Investigation of Bara-Baruga (mangrove) ecosystem recovery after a hail storm in Boambee Creek, Gumbaynggirr, NSW
Kirsten Benkendorff
A
B
C
D
E
Abstract
Understanding the causes of decline in mangrove forests requires on-ground investigations, which are best conducted in collaboration with Traditional Knowledge Holders.
To compare the ecological communities and contaminants in an unhealthy and healthy mangrove forest in Boambee Creek.
Nearmap Imagery identified the timing of mangrove die-back. On-site surveys included quadrat counts of pneumatophores, seeds and seedlings, timed searches and benthic cores for invertebrates. Samples were collected for nutrient analysis of water, elemental analysis of sediment and gas chromatography–mass spectrometry for volatile contaminants in pneumatophores.
A hail storm in 2021 coincided with the mangrove die-back. Chemical analysis showed ongoing eutrophication, and high concentrations of sediment mercury, carbon disulfide and toxic hydrocarbons in pneumatophores at the unhealthy site. Invertebrate species richness and density of pneumatophores, seeds and seedlings were significantly lower at the unhealthy site. Infauna richness decreased at the unhealthy site compared with an historical baseline in 2006, but did not change at the healthy site.
These surveys confirmed contamination and a loss of ecological integrity at the unhealthy mangrove site.
Intervention may be required at the unhealthy site to assist natural recovery and restoration of mangrove ecosystem services.
Keywords: benthic invertebrates, contaminants, cultural values, eutrophication, Indigenous research, infauna, mangrove die-back, multiple stressors.
Introduction
At a meeting of world mangrove experts in Australia in 2007, there was unanimous agreement that ‘we face the prospect of a world deprived of the services offered by mangrove ecosystems, perhaps within the next 100 years’ (Duke et al. 2007, p. 41). It was estimated that, worldwide, mangrove had been disappearing at a rate of 1–2% per year. Just over 15 years on, examples of the loss and die-back of mangrove forests are still being reported at an unprecedented rate. Mangrove forests are affected by clearing for aquaculture, urbanisation and coastal landfill, pollution, upstream land use and changes in hydrology (Goldberg et al. 2020), as well as extreme weather events and climate change (Sippo et al. 2018). The fragmentation and reduction in total area of mangrove habitat is a threat to the long-term survival of these important ecosystems and their associated ecosystem services.
Mangrove ecosystems provide essential ecosystem services across all four areas of utility, which are defined in the Millennium Ecosystem Assessment framework (Percy et al. 2005, p. 4). (1) ‘Provisioning’: mangroves provide food, wood, construction and medical resources for many communities who directly rely on their resources for their subsistence or livelihoods (Friess 2016). (2) ‘Supporting’: mangroves are primary producers that facilitate nutrient cycling (Alongi 2018), carbon sequestration (Alongi 2012) and sediment accretion (Woodroffe et al. 2016); they also provide habitat for many invertebrate and fish, including nursery grounds for commercially and recreationally important species (Nagelkerken et al. 2008). (3) ‘Regulating’: mangroves protect shorelines and prevent coastal erosion during floods, storm surges and typhoons (Alongi 2008; Barbier 2016). (4) ‘Cultural’: mangroves support recreational activities, tourism and traditional cultural practices in communities around the world (Bimrah et al. 2022), supporting psychological well-being and spiritual connection to Country (Friess 2016).
The Aboriginal and Torres Strait Islander peoples of Australia have knowledge systems that reflect a deep understanding of inter-connectedness between people and the environment (Nursey-Bray 2011; Prober et al. 2011; Hill et al. 2012). Indigenous Ecological Knowledge is accumulated over time, evolving over generations, through interactions with the environment. The Gumbaynggirr people have occupied the coastal area around Coffs Harbour for many thousands of years and have always depended on the ocean for food and other resources. Traditional Knowledge about how and where to harvest shellfish and catch fish, and what bait to use, is still applied today (Arrawarra Sharing Culture 2013). The Gumbaynggirr people were known as ‘sharing’ people because of the vast abundance of resources in their local environment, which they commonly shared with other Nations. There are a number of recognised clan groups within Gumbaynggirr Nation with a long history of connection to the land and sea. However, since the 1870s, they have been forced to co-exist with European settlers (Thomas 2013), which has affected their cultural traditions, access to and stewardship of the land. Land cleared for agriculture and urban development has not only directly reduced access to terrestrial resources in this region, but also indirectly affects water quality (Conrad et al. 2021a; Laicher et al. 2022) and threatens access to aquatic resources.
The grey mangrove, Avicennia marina, known as ‘Bara-Baruga’ in Gumbaynggirr language, dominates the saline tidal areas where sediment accumulates in estuaries such as Boambee Creek. Traditionally, the area around Boambee Creek was considered especially rich in sources of food, including fish, oysters and pipis (Thomas 2013). It is now a popular recreation area for fishing, swimming and kayaking across the community. In October 2021, this area near Coffs Harbour was hit by an intense hail storm. This resulted in defoliation of many trees in the area, including the mangroves along Boambee Creek (Fig. 1). However, the lack of recovery over 1 year later at the main impact site, which is in direct line of the runway into Coffs Harbour airport, raised questions as to whether there was another underlying problem and whether it was possible to restore the mangrove ecosystem. The mangrove die-back is very distressing to the Gumbaynggirr people who have a rich relationship with the trees and the lands to which they belong to. This connection with the Bara-Baruga (mangroves) is a spiritual connection; by way of traditional Lore the Bara-Baruga is an extension of themselves and they have a responsibility to them.
Site map of Boambee Creek study sites within Gumbaynggirr Nation on the New South Wales (NSW) coast of Australia, showing changes over time at two sites: Site 1 (unhealthy) underwent a major die-back after the 2021 hailstorm, with slow recovery; Site 2 (healthy control) was partially defoliated by the hailstorm, but had fully recovered by 2023. Source Nearmap Imagery 2023 (see https://www.nearmap.com/au/products/imagery).
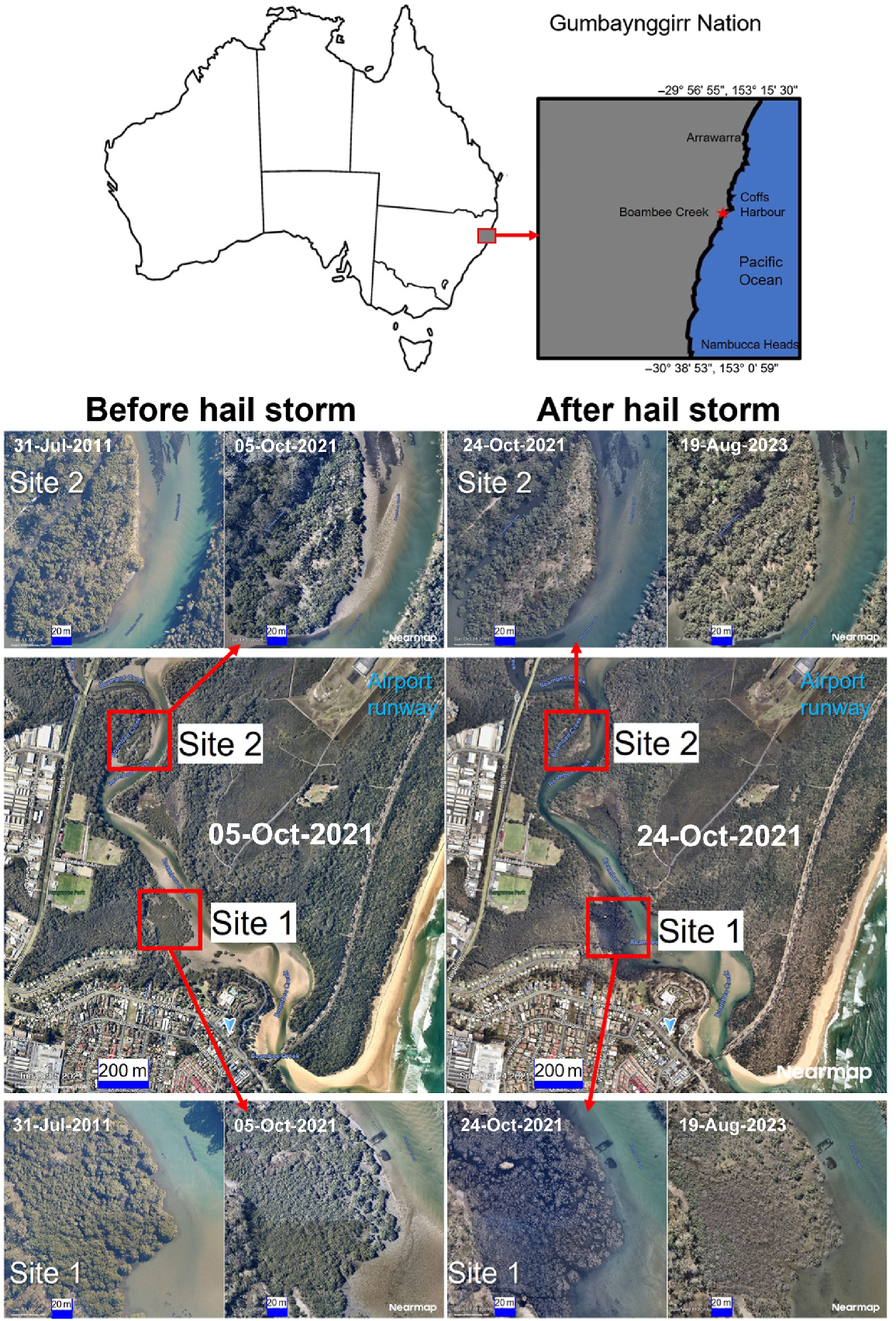
Effective restoration of mangrove habitat in die-back areas depends on what the original stressors are and whether these are ongoing. Chemical pollution can be a significant contributor to mangrove decline (Bayen 2012). A range of toxic metals and organic pollutants have been recorded from mangrove ecosystems around the world (Bayen 2012; Zhang et al. 2014; Fusi et al. 2016; Araújo et al. 2020). The fine-grained sediment in mangrove forests can sequester trace metals and other contaminants, which can then be uptaken by organisms in the mangrove community (Lewis et al. 2011). Mangroves appear to be tolerant to high concentrations of pollutants and can bioaccumulate some contaminants (Lewis et al. 2011; Kastury et al. 2023). Specifically, A. marina has been found to uptake trace metals into its roots, branches and leaves (Usman et al. 2013; Nath et al. 2014; Kastury et al. 2023) and polyaromatic hydrocarbons into its rhizosphere (Jia et al. 2016). However, exposure to heavy metals can negatively affect seedling emergence and growth (MacFarlane and Burchett 2002) and contamination by oil has been shown to adversely affect CO2 exchange and photosynthetic performance of A. marina (Jia et al. 2016).
Research partnerships between community groups and scientists can facilitate the collection of information by using complementary methods for co-management of marine ecosystems (Kaiser et al. 2019). Coastal indigenous communities play an important role in managing local ecosystems to ensure ecological and cultural integrity. Indigenous Rangers are often engaged to provide ‘eyes on country’ and undertake coastal monitoring. They can rapidly monitor changes in marine resources, using qualitative approaches based on Indigenous Ecological Knowledge (Kaiser et al. 2019), which is adaptive and built-up over long histories of practice. Scientists can help by transferring skills for quantitative data collection and standardised monitoring methods (Dobbs et al. 2016; Fredericks et al. 2025) for assessment of environmental impacts and changes in ecosystem health. The Gumbaynggirr Rangers are responsible for looking after the cultural and environmental values within the proposed Gaagal Wajaarr Sea Country Indigenous Protected Area. They have close connections to the local elders and collaborate with research scientists to apply culturally appropriate ways to investigate and support local Aboriginal people to heal and care for their country. The aim of this study was to apply traditional science, with Indigenous participation, to investigate the causes, ecological impacts and restoration potential of the mangrove die-off area in Boambee Creek. This two-way knowledge sharing provides an important basis for future co-designed research and management decisions.
Methods
Preliminary consultation and site inspections
The mangrove die-back on Boambee Creek was first noticed by one of the researchers as a passenger on a commercial flight on 27 March 2023, as the plane came into land at Coffs Harbour airport. The NSW Environmental Protection Agency (EPA) and NSW Department of Primary Industries (DPI), Fisheries were contacted, and by using high-quality aerial Nearmap Imagery (see https://www.nearmap.com/au), it was established that the timing of the mangrove die-off aligned with a hail storm event that hit the Coffs Harbour region in October 2021 (Fig. 1). Nearmap Imagery 2023 was then used to investigate recovery in the mangrove ecosystem at the main die-back site (Site 1, unhealthy) and a control site (Site 2, healthy) ~400 m upstream, which was partially defoliated during the hailstorm (Fig. 1, Supplementary Table S1a).
The Gumbaynggirr Rangers were contacted about the mangrove die-off and invited to participate in the investigation. Preliminary on-ground site inspections were undertaken independently by the Rangers and SCU researchers during April and May 2023, which confirmed that the die-back was extensive stretching from the water ~80 m landward and at least 60 m across. The ‘control’ site ~400 m upstream was inspected for comparison. No changes in hydrology were apparent, with both mangrove sites experiencing normal tidal cycles of inundation at high tide and exposure at low tide.
The Gumbaynggirr Rangers consulted with community Elders and obtained permission to survey the site. On 30 June 2023, a joint site inspection of the mangrove die-back (Site 1) was undertaken by the Gumbaynggirr Rangers and SCU researchers to share knowledge and co-design the field sampling program. Site observations were formally recorded (Table S1b) and some samples were collected for analysis. After further discussion between the Gumbaynggirr Rangers and Traditional Owners, it was agreed that culturally sensitive resources would not be targeted in the surveys. The final field sampling design (see Fig. 2) was refined during meetings at the Australian Marine Science Association Conference on the Gold Coast (AMSA 2023) and shared with team at subsequent meetings at the National Marine Science Centre (NMSC), Coffs Harbour.
Water quality
The pH, dissolved oxygen, salinity and temperature were recorded using an in situ Aquatroll 500 Multiparameter Sonde at both sites (Site 1, 16 and 24 November, and Site 2, 23 November). We measured six replicate pools on the lower shore and dug six holes within the mangrove forest, to measure ‘pore water’ from emersed sediment (Fig. 2). In addition, at Site 1 (unhealthy site), we took six replicate readings from the red-coloured water (Table S1, Supplementary Fig. S3), that was observed along the main drainage channel through the mangroves (Fig. 2).
On several site visits to the Site 1 in 2023, we collected water samples and tested these on a La Motte AquaSpin saltwater disc for alkalinity, nitrate, nitrite, ammonia and phosphorus (Table 1). At least two independent samples from the creek and pore water were tested on three occasions, with a further four samples tested from the red drainage channel on 23 November (Table 1). The nutrient readings were compared with Australian and New Zealand Environment and Conservation Council and Agriculture and Resource Management Council of Australia and New Zealand (2000) environmental trigger values for estuarine waters.
Date | Source | n | Alkalinity | Phosphate | Ammonia | Nitrite | Nitrate | |
---|---|---|---|---|---|---|---|---|
May | Creek | 2 | 248.5 ± 184.6 | 1.85 ± 0.07 | 1.15 ± 1.06 | 0.4 ± 0.57 | <1 | |
Pore water | 2 | 106 ± 2.8 | 1 ± 0.14 | 0.25 ± 0.28 | 0.125 ± 0.07 | 0.25 ± 0.71 | ||
September | Creek | 2 | 75 ± 38.2 | 0.55 ± 0.07 | 0.2 ± 0.14 | 0.05 ± 0.07 | 0.5 ± 0.71 | |
Pore water | 2 | 155 ± 38.2 | 0.2 ± 0.9 | 0.7 ± 0.28 | 0.05 ± 0.14 | 0.5 ± 0 | ||
November | Creek | 3 | 283 ± 89.5 | 0.63 ± 0.51 | 0.27 ± 0.21 | 0.2 ± 0.17 | <1 | |
Pore water | 2 | 354.5 ± 27.6 | 0.35 ± 0.35 | 0.55 ± 0.78 | <0.1 | <1 | ||
Red channel | 4 | 57.1 ± 34.4 | 0.075 ± 0.09 | 0.025 ± 0.05 | <0.1 | 1 ± 0 | ||
Default trigger values | 0.03 mg L−1 (TP) | 0.015 mg L−1 | 0.015 mg L−1 | 0.015 mg L−1 |
The environmental trigger values for nutrient in estuarine waters (Australian and New Zealand Environment and Conservation Council and Agriculture and Resource Management Council of Australia and New Zealand 2000) are provided in the bottom row for comparison. Total phosphorus (TP) is used as a guide for phosphate. <, not detected at the reporting limit for this instrument.
Chemical analysis
Four replicate 100-g sediment samples were haphazardly collected in ziplock plastic bags from the Site 1 and the healthy control Site 2 (Fig. 2) in May, 2023 and placed in the freezer at −20°C prior to transporting to the NATA accredited Southern Cross University Environmental Analysis Laboratory for contaminated soil assessment. The samples were digested in nitric acid and hydrochloric acid on a hot block at 120°C prior to analysis on an inductively coupled plasma–mass spectrometer (ICP-MS, American Public Health Association Standard Methods: 3125, see Lipps et al. 2022).
Three replicate samples of the mangrove roots and pneumatophores were collected into airtight 25-mL amber jars from both sites (Fig. 2) in June–July 2023. These samples were transported to the Southern Cross University Analytical Research Faculty for analysis by head space–gas chromatography–mass spectrometry (HS-GC-MS). An Agilent 6890 Gas Chromatograph fitted with Gerstel MPS2 headspace analyser and coupled to an Agilent 5973 Mas selective detector was used. A Zebron ZB624 capillary column (30-m length, 320-mm internal diameter, 1.8-μm film thickness) was installed. The GC run conditions employed an inlet temperature of 140°C with a 5:1 split ratio. The carrier gas used was instrument grade helium with a flow rate of 1.5 mL min−1 and column velocity of 45 cm s−1. The column oven program was 35°C, initially held for 20 min, followed by a temperature ramp rate of 10°C min−1 to 240°C, which was then held for 20 min. Total run time was 60 min. The headspace analyser employed sample heating at 105°C for 45 min and a syringe temperature of 110°C. The mass spectrum detector (MSD) used a fragmentation electron ionisation (EI) of 70 eV.
The volatile compounds were identified by matching the mass spectra against the ADAMS, NIST 14.L and Wiley databases, with accepted matches based on a minimum quality match of 80. The areas under each peak in the GC were integrated to provide the percentage composition. Mangrove root and pneumatophore samples were analysed on 3 October 2023, whereas sediment samples were analysed on 30 October after storage at room temperature.
Mangrove surveys
On 5 September 2023 at both the healthy control and mangrove die-back sites, four replicate transects were laid out perpendicular to the shore for 30 m from the edge of the water (Fig. 2). Along each transect, a 0.25-m2 quadrat was placed every 5 m and the number of pneumatophores and seeds was counted within each quadrat.
A follow-up survey was undertaken of the number of germinated seedlings at each site. The healthy control (Site 2) was surveyed on 21 November 2023 and the unhealthy die-back (Site 1) was surveyed a few days later, on 24 November 2023. In these surveys, six shore normal transects were laid out and seedlings were counted in 0.25-m2 quadrats every 5 m (Fig. 2).
Invertebrate surveys
At each site, six replicate, 5-min timed search surveys were undertaken to identify invertebrates, in non-overlapping sections of the mangrove forest (Fig. 2). During these surveys, flying insects and epifauna (>1 mm) were recorded on the surface of the substrate, mangrove trunks, pneumatophores, living branches and under fallen branches. Individual counts were tallied or estimated for >50. Semi-quantitative abundance ranks were assigned for analysis, with rare = 1–3; uncommon = 4–10; moderately common = 10–49; common = 50–499 and abundant ≥ 500 individuals per 5-min survey. These surveys were undertaken under healthy mangroves at the control Site 2 on 21 November 2023 and under unhealthy mangroves at Site 1 on 24 November 2023.
Benthic cores were also used to sample the infauna at both sites. These surveys replicated the design used in previously unpublished baseline surveys undertaken at the same sites on 14 November 2006. The cores were taken using a 15-cm diameter PVC pipe to 20-cm depth. Sixteen cores were taken along shore normal transects between 0.3 and −0.7 m below mean low water level (Fig. 2). The cores were collected into calico bags then sieved on site by using a 1-mm sieve and bilge pump. Organisms collected on the sieve were washed into ziplock bags and preserved in 70% ethanol for examination under a dissecting microscope. To ensure comparable identification between the 2006 and 2023 data, taxa were identified to family level only.
Laboratory experiment with soldier crabs
An experiment was conducted using soldier crabs (Mictyris longicarpus) in the flow-through seawater system at the National Marine Science Centre (NMSC), Coffs Harbour, New South Wales (NSW). Six experimental tanks were constructed using 50-L plastic containers (length × width × height, 570 × 356 × 328 mm, Fig. 3), modified by drilling four holes through the base and installing flyscreen netting to prevent sediment from leaking out. The containers were filled halfway with clean sand from Charlesworth Bay, NSW, and a gentle slope was constructed (15–20°, Fig. 3). To allow for daily tidal changes, two 20-mm-diameter PVC pipelines were cut to size and screwed into the drainage valve to adjust for low (80 mm) and high (140 mm) tide.
Experimental set-up for the laboratory experiment with Mictyris longicarpus. Six experimental tanks were established on a flow-through seawater system with two outlets adjusted for low and high tidal cycles. All tanks contained a slope of clean sediment and the treatment tanks were topped with a 2-cm layer of sediment collected from Boambee Site 1 (unhealthy sediment). Five crabs were randomly allocated to each tank then observed for burrowing time, crab holes and sediment balls.
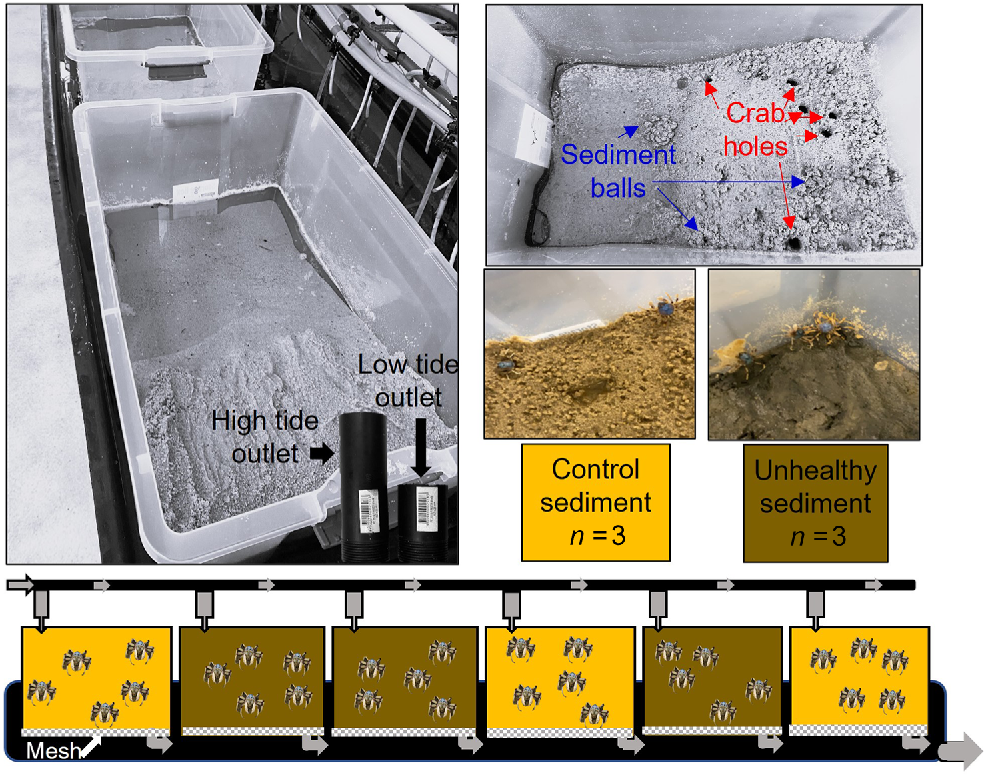
Mictyris longicarpus was collected from the sand flats ~100 m downstream of Site 1 in Boambee Creek at a low tide. In total, 30 crabs were hand netted as they emerged from their burrows. They were placed in a bucket with a small amount of sediment and transported back to NMSC, where they were placed in the experimental tanks (five per container, Fig. 3). The crabs were allowed to acclimatise for 3 weeks with 6-h high-tide cycles in the light. Observations of crab activity, holes and sediment balls (Fig. 3) were recorded before and after tidal change.
After acclimatisation, the crabs from all tanks were removed and temporarily held in separate tanks. A 2-cm layer of sediment, collected from under the unhealthy mangroves at Site 1 Boambee Creek, was placed on top of the control sediment in three randomly assigned experimental tanks (Fig. 3). Five crabs were then randomly selected and returned to each of the experimental tanks. The behaviour was observed and the time taken to burrow into the sediment was recorded with a stopwatch. This process was repeated for each of the treatment and control tanks. An Aquatroll 500 was used to measure temperature, salinity and pH at the beginning and completion of the experiment.
Statistical analysis
Statistical analyses were undertaken in Primer (ver. 7, see https://www.primer-e.com/our-software/) + PERMANOVA (Anderson et al. 2008). For all univariate analyses, Euclidean distance similarity matrices were generated and 9999 permutations were run on the raw data. To test the difference in physicochemical water properties, a two-factor nested PERMANOVA was run for ‘Site’ and ‘Location’ (low shore pool, high shore pore water, and the red channel at Site 1). Post hoc pairwise tests established which locations were different within sites. One-factor PERMANOVA was used to compare the difference in metal and metalloid concentrations between the two sites. A multivariate analysis was also run on the normalised elemental data. A principal coordinate ordination plot (PCO) was generated to illustrate differences between sites, with vector overlay based on Pearson’s correlation (>0.8).
Differences in the density of pneumatophores, mangrove seedlings and seeds were investigated in separate two-factor PERMANOVAs, to compare ‘Site’ and ‘Distance from shore’. Significant interactions were investigated using post hoc tests to compare distance from shore, within sites. Mean species richness from the timed-search surveys for invertebrates was compared between sites by using a one-factor PERMANOVA. The community composition was analysed using a Bray–Curtis similarity matrix from the rank abundance data. A PCO plot was generated with vector overlay (r > 0.8) to visualise the main species contributing to differences between sites.
To investigate differences in benthic infauna from core samples between the two sites over time (2006 v. 2023), two-factor PERMANOVAs were used to compare mean species richness, abundance, diversity (Shannons H-index) and community composition. Univariate analyses used Euclidean distance, whereas the community composition was tested using a Bray–Curtis similarity matrix. Pair-wise post hoc tests compared the change over time within each site. A bootstrap non-metric multidimensional scaling (nMDS) plot was used to illustrate the differences in community composition between years and sites.
In the laboratory experiment with solider crabs, the mean burrowing time was calculated for the five crabs within each tank and the means from three replicate tanks per treatment were used in the analysis. A two-factor PERMANOVA was used to compare differences in mean burrowing time ‘before and after’ sediment manipulation and between ‘control and unhealthy’ sediment tanks, after manipulation.
Results
Water quality
Assessment of physicochemical properties confirmed that both sites were within the expected range for lower estuary mangrove sites (Fig. 4). Comparison of the physicochemical properties of the water in shallow pools on the edge of the mangroves and pore water from among the mangroves showed similar salinity, but lower percentage of dissolved oxygen (%DO) and pH in the pore water at both sites (Fig. 4). The pore water also tended to have a lower temperature, particularly at Site 2 (Fig. 4b). PERMANOVA showed no significant (P > 0.05) differences between the two sites for any of these parameters. However, significant (P < 0.05, Table 2) differences were detected among locations within sites. DO saturation and pH were confirmed to be significantly lower in pore water within the mangroves than in the shore pools at both sites (Fig. 4b, c). At Site 1, the water in the red channel had significantly lower salinity (<5 mg L−1, Fig. 4a), confirming freshwater input and high pH (>9, Fig. 4c). Turbidity was compared only for the shore pools along the creek between sites because of disturbance digging the pore water holes. Mean turbidity was twice as high at Site 1 (414 ± 570 NTU) as Site 2 (204 ± 344 NTU), but owing to high variation within sites, there was no significant (P > 0.05) difference between the two sites (Table 2).
Comparison of the physicochemical properties in shallow pools on the shore and pore water from holes in the sediment among healthy (Site 2) and unhealthy (Site 1) mangroves in Boambee Creek, and along a freshwater drainage channel with red water at the Site 1; (a) salinity, (b) percentage of dissolved oxygen saturation, (c) pH and (d) water temperature recorded with an Aquatroll 500. There was no significant difference between the sites. Different letters above the bars indicate a statistically significant difference between the groups from pair-wise post hoc analyses within sites; upper-case letters are used for significant differences within Site 2, whereas lower-case letters are used for signifcant differences within site 1 (P < 0.05).
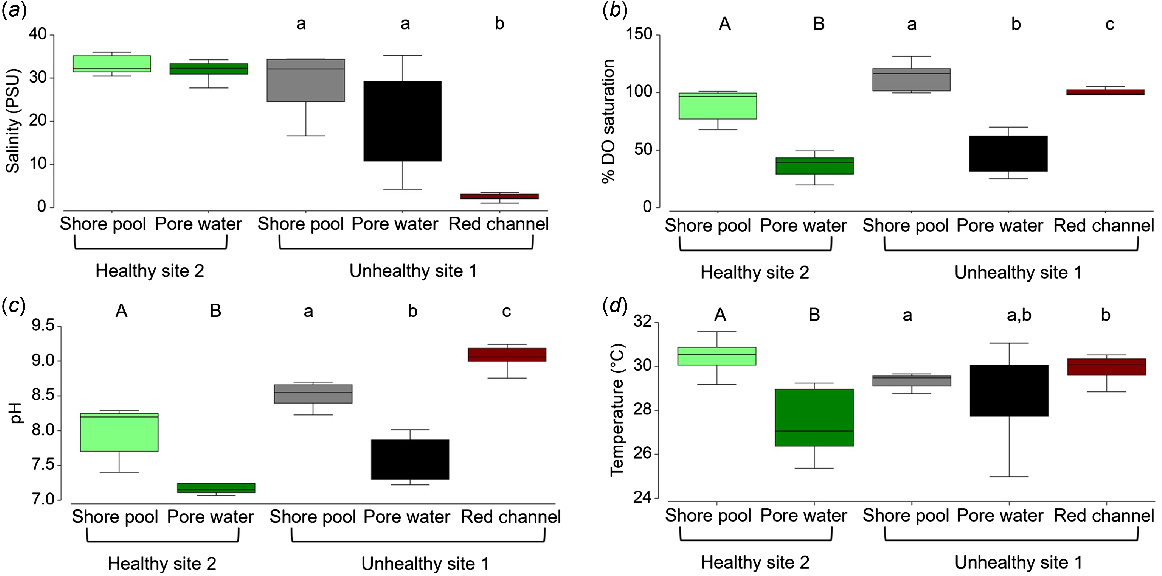
Parameter | Site | Location (site) | |||
---|---|---|---|---|---|
Pseudo-F | P-value | Pseudo-F | P-value | ||
Salinity | 2.13 | 0.1546 | 21.14 | 0.0001 | |
Dissolved oxygen | 0.47 | 0.4666 | 65.77 | 0.0001 | |
pH | 1.2 | 0.3949 | 74.58 | 0.0001 | |
Temperature | 0.11 | 0.8016 | 5.60 | 0.0039 | |
Turbidity | 0.51 | 0.5716 | NA | NA |
Turbidity was only tested for the shore pools due to disturbance from digging the porewater holes. P-vales in bold are statistically significant at α < 0.05. NA, not applicable.
The Spindisk water quality readings from Site 1 showed high dissolved phosphate in the creek and porewater on all sampling occasions, ranging from a mean of 0.2 to 1.85 mg L−1 (Table 1). This exceeds the trigger value for total phosphate (0.03 mg L−1 or 30 μg L−1) by nearly 1–1.5 orders of magnitude. Ammonia also exceeded the default environmental trigger values for estuarine waters in all samples (Table 1). The highest levels of ammonia were recorded in the pollution stream (1.15 mg L−1) in the creek adjacent to the unhealthy mangroves, at more than 75 times the default guideline value. Nitrite also exceeded the guideline values in the creek on all sampling dates (Table 1), whereas nitrate was more variable owing to a lower sensitivity of detection. The samples taken from the red water in the drainage channel showed the highest values of nitrate (67% above the default guideline values), but low phosphate and ammonia (although still in excess of the trigger values) and no nitrite (Table 1).
Chemical analysis
None of the metals or metalloids detected in the sediment from either site exceeded the safe environmental guidelines values (Table S2). Multivariate analysis of the normalised metals and metalloids in the elemental scan for contaminated sediment analysis showed no significant difference between Site 1 and Site 2 (P = 0.448, Table 3), despite tendency towards more nickel, chromium, copper and mercury at Site 1 (Fig. 5a). However, univariate analysis for mercury did confirm significantly higher levels of Hg in the sediment at Site 1 (Fig. 5b, than at Site 2 (P(MC) = 0.0185).
Parameter | Source | Pseudo-F | P(perm) P(MC) | Unique permutations | |
---|---|---|---|---|---|
Elemental composition (normalised) | Sediment | 0.8678 | 0.448 | 560 | |
As | Sediment | 0.063026 | 0.8132 | 35 | |
Pb | Sediment | 0.014155 | 0.9052 | 35 | |
Cr | Sediment | 0.029753 | 0.8648 | 35 | |
Cu | Sediment | 0.58549 | 0.4737 | 35 | |
Mn | Sediment | 0.26468 | 0.618 | 35 | |
Ni | Sediment | 3.1087 | 0.1297 | 35 | |
Zn | Sediment | 0.28339 | 0.6139 | 35 | |
Hg | Sediment | 9.7749 | 0.0185 | 15 | |
Fe | Sediment | 0.23646 | 0.6355 | 35 | |
Al | Sediment | 0.00012835 | 0.9913 | 35 | |
B | Sediment | 0.013244 | 0.9093 | 35 | |
Volatile organic compounds | Pneumatophores | 10.185 | 0.0096 | 7 |
P-vales in bold are statistically significant at α < 0.05.
Comparison of metals and metalloids in sediment from the unhealthy (Site 1) and healthy (Site 2) mangrove forests in Boambee Creek. (a) Principle components ordination plot with vector overlay based on Pearson’s correlation of >0.7; (b) box and whisker plot for mercury showing the 95% confidence intervals and range in the data at each site.
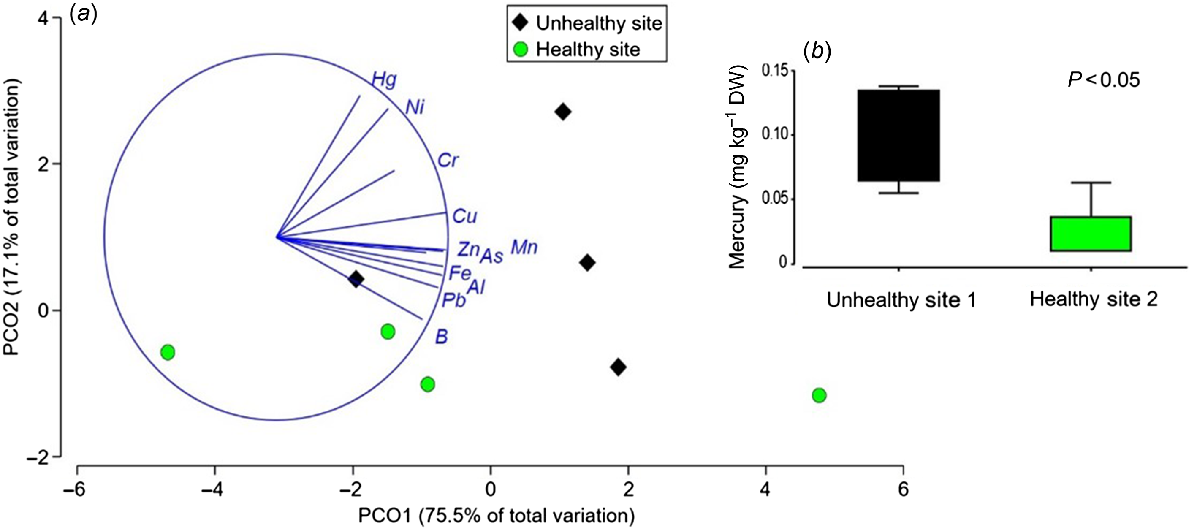
Investigation of the volatile chemicals in the mangrove pneumatophores by headspace GC/MS analysis showed a range of compounds at Site 1 (Fig. 6, Table S3). Samples from healthy pneumatophores from Site 2 (controls) contained only a solvent front (ethanol) and, in one of the three replicates, there were low concentrations of dimethyl disulfide or carbon disulfide. High concentrations of carbon disulfide were detected in all replicate samples of unhealthy pneumatophores from Site 1 (Fig. 6). In addition, samples from Site 1 contained reasonably high concentrations of naphthalene derivatives and small concentrations of furan and derivatives, phenols, including cresol, hydrocarbons including xylene and a number of butanal derivatives (Fig. 6). Multivariate PERMANOVA confirmed a significant difference in the percentage composition of volatile compounds emitted from the pneumatophores from the two sites (P(MC) = 0.0096, Table 3).
Volatile compounds detected by head space gas chromatography–mass spectrometry from the pneumatophores collected from unhealthy (Site 1) and healthy (Site 2) mangroves in Boambee Creek.
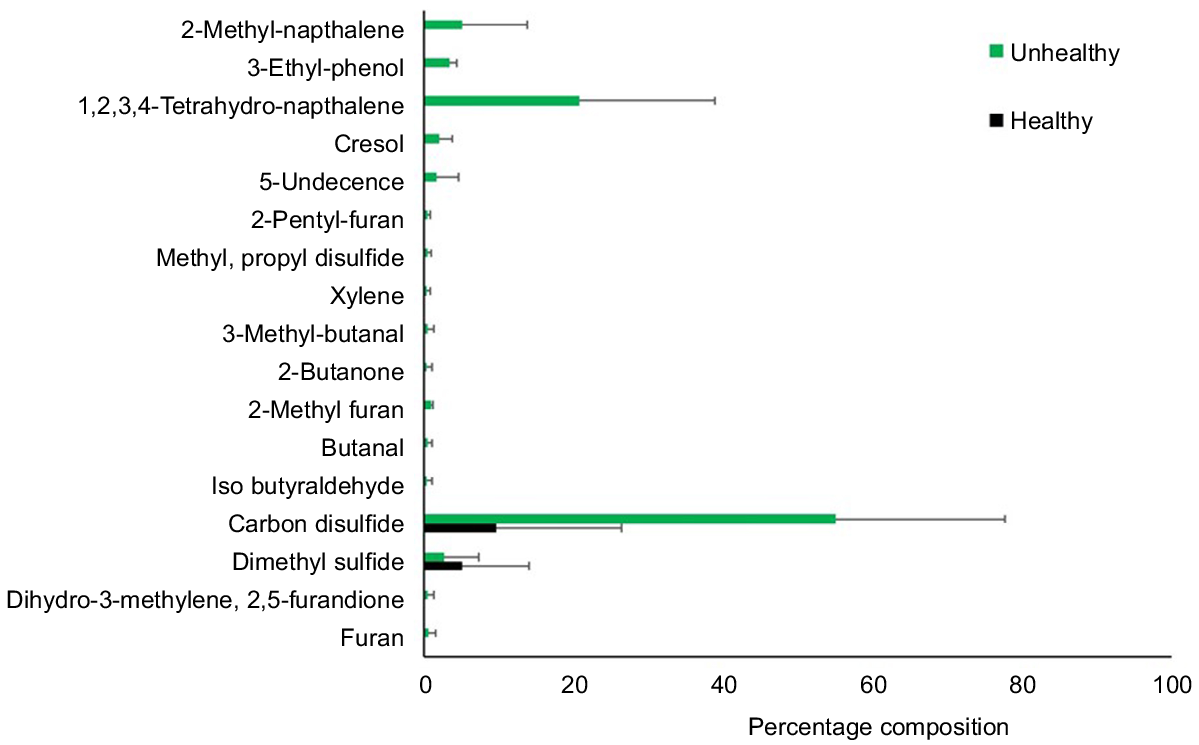
Sediment samples from both sites were subsequently analysed (after 1 month of storage). Carbon disulfide was detected in low concentrations at both Site 1 (mean 2.68 ± 0.76%) and Site 2 (mean 2.21 ± 0.75%). No other volatile contaminants were detected in these samples.
Mangrove surveys
Mangrove seeds were found on all levels of the shore at Site 2 in October 2023 (total = 68 in 36 quadrats) and tended to increase in abundance further into the forest (Fig. 7a). Only seven seeds across all transects were found among the unhealthy mangroves (Site 1), at higher levels of the shore. PERMANOVA confirmed that significantly more seeds were present at Site 2 (P = 0.0001). There was no significant change in seed abundance at different heights on the shore and no interaction with site (P < 0.05, Table 4).
Mean (+s.d.) density of mangrove (a) seeds, (b) seedlings and (c) pneumatophores among unhealthy (Site 1) and healthy (Site 2) mangroves in Boambee Creek.
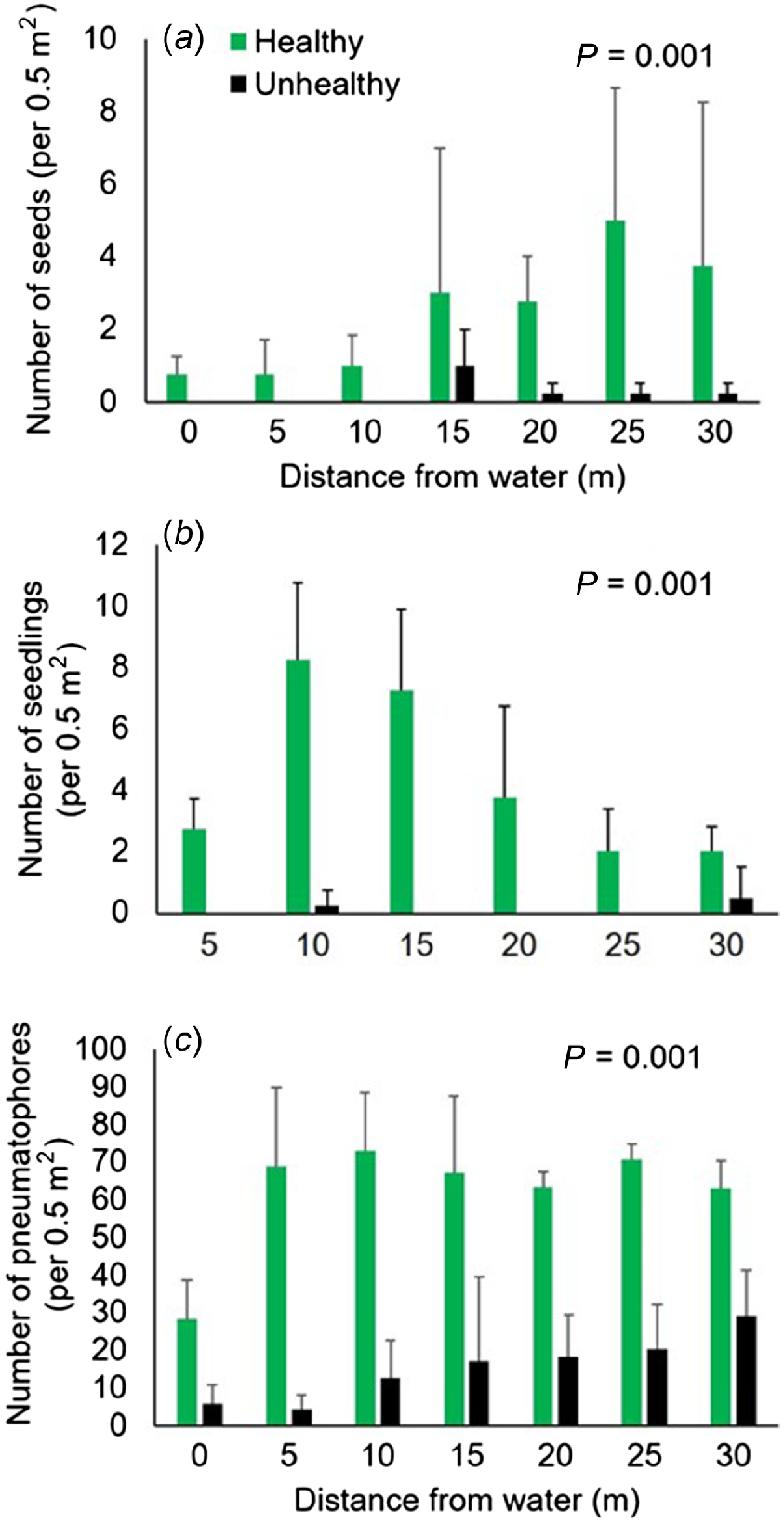
Parameter | Site | Shore height | Site × Shore height | |||||||
---|---|---|---|---|---|---|---|---|---|---|
Pseudo-F | P(perm) | Unique permutations | Pseudo-F | P(perm) | Unique permutations | Pseudo-F | P(perm) | Unique permutations | ||
Pneumatophores | 61.432 | 0.0001 | 9955 | 2.7018 | 0.0086 | 9939 | 2.2642 | 0.024 | 9933 | |
Seeds | 26.557 | 0.0001 | 9948 | 1.8419 | 0.078 | 9931 | 1.0997 | 0.3773 | 9938 | |
Seedlings | 107.65 | 0.0001 | 9948 | 1.3491 | 0.2398 | 9941 | 1.3641 | 0.2348 | 9942 |
P-vales in bold are statistically significant at α < 0.05.
Similarly, seedlings were found in all quadrats at Site 2, and the mean abundance was significantly higher than at Site 1, where seedlings were detected only in a few quadrats (Fig. 7b, P = 0.0001). Despite a trend towards more seedlings among the healthy mangroves in the middle distances from shore at Site 2, there was no significant difference according to distance and no interaction with site (P < 0.05, Table 4).
More pneumatophores were recorded in all quadrats at Site 2, than at Site 1 (Fig. 7c). PERMANOVA showed a significant interaction between distance from the shore and site (P = 0.024, Table 4). Significantly higher numbers of pneumatophores were associated with the healthy mangroves at Site 2 than the unhealthy mangroves at Site 1, at all levels of the shore (P < 0.05). There were fewer pneumatophores at the mean low water mark, than higher on the shore at Site 2 (P < 0.05, Fig. 7c) and fewer pneumatophores at 5 m at the Site 1 than other areas on the shore (P < 0.05, Fig. 7c).
Invertebrate surveys
The timed search surveys in November showed a total of 14 arthropod and 8 molluscan taxa at Site 2, compared with just 6 arthropods and 3 molluscs at Site 1 (Fig. 8a). The mean species richness was significantly higher among healthy mangroves at Site 2 (Fig. 8b, pseudo-F = 25.117, P(MC) = 0.008, 89 unique permutations) and there was a significant difference in the community composition between the two sites (pseudo-F = 6.3307, P = 0.0011, 1709 unique permutations) on the basis of semi-quantitative abundance rankings. Site 2 was characterised by higher abundance of two crabs and midges, whereas the hermit crab Pagurus sinuatus was detected only in one of the surveys at Site 1, but not at Site 2 (Fig. 8c).
Timed-search surveys for invertebrates among unhealthy (Site 1) and healthy (Site 2) mangroves in Boambee Creek, November 2023: (a) cumulative richness of invertebrate taxa; (b) mean species richness from 5-min timed-search surveys (n = 7); (c) principle components ordination plot of the invertebrate communities on the basis of semi-quantitative abundance rankings.
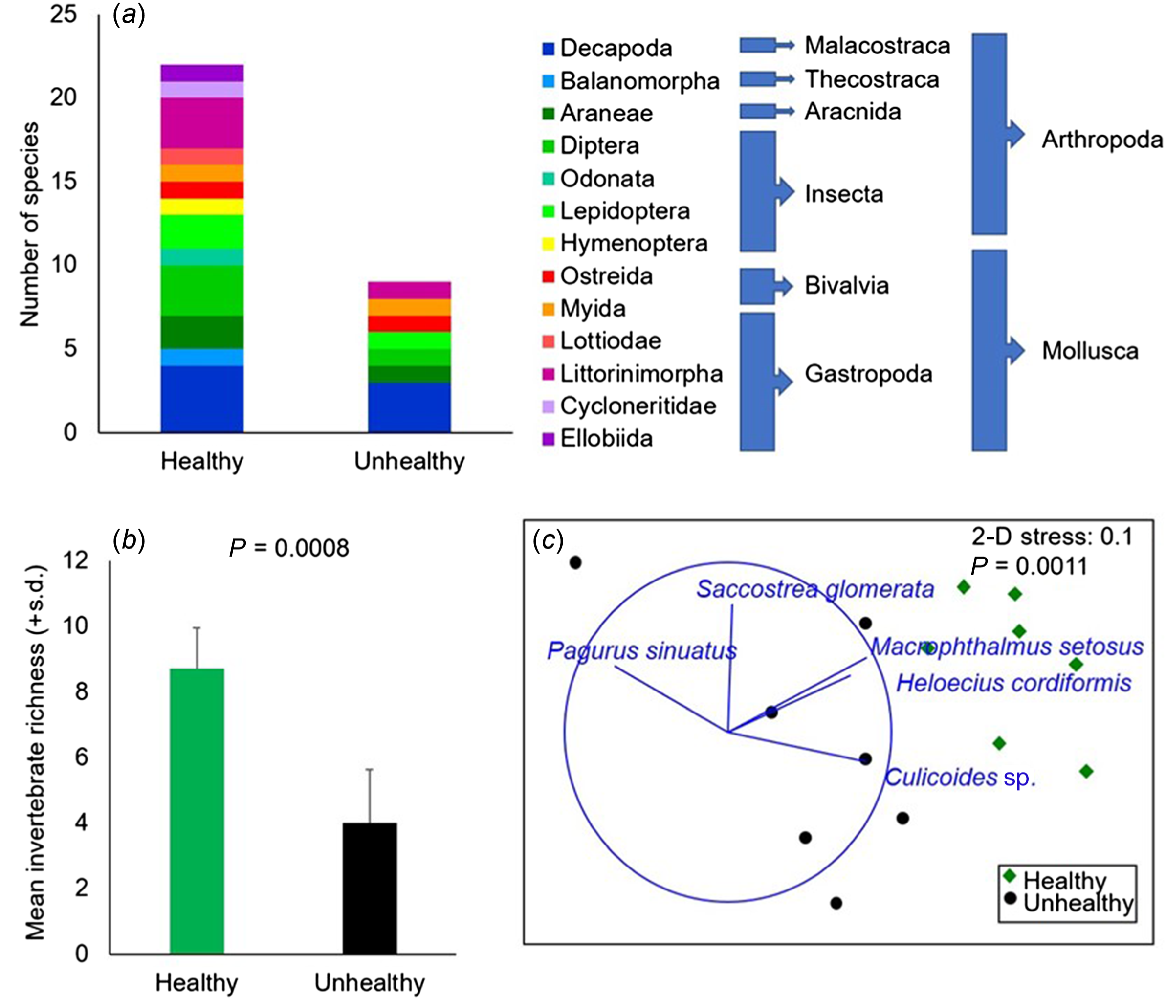
A range of infaunal taxa from six classes within four invertebrate phyla were identified from the benthic cores (Fig. 9a). A higher number of taxa were identified in the 2006 sampling than in 2023 at both sites. However, at Site 1 there was a greater decrease, from 36 taxa in 2006 to 22 taxa in 2023. In particular, no amphipods or mud whelks (Certhiidae) were recorded in cores among the unhealthy mangroves at Site 1 in 2023, (although two species of Certhiidae were observed on the edge of this site just after the timed-search surveys). At Site 2, 27 taxa were recorded in 2006, compared with 23 species in 2023.
The diversity of benthic infauna from cores taken at Site 1 and Site 2 Boambee Creek in 2006 and 2023 (n = 16); (a) cumulative richness of invertebrate taxa; (b) mean species richness; (c) mean total abundance; (d) Shannon’s H’ diversity index; and (e) multidimensional scaling bootstrap ordination of the community composition. Different letters above the bars indicate significant differences in post hoc pair-wise analyses of the interaction between year and site (P < 0.05).
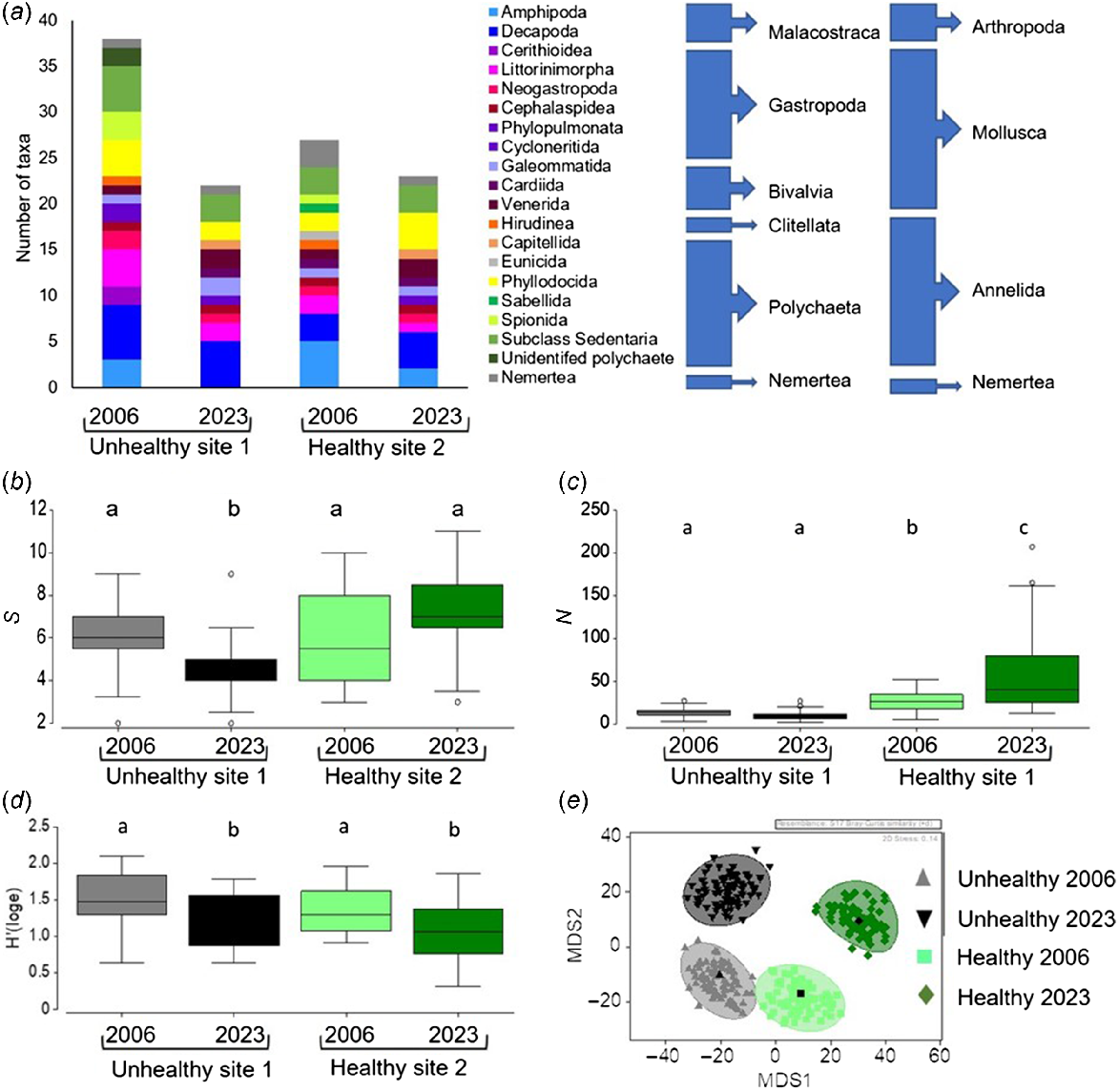
All measures of infauna biodiversity showed significant interactions between site and year (Table 5). Pairwise tests showed significantly lower mean species richness per core at Site 1 in 2023 than 2006, and at Site 1 than at Site 2, in 2023 (Fig. 9b). The highest mean species richness was recorded at Site 2 in 2023, but this was not different from that in 2006 (Fig. 9a). The abundance of invertebrates was higher at Site 2 than at Site 1 in both sampling years (Fig. 9c). Furthermore, the total abundance of invertebrates increased in 2023 at Site 2, whereas there was no change in abundance between years at Site 1 (Fig. 9c). Shannon’s H index was lower at both sites in 2023 than in 2006 (Fig. 9d). The community composition of infauna was significantly different between both sites in both years (P < 0.01). However, the bootstrap analysis showed more similarity in the communities across the two sites in 2006 than in 2023; the communities from Site 1 and 2 diverge from each other along MDS1, and from the 2006 communities along MDS2 (Fig. 9e). Similarity of percentage analysis showed that the abundance of the small bivalve Mysella vitrea is a major driver of these differences.
Parameter | Site | Year | Site × Year | |||||||
---|---|---|---|---|---|---|---|---|---|---|
Pseudo-F | P(perm) | Unique permutations | Pseudo-F | P(perm) | Unique permutations | Pseudo-F | P(perm) | Unique permutations | ||
Species richness | 2.6018 | 0.1127 | 2207 | 1.9115 | 0.1695 | 2184 | 13.593 | 0.0004 | 2211 | |
Total abundance | 17.676 | 0.0001 | 9691 | 3.6492 | 0.0584 | 9691 | 7.1253 | 0.0036 | 9686 | |
Shannon’s diversity | 5.8018 | 0.0175 | 9800 | 11.194 | 0.0016 | 9854 | 0.56585 | 0.4594 | 9824 | |
Community composition | 9.5478 | 0.0001 | 9935 | 4.1475 | 0.0003 | 9930 | 3.1918 | 0.0035 | 9914 |
P-vales in bold are statistically significant at α < 0.05.
Soldier crab response to Site 1 sediment
When first introduced to the experimental tanks, Mictyris longicarpus rapidly burrowed into the control sediment, within 25 s (Fig. 10). The crabs were active at night, generating between four and six holes per tank and many sediment balls as part of their feeding activities. After removing the crabs and adding a new layer of sediment, the soldier crabs that were returned to the control tanks continued to rapidly burrow into the sediment (within 20 s, Fig. 10). However, the soldier crabs placed in the tanks with a layer of sediment from Boambee Site 1 (unhealthy mangroves) remained active on the surface and burrowed down only after ~5 min (Fig. 10), which was ~15 times longer than in control tanks. The crabs in the treatment tanks burrowed down under the layer of unhealthy sediment and remained there without any activity for the next 3 days (as evidenced, but no sediment balls or holes on the surface). By comparison, the crabs in the control tanks remained active, generating, on average, five holes per tank and many sediment balls (e.g. Fig. 3).
The mean (+s.d.) burrowing time of Mictyris longicarpus before and after the addition of a layer of control sediment or unhealthy sediment from Site 1 at Boambee Creek. Only clean sediment from Charlesworth Bay (control sediment) was used in all tanks ‘before’ manipulation. Different letters above the bars indicate statistically significant differences from post hoc pair wise tests on the interaction between time and treatment.
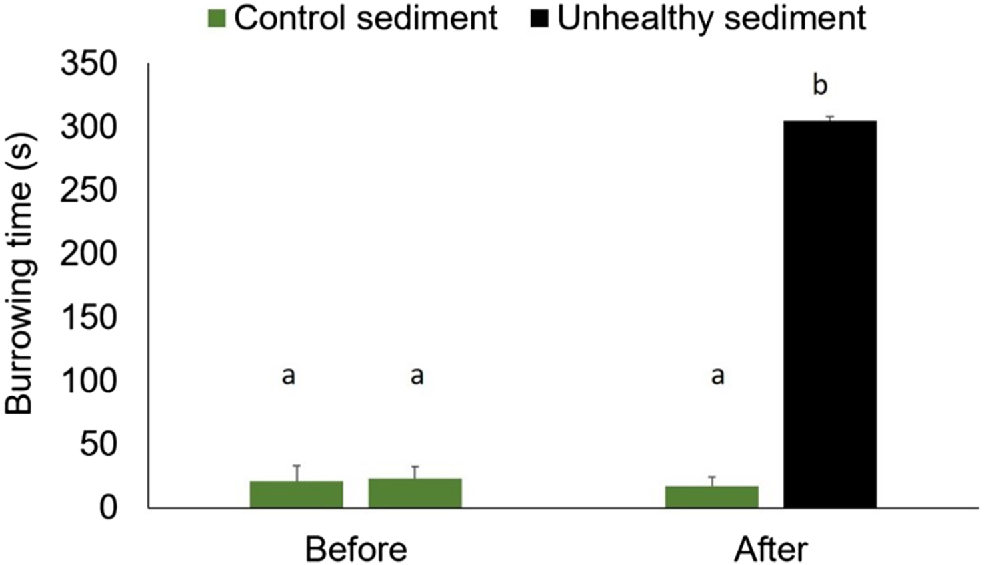
Discussion
There is much value in collaborating with Indigenous Rangers and Traditional Owners during ecological investigations. Quantitative ecological data can provide valuable insights into ecosystem health. But the ways that Aboriginal and Torres Strait Islander peoples are connected to Country, and their cultural values, are often mis-understood or not understood at all. If we can all come and work together on understanding the Lore of Aboriginal and Torres Strait Islander peoples, hopefully we can leave this world in a better condition than we found it. This investigation into the die-back of Bara-Baruga (mangroves) in Boambee Creek provides a good example of Indigenous participation in scientific research, with the combined purpose of identifying potential for healing Sea Country. Notably when NSW Government agencies were contacted about the mangrove die-back, they took a desk-top approach and identified the cause as a hail storm. Although we concur the hail storm was an important trigger for the mangrove die-back, it did not explain why the forest was not recovering after 2 years. Our comparative field-based investigation of the unhealthy site and a nearby healthy mangrove forest provided deeper insight into the extent of ecological decline. These data can now be used to inform management options and provide a baseline for future assessment of mangrove recovery.
It appears that Site 1 has been subject to multiple stressors over time, which may have all contributed to the decline in the mangrove ecosystem. In particular, a range of pollutants were detected among the unhealthy mangroves at Site 1. Healthy mangroves are remarkably resilient and have the ability to sequester pollutants (Lewis et al. 2011; Lewis and Pryor 2013) and regulate nutrients (Alongi 2018). However, defoliation after the hail storm may have negatively affected the ability of the mangroves to regulate and tolerate other stressors, such as toxic pollutants. Of particular concern was the detection of naphthalene, furan, xylene and other hydrocarbons in the pneumatophores of the unhealthy mangroves from Site 1. These are all industrial chemicals that could have come from a past spill in the area and been sequestered by the mangroves when healthy. Another possible source is aviation fuel; naphthalene and xylenes (Bernabei et al. 2003), as well as phenols including cresol (Guthrie et al. 2022), are typically found in avgas. Furans are used in synthesis of high-density aviation biofuels (Li et al. 2021). As the unhealthy mangroves at Site 1 are in direct line of the Coffs Harbour airport runway, these pollutants could be derived from occasional fuel dumping from jet planes as they come into land. Monitoring is recommended with passive air samplers to establish whether there is any ongoing chronic contamination at the site that may affect restoration and recovery.
We detected naphthalene derivatives in the pneumatophores of the grey mangroves (Avicennia marina) and these compounds may contribute to the petrochemical smell we also detected in the seeds and seedlings at Site 1 (Table S1). Napthalene, along with other hydrocarbons, was found to be uptaken and accumulated in the roots and seedlings of A. marina exposed to bunker fuel oil (Naidoo et al. 2010). These compounds can persist in the environment because of low water solubility and low degradation rates (Lewis and Pryor 2013). Other studies have shown that crude oil and dispersants can cause defoliation, affect new leaf formation, as well as damage the pneumatophores of mangroves (Wardrop et al. 1987). These impacts are all consistent with our observations in the die-back areas at Site 1. Nevertheless, there is some hope, with evidence of some seedlings germinating at the affected site, albeit significantly fewer than among the heathy mangroves at Site 2. Fewer mangrove pneumatophores and sediment holes from faunal activity are likely to contribute to lower potential for seeds to be caught and retained at Site 1 (total of seven seeds in 7 m2v. 68 seeds in the same area at Site 2). Facilitating natural restoration or replanting will be the most effective way to rehabilitate the contaminated soil at this site. Mangroves not only sequester contaminates in their roots, but they also support a diversity of microorganisms, with potential to break down contaminants for bioremediation (de Almeida et al. 2021).
Sites that have been contaminated by fuel or industrial pollution typically have multiple different types of contaminants, with potential for synergistic or cumulative effects. For example, mixtures of metals, as well as herbicides and hydrocarbon all have documented detrimental effects on mangrove seedling growth, survival and physiological responses (Lewis et al. 2011). We detected a range of metals (including cadmium) and metalloids in the mangrove sediment from Boambee Creek, but none exceeded the Australia and New Zealand default guidelines for sediment quality in aquatic ecosystems (Water Quality Australia 2020). However, mercury (Hg) was significantly higher among unhealthy mangroves at Site 1 and came very close to the default value of 0.15 mg kg−1 in two of the samples (0.14 and 0.13 mg kg−1). Elevated concentrations of Hg have been recorded in catchment waters adjacent to intensive horticulture north of Coffs Harbour and appear to be linked to treated waste water (Conrad et al. 2021b).
Mangrove ecosystems can contribute to the methylation of Hg, generating a more toxic form of methyl mercury, with potential for biomagnification up the food chain (Lei et al. 2019). Sulfur-reducing bacteria are important Hg-methylators in mangrove sediments and the detection of high concentrations of carbon disulfide in the unhealthy pneumatophores at Site 1 in Boambee Creek is indicative of significant sulfur-reducing activity. Marine ecosystems are a major natural source of carbon disulfide, although the production of dimethyl disulfide is more common in mangrove ecosystems (Cooper et al. 1987), as found in the healthy pneumatophores from Site 2. The high concentrations of carbon disulfide emitted from the pneumatophores at Site 1 are indicative of disruption in the normal microbial activities. Alternatively, it is possible that carbon disulfide is another contaminant at Site 1, because this compound is used as an industrial solvent e.g. (Drexler et al. 1994). Carbon disulfide has a short (2 day) half-life in water but has moderate acute toxicity and high chronic toxicity to aquatic life (Department of Climate Change, Energy, the Environment and Water 2022), suggesting the high concentrations emitted from Site 1 could contribute to stress in and around the mangrove die-off area.
The combined effects of aromatic hydrocarbons, carbon disulfide, mercury and other metals on mangrove ecosystems are not known. However, this mix of contaminants could have contributed to the lack of recovery of the mangroves after the 2021 hailstorm and the reduced taxonomic richness of invertebrates at Site 1 in comparison to the healthy mangroves of Site 2. Less than half the number of arthropod and molluscs species were found in the mangrove die-off area at Site 1 in timed-search surveys, which in part could be related to the reduced foliage for shelter and food. However, there was also a lack of bioturbators in the sediment under the dead mangroves and our laboratory experiment confirmed a negative behavioural response by soldier crabs to the contaminated sediments. The lack of bioturbators could create a negative feedback cycle, affecting many biogeochemical pathways for nutrient and carbon cycling (Sarker et al. 2021) and, potentially, the degradation of toxic contaminants.
Sampling of the benthic infauna in Boambee Creek adjacent to the healthy and unhealthy mangrove sites in 2023 showed some significant temporal changes in the communities since an earlier round of sampling in 2006. Fewer taxa were recorded in 2023 at both sites, which could be due to normal temporal fluctuation or different researchers identifying the species. However, there was a significant decrease in mean species richness at Site 1, which was not observed among the healthy mangroves at Site 2. Furthermore, there was an increase in benthic invertebrate abundance at Site 2 in 2023. This was largely driven by the small bivalve M. vitrea, which could be due to a recruitment event not observed to the same degree adjacent to the mangrove die-off areas at Site 1. There was also greater shift in the community structure and a noticeable absence of amphipods at Site 1 in 2023. The disappearance of amphipods has been identified as a good bioindicator for the impact of oil spills on macrobenthic communities (Gesteira and Dauvin 2000). Prolonged exposure to polyaromatic hydrocarbons, such as naphthalene, is toxic to amphipods (e.g. Landrum et al. 2003) and naphthalene has been shown to disrupt the mating pheromones of infaunal amphipods (Krång 2007). The infaunal communities are less likely to be affected by the defoliation of the mangroves in response to the hailstorm, supporting the hypothesis of multiple stressors, including contamination at the site.
The nutrient concentrations on the edge of Boambee Creek and in the pore water among unhealthy mangroves at Site 1 exceeded Australian and New Zealand Environment and Conservation Council and Agriculture and Resource Management Council of Australia and New Zealand (2000) guidelines for fresh and marine water quality on every sampling occasion. The source of the nutrients is not confirmed and could, at least in part, stem from the decomposing mangrove forest. Mangroves can retain nitrogen and phosphorus in a large reservoir of dead roots (Alongi 2018), but the disruption of normal photosynthetic and microbial activity and breakdown of roots could result in off-loading of nutrients back into the environment. However, an alternative source of nutrient input is the freshwater creek that drains through the mangrove forest from south-west to north-east. On more than one occasion, the water in this drainage channel was red in colour, with an extremely high pH (>9) and high nitrite concentrations. This is likely to be an algal bloom triggered by eutrophication. An increase in pH has been identified as a predictor of algal blooms (Wei et al. 2001) and high pH can increase the toxicity of harmful algal blooms (Valenti et al. 2010). Harmful red algal blooms in marine and freshwater are increasing globally and pose a potential threat to human health and aquatic life (Sha et al. 2021).
The potential for cumulative risks to human health from all the contaminants present in the mangrove die-off area at Site 1 in Boambee Creek also requires consideration. Carbon disulfide is a neurotoxin and can cause acute poisoning or chronic effects from inhalation, ingestion or absorption through the skin (National Institute of Health 2025). Some of the mild symptoms including headaches, nausea, itchy skin and fatigue were experienced by the researchers after some of the field trips to the unhealthy mangroves at Site 1 (Table S1). Some of the other volatile compounds detected in low amounts can also cause some of these symptoms, including xylene, methyl furan and butanal (Table S3). Furan and methyl furans are also carcinogens that induce oxidative stress and liver damage (Knutsen et al. 2017). Naphthalene is also classed as a carcinogen, but inhalation and oral exposure can cause a range of short-term and subchronic effects on the gastrointestinal, neurological, immune and pulmonary systems (Yost et al. 2021). At this stage, it is uncertain whether the concentrations of any of these contaminants are high enough to cause harmful effects to humans or the environment; so, further testing using quantitative analysis is required. We also recommend testing of seafood species, such as oysters and mud crabs for consumer safety, given the potential for biomagnification.
This study was limited by the lack of replicated baseline data before the hail storm, as well as the inclusion of only one ‘control’ site that was only partially affected by the hail storm. This prevented rigorous testing of causal effects from the hailstorm and any synergistic interactions with sediment contaminants on mangrove recovery in a well-replicated ‘before–after–control–impact’ design. It is likely that the mangroves at Site 2 regenerated faster because only part of the forest was defoliated, allowing more canopy shade and higher productivity, including seed production. Nevertheless, the study does provide valuable insights into multiple stressors, including contaminants that require consideration prior to investing in large-scale activities to restore Bara-Baruga country in Boambee Creek. Furthermore, this project has facilitated the development of a strong partnership between Indigenous Rangers and research scientists. Beneficial outcomes include the following:
building trust through two-way knowledge exchange, including respectful sharing of ecological knowledge, while protecting culturally sensitive site-based information;
empowering Indigenous Rangers with skills, including research permit applications, field-based risk assessments and techniques for recording quantitative data;
co-participation in the design and collection of ecological data, generating a baseline for future monitoring of mangrove ecosystems in Gumbaynggirr Country;
empowering early career scientists to learn on Country and make effective use of qualitative observations to refine or adapt sampling approaches when appropriate (e.g. prioritising chemical analysis of pneumatophores based on smell);
co-creation of knowledge and understanding, including the identification of potential ecological and human health risks associated with a contaminated site (with notification to the NSW Environmental Protection Agency);
sharing of data and raising awareness through engagement with Traditional Owners (Boambee Creek On-Country Day) and with the broader community (via media stories in local news on TV, radio and newspaper)
development of a shared vision and commitment to an ongoing partnership to help protect Gumbaynggirr Sea Country, including trialling methods for mangrove restoration and collaborative funding application.
Conclusions
This investigation has provided important insights into the mangrove die-off and ecological impacts within the Bara-Baruga ecosystem in Boambee Creek on Gumbaynggirr Country. Multiple stressors with negative feedback cycles have contributed to the decline. Defoliation by the hailstorm was an important trigger and most likely reduced the ability of the mangroves to withstand toxic contaminants, which may have been sequestered over a longer term within this modified urban and industrial catchment. The mangrove die-off may have then released contaminants back into the sediment and contributed to anoxic conditions, negatively affecting the fauna in the mangrove forest, as well as the water quality and benthic infauna in the adjacent creek. The lack of foliage at the unhealthy site may explain the absence of some insects, but sediment contamination is more likely to explain the reduced species richness of benthic invertebrates and impaired soldier crab burrowing behaviour in a laboratory experiment. Further research is required to fully understand the risks to seafood, human health and cultural values in Boambee Creek. Nevertheless, the germination and survival of some mangrove seedlings within the die-back area is a good sign of hope and future efforts will focus on assisting the restoration and recovery of mangroves using natural, cultural methods, with the intension of restoring the ecological balance and health of the Bara-Baruga.
Data availability
All the data for this paper is either provided in the supplementary material or are available on request to the corresponding author.
Declaration of funding
We are grateful for an Environmental Levy Grant from the City of Coffs Harbour to the Ngiyambandigay Wajaarr Aboriginal Corporation. The Gumbaynggirr Rangers and their cultural work is supported under the Australian Government Department of Climate Change, Energy, the Environment and Water Sea Country Indigenous Protected Areas Program. Southern Cross University provided field equipment and laboratory facilities for this research.
Acknowledgements
The authors acknowledge that this research was undertaken on Gumbaynggirr land and pay our respects to the Elders. Prof. Steve Smith supervised the original collection of benthic invertebrate data in 2006. We also thank Jade Farrugia, Maddison Brown, Greg Purcell and Samuel See for assistance in the field.
References
Alongi DM (2008) Mangrove forests: resilience, protection from tsunamis, and responses to global climate change. Estuarine, Coastal and Shelf Science 76, 1-13.
| Crossref | Google Scholar |
Alongi DM (2012) Carbon sequestration in mangrove forests. Carbon Management 3, 313-322.
| Crossref | Google Scholar |
Alongi DM (2018) Impact of global change on nutrient dynamics in mangrove forests. Forests 9, 596.
| Crossref | Google Scholar |
Araújo MP, Hamacher C, de Oliveira Farias C, Martinho P, de Oliveira Chaves F, Gomes Soares ML (2020) Assessment of Brazilian mangroves hydrocarbon contamination from a latitudinal perspective. Marine Pollution Bulletin 150, 110673.
| Crossref | Google Scholar |
Arrawarra Sharing Culture (2013) PROJECT FACT SHEETS – the Arrawarra Sharing Culture Project. (arrawarraculture.com) Available at https://www.yumpu.com/en/document/read/8421334/project-fact-sheets-the-arrawarra-sharing-culture-project [Verified 25 March 2025]
Australian and New Zealand Environment and Conservation Council and Agriculture and Resource Management Council of Australia and New Zealand (2000) Australian and New Zealand Guidelines for Fresh and Marine Water Quality. (ANZECC & ARMCANZ: Canberra, ACT, Australia) Available at https://www.waterquality.gov.au/anz-guidelines/resources/previous-guidelines/anzecc-armcanz-2000
Barbier EB (2016) The protective service of mangrove ecosystems: a review of valuation methods. Marine Pollution Bulletin 109, 676-681.
| Crossref | Google Scholar | PubMed |
Bayen S (2012) Occurrence, bioavailability and toxic effects of trace metals and organic contaminants in mangrove ecosystems: a review. Environment International 48, 84-101.
| Crossref | Google Scholar | PubMed |
Bernabei M, Reda R, Galiero R, Bocchinfuso G (2003) Determination of total and polycyclic aromatic hydrocarbons in aviation jet fuel. Journal of Chromatography A 985, 197-203.
| Crossref | Google Scholar | PubMed |
Bimrah K, Dasgupta R, Hashimoto S, Saizen I, Dhyani S (2022) Ecosystem services of mangroves: a systematic review and synthesis of sontemporary scientific literature. Sustainability 14, 12051.
| Crossref | Google Scholar |
Conrad SR, White SA, Santos IR, Sanders CJ (2021a) Assessing pesticide, trace metal, and arsenic contamination in soils and dam sediments in a rapidly expanding horticultural area in Australia. Environmental Geochemistry and Health 43, 3189-3211.
| Crossref | Google Scholar | PubMed |
Conrad SR, Santos IR, White SA, Woodrow RL, Sanders CJ (2021b) Cryptic night-time trace metal and metalloid contamination in an intensively cultivated coastal catchment. Environmental Pollution 276, 116685.
| Crossref | Google Scholar |
Cooper WJ, Cooper DJ, Saltzman ES, Mello WZ, Savoie DL, Zika RG, Prospero JM (1987) Emissions of biogenic sulphur compounds from several wetland soils in Florida. Atmospheric Environment (1967) 21, 1491-1495.
| Crossref | Google Scholar |
de Almeida FF, Freitas D, Motteran F, Fernandes BS, Gavazza S (2021) Bioremediation of polycyclic aromatic hydrocarbons in contaminated mangroves: understanding the historical and key parameter profiles. Marine Pollution Bulletin 169, 112553.
| Crossref | Google Scholar |
Department of Climate Change, Energy, the Environment and Water (2022) Description. In ‘Carbon disulfide’. (DCCEEW) Available at https://www.dcceew.gov.au/environment/protection/npi/substances/fact-sheets/carbon-disulfide#tabs-3 [Verified 12 September 2023]
Dobbs RJ, Davies CL, Walker ML, Pettit NE, Pusey BJ, Close PG, Akune Y, Walsham N, Smith B, Wiggan A, Cox P, Ward DP, Tingle F, Kennett R, Jackson MV, Davies PM (2016) Collaborative research partnerships inform monitoring and management of aquatic ecosystems by Indigenous Rangers. Reviews in Fish Biology and Fisheries 26, 711-725.
| Crossref | Google Scholar |
Drexler H, Göen T, Angerer J, Abou-El-Ela S, Lehnert G (1994) Carbon disulphide. International Archives of Occupational and Environmental Health 65, 359-365.
| Crossref | Google Scholar | PubMed |
Duke NC, Meynecke J-O, Dittmann S, Ellison AM, Anger K, Berger U, Cannicci S, Diele K, Ewel KC, Field CD, Koedam N, Lee SY, Marchand C, Nordhaus I, Dahdouh-Guebas F (2007) A world without mangroves? Science 317, 41-42.
| Crossref | Google Scholar | PubMed |
Fredericks J, Smith HB, Benkendorff K, Scott A, Hall KC (2025) Co-design and Traditional Owner participation in an assessment of abundance and size of Donax deltoides (garlaany, pipi) in Ngambaa Country, New South Wales, Australia. Marine and Freshwater Research 76, MF23235.
| Crossref | Google Scholar |
Friess DA (2016) Ecosystem services and disservices of mangrove forests: insights from historical colonial observations. Forests 7, 183.
| Crossref | Google Scholar |
Fusi M, Beone GM, Suciu NA, Sacchi A, Trevisan M, Capri E, Daffonchio D, Din N, Dahdouh-Guebas F, Cannicci S (2016) Ecological status and sources of anthropogenic contaminants in mangroves of the Wouri River Estuary (Cameroon). Marine Pollution Bulletin 109, 723-733.
| Crossref | Google Scholar | PubMed |
Gesteira JLG, Dauvin J-C (2000) Amphipods are good bioindicators of the impact of oil spills on soft-bottom macrobenthic communities. Marine Pollution Bulletin 40, 1017-1027.
| Crossref | Google Scholar |
Goldberg L, Lagomasino D, Thomas N, Fatoyinbo T (2020) Global declines in human-driven mangrove loss. Global Change Biology 26, 5844-5855.
| Crossref | Google Scholar | PubMed |
Guthrie JD, Romanczyk M, Kilaz G, Peretich ME, Kenttämaa HI (2022) Chemical characterization and quantitation of phenols in fuel extracts by using gas chromatography/methane chemical ionization triple quadrupole mass spectrometry. Energy & Fuels 36, 11962-11971.
| Crossref | Google Scholar |
Hill R, Grant C, George M, Robinson CJ, Jackson S, Abel N (2012) A typology of Indigenous engagement in Australian environmental management: implications for knowledge integration and social-ecological system sustainability. Ecology and Society 17, 23.
| Crossref | Google Scholar |
Jia H, Wang H, Lu H, Jiang S, Dai M, Liu J, Yan C (2016) Rhizodegradation potential and tolerance of Avicennia marina (Forsk.) Vierh in phenanthrene and pyrene contaminated sediments. Marine Pollution Bulletin 110, 112-118.
| Crossref | Google Scholar | PubMed |
Kaiser BA, Hoeberechts M, Maxwell KH, Eerkes-Medrano L, Hilmi N, Safa A, Horbel C, Juniper SK, Roughan M, Theux Lowen N, Short K, Paruru D (2019) The importance of connected ocean monitoring knowledge systems and communities. Frontiers in Marine Science 6, 309.
| Crossref | Google Scholar |
Kastury F, Cahill G, Fernando A, Brotodewo A, Huang J, Juhasz AL, Vandeleur HM, Styan C (2023) Metallic mangroves: sediments and in situ diffusive gradients in thin films (DGTs) reveal Avicennia marina (Forssk.) Vierh. lives with high contamination near a lead–zinc smelter in South Australia. Science of The Total Environment 857, 159503.
| Crossref | Google Scholar |
Knutsen HK, Alexander J, Barregård L, Bignami M, Brüschweiler B, Ceccatelli S, Cottrill B, Dinovi M, Edler L, Grasl-Kraupp B, Hogstrand C, Hoogenboom LR, Nebbia CS, Oswald IP, Petersen A, Rose M, Roudot A-C, Schwerdtle T, Vleminckx C, Vollmer G, Chipman K, De Meulenaer B, Dinovi M, Mennes W, Schlatter J, Schrenk D, Baert K, Dujardin B, Wallace H (2017) Risks for public health related to the presence of furan and methylfurans in food. EFSA Journal 15, e05005.
| Crossref | Google Scholar |
Krång A-S (2007) Naphthalene disrupts pheromone induced mate search in the amphipod Corophium volutator (Pallas). Aquatic Toxicology 85, 9-18.
| Crossref | Google Scholar | PubMed |
Laicher D, Benkendorff K, White S, Conrad S, Woodrow RL, Butcherine P, Sanders CJ (2022) Pesticide occurrence in an agriculturally intensive and ecologically important coastal aquatic system in Australia. Marine Pollution Bulletin 180, 113675.
| Crossref | Google Scholar |
Landrum PF, Lotufo GR, Gossiaux DC, Gedeon ML, Lee J-H (2003) Bioaccumulation and critical body residue of PAHs in the amphipod, Diporeia spp.: additional evidence to support toxicity additivity for PAH mixtures. Chemosphere 51, 481-489.
| Crossref | Google Scholar | PubMed |
Lei P, Zhong H, Duan D, Pan K (2019) A review on mercury biogeochemistry in mangrove sediments: hotspots of methylmercury production? Science of The Total Environment 680, 140-150.
| Crossref | Google Scholar | PubMed |
Lewis M, Pryor R (2013) Toxicities of oils, dispersants and dispersed oils to algae and aquatic plants: review and database value to resource sustainability. Environmental Pollution 180, 345-367.
| Crossref | Google Scholar | PubMed |
Lewis M, Pryor R, Wilking L (2011) Fate and effects of anthropogenic chemicals in mangrove ecosystems: a review. Environmental Pollution 159, 2328-2346.
| Crossref | Google Scholar | PubMed |
Li Z, Li Q, Wang Y, Zhang J, Wang H (2021) Synthesis of high-density aviation biofuels from biomass-derived cyclopentanone. Energy & Fuels 35, 6691-6699.
| Crossref | Google Scholar |
Lipps WC, Baxter TE, Braun-Howland E (Eds) (2022) 3125 Metals by inductively coupled plasma–mass spectrometry. In ‘Standard Methods For the Examination of Water and Wastewater’, 24th edn. (APHA Press: Washington, DC, USA) 10.2105/SMWW.2882.048
MacFarlane GR, Burchett MD (2002) Toxicity, growth and accumulation relationships of copper, lead and zinc in the grey mangrove Avicennia marina (Forsk.) Vierh. Marine Environmental Research 54, 65-84.
| Crossref | Google Scholar | PubMed |
Nagelkerken I, Blaber SJM, Bouillon S, Green P, Haywood M, Kirton LG, Meynecke J-O, Pawlik J, Penrose HM, Sasekumar A, Somerfield PJ (2008) The habitat function of mangroves for terrestrial and marine fauna: a review. Aquatic Botany 89, 155-185.
| Crossref | Google Scholar |
Naidoo G, Naidoo Y, Achar P (2010) Responses of the mangroves Avicennia marina and Bruguiera gymnorrhiza to oil contamination. Flora – Morphology, Distribution, Functional Ecology of Plants 205, 357-362.
| Crossref | Google Scholar |
Nath B, Chaudhuri P, Birch G (2014) Assessment of biotic response to heavy metal contamination in Avicennia marina mangrove ecosystems in Sydney Estuary, Australia. Ecotoxicology and Environmental Safety 107, 284-290.
| Crossref | Google Scholar | PubMed |
National Institute of Health (2025) Compound summary: carbon disulfide. In ‘PubChem’, 25 March 2025. (National Library of Medicine, National Centre for Biotrchnology Innformation, NIH) Available at https://pubchem.ncbi.nlm.nih.gov/compound/Carbon-Disulfide
Nursey-Bray M (2011) Social contexts and customary fisheries: marine protected areas and Indigenous use, Australia. Environmental Management 47, 671-683.
| Crossref | Google Scholar | PubMed |
Percy S, Lubchenco J, Almeida F, Armsworth P, Bennett A, Ebi K, Ehrmann J, Griffith J, Kumar P, Pagiola S, Prickett G, Richards D, Rivera J, Thompson V, Wenblad, A, Yohe G (2005) Ecosystems and human well-being: opportunities and challenges for business and industry. (World Resources Institute: Washington, DC, USA) Available at https://www.millenniumassessment.org/documents/document.353.aspx.pdf
Prober SM, O’Connor MH, Walsh FJ (2011) Australian Aboriginal peoples’ seasonal knowledge: a potential basis for shared understanding in environmental management. Ecology and Society 16, 12.
| Google Scholar |
Sarker S, Masud-Ul-Alam M, Hossain MS, Rahman Chowdhury S, Sharifuzzaman SM (2021) A review of bioturbation and sediment organic geochemistry in mangroves. Geological Journal 56, 2439-2450.
| Crossref | Google Scholar |
Sha J, Xiong H, Li C, Lu Z, Zhang J, Zhong H, Zhang W, Yan B (2021) Harmful algal blooms and their eco-environmental indication. Chemosphere 274, 129912.
| Crossref | Google Scholar |
Sippo JZ, Lovelock CE, Santos IR, Sanders CJ, Maher DT (2018) Mangrove mortality in a changing climate: an overview. Estuarine, Coastal and Shelf Science 215, 241-249.
| Crossref | Google Scholar |
Thomas L (2013) Indigenous history of the Coffs Harbour region. (Coffs Collection) Available at https://coffs.recollect.net.au/nodes/view/30668
Usman ARA, Alkredaa RS, Al-Wabel MI (2013) Heavy metal contamination in sediments and mangroves from the coast of Red Sea: Avicennia marina as potential metal bioaccumulator. Ecotoxicology and Environmental Safety 97, 263-270.
| Crossref | Google Scholar | PubMed |
Valenti TW, Jr., James SV, Lahousse M, Schug KA, Roelke DL, Grover JP, Brooks BW (2010) Influence of pH on amine toxicology and implications for harmful algal bloom ecology. Toxicon 55, 1038-1043.
| Crossref | Google Scholar |
Wardrop JA, Butler AJ, Johnson JE (1987) A field study of the toxicity of two oils and a dispersant to the mangrove Avicennia marina. Marine Biology 96, 151-156.
| Crossref | Google Scholar |
Water Quality Australia (2020) Default guideline values. In ‘Australian & New Zealand guidelines for fresh & marine water quality’. (Commonweath Government) Available at https://www.waterquality.gov.au/anz-guidelines/guideline-values/default
Wei B, Sugiura N, Maekawa T (2001) Use of artificial neural network in the prediction of algal blooms. Water Research 35, 2022-2028.
| Crossref | Google Scholar | PubMed |
Woodroffe CD, Rogers K, Mckee KL, Lovelock CE, Mendelssohn IA, Saintilan N (2016) Mangrove sedimentation and response to relative sea-level rise. Annual Review of Marine Science 8, 243-266.
| Crossref | Google Scholar | PubMed |
Yost EE, Galizia A, Kapraun DF, Persad AS, Vulimiri SV, Angrish M, Lee JS, Druwe IL (2021) Health effects of naphthalene exposure: a systematic evidence map and analysis of potential considerations for dose–response evaluation. Environmental Health Perspectives 129, 076002.
| Crossref | Google Scholar |
Zhang Z-W, Xu X-R, Sun Y-X, Yu S, Chen Y-S, Peng J-X (2014) Heavy metal and organic contaminants in mangrove ecosystems of China: a review. Environmental Science and Pollution Research 21, 11938-11950.
| Crossref | Google Scholar | PubMed |