Molecular phylogenetic position and description of a new genus and species of freshwater Chaetonotidae (Gastrotricha: Chaetonotida: Paucitubulatina), and the annotation of its mitochondrial genome
Leandro Gammuto A # , Valentina Serra A # , Giulio Petroni A B C and M. Antonio Todaro
A
B
C
D
E
Handling Editor: Katrine Worsaae
Abstract
Chaetonotidae is the most diversified family of the entire phylum Gastrotricha; it comprises ~430 species distributed across 16 genera. The current classification, established mainly on morphological traits, has been challenged in recent years by phylogenetic studies, indicating that the cuticular ornamentations used to discriminate among species may be misleading when used to identify groupings, which has been the practice until now. Therefore, a consensus is developing toward implementing novel approaches to better define species identity and affiliation at a higher taxonomic ranking. Using an integrative morphological and molecular approach, including annotation of the mitogenome, we report on some freshwater gastrotrichs characterised by a mixture of two types of cuticular scales diagnostic of the genera Aspidiophorus and Heterolepidoderma. Our specimens’ overall anatomical characteristics find no correspondence in the taxa of these two genera, calling for their affiliation to a new species. Phylogenetic analyses based on the sequence of the ribosomal RNA genes of 96 taxa consistently found the new species unrelated to Aspidiophorus or Heterolepidoderma but allied with Chaetonotus aff. subtilis, as a subset of a larger clade, including mostly planktonic species. Morphological uniqueness and position along the non-monophyletic Chaetonotidae branch advocate erecting a new genus to accommodate the current specimens; consequently, the name Litigonotus ghinii gen. nov., sp. nov. is proposed. The complete mitochondrial genome of the new taxon resulted in a single circular molecule 14,384 bp long, including 13 protein-coding genes, 17 tRNA genes and 2 rRNAs genes, showing a perfect synteny and collinearity with the only other gastrotrich mitogenome available, a possible hint of a high level of conservation in the mitochondria of Chaetonotidae.
ZooBank: urn:lsid:zoobank.org:pub:9803F659-306F-4EC3-A73B-8C704069F24A
Keywords: biodiversity, fresh water, gastrotrichs, hairy-bellied worms, meiofauna, mitogenome, phylogeny, systematics, taxonomy.
Introduction
Gastrotricha Metschnikoff, 1865 is a phylum of microscopic invertebrates, ubiquitous and often abundant in various aquatic ecosystems of the world (Todaro et al. 2019). The phylum comprises ~895 species, in 2 orders: Macrodasyida Remane, 1925 (~380 species) and Chaetonotida Remane, 1925 (~515 species). Chaetonotidan gastrotrichs are unevenly split into 2 suborders: Multitubulatina d’Hondt, 1971 with only 3 species and Paucitubulatina d’Hondt, 1971 comprising ~517 species (Saponi and Todaro 2024). The latter taxon includes 2 families entirely marine or brackish (Muselliferidae Leasi & Todaro, 2008 and Xenotrichulidae Remane, 1927), 4 families entirely freshwater (Dasydytidae Daday, 1905, Dichaeturidae Remane, 1927, Neogosseidae Remane, 1927, and Proichthydidae Remane, 1927) and 1 family (Chaetonotidae Gosse, 1864) encompassing freshwater as well as marine forms (Balsamo et al. 2014; Kieneke and Schmidt-Rhaesa 2015). Chaetonotidae is also the most diversified family of the entire phylum; it comprises ~430 species distributed across 16 genera; some genera exclusively inhabit either of freshwater or marine environments, whereas others may be found in both (Todaro et al. 2019). More specifically, 9 chaetonotid genera include exclusively freshwater representatives: Arenotus Kisielewski, 1987 (1 species), Bifidochaetus Kolicka & Kisielewski, 2016 (in Kolicka et al. 2016, 2 species), Cephalionotus Garraffoni, Araujo, Lourenço, Guidi & Balsamo, 2017 (1 species), Fluxiderma d’Hondt, 1974 (3 species), Halichaetoderma Rataj Križanová & Vd’ačný, 2023 (4 species), Lepidochaetus Kisielewski, 1991 (6 species), Polymerurus Remane, 1927 (16 species), Rhomballichthys Schwank, 1990 (1 species) and Undula Kisielewski, 1991 (1 species). Two chaetonotid genera are entirely marine: Caudichthydium Schwank, 1990 (3 species) and Halichaetonotus Remane, 1936 (31 species). The remaining 5 chaetonotid genera include both freshwater and marine species, with even some taxa recorded from both environments: Aspidiophorus Voigt, 1903 (24 freshwater species and 11 marine species), Chaetonotus Ehrenberg, 1830 (191 freshwater species and 47 marines, including 6 species reported mainly from freshwater habitats), Ichthydium Ehrenberg, 1830 (29 freshwater species and 3 marines), Heterolepidoderma Remane, 1927 (25 freshwater species and 15 marines) and Lepidodermella Blake, 1933 (15 freshwater species and 2 marines, including 1 species recorded mainly from freshwater habitat) (Todaro and d’Hondt 2023; Saponi and Todaro 2024).
The above-depicted classificatory scenario, established mainly on morphological traits, has recently been challenged by phylogenetic studies especially by those based on molecular sequence data (i.e. the 18S rDNA alone or combined with the 28S rDNA and the mitochondrial COI gene). Among the controversial aspects that bear relevance for the present work, it should be noted that molecular analyses have invariably indicated the family Chaetonotidae as paraphyletic since the members of the monophyletic freshwater families Neogosseidae and Dasydytidae have always been found nested within it (e.g. Kånneby et al. 2013; Kånneby and Todaro 2015; Garraffoni et al. 2017; Kolicka et al. 2020). This scenario is not much different from the one inferred by the morphological cladistics study of Hochberg and Litvaitis (2000), where Dasydytidae form a monophyletic group within a non-monophyletic Chaetonotidae, or from the results of Kieneke et al. (2008), in which Dasydytidae and Neogosseidae outline a clade within a non-monophyletic Chaetonotidae.
Moreover, the current classification of several genera requires further confirmation through molecular-based phylogenetic analyses. Interestingly, even if they share the same habitat, representatives of the same genus have been found not to cluster together, as seen in the case of Aspidiophorus and Heterolepidoderma species (e.g. Kånneby et al. 2013; Kånneby and Todaro 2015; Garraffoni et al. 2017). Even if the habitat is the same, representatives of the same genus have been found not phylogenetically allied, as in the case of Ichthydium skandicum Kånneby, Todaro & Jondelius, 2009 and I. squamigerum Balsamo & Fregni, 1995 plus Ichthydium sp. (Kolicka et al. 2020). Additionally, the genus Chaetonotus, which is the most speciose of the entire phylum, presents a taxonomically disruptive situation as its species are scattered along the evolutionary branch of Chaetonotidae and variously associated with representatives of other genera (e.g. Bekkouche and Worsaae 2016; Kolicka et al. 2020; Rataj Križanová and Vďačný 2024).
All in all, molecular phylogenetic studies suggest that the common understanding of cuticular ornamentations, such as scales and spines, may be misleading when used to identify groupings, which has been the practice until now. Therefore, a novel approach is needed to better define species identity, especially their affiliation to higher taxonomic ranking (e.g. at genus or family levels).
Recently, four new freshwater species similar to species of the genus Heterolepidoderma were discovered by Rataj Križanová and Vďačný (2024), who established the genus Halichaetoderma to allocate them. Although closely resembling Heterolepidoderma, molecular phylogenetic analyses revealed that Halichaetoderma is closely related to the marine Halichaetonotus. Stochastic mapping indicated that the characteristic oblong, keeled scales covering the bodies of the members of the three genera evolved through convergence at least four times (Rataj Križanová and Vďačný 2024), highlighting once again the inadequacy of cuticular characteristics in diagnosing groupings until their evolutionary origins are fully understood.
In this framework, we report on some gastrotrich specimens from an undescribed species found in summer 2021 in Tuscany (Italy). The phylogenetic analysis of the nucleotide sequence of the 18S and 28S ribosomal genes involving over 96 Paucitubulatina taxa supports the uniqueness of these gastrotrichs. Consequently, as repeatedly advocated, we employ a cutting-edge approach that integrates morphological and molecular data, along with mitochondrial genome information, to ensure the accurate taxonomic classification of these gastrotrichs.
Material and methods
Sampling site and sampling
Samples containing the gastrotrichs described herein were collected on 8 July 2021 from the larger freshwater pond of the botanical garden of the University of Pisa (43°43′16.60″N, 10°23′45.75″E). It is an artificial setting hosting many small and large invertebrates, vertebrates such as frogs (Pelophylax sp.), and protists. The pond is made up of two parts connected to each other. A circular shaped part is ~12 m in diameter. The narrowest part, digitiform, is ~13 m long and 3.10 m wide (4.10 m at the point where there is a small widening). The water level is uniform and is ~60–70 cm deep. The pond, of artificial origin, was probably built at the beginning of the 20th century, in the part of the botanical garden called ‘Orto del Gratta’, located in the northernmost part of the complex. The pond hosts some aquatic plant species, such as the lotus, Nelumbo nucifera (Gaertn., 1788), Nymphaea sp., Lemna minuta Kunth, 1815 (which often forms a floating carpet above the water level), and Ceratophyllum demersum Linnaeus, 1753. The high metazoan biodiversity in the pond is likely due to repeated intentional introductions, possibly by local teachers who used the variety of fauna in zoology courses. Sampling was carried out by scooping up, with plastic jars and Falcon tubes, the thin layer of detritus covering the rocky and cemented pond edges down to a depth of ~50 cm. Two 0.5-L plastic jars and four 50-mL centrifuge tubes filled with water, some debris, and a little vegetation from the two long banks of the pond were collected. Samples were brought to the laboratory in Modena and analysed over 1 week. No special permission or permits were needed to collect the animals under study.
Sample processing and morphological analysis
Fauna was extracted by stirring the samples with a plastic pipette and aliquots of the sediment-water mixture were decanted into 5-cm diameter plastic Petri dishes and analysed under a Wild M8 stereo-microscope. Individual gastrotrichs were picked out with a hand-held micro-pipette and whole mounted on a slide in a drop of drop of 1% MgCl2 solution. The morphological survey was carried out on living, relaxed specimens under a Nikon Eclipse 90i microscope provided with Nomarski optics (DIC) and fitted with a Nikon DSFi1 digital camera driven by the Nikon NIS-Elements D software (ver. 4.20, see https://www.microscope.healthcare.nikon.com/it_EU/products/software/nis-elements). Two identified specimens were retrieved from the slides, transferred to 0.5-mL centrifuge tubes filled with 96% ethanol, and used later in the laboratory at Pisa for molecular genetic analyses (see below). The description of the new species follows the convention of Hummon et al. (1992); the position of key morphological characters is given in percentage units (U) of total body length, measured from anterior to posterior end.
DNA amplification and sequencing
Starting from a single specimen, the total DNA material was amplified via the whole-genome amplification (WGA) method, using REPLI-g Single Cell Kit (QIAGEN, Hilden, Germany). In detail, the organism was washed in distilled water three times and the last time in Phosphate-Buffered Saline (PBS) solution (reagent provided in the kit). Then, it was transferred to a 0.2-mL Eppendorf tube together with 4 µL of PBS. The WGA protocol was completed following the manufacturer’s instructions. The DNA material was processed with a Nextera XT library and sequenced at GENEWIZ Germany GmbH (Leipzig, Germany), using Illumina HiSeq X technology to generate 42,729,292 reads (paired-ends 2 × 150 bp).
Ribosomal operon and mitochondrial assembly and annotation
Preliminary assembly of resulting reads was performed using SPAdes software (ver. 3.6.0, see https://github.com/ablab/spades; Bankevich et al. 2012). Starting from this assembly, contigs (i.e. assembled sequences) belonging to either the rDNA operon and the mitochondrion were identified by blastn and tblastn analyses respectively. For the ribosomal operon, several 18S and 28S rDNA sequences were downloaded from the US National Center for Biotechnology Information (NCBI) to serve as queries for the blastn analysis, whereas the protein coding genes from the mitochondrion of Lepidodermella squamata (Dujardin, 1841) (ACC KP965862) were used as queries for the tblastn analyses. Reads mapped on those selected contigs were extracted from the original set and separately assembled using SPAdes in order to obtain the whole ribosomal operon and the whole mitochondrial genome in a single contig each. Prediction and annotation of Open Reading Frames (ORFs) was performed on the MITOS web server (see http://mitos.bioinf.uni-leipzig.de/; Bernt et al. 2013) setting the genetic code to 5, whereas prediction of rDNA genes on both the ribosomal operon and mitochondrial genome was performed with the StructRNAfinder web tool (see http://structrnafinder.integrativebioinformatics.me; Arias-Carrasco et al. 2018). Mitochondrial protein codon usage was calculated using a lab made script. The obtained 18S rDNA and 28S rDNA sequences were used for the phylogenetic analysis.
Phylogenetic analysis
The acquired 18S rDNA sequence was aligned with the automatic aligner of the ARB software package (ver. 5.5, see http://www.arb-home.de; Westram et al. 2011) on the SSU ref NR99 SILVA database. To implement the 28S rDNA sequence in our analyses, starting from the work of Kolicka et al. (2020), we selected a suitable set of Paucitubulatina representatives of the phylum Gastrotricha for which both 18S and 28S rDNA sequences were available (Supplementary Table S1). We decided to not include the COI genes in our phylogeny because for several organisms the gene is missing: using it only for some of them might have created artifacts in the branch lengths and stability of the nodes. After downloading the selected sequences, they were aligned using the MAFFT online tool (see https://mafft.cbrc.jp/alignment/server/; Katoh et al. 2019) together with the 28S rDNA sequences of the new species. For the phylogenetic analysis, 92 concatenated 18S and 28S rDNA sequences belonging to representatives of the families Chaetonotidae, Dasydytidae, and Neogosseidae were selected, plus four sequences belonging to the family Xenotrichulidae as the outgroup, for a total of 96 sequences. Both alignments were manually edited to optimise base pairing, and the 18S nucleotide matrix was also trimmed at the shortest sequence length. The 18S rDNA matrix and the 28S rDNA matrix contained respectively 1697 and 3632 nucleotide columns and were concatenated to obtain a final matrix of 5329 columns (see Supplementary File S1). We also performed an additional analysis excluding outgroup sequences and rooting the resulting tree at midpoint, to assess the influence of the outgroup as a possible cause of the Long Branch Attraction phenomenum (see Rataj Križanová and Vďačný 2024).
For our dataset, the optimal substitution model was selected with jModelTest (ver. 2.1, see https://github.com/ddarriba/jmodeltest2; Darriba et al. 2012) according to the Akaike Information Criterion (AIC). The maximum likelihood (ML) tree was calculated with PHYML (ver. 5.3.2, see http://www.atgc-montpellier.fr/phyml/; Guindon and Gascuel 2003) software using the GTR+G+I substitution model, performing 1000 pseudoreplicates. Bayesian inference (BI) tree was inferred with MrBayes (ver. 3.2, see https://github.com/NBISweden/MrBayes/; Ronquist et al. 2012), using the GTR+G+I substitution model, three runs each with one cold and three heated Monte Carlo Markov chains, with a burn-in of 25%, iterating for 3,000,000 generations.
Results
Molecular phylogeny
The ribosomal operon of the new species resulted of 5682 nucleotides and was deposited in the NCBI GenBank database under Accession number OR915722.
The best BLAST hit identity on NCBI was with Chaetonotus aff. subtilis MN496228, (99.03%; 2 gaps, 17 mismatches). The topologies identified by the ML-based tree and the BI-based tree generally aligned with each other. However, it is important to note that they differed at some points, such as the placement of the marine Aspidiophorus clade (refer to Fig. 1 v. Supplementary Fig. S1). Despite these differences, both phylogenetic analyses placed our concatenated 18S–28S rDNA sequence as sister of sequences belonging to Chaetonotus aff. subtilis (see Kolicka et al. 2020) with high statistical support and altogether inside a supported clade composed by Dasydytes spp., Stylochaeta spp., Haltidytes squamosus Kisielewski, 1991, Neogossea antennigera (Gosse, 1851), Kijanebalola devestiva Todaro, Perissinotto & Bownes, 2013, and Chaetonotus heterocanthus Remane, 1927 (Fig. 1). However, most internal nodes had weak support, casting doubt on the relationships within the supported clade.
Phylogenetic tree of suborder Paucitubulina. Phylogenetic tree based on the concatenation of 18S and 28S rDNA genes. The topology is based on the maximum-likelihood analysis. The two Accession numbers shown next to the names of the organisms are 18S rDNA and 28rDNA respectively. Number pairs associated with nodes represent bootstrap values and posterior probabilities respectively (only values above 70 and 0.80 are shown). Values referring to posterior probability are reported only if the node is present in both the maximum-likelihood and the Bayesian inference based trees (see Supplementary Fig. S1). Sequence obtained in the present work is highlighted in red.
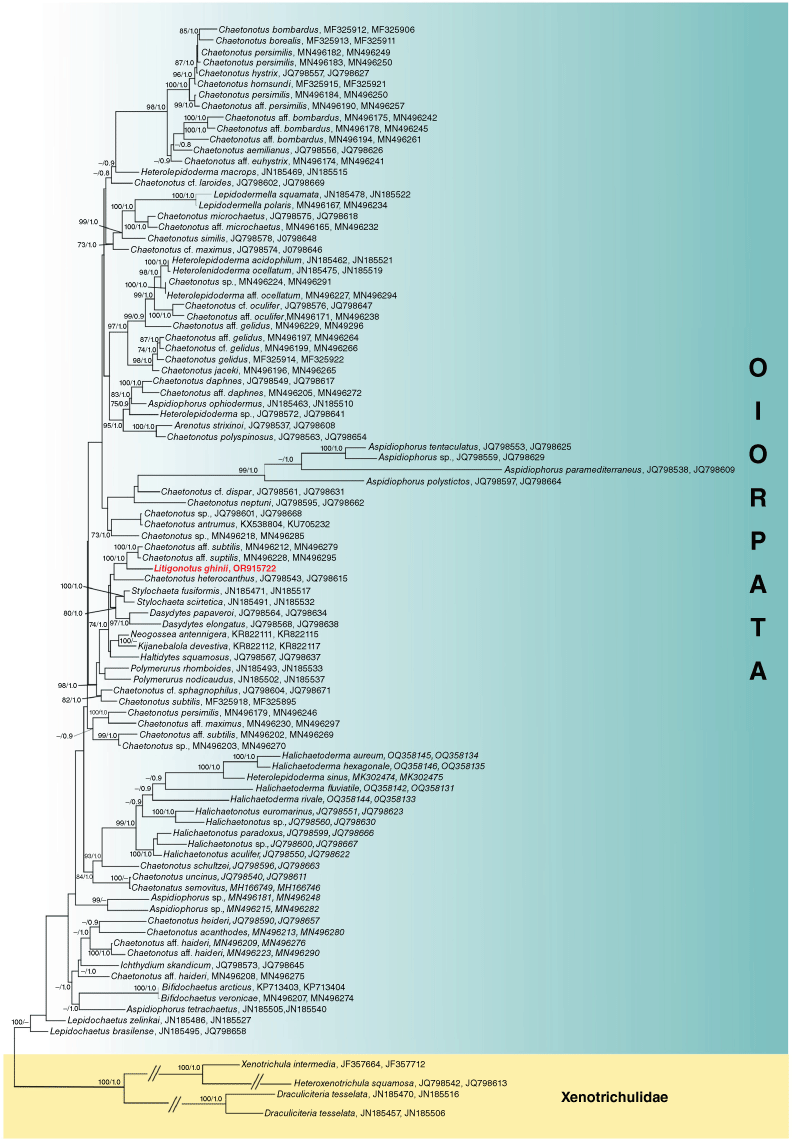
A close phylogenetic relationship between Litigonotus ghinii gen. nov., sp. nov. and members of Aspidiophorus or Heterolepidoderma genera is not supported by our analysis. In the broader framework, both the latter two genera are shown to be polyphyletic. At the same time, the family Chaetonotidae appears paraphyletic due to the nested position of members of Dasydytidae and Neogosseidae (Fig. 1). These results are consistent with the outcome of the phylogenetic analyses that excluded Xenotrichulidae as an outgroup (Supplementary Fig. S2), providing robustness to the information obtained on these specific points.
Mitochondrial genome analysis
The complete mitochondrial genome of Litigonotus ghinii gen. nov., sp. nov. resulted in a single circular molecule 14,384 bp long, and has been deposited in NCBI under the Accession number PP105008. Its genome content includes 13 protein coding genes (mainly related to energy production), 17 tRNA genes and 2 rRNAs genes (Fig. 2a). Overall, its structure does not possess any striking features or unusual genes when compared to other metazoan mitochondrial genomes. The only other available mitochondrial genome of a gastrotrich belongs to Lepidodermella squamata (Golombek et al. 2015), and comparison of their structures showed a perfect synteny and collinearity between them (Fig. 2b). Also the analysis of codon usage in both of the mitochondria showed no outstanding variation in the two organisms (Supplementary Fig. S3).
Mitochondrial gene map of Litigonotus ghinii sp. nov. The gene map of the mitochondrion of L. ghinii (a) and a schematic representation of both Litigonotus ghinii and Lepidodermella squamata mitochondria, to show the complete synteny between them (b). Arrows indicate the direction of transcription, whereas the grey inner circle indicates the GC content of the corresponding region.
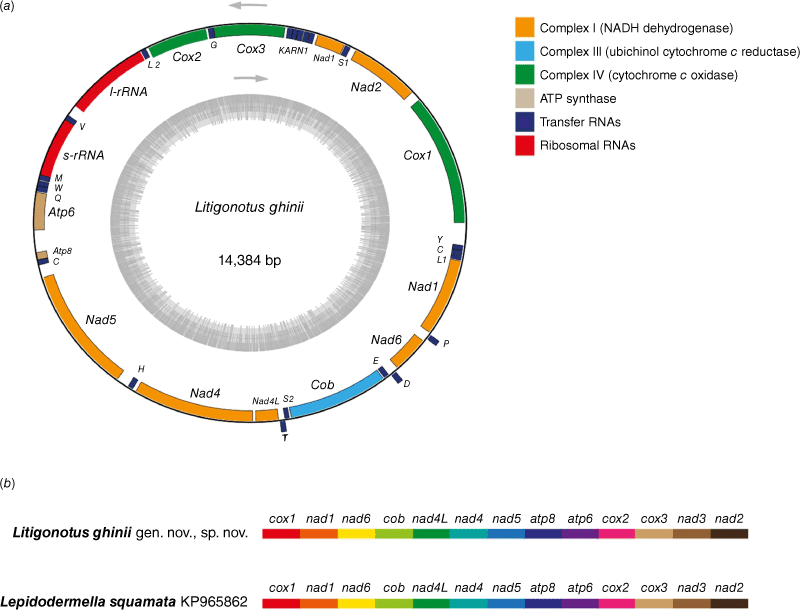
The strict similarity in terms of both codon usage and gene order between the mitochondrial genome of the new species and L. squamata is indeed remarkable (Fig. 2, Supplementary Fig. S3), especially considering the phylogenetic distance between the two taxa (Fig. 1). Even if we understand that with just two mitochondrial sequences available it is premature to draw any conclusions, this could be a hint of a high level of conservation in the mitochondria of Chaetonotidae. The high conservation could probably be linked to the habitat and the reproductive biology of these animals, which includes ubiquitous parthenogenesis and clonal lineages.
Taxonomy
Litigonotus Gammuto, Serra, Petroni & Todaro, gen. nov.
ZooBank: urn:lsid:zoobank.org:act:ABE647F5-F58F-4CFC-B0D8-39B79F0FF7A
From the Latin litigo (dispute) and notus (known), alluding to the pedunculated scales shared by several gastrotrich lineages.
Body stout, up to 148 µm long, tenpin-shaped, terminating in a furcate caudum; head slightly pentalobed, bearing cephalion, epi- and hypopleurae, and hypostomion; neck ill demarked; furca up to 18 μm long. Neck and trunk regions covered dorsally and lateroventrally by 34–45 alternating 34–36 pedunculated scales, up to 5 µm long and 3 µm wide; scales widen towards their rear end, just overlapping, and are strengthened by a weak keel extending for half their length; on dorsal side of the furcal base there are 8–10 pairs of non-pedunculated, keeled, elliptical scales; elliptic to oval keeled scales cover also the ventral proximal portion of the furcal branches; interciliary area fully covered by 9–10 alternating columns of pedunculated, keeled scales. One pair of round perianal keeled scales ~4–5 μm in size present. Sensorial ciliary elements distributed in two paired tufts of 4–8 cilia on the head and paired bristles on the neck, and on the posterior trunk region. Bristles of the neck region generally shorter, originating directly from the body; posterior bristles originating from round to triangular scales, each provided by two anteriorly converging keels. Ventral locomotor cilia distributed in two separate longitudinal bands extending from under the head to the furcal base; each band is approximately club-shaped anteriorly, but narrowing considerably from the posterior pharyngeal region. Mouth ~5 μm in diameter, projecting slightly ventrally and leading into a 31 μm long pharynx; pharynx very muscular, showing a robust bulb at each end; two cuticular teeth are visible within the anterior bulb; pharyngeo-intestinal junction (PhIJ) at U22; intestine straight, wider anteriorly, anus ventral at U88. A pair of conspicuous, tubular protonephridia are present in trunk region, extending from past the PhIJ to approximately mid-body; parthenogenetic. Laid egg, 60 × 38 μm in size with egg shell smooth, without ornamentation.
18S rRNA gene: 246 T, 287 T, 521 T, 1402 A, 1411 C, 1421 A, 1438 C.
28S rRNA gene: 123 A, 460 A, 474 G, 525 T, 610 G, 639 T, 645 C, 647 A, 664 T, 667 A, 670 C, 736 G, 764 T, 772 A, 858 C, 859 A, 887 C, 946 G, 948 A, 963 T, 1803 A.
Analysis of molecular autapomorphies was carried out using the closest sequences in the phylogeny shown in Fig. 1, namely Chaetonotus aff. subtilis MN496212, MN496279; Chaetonotus aff. subtilis MN496228, MN496295; Chaetonotus heterocanthus JQ798543, JQ798615; Dasydytes papaveroi JQ798564, JQ798634; Dasydytes elongates JQ798568, JQ798638; Stylochaeta fusiformis JN185471, JN185517; Stylochaeta scirtetica JN185491, JN185532. Nucleotide positions correspond to the reference alignments files, provided in Supplementary Files S2 and S3.
Litigonotus ghinii Gammuto, Serra, Petroni & Todaro, sp. nov.
ZooBank: urn:lsid:zoobank.org:act:EA943DD6-B6CC-43E8-AF69-7AF3ACDB73AE
The new species is named after Prof. Luca Ghini (1490–1556) founder of the Botanical Garden where the species was first found.
Italy, Pisa: the pond of the Botanical Garden (43°43′16.60″N; 10°23′45.75″E) epibenthic on organogenic detritus.
Holotype: the 146 μm long adult specimen shown in Fig. 4 and 5, no longer extant, collected on 8 July 2021 (International Code of Zoological Nomenclature, Articles 73.1.1 and 73.1.4; see also recommendation 73G–J of Declaration 45 – Addition of Recommendations to Article 73), (International Commission on Zoological Nomenclature 1999, 2017). Additional studied specimens: four adults (showing a ripe egg inside) and five subadults, collected from the type locality; all were examined in vivo and weredestroyed during the observation, except the holotype and another adult that were recovered from the slide, preserved in a 95% ethanol solution and subsequently used for molecular genetic analysis (see below). Another adult specimen was found in a sample collected from the same locality or pond on 1 December 2022.
The description is mainly based on the holotypic specimen, 146 μm in total body length. Body medium sized, tenpin-shaped; head slightly pentalobed, neck faintly narrower than head, trunk sac-like, terminating in a furcate caudum. Body widths at the head/neck/trunk/caudum and their locations along the body are 26/25/48/18 μm, at U04/22.5/56/86.5 respectively. Caudum rather short (16.7 μm long), paired laterally divergent adhesive tubes (10.5 μm long) with a slightly enlarged base (6.2 μm), covered by scales (Fig. 3a, d, 4a–c).
Head bearing frontally and dorsally an obvious, approximately pentagonal, cephalion (12 × 9 μm), laterally small (7 × 4 μm) epi- and hypo-pleurae and ventrally a trapezoidal hypostomion (7 × 3 μm) (Fig. 3b, c, 4a–c); neck and trunk covered dorsally and lateroventrally by 35 alternating columns (19 dorsal and 8 + 8 lateroventral) of 34–36 pedunculated scales (Fig. 3a, e, 4b, c, 5a, c). The scales widen towards their rear end, just overlapping, and are strengthened by a weak keel extending for half their length (Fig. 3e, 5a, c, 6e, f). The median column of scales is straight, whereas the columns on either side slowly follow the lateral body outline (Fig. 4b). Scales increase slightly in size from the head to past mid-trunk, reducing again toward the rear end. Scales on the dorsal trunk measure and 5–4 µm long and 2–3 µm wide, the peduncle is ~0.5–1.0 µm high (Fig. 3e, 6c, f). The scales of the lateral and especially ventrolateral columns are smaller, down to half the size of the dorsal ones. On posterior trunk region, at U85, are two round to subtriangular, double keeled scales (5 × 4 μm) from each of which emerge a sensorial bristle (Fig. 3a, 6a, d). On the dorsal side of the furcal base 8–10 pairs of non-pedunculated, keeled, elliptical scales are present (Fig. 3a, f, 5b); elliptic to oval keeled scales cover also the proximal portion of the ventral side of the furcal branches (Fig. 3d). The interciliary area appears fully covered from under the head to the anal region (U09–U87) by 9–10 alternating columns of pedunculated, keeled scales (Fig. 3c, d, 4c, 5c, d). The interciliary field scales appear similar to the dorsal and ventrolateral ones but smaller (~2 μm) and with the keel that spans the entire length of the scale. At the furcal indentation, two oval to round keeled scales (~4–5 μm) are present (Fig. 3d, 5d).
Head sensorial cilia are distributed into two paired tufts of 4–8 elements, 5–16 μm long (Fig. 1a–c). Two pairs of sensory bristles (13–32 μm long) are present on the dorsal side on the neck and posterior trunk region, at U26 and U87 respectively (Fig. 3a, 6a, c). The bristles of the anterior pair generally are shorter and apparently originate directly from the cuticle, whereas posterior bristles originate from scales each provided by two anteriorly converging keels. Presence of additional sensory bristles hidden among the cephalic ciliary tufts cannot be excluded. Ventral locomotor cilia are distributed in two separate longitudinal bands extending from U09 to approximately U88 (Fig. 3c, d, 6a, 7b); each band is approximately club-shaped anteriorly, but narrowing considerably from the posterior pharyngeal region; bands approach each other immediately behind the hypostomion, but remain separate throughout their entire length; individual cilia are ~8–10 μm long; two denser tufts of longer cilia (10–14 μm) are present in the posterior region (U88) (Fig. 3d, 5d).
Mouth of small size (~4–5 μm in diameter), projecting slightly ventrally (Fig. 3a, c, 7b) and leading into a 31 μm long pharynx (Fig. 3a, 4b, 7b); pharynx very muscular, showing a solid bulb anteriorly (11 μm in diameter) and posteriorly (14.5 μm); two cuticular teeth are visible within the anterior bulb (Fig. 3a); pharynx connected to a sack-like intestine; pharyngeo-intestinal junction at U22; intestine straight, wider anteriorly, narrowing posteriorly, anus ventral at U88.
A pair of conspicuous, tubular protonephridia are present in trunk region, extending from past the pharyngo-intestinal junction (U38) to approximately mid-body (U49); each protonephridium includes a proximal, straight portion containing (1–2) vibrating flagella, and somewhat convoluted posterior region that apparently empties outwards (Fig. 3a, 8a).
Adult specimens were in the parthenogenetic phase (i.e. no developing sperm were seen) showing a large egg filling much of the trunk region (Fig. 3a, 4a, 6a). During the observation, one of the specimens released its egg (60 × 38 μm). The shell of the laid egg appears smooth, without ornamentation (Fig. 6e).
Line art illustrations of Litigonotus ghinii gen. nov., sp. nov. (a) Habitus, ventral view, showing the internal organisation. (b) Anterior region, dorsal view. (c) Anterior region, ventral view. (d) Posterior region, ventral view. (e) Pedunculed scale. (f) Keeled scale. Drawings are made mostly from the holotypic specimen. At, adhesive tube; Cct, cephalic cilia tufts; Ce, cephalion; Eg, egg; Ep, epipleura; Fu, furca; I, intestine; IF, interciliary field; Hp, hypopleura; Hs, hypostomion; KS, keed scale; Lc, locomotor cilia; Mo, mouth; NB, neck bristle; PB, posterior bristle; Ph, pharynx; PhT, pharyngeal teeth; PhIJ, pharyngo–intestinal junction; Pn, protonephridium; PS, peduncled scales; PVS, posterior ventral scales.
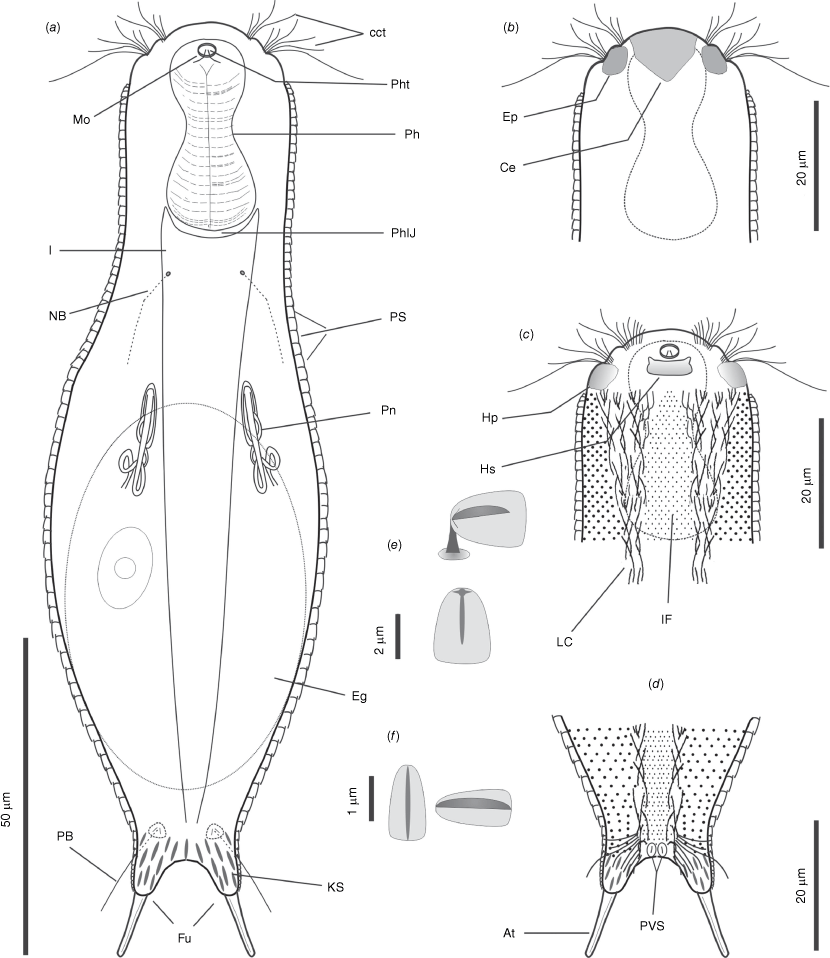
Differential interference contrast photomicrographs showing the morphology of the holotypic specimen of Litigonotus ghinii gen. nov., sp. nov. (a) Habitus outlines. (b) Habitus, dorsal view showing the cuticular covering. (c) Habitus, ventral view showing the cuticular covering including the scales of the interciliary field.
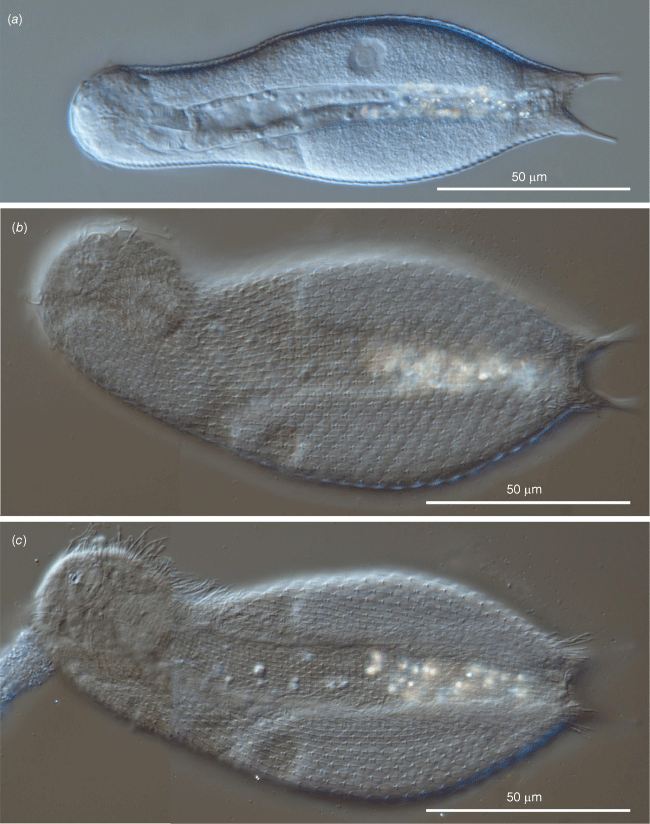
Differential interference contrast photomicrographs showing morphological details of of the holotypic specimen of Litigonotus ghinii gen. nov., sp. nov. (a) Close up of the mid-trunk, dorsal view, showing the cuticular covering made of pedunculated scales. (b) Close up of the posterior trunk region, dorsal view, showing the cuticular covering made of keeled scales; arrows indicate the double keeled scales carrying the posterior bristles. (c) Close up of the mid-trunk region, ventral view, showing the pedunculated scales covering also the interciliary field. (d) Close up of the posterior trunk region, ventral view, showing the two perianal keeled scales (arrows).
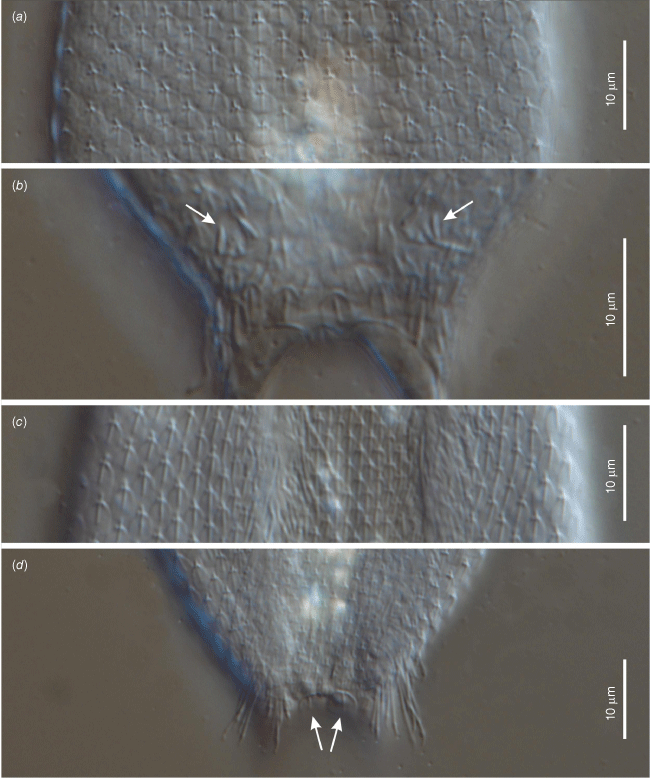
Differential interference contrast photomicrographs showing the morphology of Litigonotus ghinii gen. nov., sp. nov. (a) An adult specimen with a large egg inside, lateral view, arrows indicate neck and posterior bristles. (b) Detail of the posterior region, lateral view, showing the furca and the posterior bristle (BP). (c) Close up of the mid-trunk region, lateral view, showing the cuticular covering made of pedunculated scales. (d) Close up of a scale. (e) The egg immediately after being laid. (f) Detail of the dorsal trunk region, lateral view, showing the scales originating from a peduncle.
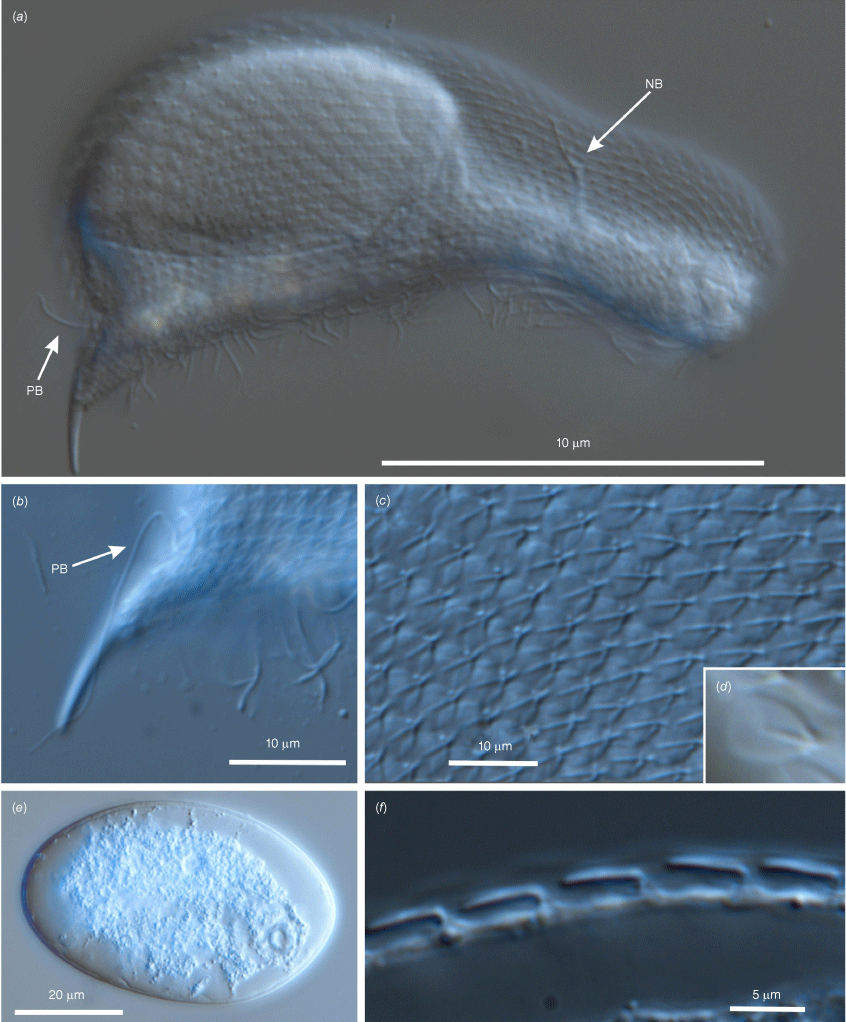
Differential interference contrast photomicrographs showing the morphology of Litigonotus ghinii gen. nov., sp. nov. (a) Habitus of an adult specimen, ventrolateral view. (b) Close up of the anterior region, ventral view, showing locomotor cilia, interciliary field, mouth and hypostomion. (c) Same region at different focal plane, showing the pharynx with two bulbs, and the pharyngeal teeth (arrows).
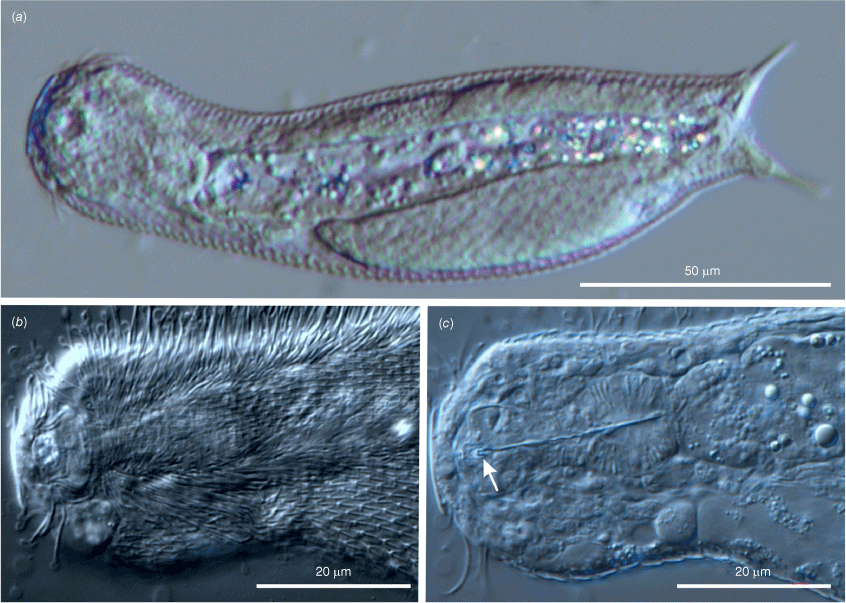
The general appearance and the cuticular characteristics of the other studied adult specimens reflect those of the holotype (e.g. head plates, pedunculated scales covering most of the body, non-pedunculated, keeled scales covering the posterior end, a rather short pharynx bearing two robust bulbs) (Fig. 6a, 7c). Some variability concerned (i) the total body length, which ranged from 135 to 148 μm (mean = 139.5 μm, s.d. = 3.4, n = 3); (ii) the furca length, spanning from 16.0 to 18.0 μm (mean = 16.8 μm, s.d. = 1.0, n = 3); notably, the size of the adhesive tubes did not vary among the measured specimens (constantly 10.2 μm long); (iii) the number of the alternating columns of scales, which numbered as low as 34 and as high as 45 (mean = 38.2 μm, s.d. = 4.6, n = 4). These data indicate the new taxon is characterised by rather limited morphological intraspecific variability. One trait seemed not to fit this statement: the maximum width at the mid trunk, which varied from 42 to 58 μm; however, this significant variation depends on the development stage of the egg inside the specimen; therefore, the trunk width is an unreliable trait for comparisons among specimens.
The general look of the five individuals without an appreciable egg inside (subadults) is very similar to that of the adults (Fig. 8); the main difference regards the body length, which varied from 114 to 128.8 μm (mean = 122.7 μm, s.d. = 6.4, n = 5); notably, the pharynx seems to reach quite soon the full length during the growth of these gastrotrichs since the smallest specimen had a pharynx 28 μm long whereas that of the longest was 30 μm, the same length as the pharynx of the holotype.
Based on morphology, and especially on the form of the cuticular scales, the studied specimen could be affiliated by current taxonomic criteria either to the genus Aspidiophorus, which includes species bearing pedunculated scales (Voigt 1902; Todaro et al. 2019), or to Heterolepidoderma, which includes species possessing keeled scales (Remane 1927; Garraffoni and Melchior 2015; Todaro et al. 2019). Since pedunculated scales cover most of the body of the studied specimens, their affiliation to Aspidiophorus would seem most appropriate. Such a taxonomic decision has been made recently also for the Swedish marine species Aspidiophorus gullmarsfjordensis Kånneby & Todaro, 2017, whose members are covered mainly by pedunculated scales but also bear a few keeled scales on the posterior trunk region (Kånneby and Todaro 2017). Although the Scandinavian and Tuscan species share the unusual combination of pedunculated scales and keeled scales, the specimens of the two taxa are distinguished by several morphometric characteristics. For example, specimens of the Swedish species are larger (186–190 μm v. 135–148 μm in total length), have a three-lobed head (v. five-lobed in the new species), and its interciliary field is bare, whereas in the Tuscan specimens are entirely covered by scales.
Among the freshwater members of the genus Aspidiophorus, there are also species showing combinations of pedunculated scales covering most of the body, and keeled scales in the posterior region, e.g. A. ophiodermus Balsamo, 1983 and A. schlitzensis Schwank, 1990 (Balsamo 1983; Schwank 1990). The specimens of the current study are easily distinguished from both of them due to a number of morphological traits, which include but are not limited to the presence of two robust bulbs in the pharynx (absent in the other two species) and the shape of the pedunculated scales. In fact, A. schlitzensis scales appear smooth (Schwank 1990), lacking the weak slight keel present in the scales of the new species, whereas in A. ophiodermus, the keel extends as a short spiny process beyond the scale itself (Balsamo 1983) in contrast with the new species, where the keel of the scale stops approximately halfway down the scale. In a comparative framework it is worth mentioning that currently none of the species affiliated to Heterolepidoderma bear pedunculated scales. Consequently, it is reasonable to consider the specimens under study belonging to an undescribed species and hence new to science. Genetic information, particularly the knowledge of the entire sequence of the mitochondrial genome, represents formidable additional traits helpful in characterising the new species.
As mentioned earlier, traditional taxonomy based on the current understanding of the anatomical traits could lead to the Tuscan species being affiliated to either Aspidiophorus or Heterolepidoderma; however, our phylogenetic analyses based on the sequences of small and large subunit ribosomal RNA genes consistently indicate that the new species is unrelated to either genus.
In all the analyses, the studied species appears sister to Chaetonotus aff. subtilis in a robustly supported subset of a larger clade, which includes mostly planktonic species belonging to the families Dasydytidae and Neogosseidae (Fig. 1, Supplementary Fig. S1, S2). Furthermore, our analyses found the genus Aspidiophorus polyphyletic, with the marine species, A. paramediterraneus Hummon, 1974, A. polystictos Balsamo & Todaro 1987, A. tentaculatus Wilke, 1954 and Aspidiophorus sp., clustering together along the non-monophyletic Chaetonotidae branch, and the freshwater taxa, A. ophiodermus Balsamo, 1983, A. tetrachaetus Kisielewski, 1986, and two Aspidiophorus spp., spread over the tree (Fig. 1, Supplementary Fig. S1, S2). Similarly, our phylogenetic analyses found Heterolepidoderma non-monophyletic (Fig. 1; Supplementary Fig. S1, S2). Previous studies based on molecular traits have also found Chaetonotidae, Aspidiophorus, and Heterolepidoderma to be non-monophyletic, highlighting the need for a revision of these taxa (e.g. Kånneby et al. 2013; Kolicka et al. 2020; Rataj Križanová and Vďačný 2024). Although such a task is beyond the scope of the present work, not including the new species in Aspidiophorus or Heterolepidoderma and creating a new genus may facilitate it.
In our analysis, the sister taxon of the new genus is Chaetonotus aff. subtilis. The morphology of the organisms corresponding to the sequences of Chaetonotus aff. subtilis (i.e. MN496212/MN496279, MN496228/MN496295) is unknown; however, the name suggests that these specimens are morphologically similar to C. subtilis Kolicka, Kotwicki & Dabert, 2018. Specimens of the latter species have the body covered with three-lobed scales, each bearing a simple spine; pedunculated scales are absent. The striking cuticular dissimilarities and the relatively high number of molecular differences suggest that L. ghinii and C. aff. subtilis belong to two distinct evolutionary lines. Consequently, we propose the name Litigonotus ghinii gen. nov., sp. nov. for the specimens found in Tuscany.
It is fascinating to note that the ancestral homeland of the new species found in the botanical garden of the University of Pisa remains a mystery, as the geographic origin of the founding population is unknown. Interestingly, this phenomenon is not unique to this particular species; other species found in ponds at different botanical gardens or Palm houses share similar stories (e.g. Kolicka et al. 2013). By contrast, the significant difference in the number of specimens collected on the two sampling dates left no doubt that the new species reaches a higher abundance in summer than in winter. These contrasting observations further indicate the need for ongoing research and monitoring to understand better our environment’s biodiversity and ecological changes (Saponi et al. 2024, in press).
The state-of-the-art analysis of gastrotrichs’ mitochondria is certainly very preliminary given the lack of sequences from other representatives of the phylum; therefore, we would like to underline how important it is to obtain mitochondrial sequences from other gastrotrich species to resolve the intricate systematic history of these organisms. Indeed, it has already been demonstrated that in other groups of metazoans a phylogenomic approach based on the entire mitochondrial marker allowed the resolution of similar debated taxonomic questions (Timmermans et al. 2014; López-López and Vogler 2017; Poliseno et al. 2017). Furthermore, the possibility of verifying any similarities or differences in the energy metabolism of gastrotrichs that have colonised ecologically different environments and have different reproductive modalities would be fascinating (Monnens et al. 2020).
Finally, the parallel between the highly conserved mitochondrial genome of the two freshwater Chaetonotidae studied so far, and the generally short length of the phylogenetic branches leading to the different lineages of freshwater gastrotrichs (also symptomatic of little variability) has not escaped our notice. The acquisition and analysis of mitogenomic data of additional taxa showing a different trend could shed light on the origin of Chaetonotidae and help clarify the deep phylogenetic relationships within the family (Kolicka et al. 2020). To simplify communication during this challenging task, we propose the name Oiorpata nom. nov. to define the clade that includes members of the current families Chaetonotidae, Dasydytydae, and Neogosseidae as it emerges consistently from the cladistic analyses performed so far (e.g. Hochberg and Litvaitis 2000; Kieneke et al. 2008; Kånneby et al. 2013; Kånneby and Todaro 2015; Garraffoni et al. 2017; Kolicka et al. 2020; Rataj Križanová and Vďačný 2024). Oiorpata is an alternative term (in Scythian language) for the Amazons, the mythical female warriors. Here, the name alludes to the primary female status (parthenogenetic) of the members of this clade. Oiorpata could also be extended to include members of the families Dichaeturidae and Proichthydidae, although none of them have been included in the molecular phylogenetic analysis conducted so far.
Data availability
The data that support this study are available in the article and accompanying online supplementary material. Sequence of the ribosomal operon of the new species has been deposited in the NCBI GenBank database under Accession number OR915722. The complete mitochondrial genome of the new species has been deposited in NCBI under Accession number PP105008.
Declaration of funding
The research leading to these results has received funding from the European Community’s H2020 Programme H2020-MSCA-RISE 2019 under grant agreement number 872767. This project is partially funded under the National Recovery and Resilience Plan (NRRP), Mission 4 Component 2 Investment 1.4 – Call for tender number 3138 of 16 December 2021, rectified by Decree number 3175 of 18 December 2021 of the Italian Ministry of University and Research funded by the European Union – NextGenerationEU. Project Code CN_00000033, Concession Decree number 1034 of 17 June 2022 adopted by the Italian Ministry of University and Research, CUP E93C22001090001, Project title ‘National Biodiversity Future Center – NBFC’.
Acknowledgements
We thank N. Bekkouche and an anonymous reviewer for their constructive comments on an early draft of the manuscript.
References
Arias-Carrasco R, Vásquez-Morán Y, Nakaya HI, Maracaja-Coutinho V (2018) StructRNAfinder: an automated pipeline and web server for RNA families prediction. BMC Bioinformatics 19, 55.
| Crossref | Google Scholar | PubMed |
Balsamo M (1983) Three new gastrotrichs from a Tuscan–Emilian Apennine lake. Bolletino di Zoologia 49(3–4), 287-295 [1982 volume, published 17 May 1983].
| Crossref | Google Scholar |
Balsamo M, Grilli P, Guidi L, d’Hondt JL (2014) Gastrotricha – biology, ecology and systematics. Families Dasydytidae, Dichaeturidae, Neogosseidae, Proichthydiidae. In ‘Identification guides to the plankton and benthos of inland waters. Vol 24’. (Ed. HJF Bumont) pp. 1–187. (Backhuys Publishers: Weikersheim, Germany)
Bankevich A, Nurk S, Antipov D, Gurevich AA, Dvorkin M, Kulikov AS, Pyshkin AV (2012) SPAdes: a new genome assembly algorithm and its applications to single-cell sequencing. Journal of Computational Biology 19, 455-477.
| Crossref | Google Scholar | PubMed |
Bekkouche N, Worsaae K (2016) Neuromuscular study of early branching Diuronotus aspetos (Paucitubulatina) yields insights into the evolution of organs systems in Gastrotricha. Zoological Letters 2, 21.
| Crossref | Google Scholar | PubMed |
Bernt M, Donath A, Jühling F, Externbrink F, Florentz C, Fritzsch G, Pütz J, Middendorf M, Stadler PF (2013) MITOS: Improved de novo metazoan mitochondrial genome annotation. Molecular Phylogenetics and Evolution 69, 313-319.
| Crossref | Google Scholar | PubMed |
Darriba D, Taboada GL, Doallo R, Posada D (2012) jModelTest 2: more models, new heuristics and parallel computing. Nature Methods 9(8), 772.
| Crossref | Google Scholar |
Garraffoni ARS, Melchior RP (2015) New species and new records of freshwater Heterolepidoderma (Gastrotricha: Chaetonotidae) from Brazil with an identification key to the genus. Zootaxa 4057, 551-568.
| Crossref | Google Scholar | PubMed |
Garraffoni ARS, Araújo TQ, Lourenço AP, Guidi L, Balsamo M (2017) A new genus and new species of freshwater Chaetonotidae (Gastrotricha: Chaetonotida) from Brazil with phylogenetic position inferred from nuclear and mitochondrial DNA sequences. Systematics and Biodiversity 15, 49-62.
| Crossref | Google Scholar |
Golombek A, Tobergte S, Struck TH (2015) Elucidating the phylogenetic position of Gnathostomulida and first mitochondrial genomes of Gnathostomulida, Gastrotricha and Polycladida (Platyhelminthes). Molecular Phylogenetics and Evolution 86, 49-63.
| Crossref | Google Scholar | PubMed |
Guindon S, Gascuel O (2003) A simple, fast, and accurate algorithm to estimate large phylogenies by maximum likelihood. Systematic Biology 52, 696-704.
| Crossref | Google Scholar | PubMed |
Hochberg R, Litvaitis MK (2000) Phylogeny of Gastrotricha: a morphology-based framework of gastrotricha relationships. Biological Bulletin 198, 299-305.
| Crossref | Google Scholar | PubMed |
Hummon WD, Balsamo M, Todaro MA (1992) Italian marine Gastrotricha. I. Six new and one redescribed species of Chaetonotida. Bollettino di Zoologia 59, 499-516.
| Crossref | Google Scholar |
International Commission on Zoological Nomenclature (2017) Declaration 45 – addition of recommendations to article 73 and of the term “specimen, preserved” to the glossary. Bullettin of Zoological Nomenclature 73, 2-4.
| Crossref | Google Scholar |
Kånneby T, Todaro MA (2015) The phylogenetic position of Neogosseidae (Gastrotricha: Chaetonotida) and the origin of planktonic Gastrotricha. Organisms Diversity and Evolution 15, 459-469.
| Crossref | Google Scholar |
Kånneby T, Todaro MA (2017) A new species of Aspidiophorus (Gastrotricha: Chaetonotidae) from the Swedish west coast. Zootaxa 4290, 390-394.
| Crossref | Google Scholar |
Kånneby T, Todaro MA, Jondelius U (2009) One new species and records of Ichthydium Ehrenberg, 1830 (Gastrotricha: Chaetonotida) from Sweden with a key to the genus. Zootaxa 2278, 26-46.
| Crossref | Google Scholar |
Kånneby T, Todaro MA, Jondelius U (2013) Phylogeny of Chaetonotidae and other Paucitubulatina (Gastrotricha: Chaetonotida) and colonization of aquatic ecosystems. Zoologica Scripta 42, 88-105.
| Crossref | Google Scholar |
Katoh K, Rozewicki J, Yamada KD (2019) MAFFT online service: multiple sequence alignment, interactive sequence choice and visualization. Briefings in bioinformatics 20, 1160-1166.
| Crossref | Google Scholar | PubMed |
Kieneke A, Riemann O, Ahlrichs WH (2008) Novel implications for the basal internal relationships of Gastrotricha revealed by an analysis of morphological characters. Zoologica Scripta 37, 429-460.
| Crossref | Google Scholar |
Kolicka M, Kisielewski J, Nesteruk T, Zawierucha K (2013) Gastrotricha from the Poznan Palm House – one new subgenus and three new species of freshwater Chaetonotida (Gastrotricha). Zootaxa 3717, 231-279.
| Crossref | Google Scholar | PubMed |
Kolicka M, Dabert M, Dabert J, Kånneby T, Kisielewski J (2016) Bifidochaetus, a new Arctic genus of freshwater Chaetonotida (Gastrotricha) from Spitsbergen revealed by an integrative taxonomic approach. Invertebrate Systematics 30, 398-419.
| Crossref | Google Scholar |
Kolicka M, Dabert M, Olszanowski Z, Dabert J (2020) Sweet or salty? The origin of freshwater gastrotrichs (Gastrotricha, Chaetonotida) revealed by molecular phylogenetic analysis. Cladistics 36, 458-480.
| Crossref | Google Scholar |
Leasi F, Todaro MA (2008) The muscular system of Musellifer delamarei (Renaud-Mornant, 1968) and other chaetonotidans with implications for the phylogeny and systematisation of the Paucitubulatina (Gastrotricha). The Biological Journal of the Linnean Society 94, 379-398.
| Crossref | Google Scholar |
López-López A, Vogler AP (2017) The mitogenome phylogeny of Adephaga (Coleoptera). Molecular Phylogenetics and Evolution 114, 166-174.
| Crossref | Google Scholar | PubMed |
Monnens M, Thijs S, Briscoe AG, Clark M, Frost EJ, Littlewood DTJ, et al. (2020) The first mitochondrial genomes of endosymbiotic rhabdocoels illustrate evolutionary relaxation of atp8 and genome plasticity in flatworms. International Journal of Biological Macromolecules 162, 454-469.
| Crossref | Google Scholar | PubMed |
Poliseno A, Feregrino C, Sartoretto S, Aurelle D, Wörheide G, McFadden CS, Vargas S (2017) Comparative mitogenomics, phylogeny and evolutionary history of Leptogorgia (Gorgoniidae). Molecular Phylogenetics and Evolution 115, 181-189.
| Crossref | Google Scholar | PubMed |
Rataj Križanová F, Vďačný P (2024) A Heterolepidoderma and Halichaetoderma gen. nov. (Gastrotricha: Chaetonotidae) riddle: integrative taxonomy and phylogeny of six new freshwater species from central Europe. Zoological Journal of the Linnean Society 200(2), 283-335.
| Crossref | Google Scholar |
Remane A (1925) Organisation und systematische Stellung der aberranten Gastrotrichen. Verhandlungen der Deutschen Zoologische Gesellschaft 30, 121-128 [In German].
| Google Scholar |
Ronquist F, Teslenko M, Van Der Mark P, Ayres DL, Darling A, Höhna S, Huelsenbeck (2012) MrBayes 3.2: efficient Bayesian phylogenetic inference and model choice across a large model space. Systematic Biology 61, 539-542.
| Crossref | Google Scholar | PubMed |
Saponi F, Todaro MA (2024) Status of the Italian freshwater Gastrotricha biodiversity, with the creation of an interactive GIS-based web map. Diversity 16, 17.
| Crossref | Google Scholar |
Saponi F, Kosakyan A, Cesaretti A, Todaro MA (2024) How anthropogenic actions affect gastrotrichs diversity in Italian freshwaters. In ‘Symposium: Cambiamento della biodiversità nell’Antropocene: priorità per la Ricerca’, 10–11 April 2024, Fano, Italy. Poster presentation 62. (Consiglio Nazionale delle Ricerche, CNR: Rome, Italy) Available at https://drive.google.com/drive/folders/1gEZWs2B-mRQ1jkm8b_hyrft4k66nSYt5
Saponi F, Kosakyan A, Cesaretti A, Todaro MA (in press) A contribution to the taxonomy and phylogeny of the genus Chaetonotus (Gastrotricha, Paucitubulatina, Chaetonotidae), with the description of a new species from Italian inland waters. European Zoological Journal .
| Google Scholar |
Timmermans MJ, Lees DC, Simonsen TJ (2014) Towards a mitogenomic phylogeny of Lepidoptera. Molecular Phylogenetics and Evolution 79, 169-178.
| Crossref | Google Scholar | PubMed |
Todaro MA, d’Hondt J-L (2023) Gastrotricha. In ‘WoRMS: World Register of Marine Species’. (Flanders Marine Institute) Available at https://www.marinespecies.org/aphia.php?p=taxdetails&id=2078
Todaro MA, Perissinotto R, Bownes SJ (2013) Neogosseidae (Gastrotricha, Chaetonotida) from the iSimangaliso Wetland Park, KwaZulu–Natal, South Africa. Zookeys 315, 77-94.
| Crossref | Google Scholar | PubMed |
Todaro MA, Sibaja-Cordero JA, Segura-Bermúdez OA, Coto-Delgado G, Goebel-Otarola N, et al. (2019) An introduction to the study of Gastrotricha, with a taxonomic key to families and genera of the group. Diversity 11, e117.
| Crossref | Google Scholar |
Voigt M (1902) Diagnosen bisher unbeschreibener Organismen aus Piöner Gewässern. Die Rotatorien und Gastrotrichen Der Umbbung von Ploen. Zoologisher Anzeiger 25, 35-39 [In German].
| Google Scholar |
Westram R, Bader K, Pruesse E, Kumar Y, Meier H, Glöckner FO, Ludwig W (2011) ARB: a software environment for sequence data. In ‘Handbook of Molecular Microbial Ecology I: Metagenomics and Complementary Approaches’. (Ed. FJ Bruijn) pp. 399–406. (Wiley–Blackwell Publishing) 10.1002/9781118010518.ch46