Altitudinal variations and local emission contributions to black carbon and ion deposition on Tibetan Plateau glaciers
Yixi Liu
A
B
C
Abstract
Impurities deposited on glaciers can significantly accelerate their melting. This study investigated the spatial variability of concentrations of impurities across three glaciers on the Tibetan Plateau, revealing a pronounced concentration increase with decreasing altitude and the influence of local emissions. To accurately evaluate the potential for glacier melt, it is necessary to account for local emissions and altitude-dependent variations of impurities in individual glaciers.
Glaciers in the Tibetan Plateau (TP), especially in the Himalayas, are retreating rapidly due to rising air temperature and increasing anthropogenic emissions from nearby regions. Traditionally, pollutants deposited on the glaciers have been assumed to originate from long-range transport from its outside.
This study investigated the concentrations of black carbon (BC) and major ions in snowpit samples collected from two glaciers in the south-eastern TP (Demula and Palongzangbu) and one glacier in the west Himalayas (Jiemayangzong). The radiative forcing of BC was calculated based on BC concentration and glacier characteristics.
The results revealed that the BC/Ca2+ concentration ratio in snowpit samples from Palongzangbu, located near residential villages, is ~2.05 times higher than that of Demula, which is mainly influenced by long-range transported pollutants. Furthermore, on Jiemayangzong glacier, snowpit samples collected with 100-m vertical resolution exhibited that BC-induced radiative forcings at low altitude are ~2.37 ± 0.16 times greater than those at high altitude.
These findings demonstrated that in addition to long-range transport, emissions from local residents also make substantial contributions to BC and certain major ions (e.g. ). To accurately assess the sources and radiative forcing of BC and other light-absorbing impurities on glaciers of the TP, it is necessary to consider the impact of local populations and altitude-dependent variations.
Keywords: anthropogenic emissions, black carbon, dust, glacier, major ions, radiative forcing, sources, Tibetan Plateau.
Introduction
Glaciers, typically located in high-altitude remote regions, act as receptors for atmospheric pollutants that are transported over long distances. The extensive glacier regions spreading across the Himalayas and the Tibetan Plateau (TP) represent critical areas for such deposition (Yao et al. 2012; Liu S et al. 2015). Under the influence of global warming, the increased deposition of pollutants, such as black carbon (BC), has been considered a significant factor accelerating glacier retreat (Flanner et al. 2007; He et al. 2018; Kang et al. 2020; Shijin et al. 2021). BC can absorb solar radiation directly or indirectly, leading to a reduction in surface albedo and intensified glacier melting. Consequently, BC has attracted considerable attention in recent studies (Kopacz et al. 2011; Li C et al. 2016, 2018; Zhao et al. 2017). During summer, high temperatures cause rapid snowmelt on glaciers, making spring the optimal time to collect snowpit samples to investigate the concentration of BC and associated radiative forcing (Ming et al. 2009; Zhang Y et al. 2017; Yan et al. 2023). However, studies typically focus on snow collected at glacier cols, ignoring the spatial variations of BC concentrations on glacier surfaces. Evidence suggests that concentrations of BC and other pollutants decrease with increasing altitude of glaciers (Li Z et al. 2009; Li X et al. 2018). Therefore, neglecting this spatial variation may lead to an underestimation of glacier melting caused by BC, yet few studies have quantitatively assessed these variations within individual glaciers.
In addition to BC, major ions are also important pollutants in glacier snow and ice, providing insight into air quality and pollution sources. The study of major ions in precipitation contributes to the understanding of air quality by exploring the sources of air pollutants based on the different physical and chemical processes of these pollutants. Typically, previous studies have categorised the sources of stormwater chemistry (major ions) into three groups: sea salt, crustal sources, and anthropogenic emissions (Liu B et al. 2013; Cereceda-Balic et al. 2020). Historical variations of major ions, recorded in ice cores, can serve as indicators of past emission trends (Ming et al. 2008; Kaspari et al. 2011). For example, a rise of SO42− concentrations in an Everest ice core mirrored emissions from seriously polluted South Asia (Duan et al. 2007), reflecting the impact of long-range transported pollutants to the glacier of the Himalayas. Glaciers near inhabited areas may experience a more substantial contribution from local emissions (Li C et al. 2021a). For instance, in the south-eastern TP, where some glacier terminals are at low altitudes due to heavy snowfall, emissions from local activities have a greater potential to impact nearby glaciers. A previous study in the TP showed that combustion activities by local residents directly influence atmospheric pollutant levels in glacier regions (Hu et al. 2018). Direct evidence of this influence can be achieved by comparing the snowpit pollutants from glaciers located at varying distances from local resident centres.
To address these knowledge gaps, this study analysed the concentrations of BC and major ions in snowpit samples collected in 2021 from two glaciers (Demula (DM) and Palongzangbu (PL)) in the south-eastern TP (Fig. 1, Table 1). The aim was to evaluate the impact of local emissions on BC and major ion concentrations and to investigate the spatial variations of these pollutants within the same glaciers, extending the comparison to the western Himalayas (Jiemayangzong, JM) (Fig. 1, Table 1). The findings will emphasise the significance of local emissions in glacier retreat and provide a more accurate basis for estimating albedo reduction caused by BC and other light absorption particles in the glacier regions of the TP.
(a) Location of three glaciers investigated in this study on the TP. (b) Sampling sites at Jiemayangzong glacier. (c) Sampling sites at Palongzangbu and Demula glaciers in the south-eastern TP.
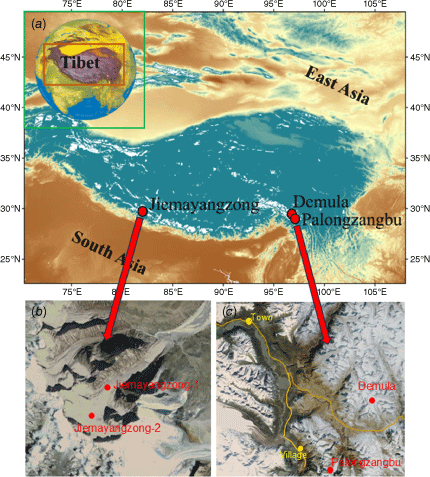
Glacier | Snowpit samples | Latitude (°N) | Longitude (°E) | Altitude (m ASL) | Depth (cm) | Snow density (kg m−3) | Collection date | |
---|---|---|---|---|---|---|---|---|
DM | 10 | 29.37 | 97.02 | 5295 | 96 | 300 | 6 April 2021 | |
PL | 13 | 29.25 | 96.93 | 4835 | 136 | 300 | 5 April 2021 | |
JM1 | 10 | 30.23 | 82.17 | 5440 | 104 | 380 | 29 April 2021 | |
JM2 | 10 | 30.21 | 82.16 | 5540 | 170 | 380 | 29 April 2021 |
ASL, above sea level.
Methods
Sampling sites and sample collection
Due to dry weather and limited snowfall in winter, snowpit samples were collected from three glaciers in different regions in spring 2021 (Table 1, Fig. 1). Snow in the snowpits had not melted because the temperature was very low at that time. Demula and Palongzangbu are ~36 km from each other (Fig. 1). Because of the low altitude of the glacier terminal of Palongzangbu, several small villages are distributed in the valley directly leading to this glacier. Palongzangbu glacier is also close to a town (Ranwu) and a highway (S201) (Fig. 1c). By contrast, Demula glacier is located in a remote area and far from the villages of local residents. These differences provide a precious opportunity to study the potential influence of emissions from local activities on glaciers. Jiemayangzong is a large glacier over 10 km long (Fig. 1b). To study the variations of BC and major ion concentrations at different altitudes of the Jiemayangzong glacier, two snowpits were dug, one at 5440 m ASL (JM1) and one at 5540 m ASL (JM2) (Table 1). Jiemayangzong glacier is the source of the Brahmaputra River and is located on the north slope of the western Himalayas, far from local Tibetan residents. As a result, pollutants deposited on this glacier are primarily long-range transported from sources such as the Indo-Gangetic Plain (Xu et al. 2013).
BC and ionic composition analysis
A total of 43 snowpit samples were collected using 100 mL of pre-cleaned polycarbonate bottles (Table 1) (Li C et al. 2017). Approximately 20 mL of thoroughly shock melted collected samples were filtered through 0.45-μm pore-size PTFE filters for major ion analysis (Zhang C et al. 2021). The remaining portion was used for measuring the BC concentration using a single particle soot photometer (SP2) (DMT, USA) after ultrasonic treatment for 10 min (Kaspari et al. 2014). Three blank samples were prepared and subjected to the same processes as those of the samples at each glacier. The average blank concentrations of Ca2+, Mg2+, Na+ and K+ were 1.25 ng L−1. The corresponding values of , Cl− and were all less than 1 ng L−1. BC concentrations of the blanks were near zero. All the reported data have been adjusted for blank values.
Calculation of radiative forcing from BC
Based on BC concentrations and glacier snowpack properties (snow depth, density, etc.) (Table 1), an updated Snow, Ice, and Aerosol Radiative (SNICAR) model was utilised for BC-induced albedo reductions (see https://github.com/mflanner/SNICARv3) (Gul et al. 2018; He et al. 2018, 2019). Eight simulation scenarios with a combination of two snowgrain shapes (spherical and non-spherical), two effective snowgrain radii, and two coating states of carbonaceous particles were considered during calculations. The radiative forcing of BC was subsequently calculated based on the computed albedo reductions and the measured surface incoming solar radiation from the National Oceanic and Atmospheric Administration and National Centers for Environmental Information.
Results and discussion
In the analysed snowpit samples, BC has the lowest concentration whereas Ca2+ exhibits the highest (Table 2). The concentrations of major ions and BC in snowpit samples at PL were higher than those at DM (Fig. 2b, 3), attributable to a combination of lower altitude and local emissions at PL. Typically, precipitation increases with altitude in mountainous regions, leading to lower concentrations of major ions and BC in snowpit samples from higher-altitude glaciers. However, the BC concentration at PL was ~3 times that at DM (Fig. 3), whereas the Ca2+ concentration at PL was only ~1.5 times that at DM. This disparity indicates a higher BC/Ca2+ ratio in the atmospheric aerosol over PL compared to DM (Fig. 4a). BC is mainly emitted from combustion activities (Bond et al. 2013; Yuan et al. 2020; Li X et al. 2022, 2023), whereas Ca2+ mainly comes from mineral dust (Rao et al. 2016; Adhikari et al. 2019). The elevated BC/Ca2+ ratio at PL also provides direct evidence of a greater influence from combustion-related emissions, particularly those associated with local residents. Similarly, , another key combustion byproduct (Duan et al. 2007), exhibited a higher /Ca2+ ratio at PL compared to DM (Fig. 4b). These findings further support the greater contribution of combustion emissions at PL. In addition to village emissions, other local sources likely contribute to these observations. For instance, the proximity of PL to Ranwu town and the S201 highway plays a significant role (Fig. 1c). The S201 highway is a major transportation route from south-eastern TP to other provinces, with heavy daily traffic. Furthermore, Ranwu town hosts over 30 coal-burning restaurants, which release particulate emissions readily transported to the PL glacier nearby. Seasonal population movement patterns further explain the variations observed. Many villagers in the area migrate seasonally, leaving their villages during spring and autumn and returning in winter for livelihood purposes (Zhang C et al. 2021). This seasonal mitigation results in peak emissions during winter, which are subsequently recorded in snowpit samples collected in early spring, reflecting conditions from winter to early spring. The lower altitude of the PL snowpit further amplifies its vulnerability to local emissions compared to the DM snowpit (Table 1). This enhanced deposition of BC and other light-absorbing particles at PL leads to a more pronounced reduction in snow albedo at the PL glacier, accelerating its melting process and contributing to the retreat. In summary, the additional BC deposition from local emissions at PL likely plays a significant role in the enhanced glacier melting observed at this site, underscoring the importance of considering both altitude and proximity to anthropogenic sources in assessing the impacts of pollutants on TP glaciers.
JM1 | JM2 | PL | DM | ||||||
---|---|---|---|---|---|---|---|---|---|
Mean | s.d. | Mean | s.d. | Mean | s.d. | Mean | s.d. | ||
BC | 17.46 | 12.70 | 14.23 | 19.17 | 22.14 | 12.72 | 7.13 | 5.81 | |
Cl− | 100.70 | 59.89 | 34.30 | 26.61 | 58.52 | 59.56 | 46.56 | 36.44 | |
143.50 | 84.24 | 99.00 | 99.84 | 211.73 | 163.59 | 93.60 | 62.62 | ||
197.65 | 99.47 | 153.92 | 127.42 | 150.52 | 50.20 | 106.16 | 52.00 | ||
Na+ | 147.82 | 118.59 | 30.62 | 32.30 | 105.80 | 113.49 | 85.83 | 61.43 | |
NH4+ | 799.41 | 521.08 | 655.32 | 607.70 | 487.21 | 279.84 | 404.98 | 346.57 | |
K+ | 95.28 | 59.70 | 48.58 | 60.57 | 59.67 | 43.50 | 39.79 | 25.45 | |
Mg2+ | 57.65 | 40.92 | 39.20 | 46.91 | 193.13 | 136.30 | 122.58 | 69.81 | |
Ca2+ | 1787.12 | 2089.03 | 732.43 | 791.77 | 1400.21 | 983.34 | 923.93 | 694.38 |
Comparison of BC and major ion concentrations in snowpits of (a) JM1 and JM2 and (b) PL and DM.
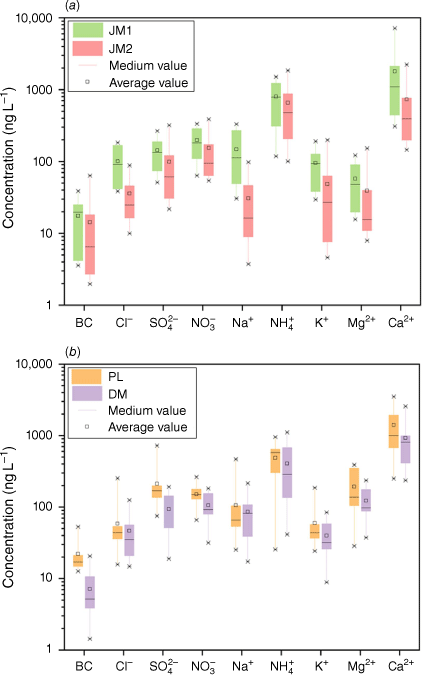
Ratios of (a) BC and Ca2+concentrations and (b) and Ca2+ concentrations in studied snowpit samples.
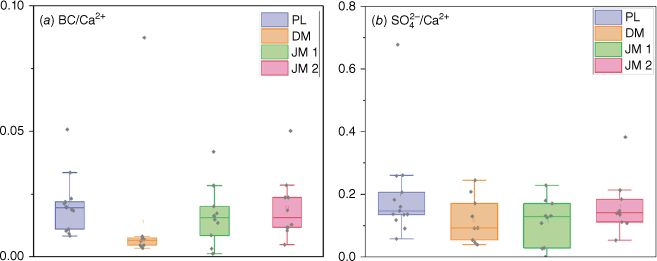
Due to its lower altitude, the JM1 snowpit exhibited higher concentrations for both major ions and BC compared to the JM2 snowpit (Fig. 2a, 3). However, the /Ca2+ and BC/Ca2+ ratios were nearly identical between the two snowpits (Fig. 4), indicating reasonably consistent aerosol composition across different altitudes within the same glacier. Interestingly, despite the JM glacier being located in a remote region without nearby human settlements, the BC/Ca2+ ratio in its snowpit samples was comparable to that of PL and higher than that of DM. This suggests an influence of cross-Himalayan transport of pollutants from the southern slope of the Himalayas, which are subjected to significant atmospheric pollution (Xu et al. 2013). Similar findings have been reported in studies on light-absorbing particles in glacier snowpits in the TP (Yan et al. 2023) and in the seasonal variations of BC concentration observed at a monitoring station on the northern slope of Everest (Chen et al. 2018). Previous research has shown that glaciers in the Himalayas are experiencing the most significant retreat in the TP region (Yao et al. 2012; Nie et al. 2021). One of the critical triggers is the increased deposition of light-absorbing particles transported from the Indo-Gangetic Plain to glaciers (Ming et al. 2008). Radiative forcing induced by BC has been calculated using BC concentrations from previous studies (Li C et al. 2021b). The calculated radiative forcing effects of BC were 3.77 ± 2.11 W m−2 for DM, 6.45 ± 3.84 W m−2 for PL, 8.84 ± 4.63 W m−2 for JM1, and 3.82 ± 2.23 W m−2 for JM2. The radiative forcing of BC at PL was ~1.68 ± 0.13 times that of DM, primarily attributed to its lower altitude and additional contributions from local emissions. Meanwhile, the radiative forcing at JM1 was 2.37 ± 0.16 times that of JM2, driven predominantly by altitude difference and reduced precipitation at JM1.
Conclusions
The hypothesis that pollutants are transported to TP glaciers via valley winds has long been proposed (Hindman and Upadhyay 2002). This study provides direct evidence supporting this assumption. Currently, a significant number of residents inhabit the valleys leading to the Himalayas, particularly along its southern slope. Although the population in these areas is considerably smaller than that of urban regions, the proximity of these valleys to TP glaciers makes emissions from local residents a crucial contributor to the deposition of particles on these glaciers, potentially surpassing the impact of long-range transported aerosols. Unlike urban areas where fossil fuels dominate energy use, valley residents predominantly rely on biomass fuels such as dung and wood. Biomass combustion is characterised by low efficiency and high emission factors (Szidat et al. 2006), leading to substantial atmospheric particle emissions during cooking and heating. For example, aerosols collected at the International Pyramid Laboratory-Observatory based in Nepal on the southern slope of the Himalayas primarily originate from Nepal rather than the Indo-Gangetic Plain (Decesari et al. 2010). This highlights the significant role of local emissions. Consequently, reducing particle emissions from residents living near glaciers is an important strategy for mitigating glacier retreat in the Himalayas and the TP.
Accurate evaluation of the radiative forcing exerted by light-absorbing particles on glaciers is essential for understanding the process driving glacier retreat and predicting future changes. This study reveals a pronounced increase in BC and major ion concentrations from higher to lower altitudes within the same glacier. Therefore, differences in the radiative forcing of BC between snowpits at the glacier col and terminal should be considered in future studies. To improve the accuracy of radiative forcing assessments for a glacier, it is crucial to analyse samples collected from multiple snowpits at different altitudes.
Data availability
All data included in this study are available on request by contacting the corresponding author.
Declaration of funding
This work was supported by the Second Tibetan Plateau Scientific Expedition and Research Program (STEP) (2019QZKK0605 to Chaoliu Li), the NSFC (41971096, 41977397 to Chaoliu Li), CAS ‘Light of West China’ Program (E0900104 to Chaoliu Li), and the State Key Laboratory of Cryospheric Science (SKLCS-ZZ-2024 to Chaoliu Li).
Acknowledgements
The authors thank the staff of the State Key Laboratory of Cryospheric Science for their help.
References
Adhikari S, Zhang F, Zeng C, Tripathee L, Adhikari NP, Xu J, Wang G (2019) Precipitation chemistry and stable isotopic characteristics at Wengguo in the northern slopes of the Himalayas. Journal of Atmospheric Chemistry 76(4), 289-313.
| Crossref | Google Scholar |
Bond TC, Doherty SJ, Fahey DW, Forster PM, Berntsen T, DeAngelo BJ, Flanner MG, Ghan S, Kaercher B, Koch D, Kinne S, Kondo Y, Quinn PK, Sarofim MC, Schultz MG, Schulz M, Venkataraman C, Zhang H, Zhang S, Bellouin N, Guttikunda SK, Hopke PK, Jacobson MZ, Kaiser JW, Klimont Z, Lohmann U, Schwarz JP, Shindell D, Storelvmo T, Warren SG, Zender CS (2013) Bounding the role of black carbon in the climate system: a scientific assessment. Journal of Geophysical Research-Atmospheres 118(11), 5380-5552.
| Crossref | Google Scholar |
Cereceda-Balic F, de la Gala-Morales M, Palomo-Marín R, Fadic X, Vidal V, Funes M, Rueda-Holgado F, Pinilla-Gil E (2020) Spatial distribution, sources, and risk assessment of major ions ad trace elements in rainwater at Puchuncaví Valley, Chile: the impact of industrial activities. Atmospheric Pollution Research 11(6), 99-109.
| Crossref | Google Scholar |
Chen X, Kang S, Cong Z, Yang J, Ma Y (2018) Concentration, temporal variation, and sources of black carbon in the Mt Everest region retrieved by real-time observation and simulation. Atmospheric Chemistry and Physics 18(17), 12859-12875.
| Crossref | Google Scholar |
Decesari S, Facchini MC, Carbone C, Giulianelli L, Rinaldi M, Finessi E, Fuzzi S, Marinoni A, Cristofanelli P, Duchi R (2010) Chemical composition of PM10 and PM1 at the high-altitude Himalayan station Nepal Climate Observatory-Pyramid (NCO-P)(5079 m ASL). Atmospheric Chemistry and Physics 10(10), 4583-4596.
| Crossref | Google Scholar |
Duan K, Thompson L, Yao T, Davis M, Mosley‐Thompson E (2007) A 1000 year history of atmospheric sulfate concentrations in southern Asia as recorded by a Himalayan ice core. Geophysical Research Letters 34(1), L01810.
| Crossref | Google Scholar |
Flanner MG, Zender CS, Randerson JT, Rasch PJ (2007) Present‐day climate forcing and response from black carbon in snow. Journal of Geophysical Research: Atmospheres 112(D11), D11202.
| Crossref | Google Scholar |
Gul C, Puppala SP, Kang S, Adhikary B, Zhang Y, Ali S, Li Y, Li X (2018) Concentrations and source regions of light-absorbing particles in snow/ice in northern Pakistan and their impact on snow albedo. Atmospheric Chemistry and Physics 18(7), 4981-5000.
| Crossref | Google Scholar |
He C, Flanner MG, Chen F, Barlage M, Liou K-N, Kang S, Ming J, Qian Y (2018) Black carbon-induced snow albedo reduction over the Tibetan Plateau: uncertainties from snow grain shape and aerosol–snow mixing state based on an updated SNICAR model. Atmospheric Chemistry and Physics 18, 11507-11527.
| Crossref | Google Scholar |
He C, Liou KN, Takano Y, Chen F, Barlage M (2019) Enhanced snow absorption and albedo reduction by dust‐snow internal mixing: modeling and parameterization. Journal of Advances in Modeling Earth Systems 11(11), 3755-3776.
| Crossref | Google Scholar |
Hindman EE, Upadhyay BP (2002) Air pollution transport in the Himalayas of Nepal and Tibet during the 1995–1996 dry season. Atmospheric Environment 36(4), 727-739.
| Crossref | Google Scholar |
Hu T, Cao J, Zhu C, Zhao Z, Liu S, Zhang D (2018) Morphologies and elemental compositions of local biomass burning particles at urban and glacier sites in southeastern Tibetan Plateau: results from an expedition in 2010. Science of The Total Environment 628-629, 772-781.
| Crossref | Google Scholar | PubMed |
Kang S, Zhang Y, Qian Y, Wang H (2020) A review of black carbon in snow and ice and its impact on the cryosphere. Earth-Science Reviews 210, 103346.
| Crossref | Google Scholar |
Kaspari SD, Schwikowski M, Gysel M, Flanner MG, Kang S, Hou S, Mayewski PA (2011) Recent increase in black carbon concentrations from a Mt Everest ice core spanning 1860–2000 AD. Geophysical Research Letters 38, L04703.
| Crossref | Google Scholar |
Kaspari S, Painter TH, Gysel M, Skiles S, Schwikowski M (2014) Seasonal and elevational variations of black carbon and dust in snow and ice in the Solu-Khumbu, Nepal and estimated radiative forcings. Atmospheric Chemistry and Physics 14(15), 8089-8103.
| Crossref | Google Scholar |
Kopacz M, Mauzerall DL, Wang J, Leibensperger EM, Henze DK, Singh K (2011) Origin and radiative forcing of black carbon transported to the Himalayas and Tibetan Plateau. Atmospheric Chemistry and Physics 11(6), 2837-2852.
| Crossref | Google Scholar |
Li C, Bosch C, Kang S, Andersson A, Chen P, Zhang Q, Cong Z, Chen B, Qin D, Gustafsson Ö (2016) Sources of black carbon to the Himalayan–Tibetan Plateau glaciers. Nature Communications 7, 12574.
| Crossref | Google Scholar | PubMed |
Li C, Yan F, Kang S, Chen P, Hu Z, Han X, Zhang G, Gao S, Qu B, Sillanpää M (2017) Deposition and light absorption characteristics of precipitation dissolved organic carbon (DOC) at three remote stations in the Himalayas and Tibetan Plateau, China. Science of The Total Environment 605-606, 1039-1046.
| Crossref | Google Scholar | PubMed |
Li C, Kang S, Yan F (2018) Importance of local black carbon emissions to the fate of glaciers of the Third Pole. Environmental Science & Technology 52(24), 14027-14028.
| Crossref | Google Scholar | PubMed |
Li C, Yan F, Kang S, Yan C, Hu Z, Chen P, Gao S, Zhang C, He C, Kaspari S, Stubbins A (2021a) Carbonaceous matter in the atmosphere and glaciers of the Himalayas and the Tibetan Plateau: an investigative review. Environment International 146, 106281.
| Crossref | Google Scholar |
Li C, Yan F, Zhang C, Kang S, Rai M, Zhang H, Hu S, He C (2021b) Coupling of decreased snow accumulation and increased light-absorbing particles accelerates glacier retreat in the Tibetan Plateau. Science of The Total Environment 809, 151095.
| Crossref | Google Scholar | PubMed |
Li X, Kang S, Zhang G, Qu B, Tripathee L, Paudyal R, Jing Z, Zhang Y, Yan F, Li G, Cui X, Xu R, Hu Z, Li C (2018) Light-absorbing impurities in a southern Tibetan Plateau glacier: variations and potential impact on snow albedo and radiative forcing. Atmospheric Research 200, 77-87.
| Crossref | Google Scholar |
Li X, Fu P, Tripathee L, Yan F, Hu Z, Yu F, Chen Q, Li J, Chen Q, Cao J, Kang S (2022) Molecular compositions, optical properties, and implications of dissolved brown carbon in snow/ice on the Tibetan Plateau glaciers. Environment International 164, 107276.
| Crossref | Google Scholar | PubMed |
Li X, Guo J, Yu F, Tripathee L, Yan F, Hu Z, Gao S, He X, Li C, Kang S (2023) Concentrations, sources, fluxes, and absorption properties of carbonaceous matter in a central Tibetan Plateau river basin. Environmental Research 216, 114680.
| Crossref | Google Scholar | PubMed |
Li Z, He Y, Pang H, Theakstone WH, Zhang N, He X, Wang S, Pu T, Du J (2009) Environmental significance of snowpit chemistry in the typical monsoonal temperate glacier region, Baishui glacier no. 1, Mt Yulong, China. Environmental Geology 58(6), 1319-1328.
| Crossref | Google Scholar |
Liu B, Kang S, Sun J, Zhang Y, Xu R, Wang Y, Liu Y, Cong Z (2013) Wet precipitation chemistry at a high-altitude site (3,326 m ASL) in the southeastern Tibetan Plateau. Environmental Science and Pollution Research 20(7), 5013-5027.
| Crossref | Google Scholar | PubMed |
Liu S, Yao X, Guo W, Xu J, Shangguan D, Wei J, Bao W, Wu L (2015) The contemporary glaciers in China based on the Second Chinese Glacier Inventory. Acta Geographica Sinica 70(1), 3-16.
| Crossref | Google Scholar |
Ming J, Cachier H, Xiao C, Qin D, Kang S, Hou S, Xu J (2008) Black carbon record based on a shallow Himalayan ice core and its climatic implications. Atmospheric Chemistry and Physics 8(5), 1343-1352.
| Crossref | Google Scholar |
Ming J, Xiao C, Cachier H, Qin D, Qin X, Li Z, Pu J (2009) Black carbon (BC) in the snow of glaciers in west China and its potential effects on albedos. Atmospheric Research 92(1), 114-123.
| Crossref | Google Scholar |
Nie Y, Pritchard HD, Liu Q, Hennig T, Wang W, Wang X, Liu S, Nepal S, Samyn D, Hewitt K (2021) Glacial change and hydrological implications in the Himalaya and Karakoram. Nature Reviews Earth & Environment 2(2), 91-106.
| Crossref | Google Scholar |
Rao PSP, Tiwari S, Matwale JL, Pervez S, Tunved P, Safai PD, Srivastava AK, Bisht DS, Singh S, Hopke PK (2016) Sources of chemical species in rainwater during monsoon and non-monsoonal periods over two mega cities in India and dominant source region of secondary aerosols. Atmospheric Environment 146, 90-99.
| Crossref | Google Scholar |
Shijin W, Yanjun C, Yanqiang W (2021) Spatiotemporal dynamic characteristics of typical temperate glaciers in China. Scientific Reports 11(1), 657.
| Crossref | Google Scholar | PubMed |
Szidat S, Jenk TM, Synal H-A, Kalberer M, Wacker L, Hajdas I, Kasper-Giebl A, Baltensperger U (2006) Contributions of fossil fuel, biomass-burning, and biogenic emissions to carbonaceous aerosols in Zurich as traced by 14C. Journal of Geophysical Research: Atmospheres (1984-2012) 111(D7), D07206.
| Crossref | Google Scholar |
Xu J, Zhang Q, Li X, Ge X, Xiao C, Ren J, Qin D (2013) Dissolved organic matter and inorganic ions in a central Himalayan glacier – insights into chemical composition and atmospheric sources. Environmental Science & Technology 47(12), 6181-6188.
| Crossref | Google Scholar | PubMed |
Yan F, Li C, Kang S, Hu Z, Zhang C, Yang C, Chen P, Yang J, Xu Y, Li Y, Gao S, He C (2023) Dust dominates glacier darkening across majority of the Tibetan Plateau based on new measurements. Science of The Total Environment 891, 164661.
| Crossref | Google Scholar | PubMed |
Yao T, Thompson L, Yang W, Yu W, Gao Y, Guo X, Yang X, Duan K, Zhao H, Xu B, Pu J, Lu A, Xiang Y, Kattel DB, Joswiak D (2012) Different glacier status with atmospheric circulations in Tibetan Plateau and surroundings. Nature Climate Change 2(9), 663-667.
| Crossref | Google Scholar |
Yuan Q, Wan X, Cong Z, Li M, Liu L, Shu S, Liu R, Xu L, Zhang J, Ding X, Li W (2020) In situ observations of light-absorbing carbonaceous aerosols at himalaya: analysis of the South Asian sources and trans-Himalayan valleys transport pathways. Journal of Geophysical Research: Atmospheres 125(18), e2020JD032615.
| Crossref | Google Scholar |
Zhang C, Chen M, Kang S, Yan F, Han X, Gautam S, Hu Z, Zheng H, Chen P, Gao S, Wang P, Li C (2021) Light absorption and fluorescence characteristics of water-soluble organic compounds in carbonaceous particles at a typical remote site in the southeastern Himalayas and Tibetan Plateau. Environmental Pollution 272, 116000.
| Crossref | Google Scholar | PubMed |
Zhang Y, Kang S, Cong Z, Schmale J, Sprenger M, Li C, Yang W, Gao T, Sillanpää M, Li X (2017) Light‐absorbing impurities enhance glacier albedo reduction in the southeastern Tibetan Plateau. Journal of Geophysical Research: Atmospheres 122(13), 6915-6933.
| Crossref | Google Scholar |
Zhao Z, Wang Q, Xu B, Shen Z, Huang R, Zhu C, Su X, Zhao S, Long X, Liu S, Cao J (2017) Black carbon aerosol and its radiative impact at a high-altitude remote site on the southeastern Tibet Plateau. Journal of Geophysical Research: Atmospheres 122(10), 5515-5530.
| Crossref | Google Scholar |