Carp carcass decomposition and water quality: implications for the release of CyHV-3 as a biocontrol agent for common carp in Australia
Richard Walsh A B , Tyler N. Dornan
A
B
C
D
E
Abstract
In Australia’s Murray–Darling Basin, the invasive common carp (Cyprinus carpio) poses a significant ecological threat. Efforts to manage their population include the potential use of cyprinid herpesvirus (CyHV-3) as a biological control agent. The environmental benefits of carp elimination could be substantial in the long term; our knowledge of the immediate ecological consequences resulting from large-scale fish die-offs remains limited.
This study sought to investigate the effect of a large-scale fish-mortality event on water quality.
Experiments of an increasing scale (bucket, mesocosm and whole wetland) were conducted to assess how decomposing carp carcasses alter dissolved oxygen and nutrient concentrations in water.
In mesocosms, dead carp decayed more rapidly at 18 than 12°C, yielding oxygen demands of 1.022 ± 0.029 and 0.496 ± 0.239 mg kg−1 min−1 respectively. This carp decay released phosphorus, yielding 2121.1 ± 140.4 mg kg−1. In the wetland experiment, carp addition of 2400 kg ha−1 resulted in anoxic conditions over the following 2 weeks. The release of dissolved organic carbon and lipids led to a peak biological oxygen demand of 95.3 mg L−1.
Carp decomposition considerably contributes to biological oxygen demand and algal growth through nutrient enrichment and is strongly influenced by carp density.
The finding highlights key factors to consider before using biocontrol agents causing mass carp mortality, including hypoxia, anoxia and increased risk of harmful algal blooms.
Keywords: biogeochemistry, CyHV-3, Cyprinus carpio, ecology, eutrophication, fish, freshwater, introduced species, limnology, Murray–Darling Basin.
Introduction
The common carp (Cyprinus carpio; hereafter carp) is a large-bodied cyprinid that is invasive in Australia, becoming a particularly widespread and damaging pest in the Murray–Darling Basin (MDB). The expansion and establishment of substantial carp populations within the MDB waterways have been facilitated by several factors, including altered river management, reproductive advantages and carp’s tolerance to suboptimal water conditions (Harris and Gehrke 1997; Koehn 2004; Stuart et al. 2021). This has led to degraded water quality and adverse effects on invertebrate, zooplankton and macrophyte communities (King et al. 1997; Koehn et al. 2000; Vilizzi et al. 2014; Marshall et al. 2019). Increasing carp populations along with alterations to water regimes (which are likely to have played a larger role) have been suggested as major drivers of native fish population declines (Clunie and Koehn 1997; Kopf et al. 2019a; Stocks et al. 2021). In terms of biomass, carp has become the predominant fish species across numerous freshwater ecosystems in Australia and is widely distributed across the entire MDB (Stuart et al. 2019). Without intervention, carp populations are expected to expand toward the upper sectors of the MDB, the remaining south-eastern river systems along the coast and across river systems in Tasmania (Koehn 2004; Koehn et al. 2018). Carp would, consequently, dominate a large part of the Australian freshwater environment.
Cyprinid herpesvirus 3 (CyHV-3) is under evaluation in Australia as a potential biological control agent for managing carp population sizes (McColl et al. 2017; Becker et al. 2019). CyHV-3 induces rapid and significant carp mortality and morbidity between the effective temperature window of 18–28°C (Rakus et al. 2013), with up to 75% death recorded in experimental aquarium treatments (Sunarto et al. 2014). At temperatures below the effective temperature range (e.g. 12°C), the virus can persist in infected carp and reactivate when temperatures rise, leading to mortality (Sunarto et al. 2014). CyHV-3 outbreaks have been documented in a number of countries, with differences in carp populations, strains and habitat all contributing to varying degrees of carp mortality and population suppression (Gotesman et al. 2013; Kopf et al. 2019b; Samsing et al. 2021). There are concerns that following extensive carp mortality, decaying carp biomass has the potential to considerably reduce dissolved oxygen (DO) concentrations in the water, resulting in hypoxic or anoxic conditions (Kopf et al. 2019b). Elevated microbial activity linked to the decomposition of organic matter is reported to be a key contributor to oxygen-depleted water in the MDB (King et al. 2012). Additionally, water temperature also significantly affects DO reduction in the water column by affecting the development, growth and respiration of microbial community, as well as influencing aquatic organisms’ metabolic requirements (Howitt et al. 2007). Elevated temperatures worsen the effects of oxygen depletion on aquatic organisms by increasing their metabolic oxygen demand and reducing oxygen solubility in water (Lewis 1970), thus affecting other aquatic organisms, including native fish populations. Extensive research has been undertaken on anoxia induced by vegetation (e.g. Gehrke et al. 1993; McMaster and Bond 2008), including studies investigating how temperature influences this process (Whitworth et al. 2014). However, the potential for deoxygenation from decomposing carp carcases and the role of temperature in this process remain largely unexplored in Australian waters. This is particularly true for carp decomposition under cold-water scenarios (e.g. 12°C), which may occur when dead carp sink to cooler waters in thermally stratified waterbodies. Recent mesocosm experiments have demonstrated that the decomposition of carp at a density of 500 kg ha−1 is sufficient for the onset of anoxia, with nutrient release exhibiting a strong linear relationship with carp biomass (Pera et al. 2022). Given the potential risk that mass carp mortality has on water quality (Lighten and van Oosterhout 2017), further investigations into the impact of temperature on carp decomposition rate and the resulting nutrient dynamics within sediment, such as those reported here, will provide complementary information to assist in carp management.
Australian native fish and other aquatic organisms may experience stress when DO concentrations fall below 4 mg L−1 and mortality may occur at DO of <2 mg L−1 (Gehrke 1988). For instance, Australian smelt (Retropinna semoni) and flat-headed galaxias (Galaxias rostratus) responded quickly to low DO, with 10% of individuals exhibiting aquatic surface respiration at 2.55 and 2.21 mg O2 L−1 respectively (McNeil and Closs 2007). More recent research suggests that certain vulnerable species may have even lower tolerances than these values (Gilmore et al. 2015). Dissolved oxygen thresholds estimated for four predatory fish species found in Australian lowland rivers are presented in Table 1 (Small et al. 2014).
Fish species | DO threshold estimate (mg L−1) | s.e. | |
---|---|---|---|
Murray cod (Maccullochella peelii) | 4.80 | 0.74 | |
Golden perch (Macquaria ambigua) | 1.72 | 0.63 | |
Silver perch (Bidyanus bidyanus) | 2.65 | 0.60 | |
Eel-tailed catfish (Tandanus tandanus) | 1.85 | 0.53 |
Native fish and exotic species alike may experience fatal consequences when exposed to oxygen concentrations below critical thresholds, even for brief durations, despite most fish having developed mechanisms to handle oxygen fluctuations. Research conducted in New Zealand has shown that exposing New Zealand smelt (Retropinna retropinna) and rainbow trout (Oncorhynchus mykiss) populations to DO concentrations of 1 mg L−1 for under 1 h can result in a 50% mortality rate (Dean and Richardson 1999). Murray crayfish (Euastacus armatus) exhibits heightened vulnerability to oxygen-depleted environments because of its limited mobility. Murray crayfish tends to escape from waters with DO concentrations approaching 2 mg L−1, because extended exposure to hypoxic conditions significantly diminishes their numbers owing to heightened predation and risk of desiccation (McKinnon 1995; King et al. 2012). The species’ slow growth rate, delayed sexual maturity and poor dispersal capabilities result in prolonged recovery periods following mass die-offs, rendering Murray crayfish populations susceptible to localised extinctions (McCarthy et al. 2014). Additionally, some freshwater shrimp (Macrobrachium spp.) and yabbies (Cherax spp.) have demonstrated high mortality rates when exposed to DO concentrations of 2 mg L−1 (King et al. 2012). Given their crucial role in the Murray River food web, population declines in these species may lead to direct and indirect effects on the populations of Australian fish and invertebrates (Ebner 2006; Gilligan et al. 2007).
A significant issue in carp biocontrol is the scale of nutrient enrichment from carp decomposition, the subsequent nutrient pathways and the possibility of resultant harmful algal blooms. Nutrient enrichment is a primary factor driving harmful algal blooms (Brookes et al. 2000), which directly affect native fish and other aquatic organisms through mechanisms such as increased water toxicity and modifications to the food web (Paerl et al. 2001). Genera of cyanobacteria such as Dolicospermum (Anabaena), Aphanizomenon and Microcystis are highly productive in warm, turbid waters and frequently form harmful cyanobacterial blooms throughout the MDB (Baker et al. 2000). These cyanobacteria produce toxins that pose significant risks to aquatic and terrestrial organisms, including humans and livestock (Chorus and Bartram 1999; World Health Organization 2011). Additionally, because of their low nutritional content, cyanobacteria are deemed a substandard food source for certain zooplankton (Gulati and Demott 1997, Müller-Navarra et al. 2000). Specifically, cyanobacteria lack some essential fatty acids and sterols required for zooplankton growth and reproduction (Ger et al. 2016), whereas their structure makes them difficult to consume for most grazers (Ghadouani et al. 2004). Although cyanobacterial carbon can be trophically upgraded through heterotrophic microbes (Luo et al. 2015), compared with more nutritionally rich algae, cyanobacteria are a suboptimal pathway for carbon flow to grazers and the broader aquatic food webs (Lampert 1987; Brookes et al. 2005). Although the relationship between nutrient enrichment and large-scale fish mortality has been investigated (e.g. Stevenson and Childers 2004; Schoenebeck et al. 2012; Killberg-Thoreson et al. 2014), there is a clear lack of studies exploring nutrient enrichment associated with large-scale carp mortality in Australian freshwater systems.
The Australian National Carp Control Plan was tasked with evaluating the efficacy of CyHV-3 as a potential biocontrol agent for Australian carp populations. Several reviews have been undertaken, outlining the potential risks, benefits and outstanding research questions (e.g. Boutier et al. 2019, McColl and Sunarto 2020). Some key factors that need to be understood in association with mass carp mortality are the epidemiology of CyHV-3, carp abundance, impacts on local fish, the potential for cross-infectivity and impacts on water quality. This study examines how the decomposition of carp carcasses influences DO concentration and nutrient loading in the water column. This involved a sequence of field and laboratory experiments, culminating in a large-scale wetland experiment in the lower Murray River, South Australia. It is hypothesised that carp decomposition will rapidly enrich the surrounding water with nutrients until reaching a plateau, with the rate of nutrient release increasing with temperature. Consequently, microorganisms will quickly utilise these nutrients, raising the biological oxygen demand and resulting in prolonged hypoxia and anoxia. Whereas some of the potential water-quality implications of mass carp die-off have been explored (Boutier et al. 2019; Kopf et al. 2019b; Pera et al. 2022), this research provides unique insights by performing a large-scale experiment in a natural wetland with high densities of carp.
Materials and methods
Three experiments of an increasing scale (bucket, mesocosm and wetland experiments) were conducted to assess how decomposing carp carcasses alter DO and nutrient concentrations in closed waterbodies. The first experiment was a small-scale bucket experiment conducted under controlled laboratory conditions to measure phosphorus and chlorophyll-a concentrations during carp decomposition. The second experiment involved introducing dead carp to mesocosms with water that did not contain sediment, phytoplankton or any other features that affect oxygen dynamics. In a third experiment, a mass of dead carp representative of the high densities observed from wild carp surveys (Hicks et al. 2005; Stuart et al. 2021) was introduced into a wetland recently disconnected from the main channel of the Murray River.
Work was conducted under ethics number 0000021588.
Bucket experiments
A laboratory experiment was conducted to measure the release of phosphorus (P) from carp carcasses into surrounding water. The study utilised 15-L buckets filled with reverse osmosis (RO) water, with five replicates each for the carp treatment and control. Single carp specimens (~2.5 kg) were added to treatment buckets, whereas control buckets remained empty. The carp were obtained by electro-fishing from the River Torrens on 9 June 2016 by SARDI Aquatic Sciences.
The experiment was conducted in a temperature-controlled environment maintained at 20°C for 45 days. Sampling took place on Days 0, 1, 2, 4, 7, 14, 23, 30, 37 and 45. Before sample collection, water in each bucket was thoroughly mixed to ensure homogeneity. Filtered reactive phosphorus (FRP) and total phosphorus (TP) were measured using 0.45-μm syringe filters and 50-mL syringes respectively. Samples were stored in 50-mL cryogenic vials and immediately frozen.
FRP and TP concentrations were determined using a Biochrom Libra S22 UV/Vis Spectrophotometer, following the ascorbic acid colorimetric method described in Water Analysis: Some Revised Methods for Limnologists (Mackereth et al. 1978). Concentrations were measured in milligrams per litre, with replicate samples being analysed on Days 30 and 45. For each analysis, standards with known P concentrations were prepared to develop a model correlating P concentration with absorbance.
To estimate the potential maximum chlorophyll-a concentration resulting from P released by carp, the study employed the relationship between maximum TP (mg L−1) and chlorophyll-a concentration (μg L−1) established for Myponga Reservoir, South Australia (Linden et al. 2004), as follows:
The biomass estimates utilised in this study were derived from a comprehensive review of carp biomass data in Australia. These estimates were based on a calibration experiment conducted on the Bogan River, which established a catch efficiency of 21% (Gehrke et al. 1995). This catch efficiency was subsequently applied to calculate total biomass at various sites, assuming similar conditions, as follows:
‘Low’: 265 kg ha−1 in MDB regulated lowland (altitude <300 m) (Driver et al. 2005)
‘Moderate’: 696 kg ha−1 in MDB reach (altitude 300–700 m) (Driver et al. 2005)
‘High’: 3144 kg ha−1 in Lachlan River reach (altitude 460 m) (Driver et al. 1997)
Mesocosm experiments
Eleven large plastic mesocosms, each holding 713 L of tap water, were set aside for 5 days to allow the chlorine to dissipate. Chlorine is routinely added to potable tap water as a disinfectant and was removed from this experiment so as not to affect microbial growth. Subsequently, a 1% inoculum (7 L) of water from Lake Torrens, South Australia, was added, bringing the total volume to 720 L.
To establish the cold treatment, three mesocosms received one carp carcass each, weighing 3.68, 3.89 and 2.56 kg respectively, whereas three mesocosms containing only water were left as controls. The six cold mesocosms were left outside at an ambient temperature of 12°C (12.27 ± 0.55°C), representing a low-risk carp-mortality scenario and a baseline for the warm-water mesocosms. Similarly, to establish the warm treatment, a single carp carcass was added to three additional mesocosms, weighing 2.89, 2.07 and 3.29 kg respectively, whereas two mesocosms containing only water were left as controls. The five mesocosms were placed in a temperature-controlled room set to 20°C, although the actual temperature of the water measured 18°C (18.07 ± 0.16°C).
Carp for the cold-water mesocosm were obtained from commercial net fishers in Lake Alexandrina, South Australia, whereas carp used in warm-water mesocosms were obtained from a Williams’ Cage at Lock 1, Blanchetown, South Australia. DO concentrations and temperature were measured every 5 min with ‘D-Opto’ Optical DO sensors (Zebra Technologies, Nelson, New Zealand), calibrated according to manufacturer’s recommendations.
Wetland experiment
Little Duck Lagoon (LDL) is a 2.5-ha, 10-ML managed wetland ~4 km south of Berri, South Australia. LDL is a component of the Causeway Wetland Complex within the larger Gurra Gurra Wetland Complex. LDL was chosen for the carp wetland experiment because of its suitable dimensions and isolation from populated areas. Most critically, LDL was scheduled for a managed drawdown phase, achieved by closing of the sluice gate between LDL and Gurra Gurra Creek, timed to align with the carp experiment. The wetland was filled with 10 ML of river water before the sluice gate was closed prior to carp addition. The wetland was allowed to completely dry out after the experiment to minimise post-experiment environmental impacts, such as the discharge of poor-quality water into the main Murray River system.
A total of 6 Mg of dead carp, sourced from the Williams’ Cage at Lock 1, South Australia, were frozen for storage and, subsequently, added to the south-eastern end of LDL by using a front-end loader. The resulting carp biomass in LDL was 2400 kg ha−1. Because of access limitations, carp carcasses were unevenly distributed, creating a density gradient across the wetland. Six sampling sites were established along the wetland length. DO concentrations were continuously monitored at Sites 1–5 using ‘D-Opto’ Optical DO sensors, with mid-experiment recalibration. Water samples were collected from all sites before carp addition (Day 0) and on Days 2, 4, 7, 14, 21, 28 and 42. Samples were ice-packed for laboratory transport, where they were processed, filtered or frozen as required. Total nutrients, dissolved nutrients and dissolved organic carbon analyses were conducted by Environmental Analysis Laboratory (EAL, Lismore, NSW, Australia). Biological oxygen demand (BOD) was measured using the standard 5-day method outlined in Standard Methods for the Examination of Water and Wastewater (Water Environmental Federation and American Public Health Association 2005). Chlorophyll-a concentration was analysed at Sites 1 and 5 following the spectrophotometric determination method from the same publication. Water-column lipids were analysed using methods described by Bligh and Dyer (1959).
Linear regression analyses were conducted to estimate potential nutrient concentrations in the wetland when scaled to lower carp densities. Mean maximum nutrient concentrations detected before (Day 0) and after the additions of carp at a density of 2400 kg ha−1 were used as data points to interpolate potential nutrient concentrations at carp densities of 1000, 500 and 150 kg ha−1. Nutrients and chlorophyll a were assumed to have a linear relationship with carp biomass, demonstrated in mesocosm experiments by Pera et al. (2022). Standard deviations for each nutrient interpolation were assumed to be equal to that observed at a carp density of 2400 kg ha−1.
Results
Bucket experiments: phosphorus and chlorophyll-a concentrations
The release of TP and FRP from carp carcasses exhibited an increasing trend over time at an ambient temperature of 20°C (Fig. 1). TP flux into the surrounding water began immediately and rose rapidly, reaching a mean (±s.d.) of 1749.8 ± 169.4 mg kg−1 by Day 14. Subsequently, TP concentrations stabilised, suggesting complete decomposition of carp tissue and nutrient release into the water column.
The filterable reactive phosphorus (FRP) and total phosphorus (TP) concentrations fluxing from carp carcasses in milligrams per kilogram of carp incubated in buckets. The FRP and TP data are slightly offset to allow clearer visualisation. Error bars represent standard deviation.
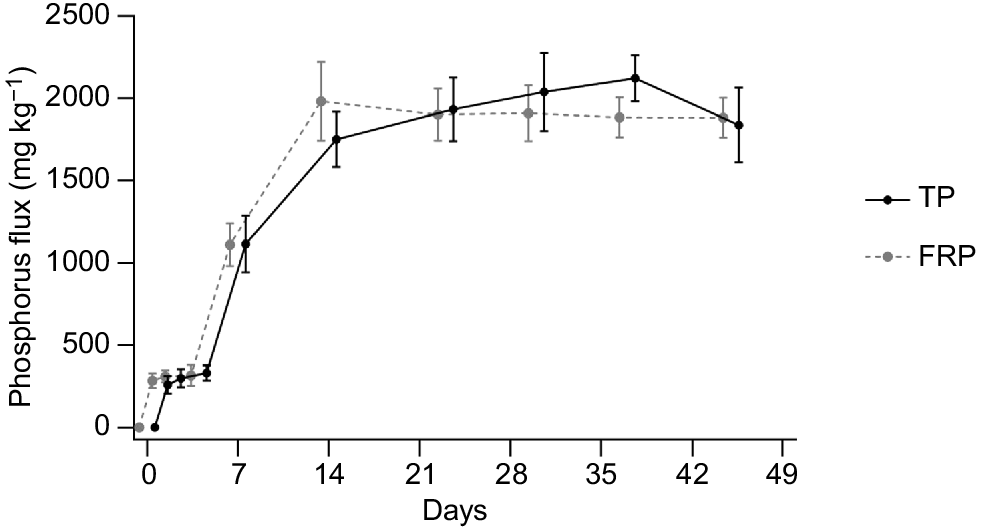
The peak mean TP leached from carp carcasses was observed on Day 37, measuring 2121.1 ± 140.4 mg kg−1. This maximum TP value was utilised to determine potential TP load and chlorophyll-a concentrations for various wetland inundation depths at different biomass levels. For biomasses of 3144, 696 and 265 kg ha−1, the projected TP loading from carp carcasses was calculated to be 6.67, 1.48 and 0.56 kg ha−1 respectively (Table 2). At the highest biomass (3144 kg ha−1), carp carcasses could potentially result in TP concentrations of 0.667, 0.333 and 0.222 mg L−1 for water depths of 1, 2 and 3 m respectively. These TP concentrations may lead to corresponding chlorophyll-a concentrations of 0.131, 0.066 and 0.045 mg L−1.
Biomass (kg ha−1) | TP load (kg ha−1) | TP load (mg m−2) | Assumed depth (m) | TP load (mg L−1) | Chl-a (mg L−1) | |
---|---|---|---|---|---|---|
265 | 0.56 | 56.21 | 1 | 0.056 | 0.013 | |
2 | 0.028 | 0.007 | ||||
3 | 0.019 | 0.005 | ||||
696 | 1.48 | 147.63 | 1 | 0.148 | 0.030 | |
2 | 0.074 | 0.016 | ||||
3 | 0.049 | 0.011 | ||||
3144 | 6.67 | 666.87 | 1 | 0.667 | 0.131 | |
2 | 0.333 | 0.066 | ||||
3 | 0.222 | 0.045 |
TP load at each biomass was calculated from TP leached during bucket experiments (2121.1 mg kg−1). TP load at each biomass was subsequently used to calculate maximum Chl-a by using Eqn 1.
Mesocosm experiments: the effect of temperature on BOD
Comparison between warm (18°C) and cold (12°C) mesocosms containing carp carcasses indicated that the rate of oxygen demand, measured as BOD, was higher with increased temperature, averaging 1.022 ± 0.029 and 0.496 ± 0.239 mg kg−1 min−1 respectively. Consequently, anoxia (DO < 0.2 mg L−1) occurred more than twice as quickly in the warm mesocosms than in the cold mesocosms (Fig. 2), averaging 59.45 (±6.1) and 127.62 (±3.4) h respectively.
Wetland experiment
The instances and severity of hypoxia and anoxia (<0.2 mg L−1) decreased with distance from where the dead carp were initially added. After carp carcasses were added to the wetland, Site 1, the closest to the carp carcasses, experienced anoxic conditions during the first night, whereas all other sites experienced DO concentrations of ~2 mg L−1 during the same time (Fig. 3, Supplementary Fig. S1). At Site 1, two periods of prolonged anoxia occurred, beginning on Days 2 and 28 and lasting for 10.5 and 4.1 days respectively. Similarly, Site 2 experienced two prolonged periods of anoxia beginning on Days 30 and 36, lasting 10.4 and 5.7 days respectively. Prolonged periods of anoxia did not occur at sites farther from the carp carcasses (Sites 3–5), although nocturnal anoxia was a common for all sites.
Dissolved oxygen concentrations (black line) and water temperature (red line) for Sites 1 and 5 for the duration of the wetland experiment. To demonstrate the variability in DO concentration, only data from Site 1 and Site 5 (closest and farthest from the carp) are visualised. A complete summary of wetland DO is presented in Fig. S1.
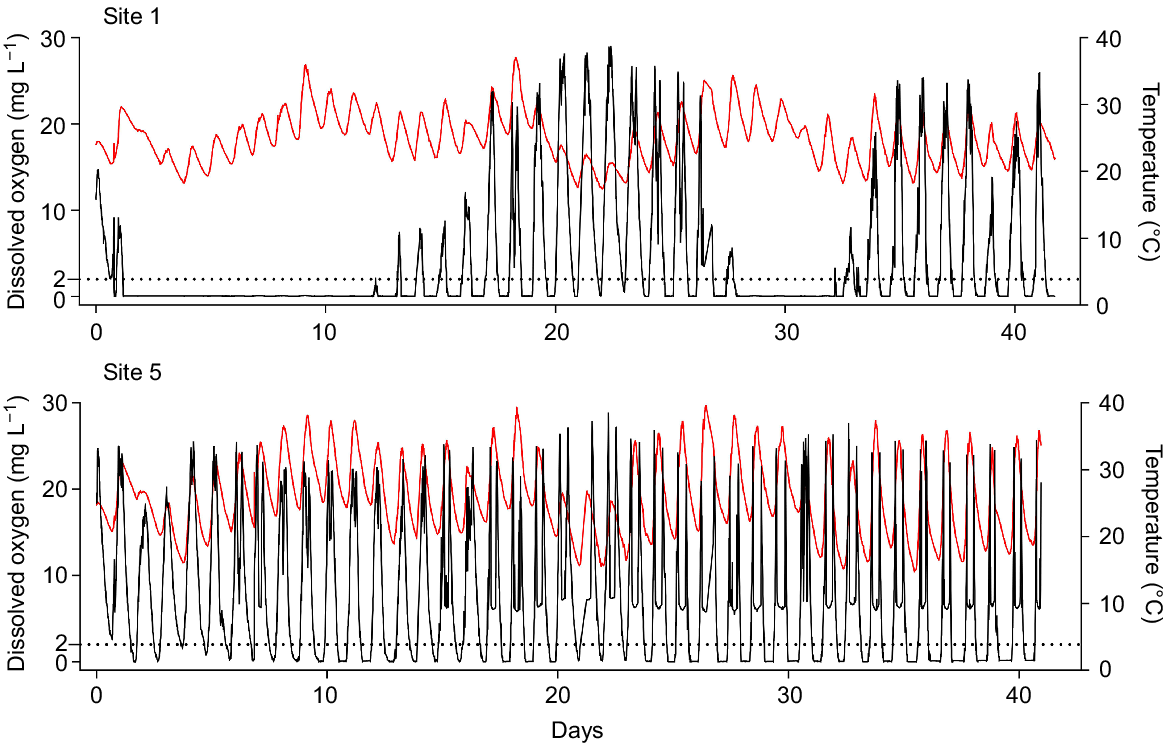
A rapid spike in total and dissolved nutrients was evident within the first 2 weeks for Sites 1, 2 and 3 (Fig. 4, Supplementary Table S1), exceeding relevant freshwater guidelines values outlined in the Table 3.
Nutrient concentrations for the duration of the wetland experiment. DOC, dissolved organic carbon; BOD, biological oxygen demand; and Chl-a, chlorophyll-a. To demonstrate the variability in nutrient concentration, only data from Site 1 and Site 5 (closest and farthest from the carp) are visualised. A complete summary of wetland nutrients is presented in Table S1.
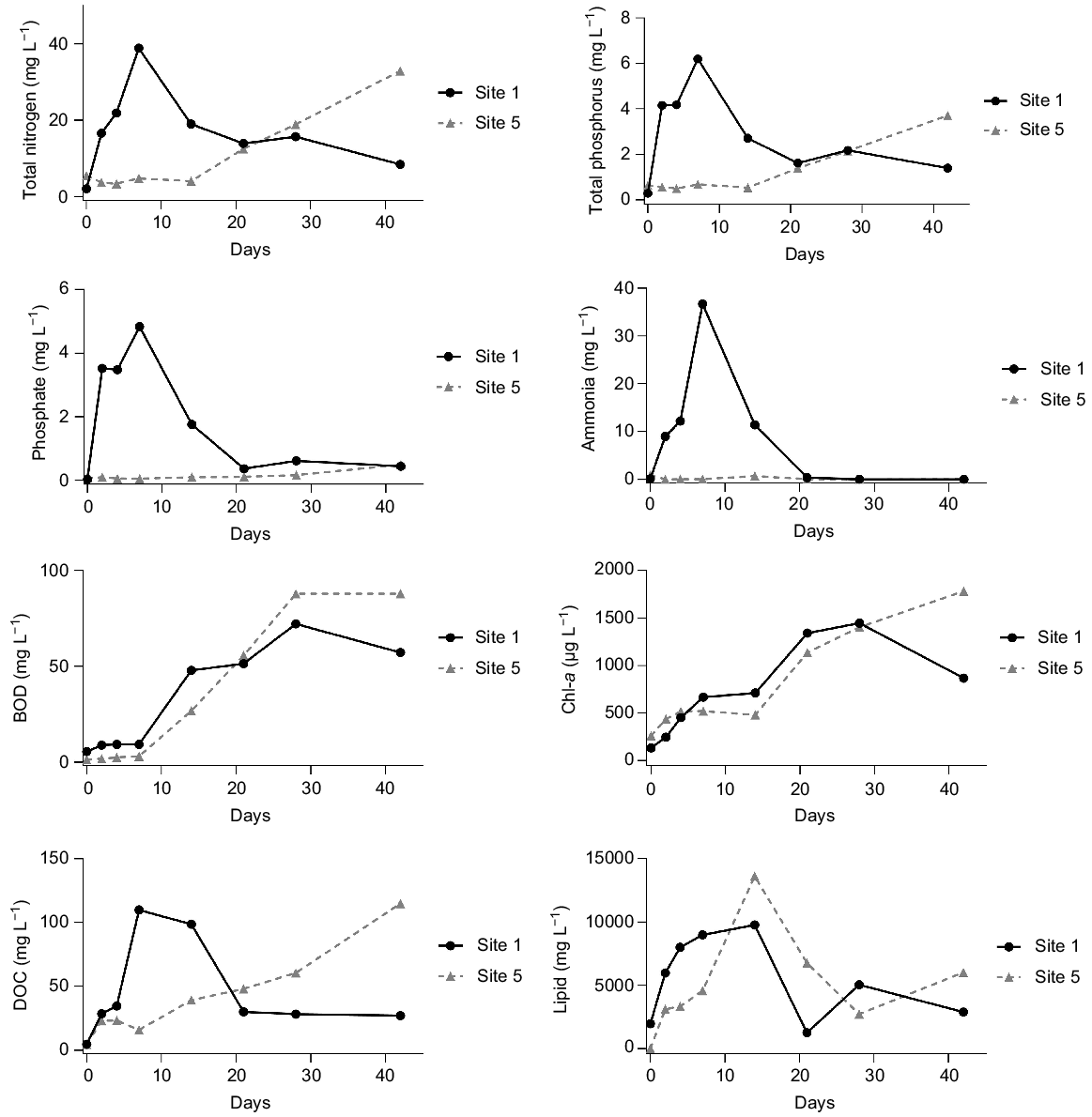
Item | Day 0 maximum concentration (mg L−1) | Maximum concentration detected (mg L−1) | Default guideline value (mg L−1) | |
---|---|---|---|---|
Total phosphorus | 0.639 | 6.2 | 0.1 | |
Total nitrogen | 5.5 | 38.9 | 1 | |
Phosphate | 0.07 | 4.8 | 0.04 | |
Ammonia | 0.886 | 36.7 | 0.9A | |
Nitrate | 0.015 | 0.07 | 0.1 | |
Nitrite | 0.006 | 0.03 | 0.1 | |
DOC | 4.8 | 196 | – | |
BOD | 5.52 | 95.3 | 15B | |
Chl-a (μg L−1) | 261.1 | 1854 | 0.005C | |
Lipid | 1960 | 13,600 | – |
Taken from Australia and New Zealand Guidelines for Fresh and Marine Water Quality (Australian and New Zealand Environment and Conservation Council and Agriculture and Resource Management Council of Australia and New Zealand 2000). All default guideline values are for lowland-river systems of southern-central Australia unless otherwise specified. DOC, dissolved organic carbon; BOD, biological oxygen demand; Chl-a, chlorophyll-a.
The magnitude of this increase correlated with proximity to the carp release point or areas of carp accumulation owing to strong northerly winds. The highest concentrations of TP, total nitrogen (TN), phosphate and ammonia were observed at Site 1, followed by Site 2 and Site 3 respectively. Peak concentrations recorded for TP, TN, phosphate and ammonia were 6.2, 38.9, 4.8 and 36.7 mg L−1 respectively. These values markedly contrast with the initial Day 0 concentrations of 0.64, 5.5, 0.07 and 0.89 mg L−1 for TP, TN, phosphate and ammonia respectively (Table 3). After the initial surge, TP and TN concentrations experienced a temporary decline at Day 14, before gradually rising towards the conclusion of the experiment. Conversely, ammonia and phosphate concentrations decreased significantly following the Day 7 peak and remained low for the duration of the study. This reduction is likely to have resulted from phytoplankton uptake and the oxidation of ammonia to nitrate. Sites 4, 5 and 6 exhibited a gradual increase in nutrient concentrations throughout the experiment, presumably owing to the exchange of water from areas with higher carp density across the wetland.
Sites 1, 2, 3 and 4 exhibited a spike in DOC concentrations within the initial 2 weeks, reaching a peak of 196 mg L−1 at Sites 3 and 4 on Day 14 (Fig. 4, Table S1). DOC concentrations declined when approaching Day 21, before increasing consistently for the rest of the experiment. Sites 5 and 6 experienced a continuous rise in DOC concentration throughout the experiment.
BOD increased following the introduction of the carp carcasses, which was likely to be a result from the release of bioavailable carbon compounds from the carp. BOD across all sites rose throughout the experiment, with Site 6 exhibiting the highest concentrations of 95.3 mg L−1 on Day 28.
Lipid concentrations reached their highest values on Day 14, with Site 5 recording a peak at 13,600 mg L−1. The release of nutrients from decomposing carp carcasses is likely to have spurred algal growth, as evidenced by a consistent rise in chlorophyll-a at all sites throughout the experiment. The highest chlorophyll-a concentration, 1854 μg L−1, was observed at Site 3 on Day 42.
Even when scaled to a carp biomass of 150 kg ha−1, interpolated wetland chlorophyll-a, TN, TP, phosphate and ammonia concentrations were extremely high, reaching estimated mean values of 331.6 ± 68.0, 6.96 ± 1.61, 0.83 ± 0.28, 0.155 ± 0.183 and 1.41 ± 2.08 mg L−1 respectively (Table 4). At a scaled carp biomass of 500 kg ha−1, interpolated BOD was calculated to reach 22.0 ± 2.26 mg L−1. Interpolated nitrite and nitrate remained at low levels for all scales of carp biomass.
Nutrient | Biomass | ||||
---|---|---|---|---|---|
2400 kg ha−1 | 1000 kg ha−1 | 500 kg ha−1 | 150 kg ha−1 | ||
Total phosphorus (mg L−1) | 3.76 ± 1.25 | 1.94 ± 0.64 | 1.29 ± 0.43 | 0.83 ± 0.28 | |
Total nitrogen (mg L−1) | 28.90 ± 6.70 | 15.25 ± 3.54 | 10.38 ± 2.41 | 6.96 ± 1.61 | |
Phosphate (mg L−1) | 1.43 ± 1.69 | 0.637 ± 0.752 | 0.353 ± 0.418 | 0.155 ± 0.183 | |
Ammonia (mg L−1) | 9.30 ± 13.7 | 4.39 ± 6.47 | 2.64 ± 3.89 | 1.41 ± 2.08 | |
Nitrate (mg L−1) | 0.022 ± 0.022 | 0.018 ± 0.018 | 0.016 ± 0.016 | 0.015 ± 0.015 | |
Nitrite (mg L−1) | 0.014 ± 0.009 | 0.009 ± 0.006 | 0.008 ± 0.005 | 0.006 ± 0.004 | |
DOC (mg L−1) | 155.3 ± 43.3 | 67.5 ± 18.8 | 36.2 ± 10.08 | 14.2 ± 3.96 | |
BOD (mg L−1) | 84.8 ± 8.70 | 38.6 ± 3.96 | 22.0 ± 2.26 | 10.5 ± 1.07 | |
Chl-a (μg L−1) | 1389.2 ± 285 | 731.2 ± 150 | 496.1 ± 101.8 | 331.6 ± 68.0 | |
Lipid (mg L−1) | 10,337 ± 3155 | 5456 ± 1665 | 3712 ± 1133 | 2492 ± 761 |
Values below detection threshold (<0.005) were considered to be equal to 0.004. Standard deviations of interpolated concentrations were assumed to be equal to those observed at 2400 kg ha−1. DOC, dissolved organic carbon; BOD, biological oxygen demand; Chl-a, chlorophyll-a.
Discussion
Our study has highlighted key factors to consider before implementing methods that cause mass carp mortality in Australia. These include potential deoxygenation of the water column and increased algal productivity (inferred from chlorophyll-a concentrations) owing to the decomposition of carp carcasses and nutrient loading from carp-derived compounds such as dissolved organic carbon, lipids and proteins (Fig. 4). When utilised by microbes, these compounds exert a considerable BOD.
Effect of carp decomposition on DO
Mesocosm experiments demonstrated that temperature plays a major role in the onset of hypoxia and anoxia following carp mortality, by influencing the development, growth and respiration of microbial communities (Stevenson and Childers 2004). Carp mesocosms at 18°C became anoxic approximately twice as rapidly as the 12°C mesocosms, generally aligning with literature stating that a 10°C increase doubles oxygen demand (Howitt et al. 2007). The 12°C mesocosm, being below the virus’ permissive temperature range of 18–28°C, primarily served as the low-risk scenario. However, decomposition at these temperatures is important for understanding fish mortalities in deep, thermally stratified lakes, where dead carp may sink to cooler waters. In such cases, nutrient release may be extended over a greater time period, prolonging algal blooms. Nevertheless, there is a heightened risk of hypoxic and anoxic conditions in regions where elevated water temperatures overlap with high carp biomass, such as in wetland systems where water temperatures can reach 30°C during summer (King et al. 2009; Whitworth et al. 2012). This is particularly true in areas of low or no flow, including backwaters and floodplains, which may facilitate the aggregation of carp carcasses, resulting in significantly higher carp biomass accumulations than current estimates (Monaghan and Milner 2008). The wetland experiment simulated these conditions, dumping 6000 kg of carp in one location and observing the most rapid and severe increases in nutrient concentrations and BOD occurring closest to the dump site.
Although an attempt was made to evenly distribute carp throughout the wetland, prevailing winds resulted in an uneven distribution. The result was a carp carcass-density gradient across the sample sites, with the highest density at Site 1 and the lowest at Site 5. This uneven distribution is likely to have caused the slightly earlier onset and prolonged presence of anoxic conditions at Site 1 relative to mesocosm experiments of Pera et al. (2022). At the highest carp density, anoxic conditions lasted for 13 days. Additionally, overnight anoxic conditions were common, with DO regularly falling below 0.1 mg L−1, even at Site 5, driven by high DOC concentrations and phytoplankton respiration. The wetland experiment confirmed that microbial decomposition of carp carcasses after a mass-mortality event can severely draw down DO concentrations to severity that precludes the survival of local native aquatic biota, potentially disrupting food-web stability.
Effect of carp decomposition on nutrients and chlorophyll-a
Bucket experiments showed that most nutrients are liberated from carp carcasses within the first 2 weeks, consistent with Pera et al. (2022). This pattern was also observed in the wetland experiment, where nutrient concentrations spiked initially as carp-derived nutrients entered the water column, particularly at Site 1 where carp density was higher than in the other sampling sites.
Over the duration of the wetland experiment, a notable increase in chlorophyll-a concentration was recorded, corresponding with progressively more widespread algal blooms within LDL. The relationship between surplus nutrients and increased phytoplankton biomass has been robustly established (e.g. Correll 1998). In freshwater environments, phosphorus is typically the constraining nutrient, with phytoplankton blooms occurring at phosphorus concentrations as low as 0.01–0.06 mg L−1 (Vuorio et al. 2020). The bucket experiments recorded phosphorus concentrations varying from 0.02 mg L−1 in the low-biomass and deep-water scenario, to 0.67 mg L−1 in the high-biomass and shallow-water scenario. Furthermore, the wetland experiment recorded peak phosphorus and nitrogen concentrations of 6.2 and 38.9 mg L−1 respectively, vastly exceeding the guideline values for lowland rivers of southern-central Australia (0.1 mg L−1 for TP and 1 mg L−1 for TN; Australian and New Zealand Environment and Conservation Council and Agriculture and Resource Management Council of Australia and New Zealand 2000).
The TP concentrations between Site 1 and Site 5 reflected the uneven distribution of carp, although they remained consistent with the mesocosm experiments of Pera et al. (2022). However, the maximum chlorophyll-a value of 1854 μg L−1 in the wetland experiment was far greater than the ~700 μg L−1 reported in Pera et al. (2022) under similar carp densities. Furthermore, although initial TP, TN, phosphate and chlorophyll-a already exceeded recommended guidelines, the addition of carp greatly exacerbated these issues, resulting in calculated ammonia and BOD exceeding guideline values at carp densities of 150 and 500 kg ha−1 respectively (Australian and New Zealand Environment and Conservation Council and Agriculture and Resource Management Council of Australia and New Zealand 2000).
Although the initial flux of carp-derived nutrients into the water column was evident in algal blooms over the duration of the wetland experiment, consideration also needs to be given to the contribution of sedimented organic matter on collapse of the bloom. The decomposition of this organic matter can increase the potential for oxygen depletion in the sediment (Paerl 2008). This may have caused the extended anoxia at Sites 1 and 2 on c. Day 28. Furthermore, sedimentation of nutrients in algal biomass may have strong positive feedback on future events. These legacy nutrients may stay in sediment for some time and contribute to future cyanobacterial blooms. Therefore, in addressing nutrient release from carp carcasses, it is critical to consider not only the ambient hydrodynamics at the time of CyHV-3 implementation, but also the flow and hydrodynamics in the following years. These future conditions could enable legacy nutrients to support algal blooms and must be considered in management strategies.
Algal blooms are not the only concern following carp decomposition. During the wetland experiment, a secondary fish-mortality event was observed between Day 14 and Day 21, where ~150 large (>40 cm) floating carp individuals were observed distributed throughout LDL. Given carp’s tolerance and ability to withstand poor water quality, the death of these fish is particularly notable. During this time, heightened ammonia concentrations were detected, which may have played a role in the fish deaths. Ammonia toxicity, primarily owing to un-ionised ammonia (NH3), is influenced by numerous factors, including DO, pH and temperature (Randall and Tsui 2002). Ammonia concentrations exceeding a 30-day rolling average of 1.9 mg L−1 at a pH of 7 and a temperature of 20°C poses a severe ammonia toxicity risk for freshwater fish, with common carp exhibiting a threshold value of 16.53 mg L−1 (United States Environmental Protection Agency 2013). Alarmingly, ammonia concentrations during the wetland experiment peaked at 36.7 mg L−1. This high concentration, combined with low DO, high pH and elevated temperature, could be sufficient to cause the secondary fish-mortality event (Parvathy et al. 2023).
The carp wetland trial provided a partial demonstration of a ‘worst-case scenario’ following the implementation of CyHV-3 as a carp biocontrol. Although the biomass of 2400 kg ha−1 used in this experiment may be representative of parts of the system, it is not representative of the whole system. Carp biomass density in Australian waters is highly variable, with mean lowland and mid-slope biomasses estimated at 265 to 3144 kg ha−1 respectively (Harris and Gehrke 1997; Driver et al. 2005; Gehrke et al. 2010; Thwaites et al. 2010; Stuart et al. 2021). There is also potential for carp carcasses to aggregate in slow-flowing wetlands and backwaters, which may increase biomass by an order of magnitude in these areas (Monaghan and Milner 2008). This variation may affect the risks of deoxygenation, nutrient loading and the potential for harmful algal blooms to form. As such, using the findings of spatially or temporally restricted studies alone to assess these risks across the whole system may mis-estimate the likelihood and severity of such events.
Although majority of the default guideline thresholds were surpassed, including at the lowest biomass concentration, these calculations assume 100% mortality of carp biomass and do not account for the potential mortality of other aquatic organisms. In contrast, real-world mortality rates will likely be lower, with typical mortality rates reported by studies ranging 50–95% (Thresher et al. 2018; Samsing et al. 2021); however, secondary mortality events for carp and other aquatic biota resulting from poor water quality are a possibility. The size of the carp population and the extent of mortality will have an influence over the pool of nutrients made available, thereby affecting the severity of environmental side effects. Similarly, each distinct habitat may be characterised by unique hydrodynamic properties that allow for varying amounts of carp biomass. Factors such as water temperature, residence times and the frequency of eutrophication play crucial roles in regulating oxygen consumption, nutrient release and algal proliferation. A thorough understanding of these dynamics is essential to accurately predict both the immediate and persistent ecological impacts following the introduction of the CyHV-3 biocontrol agent.
Accounting for water-quality concerns in a CypHV-3 release strategy
Decomposing carp carcasses can promote considerable BOD and algal proliferation by enriching the water with nutrients. The degree of BOD and nutrient enrichment is influenced by the density of carp, which differs across habitats. Recent estimates across the Murray–Darling Basin show a carp density gradient, with lower abundances in headwater streams (0–50 kg ha−1) and higher densities in the low-lying lower Murray River (400–1000 kg ha−1; Stuart et al. 2019). Upland rivers typically had a carp density of less than 200 kg ha−1, aligning with the low biomass density in the bucket experiments reported here. At these carp densities, water is likely to suffer oxygen drawdown if there was a mass carp mortality event, but anoxia is unlikely to result. In lowland-river reaches, a mass carp-mortality event from CypHV-3 release could cause hypoxic conditions.
The high concentrations of lipids, proteins and other organic compounds from decaying carp may also pose a problem for water treatment following mass carp die-off in source water for potable supply. These dissolved organic compounds and high concentrations of phytoplankton can contribute to disinfection by-product formation in drinking-water treatment (Tomlinson et al. 2016: Brookes and Tomlinson 2019).
Our study, alongside Pera et al. (2022), has demonstrated that the rate of carp decay is rapid, increases with temperature and nutrient release is mostly completed within 2 weeks. These findings have implications for clean-up protocols if CyHV-3 is to be deployed as a biocontrol agent. To prevent nutrient and DOC flux into the water, it is critical to remove carp biomass promptly, ideally within a few days. The nutrients released from decomposing carp are likely to be bioavailable, providing the resources needed for substantial algal blooms. However, the species of algae that proliferates may vary depending on seasonal conditions and local hydrodynamics. The vast biomass of carp spread across Australian waterways represent a substantial pool of nutrients that, following mass carp mortality, could be incorporated into phytoplankton biomass and enrich sediment nutrient concentrations. Such legacy nutrients may sustain algal growth for an extended period after a mass-mortality event, posing risks to native fish habitat and potable water supplies. Detailed knowledge of the risk and planning is applied at the reach, wetland and reservoir scale. Clean-up of fish carcasses will likely remove only the floating carcasses, as submerged carcasses are not visible in the turbid river system. The scale of clean-up, remoteness of many river reaches and disposal of dead fish biomass present considerable logistical challenges. However, the ability to predict the extent and severity of increased BOD and nutrient enrichment after mass carp mortality requires a more comprehensive understanding of the density and distribution of carp throughout the MDB.
Given the typical densities in the upper- and mid-river reaches (0–400 kg ha−1; Stuart et al. 2021), mass carp mortality is unlikely to cause widespread anoxia, although localised hypoxia or anoxia may occur. Releasing the virus within the cooler range of its permissive range would slow the rate of decay, gradually reducing oxygen concentrations.
Nutrient release could have both immediate and long-lasting effects. Rapid nutrient release could fuel phytoplankton growth, while also forming a legacy pool of nutrients in the sediment, which may be remobilised under certain redox conditions and resuspensions, further supporting phytoplankton growth. The problematic cyanobacteria typically occur during low flow when thermal stratification can establish (Baker et al. 2000), supported by the presence of gas vesicles that provide buoyancy (Brookes et al. 2000), which is particularly advantageous to reduce settling during calm periods. Finding a productive use for harvested fish carcasses, such as fertiliser production, would aid in reducing legacy nutrients in the system.
Successful biocontrol relies on decreasing populations of the target species to levels that reduce the degree of environmental harm. The success of carp control in Australia using CypHV-3 is debated (Boutier et al. 2019). If pursued, water-quality impacts must be managed, with nutrient-release effects potentially taking months to years to manifest.
Conclusions
We have demonstrated that carp decomposition considerably contributes to BOD and algal growth through nutrient enrichment and is strongly influenced by carp density. Decomposition and nutrient release happen rapidly, predominantly within the first 2 weeks, necessitating prompt clean-up measures. Within the upper- and mid-river reaches, mass carp mortality may lead to localised areas of hypoxia or anoxia, although an improved understanding of carp density and distribution is needed to better predict the implications of large-scale carp-mortality events.
Data availability
The data that support this study will be shared upon reasonable request to the corresponding author.
Declaration of funding
Funding for this project was provided by the Australian Government through the Fisheries Research and Development Corporation (project numbers 2017-055 and 2017-056) and The Department of Primary Industries and Regions (PIRSA).
References
Baker PD, Brookes JD, Burch MD, Maier HR, Ganf GG (2000) Advection, growth and nutrient status of phytoplankton populations in the lower River Murray, South Australia. Regulated Rivers: Research & Management 16, 327-344.
| Crossref | Google Scholar |
Becker JA, Ward MP, Hick PM (2019) An epidemiologic model of koi herpesvirus (KHV) biocontrol for carp in Australia. Australian Zoologist 40, 25-35.
| Crossref | Google Scholar |
Bligh EG, Dyer WJ (1959) A rapid method of total lipid extraction and purification. Canadian Journal of Biochemistry and Physiology 37, 911-917.
| Crossref | Google Scholar | PubMed |
Boutier M, Donohoe O, Kopf RK, Humphries P, Becker JA, Marshall J, Vanderplasschen A (2019) Biocontrol of carp: the Australian plan does not stand up to a rational analysis of safety and efficacy. Frontiers in Microbiology 10, 882.
| Crossref | Google Scholar |
Brookes JD, Tomlinson A (2019) Pick your poison: do cyanotoxins or disinfection by-products pose the greater risk? Inland Waters 9, 345-347.
| Crossref | Google Scholar |
Brookes JD, Ganf GG, Oliver RL (2000) Heterogeneity of cyanobacterial gas-vesicle volume and metabolic activity. Journal of Plankton Research 22, 1579-1589.
| Crossref | Google Scholar |
Brookes JD, Aldridge K, Wallace T, Linden L, Ganf GG (2005) Multiple interception pathways for resource utilisation and increased ecosystem resilience. Hydrobiologia 552, 135-146.
| Crossref | Google Scholar |
Clunie P, Koehn J (1997) Recovery plans for silver perch and catfish. In ‘Riverine Environment Research Forum. Proceedings of the inaugural River Environment Research Forum of Murray–Darling Basin Commission Natural Resource Management Strategy’, 4–6 October 1995, Attwood, Melboune, Vic, Australia. (Eds RJ Banens, R Lehane) pp. 13–24. (Murray–Darling Basin Commission: Canberra, ACT, Australia)
Correll DL (1998) The role of phosphorus in the eutrophication of receiving waters: a review. Journal of Environmental Quality 27, 261-266.
| Crossref | Google Scholar |
Dean TL, Richardson J (1999) Responses of seven species of native freshwater fish and a shrimp to low levels of dissolved oxygen. New Zealand Journal of Marine and Freshwater Research 33, 99-106.
| Crossref | Google Scholar |
Driver PD, Harris JH, Norris RH, Closs GP (1997) The role of the natural environment and human impacts in determining biomass densities of common carp in New South Wales rivers. In ‘Fish and rivers in stress: the NSW rivers survey’. (Eds JH Harris, P Gehrke) pp. 225–269. (NSW Fisheries Office of Conservation and the Cooperative Research Centre for Freshwater Ecology: Sydney, NSW, Australia)
Driver PD, Harris JH, Closs GP, Koen TB (2005) Effects of flow regulation on carp (Cyprinus carpio L.) recruitment in the Murray–Darling Basin, Australia. River Research and Applications 21, 327-335.
| Crossref | Google Scholar |
Ebner B (2006) Murray cod an apex predator in the Murray River, Australia. Ecology of Freshwater Fish 15, 510-520.
| Crossref | Google Scholar |
Gehrke PC (1988) Response surface analysis of teleost cardio-respiratory responses to temperature and dissolved oxygen. Comparative Biochemistry and Physiology – A. Physiology 89, 587-592.
| Crossref | Google Scholar |
Gehrke PC, Revell MB, Philbey AW (1993) Effects of river red gum, Eucalyptus camaldulensis, litter on golden perch, Macquaria ambigua. Journal of Fish Biology 43, 265-279.
| Crossref | Google Scholar |
Gehrke PC, Brown P, Schiller CB, Moffatt DB, Bruce AM (1995) River regulation and fish communities in the Murray–Darling River system, Australia. Regulated Rivers: Research & Management 11, 363-375.
| Crossref | Google Scholar |
Ger KA, Urrutia-Cordero P, Frost PC, Hansson L-A, Sarnelle O, Wilson AE, Lürling M (2016) The interaction between cyanobacteria and zooplankton in a more eutrophic world. Harmful Algae 54, 128-144.
| Crossref | Google Scholar | PubMed |
Ghadouani A, Pinel-Alloul B, Plath K, Codd GA, Lampert W (2004) Effects of Microcystis aeruginosa and purified microcystin-LR on the feeding behavior of Daphnia pulicaria. Limnology and Oceanography 49, 666-679.
| Crossref | Google Scholar |
Gotesman M, Kattlun J, Bergmann SM, El-Matbouli M (2013) CyHV-3: the third cyprinid herpesvirus. Diseases of Aquatic Organisms 105, 163-174.
| Crossref | Google Scholar | PubMed |
Gulati R, Demott W (1997) The role of food quality for zooplankton: remarks on the state-of-the-art, perspectives and priorities. Freshwater Biology 38, 753-768.
| Crossref | Google Scholar |
Hicks BJ, Osborne MW, Ling N (2005) Quantitative estimates of fish abundance from boat electro-fishing. In ‘A guide to monitoring fish stocks and aquatic ecosystems’, 11–15 July 2005, Darwin, NT, Australia. (Eds MJ Phelan, H Bajhau) pp. 104–111. (Northern Territory Department of Primary Industry, Fisheries and Mines)
Howitt JA, Baldwin DS, Rees GN, Williams JL (2007) Modelling blackwater: predicting water quality during flooding of lowland river forests. Ecological Modelling 203, 229-242.
| Crossref | Google Scholar |
Killberg-Thoreson L, Sipler RE, Heil CA, Garrett MJ, Roberts QN, Bronk DA (2014) Nutrients released from decaying fish support microbial growth in the eastern Gulf of Mexico. Harmful Algae 38, 40-49.
| Crossref | Google Scholar |
King AJ, Robertson AI, Healey MR (1997) Experimental manipulations of the biomass of introduced carp (Cyprinus carpio) in billabongs. I. Impacts on water-column properties. Marine and Freshwater Research 48, 435-443.
| Crossref | Google Scholar |
King AJ, Tonkin Z, Mahoney J (2009) Environmental flow enhances native fish spawning and recruitment in the Murray River, Australia. River Research and Applications 25, 1205-1218.
| Crossref | Google Scholar |
King AJ, Tonkin Z, Lieshcke J (2012) Short-term effects of a prolonged blackwater event on aquatic fauna in the Murray River, Australia: considerations for future events. Marine and Freshwater Research 63, 576-586.
| Crossref | Google Scholar |
Koehn JD (2004) Carp (Cyprinus carpio) as a powerful invader in Australian waterways. Freshwater Biology 49, 882-894.
| Crossref | Google Scholar |
Koehn JD, Todd CR, Zampatti BP, Stuart IG, Conallin A, Thwaites L, Ye Q (2018) Using a population model to inform the management of river flows and invasive carp (Cyprinus carpio). Environmental Management 61, 432-442.
| Crossref | Google Scholar | PubMed |
Kopf RK, Humphries P, Bond NR, Sims NC, Watts RJ, Thompson RM, Hladyz S, Koehn JD, King AJ, McCasker N, McDonald S (2019a) Macroecology of fish community biomass – size structure: effects of invasive species and river regulation. Canadian Journal of Fisheries and Aquatic Sciences 76, 109-122.
| Crossref | Google Scholar |
Kopf RK, Boutier M, Finlayson CM, Hodges K, Humphries P, King A, Kingsford RT, Marshall J, McGinness HM, Thresher R, Vanderplasschen A (2019b) Biocontrol in Australia: can a carp herpesvirus (CyHV-3) deliver safe and effective ecological restoration? Biological Invasions 21, 1857-1870.
| Crossref | Google Scholar |
Lampert W (1987) Laboratory studies on zooplankton-cyanobacteria interactions. New Zealand Journal of Marine and Freshwater Research 21, 483-490.
| Crossref | Google Scholar |
Lewis WM, Jr (1970) Morphological adaptations of cyprinodontoids for inhabiting oxygen deficient waters. Copeia 1970, 319-326.
| Crossref | Google Scholar |
Lighten J, van Oosterhout C (2017) Biocontrol of common carp in Australia poses risks to biosecurity. Nature Ecology & Evolution 1(3), 0087.
| Crossref | Google Scholar |
Linden LG, Lewis DM, Burch MD, Brookes JD (2004) Interannual variability in rainfall and its impact on nutrient load and phytoplankton in Myponga Reservoir, South Australia. International Journal of River Basin Management 2, 169-179.
| Crossref | Google Scholar |
Luo X, Liu Z, Gulati RD (2015) Cyanobacterial carbon supports the growth and reproduction of Daphnia: an experimental study. Hydrobiologia 743, 211-220.
| Crossref | Google Scholar |
McCarthy B, Zukowski S, Whiterod N, Vilizzi L, Beesley L, King A (2014) Hypoxic blackwater event severely impacts Murray crayfish (Euastacus armatus) populations in the Murray River, Australia. Austral Ecology 39, 491-500.
| Crossref | Google Scholar |
McColl KA, Sunarto A (2020) Biocontrol of the common carp (Cyprinus carpio) in Australia: a review and future directions. Fishes 5, 17.
| Crossref | Google Scholar |
McColl KA, Sunarto A, Slater J, Bell K, Asmus M, Fulton W, Hall K, Brown P, Gilligan D, Hoad J, Williams LM, Crane MSJ (2017) Cyprinid herpesvirus 3 as a potential biological control agent for carp (Cyprinus carpio) in Australia: susceptibility of non-target species. Journal of Fish Diseases 40, 1141-1153.
| Crossref | Google Scholar | PubMed |
McKinnon LJ (1995) Emersion of Murray crayfish, Euastacus armatus (Decapoda: Parastacidae), from the Murray River due to post-flood water quality. Proceedings-Royal Society of Victoria 107, 31-37.
| Google Scholar |
McMaster D, Bond N (2008) A field and experimental study on the tolerances of fish to Eucalyptus camaldulensis leachate and low dissolved oxygen concentrations. Marine and Freshwater Research 59, 177-185.
| Crossref | Google Scholar |
McNeil DG, Closs GP (2007) Behavioural responses of a south-east Australian floodplain fish community to gradual hypoxia. Freshwater Biology 52, 412-420.
| Crossref | Google Scholar |
Marshall JC, Blessing JJ, Clifford SE, Hodges KM, Negus PM, Steward AL (2019) Ecological impacts of invasive carp in Australian dryland rivers. Aquatic Conservation: Marine and Freshwater Ecosystems 29, 1870-1889.
| Crossref | Google Scholar |
Monaghan KA, Milner AM (2008) Salmon carcass retention in recently formed stream habitat. Fundamental and Applied Limnology 170, 281-289.
| Crossref | Google Scholar |
Müller-Navarra DC, Brett MT, Liston AM, Goldman CR (2000) A highly unsaturated fatty acid predicts carbon transfer between primary producers and consumers. Nature 403, 74-77.
| Crossref | Google Scholar | PubMed |
Paerl H (2008) Nutrient and other environmental controls of harmful cyanobacterial blooms along the freshwater–marine continuum. In ‘Cyanobacterial harmful algal blooms: state of the science and research needs. Vol. 619’. Advances in Experimental Medicine and Biology. (Ed. HK Hudnell) pp. 217–237. (Springer)
Paerl HW, Fulton RS, Moisander PH, Dyble J (2001) Harmful freshwater algal blooms, with an emphasis on cyanobacteria. The Scientific World Journal 1, 76-113.
| Crossref | Google Scholar | PubMed |
Parvathy AJ, Das BC, Jifiriya MJ, Varghese T, Pillai D, Rejish Kumar VJ (2023) Ammonia induced toxico-physiological responses in fish and management interventions. Reviews in Aquaculture 15, 452-479.
| Crossref | Google Scholar |
Pera JB, Davie AW, Rohlfs A-M, Mitrovic SM (2022) Simulating the potential effects of a carp virus fish kill on water quality and phytoplankton in lentic environments. Marine and Freshwater Research 73, 178-192.
| Crossref | Google Scholar |
Rakus K, Ouyang P, Boutier M, Ronsmans M, Reschner A, Vancsok C, Jazowiecka-Rakus J, Vanderplasschen A (2013) Cyprinid herpesvirus 3: an interesting virus for applied and fundamental research. Veterinary Research 44, 85.
| Crossref | Google Scholar |
Randall DJ, Tsui TKN (2002) Ammonia toxicity in fish. Marine Pollution Bulletin 45, 17-23.
| Crossref | Google Scholar | PubMed |
Samsing F, Hopf J, Davis S, Wynne JW, Durr PA (2021) Will Australia’s common carp (Cyprinus carpio) populations develop resistance to cyprinid herpesvirus 3 (CyHV-3) if released as a biocontrol agent? Identification of pathways and knowledge gaps. Biological Control 157, 104571.
| Crossref | Google Scholar |
Schoenebeck CW, Brown ML, Chippss SR, German DR (2012) Nutrient and algal responses to winterkilled fish-derived nutrient subsidies in eutrophic lakes. Lake and Reservoir Management 28, 189-199.
| Crossref | Google Scholar |
Small K, Kopf RK, Watts RJ, Howitt J (2014) Hypoxia, blackwater and fish kills: experimental lethal oxygen thresholds in juvenile predatory lowland river fishes. PLoS ONE 9, e94524.
| Crossref | Google Scholar |
Stevenson C, Childers DL (2004) Hydroperiod and seasonal effects on fish decomposition in an oligotrophic everglades marsh. Wetlands 24, 529-537.
| Crossref | Google Scholar |
Stocks JR, Davis S, Anderson MJ, Asmus MW, Cheshire KJM, van der Meulen DE, Walsh CT, Gilligan DM (2021) Fish and flows: abiotic drivers influence the recruitment response of a freshwater fish community throughout a regulated lotic system of the Murray–Darling Basin, Australia. Aquatic Conservation: Marine and Freshwater Ecosystems 31, 3228-3247.
| Crossref | Google Scholar |
Stuart I, Fanson B, Lyon J, Stocks J, Brooks S, Norris A, Bennett A (2019) A national estimate of carp biomass for Australia. Unpublished Client Report for the Fisheries Research and Development Corporation Arthur Rylah Institute for Environmental Research, Department of Environment, Land, Water and Planning, Melbourne, Vic., Australia. Available at https://www.ari.vic.gov.au/__data/assets/pdf_file/0032/442877/ARI-Client-Report-National-estimate-of-carp-biomass-for-Australia.pdf
Stuart IG, Fanson BG, Lyon JP, Stocks J, Brooks S, Norris A, Thwaites L, Beitzel M, Hutchison M, Ye Q, Koehn JD, Bennett AF (2021) Continental threat: how many common carp (Cyprinus carpio) are there in Australia? Biological Conservation 254, 108942.
| Crossref | Google Scholar |
Sunarto A, McColl KA, Crane MSJ, Schat KA, Slobedman B, Barnes AC, Walker PJ (2014) Characteristics of cyprinid herpesvirus 3 in different phases of infection: implications for disease transmission and control. Virus Research 188, 45-53.
| Crossref | Google Scholar | PubMed |
Thresher RE, Allman J, Stremick-Thompson L (2018) Impacts of an invasive virus (CyHV-3) on established invasive populations of common carp (Cyprinus carpio) in North America. Biological Invasions 20, 1703-1718.
| Crossref | Google Scholar |
Thwaites L, Fleer D, Smith B (2010) Estimating the biomass of adult common carp in Lake Albert, South Australia: a progress report for biosecurity SA. SARDI Publication Number F2010/000681-1, SARDI Research Report Series Nyumber 490. (SARDI Aquatic Sciences: Adelaide, SA, Australia) Available at https://pir.sa.gov.au/__data/assets/pdf_file/0003/232059/No_490_Estimating_the_biomass_of_adult_common_carp_in_Lake_Albert_South_Australia.pdf
Tomlinson A, Drikas M, Brookes JD (2016) The role of phytoplankton as pre-cursors for disinfection by-product formation upon chlorination. Water Research 102, 229-240.
| Crossref | Google Scholar | PubMed |
Vilizzi L, Thwaites LA, Smith BB, Nicol JM, Madden CP (2014) Ecological effects of common carp (Cyprinus carpio) in a semi-arid floodplain wetland. Marine and Freshwater Research 65, 802-817.
| Crossref | Google Scholar |
Vuorio K, Järvinen M, Kotamäki N (2020) Phosphorus thresholds for bloom-forming cyanobacterial taxa in boreal lakes. Hydrobiologia 847, 4389-4400.
| Crossref | Google Scholar |
Whitworth KL, Baldwin DS, Kerr JL (2012) Drought, floods and water quality: drivers of a severe hypoxic blackwater event in a major river system (the southern Murray–Darling Basin, Australia). Journal of Hydrology 450-451, 190-198.
| Crossref | Google Scholar |
Whitworth KL, Baldwin DS, Kerr JL (2014) The effect of temperature on leaching and subsequent decomposition of dissolved carbon from inundated floodplain litter: implications for the generation of hypoxic blackwater in lowland floodplain rivers. Chemistry and Ecology 30, 491-500.
| Crossref | Google Scholar |