Antibacterial hydrogel therapy for eradication of wound associated polymicrobial biofilms
Hanif Haidari A and Zlatko Kopecki A *A Future Industries Institute, University of South Australia, Mawson Lakes, SA 5095, Australia.
![]() Dr Hanif Haidari is a Research Associate at University of South Australia. His research interests include inorganic nanoparticles synthesis and applications against antibacterial resistance using latest in vitro and in vivo models. He also has strong interest in the development of smart drug-delivery systems for prevention of antimicrobial resistance and wound infection development. |
![]() Dr Zlatko Kopecki is a Senior Research Fellow at the University of South Australia and a Channel 7 Children’s Research Foundation Mid‐Career Fellow for Childhood Wound Infections. Dr Kopecki’s research is focussed on developing novel therapeutics for wound repair and working on understanding the mechanisms involved in wound healing, scar formation and fragile skin syndromes. He is also interested in integration of different approaches and biomaterials for the development of novel wound dressings and therapeutic approaches to improve healing and combat wound infection. |
Microbiology Australia 44(2) 104-108 https://doi.org/10.1071/MA23029
Submitted: 25 March 2023 Accepted: 20 April 2023 Published: 19 May 2023
© 2023 The Author(s) (or their employer(s)). Published by CSIRO Publishing on behalf of the ASM. This is an open access article distributed under the Creative Commons Attribution-NonCommercial-NoDerivatives 4.0 International License (CC BY-NC-ND)
Abstract
The recalcitrance of bacterial biofilms to current antimicrobials has presented a major cause of clinical recurrence of wound infections. These biofilm-associated infections are often present in polymicrobial nature associated with the presence of Pseudomonas aeruginosa and Staphylococcus aureus creating a large heterogeneity that shares a common resistance to current antimicrobials making pathogen eradication extremely challenging. In this study, we overcome the intrinsic biofilm barriers by delivering ultrasmall-sized silver nanoparticles (AgNP) using a smart hydrogel system that allows slow and sustained release of silver ions mediating successful accumulation and penetration of bacterial biofilms. The antibiofilm efficacy of the AgNP hydrogel was assessed using ex vivo porcine wound polymicrobial biofilms. Treatment with AgNP hydrogel resulted in significant dispersion of early to mature biofilms, 2–5-log reduction of bacteria compared to untreated controls. This approach overcomes the enhanced tolerance and resistance of polymicrobial biofilms by using the combined benefits of smart delivery system and the antibiofilm properties of ultrasmall AgNPs to ensure biofilm complete destruction and elimination.
Keywords: antimicrobial resistance, biofilm, hydrogel drug delivery, polymicrobial infection, silver nanoparticles.
Introduction
Infected chronic wounds remain a major global health threat with up to 80% of wound infections being associated with biofilm development. Biofilms are a community of pathogenic bacteria attached to a surface surrounded by a self-produced extracellular polymeric substance (EPS).1 The complex three-dimensional structure of biofilms act as a mechanical and physical shelter to protect bacterial cells against antimicrobials and host immune responses making elimination extremely difficult.2 This inevitable emergence of resistance to conventional antibiotics contributes to widespread treatment failure.3 Further, most wound infections are polymicrobial in nature, adding further challenge to our ability to manage wound infections due to the recalcitrance of biofilms to the current antimicrobials.4 Polymicrobial pathogen interactions lead to much harder biofilms with increased pathogenicity compared to singles species. Current strategies are limited by the poor penetration of antimicrobial agents through physical biofilm barriers reducing their efficacy. Interspecies interaction of Pseudomonas aeruginosa and Staphylococcus aureus are commonly associated with nosocomial infection bacteria and most common pathogens in chronic wounds, playing a major role in disease progression, virulence factors and biofilm recalcitrance, all contributing to impaired wound healing.5
Polymicrobial wound biofilms are highly resistant to antimicrobials which necessitates an improved understanding to regulate the damaging impact on healthcare-associated infections. As an alternative, nanomaterial based products have been investigated against bacteria including two-dimensional black phosphorus,6 gold nanoparticles,7 zinc oxide nanoparticles8 and silver nanoparticles (AgNPs).9 Among all, AgNPs antibacterial formulations have been most studied in wound dressings, coatings, and hydrogels. AgNPs are best known for the multifaceted mechanism of action showing activity against a broad spectrum of pathogens with the potential to overcome drug-resistant infections and with clear applications in clinical wound management. However the major challenge with the use of current AgNPs include toxicity to surrounding cells, uncontrolled release, lack of activity against mature biofilms especially biofilms associated with multiple species, and the possibility of developing resistance over time.10 Previously we have shown that AgNPs can be developed to overcome some of the current limitations by structural adjustment,11 optimisation of mode of delivery,12 and exposure to the target site presenting an ideal platform for antibacterial therapy without bacterial resistance.13 We have also shown that biofilm integrity can be dispersed using structurally modified AgNPs targeting biofilm development process.14 Here we speculate that ultrasmall-sized AgNPs with biofilm-modified structural properties may facilitate enhanced permeation through the cell membrane to induce overall biofilm dispersion. The slow and sustained release of silver ions will present high localised concentrations at the biofilm interface providing durable and effective activity against mature polymicrobial biofilms.
In this study, we tested the activity of advanced antibacterial AgNP hydrogel using ex vivo porcine skin polymicrobial wound biofilm model. The use of ex vivo porcine culture of mature biofilms provides more strictly controlled experimental conditions than those of in vivo models. Additionally, using porcine skin as both the substrate for bacterial attachment and the source of nutrition would produce a microbial biofilm that more closely resembles the characteristics of clinical wound biofilms.15 The porcine skin was cleaned and sterilised prior to creating a 10-mm2 excisional wound using a punch biopsy.16 The skin was partially submerged in DMEM supplemented with FCS (10%) at the air–liquid interface (Fig. 1a). The created wound bed was inoculated with a combination of P. aeruginosa (Xen 41) and S. aureus (Xen 29) to establish an early (24 h) and mature (48 h) polymicrobial biofilm in a 37°C incubator (Fig. 1b). After each time point, the planktonic bacteria were gently washed, and the wounds were treated with AgNP hydrogel (100 mg of AgNP hydrogel per wound sample equating to 20 µg of silver per wound), blank hydrogel, silver sulfadiazine (Ag SD), and PBS as described previously.17 The biofilm eradication capacity was assessed using selective bacterial counts supported by bacterial staining to analyse 3-dimensional (3-D) structure of biofilm integrity after the treatments.
(a) Schematic illustration of air–liquid interface biofilm set up using porcine skin. (b) Digital photographs of biofilm growth within the wound region after 24 h.
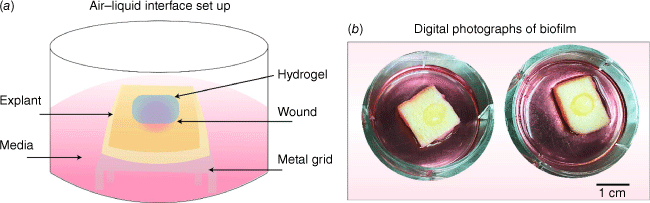
The efficiency of the AgNP hydrogel was first assessed using selective counts for P. aeruginosa and S. aureus. The initial observation indicated that the bacterial species detected within biofilms are predominantly P. aeruginosa. This is consistent with previous studies showing that the majority of the interaction of P. aeruginosa in a mixed species is competitive in nature with only a few interactions of a cooperative nature.18 In general, P. aeruginosa is a strong biofilm former and has been associated with the inhibition of other pathogenic microbes including Staphylococcus and Streptococcus species. P. aeruginosa has numerous counter attacking mechanisms mediated by its motile properties and ability to produce toxins including pyocyanin, and hydrogen cyanide affecting the electron transport pathway and thereby slowing the growth of S. aureus.18 These two wound pathogens are commonly found together in a clinical setting, however when co-cultured P. aeruginosa thrives far better. This was also observed in our study where we saw higher colony forming unit (CFU) counts for untreated P. aeruginosa (1 × 1012 and 2 × 1013 CFUs per tissue) within 24 and 48 h respectively compared to 3 × 106 and 9 × 105 CFUs per tissue) for S. aureus (Fig. 2a–d).
Colony counts of polymicrobial biofilm bacteria selective for Pseudomonas aeruginosa and Staphylococcus aureus. (a) Representative P. aeruginosa colony forming unit (CFU) plates of 24 h after treatment, (b) the corresponding quantitative analysis of P. aeruginosa bacteria. (c) Representative S. aureus CFU plates of 24 h after treatment, (d) the corresponding quantitative analysis of S. aureus bacteria. Data presented as mean ± s.d.
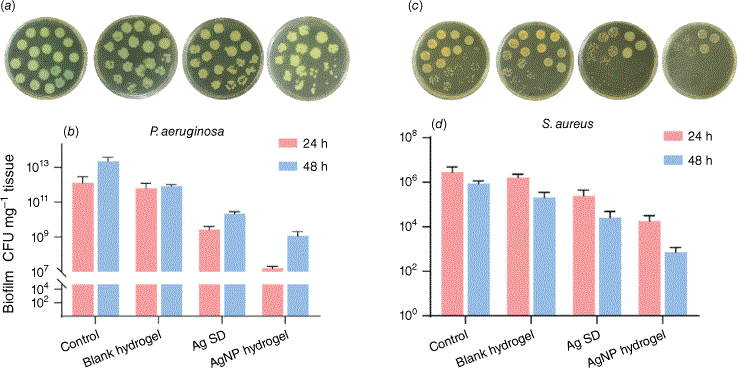
However, bacterial biofilms were highly sensitive to the treatments where Ag SD and AgNP hydrogel was able to induce a 2.8- and 4.9-log reduction of early biofilms of P. aeruginosa respectively compared to untreated control (Fig. 2a, b). The log reduction for the mature biofilms was 2 and 3.28. The reduced susceptibility of mature biofilm is expected due to the increased biofilm biomass supported with tougher structural integrity including overexpression of EPS production.19 Providing an optimal environment allows biofilms to persist with enhanced resistance mechanism to external agents including low penetration of antimicrobials.20 A similar trend of bacterial elimination was also observed against S. aureus where Ag SD and AgNP hydrogel was able to induce a 1.1- and 2.1-log reduction and 1.4- and 3.1-log reduction after 48 h (Fig. 2c, d). Blank hydrogel with no AgNP showed negligible activity against these polymicrobial biofilms (Fig. 2a–d). The increased susceptibility of P. aeruginosa and S. aureus biofilms accounts for potent antibiofilm capacity of ultrasmall AgNPs that can accumulate within biofilm structure facilitating structural damage and enhanced bacterial penetration.14,21 We have previously shown that the small sized AgNPs can overcome single species biofilm structural barrier with favourable uptake resulting in biofilm disintegration, against both Gram-negative and -positive bacteria using clinically relevant in vitro and preclinical animal infection wound models where their application was safe and no adverse effects were observed.14,21 Nanoparticle’s size dependent antibacterial effect of silver has also been studied using mesoporous silica nanoparticles,22 biofilm responsive nanoantibiotic containing small sized AgNPs23 and surface coated ultrasmall AgNPs to boost antibacterial efficacy.24 It is noteworthy that ultrasmall size AgNPs are highly susceptible to fast oxidation and dissolution, facilitating fast leaching of silver ions and short antibacterial duration with associated potential toxicity.25 The use of biocompatible hydrogel delivery system is therefore a promising approach to overcome these deficiencies providing a localised and targeted delivery of AgNPs which contributes to efficient dispersion and elimination of wound infection.
The biofilm structural integrity was also analysed using fluorescence staining through confocal microscopy. The biofilms were stained with live–dead viability kit containing SYTO9 that stains the nucleic acids of all bacteria fluorescent green, whereas propidium iodide rapidly penetrates bacteria with damaged membranes fluorescing red.26 The 3-D biofilm was reconstructed through Imaris Software (ver. 9.3.0, Bitplane AG) to visualise total cell viability as well as biofilm biovolume (Fig. 3a, b). We saw a reduced total bacterial cell viability in response to Ag SD 55% and AgNPs 40% within 24 h followed by further reduction after 48 h resulting in 42 and 26% bacterial cell viability respectively (Fig. 3c). Consistent with the CFU, AgNP treatment resulted in dramatic reduction in cell viability. We also observed progressively reduced biovolume over the 2 days of AgNP hydrogel while the untreated control progressively matured into thicker biofilm (90 µm) (Fig. 3b, d). This is because P. aeruginosa and S. aureus readily form mature biofilms and are notoriously resistant to current antimicrobials if left untreated.
Three-dimensional (3-D) fluorescence reconstruction of polymicrobial biofilm in response to treatment groups after 24 and 48 h. (a, b) representation of 3-D biofilm biovolume after 24 and 48 h of treatment compared to control. (c) Quantitative analysis of total cell viability after each time points. (d) Quantitative analysis of total biovolume of each treatment group after 24 and 48 h of treatment.
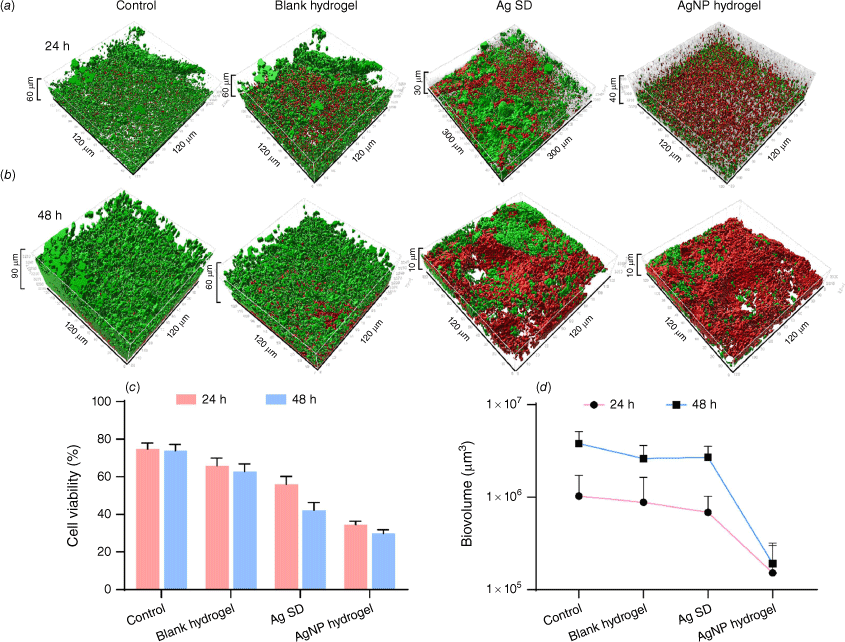
Despite the great potential of AgNPs to kill bacterial pathogens, the complex defence mechanism of biofilms has challenged most of the current AgNP composites, failing to overcome the barriers resulting in reduced response against mature biofilms. In this study we have shown that AgNP hydrogel presents effective strategy to serve as AgNP reservoir by providing controlled, sustained release for biofilm treatment. We have shown that ultrasmall nature of AgNPs could reach the biofilm interface resulting in enhanced penetration, and bacterial death. The significantly dispersed biofilm biomass as well as reduced cell viability indicates a promising antibiofilm capacity of AgNP hydrogel to eliminate a clinical chronic wound biofilm. Interestingly, it performed significantly better than the Ag SD which remains the gold standard antibacterial topical treatment for infection wound management in a clinical setting. The difference is attributed to the carrier, which can safely reach the bacterial site of infection, can penetrate, accumulate in high concentration, and display antimicrobial activity deep inside an infectious biofilm triggered by the antibiofilm properties of delivered silver ions.
In summary, biologically adhered mature biofilms are generally very difficult to eradicate because of acquired resistance and tolerance to current antimicrobials, and their persistence and continuous pathogenicity has challenged the healthcare system. The ever-growing nanoparticle therapy has been re-purposed taking advantage of the multifunctional roles against bacteria to fight against biofilm associated maturation and resistance where the antibiotics have failed greatly. Using ultrasmall silver nanoparticles delivery mechanism, we have exploited the combination of ultrasmall size and controlled release mechanism to overcome biofilm barriers hence resulting in a significant capacity to eradicate both early and mature polymicrobial biofilms similar to those encountered in chronic wounds in a clinical setting. Daily application of treatment results in progressive loss of biofilm integrity and biovolume. Therefore, this study presents a promising translational clinical pathway to fight against drug resistant chronic wound associated biofilm infections.
Data availability
The data that support this study will be shared upon reasonable request to the corresponding author.
Declaration of funding
Z. Kopecki is supported by the Channel 7 Children’s Research Foundation Fellowship. The authors also acknowledge Microscopy Australia at the University of South Australia, Mawson Lakes Campus, for scientific and technical assistance and the use of instruments. Microscopy Australia is funded by the University, and State and Federal Governments. The project is funded by Channel 7 Children’s Research Foundation Research Grant and the EB Research Network Grant (#Kopecki).
References
[1] Donlan, RM and Costerton, JW (2002) Biofilms: survival mechanisms of clinically relevant microorganisms. Clin Microbiol Rev 15, 167–193.| Biofilms: survival mechanisms of clinically relevant microorganisms.Crossref | GoogleScholarGoogle Scholar |
[2] Haidari, H et al. (2021) Eradication of mature bacterial biofilms with concurrent improvement in chronic wound healing using silver nanoparticle hydrogel treatment. Biomedicines 9, 1182.
| Eradication of mature bacterial biofilms with concurrent improvement in chronic wound healing using silver nanoparticle hydrogel treatment.Crossref | GoogleScholarGoogle Scholar |
[3] Frieri, M et al. (2017) Antibiotic resistance. J Infect Public Health 10, 369–378.
| Antibiotic resistance.Crossref | GoogleScholarGoogle Scholar |
[4] Stacy, A et al. (2016) The biogeography of polymicrobial infection. Nat Rev Microbiol 14, 93–105.
| The biogeography of polymicrobial infection.Crossref | GoogleScholarGoogle Scholar |
[5] DeLeon, S et al. (2014) Synergistic interactions of Pseudomonas aeruginosa and Staphylococcus aureus in an in vitro wound model. Infect Immun 82, 4718–4728.
| Synergistic interactions of Pseudomonas aeruginosa and Staphylococcus aureus in an in vitro wound model.Crossref | GoogleScholarGoogle Scholar |
[6] Shaw, ZL et al. (2021) Broad-spectrum solvent-free layered black phosphorus as a rapid action antimicrobial. ACS Appl Mater Interfaces 13, 17340–17352.
| Broad-spectrum solvent-free layered black phosphorus as a rapid action antimicrobial.Crossref | GoogleScholarGoogle Scholar |
[7] Arafa, MG et al. (2018) Thermoresponsive gels containing gold nanoparticles as smart antibacterial and wound healing agents. Sci Rep 8, 13674.
| Thermoresponsive gels containing gold nanoparticles as smart antibacterial and wound healing agents.Crossref | GoogleScholarGoogle Scholar |
[8] Sirelkhatim, A et al. (2015) Review on zinc oxide nanoparticles: antibacterial activity and toxicity mechanism. Nanomicro Lett 7, 219–242.
| Review on zinc oxide nanoparticles: antibacterial activity and toxicity mechanism.Crossref | GoogleScholarGoogle Scholar |
[9] Ravindran Girija, A et al. (2019) Ultrasmall gold nanocluster based antibacterial nanoaggregates for infectious wound healing. ChemNanoMat 5, 1176–1181.
| Ultrasmall gold nanocluster based antibacterial nanoaggregates for infectious wound healing.Crossref | GoogleScholarGoogle Scholar |
[10] Haidari, H et al. (2020) Silver-based wound dressings: current issues and future developments for treating bacterial infections. Wound Pract Res 28, 176–183.
| Silver-based wound dressings: current issues and future developments for treating bacterial infections.Crossref | GoogleScholarGoogle Scholar |
[11] Haidari, H et al. (2019) The interplay between size and valence state on the antibacterial activity of sub-10 nm silver nanoparticles. Nanoscale Adv 1, 2365–2371.
| The interplay between size and valence state on the antibacterial activity of sub-10 nm silver nanoparticles.Crossref | GoogleScholarGoogle Scholar |
[12] Haidari, H et al. (2020) Ultrasmall AgNP-impregnated biocompatible hydrogel with highly effective biofilm elimination properties. ACS Appl Mater Interfaces 12, 41011–41025.
| Ultrasmall AgNP-impregnated biocompatible hydrogel with highly effective biofilm elimination properties.Crossref | GoogleScholarGoogle Scholar |
[13] Haidari, H et al. (2021) Multifunctional ultrasmall AgNP hydrogel accelerates healing of S. aureus infected wounds. Acta Biomater 128, 420–434.
| Multifunctional ultrasmall AgNP hydrogel accelerates healing of S. aureus infected wounds.Crossref | GoogleScholarGoogle Scholar |
[14] Haidari, H et al. (2022) Polycationic silver nanoclusters comprising nanoreservoirs of Ag+ ions with high antimicrobial and antibiofilm activity. ACS Appl Mater Interfaces 14, 390–403.
| Polycationic silver nanoclusters comprising nanoreservoirs of Ag+ ions with high antimicrobial and antibiofilm activity.Crossref | GoogleScholarGoogle Scholar |
[15] Yang, Q et al. (2013) Development of a novel ex vivo porcine skin explant model for the assessment of mature bacterial biofilms. Wound Repair Regen 21, 704–714.
| Development of a novel ex vivo porcine skin explant model for the assessment of mature bacterial biofilms.Crossref | GoogleScholarGoogle Scholar |
[16] Haidari, H et al. (2017) Development of topical delivery systems for flightless neutralizing antibody. J Pharm Sci 106, 1795–1804.
| Development of topical delivery systems for flightless neutralizing antibody.Crossref | GoogleScholarGoogle Scholar |
[17] Haidari, H et al. (2022) Bacteria-activated dual pH- and temperature-responsive hydrogel for targeted elimination of infection and improved wound healing. ACS Appl Mater Interfaces 14, 51744–51762.
| Bacteria-activated dual pH- and temperature-responsive hydrogel for targeted elimination of infection and improved wound healing.Crossref | GoogleScholarGoogle Scholar |
[18] Nair, N et al. (2014) Impact of Staphylococcus aureus on pathogenesis in polymicrobial infections. Infect Immun 82, 2162–2169.
| Impact of Staphylococcus aureus on pathogenesis in polymicrobial infections.Crossref | GoogleScholarGoogle Scholar |
[19] Sanyasi, S et al. (2016) Polysaccharide-capped silver nanoparticles inhibit biofilm formation and eliminate multi-drug-resistant bacteria by disrupting bacterial cytoskeleton with reduced cytotoxicity towards mammalian cells. Sci Rep 6, 24929.
| Polysaccharide-capped silver nanoparticles inhibit biofilm formation and eliminate multi-drug-resistant bacteria by disrupting bacterial cytoskeleton with reduced cytotoxicity towards mammalian cells.Crossref | GoogleScholarGoogle Scholar |
[20] Wu, S et al. (2021) Biofilm-sensitive photodynamic nanoparticles for enhanced penetration and antibacterial efficiency. Adv Funct Mater 31, 2103591.
| Biofilm-sensitive photodynamic nanoparticles for enhanced penetration and antibacterial efficiency.Crossref | GoogleScholarGoogle Scholar |
[21] Uroro, EO et al. (2022) Biocompatible polycationic silver nanocluster-impregnated PLGA nanocomposites with potent antimicrobial activity. ChemNanoMat 8, e202200349.
| Biocompatible polycationic silver nanocluster-impregnated PLGA nanocomposites with potent antimicrobial activity.Crossref | GoogleScholarGoogle Scholar |
[22] Liu, J et al. (2019) Boosting antibacterial activity with mesoporous silica nanoparticles supported silver nanoclusters. J Colloid Interface Sci 555, 470–479.
| Boosting antibacterial activity with mesoporous silica nanoparticles supported silver nanoclusters.Crossref | GoogleScholarGoogle Scholar |
[23] Wu, J et al. (2019) Responsive assembly of silver nanoclusters with a biofilm locally amplified bactericidal effect to enhance treatments against multi-drug-resistant bacterial infections. ACS Cent Sci 5, 1366–1376.
| Responsive assembly of silver nanoclusters with a biofilm locally amplified bactericidal effect to enhance treatments against multi-drug-resistant bacterial infections.Crossref | GoogleScholarGoogle Scholar |
[24] Yuan, X et al. (2014) Ultrasmall Ag+-rich nanoclusters as highly efficient nanoreservoirs for bacterial killing. Nano Res 7, 301–307.
| Ultrasmall Ag+-rich nanoclusters as highly efficient nanoreservoirs for bacterial killing.Crossref | GoogleScholarGoogle Scholar |
[25] Uroro, EO et al. (2023) Enzyme-responsive polycationic silver nanocluster-loaded PCL nanocomposites for antibacterial applications. Mater Today Chem 28, 101376.
| Enzyme-responsive polycationic silver nanocluster-loaded PCL nanocomposites for antibacterial applications.Crossref | GoogleScholarGoogle Scholar |
[26] Bright, R et al. (2022) Surfaces containing sharp nanostructures enhance antibiotic efficacy. Nano Lett 22, 6724–6731.
| Surfaces containing sharp nanostructures enhance antibiotic efficacy.Crossref | GoogleScholarGoogle Scholar |