Towards a systematic revision of the superfamily Cyrenoidea (Bivalvia: Imparidentia): species delimitation, multi-locus phylogeny and mitochondrial phylogenomics
Ruiwen Wu


A
B
C
D
E
Handling Editor: Gonzalo Giribet
Invertebrate Systematics 37(9) 607-622 https://doi.org/10.1071/IS23015
Submitted: 28 March 2023 Accepted: 21 August 2023 Published: 11 September 2023
Abstract
Cyrenoidea is a superfamily of bivalves (Bivalvia: Imparidentia) currently comprising three families (Cyrenidae, Cyrenoididae and Glauconomidae). The superfamily is widely distributed in marine, brackish and freshwater environments, with an estimated 60 or more living species. Recent phylogenetic results have confirmed the monophyly of Cyrenoidea and placement in Venerida. Nevertheless, a comprehensive phylogenetic analysis of Cyrenoidea remains elusive and the phylogeny is unresolved due to inadequate sampling in previous studies. Moreover, the taxonomy and delimitation of most species, originally based on shell morphology, have not yet been tested with molecular data. Here, we constructed three molecular datasets by sequencing three markers (COI + 16S rRNA + 28S rRNA) and complete mitogenomes for Geloina coaxans (Gmelin, 1791) and Glauconome virens (Linnaeus, 1767). COI barcoding clarifies the validity of Geloina coaxans and Geloina erosa that have been subject to controversy regarding synonymy. Additionally, the barcoding supports the existence of multiple cryptic species within the Geloina expansa complex. A multi-locus dataset (COI + 16S rRNA + 28S rRNA) provides the most comprehensive phylogeny of all eight recognised genera of Cyrenoidea to date. Phylogenetic results indicate that the currently recognised family Cyrenidae is polyphyletic. The type species Geloina coaxans, Cyanocyclas limosa (Maton, 1811) and Polymesoda caroliniana (Bosc, 1801) that have long been classified within the family Cyrenidae based on shell morphology, have a closer relationship with Cyrenoida floridana Dall, 1896 than with other Cyrenidae. Therefore we transfer the genera Geloina, Cyanocyclas and Polymesoda from the family Cyrenidae to the family Cyrenoididae. The mitochondrial phylogenomics further support the family-level relationships in Cyrenoidea obtained from the three-gene analyses, confirming that the newly defined Cyrenoididae is closely related to Glauconomidae as the sister group. We observed a novel gene arrangement in Glauconome virens, the first report on the mitogenome of the family Glauconomidae, by comparing gene arrangements. Three patterns of gene rearrangement identified in Cyrenoidea are shared by the families Glauconomidae, Cyrenoididae and Cyrenidae, suggesting that gene arrangements can be a valuable tool for phylogenetic studies.
Keywords: Cyrenidae, Cyrenoididae, COI barcoding, gene order, Glauconomidae, Glauconome virens, mitochondrial genomes, molecular phylogeny, taxonomic revision.
Introduction
Bivalves are the second most species-rich molluscan class after Gastropoda (Bieler et al. 2014) and are regarded as commercially and ecologically important groups of aquatic fauna (Kocot et al. 2011; Smith et al. 2011; Vaughn and Hoellein 2018). After decades of puzzling efforts by anatomists, palaeontologists and molecular biologists, bivalve systematics are stabilising, especially at the family level and higher levels (e.g. Newell and Boyd 1978; Popham 1979; Steiner and Müller 1996; Johnston et al. 1998; Carter et al. 2000; Cope 2000; Giribet and Wheeler 2002; Sharma et al. 2012; Plazzi et al. 2013; Bieler et al. 2014; González et al. 2015; Combosch et al. 2017; Lemer et al. 2019; Valentas-Romera et al. 2019; Crouch et al. 2021).
Cyrenoidea Gray, 1840 is a superfamily of bivalves (Bivalvia: Imparidentia) (Bieler et al. 2010). As currently recognised, Cyrenoidea consists of three families: Cyrenidae Gray, 1840, Cyrenoididae H. Adams & A. Adams, 1857 and Glauconomidae Gray, 1853 and includes more than 60 extant species in 8 genera (i.e. Corbicula, Geloina, Cyanocyclas, Cyrenoida, Batissa, Polymesoda, Villorita and Glauconome) (Bieler et al. 2014; MolluscaBase 2022). Cyrenidae is the most diverse (~50 species) of these 3 families and is widely distributed on all continents except Antarctica; Cyrenoididae includes 6 species and is found across the Afrotropics, Central America and North America; Glauconomidae contains ~12 species, mainly distributed in the coastal areas of southern China, India and the Philippines (note: all numbers are for extant species; Bieler et al. 2010, 2014; Bouchet and Rocroi 2010; Coan and Valentich-Scott 2012; Pereira et al. 2014; Huber 2015; Breure et al. 2022). The habitat of most Cyrenoidea taxa is the fringe area between freshwater and saltwater environments, resulting in this group receiving little attention and being left out of larger-scale treatments of marine (e.g. Roy et al. 2009) and freshwater molluscs (e.g. Bogan 2013; Böhm et al. 2021; Graf and Cummings 2021).
Traditionally, the classification of Cyrenoidea at the family level has mainly been based on the anatomy of gills, stomachs, hinge teeth and muscle scar arrangements, and the classification and identification of species are mainly based on comparative morphology of the shell and body (e.g. Newell 1965; Taylor et al. 1969; Beesley et al. 1998; Allen 2000; Schneider 2001; Williams et al. 2004; Taylor and Glover 2006; Valentas-Romera et al. 2019). Due to morphological convergences and phenotypic plasticity, shell morphology-based taxonomy and species synonymy in Cyrenoidea are often controversial (Gray 1853; Dall 1896; Liu 2008; He and Zhuang 2013) and this seriously hinders the assessment of species diversity and the enactment of protection measures for this group.
Over the past 20 years, the molecular phylogenetic framework for bivalves has been updated and improved based on the combined work of several researchers (e.g. Matsumoto 2003; Williams et al. 2004; Taylor et al. 2007, 2009; Sharma and Wheeler 2013; Bieler et al. 2014; Combosch et al. 2017) and the monophyly of Cyrenoidea has been strongly supported. However, previous molecular studies have been lacking in sampling of Cyrenoidea taxa. To date, only four Cyrenoidea (Corbicula fluminea (O. F. Müller, 1774), Polymesoda caroliniana, Glauconome rugosa and Cyrenoida floridana Dall, 1896) have been sampled in the latest bivalve trees (Lemer et al. 2019; Crouch et al. 2021). Therefore, a comprehensive phylogeny of Cyrenoidea is lacking and the species taxonomy and delimitation originally based on morphology and anatomy have not yet been thoroughly tested by molecular analyses.
To confirm the species validity, taxonomic position and phylogenetic relationships, we established three molecular datasets of the Venerida (Bivalvia: Heteroconchia), focusing on Cyrenoidea. A DNA barcoding (cytochrome c oxidase subunit I, COI) dataset was initially used. COI is commonly used for DNA barcoding and widely used for species delimitation and species discovery (Bolotov et al. 2017; Araujo et al. 2018; Wu RW et al. 2018; Smith et al. 2019). We expanded the COI dataset and explored the capability of resolving deeper nodes at the genus level based on a three-gene (i.e. the mitochondrial 16S rRNA and COI, and the nuclear 28S rRNA) dataset. Complete mitogenomes with conserved gene order and low recombination are particularly useful tools for higher taxonomy (Huang et al. 2013; Lopes-Lima et al. 2017; Froufe et al. 2020; Wu RW et al. 2021; Wu XP et al. 2022). Complete mitochondrial genomes might be valuable for enhancing the comprehensiveness of Venerida phylogeny.
The objectives of this study are to (1) determine the validity of the nominal species in Cyrenoidea Gray, 1840 using DNA barcoding; (2) resolve phylogenetic relationships of the eight genera of Cyrenoidea based on multi-locus data and revise the current taxonomy; and (3) produce comprehensiveness of Venerida phylogeny based on complete mitogenomes.
Materials and methods
Taxon sampling and generation of the three molecular datasets
Geloina coaxans (Fig. 1) (SXNU_22071001) was collected in the Dutou River (114.543513°E, 22.740437°N), Huizhou City, Guangdong Province, China. Two specimens of Glauconome virens (Fig. 2) (SXNU_22112301, SXNU_22112302) were collected in the Shankou Mangrove National Ecological Nature Reserve (109.116308°E, 21.448125°N), Beihai City, Guangxi Zhuang Autonomous Region, China. Morphological identification of the species is based on publications (Gmelin 1791; Reeve 1843; Liu 2008) and the WORMS Web Site (MolluscaBase 2023a, 2023b). The identified specimens are deposited as vouchers in the Museum of Zoology, Shanxi Normal University, China.
Shells of Geloina coaxans. View of shells from top to bottom: exterior of right and left valves, dorsal view of articulated valves, interior of right and left valves.
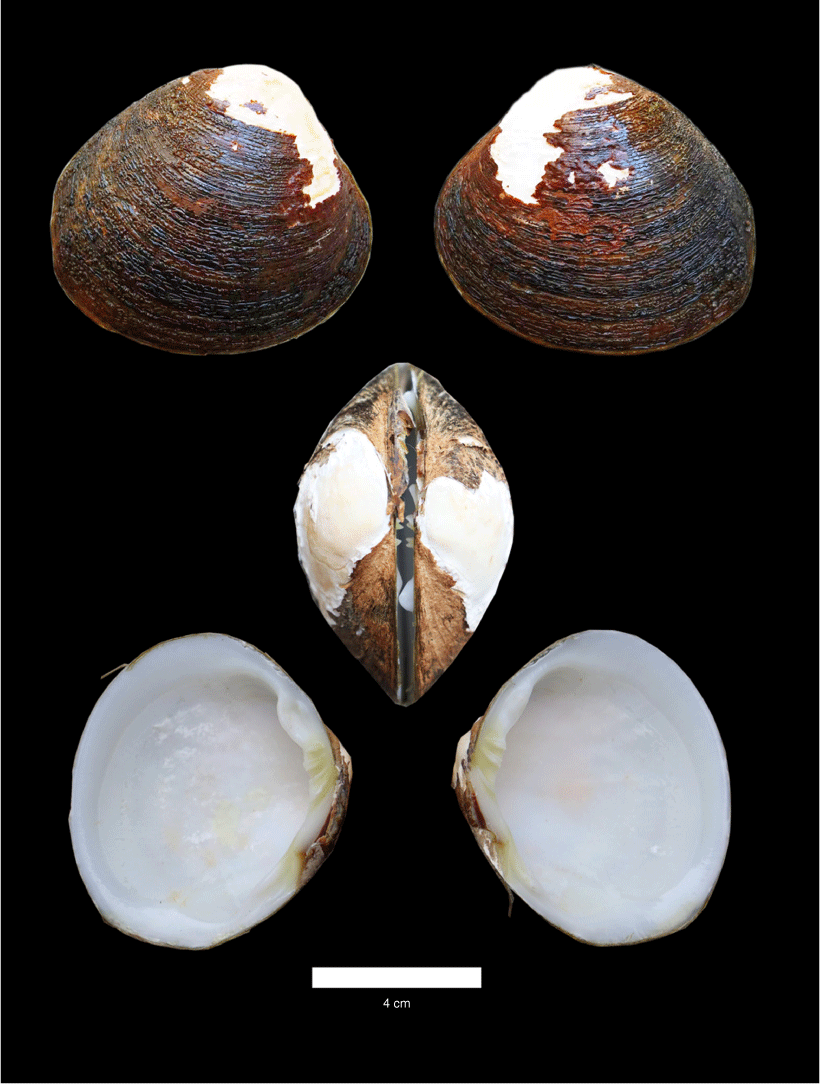
Shells of Glauconome virens. View of shells from top to bottom: exterior of right and left valves, dorsal view of articulated valves, interior of right and left valves.
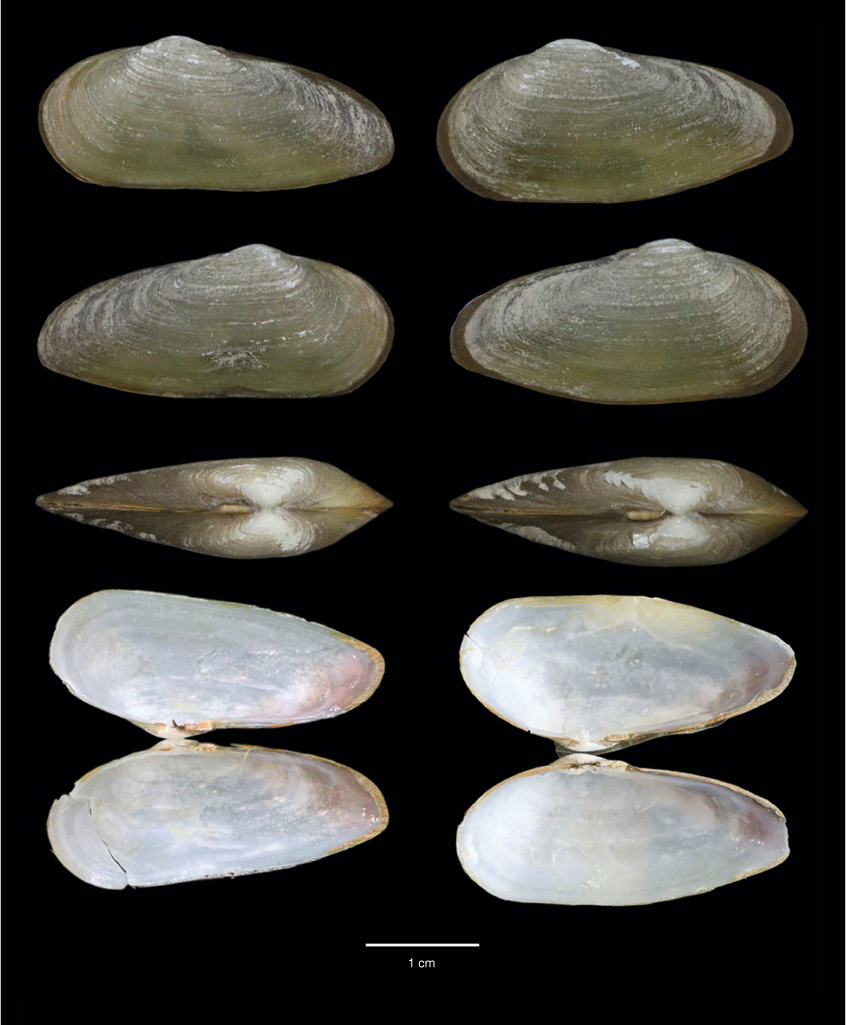
To achieve the objectives of this study, three molecular datasets were constructed: (1) a DNA barcoding (COI) dataset (Table 1): we downloaded the currently available 56 COI sequences representing 21 nominal species in Cyrenoidea from GenBank and combined these with the newly obtained sequences in the present study to construct the DNA barcoding dataset and the sequences from two species in Veneroidea from GenBank were selected as outgroups; (2) we constructed a molecular dataset comprising 13 taxa from 8 genera in Cyrenoidea, using 3 genes (COI, 16S rRNA and 28S rRNA) to clarify phylogenetic relationships (Table 2) and 5 species sequences in Veneridae, Sphaeriidae, Arcticidae and Pharidae were also downloaded from GenBank as outgroups; (3) all 53 complete available mtDNA sequences representing 8 families in Venerida (not including unverified sequences) and 2 Cardiida taxa, i.e. Acanthocardia tuberculata (Linnaeus, 1758) and Cerastoderma edule (Linnaeus, 1758), selected as outgroups were downloaded from GenBank. Two complete new mtDNA sequences (i.e. Geloina coaxans and Glauconome virens) were obtained from this study, of which G. virens is the first mitogenome in the family Glauconomidae. Finally, a mitogenomic dataset was constructed for 57 sequences.
Order | Superfamily | Species | GenBank numbers | References | |
---|---|---|---|---|---|
Venerida Gray, 1854 | Cyrenoidea Gray, 1840 | Corbicula fluminea | MK308235 | Unpublished | |
Corbicula leana | AB845591 | Yamada et al. (2014) | |||
Corbicula leana | MN746814 | Bespalaya et al. (2021) | |||
Corbicula sandai | AB845590 | Yamada et al. (2014) | |||
Corbicula sandai | OM912155 | Unpublished | |||
Corbicula sandai | OM912165 | Unpublished | |||
Corbicula madagascariensis | OM912260 | Unpublished | |||
Batissa violacea | DQ837726 | Glaubrecht et al. (2006) | |||
Batissa violacea | DQ837727 | Glaubrecht et al. (2006) | |||
Villorita cornucopia | MH593260 | Unpublished | |||
Villorita cornucopia | MH593261 | Unpublished | |||
Villorita cornucopia | MH593262 | Unpublished | |||
Villorita cyprinoides | JQ773442 | Unpublished | |||
Villorita cyprinoides | KT347297 | Unpublished | |||
Villorita cyprinoides | MH593257 | Unpublished | |||
Villorita cyprinoides | MH593258 | Unpublished | |||
Villorita cyprinoides | MH593259 | Unpublished | |||
Glauconome angulata | OM292862 | Unpublished | |||
Glauconome angulata | OM292863 | Unpublished | |||
Glauconome chinensis | DQ184851 | Mikkelsen et al. (2006) | |||
Glauconome chinensis | OL877112 | Unpublished | |||
Glauconome chinensis | OL877113 | Unpublished | |||
Glauconome chinensis | OL877114 | Unpublished | |||
Glauconome rugosa | KC429140 | Unpublished | |||
Glauconome rugosa | DQ184852 | Unpublished | |||
Glauconome straminea | MN608341 | Unpublished | |||
Glauconome virens | OQ569915 | This study | |||
Glauconome virens | OQ569916 | This study | |||
Geloina coaxans | OQ569914 | This study | |||
‘Geloina expansa’ | AB722087 | Unpublished | |||
‘Geloina expansa’ | AB722090 | Unpublished | |||
‘Geloina expansa’ | MN608338 | Unpublished | |||
Geloina erosa | OM791690 | Unpublished | |||
Geloina erosa | OM791691 | Unpublished | |||
Geloina erosa | OM791692 | Unpublished | |||
Geloina erosa | OM791693 | Unpublished | |||
Geloina erosa | OM791694 | Unpublished | |||
Geloina expansa | AB498812 | Unpublished | |||
Geloina expansa | AB722089 | Unpublished | |||
Geloina expansa | MW311111 | Unpublished | |||
Geloina expansa | MW311112 | Unpublished | |||
Geloina expansa | AB722088 | Unpublished | |||
Geloina expansa | OM791695 | Unpublished | |||
Geloina expansa | OM791696 | Unpublished | |||
Geloina expansa | OM791697 | Unpublished | |||
Geloina expansa | OM791698 | Unpublished | |||
Geloina expansa | OM791699 | Unpublished | |||
Geloina sp. | KX608980 | Unpublished | |||
Geloina sp. | KX608982 | Unpublished | |||
Geloina sp. | KX608983 | Unpublished | |||
Geloina sp. | MK481951 | Unpublished | |||
Geloina sp. | MK481952 | Unpublished | |||
Cyanocyclas limosa | AF196277 | Siripattrawan et al. (2000) | |||
Cyrenoida floridana | KC429123 | Sharma et al. (2013) | |||
Cyrenoida sp. | MK308013 | Unpublished | |||
Cyrenoida sp. | MK308062 | Unpublished | |||
Cyrenoida sp. | MK308266 | Unpublished | |||
Cyrenoida sp. | MK308305 | Unpublished | |||
Polymesoda caroliniana | AF196276 | Siripattrawan et al. (2000) | |||
Veneroidea Rafinesque, 1815 | Mercenaria mercenaria | KX713477 | Combosch et al. (2017) | ||
Chamelea striatula | KY547747 | Unpublished |
Order | Supfamily | Family | Species | COI | 16S | 28S | |
---|---|---|---|---|---|---|---|
Venerida Gray, 1854 | Cyrenoidea Gray, 1840 | Cyrenidae Gray, 1840 | Corbicula fluminea | MK308235 | MG759516 | DQ343848 | |
Corbicula leana | AB845591 | JX399587 | AB661754 | ||||
Corbicula madagascariensis | OM912260 | AF152022 | KM598271 | ||||
Corbicula sandai | AB845590 | NC_061685 | AB661660 | ||||
Batissa violacea | DQ837726 | KU318348 | – | ||||
Villorita cyprinoides | JQ773442 | KF638716 | – | ||||
Glauconomidae Gray, 1853 | Glauconome rugosa | KC429140 | KC429302 | KC429500 | |||
Glauconome virens | OQ569915 | OQ570957 | OQ608006 | ||||
Glauconome chinensis | DQ184851 | DQ184753 | DQ184798 | ||||
Cyrenoididae H. Adams & A. Adams, 1857 | Cyrenoida floridana | KC429123 | KC429280 | KC429470 | |||
Geloina coaxans | OQ569914 | OQ570956 | OQ608005 | ||||
Cyanocyclas limosa | AF196277 | AF152025 | AF131012 | ||||
Polymesoda caroliniana | AF196276 | KX713250 | KX713425 | ||||
Veneroidea Rafinesque, 1815 | Veneridae Rafinesque, 1815 | Chamelea striatula | KY547747 | KY547771 | KX713366 | ||
Arcticoidea Newton, 1891 | Arcticidae Newton, 1891 | Arctica islandica | KX713445 | KC429288 | AM779737 | ||
Sphaeriida Lemer, Bieler & Giribet, 2019 | Sphaerioidea Deshayes, 1855 | Sphaeriidae Deshayes, 1855 | Musculium indicum | KF483411 | KU376173 | KF483345 | |
Pisidium costulosum | KF483422 | KF483323 | KU376194 | ||||
Adapedonta Cossmann & Peyrot, 1909 | Solenoidea Lamarck, 1809 | Pharidae H. Adams & A. Adams, 1856 | Sinonovacula constricta | AB076949 | AB751361 | AB746907 |
DNA extraction, PCR sequencing and mitogenome assembly
The total genomic DNA of specimens was extracted from the dissected somatic tissues using TIANamp Marine Animals DNA Kit (Tiangen Biotech, Beijing, China) according to the manufacturer’s instructions.
For the newly acquired specimens, COI (LCO22me2 5′-GGTCAACAAAYCATAARGATATTGG-3′ and HCO700dy2 5′-TCAGGGTGACCAAAAAAYCA-3′, ~680 bp) (Walker et al. 2007), 16S rRNA (16SarL 5′-CGCCTGTTTATCAAAAACAT-3′ and 16SbrH 5′-CCGGTCTGAACTCAGATCACGT-3′, ~500 bp) (Wu RW et al. 2019) and 28S rRNA (D23F 5′-GAGAGTTCAAGAGTACGTG-3′ and D4RB 5′-TGTTAGACTCCTTGGTCCGTGT-3′, ~420 bp) (Park and Ó Foighil 2000) were amplified and sequenced. PCR conditions were as follows: 98°C for 10 s, 35 cycles 94°C for 1 min, 50°C for 1 min, 72°C for 1 min and a final extension of 72°C for 7 min, following the TaKaRa Ex manufacturer’s protocol. Amplified PCR products were purified and sequenced by Sangon Biotech (Shanghai). The newly obtained sequences have been uploaded to GenBank (OQ569914–OQ569916 for COI, OQ570956–OQ570957 for 16S, OQ608005–OQ608006 for 28S).
The quality of genomic DNA was detected by agarose gel electrophoresis. We sent samples of high-quality DNA to Novogene Co., Ltd (China) for library construction and sequencing. Paired-end reads of 2 × 150 bp were generated on the Illumina NovaSeq 6000 sequencing platform. Raw data filtration, cleaning and assembly were performed using the CLC Genomic Workbench (ver. 12.0, Qiagen). Mitochondrial genome sequences were designated from resulting contigs using BLAST (ver. 2.14.1, see http://blast.ncbi.nlm.nih.gov/) and concatenated into the complete mitogenome using Geneious (ver. 11, Biomatters, see https://www.geneious.com/). Mitogenomes were annotated by MITOS WebServer (ver. 2.0, see http://mitos2.bioinf.uni-leipzig.de/index.py; Donath et al. 2019) and submitted to GenBank using BankIt (Accession numbers: OQ595194–OQ595195).
Alignments, partitioning strategies and model selection
Protein-coding genes (PCGs) were translated to the corresponding amino acid under the invertebrate codon translation mode in MEGA (ver. 7.0, see http://www.megasoftware.net; Kumar et al. 2016) and aligned based on the amino acid sequences using the built-in MUSCLE (ver. 5.0, see http://www.drive5.com/muscle; Edgar 2004) with default settings. The ribosomal genes 16S rRNA and 28S rRNA were aligned using MAFFT (ver. 7.2, see https://mafft.cbrc.jp/alignment/server/; Katoh and Standley 2013) with the L-INS-i algorithm. Ambiguous alignment areas were trimmed by Gblocks (ver. 0.91b, see http://molevol.cmima.csic.es/castresana/Gblocks.html; Castresana 2000), the parameter ribosomal gene block with a minimum length was set to 2 base pairs (bp), allowed gap position was selected with half; the minimum length of protein-coding gene block was set to 3 bp, allowed gap position was also selected with half.
The COI sequence fragment length was 657 bp after alignment and trimming for the barcoding dataset. The COI, 16S rRNA and 28S rRNA sequences were 459, 1069 and 576 bp respectively after alignment and trimming for the three-gene dataset. The resulting alignment of 2104 nucleotides was concatenated in Phylosuite (ver. 1.2.3, see http://phylosuite.jushengwu.com/; Zhang et al. 2020).
We analysed two data sets for the mitogenomic data: (1) nucleotide sequences of 12 PCGs (NUC dataset); and (2) amino acid sequences of 12 PCGs (AA dataset). The concatenated NUC and AA datasets consisted of 10 698 bp and 3566 aa. Atp8 was removed for the mitogenomic dataset due to high sequence variation and a lack of annotation in some species.
The multi-locus dataset was performed with five partition schemes based on genes and codons, i.e. COI (3 codons) + 16S rRNA + 28S rRNA. PartitionFinder (ver. 2.1.1, see http://www.robertlanfear.com/partitionfinder/; Lanfear et al. 2012) was used to select Bayesian inference (BI) analysis models for partitioning schemes. ModelFinder (ver. 1.4.2, see http://www.iqtree.org/ModelFinder/; Kalyaanamoorthy et al. 2017) was used to select the maximum likelihood (ML) analysis models in IQ-TREE. The selection for best-fit models was based on the corrected Akaike Information Criterion (AICc). Substitution models assigned to each partition by PartitionFinder and ModelFinder are listed in Supplementary Table S2.
The mitochondrial genome NUC dataset was partitioned into 36 partitions according to the codon position and each PCG; and the AA dataset was partitioned into 12 partitions according to each PCG for phylogenetic analyses. Selection for best-fit models using ModelFinder and PartitionFinder was based on AICc. The best-fit partitioning schemes and subset models were shown in Supplementary Table S3.
Neighbour-joining clustering and species delimitation approaches
Molecular species delimitation is mainly determined by the barcode gap and for this purpose, we performed a neighbour-joining (NJ) analysis of the COI dataset. An NJ tree based on p-distances was generated in MEGA (ver. 7.0; Kumar et al. 2016) with 1000 bootstrap replicates (Minh et al. 2013) using the ‘Pairwise deletion’ option to treat gaps and missing data.
To view the validity of the nominal species and examine the possible presence of cryptic species, we implemented the following three species delimitation tools on the barcoding data: Automatic Barcode Gap Discovery (ABGD), multi-rate Poisson Tree Processes (mPTP) and Species Tree And Classification Estimation, Yarely (STACEY).
ABGD analyses (Puillandre et al. 2012) were performed at the webserver (see http://wwwabi.snv.jussieu.fr/public/abgd/) using the default value of prior intraspecific divergence values (Pmin = 0.001 and Pmax = 0.1); relative gap width (X) was set to 1.0; the matrix computes of pairwise distances were selected p-distances models. mPTP analyses (Kapli et al. 2017) were performed on the NJ tree of COI data using the online server (http://mptp.h-its.org). STACEY (ver. 1.2.4, see https://beast2.blogs.auckland.ac.nz/tag/stacey/; Jones 2017) was implemented in BEAST (ver. 2.0, see http://www.beast2.org/; Bouckaert et al. 2014); and parameter settings included collapse-height = 0.0001, simcutoff = 1.0 and burn-in 50%.
Phylogenetic analyses
We employed both Bayesian inference (BI) and maximum likelihood (ML) methods with various software packages to produce a robust set of phylogenetic hypotheses based on the comprehensive three-gene and mitogenome datasets.
Bayesian analysis was performed using MrBayes (ver. 3.2.6, see http://nbisweden.github.io/MrBayes/; Ronquist et al. 2012). Four simultaneous runs with four chains each were run for 10 million generations, sampling every 1000 trees. The first 25% of these trees was discarded as burn-in when computing the consensus tree (50% majority rule). Sufficient mixing of the chains was considered to be reached when the average standard deviation of split frequencies was below 0.01.
IQ-TREE (Nguyen et al. 2015) was used for ML tree reconstruction, using partition models with 1000 ultrafast bootstrap replicates (Minh et al. 2013). Additionally, a ML analysis was conducted in RAxML (ver. 2.0, see https://antonellilab.github.io/raxmlGUI/; Stamatakis 2014), with the search strategy including rapid bootstrapping. Clade support was evaluated by 1000 bootstrap replicates, assuming a generalised time reversible (GTR) + gamma (G) + proportion of invariable sites (I) model for each partition.
Gene arrangement comparisons
We batch-downloaded 53 complete mitochondrial genomes in Venerida from GenBank using PhyloSuite (Zhang et al. 2020) and assessed genomic features and gene order. All complete mitogenome sequences were also re-annotated by MITOS (Donath et al. 2019) to avoid the influence of different annotation methods on the analysis results.
Phylograms and gene orders were visualised in iTOL (ver. 6.0, see https://itol.embl.de/itol.cgi; Letunic and Bork 2007). For visualisation, we arbitrarily designated the beginning of the COI gene as position 1 in each genome (pointing in the direction of COII).
Results
Species delimitation
We examined the COI dataset of 59 sequences representing 21 nominal species in Cyrenoidea. The three species delimitation analyses consistently identified 19 OTUs (molecular operational taxonomic units) (Fig. 3).
Neighbour-joining tree inferred from 59 COI sequences representing 21 nominal species in Cyrenoidea based on p-distances. Taxa marked in red were obtained in this study. Taxon blocks represent the OTUs defined by three delimitation analyses. The blue branches represent four clades separated by the Geloina expansa complex, each of which corresponds to an OTU.
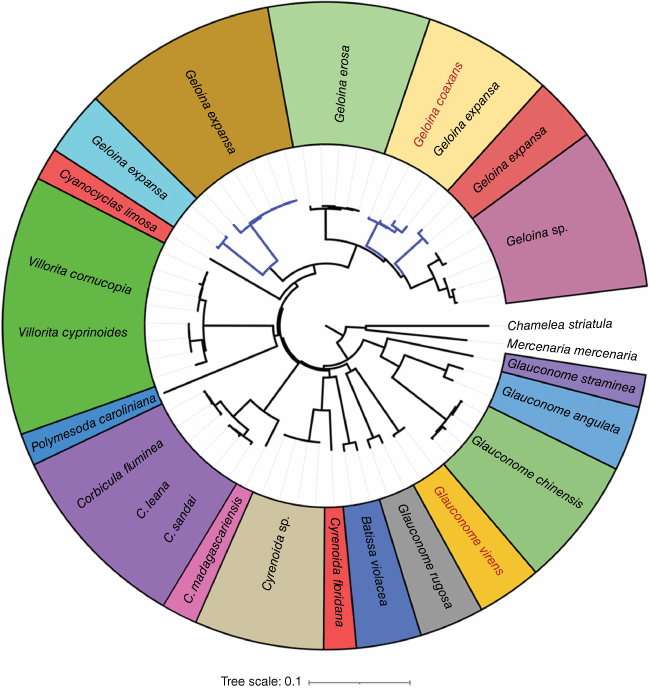
Based on ABGD, the barcode gap distance was 0.04. Estimates identified that OTU divergence (p-distances) ranged between 0.06 (Corbicula madagascariensis v. Corbicula spp.) and 0.26 (Corbicula madagascariensis v. Polymesoda caroliniana) within Cyrenoidea.
The following congeneric species were indistinguishable according to these methods: Villorita cornucopia + Villorita cyprinoides (divergence = 0.02) and Corbicula fluminea + Corbicula leana + Corbicula sandai (average divergence = 0.01), providing evidence that there are possible errors in synonymisation from previous taxonomists. Interestingly, the Geloina expansa sequences retrieved from GenBank were clustered into four distinct clades and the Geloina coaxans specimens identified in this study formed a shallow branch with one of these clades.
Phylogenetic relationships of Cyrenoidea
BI and ML analyses based on the three-gene dataset showed a congruent topology (Fig. 4). Cyrenoidea was the sister-group to Arcticoidea with strong support (BS = 80%, PP = 0.98). Both phylogenetic trees confirmed that the currently recognised family Cyrenidae was polyphyletic. Geloina coaxans, Polymesoda caroliniana and Cyanocyclas limosa, previously classified in the family Cyrenidae, formed a sister group relationship with Cyrenoida floridana that belongs to the family Cyrenoididae (Fig. 4). With the exception of Batissa violacea and Cyrenoida floridana, our taxa contained type species for each genus. BI and ML trees showed that the phylogenetic relationships among genera were as follows: (((Batissa + Villorita) + Corbicula) + (Glauconome + (Cyrenoida + (Geloina + (Cyanocyclas + Polymesoda))))) (Fig. 4).
Maximum likelihood (ML) and Bayesian inference (BI) trees of Imparidentia based on the three-gene dataset (five partitions: three codons of COI + 16S rDNA + 28S rDNA). BI tree generated by Bayesian analysis and ML tree generated by IQ-TREE analysis and RAxML produce completely congruent topology. We only show bootstrap values executed by IQ-TREE here because bootstrap values inferred from RAxML are less than 50 in some nodes. Numbers at nodes are statistical support values for ML (bootstrap support, BS)/BI (posterior probability, PP). Three clades representing three families within Cyrenoidea are coloured accordingly. The colour-coded taxa represent the corresponding eight genera in Cyrenoidea.
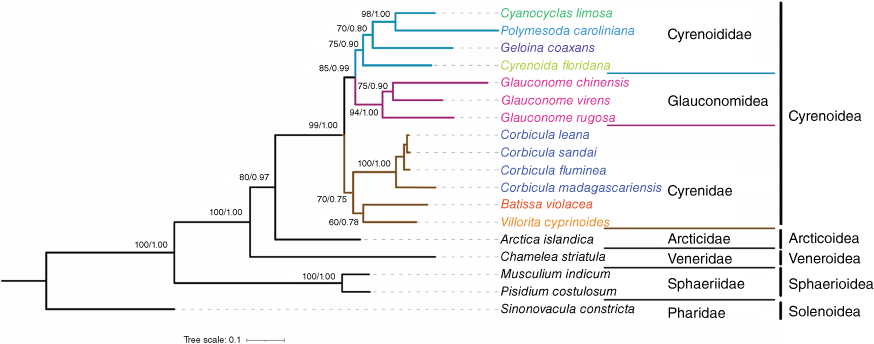
Mitogenomics
We constructed five phylogenetic trees inferred from 12 mitochondrial protein genes. ML and BI analyses of the NUC data and ML analyses of the AA data yielded congruent topologies. Most relationships across the Venerida were well-supported, with most nodes having high bootstrap support values and Bayesian posterior probabilities (BS ≥ 75%, PP ≥ 0.95; Fig. 5). The AA-based BI tree differed from the abovementioned topology and the only distinction was the position of Ruditapes decussatus (Supplementary Fig. S1). In all phylogenetic trees, Cyrenidae, Cyrenoididae and Glauconomidae formed a clade with strong nodal support (BS = 100%, PP = 1.0) and obtained topologies consistent with the three-gene dataset.
Gene arrangement of the complete mitogenomes of Venerida mapped on the phylogenetic trees that were inferred from 12 mitochondrial protein genes (except atp8). We show the consistent phylogenetic tree built by Bayesian Inference (BI) based on the NUC dataset and Maximum Likelihood (ML) analyses conducted by IQ-TREE based on both NUC and AA datasets, and RAxML based on the NUC dataset. The dotted lines represent the branch at which the BI tree based on AA data is topologically inconsistent with other trees. Numbers close to the branching points indicate ML bootstrap support (BS) values (above, AA_IQ-TREE/NUC_IQ-TREE/NUC_RAxML) and Bayesian posterior probabilities (PP) (below, AA/NUC). Nodes without support values and an asterisk (*) are 100 BS/PP across all five analyses. The newly generated mitogenomes are marked by red star signs. Coloured clades represent the corresponding families in Venerida. GO, Gene order. The number after the gene arrangement is the complete mitogenome length.
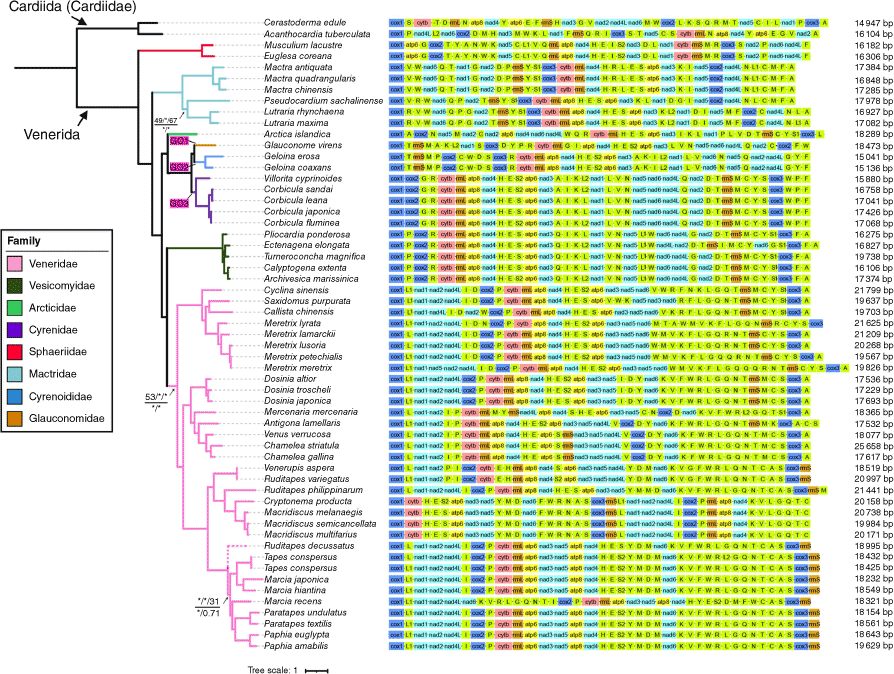
Within Venerida, BI and ML analyses revealed the phylogenetic relationships of eight families as follows: ((((((Glauconomidae + Cyrenoididae) + Cyrenidae) + Arcticidae) + (Veneridae + Vesicomyidae)) + Mactridae) + Sphaeriidae). Cyrenoidea was the sister group to Arcticidae. Sphaeriidae was the sister group to all the remaining Venerida in all IQ-TREE, MrBayes and RAxML analyses (Fig. 5, Supplementary Fig. S1).
Novel mitochondrial gene arrangements
Mitogenomes of Geloina coaxans and Glauconome virens were newly generated in this study. Both sequenced mitogenomes displayed a typical structure composed of 13 protein-coding genes, 22 tRNA genes and 2 rRNA genes (Fig. 5). All 37 genes were located on the plus strand. The mitogenomes ranged from 15 136 bp in Geloina coaxans to 18 473 bp in Glauconome virens. The base composition of G. coaxans was A = 23.57%, T = 45.98%, C = 7.87% and G = 22.58% that showed a high AT-bias (69.55%). The base composition of virens was A = 26.47%, T = 42.45%, C = 9.01% and G = 22.08%, also with an AT-bias of AT (68.92%). AT skews in sequenced G. coaxans and G. virens mitogenomes were −0.32 and −0.23 respectively. GC skew values were similar and these were 0.48 for G. coaxans and 0.42 for G. virens.
Geloina coaxans exhibited the identical gene rearrangement as that previously reported for Geloina erosa (Liao et al. 2020). However, we found a novel and unique mitochondrial gene order in Glauconome virens that differed significantly from the gene order reported for other species in Venerida (Fig. 5). We mapped the gene order of these mitogenomes onto the Venerida phylogeny based on 12 PCGs.
Discussion
Species delimitation
OTU results obtained from the three methods (ABGD, mPTP and STACEY) suggest that species may require revision, including the possible existence of a species complex in the currently known Geloina expansa.
Based on morphological similarities, Huber (2015) proposed Villorita cornucopia as a variant of Villorita cyprinoides. V. cornucopia is currently accepted as a synonym of V. cyprinoides in the World Register of Marine Species (https://www.marinespecies.org). Our species-delimitation methods using COI sequences also support this synonymisation.
The genus Corbicula is of great concern for malacologists worldwide due to the high invasive potential (e.g. Pigneur et al. 2014; Gomes et al. 2016; Benson and Williams 2021; Bespalaya et al. 2021, 2023). Species descriptions in this group were typically based on slight differences in shell shape, size and colouration (e.g. Lamarck 1818; Dall 1903; Ota and Ohta 1970). At present, 91 species of the genus Corbicula have been described globally (Bieler and Mikkelsen 2019; Graf and Cummings 2021; MolluscaBase, see https://www.molluscabase.org). Although subsequent studies have attempted to clarify the nominal species, the validity of many of these remains controversial (Okamoto and Arimoto 1986; Park and Kim 2003; Benson and Williams 2021). In our study, the divergences of Corbicula fluminea, Corbicula leana and Corbicula sandai were so small that the three methods employed for species delimitation failed to distinguish among these species. Our analysis focused exclusively on the molecular data of these species without considering voucher-specimen information. We do not support synonymising species based on a single locus analysis. The aim of this study was not to provide integrative evidence to resolve the synonymisation of the Corbicula complex. Rather, we intend this group to remain in the spotlight, awaiting comprehensive examination, after our casual molecular analysis.
The species validity and synonymy of Geloina coaxans, G. erosa and G. expansa have been controversial since descriptions were typically inferred only from shell characters in the absence of comparative soft-anatomy and molecular data (e.g. Morton 1976; He and Zhuang 2013; Huber 2015). Morton (1976) and He and Zhuang (2013) believed that G. erosa was not morphologically distinct from G. coaxans and regarded G. erosa as a variety of the latter. But Huber (2015) proposed G. erosa as a junior synonym of G. expansa by comparing the conchological characters. Currently, two of the world’s most comprehensive bivalve species websites, i.e. World Register of Marine (https://www.marinespecies.org) and the MUSSEL Project (http://www.mussel-project.net/), support inconsistent species synonyms. We downloaded all available barcode data of Geloina from GenBank and combined with sequences of G. coaxans obtained in this study, species delimitation supported the validity of G. erosa and G. coaxans and the existence of multiple cryptic species in the G. expansa complex.
Synonymising species or proposing new species in controversial congeneric species would be tentative, solely relying on shell morphological variation and insufficient molecular evidence. The addition of unlinked markers and integration of reproductive characteristics, and ecological and behavioural data should be underatken to further clarify the above concept of species before a comprehensive taxonomic classification of the group can be established.
Phylogenetic relationships and taxonomic revision
Valentas-Romera et al. (2019) conducted a comprehensive review of the anatomical data of Cyrenoidea and provided a succinct summary of the relevant research history. The monophyly of the superfamily Cyrenoidea including the freshwater–brackish water families Cyrenoididae, Cyrenidae and Glauconomidae in Imparidentia has been confirmed (Taylor et al. 2009; Sharma et al. 2012; Bieler et al. 2014; Combosch et al. 2017). Subsequent studies (Rahuman et al. 2020; Crouch et al. 2021) further confirmed the close relationship of three families in Cyrenoidea as (Cyrenidae + (Cyrenoididae + Glauconomidae)). More recently, the transcriptomic study conducted by Lemer et al. (2019) included representatives from Cyrenidae (Corbicula fluminea (O. F. Müller, 1774) and Polymesoda caroliniana (Bosc, 1801)), Glauconomidae (Glauconome rugosa Hanley, 1843) and Cyrenoididae (Cyrenoida floridana). Notably, P. caroliniana grouped with G. rugosa instead of C. fluminea (Lemer et al. 2019), indicating that the monophyly of the Cyrenidae is questionable and requires revision.
In this study, we collected 13 taxa, representing the currently recognised eight genera, to construct the most comprehensive phylogenetic framework of Cyrenoidea (Fig. 4). Multi-locus phylogenetic analyses provide compelling evidence to question the traditional taxonomy based on shell characteristics. Geloina coaxans, Cyanocyclas limosa and Polymesoda caroliniana as type taxon of the respective genera have been classified in the family Cyrenidae based on shell morphology (e.g. Gray 1840; Keen and McLean 1971; Turgeon et al. 1998). Our study shows that these three species are more closely allied to Cyrenoida floridana that belongs to the family Cyrenoididae, rather than Corbicula fluminea, the type taxon of the family Cyrenidae (Fig. 4). Previous phylogenetic studies (González et al. 2015; Kondakov et al. 2020) based on different datasets also showed that P. caroliniana was more closely related to C. floridana, albeit with a sampling strategy not designed to resolve cyrenoidean relationships. Incorporating the type species of Cyrenoididae, Cyrenoida dupontia Joannis, 1835 from West Africa, into the molecular analysis would have been preferable. However, no suitably preserved specimens were available. Taylor et al. (2009) compared the shell characteristics of C. floridana and C. dupontia, including shell sculpture, cardinal teeth and lateral teeth, and were convinced that these were members of the same group.
Consequently, with the reinforcement of the three-gene dataset, our phylogenetic studies provide strong evidence to transfer Geloina, Cyanocyclas and Polymesoda from the family Cyrenidae to the family Cyrenoididae. Multi-locus phylogeny and mitochondrial phylogenomics consistently support the family-level placement of the newly delimited Cyrenoididae as sister group to Glauconomidae (Fig. 4 and 5).
Gene rearrangement of Venerida
In contrast to the conserved mitochondrial gene order observed in most vertebrate groups, bivalves exhibit a remarkably diverse mitochondrial gene arrangement (Boore and Brown 1994; Boore et al. 1999; Kurabayashi and Ueshima 2000; Froufe et al. 2020) that is attributed to various mechanisms such as gene transpositions and reverse, tandem duplication and random loss events (Boore 2000; Wu RW et al. 2021). Although homoplastic rearrangements have been reported in some invertebrate groups, such as insects (Dowton and Austin 1999; Babbucci et al. 2014), the complete mitochondrial gene order of bivalves exhibits a unique and specific diagnostic signal that can provide powerful indicators for inferring phylogenetic and evolutionary relationships (Yuan et al. 2012; Lv et al. 2018; Froufe et al. 2020).
In this study, the mitochondrial genomes of Geloina coaxans and Glauconome virens were obtained for the first time. Notably, G. virens represents the first complete mitochondrial genome of Glauconomidae. In terms of length and nucleotide composition, the mitochondrial genome of G. virens in Venerida is not atypical; however, this does possess a distinctive gene arrangement structure. Consequently, three distinct mitochondrial gene orders (GO1, GO2 and GO3) are identified in Cyrenoidea, each of which is shared by the different families: Glauconomidae, Cyrenoididae and Cyrenidae (Fig. 5). We anticipate that these three mitogenome gene orders may serve as a diagnostic tool for family-level classification but further validation will be required through incorporating additional mitogenomes for this group in the future.
In conclusion, we establish a first modern phylogenetic framework for the superfamily Cyrenoidea and revise the taxonomic placement of three genera using a multi-locus phylogeny. We clarify the phylogenetic relationship of the family within Venerida based on mitochondrial phylogenomics and describe a novel gene arrangement from the complete mitochondrial genome of the Glauconome virens. Cyrenoidea has a wide distribution range that covers marine, brackish- and fresh-water habitats. This diversity of habitats could constitute a sound model for understanding transitions from ocean to freshwater habitats in bivalves. In addition, due to the imbalance in bivalve studies around the world, the multiple data of type taxon Batissa tenebrosa (Hinds, 1842) from Fiji (Hinds 1842) and Cyrenoida dupontia Joannis, 1835 from the Senegal River (De Joannis 1835) were only conchological descriptions. We are committed to exploring the morphological diagnosis, character evolution and biogeographic history of Cyrenoidea by expanding the sampling and strengthening international cooperation.
Data availability
The sequences obtained through this study have been uploaded in the GenBank Nucleotide Database and the accession numbers are OQ569914–OQ569916 for COI, OQ570956–OQ570957 for 16S, OQ608005–OQ608006 for 28S and OQ595194–OQ595195 for complete mtDNA.
Declaration of funding
This work was funded by the National Natural Science Foundation of China (number 32200370), the Basic Research Program of Shanxi Province, China (number 20210302124253) and the Special Foundation for National Science and Technology Basic Research Program of China (2019FY101903).
Author contributions
Ruiwen Wu designed the study. Ruiwen Wu, Lili Liu, Xiongjun Liu, Yingying Ye, Zhicai Xie, Zhenyuan Liu and Zhengfei Li undertook field research and sampling. Xiaoping Wu, Lili Liu, Xiongjun Liu and Zhenyuan Liu conducted the data analysis. Ruiwen Wu, Zhicai Xie, Xiaoping Wu and Zhengfei Li wrote and edited the manuscript. All authors read and approved the final version of the manuscript.
Acknowledgements
We express our sincere gratitude to the editor Dr Gonzalo Giribet for his insightful comments. Thanks also to the anonymous reviewers for positive and constructive comments on the manuscript.
References
Allen JA (2000) An unusual suctorial montacutid bivalve from the deep Atlantic. Journal of the Marine Biological Association of the United Kingdom 80(5), 827-834.
| Crossref | Google Scholar |
Araujo R, Buckley D, Nagel KO, García-Jiménez R, Machordom A (2018) Species boundaries, geographic distribution and evolutionary history of the Western Palaearctic freshwater mussels Unio (Bivalvia: Unionidae). Zoological Journal of the Linnean Society 182(2), 275-299.
| Crossref | Google Scholar |
Babbucci M, Basso A, Scupola A, Patarnello T, Negrisolo E (2014) Is it an ant or a butterfly? Convergent evolution in the mitochondrial gene order of Hymenoptera and Lepidoptera. Genome Biology and Evolution 6(12), 3326-3343.
| Crossref | Google Scholar | PubMed |
Bespalaya YV, Aksenova OV, Gofarov MY, Kondakov AV, Kropotin AV, Kononov OD, Bolotov IN (2021) Who inhabits the world’s deepest crater lake? A taxonomic review of Corbicula (Bivalvia: Cyrenidae) clams from Lake Toba, North Sumatra, Indonesia. Journal of Zoological Systematics and Evolutionary Research 59, 400-410.
| Crossref | Google Scholar |
Bespalaya YV, Kropotin AV, Kondakov AV, Aksenova OV, Gofarov MY, Kim SK, Lee JH, Travina OV, Vikhrev IV, Vinarski MV, Bolotov IN (2023) A taxonomic reassessment of native and invasive species of Corbicula clams (Bivalvia: Cyrenidae) from the Russian Far East and Korea. Zoological Journal of the Linnean Society 197(1), 104-126.
| Crossref | Google Scholar |
Bieler R, Carter JG, Coan EV (2010) Classification of Bivalve families. Malacologia 52(2), 113-133.
| Google Scholar |
Bieler R, Mikkelsen PM, Collins TM, Glover EA, González VL, Graf DL, Harper EM, Healy J, Kawauchi GY, Sharma PP, Staubach S, Strong EE, Taylor JD, Tëmkin I, Zardus JD, Clark S, Guzmán A, McIntyre E, Sharp P, Giribet G (2014) Investigating the Bivalve Tree of Life-an exemplar-based approach combining molecular and novel morphological characters. Invertebrate Systematics 28(1), 32-115.
| Crossref | Google Scholar |
Böhm M, Dewhurst-Richman NI, Seddon M, Ledger SEH, Albrecht C, Allen D, Bogan AE, Cordeiro J, Cummings KS, Cuttelod A, Darrigran G, Darwall W, Fehér Z, Gibson C, Graf DL, Köhler F, Lopes-Lima M, Pastorino G, Perez KE, Smith K, van Damme D, Vinarski MV, von Proschwitz T, von Rintelen T, Aldridge DC, Aravind NA, Budha PB, Clavijo C, Van Tu D, Gargominy O, Ghamizi M, Haase M, Hilton-Taylor C, Johnson PD, Kebapçı Ü, Lajtner J, Lange CN, Lepitzki DAW, Martínez-Ortí A, Moorkens EA, Neubert E, Pollock CM, Prié V, Radea C, Ramirez R, Ramos MA, Santos SB, Slapnik R, Son MO, Stensgaard A-S, Collen B (2021) The conservation status of the world’s freshwater molluscs. Hydrobiologia 848, 3231-3254.
| Crossref | Google Scholar |
Bolotov IN, Vikhrev IV, Kondakov AV, Konopleva ES, Gofarov MY, Aksenova OV, Tumpeesuwan S (2017) New taxa of freshwater mussels (Unionidae) from a species-rich but overlooked evolutionary hotspot in Southeast Asia. Scientific Reports 7, 11573.
| Crossref | Google Scholar | PubMed |
Boore JL, Brown WM (1994) Mitochondrial genomes and the phylogeny of mollusks. The Nautilus 108(2), 61-78.
| Google Scholar |
Boore JL, Daehler LL, Brown WM (1999) Complete sequence, gene arrangement, and genetic code of mitochondrial DNA of the cephalochordate Branchiostoma floridae (Amphioxus). Molecular Biology and Evolution 16(3), 410-418.
| Crossref | Google Scholar | PubMed |
Bouchet P, Rocroi JP (2010) Nomenclator of bivalve families with a classification of bivalve families by R. Bieler, J.G. Carter & E.V. Coan. Malacologia 52(2), 1-184.
| Google Scholar |
Bouckaert R, Heled J, Kühnert D, Vaughan T, Wu CH, Xie D, Suchard MA, Rambaut A, Drummond AJ (2014) BEAST 2: a software platform for Bayesian evolutionary analysis. PLoS Computational Biology 10(4), e1003537.
| Crossref | Google Scholar | PubMed |
Breure ASH, Roosen MT, Ablett JD (2022) Land and freshwater molluscs of mainland Ecuador: an illustrated checklist. Iberus 40(1), 1-290.
| Google Scholar |
Carter JG, Campbell DC, Campbell MR (2000) Cladistic perspectives on early bivalve evolution. Geological Society, London, Special Publications 177(1), 47-79.
| Crossref | Google Scholar |
Castresana J (2000) Selection of conserved blocks from multiple alignments for their use in phylogenetic analysis. Molecular Biology and Evolution 17, 540-552.
| Crossref | Google Scholar | PubMed |
Coan EV, Valentich-Scott P (2012) Bivalve seashells of tropical West America. Marine bivalve mollusks from Baja California to northern Peru. Santa Barbara Museum of Natural History 2, 1258.
| Google Scholar |
Combosch DJ, Collins TM, Glover EA, Graf DL, Harper EM, Healy JM, Kawauchi GY, Lemer S, Mcintyre E, Strong EE, Taylor JD, Zardus JD, Mikkelsen PM, Giribet G, Bieler R (2017) A family-level Tree of Life for bivalves based on a Sanger-sequencing approach. Molecular Phylogenetics and Evolution 107, 191-208.
| Crossref | Google Scholar | PubMed |
Crouch NMA, Edie SM, Collins KS, Bieler R, Jablonski D (2021) Calibrating phylogenies assuming bifurcation or budding alters inferred macroevolutionary dynamics in a densely sampled phylogeny of bivalve families. Proceedings of the Royal Society of London – B. Biological Sciences 288, 20212178.
| Crossref | Google Scholar | PubMed |
Dall WH (1896) On the American species of Cyrenoidea. The Nautilus 10(5), 51-52.
| Google Scholar |
Dall WH (1903) Review of the classification of the Cyrenacea. Proceedings of the Biological Society of Washington 16, 5-8.
| Google Scholar |
Donath A, Jühling F, Al-Arab M, Bernhart SH, Reinhardt F, Stadler PF, Middendorf M, Bernt M (2019) Improved annotation of protein-coding genes boundaries in metazoan mitochondrial genomes. Nucleic Acids Research 47(20), 10543-10552.
| Crossref | Google Scholar | PubMed |
Dowton M, Austin AD (1999) Evolutionary dynamics of a mitochondrial rearrangement “hot spot” in the hymenoptera. Molecular Biology and Evolution 16(2), 298-309.
| Crossref | Google Scholar | PubMed |
Edgar RC (2004) Muscle: multiple sequence alignment with high accuracy and high throughput. Nucleic Acids Research 32(5), 1792-1797.
| Crossref | Google Scholar | PubMed |
Froufe E, Bolotov I, Aldridge DC, Bogan AE, Breton S, Gan HM, Kovitvadhi U, Kovitvadhi S, Riccardi N, Secci-Petretto G, Sousa R, Teixeira A, Varandas S, Zanatta D, Zieritz A, Fonseca MM, Lopes-Lima M (2020) Mesozoic mitogenome rearrangements and freshwater mussel (Bivalvia: Unionoidea) macroevolution. Heredity 124, 182-196.
| Crossref | Google Scholar | PubMed |
Giribet G, Wheeler W (2002) On bivalve phylogeny: a high-level analysis of the Bivalvia (Mollusca) based on combined morphology and DNA sequence data. Invertebrate Biology 121(4), 271-324.
| Crossref | Google Scholar |
Glaubrecht M, Fehér Z, Von Rintelen T (2006) Brooding in Corbicula madagascariensis (Bivalvia, Corbiculidae) and the repeated evolution of viviparity in corbiculids. Zoologica Scripta 35, 641-654.
| Crossref | Google Scholar |
Gmelin JF (1791) ‘Caroli a Linné. Systema naturae per regna tria naturae: secundum classes, ordines, genera, species, cum characteribus, differentiis, synonymis, locis.’ [‘Charles Linnaeus. The system of nature through the three kingdoms of nature: according to classes, orders, genera, species, with characters, differences, synonyms, places.’] (Lipsiae, impensis Georg. Emanuel) [In Latin]
Gomes C, Sousa R, Mendes T, Borges R, Vilares P, Vasconcelos V, Guilhermino L, Antunes A (2016) Low genetic diversity and high invasion success of Corbicula fluminea (Bivalvia, Corbiculidae) (Müller, 1774) in Portugal. PLoS One 11, e0158108.
| Crossref | Google Scholar | PubMed |
González VL, Andrade SCS, Bieler R, Collins TM, Dunn CW, Mikkelsen PM, Taylor JD, Giribet G (2015) A phylogenetic backbone for Bivalvia: an RNA-seq approach. Proceedings of the Royal Society of London – B. Biological Sciences 282(1801), 20142332.
| Crossref | Google Scholar | PubMed |
Graf DL, Cummings KS (2021) A ‘big data’ approach to global freshwater mussel diversity (Bivalvia: Unionoida), with an updated checklist of genera and species. Journal of Molluscan Studies 87(1), eyaa034.
| Crossref | Google Scholar |
Gray JE (1853) V.—A revision of the genera of some of the families of Conchifera or bivalve shells. Annals and Magazine of Natural History 11(2), 33-44.
| Crossref | Google Scholar |
Hinds RB (1842) XIII.—Descriptions of new shells. Annals and Magazine of Natural History 10(63), 81-84.
| Crossref | Google Scholar |
Huang XC, Rong J, Liu Y, Zhang MH, Wan Y, Ouyang S, Zhou CH, Wu XP (2013) The complete maternally and paternally inherited mitochondrial genomes of the endangered freshwater mussel Solenaia carinatus (Bivalvia: Unionidae) and implications for Unionidae taxonomy. PLoS One 8(12), e84352.
| Crossref | Google Scholar |
Jones G (2017) Algorithmic improvements to species delimitation and phylogeny estimation under the multispecies coalescent. Journal of Mathematical Biology 74, 447-467.
| Crossref | Google Scholar | PubMed |
Kalyaanamoorthy S, Minh BQ, Wong TKF, von Haeseler A, Jermiin LS (2017) ModelFinder: fast model selection for accurate phylogenetic estimates. Nature Methods 14, 587-589.
| Crossref | Google Scholar | PubMed |
Kapli P, Lutteropp S, Zhang J, Kobert K, Pavlidis P, Stamatakis A, Flouri T (2017) Multi-rate Poisson tree processes for single-locus species delimitation under maximum likelihood and Markov chain Monte Carlo. Bioinformatics 33(11), 1630-1638.
| Crossref | Google Scholar | PubMed |
Katoh K, Standley DM (2013) MAFFT multiple sequence alignment software version 7: improvements in performance and usability. Molecular Biology and Evolution 30(4), 772-780.
| Crossref | Google Scholar | PubMed |
Kocot KM, Cannon JT, Todt C, Citarella MR, Kohn AB, Meyer A, Santos SR, Schander C, Moroz LL, Lieb B, Halanych KM (2011) Phylogenomics reveals deep molluscan relationships. Nature 477(7365), 452-456.
| Crossref | Google Scholar | PubMed |
Kondakov AV, Konopleva ES, Adesanya AJ, Bespalaya YV, Braun JJ, Gofarov MY, Lyubas AA, Tomilova AA, Vikhrev IV, Pokrovsky OS, Bolotov IN (2020) The global freshwater bivalve checklist’s extension: Freshwater occurrences and phylogenetic position of Galatea clams from West Africa (Venerida: Donacidae). Ecologica Montenegrina 35, 144-158.
| Crossref | Google Scholar |
Kumar S, Stecher G, Tamura K (2016) MEGA7: molecular evolutionary genetics analysis version 7.0 for bigger datasets. Molecular Biology and Evolution 33(7), 1870-1874.
| Crossref | Google Scholar | PubMed |
Kurabayashi A, Ueshima R (2000) Complete sequence of the mitochondrial DNA of the primitive opisthobranch gastropod Pupa strigosa: systematic implication of the genome organization. Molecular Biology and Evolution 17(2), 266-277.
| Crossref | Google Scholar | PubMed |
Lanfear R, Calcott B, Ho SY, Guindon S (2012) PartitionFinder: combined selection of partitioning schemes and substitution models for phylogenetic analyses. Molecular Biology and Evolution 29(6), 1695-1701.
| Crossref | Google Scholar | PubMed |
Lemer S, Bieler R, Giribet G (2019) Resolving the relationships of clams and cockles: dense transcriptome sampling drastically improves the bivalve tree of life. Proceedings of the Royal Society of London – B. Biological Sciences 286, 20182684.
| Crossref | Google Scholar | PubMed |
Letunic I, Bork P (2007) Interactive tree of life (iTOL): an online tool for phylogenetic tree display and annotation. Bioinformatics 23(1), 127-128.
| Crossref | Google Scholar | PubMed |
Liao X, Zhu X, Yang M (2020) Complete mitochondrial DNA genomes for Geloina erosa (Veneroida: Corbiculidae). Mitochondrial DNA – B 5(2), 1590-1591.
| Crossref | Google Scholar |
Lopes-Lima M, Fonseca MM, Aldridge DC, Bogan AE, Gan HM, Ghamizi M, Sousa R, Teixeira A, Varandas S, Zanatta D, Zieritz A, Froufe E (2017) The first Margaritiferidae male (M-type) mitogenome: mitochondrial gene order as a potential character for determining higher-order phylogeny within Unionida (Bivalvia). Journal of Molluscan Studies 83(2), 249-252.
| Crossref | Google Scholar |
Lv C, Li Q, Kong L (2018) Comparative analyses of the complete mitochondrial genomes of Dosinia clams and their phylogenetic position within Veneridae. PLoS One 13(5), e0196466.
| Crossref | Google Scholar |
Matsumoto M (2003) Phylogenetic analysis of the subclass Pteriomorphia (Bivalvia) from mtDNA COI sequences. Molecular Phylogenetics and Evolution 27, 429-440.
| Crossref | Google Scholar | PubMed |
Mikkelsen PM, Bieler R, Kappner I, Rawlings TA (2006) Phylogeny of Veneroidea (Mollusca: Bivalvia) based on morphology and molecules. Zoological Journal of the Linnean Society 148, 439-521.
| Crossref | Google Scholar |
Minh BQ, Nguyen MAT, von Haeseler A (2013) Ultrafast approximation for phylogenetic bootstrap. Molecular Biology and Evolution 30(5), 1188-1195.
| Crossref | Google Scholar | PubMed |
MolluscaBase (2022) Cyrenoidea J. E. Gray, 1840. In ‘MolluscaBase’. (World Register of Marine Species) Available at https://www.molluscabase.org/aphia.php?p=taxdetails&id=238370
MolluscaBase (2023a) Geloina coaxans (Gmelin, 1791). In ‘MolluscaBase’. (World Register of Marine Species) Available at https://www.molluscabase.org/aphia.php?p=taxdetails&id=991955
MolluscaBase (2023b) Glauconome J. E. Gray, 1828. In ‘MolluscaBase’. (World Register of Marine Species) Available at https://www.molluscabase.org/aphia.php?p=taxdetails&id=492515
Morton B (1976) The biology and functional morphology of the Southeast Asian mangrove bivalve, Polymesoda (Geloina) erosa (Solander, 1786) (Bivalvia: Corbiculidae). Canadian Journal of Zoology 54(4), 482-500.
| Crossref | Google Scholar |
Newell ND (1965) Classification of the Bivalvia. American Museum Novitates 2206, 1-25.
| Google Scholar |
Newell ND, Boyd DW (1978) A palaeontologist’s view of bivalve phylogeny. Philosophical Transactions of the Royal Society of London – B. Biological Sciences 284(1001), 203-214.
| Crossref | Google Scholar |
Nguyen LT, Schmidt HA, von Haeseler A, Minh BQ (2015) IQ-TREE: a fast and effective stochastic algorithm for estimating maximum-likelihood phylogenies. Molecular Biology and Evolution 32(1), 268-274.
| Crossref | Google Scholar | PubMed |
Okamoto A, Arimoto B (1986) Chromosomes of Corbicula japonica, Corbicula sandai and Corbicula leana (Bivalvia: Corbiculidae). Venus the Japanese Journal of Malacology 45(3), 194-202.
| Google Scholar |
Ota Y, Ohta Y (1970) A review of some Cretaceous corbiculids in North America. Transactions and Proceedings of the Palaeontological Society of Japan, New Series 79, 291-315.
| Google Scholar |
Park JK, Kim W (2003) Two Corbicula (Corbiculidae: Bivalvia) mitochondrial lineages are widely distributed in Asian freshwater environment. Molecular Phylogenetics and Evolution 29(3), 529-539.
| Crossref | Google Scholar | PubMed |
Park JK, Ó Foighil D (2000) Sphaeriid and corbiculid clams represent separate heterodont bivalve radiations into freshwater environments. Molecular Phylogenetics and Evolution 14(1), 75-88.
| Crossref | Google Scholar | PubMed |
Pereira D, Mansur MCD, Duarte LDS, de Oliveira AS, Pimpão DM, Callil CT, Ituarte C, Parada E, Peredo S, Darrigran G, Scarabino F, Clavijo C, Lara G, Miyahir IC, Rodriguez MTR, Lasso C (2014) Bivalve distribution in hydrographic regions in South America: historical overview and conservation. Hydrobiologia 735, 15-44.
| Crossref | Google Scholar |
Pigneur LM, Etoundi E, Aldridge DC, Marescaux J, Yasuda N, Van Doninck K (2014) Genetic uniformity and long-distance clonal dispersal in the invasive androgenetic Corbicula clams. Molecular Ecology 23, 5102-5116.
| Crossref | Google Scholar | PubMed |
Plazzi F, Ribani A, Passamonti M (2013) The complete mitochondrial genome of Solemya velum (Mollusca: Bivalvia) and its relationships with Conchifera. BMC Genomics 14, 409.
| Crossref | Google Scholar | PubMed |
Popham JD (1979) Comparative spermatozoon morphology and bivalve phylogeny. Malacological Review 12(1–2), 1-20.
| Google Scholar |
Puillandre N, Lambert A, Brouillet S, Achaz G (2012) ABGD, Automatic Barcode Gap Discovery for primary species delimitation. Molecular Ecology 21(8), 1864-1877.
| Crossref | Google Scholar | PubMed |
Rahuman S, Jeena NS, Asokan PK, Vidya R, Vijayagopal P (2020) Mitogenomic architecture of the multivalent endemicblack clam (Villorita cyprinoides) and its phylogenetic implications. Scientific Reports 10, 15438.
| Crossref | Google Scholar | PubMed |
Reeve LA (1843) Monograph of the genus Glauconome. In ‘Conchologia iconica, or, illustrations of the shells of molluscous animals. Vol. 2’. (L. Reeve & Co.: London, UK) 10.5962/bhl.title.8129
Ronquist F, Teslenko M, van der Mark P, Ayres DL, Darling A, Höhna S, Larget B, Liu L, Suchard MA, Huelsenbeck JP (2012) MrBayes 3.2: efficient Bayesian phylogenetic inference and model choice across a large model space. Systematic Biology 61(3), 539-42.
| Crossref | Google Scholar | PubMed |
Roy K, Hunt G, Jablonski D, Krug AZ, Valentine JW (2009) A macroevolutionary perspective on species range limits. Proceedings of the Royal Society of London – B. Biological Sciences 276, 1485-1493.
| Crossref | Google Scholar | PubMed |
Schneider JA (2001) Bivalve systematics during the 20th century. Journal of Paleontology 75(6), 1119-1127.
| Crossref | Google Scholar |
Sharma PP, Wheeler WC (2013) Revenant clades in historical biogeography: the geology of New Zealand predisposes endemic clades to root age shifts. Journal of Biogeography 40, 1609-1618.
| Crossref | Google Scholar |
Sharma PP, González VL, Kawauchi GY, Andrade SC, Guzmán A, Collins TM, Glover EA, Harper EM, Healy JM, Mikkelsen PM, Taylor JD, Bieler R, Giribet G (2012) Phylogenetic analysis of four nuclear protein-encoding genes largely corroborates the traditional classification of Bivalvia (Mollusca). Molecular Phylogenetics and Evolution 65(1), 64-74.
| Crossref | Google Scholar | PubMed |
Sharma PP, Zardus JD, Boyle EE, González VL, Jennings RM, McIntyre E, Wheeler WC, Etter RJ, Giribet G (2013) Into the deep: a phylogenetic approach to the bivalve subclass Protobranchia. Molecular Phylogenetics and Evolution 69(1), 188-204.
| Crossref | Google Scholar | PubMed |
Siripattrawan S, Park JK, Foighil DÓ (2000) Two lineages of the introduced Asian freshwater clam Corbicula occur in North America. Journal of Molluscan Studies 66, 423-429.
| Crossref | Google Scholar |
Smith SA, Wilson NG, Goetz FE, Feehery C, Andrade SCS, Rouse GW, Giribet G, Dunn CW (2011) Resolving the evolutionary relationships of molluscs with phylogenomic tools. Nature 480, 364-367.
| Crossref | Google Scholar | PubMed |
Smith CH, Johnson NA, Inoue K, Doyle RD, Randklev CR (2019) Integrative taxonomy reveals a new species of freshwater mussel, Potamilus streckersoni sp. nov. (Bivalvia: Unionidae): Implications for conservation and management. Systematics and Biodiversity 17(4), 331-348.
| Crossref | Google Scholar |
Stamatakis A (2014) RAxML version 8: a tool for phylogenetic analysis and post-analysis of large phylogenies. Bioinformatics 30(9), 1312-1313.
| Crossref | Google Scholar | PubMed |
Steiner G, Müller M (1996) What can 18S rDNA do for bivalve phylogeny? Journal of Molecular Evolution 43, 58-70.
| Crossref | Google Scholar | PubMed |
Taylor JD, Glover EA (2006) Lucinidae (Bivalvia) – the most diverse group of chemosymbiotic molluscs. Zoological Journal of the Linnean Society 148, 421-438.
| Crossref | Google Scholar |
Taylor JD, Kennedy WJ, Hall A (1969) The shell structure and mineralogy of the Bivalvia Introduction. Nuculacea—Trigonacea. Bulletin of the British Museum (Natural History) Zoology 3, 1-125.
| Google Scholar |
Taylor JD, Williams ST, Glover EA, Dyal P (2007) A molecular phylogeny of heterodont bivalves (Mollusca: Bivalvia: Heterodonta): new analyses of 18S and 28S rRNA genes. Zoologica Scripta 36, 587-606.
| Crossref | Google Scholar |
Taylor JD, Glover EA, Williams ST (2009) Phylogenetic position of the bivalve family Cyrenoididae—removal from (and further dismantling of) the superfamily Lucinoidea. The Nautilus 123(1), 9-13.
| Google Scholar |
Turgeon DD, Quinn JF, Bogan AE, Coan EV, Hochberg FG, Lyons WG, Mikkelsen PM, Neves RJ, Roper CFE, Rosenberg G, Roth B, Scheltema A, Thompson FG, Vecchione M, Williams JD (1998) ‘Common and Scientific Names of Aquatic Invertebrates from the United States and Canada: Mollusks.’ Special Publication 26. (American Fisheries Society) 10.47886/9781888569018
Valentas-Romera BL, Simone LRL, Mikkelsen PM, Bieler R (2019) Anatomical redescription of Cyrenoida floridana (Bivalvia, Cyrenoididae) from the Western Atlantic and its position in the Cyrenoidea. Zoosystematics and Evolution 95(2), 517-534.
| Google Scholar |
Vaughn CC, Hoellein TJ (2018) Bivalve impacts in freshwater and marine ecosystems. Annual Review of Ecology, Evolution, and Systematics 49(1), 183-208.
| Crossref | Google Scholar |
Walker JM, Bogan AE, Bonfiglio EA, Campbell DC, Christian AD, Curole JP, Harris JL, Wojtecki RJ, Hoeh WR (2007) Primers for amplifying the hypervariable, male-transmitted COII–COI junction region in amblemine freshwater mussels (Bivalvia: Unionoidea: Ambleminae). Molecular Ecology Notes 7(3), 489-491.
| Crossref | Google Scholar |
Williams ST, Taylor JD, Glover EA (2004) Molecular phylogeny of the Lucinoidea (Bivalvia): non-monophyly and separate acquisition of bacterial chemosymbiosis. Journal of Molluscan Studies 70(2), 187-202.
| Crossref | Google Scholar |
Wu RW, Liu YT, Wang S, Liu XJ, Zanatta DT, Roe KJ, Song XL, An CT, Wu XP (2018) Testing the utility of DNA barcodes and a preliminary phylogenetic framework for Chinese freshwater mussels (Bivalvia: Unionidae) from the middle and lower Yangtze River. PLoS One 13(8), e0200956.
| Crossref | Google Scholar |
Wu RW, Liu XJ, Wang S, Roe KJ, Ouyang S, Wu XP (2019) Analysis of mitochondrial genomes resolves the phylogenetic position of Chinese freshwater mussels (Bivalvia, Unionidae). ZooKeys 812(6), 23-46.
| Crossref | Google Scholar | PubMed |
Wu RW, An JM, Chen R, Ouyang S, Wu XP (2021) 基于多位点分子标记和线粒体基因组学中国蚌类(蚌目:蚌超科)物种分类与系统进化研究 [Taxonomy, phylogeny and evolution of freshwater mussels (Unionoida: Unionoidea) in China revealed by multilocus phylogenetic analyses and mitochondrial phylogenomics.]. 湖泊科学 [Journal of Lake Sciences] 33(6), 1788-1804 [In Chinese with English title and abstract].
| Crossref | Google Scholar |
Wu XP, Dai YT, Yin N, Shu FY, Chen ZG, Guo L, Zhou CH, Ouyang S, Huang XC (2022) Mitogenomic phylogeny resolves Cuneopsis (Bivalvia: Unionidae) as polyphyletic: the description of two new genera and a new species. Zoologica Scripta 51(2), 173-184.
| Crossref | Google Scholar |
Yamada M, Ishibashi R, Toyoda K, Kawamura K, Komaru A (2014) Phylogeography of the brackish water clam Corbicula japonica around the Japanese archipelago inferred from mitochondrial COII gene sequences. Zoological Science 31(3), 168-79.
| Crossref | Google Scholar | PubMed |
Yuan Y, Li Q, Yu H, Kong L (2012) The complete mitochondrial genomes of six heterodont bivalves (Tellinoidea and Solenoidea): variable gene arrangements and phylogenetic implications. PLoS One 7, e32353.
| Crossref | Google Scholar |
Zhang D, Gao FL, Jakovlić I, Zou H, Zhang J, Li WX, Wang GT (2020) PhyloSuite: an integrated and scalable desktop platform for streamlined molecular sequence data management and evolutionary phylogenetics studies. Molecular Ecology Resources 20, 348-355.
| Crossref | Google Scholar | PubMed |