Impact of inundation on soil microbiology
Timothy J. Ralph

A
B
![]() Tim Ralph is Senior Lecturer at Macquarie University. He has been studying the geomorphology and ecology of rivers and wetlands for >20 years. |
![]() Tsuyoshi (Yoshi) Kobayashi is Senior Research Scientist at the NSW Department of Planning and Environment. He has been studying the ecology of microbial communities (plankton and bacteria) in freshwater systems for >25 years. |
Abstract
Genetic sequencing as well as culture-based studies have revealed diverse aerobic and anaerobic microbes across a range of aquatic environments in floodplain wetlands. Hydrological conditions related to riverine inundation are a predominant factor determining the structure and function of soil bacterial communities in floodplain wetlands. Despite their complex mosaics of topography, landforms and aquatic habitats, some consistent response patterns are observed among soil bacterial communities with changing inundation patterns and history. Considering hydrological events and changes as a form of disturbance, Connell’s ‘intermediate disturbance hypothesis’ has been used to explain the observed bell-shaped response of soil microbial communities with varying hydrological conditions. Further application and testing of general ecological theories and hypotheses may help advance our understanding and predictive modelling capability for the dynamics of floodplain soil bacterial communities with changing hydrological conditions.
Keywords: Actinobacteria, Cyanobacteria, flooding, floodplain microbes, floodplain wetlands, fluvial geomorphology, hydrological regimes, Proteobacteria, relative abundances, taxonomic and functional diversity.
Introduction
Based on 16S rRNA gene sequencing and other molecular methods, as well as culture-based techniques, diverse aerobic and anaerobic microbes are known to inhabit wetland ecosystems.1–5 They contribute to essential metabolic and biogeochemical processes, such as the mineralisation of organic compounds and the cycling of carbon, nitrogen, phosphorus, sulfur, iron and other elements.2,3,5–7 In inland floodplain wetlands that receive water primarily from riverine flows and flooding, including those in Australia, soil microbial communities may be typically dominated by members of the phyla Proteobacteria,2,3,8,9 Acidobacteria,2,3,9,10 Actinobacteria,2,3,8–10 Bacteroidetes,2 Verrucomicrobia2 and Firmicutes.8 Proteobacteria is also known to be the most abundant in natural as well as constructed wetlands.11 Floodplain soil microbes are subject to varying wet and dry conditions.4,9 Observational and experimental studies have been conducted to understand the association of the microbial community structure and function with varying wet and dry conditions in floodplain wetlands.2,6,8,12,13 Hydrological conditions (including a gradient of hydrological connectivity, water depth fluctuations and inundation frequency) are considered critically important in determining the microbial community structure and function in floodplain wetlands.6,8,9,12 In this article, we briefly review the recent studies on the relationships of soil bacteria with hydrological conditions in floodplain wetlands including those in Australia, with a focus on the bacterial relative abundances, taxonomic diversity and functional diversity.
Relative abundances
There are studies that explicitly report the relationships between relative abundances of soil bacteria and hydrological conditions in floodplain wetlands2,8 including those in semi-arid Australia.9 Although these studies were conducted in different geographical regions and have dealt with different aspects of hydrological conditions, the soil bacteria show consistent taxon-specific patterns in terms of how their relative abundances change with increasing wet (or dry) conditions. They can be categorised into positive, negative and neutral (independent) groups in terms of their relative abundances in communities increasing with increasing wet conditions.9 For example, the positive-response group of bacteria includes Bacteroidetes,8 Chloroflexi,8 Euryarchaeota,8 Firmicutes8 and Proteobacteria.8,9 The negative-response group of bacteria includes Acidobacteria,2,8 Actinobacteria2,3,8,9 and Planctomycetes.8 The neutral-response group of bacteria includes Cyanobacteria.9
Increased wet conditions are likely to lead to more anaerobic conditions than aerobic conditions in floodplain soils, favouring anaerobic taxa over aerobic taxa in microbial communities.2,8 In the positive-response group, Bacteroides is obligately anaerobic.14 Many members of the Proteobacteria and Chloroflexi are obligately or facultatively anaerobic,15,16 although the members of Euryarchaeota and Firmicutes may be more heterogeneous in terms of oxygen preference.17,18 In the negative-response group, many members of Actinobacteria, Acidobacteria and Planctomycetes are aerobic.19–21 In the neutral-response group, Cyanobacteria are capable of anoxic, anoxygenic photosynthesis22,23 and are likely to grow even in increased wet conditions. In addition, Cyanobacteria can exist in the form of dormant cells, such as akinetes, during dry times.24,25 Such physiological adaptations by Cyanobacteria may render the presence of Cyanobacteria independent of hydrological conditions (e.g. inundation frequency) in floodplain soils, relative to other members of the microbes (Fig. 1).9
Examples of dry aquatic environments in floodplain wetlands of the Macquarie Marshes, Australia (a), with associated elevation (b), inundation frequency (c) and logit-transformed relative abundances of the floodplain Proteobacteria (d), Actinobacteria (e) and Cyanobacteria (f) against inundation frequency from the period 1979–2006.37 Fitted regression lines for Proteobacteria and Actinobacteria (d, e) are significant at P = 0.05.9
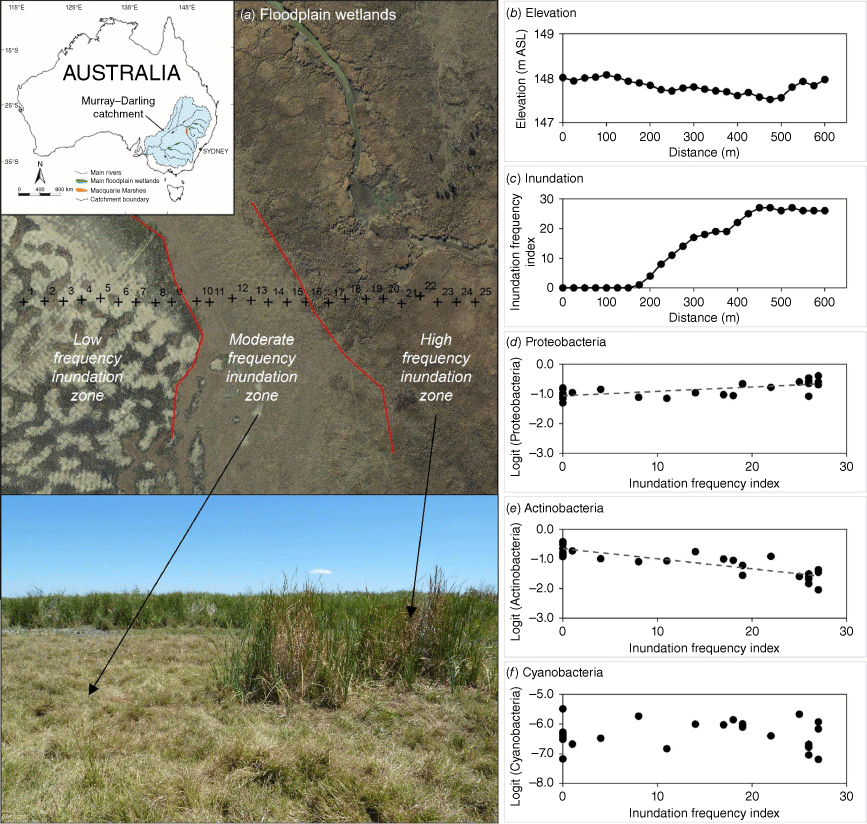
Because floodplain wetlands are comprised of a suite of landforms that are geomorphologically dynamic and underpin a mosaic of aquatic habitats,26,27 small, spatial heterogeneity in hydrological conditions (e.g. due to elevational differences within <1-km distance) can produce taxon-specific effects on their relative abundances of soil bacteria in floodplain wetlands.4,9
Taxonomic diversity
The fluctuations in hydrological conditions are known to affect the diversity of floodplain soil bacteria. For example, Peralta et al.28 have shown that bacterial communities originating from fluctuating hydrological conditions were more diverse and capable of greater changes in community composition in response to changes in soil moisture than bacterial communities shaped by stable, less dynamic, hydrological conditions (i.e. more permanently wet or dry conditions). Shen et al.4 also reported that the diversity of soil bacteria was high at middle elevation sites (corresponding to sites of intermediate flooding duration), relative to high and low elevation sites. They stated that the observed curvilinear relationship between the soil bacterial diversity and flooding disturbance can be explained by Connell’s ‘intermediate disturbance hypothesis’.29 Flooding or inundation is thought to be a reasonably common and important disturbance type in floodplain wetlands including those in semi-arid Australia.11,30,31 Interestingly, Rayburg et al.31 have shown that semi-arid floodplain sites subject to an intermediate level of flood disturbance also have a greater level of diversity in soil properties than those sites subject to frequent flood disturbances.
Functional diversity
Changes in hydrological conditions also influence the functional diversity of floodplain soil bacteria, by affecting soil redox, nutrient and carbon environments as well as grain size distributions in topsoil.32,33 For example, they may allow the coexistence of nitrifying and denitrifying bacteria in floodplains following inundation, with additional functional pathways of nitrogen cycling.32,34,35 In terms of carbon cycling, Limpert et al.3 reported the changes in the rates of carbon dioxide (CO2) and methane (CH4) emissions in a semi-arid Australian floodplain wetland before, during and after inundation. Overall, the total carbon emissions in the form of CO2 and CH4 are reduced during and after wetting due to increasing vegetative growth and subsequent CO2 sequestration.
By using Biolog EcoPlates (Biolog, Inc., Hayward, CA, USA), Wilson et al.12 measured the diversity of carbon substrate use by Australian floodplain soil bacteria. They reported that initially following flooding, a diverse range of carbon substrates was used by floodplain soil bacteria. However, after 24 days there was no significant difference between the carbon substrates used in flooded and non-flooded samples. They suggest that the initial rapid increase in substrate utilisation is due to opportunistic growth of r-selected species responding to a change in their immediate environmental condition, which was followed by growth of K-selected species. Similarly, using Ecoplates, Rana et al.13 investigated the intensity and diversity of carbon substrate use by soil bacterial communities in areas exposed to different historical inundation frequencies (moderate, low and very low areas) in semi-arid Australia. They detected the highest microbial activity from the soil bacteria community in a very low inundation frequency area. The diversity of carbon substrates used also differed among communities initially following inundation but became similar diversity levels after ~1 week of inundation.
Conclusion
The structure and function of soil bacteria are intrinsically related to hydrological conditions in floodplain wetlands. There are consistent response patterns among soil bacterial communities with changing hydrological conditions in floodplain wetlands, despite their complex topography, landscape and aquatic habitats. Considering hydrological changes as a form of disturbance, Connell’s ‘intermediate disturbance hypothesis’ has been used to explain the observed bell-shaped response of soil microbial communities with varying hydrological conditions.29 There is inherent difficulty in clearly defining what is meant by an ‘intermediate’ disturbance due to ecosystem-specific reference conditions such as average, minimum and maximum values.36 Nevertheless, further application and testing of general ecological theories and hypotheses may help advance our understanding and predictive modelling capability for the dynamics of floodplain soil bacterial communities with changing hydrological conditions.
References
1 Kim SY et al. (2008) Comparative analysis of soil microbial communities and their responses to the short-term drought in bog, fen, and riparian wetlands. Soil Biol Biochem 40, 2874-2880.
| Crossref | Google Scholar |
2 Ligi T et al. (2014) Characterization of bacterial communities in soil and sediment of a created riverine wetland complex using high-throughput 16S rRNA amplicon sequencing. Ecol Eng 72, 56-66.
| Crossref | Google Scholar |
3 Limpert KE et al. (2020) Reducing emissions from degraded floodplain wetlands. Front Environ Sci 8, 8.
| Crossref | Google Scholar |
4 Shen R et al. (2021) Flooding variations affect soil bacterial communities at the spatial and inter-annual scales. Sci Total Environ 759, 143471.
| Crossref | Google Scholar | PubMed |
5 Pazinato JM et al. (2010) Molecular characterization of the archaeal community in an Amazonian wetland soil and culture-dependent isolation of methanogenic archaea. Diversity 2, 1026-1047.
| Crossref | Google Scholar |
6 Baldwin DS, Mitchell AM (2000) The effects of drying and re‐flooding on the sediment and soil nutrient dynamics of lowland river–floodplain systems: a synthesis. Regul Rivers: Res Mgmt 16, 457-467.
| Crossref | Google Scholar |
7 Boon PI et al. (1997) Effects of wetting and drying on methane emissions from ephemeral floodplain wetlands in south-eastern Australia. Hydrobiologia 357, 73-87.
| Crossref | Google Scholar |
8 Argiroff WA et al. (2017) Microbial community functional potential and composition are shaped by hydrologic connectivity in riverine floodplain soils. Microb Ecol 73, 630-644.
| Crossref | Google Scholar | PubMed |
9 Kobayashi T et al. (2020) Influence of historical inundation frequency on soil microbes (Cyanobacteria, Proteobacteria, Actinobacteria) in semi-arid floodplain wetlands. Mar Freshwater Res 71, 617-625.
| Crossref | Google Scholar |
10 Hartman WH et al. (2008) Environmental and anthropogenic controls over bacterial communities in wetland soils. Proc Natl Acad Sci USA 105, 17842-17847.
| Crossref | Google Scholar | PubMed |
11 Mellado M, Vera J (2021) Microorganisms that participate in biochemical cycles in wetlands. Can J Microb 67, 771-788.
| Crossref | Google Scholar | PubMed |
12 Wilson JS et al. (2011) The effects of short-term inundation on carbon dynamics, microbial community structure and microbial activity in floodplain soil. River Res Appl 27, 213-225.
| Crossref | Google Scholar |
13 Rana A et al. (2021) Planktonic metabolism and microbial carbon substrate utilization in response to inundation in semiarid floodplain wetlands. J Geophys Res Biogeosci 126, e2019JG005571.
| Crossref | Google Scholar |
14 Garrett WS, Onderdonk AB (2015) Bacteroides, Prevotella, Porphyromonas, and Fusobacterium species (and other medically important anaerobic Gram-negative bacilli). In Mandell, Douglas, and Bennett’s Principles and Practice of Infectious Diseases (Bennett JE, et al., eds). pp. 2773–2780. Saunders, an imprint of Elsevier Inc., Philadelphia, PA, USA.
17 Oren A (2023) Euryarchaeota. In Encyclopedia of Life Sciences (eLS). pp. 1–17. John Wiley & Sons, Ltd. 10.1002/9780470015902.a0004243.pub3
18 Gavande PV et al. (2021) Functional characterization of thermotolerant microbial consortium for lignocellulolytic enzymes with central role of Firmicutes in rice straw depolymerization. Sci Rep 11, 3032.
| Crossref | Google Scholar | PubMed |
19 Fuerst JA, Sagulenko E (2011) Beyond the bacterium: planctomycetes challenge our concepts of microbial structure and function. Nat Rev Microbiol 9, 403-413.
| Crossref | Google Scholar | PubMed |
20 Barka EA et al. (2016) Taxonomy, physiology, and natural products of Actinobacteria. Microbiol Mol Biol Rev 80, 1-43.
| Crossref | Google Scholar | PubMed |
21 Eichorst SA et al. (2018) Genomic insights into the Acidobacteria reveal strategies for their success in terrestrial environment. Environ Microbiol 20, 1041-1063.
| Crossref | Google Scholar | PubMed |
22 Shi L et al. (2010) Diversity and abundance of aerobic anoxygenic phototrophic bacteria in two cyanobacterial bloom-forming lakes in China. Int J Limnol 46, 233-239.
| Crossref | Google Scholar |
23 Fujita Y et al. (2015) Evolutionary aspects and regulation of tetrapyrrole biosynthesis in Cyanobacteria under aerobic and anaerobic environments. Life 5, 1172-1203.
| Crossref | Google Scholar | PubMed |
24 Agrawal SC, Singh V (2002) Viability of dried filaments, survivability and reproduction under water stress, and survivability following heat and UV exposure in Lyngbya martensiana, Oscillatoria agardhii, Nostoc calcicola, Hormidium fluitans, Spirogyra sp. and Vaucheria geminata. Folia Microbiol 47, 61-67.
| Crossref | Google Scholar | PubMed |
26 Ralph TJ, Hesse PP (2010) Downstream hydrogeomorphic changes along the Macquarie River, southeastern Australia, leading to channel breakdown and floodplain wetlands. Geomorphology 118, 48-64.
| Crossref | Google Scholar |
27 Ralph TJ et al. (2016) Wandering wetlands: spatial patterns of historical channel and floodplain change in the Ramsar-listed Macquarie Marshes, Australia. Mar Freshwater Res 67, 782-802.
| Crossref | Google Scholar |
28 Peralta AL et al. (2014) Bacterial community response to changes in soil redox potential along a moisture gradient in restored wetlands. Ecol Eng 73, 246-253.
| Crossref | Google Scholar |
29 Connell JH (1978) Diversity in tropical rain forests and coral reefs: high diversity of trees and corals is maintained only in a nonequilibrium state. Science 199(4335), 1302-1310.
| Crossref | Google Scholar |
30 Colloff MJ, Baldwin DS (2010) Resilience of floodplain ecosystems in a semi-arid environment. Rangel J 32, 305-314.
| Crossref | Google Scholar |
31 Rayburg S et al. (2023) The impact of flood frequency on the heterogeneity of floodplain surface soil properties. Soil Syst 7, 63.
| Crossref | Google Scholar |
32 Hoagland B et al. (2019) Controls on nitrogen transformation rates on restored floodplains along the Cosumnes River, California. Sci Total Environ 649, 979-994.
| Crossref | Google Scholar | PubMed |
33 Zhang Z, Furman A (2021) Soil redox dynamics under dynamic hydrologic regimes – a review. Sci Total Environ 763, 143026.
| Crossref | Google Scholar | PubMed |
34 Boon PI, Mitchell A (1995) Methanogenesis in the sediments of an Australian freshwater wetland: comparison with aerobic decay, and factors controlling methanogenesis. FEMS Microbiol Ecol 18, 175-190.
| Crossref | Google Scholar |
35 Burgin AJ, Hamilton SK (2007) Have we overemphasized the role of denitrification in aquatic ecosystems? A review of nitrate removal pathways. Front Ecol Environ 5, 89-96.
| Crossref | Google Scholar |
36 Shea K et al. (2004) Moving from pattern to process: coexistence mechanisms under intermediate disturbance regimes. Ecol Lett 7, 491-508.
| Crossref | Google Scholar |
37 Thomas RF et al. (2011) Landsat mapping of annual inundation (1979–2006) of the Macquarie Marshes in semi-arid Australia. Int J Remote Sens 32(16), 4545-4569.
| Crossref | Google Scholar |
![]() Tim Ralph is Senior Lecturer at Macquarie University. He has been studying the geomorphology and ecology of rivers and wetlands for >20 years. |
![]() Tsuyoshi (Yoshi) Kobayashi is Senior Research Scientist at the NSW Department of Planning and Environment. He has been studying the ecology of microbial communities (plankton and bacteria) in freshwater systems for >25 years. |