A deep dive into the ecology of Gamay (Botany Bay, Australia): current knowledge and future priorities for this highly modified coastal waterway
T. P. Stelling-Wood







A School of Life and Environmental Sciences, The University of Sydney, Sydney, NSW 2006, Australia.
B Sydney Institute of Marine Science, 19 Chowder Bay Road, Mosman, NSW 2088, Australia.
C Centre of Marine Science and Innovation, UNSW Sydney, Sydney, NSW 2052, Australia.
D School of Biological, Earth and Environmental Sciences, UNSW Sydney, Sydney, NSW 2052, Australia.
E Geocoastal Research Group, School of Geosciences, University of Sydney, Sydney, NSW 2006, Australia.
F School of Natural Sciences, Macquarie University, Sydney, NSW 2109, Australia.
G Stantec Australia Pty Ltd, PO Box 19, Saint Leonards, NSW 2065, Australia.
H School of Life Sciences, University of Technology, Sydney, NSW 2007, Australia.
I School of Medical Sciences and School of Life and Environmental Sciences, University of Sydney, Sydney, NSW 2006, Australia.
J Environmental Protection Authority Victoria, Carlton, Vic. 3053, Australia.
K Climate Change Cluster, University of Technology Sydney, Ultimo, NSW 2007, Australia.
L NSW Department of Primary Industries, Sydney Institute of Marine Science, Chowder Bay Road, Mosman, NSW 2088, Australia.
M School of History and Philosophy of Science, The University of Sydney, Sydney, NSW 2006, Australia.
N Water Research Laboratory, School of Civil and Environmental Engineering, UNSW Sydney, Sydney, NSW 2093, Australia.
O NSW Department of Primary Industries, Port Stephens Fisheries Institute, Nelson Bay, NSW 2315, Australia.
P Australian Museum Research Institute, Australian Museum, Sydney, NSW 2010, Australia.
Q National Marine Science Centre, Southern Cross University, Coffs Harbour, NSW 2450, Australia.
R Marine Ecosystems Research, NSW Department of Primary Industries, PO Box 89, Huskisson, NSW 2540, Australia.
S Sydney School of Veterinary Science, Faculty of Science, The University of Sydney, Sydney, NSW 2006, Australia.
T Marine Studies Institute, School of Geosciences, University of Sydney, Sydney, NSW 2006, Australia.
U Present address: CSIRO Environment, Saint Lucia, Qld 4067, Australia.
Marine and Freshwater Research 74(12) 1003-1025 https://doi.org/10.1071/MF22268
Submitted: 15 December 2022 Accepted: 22 May 2023 Published: 19 July 2023
© 2023 The Author(s) (or their employer(s)). Published by CSIRO Publishing. This is an open access article distributed under the Creative Commons Attribution-NonCommercial-NoDerivatives 4.0 International License (CC BY-NC-ND)
Abstract
Context: Gamay is a coastal waterway of immense social, cultural and ecological value. Since European settlement, it has become a hub for industrialisation and human modification. There is growing desire for ecosystem-level management of urban waterways, but such efforts are often challenged by a lack of integrated knowledge.
Aim and methods: We systematically reviewed published literature and traditional ecological knowledge (TEK), and consulted scientists to produce a review of Gamay that synthesises published knowledge of Gamay’s aquatic ecosystem to identify knowledge gaps and future research opportunities.
Key results: We found 577 published resources on Gamay, of which over 70% focused on ecology. Intertidal rocky shores were the most studied habitat, focusing on invertebrate communities. Few studies considered multiple habitats or taxa. Studies investigating cumulative human impacts, long-term trends and habitat connectivity are lacking, and the broader ecological role of artificial substrate as habitat in Gamay is poorly understood. TEK of Gamay remains a significant knowledge gap. Habitat restoration has shown promising results and could provide opportunities to improve affected habitats in the future.
Conclusion and implications: This review highlights the extensive amount of knowledge that exists for Gamay, but also identifies key gaps that need to be filled for effective management.
Keywords: Australia, Botany Bay, Cooks River, estuary, First Nations people, Gamay, Georges River, Kamay, traditional ecological knowledge, urbanisation.
Introduction
Botany Bay, or Gamay (also pronounced Kamay) as it is called by First Nations people of Australia, has a long history with humans and holds great cultural significance to both First Nations people as well as to those who migrated or were transported to Australia from abroad. The waters of Gamay and its tributaries provided food and other resources for First Nations people of Gamay for thousands of years prior to European settlement, with the harvest and use of its resources being deeply entwined with their identity, culture and traditions (Australian Littoral Society 1977; National Oceans Office 2002). In 1770, Gamay was the landing place of British explorers when they first arrived in Australia. The British first called Gamay ‘Stingray Bay’ due to the large number of stingrays they saw in its shallow waters, but soon after changed it to Botanist Bay (later shortened to Botany Bay) due to the diversity of botanical specimens they were able to collect from the bay’s shoreline on that first visit (Adam 1997).
In the years since British colonisation, Gamay has become a hub for industrialisation in Sydney, which has led to extensive modification of the bay (Australian Littoral Society 1977). Urbanisation and land-use changes have exacerbated these modifications, resulting in the waterway being largely unrecognisable from the one First Nations people would have interacted with prior to European settlement (see Reid (2020) for an in-depth review of land-use changes in Gamay). Gamay’s long and complex history with humans means that successful and sustainable management of the bay requires consideration of the often competing interests of many different stakeholders, including government, industry, national and international transport, recreational users, residents, local Indigenous communities and the environment. Management efforts are also challenged by a lack of integrated knowledge of how the physical, geochemical and biological processes of Gamay as a whole interact to ultimately drive its functioning (Christensen et al. 1996). An important first step in the sustainable management of Gamay is therefore a thorough understanding of its current biophysical state.
To this day, the most comprehensive study of Gamay and its tributaries is the Environmental Control Study undertaken by the State Pollution Control Commission in conjunction with the Maritime Services Board of New South Wales (NSW) and the Port Authority of Botany Bay, which produced a series of technical reports on the processes, ecological communities and habitats of Gamay and its tributaries, published between 1978 and 1981. Although extensive in scope, this series of reports predates many of the recent human modifications to Gamay and, as a result, many of the processes, communities and habitats originally assessed in these reports either no longer exist or no longer occur as they once did. A recent review summarised published literature of some of the habitats within Gamay (Reid 2021); yet, a detailed synthesis of the complete body of biophysical studies on Gamay has not been completed. Such a synthesis is key to determining knowledge gaps and prioritising future research efforts so as to develop an integrated management strategy for Gamay. The present review therefore builds on previous reviews by synthesising all published knowledge on Gamay, including its physico-chemical characteristics and all its habitats (natural and human-made), to identify critical knowledge gaps and highlighting research opportunities, which current work has generated to ensure a sustainable future for Gamay.
First Nations people have actively managed and modified natural landscapes for tens of thousands of years (Rowland 2004). This includes the lands and water of the Gamay catchment, which First Nations people of Gamay fished and farmed for thousands of years before European settlement. The local ecological knowledge held by First Nations peoples (hereafter traditional ecological knowledge, TEK) encompasses their experiences with the environment over time (Berkes 1993). Historically, TEK from First Nations peoples of Australia has been overlooked; however, recent shifts in perspectives have meant that TEK is becoming increasingly recognised as an important and valuable source of ecological information (Fischer et al. 2022). In recognition of the importance of TEK in understanding ecological systems, where available, we have included this knowledge sourced from written archival or academic articles (this does not include oral traditions from community members), in addition to scientific literature identified in our literature review process, to produce the most comprehensive review of Gamay to date.
This review of Gamay is the second in a series of reviews that summarise the current state of knowledge on the ecology of the aquatic ecosystem and highlight key knowledge gaps for urban waterways in the Sydney region (excluding knowledge on anthropogenic threats to these waterways, because this will be published separately; see Johnston et al. (2015) for a review of Sydney Harbour’s aquatic ecology and Mayer-Pinto et al. (2015) for a review of threats to Sydney Harbour). These reviews can assist in planning research and management actions for the waterways on which they focus and inform similar practices for urban waterways around the world. In this review, we collated information regarding the geology, hydrology, chemistry and ecology of Gamay. Historical literature was also included because these early accounts contain some of the only written accounts of First Nations people of Gamay, Gamay’s aquatic ecosystem and their interactions prior to European settlement. We used the following three search methods: a systematic online literature search, a questionnaire and a workshop with scientists. The information we uncovered was then analysed and synthesised into a review of Gamay, summarised by habitat type, which quantified areas of high and low research effort, discussing what we know and do not know about this urban waterway, and highlighting future opportunities for science and management in the bay. On occasions where up-to-date syntheses on a habitat or an ecological community in Gamay already existed in the published literature (e.g. those reviewed in Reid 2021), we provided only a brief summary and referred to that source.
Systematic literature review
Our review used the following three search methods: (1) on 22 May 2021, we undertook a systematic literature search of databases (Web of Science and Google Scholar), by using the keywords: ‘Botany Bay’ and ‘Kamay’ and ‘Gamay’ and ‘Georges River’ and ‘Cooks River’; (2) on 5 August 2021, a questionnaire was distributed to ~60 scientists and managers affiliated with the Sydney Institute of Marine Science; and (3) two separate workshops and discussions were held with an interdisciplinary panel of marine scientists that have undertaken research in Gamay (on 8 and 13 October 2021). Workshop participants collated literature relating to their fields of expertise and highlighted works missing from our initial search. This allowed obscure yet important texts to be included, as well as highlighting many unpublished works and datasets not available on searchable databases. This was a key component of our search methods, as many important texts failed to include ‘Botany Bay’ or similar search terms in either the article title or keywords.
The titles, abstracts (and occasionally methods) of each article or report were examined, and the publication was included in our review if it discussed any physical, chemical or biological aspect of Gamay. Articles and reports were included in the review if they presented data wholly or partially collected from Gamay. Gamay was defined to include the main bay and the lower, estuarine regions of the Georges River and the Cooks River. This included a distance of 200 km extending seaward from the mouth of the bay, and the coastal waters off the headlands, Cape Banks and Cape Solander. It also included terrestrial areas associated with the Towra Point Aquatic Reserve (e.g. mudflats, mangroves and saltmarsh habitat), because this area is an important region for wading birds. Each publication was assigned to a field of study (e.g. ecology, oceanography) and a habitat type (e.g. rocky intertidal shores, seagrass). Some publications considered multiple habitat types. In these instances, each habitat type was considered an individual study and so was counted separately. Individual publications may therefore occur in multiple habitat-type categories. To identify areas where research was lacking, where appropriate, publications were also classified according to the major taxon they considered (e.g. fish, invertebrates). If multiple taxa or multiple habitats were considered, that publication was classified as ‘multiple’.
Results of literature review
In total, 577 different resources were included in this systematic review, including 477 journal articles, 75 reports, 13 books, as well as 12 additional resources of other types (see Supplementary Table S1 for full list of references included in this review). The earliest study included was published in 1876. There was 10 times the number of publications on the ecology of Gamay (70.7% of publications, 408 publications in total, Fig. 1) compared with those describing any other field of study. Despite First Nations people having inhabited Gamay for tens of thousands of years, and Gamay being the location that European colonists first made contact with First Nations people of Gamay, there was little in the way of published information on First Nations people of Gamay (‘historical’, 3.5% of publications, 20 publications in total, Fig. 1) or written TEK from First Nations people of Gamay. Similarly, there has been little scientific examination of Gamay’s fisheries, despite the long history of commercial fishing in the bay (until it was banned in 2002) and the continued use of the bay by recreational fishers (‘fisheries’, 3.5% of publications, 20 publications in total, Fig. 1).
Number of publications describing studies conducted in Gamay, categorised by discipline. ‘Other’ represents categories with fewer than seven publications (including taxonomic-based papers and studies detailing industrial developments).
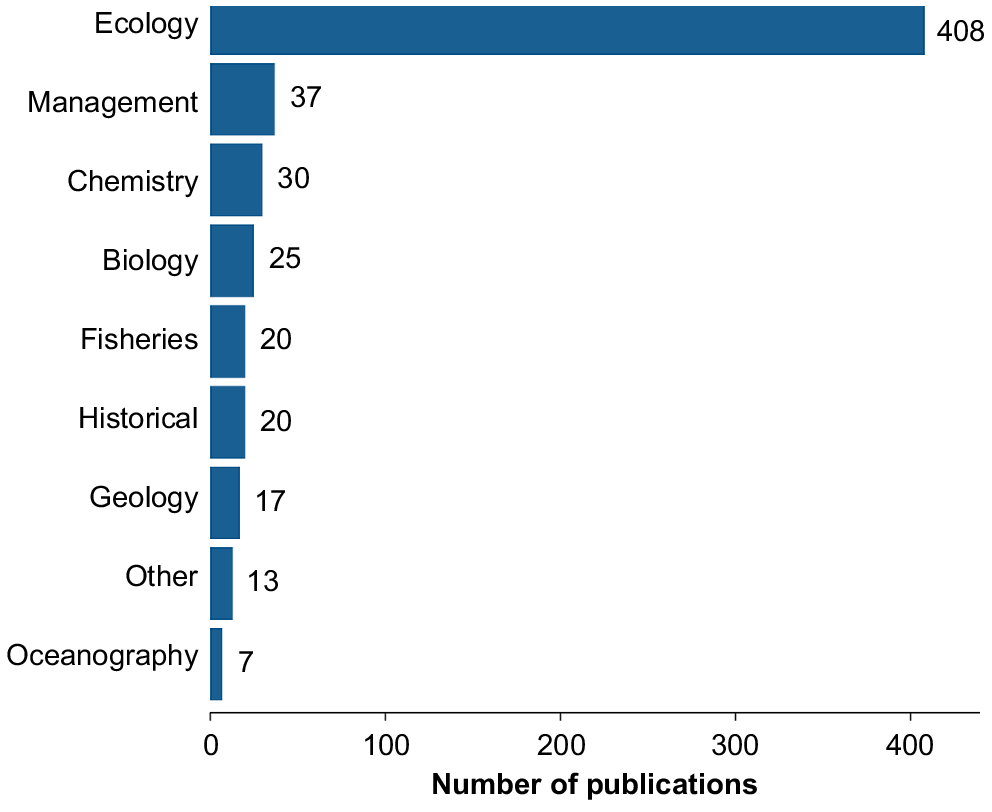
Rocky intertidal shores were the most common habitat featured in single-habitat studies (14.9% of all publications, 86 publications in total, Fig. 2), with much of this research being undertaken at the Cape Banks Aquatic Reserve on the northern headland of the bay. By contrast, freshwater habitats represented the most poorly studied habitats in single-habitat studies (0.7% of all publications, four publications in total, Fig. 2), whereas terrestrial habitats were the most poorly studied habitat overall (including both single- and multi-habitat studies, 17 studies in total, Fig. 2). This is particularly concerning because these terrestrial habitats are dominated by littoral vegetation such as tuckeroo (Cupaniopsis anacardioides) and magenta lilly pilly (Syzygium paniculatum), which is endemic to the coastal margin of NSW and is listed as vulnerable under the Commonwealth Environment Protection and Biodiversity Conservation Act 1999 and endangered under the NSW Threatened Species Conservation Act 1995 (NSW Office of Environment and Heritage 2012). Single-habitat studies were by far most common overall (Fig. 2, insert plot). Oyster-reef habitats featured least often in multi-habitat studies (Fig. 2), which was expected given that most oyster reefs have been lost for over a century, before the development of modern science (Alleway and Connell 2015). Because these are currently the focus of growing restoration efforts, this lack of information will hinder those efforts. The habitat types most commonly studied in combination were soft sediment and beach habitats (six studies, Fig. 2), followed by soft sediment and rocky shore habitats (four studies, Fig. 2), and subtidal reef habitat and artificial substrates (four studies, Fig. 2).
Studies were separated by habitat type. The number of studies that considered individual habitat types in isolation are shown in dark blue (‘single’). The number of studies that featured a given habitat type in addition to one or more other habitat types is shown in light blue (‘multiple’). Individual publications may therefore appear in multiple columns and thus the sum of all bars will be greater than the total number of publications included in the literature review. ‘Terrestrial’ represents habitats dominated by littoral vegetation associated with aquatic habitats. Inset plot in the lower right corner is a histogram of the number of different habitat types considered in studies.
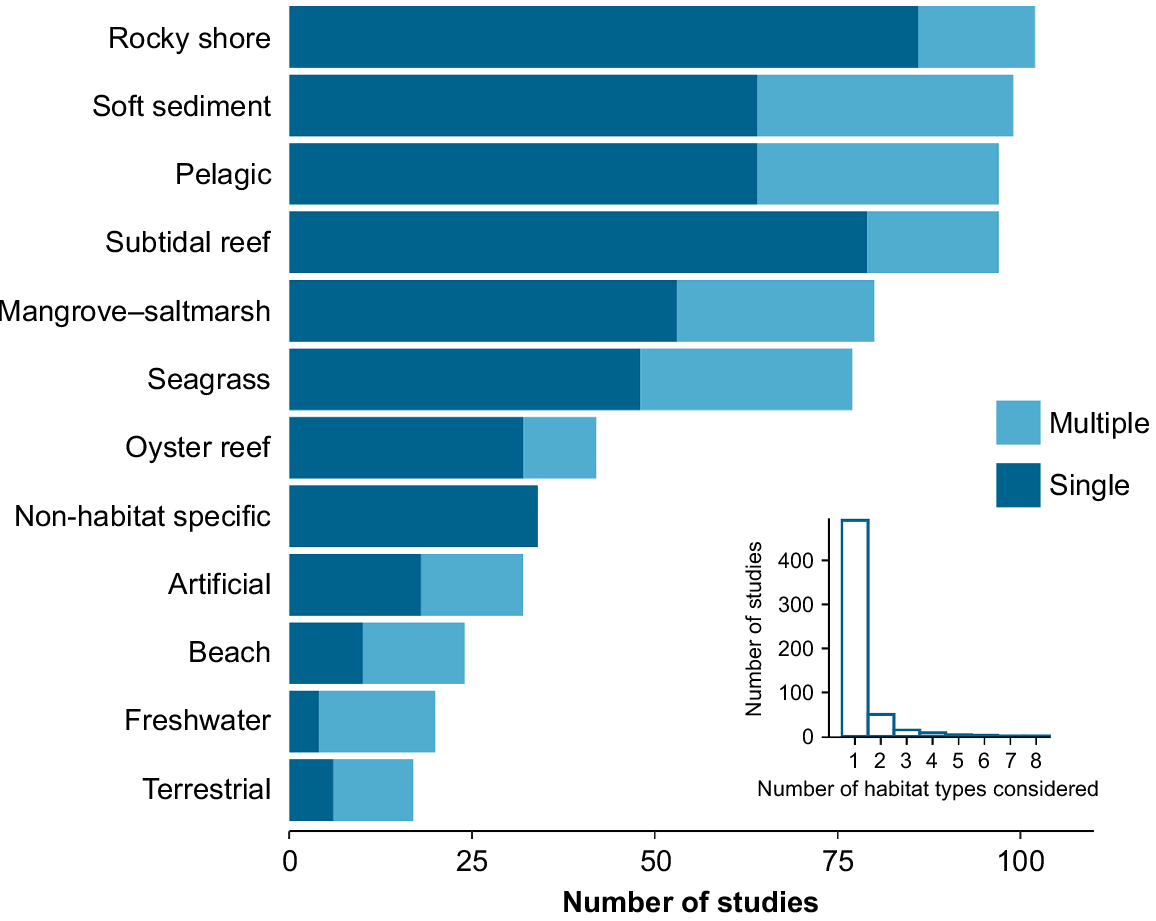
In the following pages, we present a synopsis of current knowledge of the Gamay ecosystem that arose from our literature search. All literature cited in this review is listed under References (this includes studies from outside Gamay), whereas the full list of all references uncovered during our systematic literature review is included separately in Supplementary material (contains only studies directly relating to Gamay).
Physico-chemical characteristics of Gamay
The Gamay environment
Gamay lies ~13 km south of the CBD of Sydney and is centred at ~34°S, 151°12′E. It is a semi-enclosed, low-flow embayment fed by two major freshwater tributaries, the Georges and Cooks rivers (Fig. 3), with a combined catchment area of ~1165 km2 (State Pollution Control Commission 1977). The majority of the catchment (~90%) is drained by the Georges and Woronora rivers, whereas the Cooks River and other minor tributaries drain the remainder (State Pollution Control Commission 1977). The average depth of Gamay is 11 m; however, much of the bay is significantly shallower at 5 m, except for the bay entrance, which was dredged to ~25 m to allow large-vessel passage into the bay and to gather sediment for reclamation works associated with the Sydney Airport and Port Botany expansion.
The distribution of vegetated marine habitat in Gamay, including the lower sections of its two major freshwater tributaries, Georges and Cooks rivers. Macrophyte (seagrass, mangrove, saltmarsh) distribution data were collected in 2019 and were provided by NSW Department of Primary Industries (2020a). Subtidal rocky reef has been mapped by depth only in Gamay. ‘Shallow rocky reef’ refers to reef less than 20 m deep, whereas ‘Deep rocky reef’ refers to reef 20–60 m deep. Subtidal reef data were provided by Stantec. The map also includes significant locations around the bay that are discussed in this review.
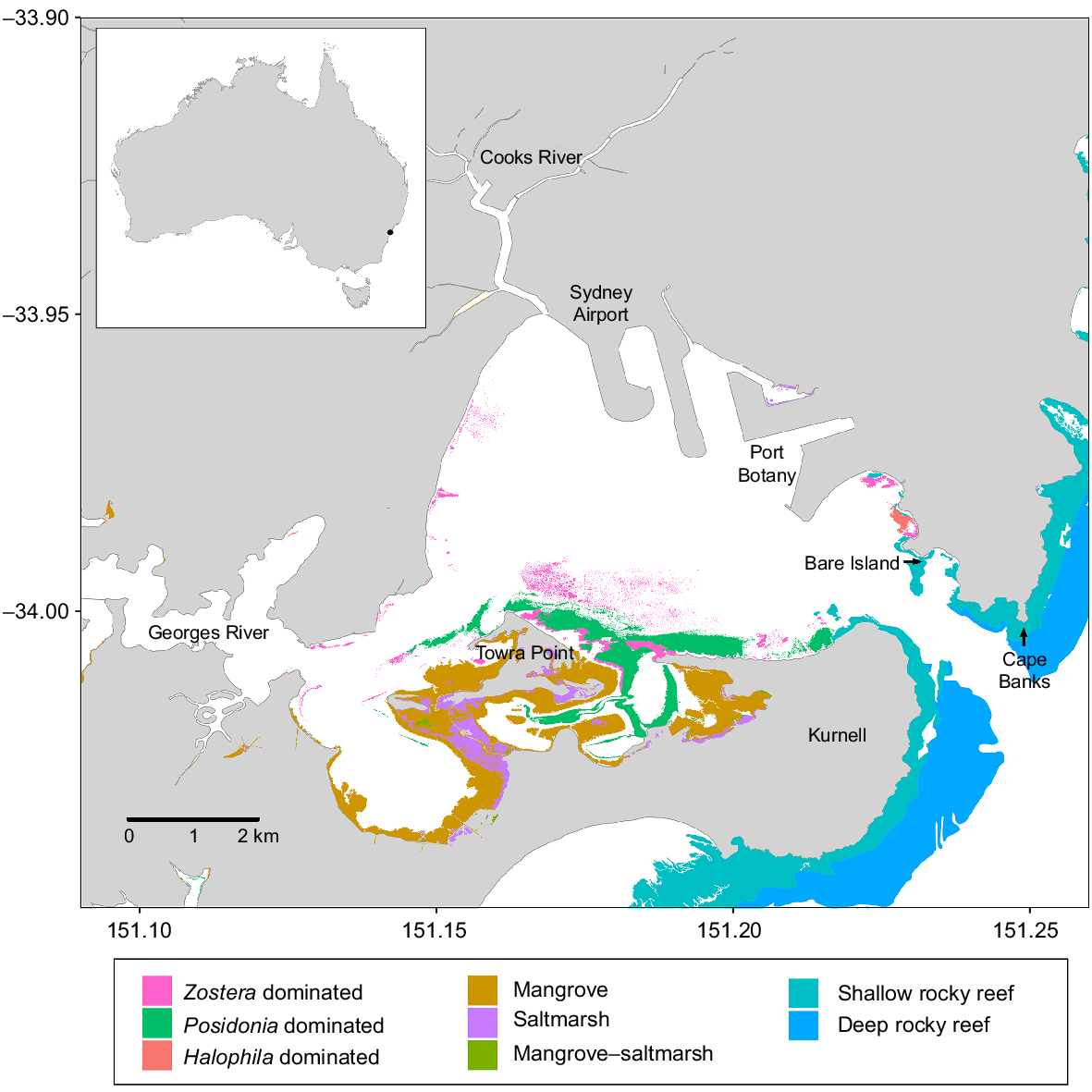
Geological history
Gamay is part of the greater Botany Basin (Roy and Crawford 1981), originally carved out of Hawkesbury sandstone by three ancient river systems, namely, the Cooks River, Georges River and a third, smaller ancient (unnamed) river (Albani et al. 1976). At 9000 years before present, sea level rose and the combined Cooks and Georges paleo-rivers joined the ancient Port Hacking channel and exited to the ocean from Bate Bay. Gamay’s geological history has been strongly influenced by climatic events of the Quaternary Period (2.58 million years before present to the present), where oscillations between low and high sea-level conditions caused the respective deposition and mobilisation of sediments in the Botany Basin (Albani 1981).
Our review found that geology was one of the least-studied fields of study (2.9% of all publications, 17 publications in total, Fig. 1), with an approximately equal number of publications discussing the geology and geological history of Gamay as those that focussed on sedimentology in the bay.
Hydrology and oceanography
Extensive development and urbanisation in the years since British colonisation have substantially altered the broader catchment of Gamay (Fig. 4), changing the hydrology of the bay, including rainfall-runoff patterns, hydrodynamics and hydraulics. Associated with this is an increase in catchment imperviousness, readily connecting urban areas directly to the bay through stormwater infrastructure (Sydney Metropolitan Catchment Management Authority 2011). This increases the likelihood that runoff (including pollutants and contaminants) directly enters the bay, rather than being filtered through soil and vegetation, potentially causing significant problems for the Gamay catchment (Sydney Metropolitan Catchment Management Authority 2011).
Aerial image of Gamay in 1945 (left) v. 2022 (right), highlighting urbanisation changes and alterations to the coastline, which have affected circulation flow paths. Major changes include the additions of the airport runways, the Port Botany expansion and the re-alignment of the Cooks River entrance (marked with red ‘x’). Image source is SixMaps.com.
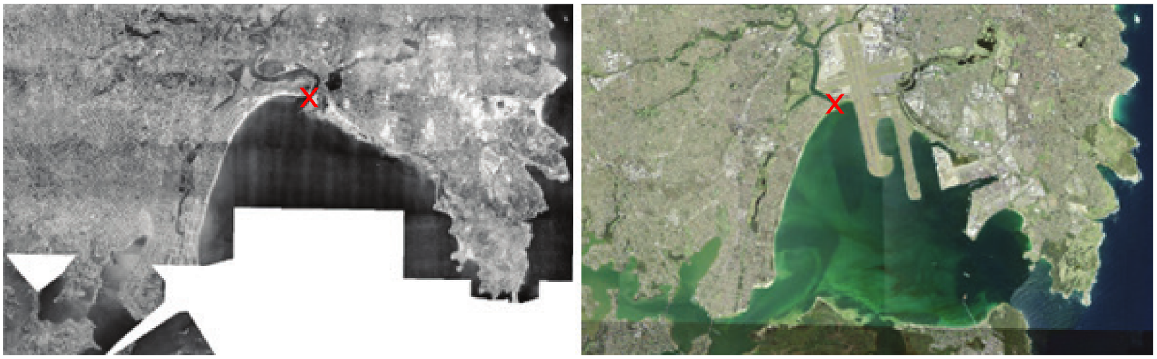
Oceanography was the field of study with the lowest number of publications, comprising just 1.2% of all publications, or seven publications in total (Fig. 1). Of those studies that have investigated Gamay’s hydrology and circulation, most have focused only on the main bay, with its two major tributaries (Georges River and Cooks River) often being excluded from studies owing to their low inflow (e.g. Roughan and Hallam 2022) and lack of data. However, freshwater input from these rivers into Gamay can be significant during periods of intense rainfall. The inclusion of these freshwater tributaries in future work on Gamay’s hydrology will be important as climate change and urbanisation are predicted to alter freshwater inflow into the main bay (Gillanders et al. 2011).
The habitats of Gamay
Soft-bottom and beach habitats
Soft-bottom habitats in Gamay are diverse, including intertidal beaches, sandflats and mudflats, as well as extensive subtidal sediment habitats. Excluding beach habitats, soft-bottom habitats were among the most highly studied habitats (11.1% of studies, 64 studies in total, Fig. 2) and were commonly included in multi-habitat studies (35 studies, Fig. 2). Beach habitats, by contrast, were some of the most understudied habitats (1.7% of studies, 10 studies in total, Fig. 2).
Intertidal mudflats are prevalent in sheltered coves, estuarine areas, rivers and creeks, as well as among mangroves, oyster reefs and built infrastructure. Theses habitats are particularly significant in Gamay because they support resident and migratory wading bird populations, some of which are in serious decline (Australian Littoral Society 1977; NSW Threatened Species Scientific Committee 1998). Gamay sediments and mudflats are productive habitats rich in organic materials, which underpin substantial communities of heterotrophic protists that consume up to 65% of bacterial production daily (Lee and Patterson 2002) and underpin detritus-based food webs (Bishop and Kelaher 2008; Kelaher et al. 2013). Detrital dynamics on Gamay mudflats are highly susceptible to detrital sources and environmental conditions, with changes in either influencing a range of processes including primary productivity, decomposition rates and nutrient cycling, and also affecting macrofaunal communities (Bishop and Kelaher 2008, 2013; Kelaher et al. 2013, 2018; Litchfield et al. 2020).
Land-use changes associated with European colonisation have significantly changed the physico-chemical characteristics of subtidal soft sediment habitats in Gamay (Reid 2020). Sand dominates much of the subtidal benthos in Gamay, with muddy areas associated with dredged areas near the airport runways and port infrastructure and at the bay’s entrance. By contrast, the Georges River has predominantly muddy embayments, whereas the channels have muddy sand (Reid 2021). Although Gamay beaches are generally considered low energy when compared with open ocean ones, they can still experience significant storm erosion, as seen following notable storms in 1974 (Fellowes et al. 2021) and 2016 (Gallop et al. 2020). Little is known about sediment-transport patterns and magnitudes in Gamay. However, it is thought that the presence or absence of channels or shoals next to beaches controls beach recovery after erosive events in Gamay.
Gamay’s soft-sediment habitats support a wide range of organisms. The diversity of fish and invertebrate species associated with benthic sediment habitats in Gamay have been discussed in detail by Reid (2021) and so are only briefly summarised here. Infaunal communities in sandy beaches are dominated by peracarid crustaceans, with biodiversity being greatest at the lower intertidal and in areas protected from wave action (Dexter 1984, 1985). At Quibray Bay, infaunal communities in sandy flats are more diverse that those from sandy beach habitats, likely owing to reduced wave action in this area of the bay (Rossi and Underwood 2002; Bugnot et al. 2022). By-catch data from prawn trawling represents the only information on demersal fish and macrobenthic communities in Gamay, finding 137 taxa associated with sediment habitats, including 115 finfish taxa, 11 crustaceans, 10 molluscs and 1 echinoderm (Liggins et al. 1996). Of these, the eastern fortesque (Centropogon australis), snapper (Chrysophrys auratus), two spot crab (Ovalipes australiensis), blue swimmer crab (Portunus pelagicus) and mantis shrimp (Squillidae spp.) were found in numbers exceeding a mean of 100 individuals caught per fisher-day of prawn trawling (or gill-netting).
Gamay’s sediment microbial communities have been assessed in a number of comparative studies across NSW estuaries (Lee and Patterson 2002; Sun et al. 2012, 2013; Dafforn et al. 2014). Community composition of microbial communities in soft sediments and phytoplankton was comprehensively sampled as part of surveys for marine pests (see Pollard and Pethebridge 2002). Over 50 species of dinoflagellates, including cysts have been identified in Gamay sediments, including Gonyaulacids, Protoperidinids and Diplopsalids, including multiple species potentially producing harmful toxins (Murray 2009; Tian et al. 2018). Sediment organic carbon content has been found to correlate with dinocyst occurrence (Tian et al. 2018). Most research on sediment bioturbators has focused on macroinvertebrates because these can be easily captured from surface layers using grabs; consequently, we have little understanding of the role that large sediment macrofauna, rays and fish play in the functioning of sediments and nutrient cycling in Gamay.
Seagrasses
Seagrass habitats in Gamay are dominated by Posidonia australis, Zostera muelleri spp. capricorni and species of Halophila, with these habitats having played a significant role in Gamay’s ecology for thousands of years (State Pollution Control Commission 1978). The P. australis population in Gamay is one of six NSW populations listed as endangered under state legislation (Fisheries Management Act 1994) and was listed as a threatened ecological community under Commonwealth legislation (Environmental Protection Biodiversity Conservation Act 1999). However, despite this, seagrass habitats were the most poorly studied vegetated habitats in Gamay (8.3% of all studies, 48 studies in total, Fig. 2), with research primarily focusing on seagrass physiology and temporal changes to seagrass cover. Few studies have investigated interactions between seagrass and their associated communities in Gamay, or connections between seagrass and other habitats.
The current extent of seagrasses in Gamay is likely to be only a fraction of its past extent prior to European colonisation, and today seagrasses cover just a little over 3 km2 of Gamay, primarily along the southern shoreline (Fig. 3). Since the 1940s, aerial photos have documented a steady decline in the cover of all seagrasses (Fig. 5; NSW Department of Primary Industries 2020a; Larkum and West 1990; West and Glasby 2022; NSW Department of Primary Industries, unpubl. data, 1972, 1986), with the largest losses occurring along the northern shoreline, possibly owintg to historical contamination in the 1800s and early 1900s (Larkum and West 1990) and, more recently, because of disruptions associated with the construction of both Port Botany (and its expansion) and the second and third airport runways (Reeds 2018; Macbeth 2019). Over the past 80 years, the rates of decline of seagrass has been 0.75% year−1 for total seagrass (Fig. 5) and 0.64% year−1 for P. australis-dominated meadows (Fig. 5), which, although on the lower range of rates recorded globally (Waycott et al. 2009; Dunic et al. 2021), are still a cause for concern. These meadows are prone to a range of natural and anthropogenic disturbances, including storms and erosion, occasional large-scale grazing from sea urchins and port development including dredging (Larkum and West 1990). Once meadows get too small (<50% of their maximum mapped area), or become fragmented, rates of decline can increase markedly (Larkum 1976; Dunic et al. 2021). Anthropogenic impacts to seagrasses are ongoing and a recent analysis has found that Gamay has some of the most fragmented Posidonia meadows in NSW (Swadling et al. 2023).
Hectares of seagrass in Gamay (excludes Georges River). Data source: Larkum and West (1990), West and Glasby (2022) and aerial photos of macrophytes in Botany Bay (NSW Department of Primary Industries, unpubl. data, 1972, 1986).
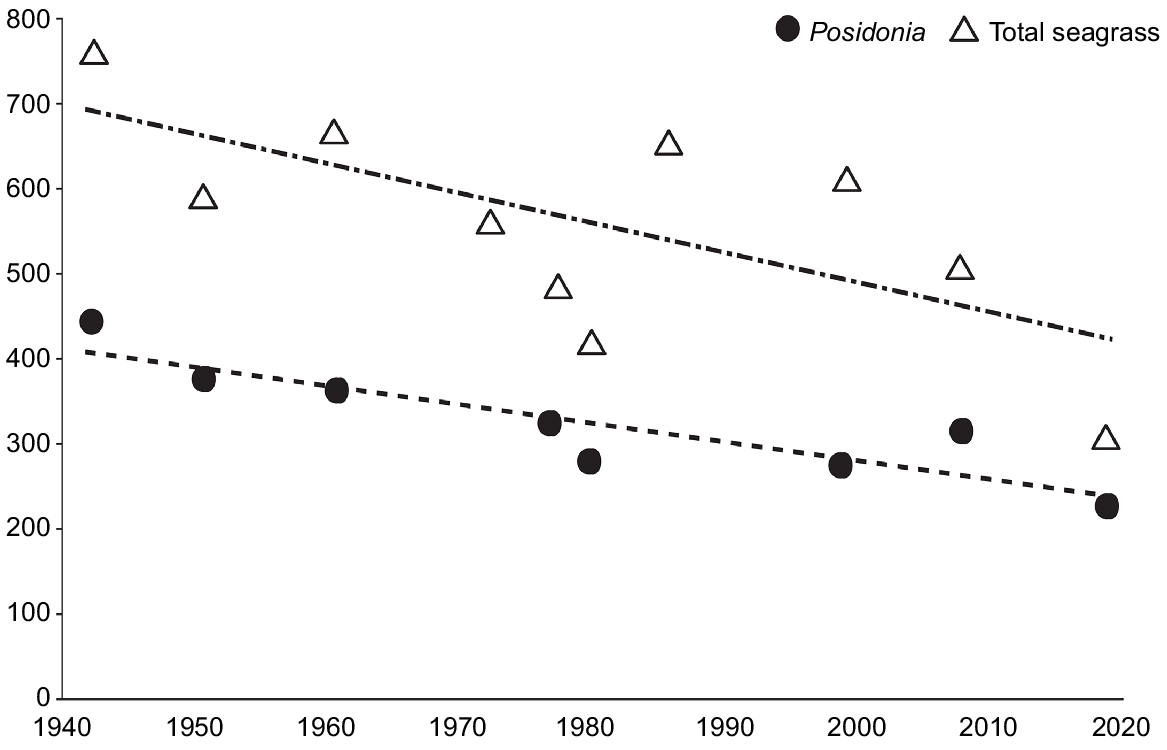
Within estuaries seagrass habitats represent some of the most ecologically important habitats, providing nursery habitat for fish and invertebrate species in Gamay (Bell and Pollard 1989; Reid 2021), many of which have commercial and recreational importance (Bell and Pollard 1989; Larkum and West 1990; Smith and Suthers 2000; Smith and Sinerchia 2004; Reid 2021). In comparison to other plant taxa, research investigating the role of symbiotic microorganisms (bacteria, fungi and archaea) in seagrass ecology is in its infancy (Nguyen et al. 2021); however, early evidence suggests that these microbial communities have close relationships with seagrasses, assisting in the acquisition of resources and helping buffer against environmental stress (Brodersen et al. 2014; Gribben et al. 2017; Tarquinio et al. 2019; Martin et al. 2020; Piercey et al. 2021). Additional gaps in Australia’s seagrass research, many of which are directly relevant to seagrasses in Gamay, are summarised in York et al. (2017).
Mangroves and saltmarsh
The Ramsar listed Towra Point Aquatic Reserve on Gamay’s southern shoreline (Fig. 3) contains the majority of the wetland habitat in the bay, with the rest being found in small patches in Penrhyn estuary and the upper regions of the Georges and Cooks rivers. The extensive wetlands at Towra Point equate to ~25% of the area of Gamay. The ecology of the Towra Point Nature Reserve has been reviewed elsewhere, and so is not included in this review (e.g. Australian Littoral Society 1977; Department of Environment, Climate Change and Water NSW, Sydney Metropolitan Catchment Management Authority 2010; Reid 2021).
Mangrove and saltmarsh habitats in Gamay support the aquatic ecosystem throughout the broader bay via both provision of hard substrata for sessile and mobile invertebrates (McGuinness 1990; Warren 1990; Ross and Underwood 1997; Minchinton and Ross 1999; Ross 2006) and nursery habitats for fish (Ross et al. 2009; Mazumder et al. 2011; Reid 2021). Saltmarsh plants are important habitats for high densities of molluscs (McGuinness 1990; Roach 1998) and are a primary source of dietary carbon for the resident grazing herbivores in the Towra Point wetlands, as shown in stable isotope analyses (Mazumder et al. 2011). These habitats can also support high densities of zooplankton in Gamay (Ross 2001), which are a major source of food for estuarine fish (Mazumder et al. 2009, 2011). Ground-truthed mapping of Gamay shows that mangrove and saltmarsh cover has changed over the past 40 years, with saltmarsh cover steadily decreasing and mangrove cover increasing (Fig. 6). Aerial photographs from the 1930s and maps from as early as 1882 show that these changes are not a recent event and, instead, suggest that this is a long-term trend for the bay (Mitchell and Adam 1989a, 1989b).
Hectares of mangrove and saltmarsh in Gamay (excludes Cooks and Georges rivers). Data source: NSW Department of Primary Industries (2020b).
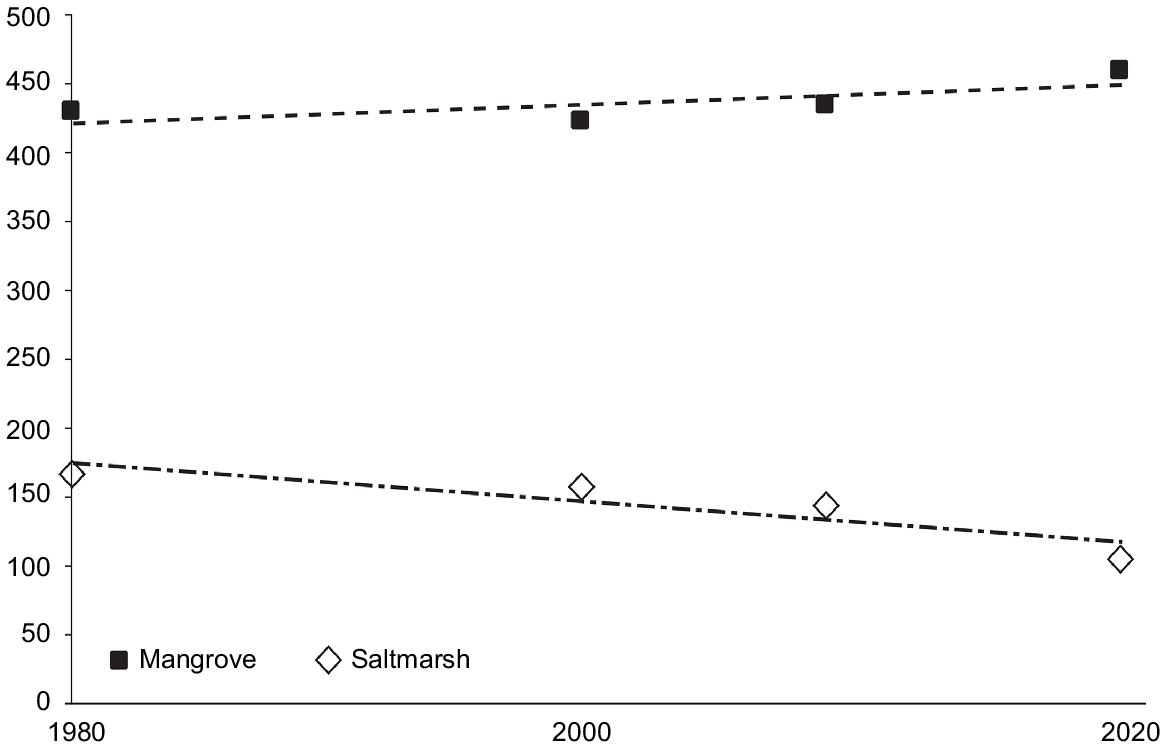
Early reports of Gamay by those that arrived on the first fleet described First Nations people of Gamay using resources from coastal wetland habitats (Australian Littoral Society 1977). Unfortunately, reports of how these wetland resources were specifically used are lacking for First Nations people of Gamay, and so it is necessary to look to reports on First Nations people from outside of Gamay, which have been recorded in more detail. Wood from the mangrove tree (Avicennia marina; known as egaie, Friess 2016) was used by First Nations people to make wood carvings (Hegerl 1982) and build canoes (Wilson 1858; Martin 1865). Mangrove fruit was also baked over coals and eaten by First Nations people of Australia (Hegerl 1982; Thozet 1985).
Subtidal rocky reef
The majority of Gamay’s subtidal rocky reef is located around the mouth of the bay, surrounding both headlands and extending along the oceanic coastline to the north and south (Fig. 3). Inside the bay, much of the subtidal reef habitat is shallow (<24 m deep), with deeper reef (20–60 m) occurring in small, isolated patches. By comparison, outside the bay, on the oceanic side of each headland, deep reef is the dominant habitat (Fig. 3). Subtidal reef habitats were the second-most studied habitat type (13.7% of studies, 79 studies in total, Fig. 1) in Gamay and were most often studied in combination with artificial-substrate habitats.
As for nearshore reefs in NSW generally, subtidal benthic reef assemblages in Gamay could be considered to be depth-driven, with various macroalgae dominating the shallowest parts, often transitioning to urchin barrens at ~5 m and then to sponge- or kelp-dominated deeper areas (Underwood et al. 1991). Much of the research on subtidal reefs in Gamay has occurred at Bare Island and Cape Banks, on the northern shore of the bay. This includes a focus on ecological interaction between macroalgae and herbivores (e.g. sea urchins, amphipods) with respect to grazing impacts and chemical ecology (Andrew and Underwood 1989; Andrew 1991; Underwood et al. 1991; Poore et al. 2000; Kingsford and Byrne 2023). The macroalgal community at Bare Island is diverse; for example, 45 species of Rhodophyta, 13 species of Phaeophyta and 3 species of Chlorophyta have been documented (Van Der Velde and King 1984). The fucoid Phyllospora comosa was once abundant along shallow reefs on the northern headland, but disappeared from the Sydney region in the 1970–1980s, likely in response to near-shore sewage outfall discharges (Coleman et al. 2008). Recent restoration efforts for P. comosa populations in the Sydney region have had some success in Gamay (e.g. at Kurnell; Mead 2020).
Bare Island is also known for its sessile invertebrate diversity, with many species of sponge, soft coral and bryozoans being found here (State Pollution Control Commission 1981a; Knott et al. 2004, 2006). Areas of deeper reef to the east of Bare Island also support colonies of cool-temperate hard coral (State Pollution Control Commission 1981a). The structure provided by these invertebrates, together with macroalgae, create complex reefs that support abundant and diverse mobile invertebrate communities dominated by crustaceans, polychaetes and molluscs (Van Der Velde and King 1984; Underwood et al. 1991; Poore et al. 2000). The highly prized abalone (Haliotis brazeri, H. coccoradiata and H. rubra) are also known to occur in Gamay. Abalone were historically harvested for trade purposes around the La Perouse area and remains an important food source for First Nations people of Australia (Cruse et al. 2005). Unfortunately, no recent comprehensive assessments of subtidal rocky reef fish assemblages in Gamay exist (but see Reid 2021). However, surveys conducted in the 1970–1980s did find that hard substrata (representing natural and artificial rocky reef) were among the most specious geomorphic zones in Gamay for fish (behind seagrass Z. muelleri spp. capricorni and deep soft substrate in the western region of the bay) (State Pollution Control Commission 1981a, 1981b, 1981c, 1981d). Kurnell Reef is an offshore subtidal reef that occurs just off Inscription Point on the southern shoreline of the bay. The reef here is deeper (~20 m) and diversity is low compared with Bare Island (State Pollution Control Commission 1981a). The area was traditionally dominated by sponges (Davis et al. 1997); however, more recently extensive Ecklonia beds at ~10-m depth have been observed growing in close proximity to the cliffs (Marzinelli et al. 2015). This area is a very popular recreational dive spot (A. Trevor-Jones, pers. comm.), particularly well known as a hotspot for weedy seadragons, which have been researched there for over 20 years (Sanchez-Camara et al. 2005, 2006, 2011; Klanten et al. 2020; Allan et al. 2022a, 2022b).
For the past 20 years, Gamay’s subtidal reefs have been at the centre of marine microbial research in Australia, with the aim of advancing our understanding of the ecology of marine free-living and host-associated microbes, and how these interact with benthic macroorganisms (e.g. Huggett et al. 2006; Swanson et al. 2006; Longford et al. 2007; Zozaya-Valdes et al. 2015; Taylor et al. 2022). Temporal sampling of kelp, sponges and coral-associated microbial communities from Bare Island (in addition to water, sediments and seagrass from elsewhere in the bay) have produced a first of its kind dataset that is being used to understand connectivity among, and environmental drivers of marine microbiomes (Phelps et al. 2021; Taylor et al. 2022). Kelp-associated microbes from Cape Banks and Kurnell have also been included in a global effort to characterise microbial diversity and function across all habitats on Earth as part of the Earth Microbiome Project (Thompson et al. 2017). This research has also uncovered strong links between microbiota and host kelp health (Marzinelli et al. 2015; Qiu et al. 2019). However, it should be noted that much of this research has focused on bacteria and archaea, and so we still have only limited understanding of the role of other microorganisms such as fungi, microalgae and viruses in these systems (but see Ferrari et al. 2021; McLennan et al. 2021).
Rocky intertidal shore
Approximately 30% of Gamay’s shoreline is rocky intertidal habitat (State Pollution Control Commission 1981a), with much of it located being around the two headlands (specifically at Cape Banks and Sutherland Point, Fig. 3), with some additional sections around Bare Island and La Perouse (Fig. 3), and a few patchy smaller intertidal shores along the Cooks River and Georges River estuary (Courtenay et al. 2005; Gall et al. 2013). Rocky intertidal habitats were the most well studied of all habitat types in Gamay, being considered in 86 single habitat studies (14.9% of studies, Fig. 2), with an additional 10 studies that considered rocky shores in addition to other habitat types (multiple-habitat studies).
The intertidal communities of the rocky shores found around the Gamay headlands generally display distribution patterns similar to those found on other rocky shores in NSW (State Pollution Control Commission 1981a; Underwood et al. 1983). The rocky shore communities of Gamay are discussed in detail in Reid (2021) and, so, are only briefly summarised here. At Cape Banks, sea-squirts (Pyura stolonifera), various macroalgae or tube-dwelling polychaetes cover the low levels of the shore and provide important habitat for associated macrofaunal assemblages, especially an abundance of gastropods and small bivalves (Kelaher et al. 2001; Kelaher 2003a, 2003b). The subtidal boulder fields are well studied. This habitat supports a diverse assemblage of mobile invertebrates that shelter under the boulders including ophiuroids, chitons and sea urchins, to name a few (Chapman and Underwood 1996). The mid-levels in comparison are characterised by encrusting algae, gastropods, seas urchins, sea stars and barnacles, with periwinkles dominating high levels on the shore (Denley and Underwood 1979; Arrontes and Underwood 1991; Underwood and Chapman 1996; Barbosa et al. 2013; Martinez et al. 2017). Overall, the range of species inhabiting the shoreline is higher at the mouth of the estuary than further up the estuary in the channels (Attenbrow 2010a).
Shellfish were an important resource for First Nations people of Gamay, usually collected directly from the rocky intertidal or subtidally by diving (Attenbrow 2010a). Excavated local middens suggest that bivalves and limpets were a particularly significant part of First Nations people’s diet prior to European settlement (Attenbrow 2010a). The diversity and relative abundances of shellfish found in different middens is highly variable, likely reflecting locational and seasonal variation in availability rather than community structure (Attenbrow 2010a). The robust shells of the turban snail (Turbo torquata) were also used to make hooks for line fishing by grinding them down into a hook shape, leaving only the pearly nacreous inside surface (Attenbrow 2010b).
Gamay’s northern headland, Cape Banks, was designated a Marine Scientific Research Area in 1975 (McGuinness 1988), before transitioning to an Aquatic Reserve in 2002. The intertidal rock platforms of Cape Banks have become a prominent site for research on key ecological processes, including inter- and intraspecific competition (e.g. Creese and Underwood 1982) and habitat–species interactions (e.g. Creese 1982). Given this, it is not surprising that invertebrate communities on intertidal rocky shores were overwhelmingly the most well studied taxon–habitat interaction (Fig. 7), with most of these studies having been undertaken at Cape Banks. However, this does mean that rocky shores in other areas of Gamay have received much less attention. Similarly, the generality of patterns and processes described for the Cape Banks area has not been sufficiently explored along neighbouring stretches of rocky shore to the north and south of Gamay.
Publications categorised by the major taxa and habitat type they investigated (quantified as the number of publications). ‘Multiple’ categories represent publications that considered more than one taxon or more than one habitat. Dark blue grid cells identify taxa–habitat interactions that have been comparatively well studied, whereas white grid cells identify taxa–habitat interactions that have not been studied. Grey grid cells identify taxa–habitat interactions that are not possible or not applicable to Gamay. The number of studies was log transformed for visualisation because invertebrates on intertidal rocky shore habitats have been an area of intense research effort (79 studies) compared to the next most well studied taxon–habitat interaction, invertebrates in soft sediment habitats (36 studies).
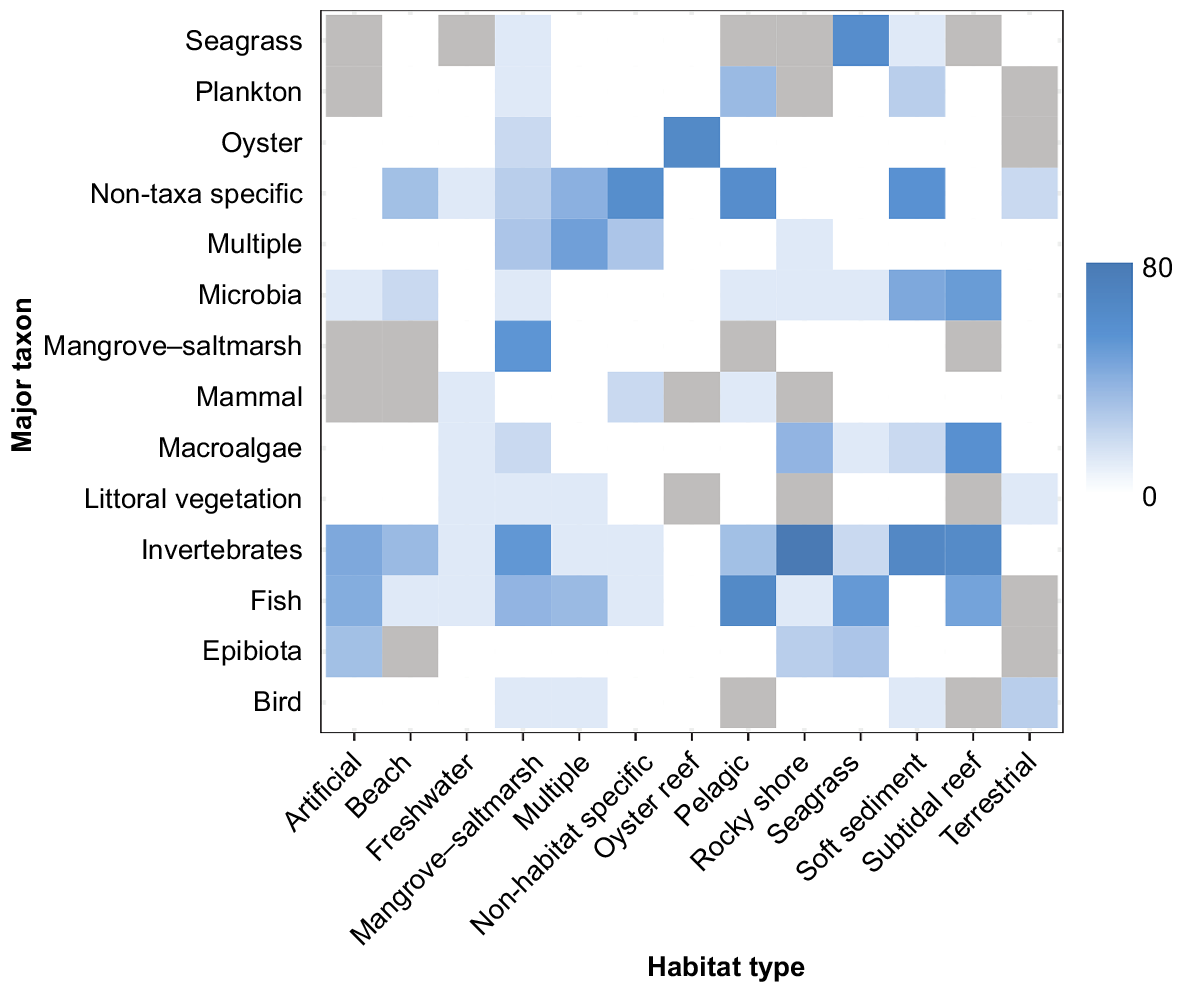
Oyster reefs
Oyster reefs historically played a significant role in the ecology of Australia’s coastal ecosystems (Roughley 1922; Gillies et al. 2020). Oysters have also been a significant food source for First Nations people of Gamay for over 6000 years, with large quantities of flat oysters (Ostrea angasi) and Sydney rock oysters (Saccostrea glomerata) found in middens in the Gamay area (Jackson and Forbes 2018). Oysters were also popular with European settlers and were ultimately exploited to near extinction. The demise of wild oyster populations in Gamay and the Georges River then stimulated the emergence of an iconic and valuable Sydney rock oyster aquaculture industry (Reid and Bone 2020). Oyster aquaculture production in the Georges River and Gamay peaked in the 1970s; however, in the following decade disease and pollution caused production to significantly decline (Reid and Bone 2020). Despite this, Gamay is still considered to support some of the best examples of remnant Sydney rock oyster (S. glomerata) reefs along the New South Wales coast.
Today, only 0.098 km2 of these remnant reefs remain in Gamay, with the majority being confined to the intertidal within the protected Towra Point Aquatic Marine Reserve (NSW Department of Primary Industries 2020b). Despite its small size, recent video sampling shows that this remnant reef has considerable biodiversity and fisheries value. In total, 44 fish species were found to be associated with oyster reefs at Quibray Bay and Carter’s Island in Gamay, a number comparable to P. australis seagrass beds (48 species), and over 60% greater than the numbers at sedimentary habitat (27 species) and mangrove habitat (29 species) (Martínez-Baena et al. 2022). The protected status of Gamay’s oyster reefs means that macroinvertebrate sampling is not possible because of destructive sampling methods. However, censuses from other NSW estuaries have found a five-fold greater density, biomass and productivity of macroinvertebrates on oyster reefs than on adjacent sediments (McLeod et al. 2020), suggesting that a similar habitat value may be expected in Gamay. Oysters have also been shown to influence nearby communities in Gamay, enhancing invertebrate abundances and richness in surrounding unvegetated sediments and mangroves (Minchinton and Ross 1999; Bishop et al. 2009, 2012; Hughes et al. 2014; Bugnot et al. 2022).
The ecosystem services provided by oyster reefs can have far-reaching benefits for entire ecosystems; yet, compared with other habitats in Gamay, these are some of the most poorly studied. Since the decline in oyster production in Gamay, much of the research effort has gone into selective breeding programs to develop disease-resistant oyster lines for aquaculture (Nell and Hand 2003; Nell and Perkins 2006; Dove et al. 2013). However, knowledge gaps still surround the transfer of disease and interbreeding between cultured and wild oysters (both Crassostrea gigas and S. glomerata). A thorough understanding of oyster disease in Gamay will be important not only for wild oyster populations and aquaculture, but also for oyster-reef restoration efforts. To maximise the ecosystem benefits of oyster reefs more broadly right across Gamay, more research is needed into methods of farming and restoration, as well as exploring optimal sites, locations and configurations for oysters to be grown within the bay.
Open water and pelagic systems
Within estuaries and marine embayments, open water or pelagic regions provide corridors for the transport and movement of species and resources, making these significant habitats for the functioning of these waterways. In line with this, these habitats were among the most highly studied of all habitats in Gamay, for both single-habitat (11.1% of studies, 64 studies in total, Fig. 2) and multi-habitat (33 studies, Fig. 2) studies.
In Gamay, a distinctive estuarine plume of more turbid water is demarcated by a tidal front and a line of flotsam, which tidally fluctuates between Bare Island and 4 km offshore (Kingsford and Suthers 1994, 1996; Reid 2021). The plume is only 1–3 m deep but brings estuarine nutrients to clear oceanic waters, which presumably enhances local planktonic production, but this is difficult to determine at the short, tidal durations. Jellyfish (Catostylus mosaicus) occur in open-water habitats in Gamay’s main bay and the Georges River (Reid 2021). Significant fish species within the pelagic environment of Gamay include schooling baitfish such as sandy sprat (Hyperlophus vittatus), Australian anchovy (Engraulis australis) and yellowtail scad (Trachurus novaezelandiae), with other significant species such as silver trevally (Pseudocaranx georgianus) and yellowtail kingfish (Seriola lalandi) also being common. Mullet (Mugil cephalus) is a particularly important species for First Nations people of Gamay, with the harvest occurring from March to June at Frenchmans Bay and Yarra Bay at La Perouse, and is associated with a time of celebration (Fay and Linkhorn 2021). Rarer sightings of larger fish such as whale shark (Rhincodon typus) and bump-head sunfish (Mola alexandrine) have also been reported in Gamay. Bones of Dugong excavated from an indigenous midden near Gamay suggest that dugongs occurred in the area historically (Haworth et al. 2004); however, recent sightings along the NSW coast are rare and none has been reported in Gamay (Allen et al. 2004). Little penguins (Eudyptula minor) can also occasionally be seen foraging for baitfish in Gamay (Carter 2020). For further details on the ecology of pelagic fish communities in Gamay, see Reid (2021).
In comparison to Gamay’s pelagic macrofauna, the microbiota of these habitats are less well documented. Planktonic microbe communities in Gamay are highly dynamic both temporally and spatially (McLennan et al. 2021), with seasonality being a strong driver of this variability. For example, the ‘red tide’-forming species Noctiluca scintillans is highly abundant in Gamay in spring and late summer, forming visible water discolouration at times, and is present only at low abundances in winter (Murray and Suthers 1999). Riverine inflow, land runoff, and other processes influence phytoplankton communities in an episodic manner, as seen by the periodic blooms of the dinoflagellate Prorocentrum minimum, which was significantly correlated with decreased salinity and water-column CO2 (McLennan et al. 2021). Multiple species of potentially harmful phytoplankton taxa have been documented at Woolooware Bay, close to oyster production leases (Ajani et al. 2013). Species producing harmful toxins are also known to appear 10–30 times per year at detectable abundances (Ajani et al. 2013) and high biomass blooms of other phytoplankton species (Murray and Suthers 1999; McLennan et al. 2021) are also common. Blooms of Alexandrium pacificum (as A. catenella), which produce the paralytic shellfish toxin (PST), occur regularly in Gamay (Murray et al. 2011).
In coastal waterways such as Gamay, planktonic bacteria are important regulators of chemical and nutrient cycling at the base of the foodweb (Falkowski et al. 2008), but also have the potential to be indicators of contamination (Newton et al. 2011). Whereas bacterial communities associated with macrophytes and sediments have been extensively characterised in Gamay (Burke et al. 2011; Sun et al. 2012, 2013; Phelps et al. 2021), planktonic bacterial communities have not been widely examined. One exception is the work by Carney et al. (2019), who performed time-series analysis of bacterial communities at Foreshore Beach and found that 388 bacterial operational taxonomic units (OTUs), spanning 35 different bacterial genera were present in these communities.
Artificial substrates
In line with urbanised estuaries globally, Gamay has been extensively modified through the addition of a large amount of artificial substrate to both the main bay and its associated river systems (e.g. breakwalls, groynes, piers, seawalls, pontoons). Estimates from the early 1980s put 20% of Gamay’s shoreline as ‘hardened’ by man-made infrastructure (State Pollution Control Commission 1981a). More recent estimates by the authors of this review using satellite images available from Google Earth suggest that ~40% of Gamay’s shoreline, excluding Georges and Cook rivers, has now been modified through the addition of artificial substrate. Seawalls are the most common form of shoreline ‘hardening’, comprising 20% of the main bay shoreline, followed by revetments (12%). Recent estimates of artificial structures in Gamay made by the NSW Department of Primary Industries (2020b) found a total of 33 jetties and pontoons in the main bay, and 39 rock groynes, which occupy an area of 22 513 and 32 196 m2 respectively. In total, 380 artificial reef units have also been added to the main bay (see below).
The ecology of artificial substrates in Gamay has not been reviewed before. Only 18 studies investigating artificial substrates in Gamay have been published (3.1% of studies, Fig. 2), of which most have focused on fish assemblages and how artificial reefs or breakwaters have influenced them. Results from these studies have been mixed (Burchmore et al. 1985; Fowler and Booth 2013; Porter et al. 2018). Assemblages were found to differ between smaller rock groynes and sandstone reefs of a similar size, driven largely by the subadult life stages of several species (Fowler and Booth 2013). By contrast, mid-water piscivores were found to be more abundant on a large revetment wall than on nearby natural rocky reefs (Porter et al. 2018). In contrast to other assemblage parameters, diversity was found to be similar between human-made rock walls and natural reefs in both studies that investigated it (Fowler and Booth 2013; Porter et al. 2018). Only two papers have studied the fouling assemblages on artificial habitats in Gamay (Knott et al. 2004; Mckenzie et al. 2011), which is a small number compared with the neighbouring estuary, Sydney Harbour, which has >20 studies on artificial habitats. Knott et al. (2004) found that many fouling invertebrate taxa showed clear differences regarding their cover between natural rocky reefs and concrete breakwalls in Gamay. No published studies have investigated the importance of intertidal and supra-littoral parts of concrete breakwalls and groynes in Gamay to shorebirds and fur-seals, despite anecdotal evidence that these structures in some parts of Gamay are regularly utilised (e.g. Taylor 2015).
In 2002, Gamay was declared a recreational fishing haven, ending over a century of commercial fishing. In response to this, NSW Department of Primary Industries Fisheries deployed artificial reef balls (0.5 m tall, 80 kg, footprint of 0.45 m2) to enhance rocky substrate and recreational fishing opportunities in Gamay. These were mostly deployed at the mouth of Yarra Bay, east of Bare Island at a depth of 5–10 m, providing ~80 m2 of artificial substrate (30-m3 reef volume) to the bay, representing a ~16% increase in area (Folpp et al. 2020). Since then, a further 200 reef balls have been deployed west of Bare Island. Reef balls have been deployed in multiple NSW estuaries, and associated fish communities have been found to differ among estuaries, (Folpp et al. 2020; Goddard et al. 2022), largely owing to the proximity of the deployment location to the estuary entrance. In Gamay, nearly half of fish biomass associated with reefs balls is based on plankton (the rest on algal or benthic production), which is similar to the adjacent coastal rocky reefs (Truong et al. 2017; Holland et al. 2020). This contrasts with Lake Macquarie where reef ball-associated fish biomass is more evenly distributed among plankton, macrophytes and detritus. Epibenthic assemblages have been assessed on Mini Bay Reef Balls in Gamay, with a total of 16 taxa found, higher than on reef balls deployed in both Lake Macquarie and Georges Basin (Mckenzie et al. 2011).
Freshwater-associated habitats
Gamay is fed by two main freshwater tributaries, Georges River and the Cooks River (Fig. 3). The larger of the two is the Georges River, which is ~96 km long, with a catchment of ~960 km2 and a discharge of >300 GL year−1 (Kingsford and Suthers 1996). The smaller Cooks River is just 23 km long, with a catchment size of 109 km2 and a discharge of ~200 GL year−1 (Kingsford and Suthers 1996). The Cooks River is mostly estuarine and is composed primarily of concrete drainage ditches and pipes (89% artificial). By contrast, the Georges River is estuarine for nearly half its length up to the Liverpool Weir, with its upper reaches being freshwater (Reid 2020). The freshwater wetlands around Gamay were used as a source of freshwater for industry from 1859 up until 1889, after which these areas dried up as a result of overuse (Sydney Metropolitan Catchment Management Authority 2011). Significant freshwater regulation still occurs in the Gamay catchment today, with water capture and regulation for drinking water, agriculture and industry occurring at several locations.
Little research exists on the communities associated with Gamay’s freshwater habitats. However, routine monitoring by the Cooks River Alliance and the Georges River Keeper council groups does collect data on water quality, benthic diatoms and macroinvertebrates. The endangered Macquarie Perch is also known to occur in the Georges River (Faulks et al. 2011). Other native freshwater fish reported to occur in the river include several species of gudgeons (Hammer et al. 2019), galaxids, smelt, Australian bass (Harris 1985), as well as long- and short-finned eels. Mullet also somehow manages to pass through the Liverpool weir in the Georges River, and make it their way into the rivers’ freshwater reaches. The river contains multiple fish ways but fish passage for anadromous and catadromous fishes is of concern. Much more research is needed into these freshwater fishes. Platypus can still be found in the Gamay catchment but are restricted to an area near Campbeltown (Hawke et al. 2019). Freshwater mussels are documented to historically have occurred in the Georges and Woronora rivers (McMichael and Hiscock 1958), and were an important food source for First Nations people of Gamay (Attenbrow 2010b). There are also reports of First Nations people of Gamay sourcing eels and wood-boring worms, which they called cahbro, from the upper reaches of the river (Attenbrow 2010b).
Research gaps for the habitats and biological communities of Gamay
So as to identify areas where fundamental research is lacking, we cross-referenced the number of publications on different taxa within each habitat type (Fig. 7). Across most habitat types, invertebrates and fish were the most thoroughly studied taxa (Fig. 7), with invertebrates on rocky shore habitats being an area of exceptionally high research effort. Much of this research was undertaken at the Cape Banks Aquatic Reserve (designated as a Scientific Marine Research Area in the 1970s). Mangrove and saltmarsh habitats are the most thoroughly studied habitat across the different taxon categories, with much of this research having been undertaken at the Ramsar-listed Towra Point Aquatic Reserve. However, how these habitat-formers interact with other habitats in Gamay remains largely unknown (Fig. 7). Although oysters and oyster reefs are an area of high research effort, the interaction of oysters with other habitat types remains a major knowledge gap (Fig. 7). The study of interactions among more than one taxon or more than one habitat type (multiple categories, Fig. 7) is also a major knowledge gap for Gamay, with most studies considering individual taxa and habitats in isolation only (Fig. 7).
Discussion, knowledge gaps and opportunities
As summarised above, there is an extensive body of published literature covering the various habitats and species of Gamay. However, the bay represents a large and complex ecosystem and we have identified a number of themes for future work on the basis of the lack of studies in some core areas, as well as highlighting areas of opportunity for research in Gamay into the future.
Human activities and development in Gamay
Gamay has and continues to see a rich array of human activities and although a thorough review of threats and impacts to Gamay is outside the scope of the present study (see Stelling-Wood et al. (2022) for more information on threats to Gamay), here we briefly discuss a few that relate most directly to the ecological topics covered in this review. There is a long history of contamination in Gamay, including a legacy of industrial discharges affecting benthic sediments (Reid 2020) and stormwater runoff into main channels and eventually the bay (Tippler et al. 2012). Whereas the contamination status of Gamay has been well documented for sediments (e.g. Brown and Maher 1992; Birch 1996; Hayes et al. 1998; Spooner et al. 2003; Albani and Kollias 2005) and is moderately well documented in terms of water contamination (e.g. Carney et al. 2019, 2020), contamination impacts on other habitats and their cumulative or synergistic effects remain largely unstudied. Gamay has also been subject to extensive modification of habitats though dredging, land reclamation and shoreline hardening in the years since European settlement. The impacts of these processes have predominantly been studied in artificial (e.g. Clark et al. 2015; Porter et al. 2018) and soft sediment habitats (e.g. Jones 1981; Fraser et al. 2006), with little consideration given to how other habitats and communities in Gamay may be affected.
As a focus of intensive shipping activity combined with increasing habitat modification (e.g. the recent port expansion and proposed ferry wharves) resulting in more artificial structures, Gamay is also vulnerable to marine invasive species. Although we know that artificial structures are often preferentially colonised by invasive species (Glasby et al. 2007; Dafforn et al. 2012), there is little information on this in Gamay, representing an important knowledge gap (but see Pollard and Pethebridge 2002; Piola and Johnston 2006; Murray and Keable 2013). The extensively modified nature and well-connectedness of Gamay also makes it highly susceptible to pathogens and disease (Glasby and Lobb 2008). Harmful algal blooms are known to occur in Gamay and have the potential to poison seafood or cause fish and invertebrate mortalities (Murray and Suthers 1999; Ajani et al. 2013; McLennan et al. 2021); however, we have little information on which to assess their ongoing threat. Last, the bay is the focus of intensive recreational fishing activity with anecdotal suggestions of large changes to the assemblage of catch over the past 20 years. However, the only comprehensive survey of Gamay fishes predates these observations (State Pollution Control Commission 1981b, 1981c, 1981d) and, as such, the extent to which such changes are real or may be attributable to fishing v. changes to habitats or environmental conditions remains unknown.
Long-term trends
Effective management requires a robust knowledge of environmental change over time. This includes the cause of change and its consequences, both those that are presently perceived as well as those possible in the future (Lindenmayer et al. 2012). Long-term data sets are invaluable to show changes to the environment in response to short- and long-term perturbations. Similar to Sydney Harbour (Johnston et al. 2015), few studies published on Gamay have involved time-series or temporal studies beyond a few years and thus we identify this as a significant knowledge gap and opportunity to improve our understanding of the bay. However, one exception to this is macrophyte cover in Gamay. The availability of early maps and aerial photos from as far back as 1882 has motivated several studies on changes in macrophyte cover over time in Gamay. These studies have documented large declines in the cover of seagrass and saltmarsh, whereas the cover of mangroves has increased (NSW Department of Primary Industries 2020a; Mitchell and Adam 1989a; Larkum and West 1990; Saintilan and Williams 1999, 2000; West and Glasby 2022; NSW Department of Primary Industries, unpubl. data, 1972, 1986). Long-term sea urchin monitoring records (1996–2013) have also been used to assess temperature-driven increases in the prevalence of sea urchin disease in Gamay (Sweet et al. 2016).
Although few studies have investigated long-term trends in Gamay, several long-term datasets do exist for the bay. Regular water monitoring is undertaken by Beachwatch at popular swimming sites in Gamay and the Georges River every 6 days throughout the summer swimming season (October–April), and monthly during winter (May–September). As part of the Australian Microbiome coastal project conducted by Bioplatforms Australia and the Integrated Marine Observing System, pelagic plankton and bacterial samples have also been collected fortnightly at a site close to Quibray Bay for the past 5 years (McLennan et al. 2021). In addition to this, a real-time temperature and salinity sensor has also been deployed close to the sampling site, generating publicly available data.
Where long-term data sets do not exist, Gamay’s rich history of field surveys and experiments could provide a unique opportunity to assess long-term impacts through the use of techniques such as modelling and meta-analyses. A proliferation of citizen science programs in the past decade has also increased the availability of datasets that cover large temporal or spatial scales (Devictor et al. 2010). These datasets have immense potential for scientists and managers because they often cover scales difficult to achieve in small research groups (Silvertown 2009). The Cape Solander Whale Migration Study (CSWMS), which operates in Gamay, involves volunteers recording humpback whale numbers from Cape Solander in the Gamay Botany Bay National Park and has been active for the past 20 years. Data collected by the CSWMS has permitted scientists to estimate a 10% year−1 increase in the number of humpback whales occurring off Sydney over the past 20 years (Pirotta et al. 2020). Another example is the Australasian Fishes project on the iNaturalist database, which involves submission of fish photos by citizens, which can then be verified by other users and experts (DiBattista et al. 2021). Over 70 000 images have already been submitted, with a large number from Gamay. Increased use of available citizen science datasets for scientific research in Gamay, as well as the development of more citizen science programs, represent a significant area of opportunity for Gamay into the future.
Seascape connectivity
Water-mediated movement of energy, propagules and nutrients is essential for the ecological integrity of estuaries (Pringle 2003). Few studies have investigated Gamay’s hydrology, despite human modifications significantly altering water flow within the bay. Artificial structures can disrupt the movement of water across seascapes, with the potential to influence connectivity at multiple scales (Bishop et al. 2017). These structures can act as physical barriers, preventing movement, but also have the potential to facilitate connectivity by providing channels through which movement can occur (Bishop et al. 2017). Climate change and urbanisation mean that artificial structures are being added to coastal habitats at an increasing rate (Ross and Adam 2013; Firth et al. 2020) and Gamay is no exception to this (see Artificial substrates). This makes it more important than ever to understand connectivity in this coastal waterway and how the addition of artificial substrates might influence it. Additionally, Gamay’s connection to the ocean and the influence of adjacent rapid ocean warming (Malan et al. 2021; Li et al. 2022) requires long-term datasets and further investigation to understand warming trends and its ecological implications within the embayment.
Habitat loss and increased habitat fragmentation in urban estuaries such as Gamay make it particularly important to understand how habitats and communities are connected. Unfortunately, in Gamay this information is patchy, with few studies considering more than one habitat or more than one community. One exception to this is the importance of vegetated habitats for fish movement, which has been well documented (Mazumder et al. 2011; Saintilan and Mazumder 2017). At Towra Point, several fish species are known to move from seagrass beds into mangrove and saltmarsh habitat during the high tide (Saintilan et al. 2007). However, information on present-day fish assemblages in Gamay is lacking and, as such, it is unclear whether these habitat inter-dependencies are still important. This information will be necessary if we are to understand the degree to which bay resilience depends on this, both now and into the future. The configuration of habitats, such as proximity to habitat-forming species and rocky reefs, and the connectivity among these habitats will be likely to influence sediment infauna biodiversity and functioning; however, little is known about this in Gamay (but see, Bugnot et al. 2022). Similarly, it remains largely unknown how the productivity of mudflats at Ramsar-listed Towra Point and nearby soft-sediment habitats are influenced by adjacent oyster reefs, despite this area being important foraging ground for endangered and migratory shorebirds. The contribution of oyster reefs to the present-day fisheries productivity is also unknown, despite the fact that this information will be important to ensure effective fisheries management of the bay as a designated recreational fishing haven. A thorough understanding of seascape connectivity is vital for scientists and managers as they look to find ways to effectively mediate and reverse the negative effects that humans have had in Gamay through conservation, restoration and effective fisheries management.
Habitat restoration and remediation
A legacy of extensive urbanisation has led to the degradation and loss of many habitats and communities in Gamay. Seagrass restoration is becoming increasingly viable and is seen as a crucial management tool for addressing seagrass losses (Sinclair et al. 2021). There have been some encouraging results from recent seagrass transplantation projects in Gamay. Restoration by NSW Department of Primary Industries at Kurnell found that numbers of transplanted P. australis shoots doubled over 8.5 years. This, coupled with extensive vegetative regrowth from the surrounding meadow into the rehabilitated sediments, resulted in the restoration of ~600 m2 of P. australis, albeit at a reduced density compared with natural populations (T. M. Glasby, pers. comm.). However, the success of rhizome transplants in deeper (3–4 m) parts of the meadow was substantially reduced relative to the shallower areas (2–3 m), suggesting that there are still gaps in our understanding of the mechanisms that regulate the success of restoration efforts. Seagrass and saltmarsh restoration has also been undertaken on Gamay’s northern shoreline as part of the Port Botany expansion Penrhyn Estuary habitat enhancement plan (Cardno 2019). Positive results here suggest potential opportunities for creating or restoring habitats in other areas in Gamay, provided that the threats to seagrass have first been removed.
The first large-scale oyster-reef restoration project in Gamay and the Georges River was recently established in 2021 by the Nature Conservancy, Greater Sydney Local Land Services and NSW Department of Primary Industries. Whereas at the time of writing, restoration work is yet to begin, the project’s long-term aim is to restore up to 50 000 m2 of the locally extinct Australian flat oyster (O. angasi) and depleted Sydney rock oyster (S. glomerata) reefs, across four sites, namely, Kurnell, Taren Point, Coronation Bay and Audrey Bay flat (The Nature Conservancy 2022). Through this restoration work, the project hopes to increase biodiversity, support threatened and endangered species, improve water quality, reduce erosion and enhance local fisheries in Gamay. However, disease and parasites (e.g. mudworm, QX and winter mortality) have historically plagued oyster populations in Gamay and could pose significant threats to the success of oyster-reef restoration efforts. Currently, little is known about the source or transfer of these pathogens in the bay, making this oyster-reef restoration project an acid test of how well we understand oyster-reef ecology in Gamay. Many of the restoration projects currently underway in Gamay represent pioneering work, providing significant opportunities to trial management strategies and draw on TEK and the stewardship of First Nations people in Gamay. However, flow-on effects from these projects will be inevitable and, consequently, for these projects to be successful, more needs to be known about species-specific habitat dependencies as well as transfers and linkages among different habitats.
Traditional ecological knowledge of First Nations people of Gamay
First Nations people of Gamay have lived off the lands and waters of Gamay and its tributaries for tens of thousands of years, acquiring extensive knowledge and experience over this time period (captured as TEK). Because of the nature of First Nations’ cultures in Australia being predominantly aural, where knowledge is verbally handed down through generations, colonial practices involving the separation and relocation of families away from their country, and the prohibition of their cultural practices have served to disrupt the transmission of TEK, meaning that much of it has been lost. In situations where TEK has been passed on, it remains largely undocumented in written formats, and in cases where TEK has been documented, it is often recorded as anecdotal observations by early European settlers, lacking important detail such as location, dates, organisms, practices etc., making it difficult to use such information in scientific research. This has meant that little is reported about Gamay prior to European settlement and in the early years post-European settlement.
The value of TEK for science and management has only recently begun to be realised. However, its recognition is paving the way for collaborative efforts among scientists, managers and First Nations people of Australia, creating opportunities to advance knowledge and understanding, and share power and responsibilities among these different groups (Bohensky et al. 2013). Such collaborations can facilitate the development of novel approaches to environmental management (Houde 2007). However, it is important to note that collaborations among scientists, managers and First Nations people need to be respectful. TEK from First Nations people should not be taken from the community without consent, changed or used disrespectfully. This means that the rights of this knowledge must remain with the community and cannot be owned or copyrighted by any academic space. By forming meaningful collaborations with communities, showing respect and acknowledgement of this knowledge, and letting those community elders say how the knowledge they have shared can be used, we can generate a positive space for sharing among communities, scientists and managers.
Artificial substrates as habitat
Artificial substrates are one of the few habitat types in Gamay that are increasing in extent, yet, despite this, they remain poorly studied. Studies that do exists tend to be small scale. For example, of the three studies investigating fish assemblages associated with artificial structures, all have been undertaken on the northern shoreline of Gamay (Burchmore et al. 1985; Fowler and Booth 2013; Porter et al. 2018). The superficial nature of this research in Gamay limits our understanding of the significance of these habitats, and instead there needs to be greater focus on artificial substrates right across the bay and associated rivers, with a particular emphasis on the influence of artificial substrate on the ecology of Gamay. This information is important to understand the type and magnitude of ecological change that may already have occurred because of the addition of artificial substrates to Gamay, as well as to predict future change if further substrate is added. A first step in achieving this would be a to document the amount and distribution of artificial substrates within the bay and rivers, followed by comprehensive faunal survey on all major structure types.
Eco-engineering interventions, such as Living Seawalls (www.livingseawalls.com.au), offer the opportunity to potentially increase the functioning of artificial-substrate habitat by increasing structural complexity for the purpose of enhancing biodiversity. Although such interventions are rarely equivalent to natural habitat, at locations where the construction of infrastructure is unavoidable, such interventions can act to minimise the negative ecological effects of such developments (Strain et al. 2018). Given the extent of artificial substrates in Gamay, such approaches have the potential to increase local biodiversity, improve water quality, increase fish productivity and sequester carbon. However, the implementation of such interventions is influenced by permitting requirements and other factors, which in Gamay limit their widespread use.
Conclusions
Gamay is a place of enormous ecological and social value, making it one of the most well-studied coastal waterways in Australia. Yet, its complexity has meant that the extensive research that has been undertaken in Gamay represents just the baseline of what is needed to effectively manage the bay. This review summarised current ecological knowledge on Gamay, and identified key areas where knowledge is absent or lacking. Filling these knowledge gaps should be of the utmost importance for scientists and managers, to ensure we manage this iconic ecosystem for all its residents, including both the people that live along its shorelines and the aquatic communities that call its waters home. Below we highlight what we feel are the five foremost research priorities for Gamay to ensure its sustainable future.
Understanding Gamay’s hydrology
Water movement is important for the exchange of resources within and between waterways; however, little is known about the hydrology of Gamay, including how the bay influences its freshwater tributaries (Georges and Cooks rivers) and vice versa. Similarly, the continual modification of the shoreline of the bay (construction of rock groynes and the recent expansion of Port Botany) has potentially affected patterns of water flow and sand movement in the bay, warranting further investigation. This information will be vital to ensure a sustainable future for the bay in the face of increased environmental instability owing to climate change and intensifying pressures from urbanisation.
Promoting long-term datasets
Long-term, continuous datasets remain an important research priority for Gamay, both in terms of maintaining the few that currently exist, as well as establishing new datasets and sustaining them into the future. The longer these datasets exist, the more valuable they will become, especially for documenting trends, representing an invaluable resource for researchers and decision makers.
Artificial substrates and ecosystem functioning
With several development plans for Gamay currently being considered by the NSW Government, understanding the role artificial substrates play in Gamay’s ecology is more important than ever. This includes their role as creators of novel habitat, as well as direct (e.g. habitat loss) and indirect (e.g. sediment resuspension driven contamination) impacts associated with their implementation. A lot of uncertainty surrounds the potential indirect effects of artificial structures in marine systems because of difficulties in linking past activities (e.g. legacy contamination) with present- day processes (e.g. dredging), highlighting this as a particularly important research priority for Gamay into the future.
Habitat loss, restoration and remediation research
Disturbance events such as contamination and habitat modification are commonly associated with large-scale loss of habitat in Gamay. However, the mechanisms driving the loss of habitat are not always clear, and more research is necessary if we are to understand how these processes might be linked and to prevent further loss. In Gamay, small-scale habitat-restoration efforts have shown promising results and could provide opportunities to improve affected habitats in the future, provided that the disturbances causing those losses have first been removed. However, much more research is required before these projects can be successfully up-scaled to ecologically significant levels.
The inclusion of TEK from First Nations people of Gamay
The inclusion of TEK from First Nations people into science and management is often hindered by a lack of written documentation, for previously mentioned reasons. Gamay is no exception to this, despite the fact that TEK from First Nations people of Gamay represents the only source of ecological information about Gamay prior to arrival of Europeans in Australia. Consequently, a process of co-governance and co-management collaboration between the First Nations people of Gamay and scientists is vital to ensure that valuable TEK is included in science, management and restoration decisions about Gamay.
In addition to highlighting areas where research is lacking, this review has also determined the numerous opportunities that current investigations have generated, creating paths that stakeholders and managers can co-design and work toward a sustainable future for the bay. However, the success of many of these opportunities will rely on cooperation and collaboration, not only between scientists and managers, but also among the multitude of other stakeholder groups (e.g. First Nations people of Gamay, government agencies, commercial operators, recreational fishing groups, local councils, community groups, residents) that use and care for the bay. For it is only through cooperation, collaboration and partnerships among stakeholders that a sustainable future for Gamay can truly be realised.
Data availability
All literature included in this literature review are listed in supplementary material.
Conflicts of interest
M. Bishop and B. P. Kelaher are associate editors for Marine and Freshwater Research but did not at any stage have editor-level access to this manuscript while in peer review, as is the standard practice when handling manuscripts submitted by an editor to this journal. Marine and Freshwater Research encourages its editors to publish in the journal and they are kept totally separate from the decision-making processes for their manuscripts. The authors have no further conflicts of interest to declare.
Acknowledgements
We acknowledge and pay respects to the traditional custodians of the First Nations Lands and Sea Country on which the research included in this literature review was conducted. We acknowledge and pay respects to the generations of First Nations ancestors who have cared for and protected Gamay for tens of thousands of years and to the Elders and community who continue this tradition now and into the future. We acknowledge the importance and connection of Gamay to the lives of First Nations people.
References
Adam P (1997) Introduction to the Botany Bay symposium. Wetlands Australia 16, 1-3.
| Google Scholar |
Ajani P, Brett S, Krogh M, Scanes P, Webster G, Armand L (2013) The risk of harmful algal blooms (HABs) in the oyster-growing estuaries of New South Wales, Australia. Environmental Monitoring and Assessment 185, 5295-5316.
| Crossref | Google Scholar |
Albani AD (1981) Sedimentary environments and Pleistocene chronology of the Botany Basin, NSW, Australia. Geo-Marine Letters 1, 163-167.
| Crossref | Google Scholar |
Allan SJ, O’Connell MJ, Harasti D, Klanten OS, Booth DJ (2022a) Searching for seadragons: predicting micro-habitat use for the common (weedy) seadragon (Phyllopteryx taeniolatus) based on habitat and prey. Journal of Fish Biology 100, 935-943.
| Crossref | Google Scholar |
Allan SJ, O’Connell MJ, Harasti D, Klanten OS, Booth DJ (2022b) Space use by the endemic common (weedy) seadragon (Phyllopteryx taeniolatus): influence of habitat and prey. Journal of Fish Biology 100, 175-183.
| Crossref | Google Scholar |
Allen S, Marsh H, Hodgson A (2004) Occurrence and conservation of the dugong (Sirenia: Dugongidae) in New South Wales. Proceedings of the Linnean Society of New South Wales 125, 211-216.
| Google Scholar |
Alleway HK, Connell SD (2015) Loss of an ecological baseline through the eradication of oyster reefs from coastal ecosystems and human memory. Conservation Biology 29, 795-804.
| Crossref | Google Scholar |
Andrew NL (1991) Changes in subtidal habitat following mass mortality of sea urchins in Botany Bay, New South Wales. Australian Journal of Ecology 16, 353-362.
| Crossref | Google Scholar |
Andrew NL, Underwood AJ (1989) Patterns of abundance of the sea urchin Centrostephanus rodgersii (Agassiz) on the central coast of New South Wales, Australia. Journal of Experimental Marine Biology and Ecology 131, 61-80.
| Crossref | Google Scholar |
Arrontes J, Underwood AJ (1991) Experimental studies on some aspects of the feeding ecology of the intertidal starfish Patiriella exigua. Journal of Experimental Marine Biology and Ecology 148, 255-269.
| Crossref | Google Scholar |
Barbosa SS, Klanten SO, Puritz JB, Toonen RJ, Byrne M (2013) Very fine-scale population genetic structure of sympatric asterinid sea stars with benthic and pelagic larvae: influence of mating system and dispersal potential. Biological Journal of the Linnean Society 108, 821-833.
| Crossref | Google Scholar |
Bell JD, Pollard DA (1989) Ecology of fish assemblages and fisheries associated with seagrasses. In ‘Biology of seagrasses: a treatise on the biology of seagrasses with special reference to the Australian region’. (Eds AJ McComb, AWD Larkum, SA Shephard) pp. 565–609. (Elsevier: Amsterdam, Netherlands)
Birch GF (1996) Sediment-bound metallic contaminants in Sydney’s estuaries and adjacent offshore, Australia. Estuarine, Coastal and Shelf Science 42, 31-44.
| Crossref | Google Scholar |
Bishop MJ, Kelaher BP (2008) Non-additive, identity-dependent effects of detrital species mixing on soft-sediment communities. Oikos 117, 531-542.
| Crossref | Google Scholar |
Bishop MJ, Kelaher BP (2013) Context-specific effects of the identity of detrital mixtures on invertebrate communities. Ecology and Evolution 3, 3986-3999.
| Crossref | Google Scholar |
Bishop MJ, Morgan T, Coleman MA, Kelaher BP, Hardstaff LK, Evenden RW (2009) Facilitation of molluscan assemblages in mangroves by the fucalean alga Hormosira banksii. Marine Ecology Progress Series 392, 111-122.
| Crossref | Google Scholar |
Bishop MJ, Byers JE, Marcek BJ, Gribben PE (2012) Density-dependent facilitation cascades determine epifaunal community structure in temperate Australian mangroves. Ecology 93, 1388-1401.
| Crossref | Google Scholar |
Bishop MJ, Mayer-Pinto M, Airoldi L, Firth LB, Morris RL, Loke LH, Hawkins SJ, Naylor LA, Coleman RA, Chee SY, Dafforn KA (2017) Effects of ocean sprawl on ecological connectivity: impacts and solutions. Journal of Experimental Marine Biology and Ecology 492, 7-30.
| Crossref | Google Scholar |
Bohensky EL, Butler JRA, Davies J (2013) Integrating indigenous ecological knowledge and science in natural resource management: perspectives from Australia. Ecology and Society 18, 20.
| Crossref | Google Scholar |
Brodersen KE, Nielsen DA, Ralph PJ, Kühl M (2014) A split flow chamber with artificial sediment to examine the below-ground microenvironment of aquatic macrophytes. Marine Biology 161, 2921-2930.
| Crossref | Google Scholar |
Brown G, Maher W (1992) The occurrence, distribution and sources of polycycic aromatic hydrocarbons in the sediments of the Georges River estuary, Australia. Organic Geochemistry 18, 657-668.
| Crossref | Google Scholar |
Bugnot AB, Dafforn KA, Coleman RA, Ramsdale M, Gibbeson JT, Erickson K, Vila-Concejo A, Figueira WF, Gribben PE (2022) Linking habitat interactions and biodiversity within seascapes. Ecosphere 13, e4021.
| Crossref | Google Scholar |
Burchmore JJ, Pollard DA, Bell JD, Middleton MJ, Pease BC, Matthews J (1985) An ecological comparison of artificial and natural rocky reef fish communities in Botany Bay, New South Wales, Australia. Bulletin of Marine Science 37, 70-85.
| Google Scholar |
Burke C, Thomas T, Lewis M, Steinberg P, Kjelleberg S (2011) Composition, uniqueness and variability of the epiphytic bacterial community of the green alga Ulva australis. The ISME Journal 5, 590-600.
| Crossref | Google Scholar |
Carney RL, Labbate M, Siboni N, Tagg KA, Mitrovic SM, Seymour JR (2019) Urban beaches are environmental hotspots for antibiotic resistance following rainfall. Water Research 167, 115081.
| Crossref | Google Scholar |
Carney RL, Brown MV, Siboni N, Raina J-B, Kahlke T, Mitrovic SM, Seymour JR (2020) Highly heterogeneous temporal dynamics in the abundance and diversity of the emerging pathogens Arcobacter at an urban beach. Water Research 171, 115405.
| Crossref | Google Scholar |
Carter L (2020) Little penguin. Scientific name: Eudyptula minor. (Australian Museum) Available at https://australian.museum/learn/animals/birds/little-penguin-eudyptula-minor/ [Verified 3 July 2023]
Chapman MG, Underwood AJ (1996) Experiments on effects of sampling biota under intertidal and shallow subtidal boulders. Journal of Experimental Marine Biology and Ecology 207, 103-126.
| Crossref | Google Scholar |
Christensen NL, Bartuska AM, Brown JH, Carpenter S, D’antonio C, Francis R, Franklin JF, Macmahon JA, Noss RF, Parsons DJ, Peterson CH, Turner MG, Woodmansee RG (1996) The report of the Ecological Society of America committee on the scientific basis for ecosystem management. Ecological Applications 6, 665-691.
| Crossref | Google Scholar |
Clark GF, Kelaher BP, Dafforn KA, Coleman MA, Knott NA, Marzinelli EM, Johnston EL (2015) What does impacted look like? High diversity and abundance of epibiota in modified estuaries. Environmental Pollution 196, 12-20.
| Crossref | Google Scholar |
Coleman MA, Kelaher BP, Steinberg PD, Millar AJK (2008) Absence of a large brown macroalga on urbanized rocky reefs around Sydney, Australia, and evidence for historical decline. Journal of Phycology 44, 897-901.
| Crossref | Google Scholar |
Courtenay GC, Gladstone W, Schreider M (2005) Assessing the response of estuarine intertidal assemblages to urbanised catchment discharge. Environmental Monitoring and Assessment 107, 375-398.
| Crossref | Google Scholar |
Creese RG (1982) Distribution and abundance of the acmaeid limpet, Patelloida latistrigata, and its interaction with barnacles. Oecologia 52, 85-96.
| Crossref | Google Scholar |
Creese RG, Underwood AJ (1982) Analysis of inter- and intra-specific competition amongst intertidal limpets with different methods of feeding. Oecologia 53, 337-346.
| Crossref | Google Scholar |
Dafforn KA, Glasby TM, Johnston EL (2012) Comparing the invasibility of experimental ‘reefs’ with field observations of natural reefs and artificial structures. PLoS ONE 7, e38124.
| Crossref | Google Scholar |
Dafforn KA, Baird DJ, Chariton AA, Sun MY, Brown MV, Simpson SL, Kelaher BP, Johnston EL (2014) Faster, higher and stronger? the pros and cons of molecular faunal data for assessing ecosystem condition. Advances in Ecological Research 51, 1-40.
| Crossref | Google Scholar |
Davis A, Roberts D, Cummins S (1997) Rapid invasion of a sponge-dominated deep-reef by Caulerpa scalpelliformis (Chlorophyta) in Botany Bay, New South Wales. Australian Journal of Ecology 22, 146-150.
| Crossref | Google Scholar |
Denley EJ, Underwood AJ (1979) Experiments on factors influencing settlement, survival, and growth of two species of barnacles in New South Wales. Journal of Experimental Marine Biology and Ecology 36, 269-293.
| Crossref | Google Scholar |
Devictor V, Whittaker RJ, Beltrame C (2010) Beyond scarcity: citizen science programmes as useful tools for conservation biogeography. Diversity and Distributions 16, 354-362.
| Crossref | Google Scholar |
Dexter DM (1984) Temporal and spatial variability in the community structure of the fauna of four sandy beaches in south-eastern New South Wales. Marine and Freshwater Research 35, 663-672.
| Crossref | Google Scholar |
Dexter DM (1985) Distribution and life histories of abundant crustaceans of four sandy beaches of south-eastern New South Wales. Marine and Freshwater Research 36, 281-289.
| Crossref | Google Scholar |
DiBattista JD, West KM, Hay AC, Hughes JM, Fowler AM, McGrouther MA (2021) Community-based citizen science projects can support the distributional monitoring of fishes. Aquatic Conservation: Marine and Freshwater Ecosystems 31, 3580-3593.
| Crossref | Google Scholar |
Dove MC, Nell JA, O’Connor WA (2013) Evaluation of the progeny of the fourth-generation Sydney rock oyster Saccostrea glomerata (Gould, 1850) breeding lines for resistance to QX disease (Marteilia sydneyi) and winter mortality (Bonamia roughleyi). Aquaculture Research 44, 1791-1800.
| Crossref | Google Scholar |
Dunic JC, Brown CJ, Connolly RM, Turschwell MP, Côté IM (2021) Long-term declines and recovery of meadow area across the world’s seagrass bioregions. Global Change Biology 27, 4096-4109.
| Crossref | Google Scholar |
Falkowski PG, Fenchel T, Delong EF (2008) The microbial engines that drive Earth’s biogeochemical cycles. Science 320, 1034-1039.
| Crossref | Google Scholar |
Faulks LK, Gilligan DM, Beheregaray LB (2011) The role of anthropogenic vs. natural in-stream structures in determining connectivity and genetic diversity in an endangered freshwater fish, Macquarie perch (Macquaria australasica). Evolutionary Applications 4, 589-601.
| Crossref | Google Scholar |
Fellowes TE, Vila-Concejo A, Gallop SL, Schosberg R, de Staercke V, Largier JL (2021) Decadal shoreline erosion and recovery of beaches in modified and natural estuaries. Geomorphology 390, 107884.
| Crossref | Google Scholar |
Ferrari J, Goncalves P, Campbell AH, Sudatti DB, Wood GV, Thomas T, Pereira RC, Steinberg PD, Marzinelli EM (2021) Molecular analysis of a fungal disease in the habitat-forming brown macroalga Phyllospora comosa (Fucales) along a latitudinal gradient. Journal of Phycology 57, 1504-1516.
| Crossref | Google Scholar |
Firth LB, Airoldi L, Bulleri F, Challinor S, Chee S-Y, Evans AJ, Hanley ME, Knights AM, O’Shaughnessy K, Thompson RC, Hawkins SJ (2020) Greening of grey infrastructure should not be used as a Trojan horse to facilitate coastal development. Journal of Applied Ecology 57, 1762-1768.
| Crossref | Google Scholar |
Fischer M, Maxwell K, Nuunoq , Pedersen H, Greeno D, Jingwas N, Graham Blair J, Hugu S, Mustonen T, Murtomäki E, Mustonen K (2022) Empowering her guardians to nurture our Ocean’s future. Reviews in Fish Biology and Fisheries 32, 271-296.
| Crossref | Google Scholar |
Folpp HR, Schilling HT, Clark GF, Lowry MB, Maslen B, Gregson M, Suthers IM (2020) Artificial reefs increase fish abundance in habitat-limited estuaries. Journal of Applied Ecology 57, 1752-1761.
| Crossref | Google Scholar |
Fowler AM, Booth DJ (2013) Seasonal dynamics of fish assemblages on breakwaters and natural rocky reefs in a temperate estuary: consistent assemblage differences driven by sub-adults. PLoS ONE 8, e75790.
| Crossref | Google Scholar |
Fraser C, Hutchings P, Williamson J (2006) Long-term changes in polychaete assemblages of Botany Bay (NSW, Australia) following a dredging event. Marine Pollution Bulletin 52, 997-1010.
| Crossref | Google Scholar |
Friess DA (2016) Ecosystem services and disservices of mangrove forests: insights from historical colonial observations. Forests 7, 183.
| Crossref | Google Scholar |
Gall ML, Holmes SP, Dafforn KA, Johnston EL (2013) Differential tolerance to copper, but no evidence of population-level genetic differences in a widely-dispersing native barnacle. Ecotoxicology 22, 929-937.
| Crossref | Google Scholar |
Gallop SL, Vila-Concejo A, Fellowes TE, Harley MD, Rahbani M, Largier JL (2020) Wave direction shift triggered severe erosion of beaches in estuaries and bays with limited post-storm recovery. Earth Surface Processes and Landforms 45, 3854-3868.
| Crossref | Google Scholar |
Gillanders BM, Elsdon TS, Halliday IA, Jenkins GP, Robins JB, Valesini FJ (2011) Potential effects of climate change on Australian estuaries and fish utilising estuaries: a review. Marine and Freshwater Research 62, 1115-1131.
| Crossref | Google Scholar |
Gillies CL, Castine SA, Alleway HK, Crawford C, Fitzsimons JA, Hancock B, Koch P, Mcafee D, McLeod IM, zu Ermgassen PSE (2020) Conservation status of the oyster reef ecosystem of southern and eastern Australia. Global Ecology and Conservation 22, e00988.
| Crossref | Google Scholar |
Glasby TM, Connell SD, Holloway MG, Hewitt CL (2007) Nonindigenous biota on artificial structures: could habitat creation facilitate biological invasions? Marine Biology 151, 887-895.
| Crossref | Google Scholar |
Goddard BK, Becker A, Harasti D, Smith JA, Subramaniam RC, Suthers IM (2022) The trophic basis of fish assemblages in temperate estuarine and coastal ecosystems. Marine Biology 169, 19.
| Crossref | Google Scholar |
Gribben PE, Nielsen S, Seymour JR, Bradley DJ, West MN, Thomas T (2017) Microbial communities in marine sediments modify success of an invasive macrophyte. Scientific Reports 7, 9845.
| Crossref | Google Scholar |
Hammer MP, Adams M, Thacker CE, Johnson JB, Unmack PJ (2019) Comparison of genetic structure in co-occurring freshwater eleotrids (Actinopterygii: Philypnodon) reveals cryptic species, likely translocation and regional conservation hotspots. Molecular Phylogenetics and Evolution 139, 106556.
| Crossref | Google Scholar |
Harris JH (1985) Diet of the Australian bass, Macquaria novemaculeata (Perciformes: Percichthyidae), in the Sydney basin. Marine and Freshwater Research 36, 219-234.
| Crossref | Google Scholar |
Hawke T, Bino G, Kingsford RT (2019) A silent demise: historical insights into population changes of the iconic platypus (Ornithorhynchus anatinus). Global Ecology and Conservation 20, e00720.
| Crossref | Google Scholar |
Haworth RJ, Baker RGV, Flood PJ (2004) A 6000 year-old fossil dugong from botany bay: inferences about changes in Sydney’s climate, sea levels and waterways. Australian Geographical Studies 42, 46-59.
| Crossref | Google Scholar |
Hayes W, Anderson I, Gaffoor M, Hurtado J (1998) Trace metals in oysters and sediments of Botany Bay, Sydney. Science of The Total Environment 212, 39-47.
| Crossref | Google Scholar |
Holland MM, Smith JA, Everett JD, Vergés A, Suthers IM (2020) Latitudinal patterns in trophic structure of temperate reef-associated fishes and predicted consequences of climate change. Fish and Fisheries 21, 1092-1108.
| Crossref | Google Scholar |
Houde N (2007) The six faces of traditional ecological knowledge: challenges and opportunities for Canadian co-management arrangements. Ecology and Society 12, 34.
| Crossref | Google Scholar |
Huggett MJ, Williamson JE, De Nys R, Kjelleberg S, Steinberg PD (2006) Larval settlement of the common Australian sea urchin Heliocidaris erythrogramma in response to bacteria from the surface of coralline algae. Oecologia 149, 604-619.
| Crossref | Google Scholar |
Hughes AR, Gribben PE, Kimbro DL, Bishop MJ (2014) Additive and site-specific effects of two foundation species on invertebrate community structure. Marine Ecology Progress Series 508, 129-138.
| Crossref | Google Scholar |
Jackson G, Forbes P (2018) Oysters on the Georges River. Sutherland Shire Historical Society Inc. Bulletin 201, 31-36.
| Google Scholar |
Johnston EL, Mayer-Pinto M, Hutchings PA, Marzinelli EM, Ahyong ST, Birch G, Booth DJ, Creese RG, Doblin MA, Figueira W, Gribben PE, Pritchard T, Roughan M, Steinberg PD, Hedge LH (2015) Sydney Harbour: what we do and do not know about a highly diverse estuary. Marine and Freshwater Research 66, 1073-1087.
| Crossref | Google Scholar |
Jones G (1981) Effects of dredging and reclamation on the sediments of Botany Bay. Marine and Freshwater Research 32, 369-377.
| Crossref | Google Scholar |
Kelaher BP (2003a) Changes in habitat complexity negatively affect diverse gastropod assemblages in coralline algal turf. Oecologia 135, 431-441.
| Crossref | Google Scholar |
Kelaher BP (2003b) Effects of frond length on diverse gastropod assemblages in coralline turf. Journal of the Marine Biological Association of the United Kingdom 83, 159-163.
| Crossref | Google Scholar |
Kelaher BP, Chapman MG, Underwood AJ (2001) Spatial patterns of diverse macrofaunal assemblages in coralline turf and their associations with environmental variables. Journal of the Marine Biological Association of the United Kingdom 81, 917-930.
| Crossref | Google Scholar |
Kelaher BP, Bishop MJ, Potts J, Scanes P, Skilbeck G (2013) Detrital diversity influences estuarine ecosystem performance. Global Change Biology 19, 1909-1918.
| Crossref | Google Scholar |
Kelaher BP, Coleman MA, Bishop MJ (2018) Ocean warming, but not acidification, accelerates seagrass decomposition under near-future climate scenarios. Marine Ecology Progress Series 605, 103-110.
| Crossref | Google Scholar |
Kingsford MJ, Byrne M (2023) New South Wales rocky reefs are under threat. Marine and Freshwater Research 74, 95-98.
| Crossref | Google Scholar |
Kingsford MJ, Suthers IM (1994) Dynamic estuarine plumes and fronts: importance to small fish and plankton in coastal waters of NSW, Australia. Continental Shelf Research 14, 655-672.
| Crossref | Google Scholar |
Kingsford MJ, Suthers IM (1996) The influence of tidal phase on patterns of ichthyoplankton abundance in the vicinity of an estuarine front, Botany Bay, Australia. Estuarine, Coastal and Shelf Science 43, 33-54.
| Crossref | Google Scholar |
Klanten OS, Gaither MR, Greaves S, Mills K, O’Keeffe K, Turnbull J, McKinnon R, Booth DJ (2020) Genomic and morphological evidence of distinct populations in the endemic common (weedy) seadragon Phyllopteryx taeniolatus (Syngnathidae) along the east coast of Australia. PLoS ONE 15, e0243446.
| Crossref | Google Scholar |
Knott NA, Underwood AJ, Chapman MG, Glasby TM (2004) Epibiota on vertical and on horizontal surfaces on natural reefs and on artificial structures. Journal of the Marine Biological Association of the United Kingdom 84, 1117-1130.
| Crossref | Google Scholar |
Knott NA, Underwood AJ, Chapman MG, Glasby TM (2006) Growth of the encrusting sponge Tedania anhelans (Lieberkuhn) on vertical and on horizontal surfaces of temperate subtidal reefs. Marine and Freshwater Research 57, 95-104.
| Crossref | Google Scholar |
Larkum AWD (1976) Botany Bay: national asset or national disaster. Operculum 5, 67-75.
| Google Scholar |
Larkum AWD, West RJ (1990) Long-term changes of seagrass meadows in Botany Bay, Australia. Aquatic Botany 37, 55-70.
| Crossref | Google Scholar |
Lee WJ, Patterson DJ (2002) Abundance and biomass of heterotrophic flagellates, and factors controlling their abundance and distribution in sediments of Botany Bay. Microbial Ecology 43, 467-481.
| Crossref | Google Scholar |
Li J, Roughan M, Kerry C (2022) Drivers of ocean warming in the western boundary currents of the Southern Hemisphere. Nature Climate Change 12, 901-909.
| Crossref | Google Scholar |
Liggins GW, Kennelly SJ, Broadhurst MK (1996) Observer-based survey of by-catch from prawn trawling in Botany Bay and Port Jackson, New South Wales. Marine and Freshwater Research 47, 877-888.
| Crossref | Google Scholar |
Lindenmayer DB, Likens GE, Andersen A, Bowman D, Bull CM, Burns E, Dickman CR, Hoffmann AA, Keith DA, Liddell MJ, Lowe AJ, Metcalfe DJ, Phinn SR, Russell-Smith J, Thurgate N, Wardle GM (2012) Value of long-term ecological studies. Austral Ecology 37, 745-757.
| Crossref | Google Scholar |
Litchfield SG, Schulz KG, Kelaher BP (2020) The influence of plastic pollution and ocean change on detrital decomposition. Marine Pollution Bulletin 158, 111354.
| Crossref | Google Scholar |
Longford SR, Tujula NA, Crocetti GR, Holmes AJ, Holmström C, Kjelleberg S, Steinberg PD, Taylor MW (2007) Comparisons of diversity of bacterial communities associated with three sessile marine eukaryotes. Aquatic Microbial Ecology 48, 217-229.
| Crossref | Google Scholar |
McGuinness KA (1990) Effects of oil spills on macro-invertebrates of saltmarshes and mangrove forests in Botany Bay, New South Wales, Australia. Journal of Experimental Marine Biology and Ecology 142, 121-135.
| Crossref | Google Scholar |
Mckenzie R, Lowry M, Folpp H, Gregson M (2011) Fouling assemblages associated with estuarine artificial reefs in New South Wales, Australia. Brazilian Journal of Oceanography 59, 107-118.
| Crossref | Google Scholar |
McLennan K, Ruvindy R, Ostrowski M, Murray S (2021) Assessing the use of molecular barcoding and qPCR for investigating the ecology of Prorocentrum minimum (Dinophyceae), a harmful algal species. Microorganisms 9, 510.
| Crossref | Google Scholar |
McLeod IM, Boström-Einarsson L, Creighton C, D’Anastasi B, Diggles B, Dwyer PG, Firby L, Le Port A, Luongo A, Martinez-Baena F, McOrrie S, Heller-Wagner G, Gillies CL (2020) Habitat value of Sydney rock oyster (Saccostrea glomerata) reefs on soft sediments. Marine and Freshwater Research 71, 771-781.
| Crossref | Google Scholar |
McMichael DF, Hiscock ID (1958) A monograph of the freshwater mussels (Mollusca: Pelecypoda) of the Australian region. Australian Journal of Marine and Freshwater Research 9, 372-508.
| Crossref | Google Scholar |
Malan N, Roughan M, Kerry C (2021) The rate of coastal temperature rise adjacent to a warming western boundary current is nonuniform with latitude. Geophysical Research Letters 48, e2020GL090751.
| Crossref | Google Scholar |
Martin J (1865) Explorations in north-western Australia. The Journal of the Royal Geographical Society of London 35, 237-289.
| Crossref | Google Scholar |
Martin BC, Alarcon MS, Gleeson D, Middleton JA, Fraser MW, Ryan MH, Holmer M, Kendrick GA, Kilminster K (2020) Root microbiomes as indicators of seagrass health. FEMS Microbiology Ecology 96, fiz201.
| Crossref | Google Scholar |
Martinez AS, Queiroz EV, Bryson M, Byrne M, Coleman RA (2017) Incorporating in situ habitat patchiness in site selection models reveals that site fidelity is not always a consequence of animal choice. Journal of Animal Ecology 86, 847-856.
| Crossref | Google Scholar |
Martínez-Baena F, Lanham BS, McLeod IM, Taylor MD, McOrrie S, Luongo A, Bishop MJ (2022) Remnant oyster reefs as fish habitat within the estuarine seascape. Marine Environmental Research 179, 105675.
| Crossref | Google Scholar |
Marzinelli EM, Campbell AH, Zozaya Valdes E, Vergés A, Nielsen S, Wernberg T, De Bettignies T, Bennett S, Caporaso JG, Thomas T, Steinberg PD (2015) Continental-scale variation in seaweed host-associated bacterial communities is a function of host condition, not geography. Environmental Microbiology 17, 4078-4088.
| Crossref | Google Scholar |
Mayer-Pinto M, Johnston EL, Hutchings PA, Marzinelli EM, Ahyong ST, Birch G, Booth DJ, Creese RG, Doblin MA, Figueira W, Gribben PE, Pritchard T, Roughan M, Steinberg PD, Hedge LH (2015) Sydney Harbour: a review of anthropogenic impacts on the biodiversity and ecosystem function of one of the world’s largest natural harbours. Marine and Freshwater Research 66, 1088-1105.
| Crossref | Google Scholar |
Mazumder D, Saintilan N, Williams RJ (2009) Zooplankton inputs and outputs in the saltmarsh at Towra Point, Australia. Wetlands Ecology and Management 17, 225-230.
| Crossref | Google Scholar |
Mazumder D, Saintilan N, Williams RJ, Szymczak R (2011) Trophic importance of a temperate intertidal wetland to resident and itinerant taxa: evidence from multiple stable isotope analyses. Marine and Freshwater Research 62, 11-19.
| Crossref | Google Scholar |
Minchinton TE, Ross PM (1999) Oysters as habitat for limpets in a temperate mangrove forest. Australian Journal of Ecology 24, 157-170.
| Crossref | Google Scholar |
Mitchell ML, Adam P (1989a) The decline of saltmarsh in Botany Bay. Wetlands 8(2), 55-60.
| Google Scholar |
Mitchell ML, Adam P (1989b) The relationship between mangrove and saltmarsh communities in the Sydney region. Wetlands 8, 37-46.
| Google Scholar |
Murray A, Keable SJ (2013) First report of Sabella spallanzanii (Gmelin, 1791) (Annelida: Polychaeta) from Botany Bay, New South Wales, a northern range extension for the invasive species within Australia. Zootaxa 3670, 391-395.
| Crossref | Google Scholar |
Murray S, Suthers IM (1999) Population ecology of Noctiluca scintillans Macartney, a red-tide-forming dinoflagellate. Marine and Freshwater Research 50, 243-252.
| Crossref | Google Scholar |
Murray SA, Wiese M, Stüken A, Brett S, Kellmann R, Hallegraeff G, Neilan BA (2011) sxtA-based quantitative molecular assay to identify saxitoxin-producing harmful algal blooms in marine waters. Applied and Environmental Microbiology 77, 7050-7057.
| Crossref | Google Scholar |
Nell JA, Hand RE (2003) Evaluation of the progeny of second-generation Sydney rock oyster Saccostrea glomerata (Gould, 1850) breeding lines for resistance to QX disease Marteilia sydneyi. Aquaculture 228, 27-35.
| Crossref | Google Scholar |
Nell JA, Perkins B (2006) Evaluation of the progeny of third-generation Sydney rock oyster Saccostrea glomerata (Gould, 1850) breeding lines for resistance to QX disease Marteilia sydneyi and winter mortality Bonamia roughleyi. Aquaculture Research 37, 693-700.
| Crossref | Google Scholar |
Newton RJ, Vandewalle JL, Borchardt MA, Gorelick MH, McLellan SL (2011) Lachnospiraceae and Bacteroidales alternative fecal indicators reveal chronic human sewage contamination in an urban harbor. Applied and Environmental Microbiology 77, 6972-6981.
| Crossref | Google Scholar |
Nguyen HM, Ralph PJ, Marín-Guirao L, Pernice M, Procaccini G (2021) Seagrasses in an era of ocean warming: a review. Biological Reviews 96, 2009-2030.
| Crossref | Google Scholar |
NSW Department of Primary Industries (2020a) Fisheries NSW spatial data portal. (NSW DPI) Available at https://webmap.industry.nsw.gov.au/Html5Viewer/index.html?viewer=Fisheries_Data_Portal [Verified 20 December 2021]
NSW Department of Primary Industries (2020b) NSW Estuarine habitat dashboard. (NSW DPI) Available at https://nsw-dpi.shinyapps.io/NSW_Estuarine_Habitat/ [Verified 10 January 2022]
Phelps CM, McMahon K, Bissett A, Bernasconi R, Steinberg PD, Thomas T, Marzinelli EM, Huggett MJ (2021) The surface bacterial community of an Australian kelp shows cross-continental variation and relative stability within regions. FEMS Microbiology Ecology 97, fiab089.
| Crossref | Google Scholar |
Piercey RS, Gribben PE, Hanley TC, Moles AT, Hughes AR (2021) Incorporating marine macrophytes in plant–soil feedbacks: emerging evidence and opportunities to advance the field. Journal of Ecology 109, 614-625.
| Crossref | Google Scholar |
Piola RF, Johnston EL (2006) Differential tolerance to metals among populations of the introduced bryozoan Bugula neritina. Marine Biology 148, 997-1010.
| Crossref | Google Scholar |
Pirotta V, Reynolds W, Ross G, Jonsen I, Grech A, Slip D, Harcourt R (2020) A citizen science approach to long-term monitoring of humpback whales (Megaptera novaeangliae) off Sydney, Australia. Marine Mammal Science 36, 472-485.
| Crossref | Google Scholar |
Poore AGB, Watson MJ, de Nys R, Lowry JK, Steinberg PD (2000) Patterns of host use among alga- and sponge-associated amphipods. Marine Ecology Progress Series 208, 183-196.
| Crossref | Google Scholar |
Porter AG, Ferrari RL, Kelaher BP, Smith SDA, Coleman RA, Byrne M, Figueira W (2018) Marine infrastructure supports abundant, diverse fish assemblages at the expense of beta diversity. Marine Biology 165, 112.
| Crossref | Google Scholar |
Pringle C (2003) What is hydrologic connectivity and why is it ecologically important? Hydrological Processes 17, 2685-2689.
| Crossref | Google Scholar |
Qiu Z, Coleman MA, Provost E, Campbell AH, Kelaher BP, Dalton SJ, Thomas T, Steinberg PD, Marzinelli EM (2019) Future climate change is predicted to affect the microbiome and condition of habitat-forming kelp. Proceedings of the Royal Society of London – B. Biological Sciences 286, 20181887.
| Crossref | Google Scholar |
Reid DJ (2020) A review of intensified land use effects on the ecosystems of Botany Bay and its rivers, Georges River and Cooks River, in southern Sydney, Australia. Regional Studies in Marine Science 39, 101396.
| Crossref | Google Scholar |
Reid DJ (2021) A review of the ‘natural’ ecological features of waterways in the Botany Bay catchment, in southern Sydney, Australia. Regional Studies in Marine Science 41, 101545.
| Crossref | Google Scholar |
Reid DJ, Bone EK (2020) The rise and fall of oyster cultivation in the highly urbanized Georges River estuary, Sydney, Australia: a review of lessons learned. Regional Studies in Marine Science 35, 101246.
| Crossref | Google Scholar |
Roach AC (1998) Effects of predation on the size structure of the gastropod Salinator solida (Martens) populations at Towra Point, NSW, Australia. Marine and Freshwater Research 49, 779-784.
| Crossref | Google Scholar |
Ross PM (2001) Larval supply, settlement and survival of barnacles in a temperate mangrove forest. Marine Ecology Progress Series 215, 237-249.
| Crossref | Google Scholar |
Ross PM (2006) Macrofaunal loss and microhabitat destruction: the impact of trampling in a temperate mangrove forest, NSW Australia. Wetlands Ecology and Management 14, 167-184.
| Crossref | Google Scholar |
Ross PM, Adam P (2013) Climate change and intertidal wetlands. Biology 2, 445-480.
| Crossref | Google Scholar |
Ross PM, Underwood AJ (1997) The distribution and abundance of barnacles in a mangrove forest. Australian Journal of Ecology 22, 37-47.
| Crossref | Google Scholar |
Rossi F, Underwood AJ (2002) Small-scale disturbance and increased nutrients as influences on intertidal macrobenthic assemblages: experimental burial of wrack in different intertidal environments. Marine Ecology Progress Series 241, 29-39.
| Crossref | Google Scholar |
Roughan M, Hallam B (2022) The transport and retention of passive particles in Sydney Harbour and Botany Bay, Australia (Version 1). In Zenodo, 15 February 2022. doi:10.5281/zenodo.6096962
Rowland MJ (2004) Return of the ‘noble savage’: misrepresenting the past, present and future. Australian Aboriginal Studies 2, 2-14.
| Google Scholar |
Saintilan N, Mazumder D (2017) Mass spawning of crabs: ecological implications in subtropical Australia. Hydrobiologia 803, 239-250.
| Crossref | Google Scholar |
Saintilan N, Williams RJ (1999) Mangrove transgression into saltmarsh environments in south-east Australia. Global Ecology and Biogeography 8, 117-124.
| Crossref | Google Scholar |
Saintilan N, Williams RJ (2000) Short Note: The decline of saltmarsh in southeast Australia: results of recent surveys. Wetlands Australia 18, 49-54.
| Google Scholar |
Saintilan N, Hossain K, Mazumder D (2007) Linkages between seagrass, mangrove and saltmarsh as fish habitat in the Botany Bay estuary, New South Wales. Wetlands Ecology and Management 15, 277-286.
| Crossref | Google Scholar |
Sanchez-Camara J, Booth DJ, Turon X (2005) Reproductive cycle and growth of Phyllopteryx taeniolatus. Journal of Fish Biology 67, 133-148.
| Crossref | Google Scholar |
Sanchez-Camara J, Booth DJ, Murdoch J, Watts D, Turon X (2006) Density, habitat use and behaviour of the weedy seadragon Phyllopteryx taeniolatus (Teleostei: Syngnathidae) around Sydney, New South Wales, Australia. Marine and Freshwater Research 57, 737-745.
| Crossref | Google Scholar |
Sanchez-Camara J, Martin-Smith K, Booth DJ, Fritschi J, Turon X (2011) Demographics and vulnerability of a unique Australian fish, the weedy seadragon Phyllopteryx taeniolatus. Marine Ecology Progress Series 422, 253-264.
| Crossref | Google Scholar |
Silvertown J (2009) A new dawn for citizen science. Trends in Ecology & Evolution 24, 467-471.
| Crossref | Google Scholar |
Sinclair EA, Sherman CDH, Statton J, Copeland C, Matthews A, Waycott M, van Dijk K-J, Vergés A, Kajlich L, McLeod IM, Kendrick GA (2021) Advances in approaches to seagrass restoration in Australia. Ecological Management & Restoration 22, 10-21.
| Crossref | Google Scholar |
Smith KA, Sinerchia M (2004) Timing of recruitment events, residence periods and post-settlement growth of juvenile fish in a seagrass nursery area, south-eastern Australia. Environmental Biology of Fishes 71, 73-84.
| Crossref | Google Scholar |
Smith KA, Suthers IM (2000) Consistent timing of juvenile fish recruitment to seagrass beds within two Sydney estuaries. Marine and Freshwater Research 51, 765-776.
| Crossref | Google Scholar |
Spooner DR, Maher W, Otway N (2003) Trace metal concentrations in sediments and oysters of Botany Bay, NSW, Australia. Archives of Environmental Contamination and Toxicology 45, 92-101.
| Crossref | Google Scholar |
Stelling-Wood TP, Gribben PE, Adam P, Birch G, Bishop MJ, Blount C, Booth DJ, Brown C, Bruce E, Bugnot AB, Byrne M, Creese RG, Dafforn KA, Dahlenburg J, Doblin MA, Fellowes TE, Fowler AM, Gibbs MC, Glamore W, Glasby T, Hay A, Kelaher B, Knott NA, Larkum AWD, Parker LM, Marzinelli EM, Mayer-Pinto M, Morgan B, Murray SA, Rees M, Ross PM, Roughan M, Saintilan N, Scanes E, Seymour JR, Schaefer N, Suthers IM, Taylor MD, Williamson JE, Villa Concejo A, Whittington R, Figueira WF (2022) Science of Gamay: a systematic review of current knowledge of Botany Bay. Sydney Institute of Marine Science, Sydney, NSW, Australia.
Strain EMA, Olabarria C, Mayer-Pinto M, Cumbo V, Morris RL, Bugnot AB, Dafforn KA, Heery E, Firth LB, Brooks PR, Bishop MJ (2018) Eco-engineering urban infrastructure for marine and coastal biodiversity: which interventions have the greatest ecological benefit? Journal of Applied Ecology 55, 426-441.
| Crossref | Google Scholar |
Sun MY, Dafforn KA, Brown MV, Johnston EL (2012) Bacterial communities are sensitive indicators of contaminant stress. Marine Pollution Bulletin 64, 1029-1038.
| Crossref | Google Scholar |
Sun MY, Dafforn KA, Johnston EL, Brown MV (2013) Core sediment bacteria drive community response to anthropogenic contamination over multiple environmental gradients. Environmental Microbiology 15, 2517-2531.
| Crossref | Google Scholar |
Swadling DS, West GJ, Gibson PT, Laird RJ, Glasby TM (2023) Don’t go breaking apart: Anthropogenic disturbances predict meadow fragmentation of an endangered seagrass. Aquatic Conservation: Marine and Freshwater Ecosystems 33, 56-69.
| Crossref | Google Scholar |
Swanson RL, de Nys R, Huggett MJ, Green JK, Steinberg PD (2006) In situ quantification of a natural settlement cue and recruitment of the Australian sea urchin Holopneustes purpurascens. Marine Ecology Progress Series 314, 1-14.
| Crossref | Google Scholar |
Sweet M, Bulling M, Williamson JE (2016) New disease outbreak affects two dominant sea urchin species associated with Australian temperate reefs. Marine Ecology Progress Series 551, 171-183.
| Crossref | Google Scholar |
Tarquinio F, Hyndes GA, Laverock B, Koenders A, Säwström C (2019) The seagrass holobiont: understanding seagrass-bacteria interactions and their role in seagrass ecosystem functioning. FEMS Microbiology Letters 366, fnz057.
| Crossref | Google Scholar |
Taylor J (2015) New Zealand fur seal sightings in Sydney on the way up, trend to continue: experts. In The Daily Telegraph, 14 August 2015. Available at https://www.dailytelegraph.com.au/newslocal/city-east/experts-run-off-their-feet-with-seal-activity-in-southeast-sydney-more-to-come/news-story/7028e5c10600e38790b6eb5eeb1d1fdf
Taylor JA, Díez-Vives C, Nielsen S, Wemheuer B, Thomas T (2022) Communality in microbial stress response and differential metabolic interactions revealed by time-series analysis of sponge symbionts. Environmental Microbiology 24, 2299-2314.
| Crossref | Google Scholar |
Thompson LR, Sanders JG, McDonald D, Amir A, Ladau J, Locey KJ, Prill RJ, Tripathi A, Gibbons SM, Ackermann G, et al. (2017) A communal catalogue reveals Earth’s multiscale microbial diversity. Nature 551, 457-463.
| Crossref | Google Scholar |
Tian C, Doblin MA, Dafforn KA, Johnston EL, Pei H, Hu W (2018) Dinoflagellate cyst abundance is positively correlated to sediment organic carbon in Sydney Harbour and Botany Bay, NSW, Australia. Environmental Science and Pollution Research 25, 5808-5821.
| Crossref | Google Scholar |
Tippler C, Wright IA, Hanlon A (2012) Is catchment imperviousness a keystone factor degrading urban waterways? A case study from a partly urbanised catchment (Georges River, south-eastern Australia). Water, Air, & Soil Pollution 223, 5331-5344.
| Crossref | Google Scholar |
Truong L, Suthers IM, Cruz DO, Smith JA (2017) Plankton supports the majority of fish biomass on temperate rocky reefs. Marine Biology 164, 73.
| Crossref | Google Scholar |
Underwood AJ, Chapman MG (1996) Scales of spatial patterns of distribution of intertidal invertebrates. Oecologia 107, 212-224.
| Crossref | Google Scholar |
Underwood AJ, Denley EJ, Moran MJ (1983) Experimental analyses of the structure and dynamics of mid-shore rocky intertidal communities in New South Wales. Oecologia 56, 202-219.
| Crossref | Google Scholar |
Underwood AJ, Kingsford MJ, Andrew NL (1991) Patterns in shallow subtidal marine assemblages along the coast of New South Wales. Australian Journal of Ecology 16, 231-249.
| Crossref | Google Scholar |
Van Der Velde JT, King RJ (1984) The subtidal seaweed communities of Bare Island, Botany Bay. Wetlands Australia 4, 7-22.
| Google Scholar |
Warren JH (1990) The use of open burrows to estimate abundances of intertidal estuarine crabs. Australian Journal of Ecology 15, 277-280.
| Crossref | Google Scholar |
Waycott M, Duarte CM, Carruthers TJB, Orth RJ, Dennison WC, Olyarnik S, Calladine A, Fourqurean JW, Heck KL, Jr, Hughes AR, Kendrick GA, Kenworthy WJ, Short FT, Williams SL (2009) Accelerating loss of seagrasses across the globe threatens coastal ecosystems. Proceedings of the National Academy of Sciences 106, 12377-12381.
| Crossref | Google Scholar |
West GJ, Glasby TM (2022) Interpreting long-term patterns of seagrasses abundance: how seagrass variability is dependent on genus and estuary type. Estuaries and Coasts 45, 1393-1408.
| Crossref | Google Scholar |
Wilson JS (1858) Notes on the physical geography of north-west Australia. Journal of the Royal Geographical Society of London 28, 137-153.
| Crossref | Google Scholar |
York PH, Smith TM, Coles RG, McKenna SA, Connolly RM, Irving AD, Jackson EL, McMahon K, Runcie JW, Sherman CDH, Sullivan BK, Trevathan-Tackett SM, Brodersen KE, Carter AB, Ewers CJ, Lavery PS, Roelfsema CM, Sinclair EA, Strydom S, Tanner JE, van Dijk K-J, Warry FY, Waycott M, Whitehead S (2017) Identifying knowledge gaps in seagrass research and management: an Australian perspective. Marine Environmental Research 127, 163-172.
| Crossref | Google Scholar |
Zozaya-Valdes E, Egan S, Thomas T (2015) A comprehensive analysis of the microbial communities of healthy and diseased marine macroalgae and the detection of known and potential bacterial pathogens. Frontiers in Microbiology 6, 146.
| Crossref | Google Scholar |