Nuanced influences of subtidal artificial shellfish structures on nekton communities in urbanised estuaries
Brad Martin

A
B
Abstract
Reef installation is increasingly considered for urbanised estuaries to enhance and restore ecological functions. Restoration structures are expected to provide nekton habitat benefits, but early outcomes are poorly understood.
This study assessed nekton assemblage variation associated with an oyster reef restoration site, situated within the anthropogenically modified Port River–Barker Inlet estuary.
Nekton communities and environmental variables were measured 6 weeks before, and 14 months following restoration, and at a control site. Modelling was used to assess spatio-temporal variation.
Video monitoring detected 34 species, with harvestable nekton comprising 60.3% of total abundances. Nekton assemblages were strongly influenced by interannual effects, with few outcomes being directly related to restoration activities. Restoration structures supported non-native gobies during the study.
These results suggest that small-scale restoration can have little detectable impact on urbanised fish communities in the early stages of estuarine restoration. Nekton were influenced by environmental variables with distinct seasonal variation.
In urban estuaries characterised by pre-existing artificial structures, small trial oyster reefs may be functionally redundant as nekton habitats until sufficient spatial-scale and functional changes are achieved.
Keywords: artificial structure, estuary management, fish habitat, non-native, oyster reef, restoration, urbanisation, video monitoring.
Introduction
Estuarine environments are among the most productive and socioeconomically valuable systems worldwide (Barbier et al. 2011; Grabowski et al. 2012). Estuaries have underpinned the development of coastal cities and industry, because ports and harbours are essential for maritime trade and fisheries (Taylor and Suthers 2021). These systems also support beneficial ecosystem functions, including fish production, coastal protection, water quality maintenance and carbon sequestration (Snelgrove et al. 2014; Barbier 2017). Often, these valuable functions are supported by the persistence of biogenic habitats, including mangrove forests, seagrass meadows and shellfish reefs (Mahoney and Bishop 2017; Smith et al. 2023). However, estuarine urbanisation has accelerated in the past two centuries (Lotze et al. 2006; Todd et al. 2019). The cumulative effects of anthropogenic stressors, including pollution, channel modification and overfishing, have caused declines in natural habitats and associated functions (Halpern et al. 2008; Taylor and Suthers 2021). Thus, with the ongoing human reliance on these systems, there is a significant need to understand potential opportunities to mitigate or reverse estuarine ecosystem degradation.
In urbanised estuaries, biogenic habitats characterised by mangroves, seagrass or oyster reefs are often replaced by simplified environments such as unstructured soft-sediment or artificial infrastructure (e.g. seawalls, wharves, rock walls). These simplified habitats typically support lower species diversity, and less beneficial ecosystem functions than for natural baselines (Halpern et al. 2008; Clifton et al. 2022). Subsequently, practitioners in estuarine management are increasingly considering habitat structure and opportunities to improve estuary socioecological value through restoration and eco-engineering (Chapman et al. 2018). This includes installing ‘living seawalls’ to restore benthic complexity and biodiversity (Clifton et al. 2022), ‘artificial reefs’ to enhance fisheries and fish habitat (Chong et al. 2024), and restoring habitat-forming organisms for living shoreline defence (Morris et al. 2024).
Coastal habitat restoration efforts worldwide have demonstrated enhancements and recovery of biodiversity and ecological functions (Saunders et al. 2020; Smith et al. 2023; Sievers et al. 2024). However, restoration projects have also had mixed successes, with some efforts being insufficient in scale, or producing limited or partial ecosystem recovery (Hale and Swearer 2017; Fraschetti et al. 2021). For example, Fraschetti et al. (2021) undertook a meta-analysis of coastal restoration projects and identified that 54% are <1 ha, a scale informative for trialling techniques but not recommended for evaluating habitat restoration success. As restoration projects are labour intensive and costly (Bayraktarov et al. 2016), it is important that practitioners mitigate against unfavourable restoration outcomes and work towards informed, large-scale ecosystem restoration (Saunders et al. 2020). This can include environmental and social suitability assessments prior to restoration (Howie et al. 2024), ongoing monitoring efforts (Baggett et al. 2015), evaluation at multiple spatial scales and manipulative experiments for aspects of habitat functioning (Fraschetti et al. 2021). Such approaches are particularly relevant for urban estuaries where site suitability can be limited by affected environmental conditions, human-use conflicts and ongoing anthropogenic stressors (Howie and Bishop 2021).
Oyster reefs, composed of biogenic oyster aggregations, were once important components of temperate and tropical estuaries globally (Beck et al. 2011; Richardson et al. 2022). Today, they are absent or degraded from many estuaries because of anthropogenic pressures, including overharvesting, declining water quality and disease (e.g. Beck et al. 2011; Gillies et al. 2020; Thurstan et al. 2024). The loss of biogenic habitat and filter-feeding organisms is thought to have diminished the productivity, biodiversity and socioeconomic value of coastal ecosystems worldwide (Grabowski et al. 2012). However, increased awareness of oyster reef loss, combined with their socioecological value, has accelerated oyster reef restoration efforts within the past two decades (Fitzsimons et al. 2020; Smith et al. 2023). Oyster restoration has generally involved installing suitable substrates for larval oyster settlement (e.g. limestone, sterilised shell) and restocking populations through translocation (Fitzsimons et al. 2020; Howie and Bishop 2021). Such approaches have been used to manage wild oyster fisheries in the United States for over 60 years (Bersoza Hernández et al. 2018), with recent efforts being focused on restoring beneficial ecological functions (Smith et al. 2023). Oyster reef restoration has increasingly occurred in Australia since 2015 (McAfee et al. 2022) where natural oyster reefs have failed to recover (Gillies et al. 2020), providing opportunities to enhance fisheries (Gilby et al. 2018) and biofiltration (Ray and Fulweiler 2021).
The Port River–Barker Inlet Estuary system is among the most anthropogenically modified estuaries in southern Australia (Bossley et al. 2017). Since European settlement (est. 1836), urbanisation of the upper estuarine environment has contributed to declines in coastal and supralittoral habitats (Daniels et al. 2005). This includes subtidal flat oyster (Ostrea angasi) reefs, which became functionally extinct across South Australia by the 1950s, with one remnant reef in Tasmania (Gillies et al. 2020; Martin et al. 2025a). Today, the estuary’s subtidal environment is dominated by bare muddy benthos and artificial infrastructure (Hammer 2014). Given that oyster reefs in other regions are associated with enhanced nekton (fishes, decapods, cephalopods) biodiversity relative to degraded control sites (Gilby et al. 2018; Smith et al. 2023), the loss of O. angasi reefs from the Port River–Barker Inlet Estuary has likely affected nekton communities. However, a small-scale oyster reef restoration trial commenced in December 2022 as part of the ‘Shellfish Revolution’ initiative, led by Ozfish Unlimited (see ozfish.org.au/programs/shellfish-restoration). Modular steel gabion units filled with sterilised oyster shell were installed to restore biogenic shellfish habitat, with the objective of establishing a foundational substrate for O. angasi reef development (e.g. Walters et al. 2022).
As enhancements in fisheries-targeted species and nekton biodiversity were key objectives of the oyster reef restoration, this study aimed to evaluate changes in the nekton community during the early stages of restoration. Specifically, we hypothesised that following oyster reef installation, the restoration site would (1) maintain elevated total nekton species richness and abundances, and (2) support a different multivariate nekton community relative to the bare control site. Additionally, we evaluated temporal shifts in the environmental variables and nekton community, hypothesising that (3) nekton community metrics across sites would vary across seasons, with the lowest total richness and abundances occurring during winter, when fewer fishes are likely to be present. This approach would improve environmental baselines for the urbanised upper section of the Port River–Barker Inlet Estuary, which has been historically understudied (e.g. Hammer 2014), a limitation that may be posed in other urban estuaries.
Materials and methods
Study system and restoration
This study took place in Port Adelaide, the urbanised upper section of the Port River–Barker Inlet Estuary, situated on the eastern side of the Gulf St Vincent, South Australia (34°50′43.8″S, 138°29′51.9″E). Owing to channel modification, the system forms an inverse estuary, with seawater inflows from the north and south via Outer Harbour and the West Lakes intake pipe respectively, and intermittent freshwater inputs from the Dry Creek wetland and stormwater drains. The ~0.28-ha oyster reef restoration site was established near Jervois Bridge, abutting a quay wall and a rock ‘rip-rap’ revetment (Fig. 1). This area once sustained flat oyster (O. angasi) reefs (historically termed ‘beds’) prior to the 1920s, on the basis of historical Indigenous and European Australian records (reviewed in Martin et al. 2025a). The site also hosted the Port Adelaide Fish Market (pre-1960s) with ~40 relict remnant wooden supporting beams present across the site. A bare control site near Birkenhead Bridge was established 400 m downstream, where habitat restoration has not historically occurred. This control site abuts a quay wall and is dominated by a bare mud benthos. Both sites are situated along the main Port River channel (5–6-m depth) and located within the Port Adelaide urban centre, and are therefore likely to experience similar environmental conditions. The quay walls at both sites support pre-existing sessile invertebrate communities, including Pacific oysters (Magallana gigas), black mussels (Mytilus spp.), Mediterranean fan worms (Sabella spallanzanii) and sea squirts, whereas the bare benthos is mainly characterised by turfing algae and terebellid bristle worms. As estuarine O. angasi reefs are functionally extinct locally (Alleway and Connell 2015), we were unable to include an established oyster reef as an additional reference control.
Map of Inner Harbour in the Port River–Barker Inlet Estuary, South Australia, depicting the oyster reef restoration site (red border) and bare control site surveyed from 2022 to 2024, with red circles representing remote underwater video station (RUVS) deployment locations. Above-water photographs of the restoration and control habitats are shown, along with a representative image of the RUVS field of view.
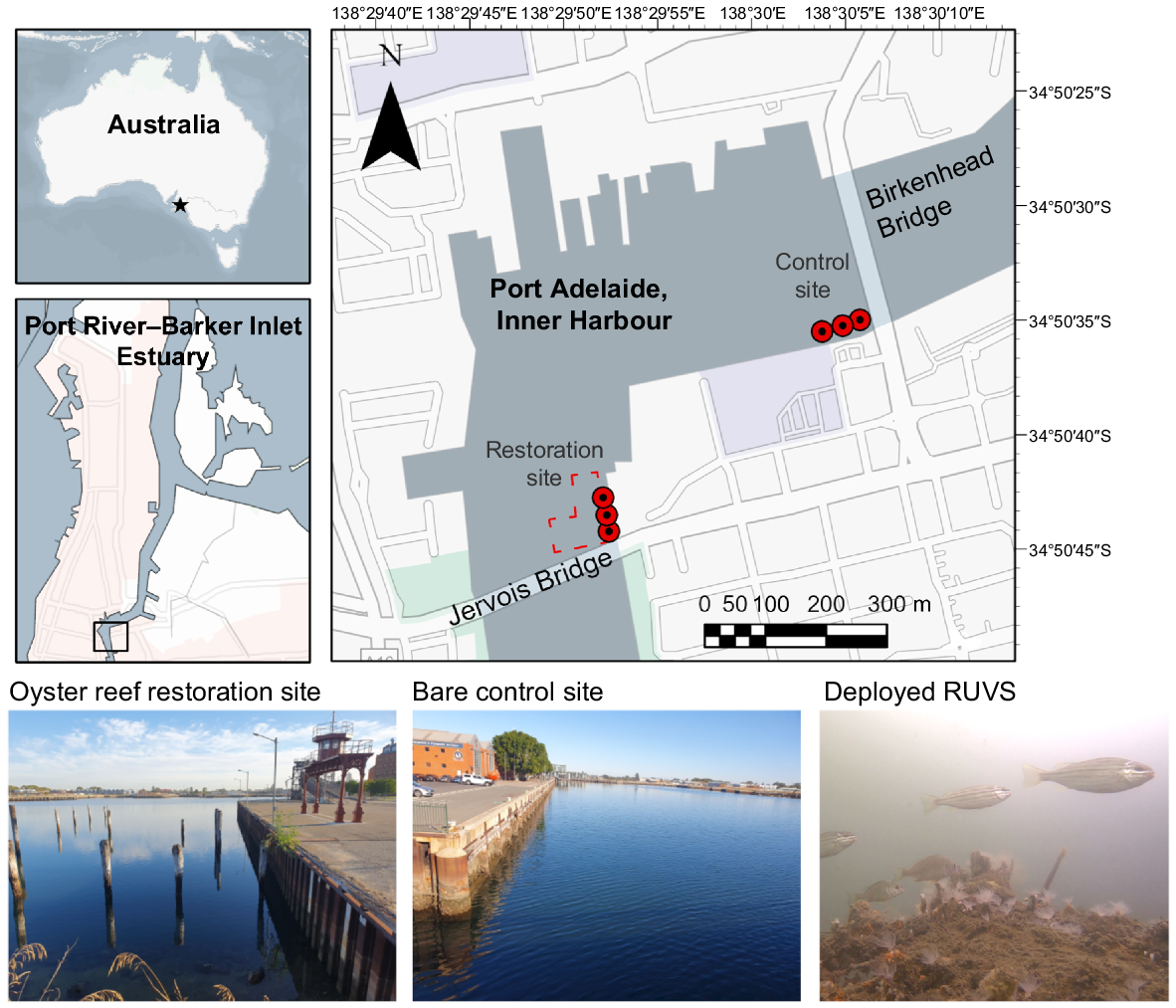
Subtidal oyster reef restoration in the Port River–Barker Inlet Estuary has occurred only at Jervois bridge, commencing in 2018, with efforts expanded in 2022, led by not-for profit organisations OzFish Unlimited and the Estuary Care Foundation. Some 80 modular gabion mesh baskets (40 × 40 × 30 cm) containing sterilised Pacific oyster shell were deployed by volunteers at the single restoration site, along with adult O. angasi broodstock (~50 individuals) during a single event in December 2022. This coincided with known O. angasi spawning periods when seawater temperatures were above 17°C (McAfee and Connell 2020). The units form a low-relief structural substrate for settlement by O. angasi larvae and other species. By 6 months post-restoration, each unit supported ~1000 O. angasi spat (n = 3 units; I. Kenny, unpubl. data, 2024). Successive settlement onto the units is expected to facilitate the re-establishment of flat oyster populations, and their associated functions.
Sampling approach
Nekton surveys began in November 2022 and occurred approximately every 3 weeks until February 2024. This included three survey periods prior to restoration, in November and December 2022, which provided a ‘before installation’ baseline. Post-restoration surveys commenced 3 weeks after installation of the modular oyster units between January 2023 and February 2024. Nekton were assessed from 20 survey timepoints across 14 months to evaluate temporal variability in the abiotic and biotic conditions associated with restoration.
The nekton communities at each site were surveyed using unbaited remote video stations (RUVS) during daylight hours (07:30 to 17:30 hours). Each RUVS consisted of a GoPro Hero7 camera attached to a weighted frame and positioned 15 cm above the benthos when deployed. The cameras were unbaited to minimise bait biases in interpreting the fish-habitat associations (Martinez-Baena et al. 2022; Martin et al. 2025b). RUVS were deployed for 35 min, because short-durations are suitable to rapidly quantify fish habitat use in complex and turbid estuary settings (Baker et al. 2022). Owing to the single oyster reef restoration site, and small restoration footprint available, three RUVS were deployed >15 m apart at each site along the adjacent wharf, with a replicate deployment occurring 1–5 h later for each timepoint (n = 12). Although this design was suboptimal for capturing broad spatial variability within the estuary, it provided a localised assessment of the monitored sites.
To minimise biases associated with sediment disturbance, only 30 min of footage was assessed, with analysis being delayed until the sediment plume had settled. Videos were processed in EventMeasure (ver. 6.45, see www.seagis.com.au), with all nekton (e.g. fishes and decapods) being identified to the lowest taxonomic level to quantify community composition and taxonomic richness (Gomon et al. 2008). Abundance was calculated as the maximum number of individuals within a single frame (MaxN) (Whitmarsh et al. 2016). Additionally, all species were categorised into water column guilds (e.g. Maus et al. 2024), consisting of (1) benthic species found on the benthos, (2) demersal species associated near the benthos, or (3) benthopelagic species that move and feed between the benthos and water column. We also calculated the number of ‘harvestable’ and ‘invasive’ nekton species on the basis of their socioecological categorisation as fisheries-targeted (i.e. FishBase) or as non-native species (i.e. Wiltshire et al. 2010; Hammer 2014).
All fieldwork was carried out according to permissions obtained from Flinders University Animal Ethics Committee (AEC:5317).
Environmental variables
Field of view composition percentages of each RUVS deployment was calculated using ImageJ (ver. 1.54j, W. S Rasband, US National Institutes of Health, Bethesda, MD, USA, see http://imagej.nih.gov/ij/) from a single screenshot to quantify the cover proportion of visible consolidated benthic structures, unstructured bare mud, wharf wall and the water column. Surface water temperature and salinity measurements were collected in situ, and a Secchi disc was used to estimate water clarity and maximum water depth. The maximum and minimum air temperature, and maximum wind speed (km h−1) and direction were obtained from the Parafield Airport weather observation station for each deployment day (see http://www.bom.gov.au/climate/averages/tables/cw_023013.shtml).
Statistical analyses
The environmental and nekton community data were statistically analysed in RStudio (ver. 4.3.1, Posit Software, PBC, Boston, MA, USA, see https://posit.co/products/open-source/rstudio/). We first tested for potential site (two levels: restoration and control) differences in the measured water variables and field of view percentages using non-parametric Mann–Whitney U-tests. The environmental conditions were assumed to be independent of restoration activities and driven by other temporal variables (e.g. weather, hydrodynamics).
Spatio-temporal variation in nekton community structure was investigated using permutational multivariate analysis of variance (PERMANOVA) from the vegan package (ver. 2.6-4, J. Oksanen et al., see https://cran.r-project.org/package=vegan/). The PERMANOVA was conducted to assess for significant differences between site (fixed factor; two levels: restoration and control sites), and stage (fixed factor; two levels: before and after restoration), with season (four levels; spring, summer, autumn and winter), replicate (two levels), and stage:time (23 survey periods) as covariates in the PERMANOVA design to account for potential temporal autocorrelation in the data. Prior to analysis, potential skewness in the data was assessed through a series of transformations and visualised using shade plots to identify whether transformation to normalise the data was needed, and then checked for overdispersion. This showed that the multivariate data required fourth-root transformation to reduce the effects of overly abundant schooling fishes before calculating Bray–Curtis dissimilarly matrices. A dummy variable of +1 was added to the data because of surveys where nekton were absent (Clarke et al. 2006). Owing to the unbalanced number of surveys by stage, we focused the analyses on its interaction with other factors to mitigate potential biases. Post hoc pairwise PERMANOVAs were used to assess the sources of significant difference within levels of the factors.
For general comparisons, the community data were visualised using two separate non-metric multidimensional scaling (nMDS) plots depicting potential groupings by site, and the interaction of site and stage to support interpretation of possible effects of the main factors. A distance-based redundancy analysis (dbRDA) was used to ordinate the transformed-community data and identify potential relationships to the environmental variables. Using the ‘envit’ function of the vegan package, vectors from the environmental variables were tested for significant correlation to the ordination axes (9999 permutations) and fitted to the dbRDA plot, with the overlays depicting the direction and strength of the relationship.
Dufrene–Legendre indicator species analysis from the labdsv package (ver. 2.1-0, D. W. Roberts, see https://CRAN.R-project.org/package=labdsv; Roberts and Roberts 2016) was used to determine indicator values for each species on the basis of their frequency and abundance, and identify species that differed across sites and restoration stages. We then used univariate linear mixed effects models from the packages glmmTMB (ver. 1.1.9, see https://cran.r-project.org/package=glmmTMB; Booth et al. 2003) and car (ver. 3.1-2, see https://CRAN.R-project.org/package=car; Fox and Weisberg 2018) to test for patterns in the (1) nekton total abundance and richness; (2) abundances of species identified from the indicator analysis, and abundance based on the (3) socioecological categories, and (4) water column position guilds. The model tested for interactions among the fixed factors of site and stage, with season, replicate and time as random factors to account for temporal variation. All models used a negative binomial distribution, and were checked for collinearity and overdispersion. For each model, we used the MuMIn package (ver. 1.48.4, see http://r-forge.r-project.org/projects/mumin/) to calculate the marginal and conditional R2-values to estimate the proportion of variance explained by the fixed factors, and in combination with the random factors to evaluate potential temporal effects.
Results
Environmental conditions
Weather conditions recorded from the Parafield Weather Station were variable across surveys (Fig. 2). Maximum wind conditions were 20–67 km h−1, typically in a westerly direction (Fig. 2a). Rainfall (mm) was relatively uncommon (17% of survey periods) and ranged from 0 to 2.6 mm (Fig. 2b). Air and water temperatures typically followed seasonal patterns, being warmer during the Austral summer (December–February), and cooler during winter (June–August; 20–40 weeks post-installation) (Fig. 2c, d). Air temperature ranged from daily minimums of 0.2–25.1°C to maximums of 14.1–35.9°C (Supplementary Table S1). Maximum depth was typically shallower at the restoration site (mean ± standard error, 4.47 ± 0.07 m) than at the control site (4.89 ± 0.05 m). Water clarity was also generally poorer at the restoration site (3.47 ± 0.07 m) than at the control sites (3.94 ± 0.06 m), regardless of tidal influence (Fig. 2e, f). Water temperature ranged from 9.3 to 32°C and showed seasonal cooling during the Austral winter period (Fig. 2d). Salinity, measured in parts per thousand (ppt) of dissolved salts, ranged from 20 to 40 ppt, with variation potentially caused by freshwater input from stormwater drains after large rainfall events (Fig. 2g), but did not vary seasonally. The Mann–Whitney U-test results found significant differences by site only for depth and clarity (P < 0.0001; Supplementary Table S1).
Environmental conditions associated with each remote underwater video station (RUVS) monitoring timepoint between November 2022 and February 2024, from the restoration (Res. site) and control (Con. site) sites in the Port River–Barker Inlet Estuary. This includes the (a) maximum (max.) wind speed and direction, (b) rainfall and (c) air temperature (temp.) recorded from the Parafield Airport; mean values of in situ measurements of (d) water temperature, (e) depth, (f) water clarity (Secchi disc depth), and (g) salinity; and (h) proportional bar graph of RUV field of view composition aggregated across sites. The vertical red dashed line delineates between the before and after restoration stages.
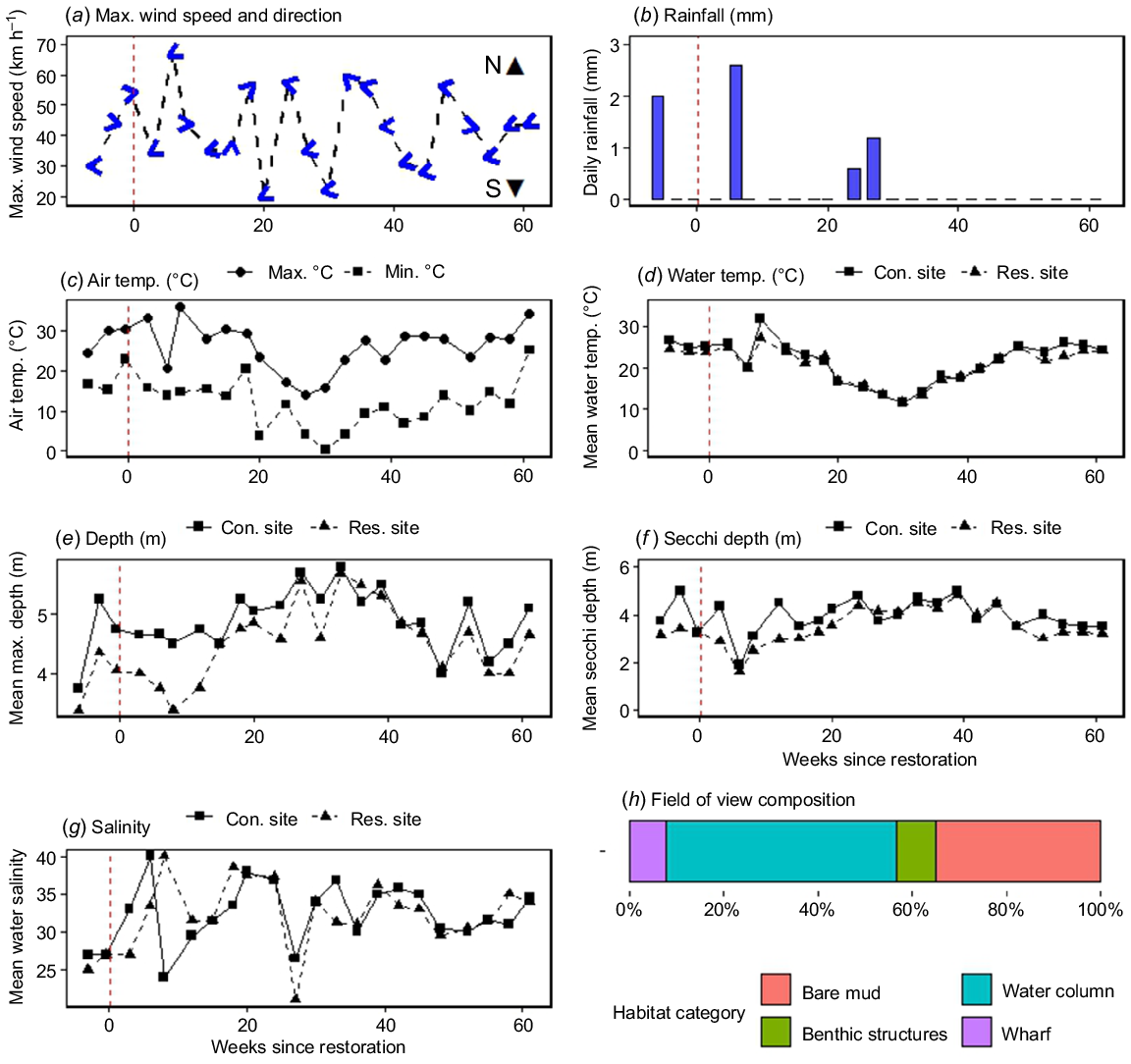
Video monitoring
Of the 276 RUVS deployments, 252 recordings were included in the analysis, consisting of 121 videos from the oyster reef restoration site, and 131 videos from the control site. Twenty-four deployments were excluded owing to recording issues (e.g. malfunctions, unsuccessful placement, tipping over).
The field of view composition was similar across sites on the basis of the Mann–Whitney U-test results for each category (P > 0.05, Table S1). The benthos was typically dominated by bare mud (mean ± standard error: 35.0 ± 1.5%) with some consolidated benthic structures (8.4 ± 0.8%) including pylons, debris (e.g. pipes, fishing gear, discarded shopping trollies) and biogenic structures, such as disarticulated Pacific oyster shells (M. gigas), spaghetti bryozoans (Amathia verticillata) and Mediterranean fan worms (S. spallanzanii). The vertical composition of the field of view consisted of the wharf wall (7.7 ± 1.2%), and the water column (48.9 ± 1.8%; Fig. 2h).
Surveyed nekton assemblages
In total, 4941 nektonic organisms were recorded from the RUVS, consisting of 31 fish species from 17 families, and 2 decapod species. From 6 weeks prior to oyster reef installation in December 2022, the RUVS detected a mean (±s.e.) of 4.6 ± 0.4 nekton species, and 22.5 ± 3.0 total abundances, across 36 deployments. In the 61 weeks following restoration activities to February 2024, the RUVS averaged 3.9 ± 0.1 nekton species, and 19.1 ± 1.16 total abundance, across 216 deployments. Most species (71%) were surveyed at both sites, with only three species exclusively observed from the restoration site, v. nine species uniquely sighted at the control site. However, these ‘site-specific’ species were rare, and were detected only in <3% of videos (Fig. 3, Table S2). Only 14 species (41%) were detected from the surveys across all four seasons (Fig. 3). The most ubiquitous species observed were black bream (Acanthopagrus butcheri, 83%), southern longfin gobies (Favonigobius lateralis, 48%) and western striped grunters (Pelates octolineatus, 42%).
Bubble plot representing the combined abundances of nekton species across sites detected during each sample period from remote underwater video station surveys, coloured by categorical values of season (spring, green; summer, orange; autumn, brown; winter, blue).
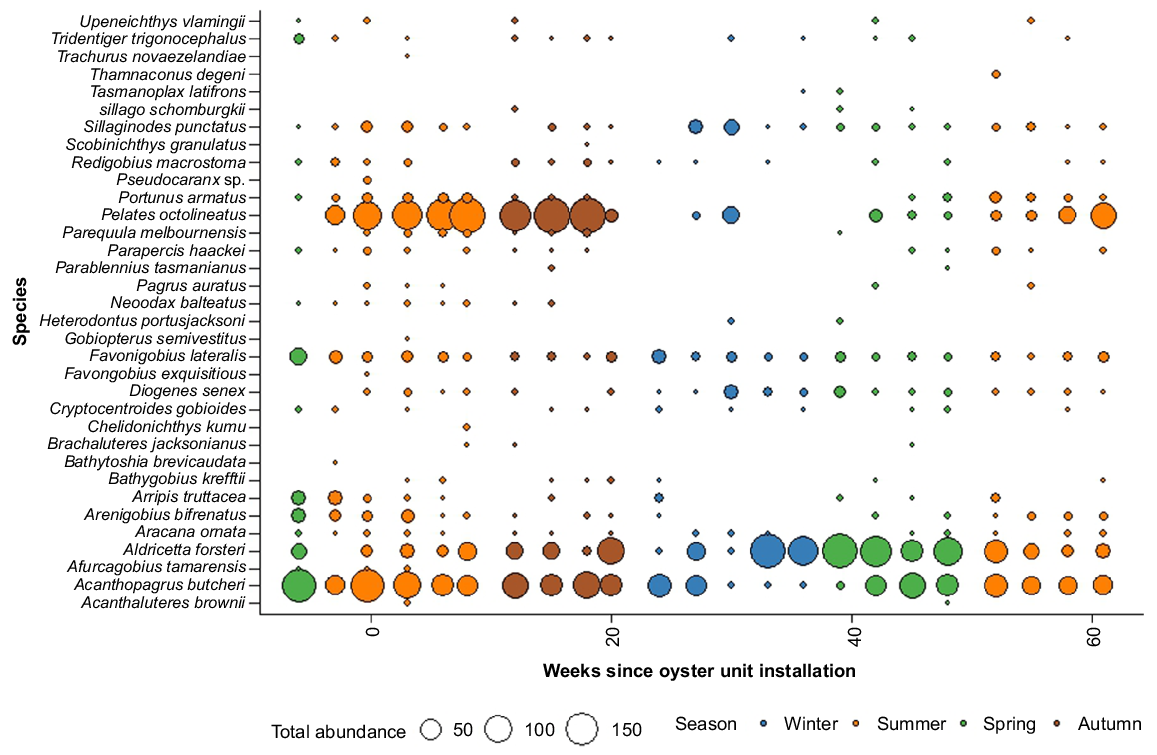
Of the observed nekton, eight were considered harvestable species, comprising 60.3% of total abundance (i.e. primarily A. butcheri and yelloweye mullet, Aldrichetta forsteri). Non-native Gobiidae species comprised 1.7% of the total abundance, and included crested oystergoby (Cryptocentroides gobioides), trident goby (Tridentiger trigonocephalus), largemouth goby (Redigobius macrostoma) and exquisite sandgoby (Favongobius exquisitious). Of the 34 nekton species, the majority were classified as demersal (38.2%) or benthic (35.3%), comprising 15.7% of total abundance across all sites and surveys. Benthopelagic species (nine species) comprised the majority of observed nekton (84.2% of total abundance).
Nekton community structure
Nekton assemblages differed among sites, and before and after oyster reef restoration, but these factors did not significantly interact (Table 1). The position of the ellipses showed some separation across surveys by both site and stage respectively, but there was considerable overlap in the combination of these factors (Fig. 4). Given that pre-restoration sampling occurred only in spring–summer, the greater variation of the post-restoration nekton community (Fig. 4b) can be attributed to the larger sample size, and seasonal nekton variability from autumn and winter surveys. The nekton assemblages were also temporally variable across survey timepoints on the basis of the significant interaction of restoration stage and time (Table 1). This was also identified with seasonal variation in the community, where peaks in the occurrence and abundances of most species were typically observed in summer and decreased into the winter period (Table 1, Fig. 3).
PERMANOVA – nekton structure | Pairwise PERMANOVAs | |||||||
---|---|---|---|---|---|---|---|---|
Factor | d.f. | SS | F-value | P-value | Comparison | F-value | P-value | |
Site | 1 | 1.572 | 11.510 | 0.0001* | Control–restoration site | 8.950104 | 0.001* | |
Stage | 1 | 1.348 | 9.8637 | 0.0001* | ||||
Season | 3 | 7.272 | 17.7438 | 0.0001* | Spring–winter | 11.217284 | 0.006* | |
Replicate | 1 | 0.272 | 1.9916 | 0.0782 | Spring–summer | 13.086304 | 0.006* | |
Site × stage | 1 | 0.260 | 1.9051 | 0.0815 | Spring–autumn | 12.327621 | 0.006* | |
Stage × time | 2 | 1.709 | 6.2558 | 0.0001* | Summer–winter | 29.351259 | 0.006* | |
Residual | 242 | 33.062 | Summer–autumn | 5.279817 | 0.006* | |||
Total | 251 | 45.496 | Autumn–winter | 25.737992 | 0.006* |
Pairwise PERMANOVA results also displayed for examining significant differences between sites and seasons. Analysis used Bray–Curtis dissimilarities of the square root-transformed nekton data, with significant results (P < 0.05) denoted by an asterisk. d.f., degrees of freedom; SS, sum of squares.
Non-metric multi-dimensional scaling (nMDS) plots of nekton community structure on the basis of Bray–Curtis dissimilarities. It depicts points categorised by (a) sites, comparing the restoration and control sites, and (b) site and restoration stage, depicting samples before and after oyster restoration, with overlaid 95% confidence ellipses. Stress value was 0.165.
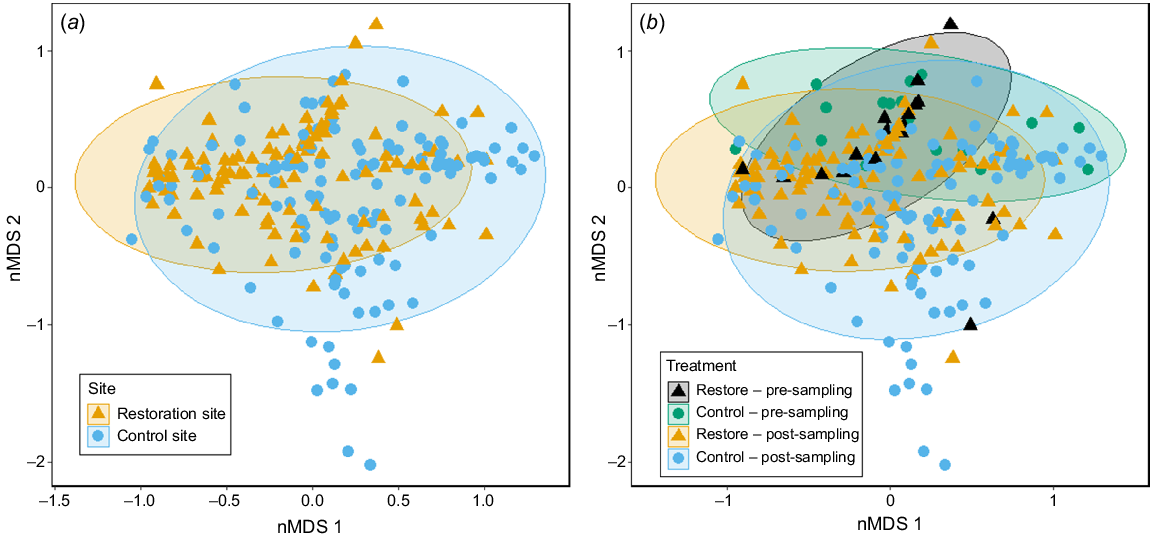
Nekton communities were primarily clustered by season, rather than site-specific clustering, showing a distinct seasonal separation between summer–autumn and winter–spring samples (Fig. 5). These patterns were strongly associated with the measured environmental variables (Fig. 2), particularly temperature, weather patterns and water clarity.
Distance-based redundancy analysis (dbRDA) plot of transformed nekton community data, with points categories across the two sites, and four seasons. Points represent nekton surveys, and are presented by different colours and shapes to represent site and seasonal patterns. Vectors indicate the direction and strength of environmental variables found to have a significant (P < 0.05) correlation with the data.
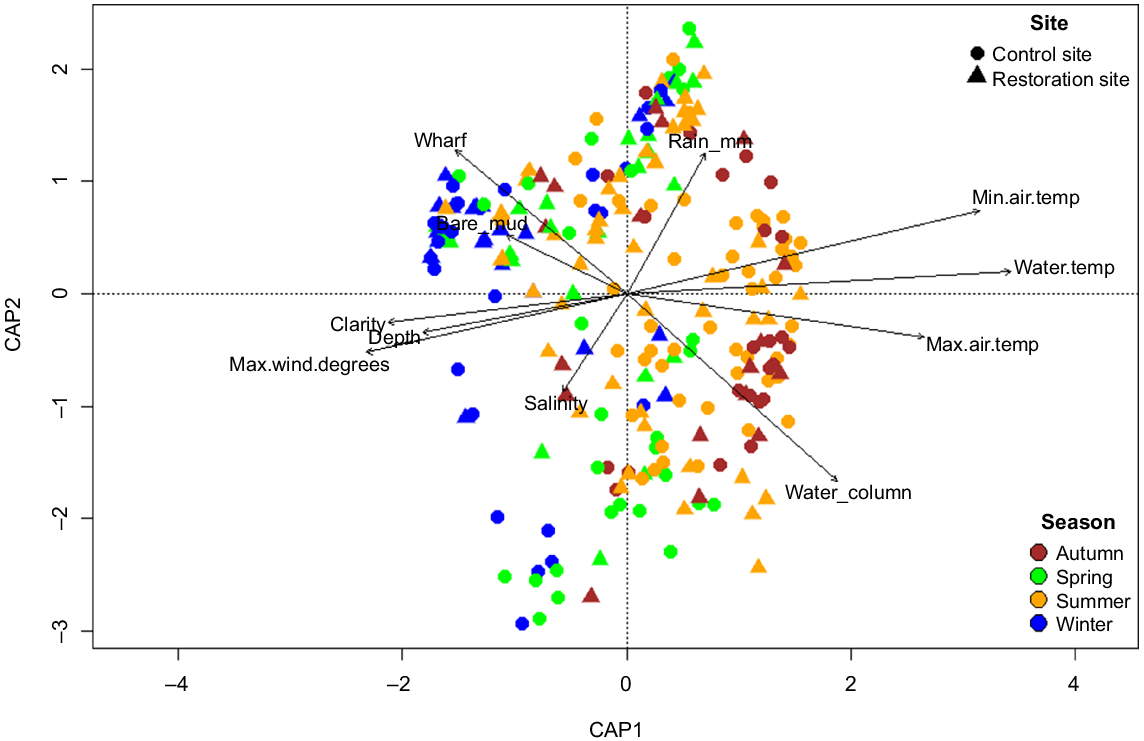
The crested oystergoby (C. gobioides) was the sole indicator species that was positively associated with the restoration site, with greater detection in the months following the oyster reef restoration activities. By contrast, western striped grunters (P. octolineatus) were associated with the control site throughout the study period (Table 2). Both yelloweye mullet (A. forsteri) and wavy grubfish (Parapercis haackei) were identified as indicator species for the control site in the post-restoration period.
Species | Restoration stage | Site | IndVal | P-value | |
---|---|---|---|---|---|
Western striped grunter (Pelates octolineatus) | Before | Control | 0.3495 | 0.038 | |
Crested oystergoby (Cryptocentroides gobioides) | After | Restoration | 0.0890 | 0.008 | |
Yelloweye mullet (Aldricetta forsteri) | After | Control | 0.3624 | 0.008 | |
Western striped grunter (Pelates octolineatus) | After | Control | 0.3682 | 0.003 | |
Wavy grubfish (Parapercis haackei) | After | Control | 0.1422 | 0.001 |
‘Restoration stage’ and ‘site’ denote the level of the factor for which that species is a significant (P < 0.05) indicator.
Nekton species richness and abundances
Variation in the multivariate community structure could be, in part, attributed to site- and seasonal-effects on species presence and abundance. Overall, the linear mixed-effect models indicated elevated total nekton abundances at the control site (F = 27.62, P < 0.001), but similar nekton richness across sites (F = 2.70, P = 0.100; Table 3). The RUVS at the restoration site detected 3.8 ± 0.2 nekton species and 14.5 ± 1.2 total abundance, which was lower than at the control site with 4.2 ± 0.2 species and 24.3 ± 1.7 total abundance (Fig. 6, Supplementary Fig. S1). Similarly, the benthopelagic guild abundances were greater at the control site (F = 27.72, P < 0.001), but both sites supported similar demersal and benthic nekton guild abundances (Fig. S1). The random effects (season, time and replicate) approximately quadrupled the amount of variation explained by the models compared with the fixed effects only (site and stage; Table 3), reflecting the influence of temporal variation in the data (Fig. 3–5). Although surveys in the weeks following restoration showed elevated nekton richness and total abundances at the restoration site, these values generally decreased during the cooler winter period and stayed below abundances at the control site over the proceeding spring–summer period (Fig. 6). Significant interactions of site and stage were identified from both yelloweye mullet (A. forsteri) and western striped grunters (P. octolineatus) (Table 3). Aldricetta forsteri was not detected prior to restoration, but was increasingly common post-restoration, with high abundances at the control site over the winter–spring period (Fig. 6). By contrast, P. octolineatus was detected at the restoration site only post-restoration and was generally detected at higher abundances at the control site throughout the study period (Fig. 6, S1).
Nekton metric | Intercept | Site | Stage | Site × stage | R2-value | ||||||
---|---|---|---|---|---|---|---|---|---|---|---|
F | P | F | P | F | P | F | P | Marginal | Conditional | ||
Total abundance | 477.99 | <0.001* | 27.62 | <0.001* | 0.07 | 0.786 | 0.69 | 0.407 | 0.104 | 0.229 | |
Total richness | 81.33 | <0.001* | 2.70 | 0.100 | 0.00 | 0.953 | 0.00 | 0.969 | 0.009 | 0.273 | |
Harvestable abundance | 404.22 | <0.001* | 16.55 | <0.001* | 0.16 | 0.690 | 3.41 | 0.065 | 0.069 | 0.141 | |
Non-native abundance | 22.31 | <0.001* | 5.20 | 0.023* | 4.23 | 0.040* | 2.02 | 0.155 | 0.071 | 0.109 | |
Crested oystergoby | 22.31 | <0.001* | 5.20 | 0.023* | 4.23 | 0.040* | 2.02 | 0.155 | 0.089 | 0.089 | |
Western striped grunters | 0.40 | 0.526 | 9.36 | 0.002* | 0.06 | 0.807 | 4.46 | 0.035* | 0.075 | 0.708 | |
Yelloweye mullet | 53.37 | <0.001* | 7.81 | 0.005* | 16.07 | <0.001* | 10.44 | 0.001* | 0.178 | 0.185 | |
Wavy grubfish | 17.04 | <0.001* | 7.71 | 0.006* | 0.48 | 0.490 | 1.79 | 0.181 | 0.158 | 0.201 | |
Demersal | 2.59 | 0.108 | 0.32 | 0.572 | 1.73 | 0.188 | 1.89 | 0.169 | 0.011 | 0.175 | |
Benthic | 23.72 | <0.001* | 0.47 | 0.491 | 15.45 | <0.001* | 3.01 | 0.083 | 0.085 | 0.167 | |
Benthopelagic | 247.58 | <0.001* | 27.72 | <0.001* | 0.10 | 0.750 | 1.41 | 0.235 | 0.100 | 0.238 |
The proportional variation explained (R2) by the fixed and random factors combined are denoted as marginal and condition values respectively. Significant (P < 0.05) results are denoted by an asterisk.
Time series plot of mean ± s.e. of the nekton (a) richness, (b) total abundance, (c) western striped grunter, and (d) yelloweye mullet observed per RUV survey timepoint at the restoration and control sites between November 2022 and February 2024. The vertical dashed line denotes restoration activities related to the installation of modular oyster units.
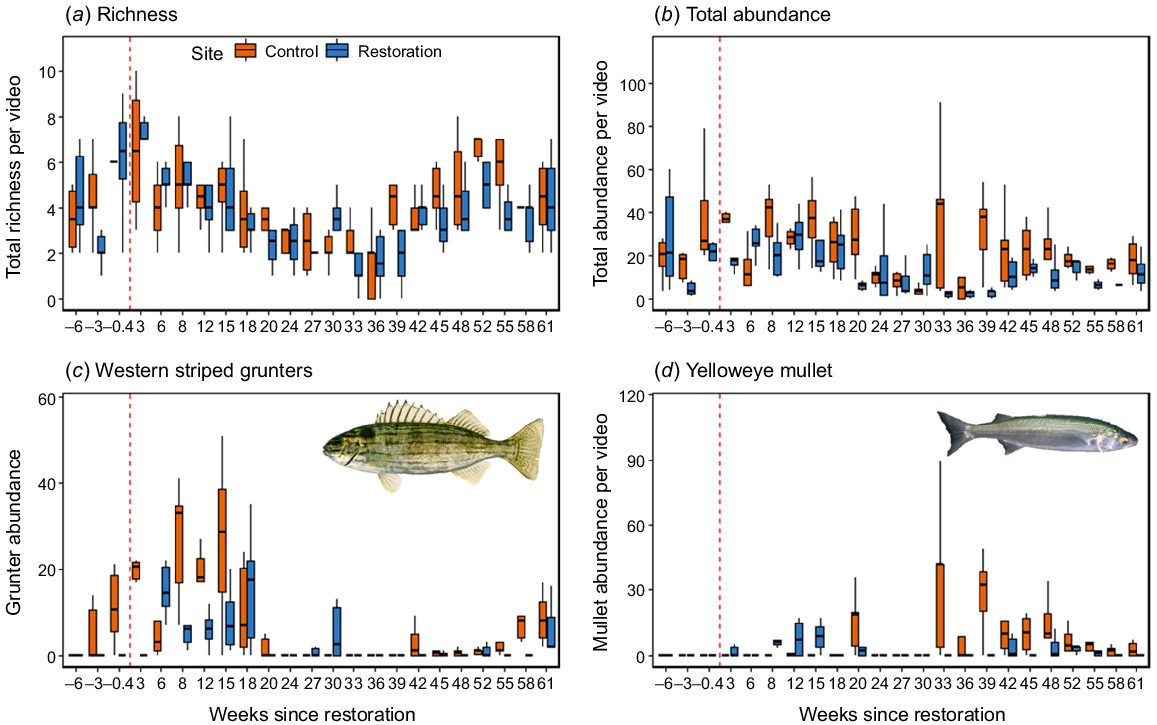
Discussion
Coastal habitat restoration is increasingly occurring worldwide, often aiming to increase nekton biodiversity (i.e. richness, abundances) and enhance fisheries-targeted species (Grabowski et al. 2012; Sievers et al. 2024). For functionally extinct oyster reefs, restoration efforts usually aim to restore these habitats and their associated ecological functions (Gillies et al. 2020; Smith et al. 2023), with small-scale trials guiding upscaled restoration efforts. During our study, the results suggest that pre-existing habitat conditions and seasonal shifts in abiotic variables decreased nekton species richness, total abundances and harvestable abundances detected from RUV surveys at the small-scale restoration site compared with our control site. Both sites supported distinct nekton communities that displayed seasonal variability. These outcomes underscore the value of understanding temporal patterns in abiotic variables for interpreting restoration outcomes, especially for trial reef sites (Baggett et al. 2015; Searles et al. 2022). By pooling observations from both sites, our results also suggest that anthropogenically modified estuaries can function as important nekton habitat (Bradley et al. 2023) and benefit fisheries-targeted species (Jackson and Jones 1999).
Our study provided the first nekton community assessment in the upper reaches of the Port River–Barker Inlet Estuary. We found fisheries-targeted species in high abundances at the surveyed locations, including yelloweye mullet (A. forsteri), black bream (A. butcheri) and King George whiting (Sillaginodes punctatus). By contrast, previous nekton studies in the Port River–Barker Inlet Estuary have predominantly focused on remnant natural habitats (e.g. Jones et al. 1996; Jackson and Jones 1999; Martin et al. 2025c), particularly vegetated sites (mangroves, seagrass, saltmarsh) in the lower sections of the Port River. Hammer (2014) provided an exception, where they monitored Gobiidae species along artificial structures in the Port River–Barker Inlet Estuary. Subsequently, our study highlighted that the fisheries-value within this estuary extends to the highly modified upper section of Port Adelaide.
Nekton community responses
Oyster reef restoration projects are expected to benefit nekton assemblages by increasing beneficial habitat functions, including foraging opportunities, refugia and reducing environmental stressors (Grabowski et al. 2012; Gilby et al. 2018). These functional enhancements may increase fish survival and recruitment, resulting in net gains to nekton biodiversity and productivity (e.g. Smith et al. 2023; Connolly et al. 2024). For example, restored eastern oyster (Crassostrea virginica) reefs in the United States support 34% greater species richness and 99% greater total community biomass compared with degraded (control) sites (Smith et al. 2023). This is further supported by a global meta-analysis of 160 oyster restoration studies, finding that restoration generally enhanced nekton abundance and diversity, albeit not across all studies (Sievers et al. 2024). In regions without remnant reefs or with understudied oyster species, expected nekton responses may be identified only following restoration trials (Zu Ermgassen et al. 2020; Martin et al. 2025b).
Estuarine shellfish restoration trials elsewhere in Australia (e.g. Gilby et al. 2021; Maus et al. 2024) have detected post-restoration enhancements in species richness and fish abundances 1–2 years post-restoration. For example, Gilby et al. (2021) found that by 30 months post-restoration, a 1.4-ha modular Sydney rock oyster (Saccostrea glomerata) reef enhanced fish richness and abundance by 3.8 and 10.7 times respectively, without depleting nekton from the surrounding seascape. Furthermore, at the ‘unit’ scale, oyster garden trials in Morton Bay using caged shellfish found higher fish and invertebrate diversity than in both control cages and non-caged areas (Boström-Einarsson et al. 2022). Contrastingly, our dataset found little observable ecological responses of the nekton community following oyster reef restoration activities. Instead, temporal and interannual variation in environmental conditions, particularly weather and water parameters, were key predictors for interpreting variation in the nekton assemblages. Trends were consistent with established seasonal changes typical of estuarine nekton assemblages (e.g. Chuwen et al. 2009; Potter et al. 2016; Harrison and Whitfield 2021). During the summer and autumn months, when temperature and salinity are generally elevated, nekton diversity was typically greater owing to migrations and spawning pulses of marine species, and lower in winter and spring (Harrison and Whitfield 2021). Similar seasonal patterns have also been recorded from estuarine fish monitoring associated with remnant oyster reefs from New South Wales (Martínez-García et al. 2022) and from 100-m2 mussel restoration sites in the Swan–Canning estuary (Maus et al. 2024). Most shellfish reef fish studies conduct monitoring for ~12 months (Martin et al. 2025b); thus, multi-year or subsequent studies would be required to determine whether some of the observed differences were caused by seasonal or annual variations or whether real ecological changes are occurring from restoration.
Several factors are likely to have contributed to the subdued nekton responses to restoration in our study, including habitat characteristics, environmental stressors and species-specific responses. The modular steel gabion baskets comprise a low-profile reef that cover a small spatial extent (~0.28 ha) across a single location. This small spatial scale of restoration may have limited enhancements in large, mobile nekton (i.e. >20 cm TL; Gilby et al. 2018; Fraschetti et al. 2021), whereas cryptobenthic species (e.g. Gobiidae spp.) that are more sensitive to structural differences (Ushiama et al. 2019) are less detectable by using RUVS (Martin et al. 2025b). Furthermore, our study occurred during the early stages of restoration when nekton are responding to the reef substrate, rather than the biological role of oysters in environmental modification. Successive flat oyster (O. angasi) recruitment combined with upscaled restoration within the estuary may be required before functional modifications (e.g. enhanced biofiltration, bio-deposits, habitat creation) lead to major restoration outcomes on nekton assemblages (Smith et al. 2023). Additional restoration efforts would also increase available shellfish habitat for monitoring, which could lessen the effects of confounding anthropogenic and environmental variables in understanding nekton responses.
In the context of the broader estuary seascape, both the control and restoration sites, although lacking remnant biogenic habitat, contain artificial vertical and benthic structures, including cement wharfs, wooden pylons and debris. These pre-existing consolidated structures may already provide valuable nekton habitat (Chapman et al. 2018), and could make the additional structures from the oyster reef restoration functionally redundant. Similar outcomes have been documented in studies comparing fish communities of oyster reefs and vegetated habitats (e.g. saltmarsh, mangroves), with the lack of differences in communities being attributed to shared functional and structural attributes (Geraldi et al. 2009; Martinez-Baena et al. 2022). Subsequently, more pronounced nekton responses may be observable from large-scale restoration efforts, particularly in seascapes dominated by unstructured habitats (Gilby et al. 2018).
As oyster reef restoration within the Port River–Barker Inlet Estuary has been confined to a single site within the estuary, localised characteristics and scale-dependent limitations of our survey design may have limited the detection of nekton community enhancements at our restoration site. The pre-existing structural habitat (remnant pylons) at the restoration site, and its selection for oyster reef restoration, may have inversely affected nekton by attracting natural predators, or functioning as socio-ecological traps, whereby installed structures result in actual or expected catchability enhancements, leading to greater recreational fisheries pressure (Chong et al. 2024). Although not quantified in our study, predators, including dolphins, cormorants, and recreational fishers, were observed at both sites (B. Martin, pers. obs.). Their presence may have influenced species detections by modifying nekton behaviour and population dynamics, including non-lethal predation effects (Kimbro et al. 2014; Pauli and Sih 2017). Similarly, the higher turbidity (poorer water clarity) at the restoration site may have influenced nekton distribution, as turbidity can disrupt predator–prey interactions, modify habitat characteristics, and increase physiological stress and mortality (Kjelland et al. 2015). High turbidity can also influence fish detectability by visual monitoring methods, with sonar or extractive sampling as potential alternatives (Martin et al. 2025b). Within the Port Adelaide catchment, stormwater drainage has been identified as a primary source of suspended particles, with an estimated 26,900 kg year−1 of pollutants being discharged annually (Southfront 2019). Monitoring additional restoration sites, should further oyster reef restoration occur within the estuary, may reduce some of these localised confounding influences and improve the detectability of potential nekton enhancements.
Variations in indicator species abundances underscores the complex responses of nekton communities to oyster reef restoration and broader environmental conditions. For example, following restoration activities, the restoration site in our study was strongly associated with crested oystergobies (C. gobioides), which was likely in response to the addition of recycled shell material used in the restoration substrate. Whereas this goby is non-native to South Australia (Wiltshire et al. 2010), recent studies have found strong habitat associations of that goby species to oyster reefs (Martinez-Baena et al. 2022; Martin et al. 2025c). Many Gobiidae species are reef-residents that use disarticulated oyster shells for nesting sites and refugia, and therefore respond positively to oyster reef restoration (Davenport et al. 2021; Lewis et al. 2021). In comparison, yelloweye mullet (A. forsteri) and western striped grunter (P. octolineatus) are highly mobile and transient fishes, and may benefit from the restoration site only for short durations associated with temporal shifts (i.e. diel, seasonal, ontogenetic) in their movement (Davenport et al. 2021). For A. forsteri, they are known to have undertaken seasonal movement into the upper section of the Port River in winter (Jones et al. 1996). The species A. forsteri is also a detrital feeder and may prefer unstructured habitats for feeding (Martin et al. 2025c), leading to higher abundances at the control site. These examples demonstrate that oyster restoration can have mixed and context-dependent outcomes for nekton species. Determining whether the observed species composition and responses change as the reef develops in future years would provide useful information of long-term outcomes, both in Port Adelaide and other urban waterways.
Considerations for shellfish reef restoration
Urbanised estuaries are complex systems for oyster reef restoration, with site suitability and reef resilience being limited by anthropogenic modification and spatial use (Howie and Bishop 2021). Although restoration initiatives are typically aimed at facilitating habitat-forming species, they may not optimise nekton habitat value (e.g. structure, prey), nor address stressors influencing their populations (e.g. fishing, pollutants, invasive species), which should be assessed as a part of developing restoration baselines (Gilby et al. 2018; Sievers et al. 2024). For example, at least 50 non-native species are established within the Port River–Barker Inlet Estuary (Wiltshire et al. 2010), including sessile marine pests that may compete with native O. angasi and challenge efforts to restore ‘natural’ shellfish reef baselines (Maus et al. 2024). In our study, restoration was associated with non-native Gobiidae presence, particularly C. gobioides. Although they are likely to have benign impacts, their co-occurrence with native gobies including F. lateralis and Arenigobius bifrenatus may result in increasing competition for benthic resources alongside the development of restoring shellfish reef systems (Hammer 2014). Other environmental stressors including sedimentation, stormwater inputs and pollution may similarly affect the capacity of restoration sites to sustain populations of habitat-forming shellfish and associated nekton communities (Gilby et al. 2018; Howie and Bishop 2021). Thus, practitioners should consider a range of abiotic and biotic variables when considering potential restoration suitability, particularly for urbanised estuary systems.
Whether shellfish reefs enhance nekton communities may depend on their capacity to provision ecological functions that modify structural and resource heterogeneity (Gilby et al. 2018). Our results indicated that in urbanised estuaries with artificial structural habitats, small-scale reef restoration can be functionally redundant for nekton communities, at least in the early stages and while reefs occupy a limited spatial footprint. Although not quantified in this study, the reef restoration may already support other beneficial functions such as enhanced biofiltration or macroinvertebrate productivity, which could indirectly benefit nekton communities; however, that would require further evaluation at smaller spatial scales (e.g. Andersson et al. 2023). The strong influence of seasonal and interannual environmental variation on the nekton community demonstrated why practitioners should also monitor abiotic variables when examining restoration outcomes (Baggett et al. 2015). Given the short duration of this study, longer-term monitoring and the installation of additional oyster reef sites is recommended to better understand nekton community dynamics and the role of abiotic variables. As shellfish reef restoration is increasingly being considered for degraded coastal habitats in urban areas, this study highlighted some of the challenges associated with the ecology of modified estuarine settings. Thus, in urbanised coastal settings, multiple, small-scale pilot shellfish reefs should be trialled to evaluate restoration risks and benefits so as to better inform upscaled habitat restoration aimed at long-term nekton enhancements.
Declaration of funding
This research was supported by Flinders University and a Holsworth Wildlife Research Endowment Grant from the Ecological Society of Australia awarded to to the lead author.
Acknowledgements
We thank C. McMahon from the Estuary Care Foundation for providing information about the study site and the volunteers that supported the fieldwork. We acknowledge and pay our respect to the Kaurna people, the Traditional Custodians of the Land and Sea Country from which this research has been obtained.
References
Alleway HK, Connell SD (2015) Loss of an ecological baseline through the eradication of oyster reefs from coastal ecosystems and human memory. Conservation Biology 29(3), 795-804.
| Crossref | Google Scholar | PubMed |
Andersson M, Cheney KL, Porter R, Gilby BL, Mos B (2023) Quantifying clearance rates of restored shellfish reefs using modular baskets. EcoEvoRxiv 2023, version 4 [Preprint, published 8 December 2023].
| Crossref | Google Scholar |
Baggett LP, Powers SP, Brumbaugh RD, Coen LD, DeAngelis BM, Greene JK, Hancock BT, Morlock SM, Allen BL, Breitburg DL, Bushek D, Grabowski JH, Grizzle RE, Grosholz ED, La Peyre MK, Luckenbach MW, McGraw KA, Piehler MF, Westby SR, Zu Ermgassen PSE (2015) Guidelines for evaluating performance of oyster habitat restoration. Restoration Ecology 23(6), 737-745.
| Crossref | Google Scholar |
Baker R, Bilbrey D, Bland A, D’Alonzo F, Ehrmann H, Havard S, Porter Z, Ramsden S, Rodriguez AR (2022) Underwater video as a tool to quantify fish density in complex coastal habitats. Diversity 14(1), 50.
| Crossref | Google Scholar |
Barbier EB (2017) Marine ecosystem services. Current Biology 27(11), R507-R510.
| Crossref | Google Scholar | PubMed |
Barbier EB, Hacker SD, Kennedy C, Koch EW, Stier AC, Silliman BR (2011) The value of estuarine and coastal ecosystem services. Ecological Monographs 81(2), 169-193.
| Crossref | Google Scholar |
Bayraktarov E, Saunders MI, Abdullah S, Mills M, Beher J, Possingham HP, Mumby PJ, Lovelock CE (2016) The cost and feasibility of marine coastal restoration. Ecological Applications 26(4), 1055-1074.
| Crossref | Google Scholar | PubMed |
Beck MW, Brumbaugh RD, Airoldi L, Carranza A, Coen LD, Crawford C, Defeo O, Edgar GJ, Hancock B, Kay MC, Lenihan HS, Luckenbach MW, Toropova CL, Zhang G, Guo X (2011) Oyster reefs at risk and recommendations for conservation, restoration, and management. BioScience 61(2), 107-116.
| Crossref | Google Scholar |
Bersoza Hernández A, Brumbaugh RD, Frederick P, Grizzle R, Luckenbach MW, Peterson CH, Angelini C (2018) Restoring the eastern oyster: how much progress has been made in 53 years? Frontiers in Ecology and the Environment 16(8), 463-471.
| Crossref | Google Scholar |
Booth JG, Casella G, Friedl H, Hobert JP (2003) Negative binomial loglinear mixed models. Statistical Modelling 3(3), 179-191.
| Crossref | Google Scholar |
Bossley MI, Steiner A, Rankin RW, Bejder L (2017) A long-term study of bottlenose dolphins (Tursiops aduncus) in an Australian industrial estuary: increased sightings associated with environmental improvements. Marine Mammal Science 33(1), 277-290.
| Crossref | Google Scholar |
Boström-Einarsson L, Martínez-Baena F, Diggles B, Firby L, McLeod IM (2022) An ecological assessment of Australia’s first community oyster gardens. Ecological Management & Restoration 23(3), 244-251.
| Crossref | Google Scholar |
Bradley M, Sheaves M, Waltham NJ (2023) Urban–industrial seascapes can be abundant and dynamic fish habitat. Frontiers in Marine Science 9, 1034039.
| Crossref | Google Scholar |
Chapman MG, Underwood AJ, Browne MA (2018) An assessment of the current usage of ecological engineering and reconciliation ecology in managing alterations to habitats in urban estuaries. Ecological Engineering 120, 560-573.
| Crossref | Google Scholar |
Chong L, Siders ZA, Lorenzen K, Ahrens RNM, Camp EV (2024) Global synthesis of effects and feedbacks from artificial reefs on socioecological systems in recreational fisheries. Fish and Fisheries 25(2), 303-319.
| Crossref | Google Scholar |
Chuwen BM, Hoeksema SD, Potter IC (2009) Factors influencing the characteristics of the fish faunas in offshore, deeper waters of permanently-open, seasonally-open and normally-closed estuaries. Estuarine, Coastal and Shelf Science 81(3), 279-295.
| Crossref | Google Scholar |
Clarke KR, Somerfield PJ, Chapman MG (2006) On resemblance measures for ecological studies, including taxonomic dissimilarities and a zero-adjusted Bray–Curtis coefficient for denuded assemblages. Journal of Experimental Marine Biology and Ecology 330(1), 55-80.
| Crossref | Google Scholar |
Clifton GA, Dafforn KA, Bishop MJ (2022) The ecological benefits of adding topographic complexity to seawalls vary across estuarine gradients. Ecological Engineering 182, 106735.
| Crossref | Google Scholar |
Connolly R, Herrera C, Rasmussen J, Buelow C, Sievers M, Jinks K, Brown C, Lopez-Marcano S, Sherman C, Martínez-Baena F, Martin B, Baring R, Reeves SE (2024) Estimating enhanced fish production on restored shellfish reefs using automated data collection from underwater videos. Journal of Applied Ecology 61(4), 633-646.
| Crossref | Google Scholar |
Davenport TM, Hughes AR, Zu Ermgassen PSE, Grabowski JH (2021) Recruitment enhancement varies by taxonomic group and oyster reef habitat characteristics. Ecological Applications 31(5), e02340.
| Crossref | Google Scholar | PubMed |
Fitzsimons JA, Branigan S, Gillies CL, Brumbaugh RD, Cheng J, DeAngelis BM, Geselbracht L, Hancock B, Jeffs A, McDonald T, McLeod IM, Pogoda B, Theuerkauf SJ, Thomas M, Westby S, Zu Ermgassen PSE (2020) Restoring shellfish reefs: global guidelines for practitioners and scientists. Conservation Science and Practice 2(6), e198.
| Crossref | Google Scholar |
Fraschetti S, McOwen C, Papa L, Papadopoulou N, Bilan M, Boström C, Capdevila P, Carreiro-Silva M, Carugati L, Cebrian E, Coll M, Dailianis T, Danovaro R, De Leo F, Fiorentino D, Gagnon K, Gambi C, Garrabou J, Gerovasileiou V, Hereu B, Kipson S, Kotta J, Ledoux J-B, Linares C, Martin J, Medrano A, Montero-Serra I, Morato T, Pusceddu A, Sevastou K, Smith CJ, Verdura J, Guarnieri G (2021) Where is more important than how in coastal and marine ecosystems restoration. Frontiers in Marine Science 8, 626843.
| Crossref | Google Scholar |
Geraldi NR, Powers SP, Heck KL, Cebrian J (2009) Can habitat restoration be redundant? Response of mobile fishes and crustaceans to oyster reef restoration in marsh tidal creeks. Marine Ecology Progress Series 389, 171-180.
| Crossref | Google Scholar |
Gilby BL, Olds AD, Peterson CH, Connolly RM, Voss CM, Bishop MJ, Elliott M, Grabowski JH, Ortodossi NL, Schlacher TA (2018) Maximizing the benefits of oyster reef restoration for finfish and their fisheries. Fish and Fisheries 19(5), 931-947.
| Crossref | Google Scholar |
Gilby BL, Olds AD, Chapman S, Goodridge Gaines LA, Henderson CJ, Ortodossi NL, Didderen K, Lengkeek W, van der Heide T, Schlacher TA (2021) Attraction versus production in restoration: spatial and habitat effects of shellfish reefs for fish in coastal seascapes. Restoration Ecology 29(7), e13413.
| Crossref | Google Scholar |
Gillies CL, Castine SA, Alleway HK, Crawford C, Fitzsimons JA, Hancock B, Koch P, McAfee D, McLeod IM, Zu Ermgassen PSE (2020) Conservation status of the oyster reef ecosystem of southern and eastern Australia. Global Ecology and Conservation 22, e00988.
| Crossref | Google Scholar |
Grabowski JH, Brumbaugh RD, Conrad RF, Keeler AG, Opaluch JJ, Peterson CH, Piehler MF, Powers SP, Smyth AR (2012) Economic valuation of ecosystem services provided by oyster reefs. BioScience 62(10), 900-909.
| Crossref | Google Scholar |
Hale R, Swearer SE (2017) When good animals love bad restored habitats: how maladaptive habitat selection can constrain restoration. Journal of Applied Ecology 54(5), 1478-1486.
| Crossref | Google Scholar |
Halpern BS, Walbridge S, Selkoe KA, Kappel CV, Micheli F, D’Agrosa C, Bruno JF, Casey KS, Ebert C, Fox HE, Fujita R, Heinemann D, Lenihan HS, Madin EM, Perry MT, Selig ER, Spalding M, Steneck R, Watson R (2008) A global map of human impact on marine ecosystems. Science 319(5865), 948-952.
| Crossref | Google Scholar | PubMed |
Hammer MP (2014) Range extensions for four estuarine gobies (Pisces: Gobiidae) in southern Australia: historically overlooked native taxa or recent arrivals? Transactions of the Royal Society of South Australia 130(2), 187-196.
| Crossref | Google Scholar |
Harrison TD, Whitfield AK (2021) A global assessment of fish estuary associations: a numerical approach to assessing estuary-associated fish functional guilds. Fish and Fisheries 22(5), 874-898.
| Crossref | Google Scholar |
Howie AH, Bishop MJ (2021) Contemporary oyster reef restoration: responding to a changing world. Frontiers in Ecology and Evolution 9, 689915.
| Crossref | Google Scholar |
Howie AH, Reeves SE, Gillies CL, Bishop MJ (2024) Integration of social data into restoration suitability modelling for oyster reefs. Ecological Indicators 158, 111531.
| Crossref | Google Scholar |
Jackson G, Jones GK (1999) Spatial and temporal variation in nearshore fish and macroinvertebrate assemblages from a temperate Australian estuary over a decade. Marine Ecology Progress Series 182, 253-268.
| Crossref | Google Scholar |
Jones GK, Baker JL, Edyvane K, Wright GJ (1996) Nearshore fish community of the Port River–Barker Inlet Estuary, South Australia. I. Effect of thermal effluent on the fish community structure, and distribution and growth of economically important fish species. Marine and Freshwater Research 47(6), 785-799.
| Crossref | Google Scholar |
Kimbro DL, Byers JE, Grabowski JH, Hughes AR, Piehler MF (2014) The biogeography of trophic cascades on US oyster reefs. Ecology Letters 17(7), 845-854.
| Crossref | Google Scholar | PubMed |
Kjelland ME, Woodley CM, Swannack TM, Smith DL (2015) A review of the potential effects of suspended sediment on fishes: potential dredging-related physiological, behavioral, and transgenerational implications. Environment Systems and Decisions 35(3), 334-350.
| Crossref | Google Scholar |
Lewis DM, Durham KE, Walters LJ, Cook GS (2021) A resident fish guild as a higher trophic level indicator of oyster reef restoration success. Sustainability 13(23), 13004.
| Crossref | Google Scholar |
Lotze HK, Lenihan HS, Bourque BJ, Bradbury RH, Cooke RG, Kay MC, Kidwell SM, Kirby MX, Peterson CH, Jackson JB (2006) Depletion, degradation, and recovery potential of estuaries and coastal seas. Science 312(5781), 1806-1809.
| Crossref | Google Scholar | PubMed |
Mahoney PC, Bishop MJ (2017) Assessing risk of estuarine ecosystem collapse. Ocean & Coastal Management 140, 46-58.
| Crossref | Google Scholar |
Martin B, Huveneers C, Reeves S, Baring R (2025a) Reviving shellfish reef socio-ecological histories for modern management and restoration. Ocean & Coastal Management 261, 107540.
| Crossref | Google Scholar |
Martin B, Huveneers C, Reeves S, Baring R (2025b) Fishing for more than shellfish: a systematic review of fish community monitoring of shellfish reefs. Restoration Ecology 33(1), e14323.
| Crossref | Google Scholar |
Martin B, Huveneers C, Reeves S, Baring R (2025c) Mangrove-associated Pacific oysters (Magallana gigas) influence estuarine biodiversity. NeoBiota 98, 1-26.
| Crossref | Google Scholar |
Martinez-Baena F, Lanham BS, McLeod IM, Taylor MD, McOrrie S, Luongo A, Bishop MJ (2022) Remnant oyster reefs as fish habitat within the estuarine seascape. Marine Environmental Research 179, 105675.
| Crossref | Google Scholar | PubMed |
Martínez-García MF, Ruesink JL, Grijalva-Chon JM, Lodeiros C, Arreola-Lizárraga JA, De la Re-Vega E, Varela-Romero A, Chávez-Villalba J (2022) Socioecological factors related to aquaculture introductions and production of Pacific oysters (Crassostrea gigas) worldwide. Reviews in Aquaculture 14(2), 613-629.
| Crossref | Google Scholar |
Maus C, Cottingham A, Bossie A, Tweedley JR (2024) Assessing the efficacy of a bouchot-style shellfish reef as a restoration option in a temperate estuary. Journal of Marine Science and Engineering 12(1), 87.
| Crossref | Google Scholar |
McAfee D, Connell SD (2020) Cuing oyster recruitment with shell and rock: implications for timing reef restoration. Restoration Ecology 28(3), 506-511.
| Crossref | Google Scholar |
McAfee D, McLeod IM, Alleway HK, Bishop MJ, Branigan S, Connell SD, Copeland C, Crawford CM, Diggles BK, Fitzsimons JA, Gilby BL, Hamer P, Hancock B, Pearce R, Russell K, Gillies CL (2022) Turning a lost reef ecosystem into a national restoration program. Conservation Biology 36(6), e13958.
| Crossref | Google Scholar | PubMed |
Morris RL, Campbell-Hooper E, Waters E, Bishop MJ, Lovelock CE, Lowe RJ, Strain EMA, Boon P, Boxshall A, Browne NK, Carley JT, Fest BJ, Fraser MW, Ghisalberti M, Gillanders BM, Kendrick GA, Konlechner TM, Mayer-Pinto M, Pomeroy AWM, Rogers AA, Simpson V, Van Rooijen AA, Waltham NJ, Swearer SE (2024) Current extent and future opportunities for living shorelines in Australia. Science of the Total Environment 917, 170363.
| Crossref | Google Scholar | PubMed |
Pauli B, Sih A (2017) Behavioural responses to human-induced change: why fishing should not be ignored. Evolutionary Applications 10(3), 231-240.
| Crossref | Google Scholar |
Potter IC, Veale L, Tweedley JR, Clarke KR (2016) Decadal changes in the ichthyofauna of a eutrophic estuary following a remedial engineering modification and subsequent environmental shifts. Estuarine, Coastal and Shelf Science 181, 345-363.
| Crossref | Google Scholar |
Ray NE, Fulweiler RW (2021) Meta-analysis of oyster impacts on coastal biogeochemistry. Nature Sustainability 4(3), 261-269.
| Crossref | Google Scholar |
Richardson MA, Zhang Y, Connolly RM, Gillies CL, McDougall C (2022) Some like it hot: the ecology, ecosystem benefits and restoration potential of oyster reefs in tropical waters. Frontiers in Marine Science 9, 873768.
| Crossref | Google Scholar |
Roberts DW, Roberts MDW (2016) Package ‘labdsv’. Ordination and Multivariate 775, 1-68.
| Google Scholar |
Saunders MI, Doropoulos C, Bayraktarov E, Babcock RC, Gorman D, Eger AM, Vozzo ML, Gillies CL, Vanderklift MA, Steven ADL, Bustamante RH, Silliman BR (2020) Bright spots in coastal marine ecosystem restoration. Current Biology 30(24), R1500-R1510.
| Crossref | Google Scholar | PubMed |
Searles AR, Gipson EE, Walters LJ, Cook GS (2022) Oyster reef restoration facilitates the recovery of macroinvertebrate abundance, diversity, and composition in estuarine communities. Scientific Reports 12(1), 8163.
| Crossref | Google Scholar | PubMed |
Sievers M, Connolly RM, Finlayson KA, Kitchingman ME, Ostrowski A, Pearson RM, Turschwell MP, Adame MF, Bugnot AB, Ditria E, Hale R, Silliman BR, Swearer SE, Valdez SR, Brown CJ (2024) Enhanced but highly variable biodiversity outcomes from coastal restoration: a global synthesis. One Earth 7(4), 623-634.
| Crossref | Google Scholar |
Smith RS, Cheng SL, Castorani MCN (2023) Meta-analysis of ecosystem services associated with oyster restoration. Conservation Biology 37(1), e13966.
| Crossref | Google Scholar | PubMed |
Snelgrove PVR, Thrush SF, Wall DH, Norkko A (2014) Real world biodiversity – ecosystem functioning: a seafloor perspective. Trends in Ecology & Evolution 29(7), 398-405.
| Crossref | Google Scholar | PubMed |
Taylor MD, Suthers IM (2021) The socio-ecological system of urban fisheries in estuaries. Estuaries and Coasts 44(7), 1744-1751.
| Crossref | Google Scholar |
Thurstan R, McCormick H, Preston J, Ashton E, Bennema F, Bratoš Cetinić A, Brown J, Cameron T, Da Costa F, Donnan D, Ewers C, Fortibuoni T, Galimany E, Giovanardi O, Grancher R, Grech D, Hayden-Hughes M, Helmer L, Jensen K, Juanes JA, Latchford J, Moore ABM, Moutopoulos D, Nielsen P, von Nordheim H, Ondiviela B, Peter C, Pogoda B, Poulsen B, Pouvreau S, Roberts C, Scherer C, Smaal A, Smyth D, Strand Å, Theodorou J, Zu Ermgassen PSE (2024) Records reveal the vast historical extent of European oyster reef ecosystems. Nature Sustainability 7, 1719-1729.
| Crossref | Google Scholar |
Todd PA, Heery EC, Loke LHL, Thurstan RH, Kotze DJ, Swan C (2019) Towards an urban marine ecology: characterizing the drivers, patterns and processes of marine ecosystems in coastal cities. Oikos 128(9), 1215-1242.
| Crossref | Google Scholar |
Ushiama S, Mayer-Pinto M, Bugnot AB, Johnston EL, Dafforn KA (2019) Eco-engineering increases habitat availability and utilisation of seawalls by fish. Ecological Engineering 138, 403-411.
| Crossref | Google Scholar |
Walters LJ, Roddenberry A, Crandall C, Wayles J, Donnelly M, Barry SC, Clark MW, Escandell O, Hansen JC, Laakkonen K, Sacks PE (2022) The use of non-plastic materials for oyster reef and shoreline restoration: understanding what is needed and where the field is headed. Sustainability 14(13), 8055.
| Crossref | Google Scholar |
Whitmarsh SK, Fairweather PG, Huveneers C (2016) What is Big BRUVver up to? Methods and uses of baited underwater video. Reviews in Fish Biology and Fisheries 27(1), 53-73.
| Crossref | Google Scholar |
Wiltshire K, Rowling KP, Deveney MR (2010) Introduced marine species in South Australia: a review of records and distribution mapping. SARDI Publication Number F2010/000305-1, SARDI Research Report Series Number 468. (SARDI Aquatic Sciences: Adelaide, SA, Australia) Available at https://pir.sa.gov.au/__data/assets/pdf_file/0003/232068/No_468_Introduced_marine_species_in_South_Australia_a_review_of_records_and_distribution_mapping.pdf
Zu Ermgassen PSE, Thurstan RH, Corrales J, Alleway H, Carranza A, Dankers N, DeAngelis B, Hancock B, Kent F, McLeod I, Pogoda B, Liu Q, Sanderson WG (2020) The benefits of bivalve reef restoration: a global synthesis of underrepresented species. Aquatic Conservation: Marine and Freshwater Ecosystems 30(11), 2050-2065.
| Crossref | Google Scholar |