout the remaining main Hawaiian Islands and had even invaded major streams upland of estuarine habitats (Edmondson and Wilson 1940). More recently, a 2004 study on the effects of fishing gear in the Northwestern Hawaiian Islands of Papahānaumokuakea reported S. serrata as by-catch off Nalukākala (Maro Island) and Mokumanamana (Necker Island) (Moffitt et al. 2006). In addition to its spread across the archipelago, S. serrata has been observed dragging wetland bird chicks under the water (Harmon, pers. comm.).
Despite being an introduced organism with a largely undocumented ecological impact in Hawai‘i, S. serrata has high market value locally in the islands and throughout the Indo-Pacific where it is also cultivated through aquaculture in many locations (Quinitio et al. 2001; Triño and Rodriguez 2002; Mirera et al. 2013). In 1999, the total global catch of S. serrata was 13 431 tonnes, with Indonesia and Thailand reporting the largest catches (8560 and 3050 tonnes respectively) (FAO 2001). As a fishery species, it is regulated by the State of Hawai‘i with a size minimum of 6 inches or 152 mm for take of males and no-take of any females, with no bag limits (Hawai‘i Administrative Rules (HAR) §13-84, HAR§13-95, Hawai‘i Revised Statutes (HRS) §188-58; DAR 2015). In addition to limits on size and sex of crabs, there are restrictions on type of gear that may be used for harvest. Gear such as nets or hard traps (i.e. plastic or metal) are limited to those nets and traps with 5-cm holes or larger (HAR§13-75). However, local management in the Hawaiian Islands should recognise the potential for negative ecological impacts of unchecked crab populations, such as coastal habitat destruction and predation on native animals (Reaser et al. 2007; Tavares and Mendonca 2011), as well as the economic value of crabs when setting rules for catch.
Current harvesting practices for S. serrata in He‘eia Fishpond appear to be sustainable because fishpond records indicate that yields have not changed. Our goal here was to codevelop an ILK-informed fisheries model to predict sustainable harvest limits within a Native Hawaiian fishpond. The loko i‘a o He‘eia, managed by the non-profit organisation Paepae o He‘eia (POH), makes an ideal model system to evaluate this hybrid approach to sustainable fisheries management because the resource managers are also cultural practitioners who enact the harvest and are able to incorporate observed changes in size, abundance and harvest efficiency directly and immediately. Recognising that fishpond managers’ goals are likely to change through time, we use data collected by POH together with new information from a more intensive survey effort to inform the model and explore the predicted population responses of the crab population within He‘eia fishpond to different harvest scenarios. By developing a model from the historical observations of the Indigenous population of the place, supplemented with parameter estimates from our mark–recapture data, we sought to codevelop a versatile tool that would enable local managers to consider a range of harvest scenarios to determine the management strategy best suited to specific goals. For example, management may currently seek to maximise sustainable harvest and secure financial benefits, but future management may aim to fish out the entire introduced population to allow for native species restoration. Such a diversity of goals requires a modelling approach that allows managers to quickly explore predicted outcomes of differing harvest levels and update their management strategy through time. Codevelopment of this tool ensures open and transparent communication and provides information for changing strategies as goals change through time at the He‘eia fishpond.
Materials and methods
Study site
He‘eia Fishpond is a 0.35-km2 Hawaiian fishpond, or loko i‘a, located on the eastern side of the island of O‘ahu, Hawai‘i (21.431584°N, 157.806970°W) (Fig. 1a). As a loko kuapā, this particular fishpond is characterised by a 2.5-km coral and basalt lava rock wall that encompasses the pond (Fig. 1b). The kuapā wall is built on the fringing reef, Malauka‘a. The semipermeable wall allows for the slow flow of seawater between the inside and outside of the pond in order to maintain water level while forcing flow to the sluice gates. The movable sluice gates fitted into the wall are used to control the flow of either fresh or salt water. He‘eia Fishpond is under active restoration and is one of the few loko i‘a producing food on O‘ahu. This loko i‘a is cared for by POH, a non-profit, Native Hawaiian community-based organisation whose mission is to maintain the fishpond as a foundation for cultural sustainability. POH manages fishpond resources according to a combination of traditional management practices, hands-on education programs, and scientific research. In 2017, He‘eia Fishpond was included in the designation of the He‘eia National Estuarine Research Reserve.
Crabbing data
The first set of data was collected from March 2017 through February 2020 at He‘eia Fishpond. Crabbing sessions occurred once a month from March 2017 through February 2018 unless the first harvest did not yield enough marketable crabs, in which case there was a second harvest (May 2017 and June 2017). The closed season was designated by POH as July 2017 to November 2017 and May 2018 to August 2018. This voluntary closure covers the period in which low numbers of marketable-sized crabs have been historically observed. Traps were deployed during the 2017 closed season to simulate normal crabbing operations; however, all crabs were released after data collection. During the closed season, it was noted which crabs would have been harvested for market before they were actually released. From March 2018 through April 2018 and September 2018 through April 2019, crabs were captured twice a month.
Crabs were harvested using Fathoms Plus (Los Angeles, CA, USA) shellfish traps, which are exclusively used for S. serrata in the fishpond due to the structural integrity of the traps. Fathoms Plus traps are lightweight, oval-shaped polyethylene traps (50.8 cm tall by 86.4 cm long) that are hinged on the bottom and open from the top for accessing captured organisms. There is a 12.7 cm by 21.5 cm conical tapering entrance on each of the long sides of the oval and the mesh size is 4 cm. These traps are restricted outside of the fishpond in public waters; however, the pond is not publicly accessible as it is on privately owned property. All harvest activities are carried out by staff for commercial sales that benefit POH, which uses the funds to support fishpond management. Although there are no catch or gear restrictions in privately owned coastal properties such as this loko i‘a, the State of Hawai‘i restricts S. serrata catch to males only (HAR§13-84, HAR§13-95, HRS§188-58) at 152 mm or larger in carapace width (left to right measurement across the widest part of the dorsal surface of the carapace) with no bag limits. To adhere as closely as possible to State law, POH staff limit their catch to 10 legal-sized male crabs per month that may be gathered in 1–2 trap soaks per session. A single soak is usually 24 h, and there may be two consecutive days in a single session. Three to seven baited traps were deployed individually throughout the fishpond for each session. The number of traps laid is chosen by considering anticipated catch per trap based on staff experience. All traps were deployed and retrieved by hand from a small boat. Depending on daily tidal variation, the traps were submerged in 0.5–1-m-deep water.
We recorded the following data at each harvest from March 2017 through April 2019: (1) deployment and retrieval date and time for each harvest session; (2) location of each trap with GPS unit (Garmin GPS 72H); (3) total number of crabs per trap; (4) carapace measurements (carapace width (CW), referred to throughout as ‘length’ in the Methods to be consistent with notation), weight, and sex of each crab; and (5) status of each crab as: removed from the fishpond (‘kept’), released back into the fishpond, or found dead. While the study completed data collection in April 2019, POH staff continued to collect catch data but not size data for the period of May 2019 through February 2020. As size data were not collected during this period, the catch data during this period were included in calculations for monthly catch per unit effort (CPUE) but not the fishery model.
Crab CWs were measured following the standard methodology across the widest point of the dorsal surface of the carapace using a Haglöf Sweden’s Mantax Blue caliper. Weights were recorded using an Ohaus Valor 4000 digital scale. Sex was determined by examining the shape of the abdominal flap on the ventral side of each crab. Males reach sexual maturity at 90–110 mm CW, and it is at this stage that they develop a distinct triangular-shaped abdominal flap. Conversely, the abdominal flap is shaped like a ‘U’ for mature females and may not be obvious until larger than this size range (Shelley and Lovatelli 2011). Immature crabs whose sex could not be determined from telson shape were weighed and measured, but sex was recorded as ‘undetermined’. These crabs were excluded from the analysis. No females caught in traps were obviously gravid (no eggs exposed). The relationship between weight and CW (W = aLbeϵ ) was fitted separately for male and female crabs using data from all crabs caught during the experiment.
Monthly catch rate (as CPUE) was calculated from catch data during the sampling period of March 2017 through February 2020 as total catch over trap hours:

with units as crabs per trap hour. CPUE is used as a proxy for abundance. CPUE standardises catch by accounting for differences in effort that result from variations in numbers of traps used and periods during which traps are deployed (soak time). For months in which multiple harvests occurred, CPUE was calculated as sums when harvests were consecutive days or averaged when harvests were not consecutive (i.e. 2 weeks apart).
Mark and recapture
In order to track adult crab movements and quantify available stock in the fishpond, we used mark and recapture methods (Krebs 1989). Beginning in March 2018, all crabs that were released back into the fishpond during crabbing sessions were tagged with T-bar anchor tags coded with unique identifiers (Hallprint, Australia). Although crabs are ecdysiasts and need to moult in order to grow, T-bar anchor tags are designed to be inserted between the carapace and first thoracic segment in order to be retained through moulting (McPherson 2002; Meynecke et al. 2015). To ensure that the maximum number of tagged crabs would be captured, a larger-scale dedicated effort to tag crabs occurred in March and April 2019. Over 3 days in each month, 12 traps were baited and deployed. All crabs captured were tagged and released.
Sustainable fishery model
Estimate of adult survival
Life history parameters used in this study are described in Table 1. Adult survival (Sa) was estimated from the mark–recapture data using a Cormack–Jolly–Seber (CJS) model (Cormack 1964; Jolly 1965; Seber 1965). The CJS model assumes that the population is open and that the population size changes over time due to natural mortality. Mark and recapture data (n = 112 individuals) were divided into sampling periods, such that every sampled month following the initial tagging represented one observation instance (with a mean time of 1.63 months between surveys). We assumed that parameters were constant, i.e. that survival probability did not change during the study period. Priors for each parameter were uniform between 0 and 1 because there was insufficient information to confidently constrain priors. The model was sampled from four chains with 1000 post-warmup draws per chain, thinning every other iteration. The CJS model was implemented in Stan (Carpenter et al. 2017; Stan Development Team 2018).
![]() |
Mortality and survivorship
Instantaneous mortality at age was estimated following O’Neill et al. (2010), who applied the Hoenig equation to Australian spanner crab (Ranina ranina). MH is calculated from the Hoenig equation for generic marine species (Hoenig 1983). Maximum longevity (tmax) is unknown for S. serrata so longevity was randomly drawn from a uniform distribution between 7 and 16, a range based on the aquaculture literature on S. serrata (Mwaluma 2002). We accommodate for uncertainty in this estimate by varying the shape parameter (z), thus introducing variation in the estimate of natural mortality and survivorship at age (constant natural mortality occurs at z = 0).


where the shape parameter is fixed at z = 0.3 and

For younger ages, natural survivorship at age was calculated as a function of the shape parameter z, which allowed natural mortality to vary across sizes. The Hoenig method for calculating M was used for young size classes, and the survival rate estimated from the CJS mark–recapture model was used to determine the mortality of individuals larger than 140 mm (the minimum size of crabs recaptured in the mark–recapture experiment).
Survivorship at age was calculated as:

where S1 is the larval survival, and St is the annual survival of adult crabs, based on mark–recapture estimates.
Lefkovitch matrix structure
The present study characterised the intrinsic population growth rate (r) for Samoan crab populations using a size-structured Lefkovitch matrix population model (Eqn 4) implemented in R (R Core Team 2019). Here, we represent size using CW, which is also the basis for the harvest rule for S. serrata in Hawai‘i. The generalised form of the Lefkovitch matrix (Lefkovitch 1965) is:
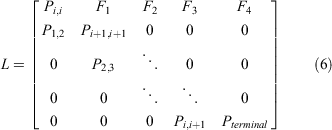
Columns represent size bins, which were grouped as follows: 11–60, 61–80, 81–100, 101–120, 121–140, 141–160, 161–180, 181–211, and ≥212 mm CW. Fertility rates are given per size bin in the top row; within-bin survival probabilities in diagonal (Pi,i); survival between size bins in subdiagonal Pi,i+1. Upper-left and lower-right values represent the probability that new recruits remain recruits, and the survivorship of the terminal size bin, respectively.
The Lefkovitch matrix approach assumes that fertility and survival rates are constant through time and unaffected by density dependence; that the demography of the population can be determined from analysis of female dynamics alone; and that the size bins specified are of appropriate resolution (Caswell 1990). The simulation framework is stochastic, thus accounting for uncertainty about certain aspects of S. serrata life history to generate an estimate for demographic parameters (Aires-da-Silva and Gallucci 2007). The initial distribution of estimates for r (calculated as the log of the dominant eigenvalue of the composite harvest and Lefkovitch matrix) and other demographic values were generated under a baseline scenario wherein fishing mortality of females was set to zero. This effectively represents a scenario with zero harvest.
Growth
The size transition matrix X was formulated as follows (Siddeek et al. 2016):
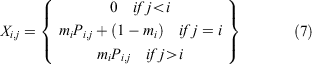
where mi is the 1-year moult probability for a crab in size bin i and Pi,j is the probability density of a given growth increment. The probability of one individual crab growing from size bin i to size bin j is drawn from a normal distribution with mean μi.
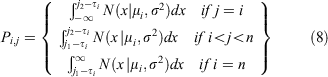

The expected growth increment for a crab in size bin i, μi was estimated using the midpoint of the contributing size bin (τi) and parameters a and b as estimated from the von Bertalanffy growth function. Von Bertalanffy growth parameters were estimated from size and age data on S. serrata collected in Kosrae, Micronesia (Bonine et al. 2008). Using the age at first capture (approximately 182 days), mean CW for each captured life stage, and estimated intermolt times reported by Bonine et al. (2008), we estimated von Bertalanffy growth parameters (asymptotic length L∞ (in millimetres), and growth rate coefficient K (in millimetres year−1), fixing t0 at –0.0015 (years). These values were then used to estimate the expected growth increment via the following:
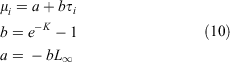
We parameterised the probability of moulting for a crab in size bin i, mi using the relationship between size and intermoult period reported by Moksnes et al. (2015):

Moult probabilities were used to generate individual variation in transition probability between size bins.
Reproduction
Egg production (eggs × 1000) was modelled as a function of size bin i:

where fi is the fecundity of crabs in size bin i, Oi is the proportion of mature females in size bin i, and EPR is the number of eggs per recruit. We assume a sex ratio of 50:50 for newly settled larvae.
Size-specific fecundities were derived from the size–egg equation proposed for S. serrata in Bangladesh (Sarower et al. 2012). Crabs below 100 mm CW were assumed to not produce any eggs.

The proportion of mature females in size bin i was calculated as:

where 15 550 is the slope of the relationship between CW and eggs produced (the additional eggs produced with a 1-mm increase in CW) (Sarower et al. 2012), t is the estimated age at size using an inverse von Bertalanffy curve, and t50 is the length at 50% maturity (t50 = 95.5 mm). This estimate equates to the expected egg output per spawning event per female in size bin i.
Current fishery selectivity
Fishery selectivity refers to the probability that crabs of a given size (CW) will be caught in fishing gear. In He‘eia fishpond, the general harvest rule for S. serrata is that only males weighing 700 g and above are kept (this corresponds to a CW of 140–160 mm). To develop a fishery selectivity scenario that was representative of current harvest practices, we developed a logistic selectivity curve based on the sizes of crabs that were kept during the mark–recapture experiment. We estimated fishery selectivity from kept crabs using length compositions following Nadon (2017):

where L is the length at which selectivity is being estimated, LS50 is the length at 50% selectivity (i.e. where crabs have a 50% chance of being caught in the trap), and LS95 is the length at 95% selectivity. Following Nadon (2017), we defined the first discontinuous break in the size composition of kept crabs as the length at 95% selectivity (LS95 = 195 mm), and LS50 is the midpoint between the minimum size kept and LS95 as the length at 50% selectivity (LS50 = 167.5 mm) (Fig. 2). The selectivity for the midpoint of each size bin was used as the selectivity for the entire size bin. This method has been used for other species in Hawai‘i with size restrictions where the ‘true’ selectivity varies around the actual size limit (DeMartini et al. 2002; Nadon 2017).
![]() |
Harvest scenario risk analysis
We investigated the response of the population intrinsic growth rate r to variations in the harvest mortality and minimum size selectivity at first capture of female crabs. Our risk analysis investigated 40 different unique harvest strategies, each characterised by a harvest mortality (ranging from 0 to 0.9) and minimum size at first capture (0, 76, 95.5, and 152 mm CW). The smallest size captured in the fishery was 76 mm; 95.5 mm is the length at 50% selectivity (LS50), and 152 mm is the state legal limit. We tested 40 mortality and size at capture combinations, for a total of 40 000 unique simulations. The distributions indicated the spread of estimated growth rates r for a specific minimum size selectivity; r values less than zero indicate a declining population. This ‘trigger point’ analysis illustrated which size cutoffs result in population declines for S. serrata in He’eia. This approach allowed for the exploration of different harvest strategies on the population growth rate.
Results
Crabbing data
A total of 885 individuals were caught and measured during this study period of March 2017 through April 2019. Catch size and CPUE (Fig. 3) varied across harvests, with a maximum of 45 crabs caught in a single session (November 2019), which corresponded to a total CPUE of 0.23 crabs per trap-hour. For the open season months (March 2017 to May 2017; December 2017 to April 2019), the greatest number of crabs removed from the fishpond was 25 individuals (March and April 2018) with a corresponding total CPUE of 0.23 crabs per trap-hour and a keeps-only CPUE of 0.16 crabs per trap-hour. The greatest number released was 36 individuals. The maximum CPUE value for total catch was 0.3 crabs per trap-hour in November 2017, which corresponded with 44 crabs caught but only 9 matched criteria for keeps. These crabs were ultimately released as November 2017 was the end of the closed season. The lowest CPUE value for total catch was 0.11 crabs per trap-hour in March 2019, which corresponded with 20 crabs caught but ultimately only 9 kept. In these cases, the CPUE values for kept crabs only was 0.062 and 0.051 respectively.
The largest individual had a CW of 200 mm, and the heaviest individual was 2.2 kg (both males). During the self-imposed closed season (June–November 2017), the median crab size was below legal limits. Outside of this period, the crab sizes are typically greater than the legal limit (Fig. 4). Weight restrictions are also part of the self-imposed restrictions at the fishpond, with a minimum weight limit of 0.7–1 kg (1.5–2.5 lb) set for crabs that are removed for sale. The catch varies through the year, with few crabs below sexual maturity and the majority of catch over legal size (152 mm) during 2018 (Fig. 4).
In addition to variation in catch size, composition of crab catch also varied seasonally. A maximum of 44 males was seen in a single month when multiple harvests occurred, or a minimum of 18 with a mean of 28 males. In contrast, the maximum number of females was 12 in a month with multiple harvests, with 4 females minimum, and a mean of 7 females. Across the entire year of sampling, 78% of catch were males and 20% were females (the remaining 2% were immature or crabs whose sex could not be determined due to damage). Based on a comparison of size and weight, males were typically found to be larger overall, and weighed more than females of the same CW (Fig. 5). The heaviest male was 2200 g at CW 194 mm while the heaviest female was 1300 g at CW 200 mm. The relationship between weight and CW was different for males and females. For males, the intercept a was 5.7 × 10−6 with slope b of 3.74. For females, the intercept a was 1.6 × 10−4 with slope b of 3.0.
![]() |
Landings (catches that were retained) consisted of marketable crabs that met the self-imposed POH weight limit of 1000 g by month. During the open season (March–June 2017, December 2017–March 2018), the maximum catch was in March 2017 with combined weight of marketable crabs at 32 800 g, and the minimum catch was in June 2017 with combined marketable weight of 8800 g. The average weight of combined marketable catch during the open season was 20 900 g. During the closed season (July–November 2017), the maximum total weight of marketable crabs was 10.8 kg in September 2017 and the minimum total weight was 2300 g in August 2017. The average weight of marketable crabs caught during the study period was 8600 g.
Mark and recapture
From March 2018 to February 2019, a total of 90 crabs was tagged and released. Of this first round of tagged crabs, there were 49 females, 30 males, and two immature (based on abdominal flap shape). Three of these crabs were recaptured, each within a month of being tagged. Due to the low number of tagged crabs and the subsequent low recapture rate of tagged crabs, there was a dedicated tagging effort in March and April 2019 that resulted in a total of 209 crabs tagged and released (52 females, 145 males, and 12 immature). From the first dedicated tagging event in March 2019, 40 tagged crabs were recaptured. June 2019 was the last time a tagged crab was recaptured. The size range of crabs for which data were collected was 76 mm to 180 mm (Fig. 2).
Estimate of adult survival
The parameter Sa is a survival rate per time period estimated from the mark–recapture data. We estimated a monthly survival rate of 0.979 [95% confidence interval 0.930, 0.999], which corresponds to an annual mortality rate of Madult = 0.224 [0.113, 0.581]. Because these parameters were estimated from unfished individuals taken from the fishpond, we assume that they are representative of natural mortality and survival rates. We estimated a recapture probability of pa = 0.014 [0.011, 0.024].
Mortality and survivorship
In the baseline model, where there was no harvest mortality, very few crabs survived the larval stage, and mortality was based on results from the mark–recapture experiment, resulted in a survivorship curve that increased with age from an average minimum for the lowest size bin of 0.0001 to an average maximum of 0.56.
Growth
We estimated von Bertalanffy growth parameters of L∞ = 200.2 and K = 0.0045. These resulted in parameters a = 134.68 and b = −0.43 for determining the expected growth increment at a given size. The growth parameters from Micronesia (Bonine et al. 2008) predict a slower individual growth rate for S. serrata than those from a similar study in East Africa (Moksnes et al. 2015).
Current fishery selectivity
Using fishery selectivity estimated from kept crabs in the fishpond (Fig. 6) resulted in a slightly lower mean growth rate than in the unfished population (r = 0.825) but higher variance in growth rates than following the state size regulations, across harvest rates (Fig. 7). Model simulations indicated that the current fishing regime under which POH practitioners harvest S. serrata is less likely to cause a decline in population growth rate with increasing harvest pressure than strict size limits (Fig. 7, Fig. 8).
![]() |
Harvest scenario risk analysis
A summary of outcomes from the demographic analysis is presented in Table 2 and the distribution of population growth rate at each harvest size i is shown in Fig. 8. For the unfished population, estimates of the intrinsic growth rate r averaged 0.811 year−1 and the population doubling time averaged 1.63 years. The mean per-generation growth rate (R0) was estimated at 52.7 with a mean generation time of 4.75 years. The stable age distribution for the unfished population was heavily skewed right, with over 95% of the population falling into the first two size bins (11–65 mm). The bisection method indicated a minimum stationary harvest mortality of 46.6% across all size bins.
![]() |
Harvest mortality rates at or below 40% produced no simulations with r values less than 0 for all minimum sizes at capture, including 0 mm. In this simulation, even extreme fishing mortality rates (90%) did not result in population shrinkage when only legal sizes (>152 mm) were harvested. Harvest rates of 70% and above did not produce any simulations with declining populations so long as the harvest maintained the State’s minimum capture size (152 mm). Notably, a fishing mortality rate of 70% indicated a 100% risk of population decline (r < 0) when all sizes were selected for (i.e. when the size cutoff is zero; light blue curve); a minimum size at capture of 76 mm, which is the smallest empirically observed catch in this dataset, produced negative r values when mortality was 70%. Thus, in our simulation framework, tested harvest mortality rates above 47.2% (the stationary harvest rate) can present a risk of population shrinkage when selectivity includes crabs less than legal size.
In addition to the above investigation into the effect of varied harvest mortality on female S. serrata, we included a risk analysis. This approach cross-examined the minimum size at capture with the incremented harvest approach described above. The minimum sizes-at-capture explored were (1) 0 mm, all crabs able to be caught; (2) 76 mm, the smallest observed size of the crabs captured for this study; (3) 95.5 mm, the L50 estimate; and (4) 152 mm (6 in), the minimum size allowed per state regulations (HAR§13-84, HAR§13-95; DAR 2015). Results from the risk analysis are presented in Fig. 8, where we show the distribution of r values for 1000 simulated populations under each of the harvest levels.
Discussion
Interest in ILK systems is increasing as managers look for innovative and practical solutions to long-standing issues of sustainability (Johnson et al. 2016; Price and Toonen 2017; Winter et al. 2020). Hawaiian fishponds, or loko i‘a, are one of the most important technological advances made by kānaka maoli because these aquaculture systems provided food security for a large population living entirely off local resources (Kikuchi 1976). As such, they provide particularly compelling insight into transforming modern conservation biology efforts through Indigenous perspectives. Here we seek to develop a fisheries model that combines Indigenous and local ecological knowledge collected in He‘eia Fishpond with new information generated from modern intensive surveys and mark–recapture to explore the relative impacts of different harvest scenarios and predict future sustainable harvest limits of S. serrata. The mud crab S. serrata is a large-bodied and highly desirable fisheries crab throughout its range in the Indo-West Pacific. With its intentional introduction throughout the Pacific, Atlantic and Indian Oceans, it has successfully established populations in several locations (Lemaitre et al. 2013; Siple and Donahue 2013; McLay 2015; Roy and Nandi 2017). In some of these locations, it has had negative effects on native ecosystems (Reaser et al. 2007; Tavares and Mendonca 2011; Yeo et al. 2011). A well-managed fishery in Hawai‘i may be able to control this non-native population while generating income and food security for local communities that simultaneously fulfills the goals of active fishpond restoration leading to cultural and ecological preservation and sustainable food production.
Despite extensive data published on the global fishery of S. serrata (Robertson 1996; Pillans et al. 2005; Butcher et al. 2012), there are no fishery data published to date in Hawai‘i to the authors’ knowledge. Our study is the first to present fishery data on S. serrata in general and in a Hawaiian fishpond in particular. Our harvest scenarios risk analysis indicates that POH, the stewards of He‘eia Fishpond, have implemented a harvest strategy of S. serrata that is sustainable, and that some less selective strategies would likely result in population decline. Because POH are able to use their own harvest guidelines for species caught within the fishpond, they can adjust their harvest quickly based on the best available science, which makes this an ideal location for testing the effectiveness of different harvest control rules. POH management of S. serrata is effective not only for the limits on crab size based on the State regulation, but also for the implementation of a bag limit that the State does not have. POH also responds to observed drops in harvest by immediately closing the fishery until CPUE in traps increases again. Traditional ecological management is responsive to changes because it is based on near continuous observations of resources. If POH were interested in reducing population productivity, for example to allow recolonisation by native crab species, our results indicate that selecting smaller crabs for harvest and harvesting more frequently could reduce the population growth rate of S. serrata sufficiently for native crabs such as Hawaiian kuahonu (Portunus sanguinolentus Herbst 1783) to compete for space and resources. Native crabs are important because many species were historically sources of food. Some also had roles in Hawaiian folktales or cultural proverbs (Titcomb et al. 1979; Pukui 1983). Native crabs are more historically and culturally significant than the introduced species and their restoration is therefore part of cultural revitalisation (Titcomb et al. 1979).
Our study supports the POH management strategy of adjusting size limits depending on desired numbers of crabs per harvest. The harvest scenarios risk analysis indicates not only that current management is sustainable, but also that increased catches (either by harvesting more crabs or harvesting more frequently) is likely still sustainable, as long as catches are limited to large individuals (>152 mm), as is currently practiced. The risk analysis exercise also shows that harvesting smaller crabs must be accompanied by a lower harvest rate, especially for the smallest size class, or population decline is likely. Data on selectivity in the recreational fishery on O’ahu are not available, but data collection practices in He‘eia fishpond allowed for the estimation of current fishery selectivity. POH’s current selection strategy, based on the crabs kept from surveys, appears to have similar effects at different harvest rates but could potentially result in declines in the population growth rate, even at relatively low harvest rates. In the larval phases, these crabs face the same predation threats as do most invertebrate plankton (Webley and Connolly 2007; Christy 2011; Alberts-Hubatsch et al. 2016). As adults, however, risk of predation drops to be almost non-existent. These crabs, due to their size and ability to move between water and land, do not have natural predators in Hawai‘i, so management does not need to account for predation pressures besides that of humans (FAO 2001; Alberts-Hubatsch et al. 2016).
The data show that crabs removed from the fishpond during harvest are replaced within the next month (Figs 6, 7). The fishpond, although enclosed by a large, 2.5-km rock and coral wall, is not entirely closed off to input from the ocean. We did not capture larvae or other smaller, subadult stages of crabs in the fishpond, and therefore we cannot comment on whether these size classes may enter and leave. However, there is anecdotal evidence to support movement into and out of the pond as adults. There have been a few eyewitness accounts of large adults walking up and over the fishpond walls, but such observations are rare and typically involve a single individual. At least two members of the public reported on their capture of tagged S. serrata outside the fishpond. Based on minimum distance calculated from shore topography, one was found 8 km south 22 days after tagging and the other 4.8 km north after 60 days at liberty. This indicates that the fishpond population may not be an entirely closed population, and justifies our use of an open population model framework.
This study is an important first step towards a stronger understanding of S. serrata population dynamics in Hawai‘i. It has also identified some areas where improved information would result in more refined estimates of productivity and growth. We emphasise that the simulation carried out in this study is a harvest scenario risk analysis, intended to show the relative impact of different harvest rules on the population growth rate. It is not a formal fishery stock assessment, so the results from different size limits can only be interpreted relative to one another. Future work should include more accurate estimates of growth, maturity, and reproduction for S. serrata in the Hawaiian Islands. For example, specific life-history parameters such as individual growth and egg production may be dependent on environmental conditions, which would violate the static assumptions of the Lefkovitch approach used here. Likewise, Lefkovitch matrix models are usually modelling female growth and reproduction, whereas the fishery in the fishpond is almost exclusively for male crabs. Female values from the literature were included where possible, but interpretation of the results should recognise this limitation, because the population growth rate is likely larger in reality than reported here.
One important finding is that productivity in this population is highly sensitive to egg production, so better data on egg production by females in the Hawaiian Islands would result in a more accurate model of population growth. While egg production is often quantified in the laboratory, estimating other parameters may not require additional facilities. For example, an extended mark–recapture effort could produce highly-resolved individual growth estimates, which could be used to refine the growth matrix for Samoan crab in the wild. Such refined parameter values could be used to produce better estimates of stock productivity statewide. More mark–recapture data would also improve estimates of adult and subadult survival. To our knowledge, these would represent the first estimates of individual growth for S. serrata in Hawai‘i and could potentially be used in a Statewide population assessment. Ultimately, this would provide data to inform Statewide policy change and aid in efforts for population control across the archipelago.
The ecological and commercial value of the mud crab S. serrata throughout its natural and introduced range makes such a population assessment worthwhile. The combination of ILK with parameter estimates generated from mark–recapture surveys allows us to develop a versatile fisheries model to explore the relative impacts of different harvest scenarios on the crab population and to predict future sustainable harvest limits within this Native Hawaiian fishpond. The loko i‘a managed by POH makes an ideal model system to evaluate our codevelopment approach to sustainable fisheries management because the resource managers are also cultural practioners who enact harvest and are able to incorporate observed changes in size, abundance and harvest efficiency directly and immediately. By developing a management tool that allows the Indigenous resource managers to quickly and easily test a range of harvest scenarios, they can adaptively determine the management strategy best suited to specific goals. Codevelopment of this tool ensures open and transparent communication and provides information for changing strategies as management goals may change through time at the site. For example, current goals seek maximum sustainable harvest of introduced S. serrata for reliable food production, but once production of native species is stable and reliable, those goals are likely to change. At some point in the future, managers may seek to eradicate the introduced species to facilitate restoration of traditionally harvested native species. Such radically different management goals are best served by a versatile codeveloped tool, such as the approach we develop here, which incoporates ILK from the managers directly with the best available data and scientific approaches to allow them to decide the most effective harvest strategy to accomplish their changing goals for their place. The reciprocal collaboration required for this project to succeed provides an example of how conservation biology efforts may be transformed through Indigenous perspectives and codevelopment of new knowledge and contemporary management tools.
Conflicts of interest
The research was conducted in part or in whole under an award from the Office for Coastal Management, National Ocean Service, National Oceanic and Atmospheric Administration.
Acknowledgements
We acknowledge Paepae o He‘eia, the stewards of He‘eia Fishpond, for inviting us to share this Hawaiian space and follow along on crab harvests to collect data. This work was funded through a combination of grants including UH Mānoa Sea Grant # NA14OAR4170071; He‘eia NERR NA17NOS4200067, NA18NOS4200025, and NA19NOS4200078; the Carol Ann and Myron K. Hayashida Scholarship, and the Colonel Willys E. Lord, DVM & Sandina L. Lord Endowed Scholarship. Thank you to the ToBo Lab at HIMB, He‘eia NERR managers, Kalei Fujihara, Nakoa Goo, Claire Lager, Sherril Leon Soon, Kanoe‘ulalani Morishige, and Mindy Mizobe for lending hands in field work, and to Kamalani Hurley and Zacheri Hee for discussion and support. In honour of the next generation of Hawaiian stewards and scientists, thank you for your inspriation, Hali‘a and La‘akea Hurley Hee. SOEST contribution no. 10983, HIMB contribution no. 1806.
References
Aires-da-Silva, A. M., and Gallucci, V. F. (2007). Demographic and risk analyses applied to management and conservation of the blue shark (Prionace glauca) in the North Atlantic Ocean. Marine and Freshwater Research 58, 570–580.| Demographic and risk analyses applied to management and conservation of the blue shark (Prionace glauca) in the North Atlantic Ocean.Crossref | GoogleScholarGoogle Scholar |
Alberts-Hubatsch, H., Lee, S. Y., Meynecke, J-O., Diele, K., Nordhaus, I., and Wolff, M. (2016). Life-history, movement, and habitat use of Scylla serrata (Decapoda, Portunidae): current knowledge and future challenges. Hydrobiologia 76, 5–21.
| Life-history, movement, and habitat use of Scylla serrata (Decapoda, Portunidae): current knowledge and future challenges.Crossref | GoogleScholarGoogle Scholar |
Anderson, A. (2009). Epilogue: changing archaeological perspectives upon historical ecology in the Pacific Islands. Pacific Science 63, 747–757.
| Epilogue: changing archaeological perspectives upon historical ecology in the Pacific Islands.Crossref | GoogleScholarGoogle Scholar |
Anon. (1936). Samoan crab held menace; protection to be removed. Honolulu Star-Bulletin, 30 June 1936, p. 1.
Apple, R., and Kikuchi, W. K. (1975). Ancient Hawaii shore zone fishponds: an evaluation of survivors for historical preservation. Office of the State Director, National Park Service, United States Department of the Interior, Honolulu
Bonine, K. M., Bjorkstedt, E. P., Ewel, K. C., and Palik, M. (2008). Population characteristics of the mangrove crab Scylla serrata (Decapoda: Portunidae) in Kosrae, Federated States of Micronesia: effects of harvest and implications for management. Pacific Science 62, 1–19.
| Population characteristics of the mangrove crab Scylla serrata (Decapoda: Portunidae) in Kosrae, Federated States of Micronesia: effects of harvest and implications for management.Crossref | GoogleScholarGoogle Scholar |
Brock, V. (1960). The introduction of aquatic animals into Hawaiian water. International Revue Hydrobiologia 45, 463–480.
Butcher, P. A., Leland, J. C., Broadhurst, M. K., Paterson, B. D., and Mayer, D. G. (2012). Giant mud crab (Scylla serrata): relative efficiencies of common baited traps and impacts on discards. ICES Journal of Marine Science 69, 1511–1522.
| Giant mud crab (Scylla serrata): relative efficiencies of common baited traps and impacts on discards.Crossref | GoogleScholarGoogle Scholar |
Carpenter, B., Gelman, A., Hoffman, M. D., Lee, D., Goodrich, B., Betancourt, M., Brubaker, M., Guo, J., Li, P., and Riddell, A. (2017). Stan: a probabilistic programming language. Journal of Statistical Software 76, 1–32.
| Stan: a probabilistic programming language.Crossref | GoogleScholarGoogle Scholar |
Caswell, H. (1990). Matrix population models. Biometrics 46, 1238.
| Matrix population models.Crossref | GoogleScholarGoogle Scholar |
Christy, J. H. (2011). Timing of hatching and release of larvae by brachyuran crabs: patterns, adaptive significance and control. Integrative and Comparative Biology 51, 62–72.
| Timing of hatching and release of larvae by brachyuran crabs: patterns, adaptive significance and control.Crossref | GoogleScholarGoogle Scholar | 21593141PubMed |
Cobb, J. N. (1901). Commercial fisheries of the Hawaiian Islands. U.S. Bureau of Fisheries, Commissioner’s Report. pp. 433–512.
Cobb, J. N. (1905). The aquatic resources of the Hawaiian Islands. Part II. Section III. The commercial fisheries. Bulletin of the United States Fish Commission 23, 715–765.
Cormack, R. (1964). Estimates of survival from sighting of marked animals. Biometrika 51, 429–438.
| Estimates of survival from sighting of marked animals.Crossref | GoogleScholarGoogle Scholar |
Costa-Pierce, B. A. (1987). Aquaculture in ancient Hawaii. BioScience 37, 320–331.
| Aquaculture in ancient Hawaii.Crossref | GoogleScholarGoogle Scholar |
DeMartini, E. E., Kleiber, P., and DiNardo, G. T. (2002). Comprehensive (1986–2001) characterization of size at sexual maturity for Hawaiian spiny lobster (Panulirus marginatus) and slipper lobster (Scyllarides squammosus) in the northwestern Hawaiian Islands. NOAA-TM-NMFS-SWSFC-344. NOAA National Marine Fisheries Service, Honolulu, HI
DHM, Inc. (1989). Hawaiian fishpond study: islands of O’ahu, Moloka’i, and Hawai‘i. Prepared by DHM Planners, Inc. and Public Archaeology Section, Applied Research Group, Bernice Pauahi Bishop Museum, Honolulu, HI
Division of Aquatic Resources, DAR (2015). Fishing regulations: marine invertebrates. Department of Aquatic Resources, State of Hawai‘i. Available at http://dlnr.hawaii.gov/dar/fishing/fishing-regulations/marine-invertebrates/
Dye, T. (1994). Population trends in Hawai‘i before 1778. The Hawaiian Journal of History 28, 1–20.
Edmondson, C. H., and Wilson, I. H. (1940). The shellfish resources of Hawaii. Proceedings 6th Pacific Science Congress 3, 241–243.
Eldredge, L. G., and Carlton, J. T. (2002). Hawaiian marine bioinvasions: a preliminary assessment. Pacific Science 56, 211–212.
| Hawaiian marine bioinvasions: a preliminary assessment.Crossref | GoogleScholarGoogle Scholar |
FAO (2001). FAO yearbook fishery statistics: capture production. Vol. 88/1. (Food and Agriculture Organization of the United Nations, Rome, Italy.)
Farber, J. M. (1997). ‘Ancient Hawaiian Fishponds: Can Restoration Succeed on Moloka’i?’ (Neptune House Publications: Encinitas, CA.)
Forskål, P. (1775). Descriptiones Animalium, Avium, Amphibiorium, Piscium, Insectorum, Vermium; quae in Itinere Orientali Observavit Petrus Forskål. Post Mortem Autoris editit Carsten Niebuhr. Adjuncta est materia Medica Kahirina. Mölleri, Hafniae, 19 + xxxiv + 164 pp.
Gopurenko, D., Hughes, J. M., and Keenan, C. P. (1999). Mitochondrial DNA evidence for rapid colonisation of the Indo-West Pacific by the mudcrab Scylla serrata. Marine Biology 134, 227–233.
| Mitochondrial DNA evidence for rapid colonisation of the Indo-West Pacific by the mudcrab Scylla serrata.Crossref | GoogleScholarGoogle Scholar |
Herbst, J. F. W. (1783). Versuch einer Naturgeschichte der Krabben und Krebse nebst einer systematischen. Beschreibung ihrer verschiedenen Arten 3, 1–46 pls 51–54.
Hoenig, J. M. (1983). Empirical use of longevity data to estimate mortality-rates. Fishery Bulletin 82, 898–903.
Howitt, R. (2002). ‘Rethinking Resource Management: Justice, Sustainability and Indigenous Peoples.’ (Taylor and Francis Group: London.)
Johnson, J. T., Howitt, R., Cajete, G., Berkes, F., Louis, R. P., and Kliskey, A. (2016). Weaving Indigenous and sustainability sciences to diversify our methods. Sustainability Science 11, 25–32.
| Weaving Indigenous and sustainability sciences to diversify our methods.Crossref | GoogleScholarGoogle Scholar |
Jolly, G. (1965). Explicit estimates from capture–recapture data with both death and immigration stochastic model. Biometrika 52, 225–247.
| Explicit estimates from capture–recapture data with both death and immigration stochastic model.Crossref | GoogleScholarGoogle Scholar | 14341276PubMed |
Kamakau, S. M. (1976). ‘The Works of the People of Old = Nā Hana a ka Po‘e Kahiko.’ Translated from Hawaiian by Mary Kawena Puku‘i. (Bishop Museum Press: Honolulu, HI.)
Keala, G., Hollyer, J., and Castro, L. (2007). ‘Loko I‘a: a manual on Hawaiian fishpond restoration and management.’ (University of Hawai‘i Press: Honolulu, HI.)
Kikuchi, W. K. (1976). Prehistoric Hawaiian fishponds. Science 193, 295–299.
| Prehistoric Hawaiian fishponds.Crossref | GoogleScholarGoogle Scholar | 17745716PubMed |
Kirch, P. V. and Hunt, T. L. (Eds) (1997). ‘Historical Ecology in the Pacific Islands: Prehistoric Environmental and Landscape Change.’ (Yale University Press: New Haven, CT.)
Krebs, C. J. (1989). ‘Ecological Methodology.’ (Harper and Row: New York, NY.)
Kurashima, N., Jeremiah, J., Whitehead, A. N., Tulchin, J., Browning, M., and Duarte, T. (2018). ‘Āina Kaumaha: the maintenance of ancestral principles for 21st century indigenous resource management. Sustainability 10, 1–21.
| ‘Āina Kaumaha: the maintenance of ancestral principles for 21st century indigenous resource management.Crossref | GoogleScholarGoogle Scholar |
Kurashima, N., Fortini, L., and Ticktin, T. (2019). The potential of indigenous agricultural food production under climate change in Hawai‘i. Nature Sustainability 2, 191–199.
| The potential of indigenous agricultural food production under climate change in Hawai‘i.Crossref | GoogleScholarGoogle Scholar |
Lefkovitch, L. P. (1965). The study of population growth in organisms grouped by stages. Biometrics 21, 1–18.
| The study of population growth in organisms grouped by stages.Crossref | GoogleScholarGoogle Scholar |
Lemaitre, R., Campos, N. H., Viloria Maestre, E., and Windsor, A. M. (2013). Discovery of an alien crab, Scylla serrata (Forsskål, 1775) (Crustacea: Decapoda: Portunidae), from the Caribbean coast of Colombia. BioInvasions Records: International Journal of Field Research on Biological Invasions 2, 311–315.
| Discovery of an alien crab, Scylla serrata (Forsskål, 1775) (Crustacea: Decapoda: Portunidae), from the Caribbean coast of Colombia.Crossref | GoogleScholarGoogle Scholar |
MacCaughey, V. (1917). The mangrove in the Hawaiian islands. Hawaiian Forester and Agriculturalist 14, 361–365.
McLay, C. L. (2015). Invasive Brachyura. In ‘Treatise on Zoology – Anatomy, Taxonomy, Biology. The Crustacea’. (Eds P. Castro, P. J. F. Davie, D. Guinot, F. R. Schram, and J. C. Von Vaupel Klein.) Volume 9, Part C, pp. 821–846. (Brill.)
McPherson, R. (2002). Assessment of T bar anchor tags for marking the blue swimmer crab Portunus pelagicus (L.). Fisheries Research 54, 209–216.
| Assessment of T bar anchor tags for marking the blue swimmer crab Portunus pelagicus (L.).Crossref | GoogleScholarGoogle Scholar |
Meynecke, J. O., Mayze, J., and Alberts-Hubatsch, H. (2015). Performance and physiological responses of combined T-bar and PIT tagged giant mud crabs (Scylla serrata). Fisheries Research 170, 212–216.
| Performance and physiological responses of combined T-bar and PIT tagged giant mud crabs (Scylla serrata).Crossref | GoogleScholarGoogle Scholar |
Mirera, D. O., Ochiewo, J., Munyi, F., and Muriuki, T. (2013). Heredity or traditional knowledge: fishing tactics and dynamics of artisanal mangrove crab (Scylla serrata) fishery. Ocean and Coastal Management 84, 119–129.
| Heredity or traditional knowledge: fishing tactics and dynamics of artisanal mangrove crab (Scylla serrata) fishery.Crossref | GoogleScholarGoogle Scholar |
Moffitt, R. B., Johnson, J., and DiNardo, G. (2006). Spatiotemporal analysis of lobster trap catches: impacts of trap fishing on community structure. Atoll Research Bulletin 543, 217–236.
Moksnes, P.-O., Mirera, D. O., Björkvik, E., Hamad, M. I., Mahudi, H. M., Nyqvist, D., Jiddawi, N., and Troell, M. (2015). Stepwise function of natural growth for Scylla serrata in East Africa: a valuable tool for assessing growth of mud crabs in aquaculture. Aquaculture Research 46, 2938–2953.
| Stepwise function of natural growth for Scylla serrata in East Africa: a valuable tool for assessing growth of mud crabs in aquaculture.Crossref | GoogleScholarGoogle Scholar |
Moulton, M. P., and Pimm, S. L. (1986). Species introductions to Hawaii. In ‘Ecology of Biological Invasions of North America and Hawaii’. (Eds H. A. Mooney, and J. A. Drake.) pp. 231–249. (Springer: New York, NY.)
Mwaluma, J. (2002). Pen culture of the mud crab Scylla serrata in Mtwapa mangrove system, Kenya. Western Indian Ocean Journal of Marine Science 1, 127–133.
Nadon, M. O. (2017). Stock assessment of the coral reef fishes of Hawaii, 2016. NMFS-PIFSC-60. NOAA National Marine Fisheries Service, Honolulu, HI
O’Neill, M. F., Campbell, A. B., Brown, I. W., and Johnstone, R. (2010). Using catch rate data for simple cost-effective quota setting in the Australian spanner crab (Ranina ranina) fishery. ICES Journal of Marine Science 67, 1538–1552.
| Using catch rate data for simple cost-effective quota setting in the Australian spanner crab (Ranina ranina) fishery.Crossref | GoogleScholarGoogle Scholar |
Pascua, P., McMillen, H., Ticktin, T., Vaughan, M., and Winter, K. B. (2017). Beyond services: a process and framework to incorporate cultural, genealogical, place-based, and indigenous relationships in ecosystem service assessments. Ecosystem Services 26, 465–475
Pillans, S., Pillans, R. D., Johnstone, R. W., Kraft, P. G., Haywood, M. D. E., and Possingham, H. P. (2005). Marine Ecology Progress Series 295, 201–213.
| Crossref | GoogleScholarGoogle Scholar |
Prasad, P. N., and Neelakantan, B. (1989). Maturity and breeding of the mud crab, Scylla serrata (Forskal) (Decapoda: Brachyura: Portunidae). Proceedings of the Indian Academy of Sciences (Animal Sciences) 98, 341–349.
| Maturity and breeding of the mud crab, Scylla serrata (Forskal) (Decapoda: Brachyura: Portunidae).Crossref | GoogleScholarGoogle Scholar |
Price, M. R., and Toonen, R. J. (2017). Scaling up restoration efforts in the Pacific Islands: a call for clear management objectives, targeted research to minimize uncertainty, and innovative solutions to a wicked problem. Pacific Science 71, 391–399.
| Scaling up restoration efforts in the Pacific Islands: a call for clear management objectives, targeted research to minimize uncertainty, and innovative solutions to a wicked problem.Crossref | GoogleScholarGoogle Scholar |
Pukui, M. K. (1983). ‘Ōlelo No‘eau : Hawaiian Proverbs & Poetical Sayings.’ (Bishop Museum Press: Honolulu, HI.)
Quinitio, E. T., Parado-Estepa, F. D., Millamena, O. M., Rodriguez, E., and Borlongan, E. (2001). Seed production of mud crab Scylla serrata juveniles. Asian Fisheries Science 14, 161–174.
R Core Team. (2019). R: a language and environment for statistical computing. R Foundation for Statistical Computing, Vienna, Austria. Available at https://www.R-project.org/
Reaser, J. K., Meyerson, L. A., Cronk, Q., De Poorter, M. A. J., Eldrege, L. G., Green, E., Kairo, M., Latasi, P., Mack, R. N., Mauremootoo, J., and O’Dowd, D. (2007). Ecological and socioeconomic impacts of invasive alien species in island ecosystems. Environmental Conservation 34, 98–111.
| Ecological and socioeconomic impacts of invasive alien species in island ecosystems.Crossref | GoogleScholarGoogle Scholar |
Robertson, W. D. (1996). Abundance, population structure and size at maturity of Scylla serrata (Forskal) (Decapoda: Portunidae) in Eastern Cape estuaries, South Africa. South African Journal of Zoology 31, 177–185.
Roy, M. K. D., and Nandi, N. C. (2017). Marine invasive alien crustaceans of India. Journal of Aquaculture and Marine Biology 5, 00115.
Sarower, M. G., Aktar, N., Mostafa, M., Sabbir, W., and Islam, M. S. (2012). Some aspects of captive breeding biology of mud crab, Scylla serrata in Bangladesh. Journal of Innovation and Development Strategy 6, 1–6.
Seber, G. (1965). A note on multiple-recapture census. Biometrika 52, 249–259.
| A note on multiple-recapture census.Crossref | GoogleScholarGoogle Scholar | 14341277PubMed |
Shelley, C. and Lovatelli, A. (2011). Part 1. Biology. In ‘Mud Crab Aquaculture: A Practical Manual’. FAO Fisheries and Aquaculture Technical Paper. (Food and Agriculture Organization of the United Nations: Rome, Italy.)
Siddeek, M. S. M., Zheng, J., Punt, A. E., and Vanek, V. (2016). Estimation of size–transition matrices with and without molt probability for Alaska golden king crab using tag–recapture data. Fisheries Research 180, 161–168.
| Estimation of size–transition matrices with and without molt probability for Alaska golden king crab using tag–recapture data.Crossref | GoogleScholarGoogle Scholar |
Siple, M. C., and Donahue, M. J. (2013). Invasive mangrove removal and recovery: food web effects across a chronosequence. Journal of Experimental Marine Biology and Ecology 448, 128–135.
| Invasive mangrove removal and recovery: food web effects across a chronosequence.Crossref | GoogleScholarGoogle Scholar |
Stan Development Team (2018). RStan: the R interface to Stan. R package version. Available at http://mc-stan.org
Stannard, D. E. (1989). ‘Before the Horror: The Population of Hawai‘i on the Eve of Western contact.’ (University of Hawaii Press: Honolulu, HI.)
Tavares, M., and Mendonca, J. B. (2011). The occurrence of the Indo-Pacific swimming crab Scylla serrata (Forskål, 1775) in the southwestern Atlantic (Crustacea: Brachyura: Portunidae). Aquatic Invasions 6, S49–S51.
| The occurrence of the Indo-Pacific swimming crab Scylla serrata (Forskål, 1775) in the southwestern Atlantic (Crustacea: Brachyura: Portunidae).Crossref | GoogleScholarGoogle Scholar |
Titcomb, M., Fellows, D. B., Pukui, M. K., and Devaney, D. M. (1979). ‘Native Use of Marine Invertebrates in old Hawaii.’ (University of Hawai‘i Press: Honolulu, HI.)
Triño, A. T., and Rodriguez, E. M. (2002). Pen culture of mud crab Scylla serrata in tidal flats reforested with mangrove trees. Aquaculture 211, 125–134.
| Pen culture of mud crab Scylla serrata in tidal flats reforested with mangrove trees.Crossref | GoogleScholarGoogle Scholar |
USA DOC (2012). Increased food security and food self-sufficiency strategy. Prepared by Office of Planning, Department of Business Economic Development and Tourism, Department of Commerce, and The Department of Agriculture, State of Hawai‘i, Honoulu, HI
Webley, J. A. C., and Connolly, R. M. (2007). Vertical movement of mud crab megalopae (Scylla serrata) in response to light: doing it differently down under. Journal of Experimental Marine Biology and Ecology 341, 196–203.
| Vertical movement of mud crab megalopae (Scylla serrata) in response to light: doing it differently down under.Crossref | GoogleScholarGoogle Scholar |
Williams, M. J., and Primavera, J. H. (2001). Choosing tropical portunid species for culture, domestication and stock enhancement in the Indo-Pacific. Asian Fisheries Science 14, 121–142.
Winter, K. B., Beamer, K., Vaughan, M. B., Friedlander, A. M., Kido, M. H., Whitehead, A. N., Akutagawa, M. K. H., Kurashima, N., Lucas, M. P., and Nyberg, B. (2018). The moku system: managing biocultural resources for abundance within social–ecological regions in Hawai‘i. Sustainability 10, 3294.
| The moku system: managing biocultural resources for abundance within social–ecological regions in Hawai‘i.Crossref | GoogleScholarGoogle Scholar |
Winter, K. B., Alegado, R. A., Bowen, B. W., Bremer, L. L., Coffman, M., Cypher, M., Deenik, J., Donahue, M. J., Falinski, K., Frank, K., Franklin, E. C., Hewitt, A., Hintzen, K., Kaluhiwa, R., Kawelo, H., Kekuewa, K., Kotubetey, K., Kukea-Shultz, K., Kurashima, N., Lee, T., Leong, J-A., Lincoln, N. K., Madden, E., McManus, M. A., Neilson, B., Nelson, C. E., Okano, R., Olegario, A., Oleson, K., Pascua, P., Price, M., Reppun, F., Rii, Y., Rivera, M., Rodgers, K., Sabine, C., Smith, C., Thomas, B., Ticktin, T., and Toonen, R. J. (2020). Ecomimicry in indigenous resource management: optimizing ecosystem services to achieve resource abundance with examples from Hawaii. Ecology and Society 25, 26.
| Ecomimicry in indigenous resource management: optimizing ecosystem services to achieve resource abundance with examples from Hawaii.Crossref | GoogleScholarGoogle Scholar |
Yeo, D. C., Carlton, J. T., Teo, S. L. and Ng, P. K. (2011). An incoming flood on a cryptic stage: understanding alien crustacean invasions in Southeast Asia. In ‘In the Wrong Place – Alien Marine Crustaceans: Distribution, Biology and Impacts’. (Eds B. Galil, P. F. Clark, and J. T. Carlton.) pp. 403–417. (Springer: Dordrecht, Netherlands.)