Daphnia reproductive impacts following chronic exposure to micro- and nano-scale particles from three types of rubber
Brittany E. Cunningham
A
B
C
Abstract
Tyre rubber particles, from both driving and reuse of tyre rubber, are pollutants that carry toxic chemicals into the environment. We investigated the long-term effects that these particles have on small aquatic organisms and found that they drastically reduce their ability to reproduce. Continued exposure of aquatic invertebrates to tyre-related pollutants, has the potential to affect the population by inhibiting reproduction into future generations.
High levels of rubber microplastics in aquatic environments are often attributed to particles from driven tyres; however, the use of recycled or crumb rubber particles in outdoor surfaces is another source. Chronic toxicity assessments with tyres are limited, and there is a need to evaluate effects of rubber particles from different sources to better understand their role in conferring toxicity.
We investigated the effect of chronic exposure of Daphnia magna to micro-sized (1–20 µm, 3.13 × 104–1.25 × 105 particles mL–1) and nano-sized (<1 µm, 1.25 × 105–1.00 × 107 particles mL–1) synthetic rubber particles. These included tyre particles (TPs) and two types of rubber from recycled tyres (called recycled rubber, RR, and crumb rubber, CR). Mortality, reproduction and moulting were assessed daily, and growth was measured at the end of the exposure. Additionally, the F1 generation was reared to assess multigenerational effects.
Chronic exposure to micro-rubber particles had severe effects, delaying, decreasing and even eliminating reproduction starting at 6.25 × 105 particles mL–1. Chronic exposure to nano-rubber particles had less severe effects, but delayed and decreased reproduction at the highest exposure level, 5.00 × 107 particles mL–1. Exposure to nano-rubber in the parental generation affected reproduction in the F1 generation.
This is the first comparison of chronic and generational toxicity between different sizes and compositions of rubber particles. The reproductive affects of chronic exposure to rubber particles could have devastating impacts on populations of Daphnia. Research is needed to identify which components leached from rubber particles affect reproductive ability.
Keywords: aquatic toxicity, chronic toxicity, Daphnia, microplastics, nanoplastics, reproduction, rubber, tyres.
Introduction
Black, rubber particles have been documented in the environment in both sediment and aquatic samples for many years (Wik and Dave 2009; Wagner et al. 2018). These microplastics are often classified as tyre particles (TPs). Tyre-related debris is one of the largest sources of terrestrial microplastics in marine environments (Boucher and Friot 2017). This may be especially problematic in the United States (US) which has the highest ratio of automobiles to inhabitants (Luo et al. 2021). Kole et al. (2017) estimated that in the US ~1,524,740 tonnes (Mg) of tyre-related particles flow into the environment each year. A more recent estimate by Luo et al. (2021), calculated that in 2019 the US produced 5.37 × 105 Mg of TPs. Little data exist on a global scale on the quantity of TPs in the environment. Estimates for micro-sized TPs range from 0.03 to 59 mg L–1 in surface waters (Wik and Dave 2009) and up to 12 mg g–1 dry weight in river sediments (Baensch-Baltruschat et al. 2020). Additionally, methods for measuring nanoplastics in environmental samples are still being developed, and currently no estimates of environmental nano-tyre or nano-rubber particles exist. TP pollution will likely continue to rise as tyre use is only expected to increase (Luo et al. 2021), and there are no current alternatives offered to primarily synthetic rubber automobile tyres. However, the creation of TPs through the friction of tyres on roadways is not the only source of anthropogenic rubber particles in the environment. Recycled rubber generated from the recycling of tyres, and crushed tyre commonly used in artificial turf, also contribute to rubber microplastics (Verschoor et al. 2021). Approximately 2 Mg year−1 of recycled rubber particles are estimated to be shed from a standard football field of artificial turf (Wang et al. 2019). Tyre-related rubber from all of these sources can enter the environment through wastewater (Sugiura et al. 2021), road and stormwater runoff (Ziajahromi et al. 2020; Werbowski et al. 2021), and atmospheric deposition (Kukutschová et al. 2011).
When it comes to synthetic rubber microplastics, TPs are the most commonly discussed and researched (Kole et al. 2017; Wagner et al. 2018; Luo et al. 2021; Cheong et al. 2023). The identity of black rubber microplastics found in environmental samples cannot always be verified through mechanisms such as spectroscopy (Sutton et al. 2019); however, they are often classified visually, based on colour and morphology, as TPs (Werbowski et al. 2021). TPs are composed of a variety of materials including natural and synthetic rubber, filling agents, oils, vulcanisation agents (e.g. sulfur), antiozonants (e.g. 6PPD) and other additives (Eisentraut et al. 2018). Tyre composition is proprietary and varies between and within tyre types and brands (Wik and Dave 2005, 2006). Over 350 chemical compounds have been potentially associated with recycled tyre crumb rubber (Thomas et al. 2019); chemicals documented in the leachate of tyres include heavy metals, polycyclic aromatic hydrocarbons (PAHs), benzothiazoles and aryl-amines (Capolupo et al. 2020; Chibwe et al. 2022). External factors such as the period of leaching (Chibwe et al. 2022), size of the particles (Stack et al. 2023), exposure to UV light (Wik and Dave 2006; Lu et al. 2021) and other weathering (Weyrauch et al. 2023) have been shown to alter the composition and rate of chemical leaching from tyre rubber. Aquatic organisms not only come into contact with the chemicals that TPs leach, but they also interact with the particles as well. Many aquatic organisms have been shown to ingest TPs; the most common of which are crustaceans (e.g. Gammarus pulex, Redondo-Hasselerharm et al. 2018; Hyalella, Khan et al. 2019; Mysid sp., Halle et al. 2020; Siddiqui et al. 2022; and Daphnia magna, Cunningham et al. 2022) and fish (e.g. Menidia beryllina, Siddiqui et al. 2022; Fundulus heteroclitus, LaPlaca and van den Hurk 2020; and Pimephales promelas, LaPlaca and van den Hurk 2020).
Another source of black rubber particles in the environment come from the recycling of tyre rubber into rubber mulch products. Recycled rubber – also called recycled crumb rubber or just crumb rubber – is commonly used as playground mulch, flooring and synthetic turf (Llompart et al. 2013; Benson et al. 2019). Although the conversion of recycled tyres into flooring is often thought of as an environmentally sustainable practice for managing tyre waste (Fiksel et al. 2011), little attention has been paid to its contribution to the tyre microplastic pollution problem. These recycled rubber particles can also find their way into the environment through wastewater and stormwater runoff (Verschoor et al. 2021) and are widely documented as a health concern for children who use playgrounds and turf fields frequently (Murphy and Warner 2022). Overall, little modelling has been done on the flow of rubber from non-driving sources into the environment; however, Sieber et al. (2020) modelled the flow of rubber particles into the environment in Switzerland and estimated that 3% of the pollution came from recycled rubber granules. The integrity of the rubber in these recycled products, as well as the chemicals in the leachate, may differ from that of traditional TPs because of the aging and processing that has occurred. For example, studies show that chemicals leached from rubber changes both over time and with the size of the rubber particles (Rhodes et al. 2012; Selbes et al. 2015). Yet, similar to TP leachate, leachate from crumb rubber has also been shown to contain zinc and PAHs (Celeiro et al. 2021; Lu et al. 2021).
Rubber toxicity studies have primarily focused on the toxicity of the leachate from tyre, tyre wear and tyre debris particles (Luo et al. 2021). We, as well as others, have found both TPs themselves and their leachate elicit toxic responses in aquatic organisms (Wik and Dave 2006; LaPlaca and van den Hurk 2020; Cunningham et al. 2022; Siddiqui et al. 2022). Additionally, 6-PPD-quinone in tyre leachate has been shown to have acute lethal effects on historically and commercially important salmonids (Tian et al. 2022). Overall, both the tyre leachate and particle toxicity assessments in the literature have occurred over acute time periods (Wagner et al. 2018; Luo et al. 2021; Cheong et al. 2023). TPs and their leachates have been shown to be acutely toxic to many freshwater organisms including green algae (Wik et al. 2009; Turner and Rice 2010; Marwood et al. 2011), Daphnia (Wik et al. 2009; Marwood et al. 2011), zebrafish (Wik et al. 2009; Varshney et al. 2022), salmon (Tian et al. 2022) and fathead minnows (Chibwe et al. 2022). Far fewer studies have investigated the toxicity of recycled rubbers, especially for aquatic organisms. Halsband et al. (2020) investigated crumb rubber leached in seawater and found that acute exposure to the leachate caused mortality in marine copepods. Additionally, Birkholz et al. (2003) leached crumb rubber in freshwater and found the leachate, with particles removed, to be toxic to bacteria (Vibrio fisheri), algae (Selenastrum capricornutum), invertebrates (Daphnia manga) and fish (P. promelas). For the particles themselves, acute toxicity of recycled crumb rubber in soil had minimal effects on earthworms and soil microbes (Pochron et al. 2017). Additionally, in conjunction with the US Federal Research Action Plan on recycled tyre crumb used on playing fields and playgrounds, in vivo studies have been done with human cell lines and mice to assess the risk of recycled tyre crumb rubber in synthetic turf (National Toxicology Program 2019). They found that, although crumb rubber was cytotoxic to human lung, skin and small intestine cells, there were minimal effects in female mice.
Due to the acute toxicity of micro and nano-TPs, it is necessary to know how that toxicity may manifest over chronic exposures. Some chronic toxicity assessments of rubber particles and leachate have been done for freshwater organisms. Panko et al. (2013) chronically exposed aquatic organisms to TPs in sediment or tyre leachate and found that Chironomus dilutus and P. promelas were affected, whereas H. azteca and Ceriodaphnia dubia were not. Chronic exposure of D. magna to tyre leachate was also found to cause mortality (Gualtieri et al. 2005). A chronic, 21-day exposure of H. azteca to TPs affected mortality, reproduction and growth (Khan et al. 2019). By contrast, a 28-day exposure of freshwater crustaceans (G. pulex and Asellus aquaticus) and worms (Lumbriculus variegatus and Tubifex spp.) to TPs did not have any effects (Redondo-Hasselerharm et al. 2018). Additionally, a chronic exposure with crumb rubber particles conducted in the mummichog, Fundulus heteroclitus, did not induce a major physiological response or mortality (LaPlaca et al. 2022). The large variation of effects in the above-summarised studies is likely due to a combination of differing rubber sources, particle size and organism sensitivity. As organisms are exposed to micro and nano-sized rubber particles in the environment over long periods of time, it is important to conduct experiments that more closely mirror these environmental exposure durations.
There remains a gap in the literature concerning the chronic exposure of aquatic organisms to micro and nano-sized rubber particles and how this toxicity may (1) differ between new and recycled tyres and (2) affect subsequent generations. Thus, we designed experiments to assess the chronic toxicity of three types of rubber to Daphnia growth and reproduction. We intentionally selected sublethal concentrations of exposure based on our earlier acute study as well as preliminary chronic exposures. Our goals were to assess the chronic effects of the particles on the exposed parental Daphnia generation as well as on their unexposed offspring (F1). The study investigated if exposure of the parental generation, and during embryo development, was sufficient to result in effects to an F1 generation adult that was not continuously exposed to the rubber.
Materials and methods
Test organism
Daphnia magna were taken from continuous cultures maintained at 21°C with a 14-h light:10-h DARK cycle under full spectrum growth lights. D. magna were reared in media (referred to as Daphnia water from this point) containing 192 mg L–1 of NaHCO3, 120 mg L–1 of CaSO4∙2H2O, 120 mg L–1 of MgSO4, 8 mg L–1 of KCl and 0.002 mg L–1 of Na2SeO3. This reconstituted hard water media was verified to have a pH between 7.8 and 8.4 and a conductivity between 580 and 620 µS cm–1. D. magna were fed rehydrated freeze-dried spirulina daily, and 3 times per week the algal food was supplemented with fermented Daphnia chow (Carolina Biologic Supply) and dried yeast suspensions. All cultures were maintained in 2-L glass beakers and offspring were removed each day. For the chronic exposures, neonates <24-h old were selected and transferred to a separate glass culture container and allowed to mature for 1 week prior to experimentation. Temporal variation in neonate age has been shown to have a large effect on toxicity, with younger neonates having far greater sensitivity to environmental pollutants (Traudt et al. 2017; Schmid et al. 2023). This is also known to be true for plastic particle exposure, as Liu et al. (2018) showed that Daphnia neonates exposed to nanoplastic were more sensitive, and had greater rates of mortality than adults. As the aim of this study was to assess sublethal exposure, the use of 1-week old Daphnia in a 28-day test, a modification of Organisation for Economic Co-operation and Development (2012) guidelines, allows for an increased number of broods to be monitored while decreasing the background mortality.
Rubber particle preparation and characterisation
To generate the TPs, a new, undriven standard passenger car tyre (all season tyre with DOT quality grades of Treadwear 480, Traction A and Temperature A) was used. This specific tyre was picked to be as representative as possible; however, all tyres differ from each other in composition (Wik and Dave 2006), the details of which are proprietary. Slices were taken from just the tread of the tyres with a stainless-steel blade. Slices were cut into 2–4-mm pieces. Although the terms crumb rubber and recycled rubber are often used synonymously and both refer to rubber material generated from recycled tyres, for the purposes of this paper, to distinguish between the two types of recycled rubber materials used, they will be referred to as recycled rubber (RR) and crumb rubber (CR) based on their product labels. The RR was acquired from Recycled Rubber Products, LLC (Joliet, IL, USA), and was received in shredded form. The CR came from the Hawaii Pacific University Center for Maine Debris Research’s Polymer Kit 1.0 (Hawaii, USA; kit rubber sourced from reRubber, LLC). The CR arrived broken down into crumbed particles, in pieces >4 mm in size.
All rubber pieces were further reduced in size using liquid nitrogen-cooled cryomilling (Retsch CryoMill, Haan, Germany). After milling, 3.25 g of milled rubber particles were suspended in 300 mL of 0.1-µm filtered Daphnia water (Pall Supor PES filters) containing 50 mg L–1 of humic acid (HA). All rubber solutions and dilutions throughout this study were created in this filtered water to minimise the chance of introducing contaminant micro or nanoplastics to the exposures. A separate suspension of particles in water was made for each of the three rubbers. HA was used throughout the experiments to minimise aggregation and keep the particles in suspension (Grillo et al. 2015). Glass beads were added to the rubber suspensions and they were autoclaved, cooled, and then rotated on a shaker table to further decrease the size of the particles. After 72 h, the glass beads were removed. The suspension was passed through a 20-µm sieve and then a 1-µm filter (Advantec mixed cellulose filter) to obtain a suspension of particles less than 1 μm. The 1-µm filters were then backflushed with fresh 0.1-µm filtered Daphnia water containing 50 mg L–1 of HA to obtain a solution of micro-sized particles between 1 and 20 μm. Particle counts were taken for the micro-sized fraction using a flow cytometer (Accuri C6 Flow Cytometer, BD Biosciences, San Jose, CA, USA) calibrated with size standards, whereas the nano-fraction was analysed for particle concentration using Nanoparticle Tracking Analysis (NTA, ver. 3.4, Malvern Panalytical Inc., Westborough, MA, USA) on a NanoSight NS500 instrument (Malvern Instruments, Westborough, MA) equipped with a 405-nm laser (Cunningham et al. 2022). Rubber particle size distributions were measured to verify that particles fell within the desired micro and nano-size ranges, and were in line with distributions described by Cunningham et al. (2022). Solutions were kept covered and refrigerated until use, at which time settled particles were resuspended through vigorous stirring with a glass rod. New micro and nano-rubber suspensions were made every 2 weeks throughout the experimental period and refrigerated until use to avoid any interference from bacterial growth on the particles.
Experimental design
Static renewal, 28-day chronic toxicity tests were conducted using an adapted version of Test 211 of the Organisation for Economic Co-operation and Development (2012) guidelines. Concentrations for the chronic exposures were selected based on acute exposures of Daphnia to TPs (Cunningham et al. 2022), RR and CR (data not shown). The concentrations selected were all below the half maximal effective concentration (EC50) values determined for acute exposures. Additionally, preliminary chronic exposures with TPs were done to ensure sublethal levels of rubber exposure (Supplementary Fig. S1). As micro- and nano-sized rubber particles have been shown to cause mortality at different particle-count based concentrations (Cunningham et al. 2022), the high, medium and low concentrations used for the exposures differed between the micro and nano-sized exposures. The high micro-size concentration and low nano-size concentration (particles mL–1) were the same to have an overlapping exposure level between the micro and nano-particles. For all three rubber types, the micro-sized exposure concentrations were 3.13 × 104, 6.25 × 104 and 1.25 × 105 particles mL–1 (~1.97, 3.94 and 7.87 mg L–1), and the nano-sized exposure concentrations were 1.25 × 105, 5.00 × 106 and 5.00 × 107 particles mL–1. All exposures, including the Daphnia water controls (0 particles mL–1), contained 50 mg L–1 of HA. All Daphnia water and HA used throughout the chronic assay were filtered to 0.1 μm to remove additional particles. It would have been desirable to include a control exposure with only rubber leachate and no particles; however, removal of the nanoscale rubber particles from the leachate (described in Cunningham et al. 2022) is a time and material intensive process and it was not possible to generate the volume of particle-free leachate necessary to do this.
As described above, the test animals were 1-week old Daphnia. Three replicates were conducted with 5 Daphnia per 50-mL beaker containing 30 mL of test media. Test media was renewed twice a week. Solution changes were limited to twice a week to reduce stress on the organisms and to conserve materials. The D. magna were fed 20 μL of spirulina solution per Daphnia every 24-h prepared by sonicating 0.05 g of dried spirulina in 10 mL of Daphnia water. Every other day, algae feedings were supplemented by adding 0.80 mL of yeast and fermented Daphnia chow suspension containing 1.9 g L–1 of total dissolved solids. The beakers were kept under a full spectrum grow light set to a 14-h light:10-h dark cycle. Mortality, immobility, moulting and reproduction (i.e. number of neonates produced) were assessed daily using an Olympus SZ61 series optical microscope. All moults and neonates, as well as any dead D. magna, were removed from the exposure beakers daily. Neonates from the third brood were kept and reared in particle-free Daphnia water for F1 generation evaluations. The beakers of the initial, exposed generation were terminated after 28 days. At 1-week old, Daphnia from the F1 generation were placed into 50-mL beakers. They were reared in identical conditions to the parental generation, five Daphnia per beaker in 30 mL of liquid; however, they were raised in clean Daphnia water with no added particles. The F1 generation was monitored daily for the same endpoints as the exposed, parental generation, and was kept on the same water change and feeding schedule. At the end of 28 days from the time they were placed in the beakers, the F1 experiment was terminated. All D. magna were preserved in 2% paraformaldehyde and imaged individually using an Olympus SC100 series optical video microscope. For growth analysis, Daphnia length measurements were taken in a single straight line from the centre of the eye to the tip of the posterior carapace, as described in Duckworth et al. (2019). Images were taken of each individual; these were then measured using Olympus cellSens Entry (ver. 1.18, Evident Corporation, Tokyo, Japan) imaging and analysis software.
Data analysis
All statistical analyses were performed using RStudio (ver. 1.0.153, Posit Software, PBC, Boston, MA, USA, see https://posit.co/products/open-source/rstudio/). Reproduction and moulting were assessed in terms of the number of neonates, or moults, produced per D. magna alive in the beaker per day, to account for mortality in the parent generation. Mortality was assessed as the percent survival out of five initial Daphnia. Levene’s test and Shapiro–Wilk’s test were used to assess homogeneity of variance and normality of distributions, respectively. The moulting and growth data met these assumptions. For these data, one-way analysis of variance (ANOVA) was used to evaluate differences across exposure concentrations of each rubber particle type, with a Dunnett test used to evaluate differences between exposure concentrations and the control. Additionally, two-way ANOVA was used to evaluate differences between exposure concentrations and rubber types, with Tukey HSD post hoc tests used to identify significant differences among treatment groups. Data normality and homogeneity of variance were not met for neonate production and reproduction timing data; therefore, the non-parametric Kruskall–Wallis and Dunn tests were used to compare means of these data to assess differences between concentrations of rubber and their respective controls, as well as among different rubber types. All tests were considered statistically significant at P ≤ 0.05. All response curves were constructed and EC50 values were calculated using the package DRC (analysis of dose–response curves) (ver. 3.0.1, see https://cran.r-project.org/package=drc; Ritz et al. 2015). Fig. 1–4 below display the results of the experiment normalised to each rubber type’s respective control, so that comparisons can be made between rubber types. The non-normalised figures with control bars can be found in the Supplementary material.
The number of neonates per Daphnia per day normalised as a percentage of the control values over the 28-day chronic micro and nano-sized rubber exposure. Significance is designated by an asterisk (*) for a difference with the control Daphnia (Kruskal–Wallis with Dunn test, P ≤ 0.05), and by a hash (#) for a difference between two rubber types at the same concentration (Kruskal–Wallis with Dunn test, P ≤ 0.05). CR, crumb rubber; RR, recycled rubber; TP, tyre particles. Non-normalised figures with control bars are shown in the Supplementary material (Supplementary Fig. S7).
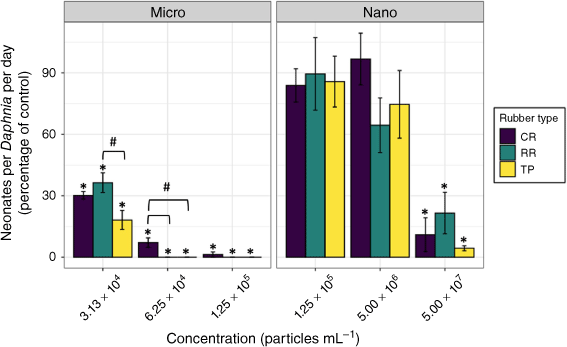
The length of time in days that it took for Daphnia to first produce neonates, normalised as a percentage of the control values. Significance is designated by an asterisk (*) for a difference with the control Daphnia (Kruskal–Wallis with Dunn test, P ≤ 0.05), and by a hash (#) for a difference between two rubber types at the same concentration (Kruskal–Wallis with Dunn test, P ≤ 0.05). CR, crumb rubber; RR, recycled rubber; TP, tyre particles. Non-normalised figures with control bars are shown in the Supplementary material (Supplementary Fig. S8). The caret symbol (^) shows where there are no bars for the higher concentrations of the micro RR and TP because they never reproduced, and there was no value for time to first reproduction.
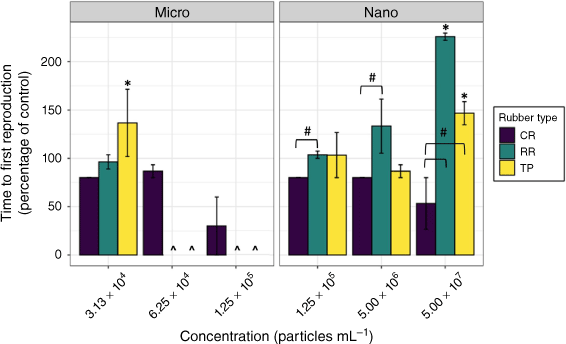
The final length of the surviving Daphnia on day 28 of the exposure to chronic micro and nano-sized rubber, normalised as a percentage of the control values. Significance is designated by an asterisk (*) for a difference from the control Daphnia length and that of the other rubbers at the same concentration (one-way ANOVA with Dunnett and two-way ANOVA with Tukey HSD, P ≤ 0.05). CR, crumb rubber; RR, recycled rubber; TP, tyre particles. Non-normalised figures with control bars are shown in the Supplementary material (Supplementary Fig. S9).
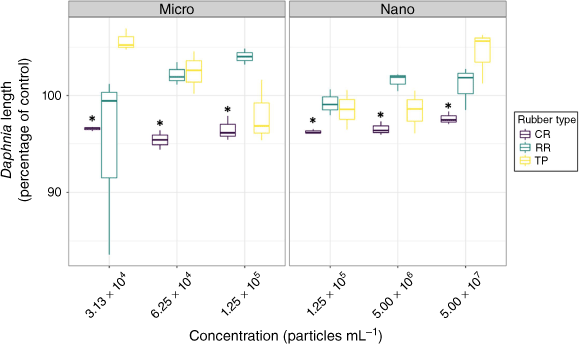
(a) The number of neonates per Daphnia per day produced by the F1 generation, normalised as a percentage of the control values. (b) The length of time in days that it took for Daphnia in the F1 generation to first produce neonates, normalised as a percentage of the control values. Significance is designated by an asterisk (*) for a difference with the control Daphnia (Kruskal–Wallis with Dunn test, P ≤ 0.05). CR, crumb rubber; RR, recycled rubber; TP, tyre particles. Non-normalised figures with control bars are shown in the Supplementary material (Supplementary Fig. S11 and S12). The caret symbol (^) shows where there are no bars because there was not enough reproduction for an F1 generation for these exposures.
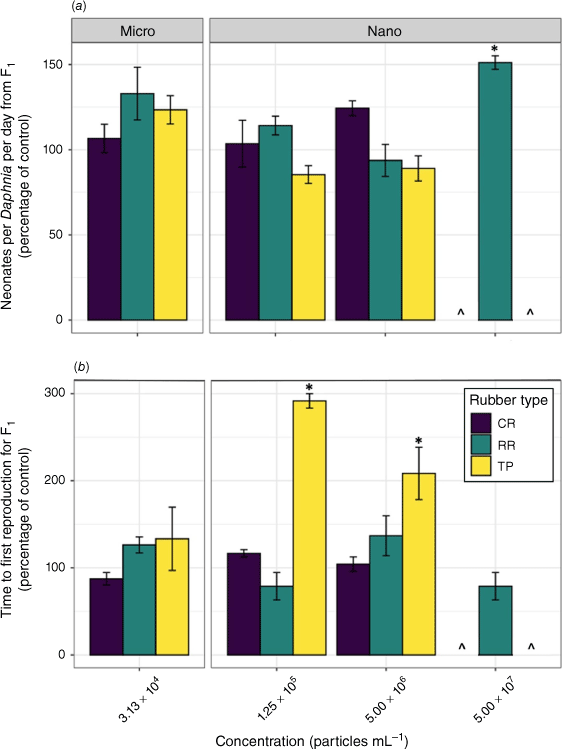
Results
Survival
Survival was not significantly affected by exposure to any of the micro or nano-rubber particles. This lack of mortality was by design, as sublethal concentrations were intentionally chosen. Supplementary Fig. S1 describes concentrations from a preliminary, range-finding experiment in which TPs became lethal before the full 28 days of the chronic exposure had passed. The range-finding experiments informed the sub-lethal concentrations of this study. For these chronic rubber exposures, all replicates began with five Daphnia, and none had fewer than four Daphnia at the end of the 28 days of exposure (Supplementary Fig. S2). No significant occurrence of immobility was observed in any of the controls or exposures during this experiment.
Reproduction
Reproduction was the endpoint most affected by exposure to sublethal concentrations of all three rubber types. The reproductive output was assessed both as the number of neonates produced per Daphnia per day and as the length of time it took for Daphnia to start producing neonates. All concentrations of micro-sized rubber particles (TPs, RR and CR) caused significant decreases in neonate production in comparison to the controls (Fig. 1, Kruskal–Wallis with Dunn’s test, P ≤ 0.05). At the lowest concentration exposure of micro-rubber, the TPs resulted in lower neonate production than the RR (Kruskal–Wallis with Dunn’s test, P ≤ 0.05), and in the medium exposure concentration to the micro-rubber the TPs and the RR had lower neonate production than the CR (Kruskal–Wallis with Dunn’s test, P ≤ 0.05). For both micro and nano-particles, a dose–dependent relationship was observed in which increasing concentrations of rubber particles resulted in decreasing amounts of neonates produced (Supplementary Fig. S3), with reproduction slowing or entyrely stopping at the highest concentrations of exposure. The EC50 values calculated for the micro-sized exposures ranged from 2.24 × 104 to 2.94 × 104 particles mL–1 and for the nano-sized exposures from 9.85 × 106 to 5.32 × 107 particles mL–1 (Table 1). For nano-sized exposures, the highest concentration (5.0 × 107 particles mL–1) of all three types of rubber particles caused a significant decrease in neonate production in comparison to the controls (Fig. 1, Kruskal–Wallis with Dunn test, P ≤ 0.05).
Particle size | Rubber type | Neonate production EC50 (particles mL–1) | Reproductive delay EC50 (particles mL–1) | |
---|---|---|---|---|
Micro (1–20 µm) | Tyre particle | 2.46 × 104 | – | |
Crumb rubber | 2.24 × 104 | – | ||
Recycled rubber | 2.94 × 104 | – | ||
Nano (<1 µm) | Tyre particle | 1.04 × 107 | 5.32 × 107 | |
Crumb rubber | 3.26 × 107 | – | ||
Recycled rubber | 1.12 × 107 | 9.85 × 106 |
Although the overall reproductive output was seen to decrease following chronic exposure to the rubber particles (Fig. 1), the amount of time in days that it took for a replicate beaker to first produce neonates increased for some of the rubbers (Fig. 2). For the Daphnia exposed to the micro-TPs, the time to first reproduction was significantly longer than control in the lowest concentration (3.13 × 104 particles mL–1) (Kruskal–Wallis with Dunn test, P ≤ 0.05). There were no values for the time to first reproduction for the higher two concentrations of the TPs or RR because those treatments never reproduced (Fig. 2). No concentration–response was observed in any of the micro-rubber exposures, or in nano-CR, for the reproduction delay (Supplementary Fig. S4). Exposure to the highest concentration of nano-TPs and RR (5.0 × 107 particles mL–1) significantly increased the time to first reproduction (Kruskal–Wallis with Dunn test, P ≤ 0.05). For the nano-rubber exposures, the time to reproduction was significantly different between the CR and RR at all concentrations, and between the CR and TPs at the highest concentration (Fig. 2, Kruskal–Wallis with Dunn tests, P ≤ 0.05). For delayed reproduction, EC50 values calculated for the nano-TPs and RR exposures ranged from 3.89 × 107 to 5.71 × 107 particles mL–1 (Table 1). No reproductive delay was observed in any of the CR exposures.
Growth and moulting
Only one rubber exposure affected the growth of the Daphnia over the 28-day chronic exposure (Fig. 3). The Daphnia exposed to the lowest concentration of the RR had the highest variation in length; however, the data were not significantly different than any of the other exposures and were all greater than 80% of the length of the control group. Though there were no significant differences in the final length of Daphnia exposed to the micro or nano-sized TPs or RR and their controls (one-way ANOVA and Dunnett, P > 0.05), the Daphnia exposed to the CR were significantly smaller than both their controls (one-way ANOVA and Dunnett, P ≤ 0.05) and those exposed to the other rubbers (two-way ANOVA and Tukey HSD, P ≤ 0.005) (Daphnia size ranges given in Supplementary Table S1). In contrast, no significant differences in comparison to the controls were found in the number of moults produced per Daphnia per day over the 28-day chronic exposure (Supplementary Fig. S6 and S10). Although the frequency of production did not differ, moults produced by micro-size exposed Daphnia frequently contained undeveloped eggs that were shed along with the moult (Supplementary Fig. S5). No Daphnia produced ephippia, including the control group, during the course of the experiments. During the measuring of the Daphnia, those exposed to rubber particles in the micro-size range were observed to have black particles in the digestive tract; however, the extent of this uptake was not quantified.
F1 generation
Owing to the lack of reproduction in the parental generation, not all exposure concentrations were able to produce neonates that could be grown out for the F1 generation. The Daphnia of the F1 generation included individuals from parents in the control, 3.13 × 104 particles mL–1 of micro-rubbers (TPs, RR and CR), 1.25 × 105 and 5.0 × 106 particles mL–1 of nano-rubbers (TPs, RR and CR) and 5.0 × 107 particles mL–1 nano-RR. Only the Daphnia from parents exposed to the highest concentration of nano-RR produced significantly more neonates per Daphnia per day (Fig. 4a, Kruskal–Wallis with Dunn test, P ≤ 0.05). Additionally, only the F1 Daphnia from parents exposed to the nano-TP treatments took significantly longer to start reproducing than those from all other parents (Fig. 4b, Kruskal–Wallis with Dunn test, P ≤ 0.05). Neither survival, number of moults per Daphnia per day, nor growth were significantly different (Supplementary Fig. S13 and S14).
Discussion
This is the first study to provide evidence of multigenerational affects of nano-rubber particles. Daphnia in this study were exposed to micro and nano-rubber particles at orders of magnitude lower than sublethal concentrations in our earlier acute exposures (Cunningham et al. 2022). It is important to assess affects of rubber particle exposures at these levels because sublethal stressors can still produce important and harmful ecological effects (Mayer‐Pinto et al. 2020). Few chronic toxicity assessments have been conducted with TPs. Magni et al. (2022) performed a 21-day chronic exposure of Daphnia to micro-crumb rubber granules from 1 to 100 mg L–1. Additionally, a chronic exposure of H. azteca found that larger TPs (<500 μm) caused mortality (Khan et al. 2019). No information could be found in the literature on subsequent generations of Daphnia from parents exposed to tyres, other rubbers or their leachates. Estimates for micro-sized TPs range from 0.03 to 59 mg L–1 in surface waters (Wik and Dave 2009). The micro-sized particle treatments in this study were ∼1.97, 3.94 and 7.87 mg L–1, which is within the estimated range of this size class of particles in the environment. However, due to the challenges associated with their size, no estimates of nano-sized rubber particles in the environment exist. Overall, sublethal effects of chronic rubber particle exposures to Daphnia were seen to differ across rubber particle types and size ranges.
Reproduction
The most severe effect observed in this study was the significant decrease in neonate production in all of the micro and some of the nano-rubber exposures. This effect on reproduction is in line with what others have reported following exposure of aquatic organisms to tyre-related materials. In exposing Daphnia to tyre leachate, Wik et al. (2009) also found reproduction to be the most sensitive endpoint. In their 9-day exposure with Ceriodaphnia dubia, they observed a decrease in reproductive output. Magni et al. (2022) found decreased reproductive output following chronic exposure of Daphnia to micro-crumb rubber granules at 9.8 mg L–1. Similarly, Khan et al. (2019) conducted a 21-day exposure of H. azteca to tyre wear particles and found that reproductive output decreased at their highest exposure, 1000 particles mL–1. Contrastingly, Carrasco-Navarro et al. (2022) performed a 28-day chronic exposure up to 750 mg kg–1 for freshwater sediment-dwelling organisms, Lumbriculus variegatus and Chironomus riparius, and found minimal effects on reproduction. However, research has shown that in soil, tyre-related rubber particles release less leachate, due to lower pH, and the resulting leachate is less toxic (Marwood et al. 2011; Panko et al. 2013); which would explain why we, and others, observed more severe effects for organisms exposed in water.
In this study, the negative effect on neonate production was dependent on the size of the rubber particles, with micro-sized particles exhibiting more severe decreases than nano-sized particles at comparable concentrations. Although the micro-CR had a small number of neonates produced even at the highest exposure concentration (1.25 × 105 particles mL–1), for the micro-TP and RR exposures, neonate production ceased starting at 6.25 × 104 particles mL–1. In comparison, for the nano-sized rubber particles, it took a much higher concentration, 5.0 × 107 particles mL–1, for a decrease in neonate production for all rubbers. In toxicity assessments of plastic particles to small aquatic organisms, typically smaller particles have been found to be more toxic than larger ones (Lee et al. 2013; Choi et al. 2020; Hazeem et al. 2020; An et al. 2021). For example, An et al. (2021) chronically exposed Daphnia to micro-sized polyethylene (PE) fragments and found that the smaller fragments reduced the number of offspring produced, whereas the larger ones did not. However, in this study, the effect of the micro-sized rubber particles were more severe than for the nano-sized rubber.
The reason that the reproductive effects of the micro-rubber were more severe than the nano-rubber could be physical, chemical or some combination of the two. One possible driver of this reversed trend is nutrient limitation due to the physical presence of the micro-particles. Nutrient quantity is linked to Daphnia reproductive ability (Bukovinszky et al. 2012). Though all Daphnia in this study were fed equivalent rations, the total volumes of the particles in the exposures varied between micro and nano-sizes. Micro-rubbers would take up a greater volume within the gut of the Daphnia and may have induced satiation (Lee et al. 2013; Straub et al. 2017), physically limiting nutrient uptake. In fact, micro and nano-sized TPs were shown to cause a decrease in growth in silversides and mysid shrimp at similar concentrations, likely due to food dilution (Siddiqui et al. 2022). Another potential explanation is that the magnitude of the effect was related to the mass of the tyre rubber in the exposures, and subsequently, the chemical compounds released through leaching. Exposures were conducted as number of particles per volume, but the mass of a micro-sized particle is much larger than that of a nano-sized particle. However, one would expect the higher surface area of smaller particles to result in higher leaching rates of chemicals (Stack et al. 2023). Tyre leachate contains endocrine-disrupting chemicals (Armada et al. 2022) that can affect reproduction (Tatarazako and Oda 2007), and the higher mass of rubber in the micro-sized exposures may have leached a greater amount of such chemicals during their suspension. Zinc and PAHs are other common components in tyre leachate (Rhodes et al. 2012; Lu et al. 2021) that have also been shown to decrease Daphnia reproductive output (De Schamphelaere et al. 2004; Feldmannová et al. 2006).
Reproductive effects also differed between the rubber types and were found to be more severe in Daphnia exposed to TPs or RR, than for those to CR. Exposure to the lowest concentration of micro-rubber particles did not cause a reproductive delay in the CR or RR, but did in the TPs. This reproductive delay could explain why neonate production at that concentration (3.13 × 104 particles mL–1) of TPs was lower than that of the other rubbers (Fig. 1). In the nano-rubber exposures, there were no differences between the rubber types for reproductive output; however, for the timing of first reproduction, only the TPs and RR had a delay whereas the CR did not. In fact, none of the CR exposures, micro or nano, showed a change in the amount of time to first reproduction. This highlights how specific differences in tyre formulations can potentially influence the effects.
Overall, the reproductive toxicity of the CR was lower than that of the other rubber types. For rubber exposures that experienced an increase in the time to first reproduction, endocrine-disrupting chemicals, which are known to delay first brood production in Daphnia (Cho et al. 2022) and have been documented in tyre leachate (Halsband et al. 2020; Armada et al. 2022), may be contributing to the observed delay. Although those same chemicals would be expected in the leachate of all the rubbers, the concentrations could vary with rubber composition. This difference in toxicity may also be related to the age and weathering of the rubber types. Aging of both tyres and crumb rubber granulates has been shown to decrease the toxicity of them and their leachates (Lu et al. 2021; Tallec et al. 2022). Full age and weathering information for the two recycled tyre samples that we used (RR and CR) is not available nor do we know the type of tyre rubber that was sourced to produce these materials. It is possible that the CR sample came from older tyres than both the TPs and RR, or from different types of tyres with differing compositions, which may have decreased its reproductive toxicity.
Growth and moulting
In this study, although the reproductive effects of the CR on the Daphnia were less than those of the TPs and RR, the CR effects on the growth of the Daphnia were greater. CR-exposed Daphnia were the only ones smaller than their controls after 28 days of exposure. This again suggests that the chemical composition of the CR differs from that of the TPs and RR. All CR exposures had the same magnitude of effect on Daphnia growth regardless of particle size or concentration. Because the effect of the CR on growth was the same for micro and nano-size exposures, it was likely not caused by food dilution or taking up physical space within the Daphnia gut. It is more likely that this effect was caused by chemicals leached from the CR particles, which may be different or in different quantities to the other rubber particles. Phthalates, found in tyre leachate (Halsband et al. 2020), have been shown to reduce Daphnia body length after 14-day chronic exposure (Seyoum and Pradhan 2019). Although phthalates would also be expected in the TPs and RR, their chemical compositions or quantities may differ.
Whether chronic exposure to plastic particles affects growth seems to be highly dependent on the composition of the particles. Although this current study only found that one type of rubber (CR) particles affected the growth of the Daphnia, other chronic exposures of aquatic invertebrates to tyre micro-particles or leachate have seen negative effects on growth (Wik et al. 2009; Khan et al. 2019; Magni et al. 2022). Additionally, conflicting information exists on the growth affects of chronic exposure of Daphnia to particles of other polymer types. For example, chronic exposure to micro low-density polyethylene (LDPE) (Jemec Kokalj et al. 2021) and nano-polystyrene (PS) (Liu et al. 2020) had no effect on growth; whereas micro-polyvinyl chloride (PVC), polylactic acid (PLA) and polyurethane (PUR) (Zimmermann et al. 2020), and a different chronic exposure to nano-PS (Pikuda et al. 2022), did reduce growth.
Although none of the rubber exposures had affected the moulting of the Daphnia throughout the 28 days of the experiment, moults produced by micro-size exposed Daphnia after reaching reproductive maturity frequently contained undeveloped eggs, though not ephippia. The reason for this high level of non-viable egg production is not known. Leachate from tyre rubber contains high levels of dissolved organic carbon (DOC) (Selbes et al. 2015). The addition of the DOC could be a contributor to egg abortion because it has also been suggested that Daphnia may use non-viable egg production to discard excess carbon (He and Wang 2008). A nutritionally inadequate diet can also result in the production of non-viable eggs (Urabe and Sterner 2001). Therefore, the micro-rubber particles taking up space within the gut of the Daphnia could also be a contributor to the non-viable egg production. The nano-size exposed Daphnia did not display the same level of non-viable egg production as the micro-size exposed, which provides support for this concept.
F1 generation
Effects on the F1 Daphnia were only seen in those produced by parents in nano-rubber exposures, and not in the lowest micro-rubber exposure, which was the only micro-rubber exposure level with enough neonates produced to have an F1 generation. Although no information could be found in the literature on subsequent generations of Daphnia from parents exposed to tyres, other rubbers or their leachates, generational effects have been reported for other nanoplastic exposures. For example, Daphnia chronically exposed to nano-PS had multigenerational effects on reproductive output and delays that lasted into the F2 generation (Nogueira et al. 2022). Additionally, it is known that Daphnia young can take up nano-sized plastics while still in the brood pouch (Brun et al. 2017). Following a chronic nano-PS exposure, Xu et al. (2020) detected nanoplastics in the brood chamber, which transferred to F1 neonates. This transfer of particles during the egg production and brooding period is likely the reason for the effects on the F1 Daphnia, as they themselves were grown in particle-free water.
F1 Daphnia from parents exposed to nano-TPs took longer to reproduce, even though their parents, who were directly exposed to the nano-TPs, did not. This trend, which was also observed by Nogueira et al. (2022) who did not see a delay in first reproduction for the parental generation exposed to nano-PS, but did see delayed reproduction in the F1 and F2 generations, merits further study. Neonate production was significantly higher for Daphnia from parents in the highest nano-size concentration of RR and not the other rubber types. A reason for the difference between the rubber types could be because the parental Daphnia in the highest nano-RR treatment took longer than the other rubbers and concentrations to first produce offspring (Fig. 2). Therefore, the F1 Daphnia in this group (from high RR parents) came from older parents than the other F1 Daphnia. It has been shown that older Daphnia produce offspring that mature earlier and are more fecund (Sakwińska 2004). Alternatively, increased offspring production can also be a stress response.
No effects were observed on the survival, moulting or growth of the F1 Daphnia. Subsequent generations of Daphnia from parents chronically exposed to other plastics have also not been affected by those parental exposures. For example, Cui et al. (2017) found that the survival was not affected for offspring of Daphnia chronically exposed to nano-PS. Additionally, Xu et al. (2020) chronically exposed Daphnia to nano-PS and found no effects on the F1 generation; however, they did note reduced reproduction in the F2 generation.
Leachate chemical analyses
In the above discussion the potential contributions of both the physical and chemical aspects of the rubber particles to the observed effects have been discussed. Because rubber leachate contains a mixture of chemicals, attributions of toxicity endpoints to specific compounds, or combinations of compounds, is very difficult. Mayer et al. (2024) compiled a comprehensive list of known chemical emissions from tyres. These compounds include additives, antioxidants, vulcaniation agents, PAHs, metals and plasticisers (Mayer et al. 2024). This list gets even larger when rubber particles are exposed to sunlight or elevated temperatures during leaching, and transformation products are formed (Foscari et al. 2023; Weyrauch et al. 2023). Müller et al. (2022) identified over 200 organics in TPs, 68% of which were classified as leachables. Additionally, Stack et al. (2023) conducted leaching experiments with larger (32 µm and 1 cm) TPs produced by the same lab that generated the particles for this study, and identified amines, benzothiazoles and quinoline-related compounds that were not previously reported in tyre-leachate literature. Although current methods are able to identify many of the compounds leached by tyre rubbers, some remain unidentified (Khan et al. 2024).
The ability of the rubber particles to continue leaching over time complicates attempts to separate effects specifically related to particles. This means that even washed, “clean” particles could produce leachate in their exposure media. One solution to this problem could be an exposure with fully leached particles. However, the leaching rates of the many chemical compounds within the rubber are not fully understood, meaning that it is not known how long the particles would need to be leached before a fully leached particle was attained. It is known that the method of tyre leachate creation will affect the final leachate product, including the type and brand of tyre used (Jeong et al. 2022; Ding et al. 2023), size and aggregation of particles (Gualtieri et al. 2005; Stack et al. 2023), length of leaching time (Halsband et al. 2020; Chibwe et al. 2022), lighting and UV during exposures (Lu et al. 2021; Foscari et al. 2023), and aging of particles in advance (Halsband et al. 2020; Weyrauch et al. 2023). For this reason, when making comparisons between particulate and leachate exposure, the leachate needs to be extracted from suspensions created in an identical manner to those used for the rubber particle exposure.
An inability to run leachate only controls was a confounding factor in this study. The largest barrier to doing this is the difficulty of removing the nano-size fraction of rubber particles from the leachate. Typically, nanoplastics are classified as particles below 1 µm (Gigault et al. 2018); however, a true nano-particle is smaller than 100 nm (0.1 µm). As detailed in Cunningham et al. (2022), for the creation of particle-free leachate we used 0.02-µm filters, which will remove all particles above 20 nm, to remove as much of the nano-rubber particles as possible. Such a fine-scale level of filtration was not possible to generate the large volume of leachate that would have been necessary for chronic exposures of the three rubber types. Other studies that have conducted exposures to tyre leachate use larger filters that do not effectively remove particles in the nano-size range (e.g. 0.2-µm cellulose filter, Boisseaux et al. 2024; 0.45-µm Whatman glass fibre filter, Chibwe et al. 2022; 0.2-μm Nalgene, Capolupo et al. 2020; 0.45-µm SimplePure nylon filter, Halle et al. 2021; and 0.45-µm filters, Magni et al. 2022). This leaves behind a fraction of nano-TPs that may continue to leach throughout the exposure, unintentionally altering the leachate composition. Future chronic experiments are needed with leachate exposures filtered to remove as much of the nano-rubber particles as possible; then chronic toxicity of the leachate can truly be assessed independently from particulate.
Conclusion
This is the first comparison of chronic and generational toxicity between different sizes and compositions of rubber particles. The most sensitive endpoint in these chronic exposures was reproduction. Overall, reproductive effects, both output and delay, were more severe for: (1) TPs and RR than for the CR tested, and (2) micro-sized rubber than for nano-sized rubber. The effects of the micro-particles may also be related to nutrient dilution due to their physical presence in the Daphnia gut. Only the offspring from nano-size exposed parents exhibited multigenerational effects on reproduction. This indicates a slower surface-area-related leaching, or size-related mechanism of toxicity not available for larger particles. Research is needed to identify which components of the rubber leachate are severely affecting reproductive ability and multigenerational effects, even at these sublethal levels of exposure.
Data availability
The data used to generate the results in the paper will be made available upon all reasonable requests.
Declaration of funding
This research was funded by the National Science Foundation Growing Convergence Research Big Idea (to S. Harper and S. M. Brander), 1935028. Research reported in this publication was supported by the National Institute of Environmental Health Sciences of the National Institutes of Health under Award Number T32ES007060. The content is solely the responsibility of the authors and does not necessarily represent the official views of the National Institutes of Health.
Author contributions
S. M. Brander, B. J. Harper and S. Harper provided conceptualisation. B. E. Cunningham provided investigation, analysis and manuscript writing. S. Harper contributed supervision, project administration, and funding acquisition (as PI). All authors were responsible for methodology, and review, editing, and approval of the accepted version of the manuscript.
References
An D, Na J, Song J, Jung J (2021) Size-dependent chronic toxicity of fragmented polyethylene microplastics to Daphnia magna. Chemosphere 271, 129591.
| Crossref | Google Scholar | PubMed |
Armada D, Llompart M, Celeiro M, Garcia-Castro P, Ratola N, Dagnac T, de Boer J (2022) Global evaluation of the chemical hazard of recycled tire crumb rubber employed on worldwide synthetic turf football pitches. Science of The Total Environment 812, 152542.
| Crossref | Google Scholar | PubMed |
Baensch-Baltruschat B, Kocher B, Stock F, Reifferscheid G (2020) Tyre and road wear particles (TRWP) – a review of generation, properties, emissions, human health risk, ecotoxicity, and fate in the environment. Science of The Total Environment 733, 137823.
| Crossref | Google Scholar | PubMed |
Benson K, Irvin-Barnwell E, Ragin-Wilson A, Breysse P (2019) Federal research action plan on recycled tire crumb used on playing fields: tire crumb rubber characterization and exposure characterization study overview. Journal of Environmental Health 82(2), 28-30.
| Google Scholar | PubMed |
Birkholz DA, Belton KL, Guidotti TL (2003) Toxicological evaluation for the hazard assessment of tire crumb for use in public playgrounds. Journal of the Air & Waste Management Association 53(7), 903-907.
| Crossref | Google Scholar | PubMed |
Boisseaux P, Rauert C, Dewapriya P, Delignette-Muller M-L, Barrett R, Durndell L, Pohl F, Thompson R, Thomas KV, Galloway T (2024) Deep dive into the chronic toxicity of tyre particle mixtures and their leachates. Journal of Hazardous Materials 466, 133580.
| Crossref | Google Scholar | PubMed |
Boucher J, Friot D (2017) ‘Primary microplastics in the oceans: a global evaluation of sources.’ (IUCN: Gland, Switzerland) 10.2305/IUCN.CH.2017.01.en
Brun NR, Beenakker MM, Hunting ER, Ebert D, Vijver MG (2017) Brood pouch-mediated polystyrene nanoparticle uptake during Daphnia magna embryogenesis. Nanotoxicology 11(8), 1059-1069.
| Crossref | Google Scholar | PubMed |
Bukovinszky T, Verschoor AM, Helmsing NR, Bezemer TM, Bakker ES, Vos M, de Senerpont Domis LN (2012) The good, the bad and the plenty: interactive effects of food quality and quantity on the growth of different Daphnia species. PLoS One 7(9), e42966.
| Crossref | Google Scholar | PubMed |
Capolupo M, Sørensen L, Jayasena KDR, Booth AM, Fabbri E (2020) Chemical composition and ecotoxicity of plastic and car tire rubber leachates to aquatic organisms. Water Research 169, 115270.
| Crossref | Google Scholar | PubMed |
Carrasco-Navarro V, Nuutinen A, Sorvari J, Kukkonen JV (2022) Toxicity of tire rubber microplastics to freshwater sediment organisms. Archives of Environmental Contamination and Toxicology 82(2), 180-190.
| Crossref | Google Scholar | PubMed |
Celeiro M, Armada D, Ratola N, Dagnac T, De Boer J, Llompart M (2021) Evaluation of chemicals of environmental concern in crumb rubber and water leachates from several types of synthetic turf football pitches. Chemosphere 270, 128610.
| Crossref | Google Scholar | PubMed |
Cheong R, Dumont ER, Thomson P, Castañeda-Cortés D, Hernandez L, Gao X, Zheng J, Baesu A, Macairan J, Smith A (2023) Nanoparticle-specific and chemical-specific effects of tire wear particle leachate on amphibian early life stages. Journal of Hazardous Materials Advances 12, 100357.
| Crossref | Google Scholar |
Chibwe L, Parrott JL, Shires K, Khan H, Clarence S, Lavalle C, Sullivan C, O’Brien AM, De Silva AO, Muir DC (2022) A deep dive into the complex chemical mixture and toxicity of tire wear particle leachate in fathead minnow. Environmental Toxicology and Chemistry 41(5), 1144-1153.
| Crossref | Google Scholar | PubMed |
Cho H, Ryu CS, Lee S-A, Adeli Z, Meupea BT, Kim Y, Kim YJ (2022) Endocrine-disrupting potential and toxicological effect of para-phenylphenol on Daphnia magna. Ecotoxicology and Environmental Safety 243, 113965.
| Crossref | Google Scholar | PubMed |
Choi JS, Hong SH, Park J-W (2020) Evaluation of microplastic toxicity in accordance with different sizes and exposure times in the marine copepod Tigriopus japonicus. Marine Environmental Research 153, 104838.
| Crossref | Google Scholar | PubMed |
Cui R, Kim SW, An Y-J (2017) Polystyrene nanoplastics inhibit reproduction and induce abnormal embryonic development in the freshwater crustacean Daphnia galeata. Scientific Reports 7(1), 12095.
| Crossref | Google Scholar | PubMed |
Cunningham B, Harper B, Brander S, Harper S (2022) Toxicity of micro and nano tire particles and leachate for model freshwater organisms. Journal of Hazardous Materials 429, 128319.
| Crossref | Google Scholar | PubMed |
De Schamphelaere K, Canli M, Van Lierde V, Forrez I, Vanhaecke F, Janssen C (2004) Reproductive toxicity of dietary zinc to Daphnia magna. Aquatic Toxicology 70(3), 233-244.
| Crossref | Google Scholar | PubMed |
Ding J, Lv M, Wang Q, Zhu D, Chen Q-L, Li X-Q, Yu C-P, Xu X, Chen L, Zhu Y-G (2023) Brand-specific toxicity of tire tread particles helps identify the determinants of toxicity. Environmental Science & Technology 57(30), 11267-11278.
| Crossref | Google Scholar | PubMed |
Duckworth J, Jager T, Ashauer R (2019) Automated, high-throughput measurement of size and growth curves of small organisms in well plates. Scientific Reports 9(1), 10.
| Crossref | Google Scholar | PubMed |
Eisentraut P, Dümichen E, Ruhl AS, Jekel M, Albrecht M, Gehde M, Braun U (2018) Two birds with one stone – fast and simultaneous analysis of microplastics: microparticles derived from thermoplastics and tire wear. Environmental Science & Technology Letters 5(10), 608-613.
| Crossref | Google Scholar |
Feldmannová M, Hilscherová K, Marsálek B, Bláha L (2006) Effects of N‐heterocyclic polyaromatic hydrocarbons on survival, reproduction, and biochemical parameters in Daphnia magna. Environmental Toxicology: An International Journal 21(4), 425-431.
| Crossref | Google Scholar | PubMed |
Fiksel J, Bakshi BR, Baral A, Guerra E, DeQuervain B (2011) Comparative life cycle assessment of beneficial applications for scrap tires. Clean Technologies and Environmental Policy 13(1), 19-35.
| Crossref | Google Scholar |
Foscari A, Schmidt N, Seiwert B, Herzke D, Sempéré R, Reemtsma T (2023) Leaching of chemicals and DOC from tire particles under simulated marine conditions. Frontiers in Environmental Science 11, 1206449.
| Crossref | Google Scholar |
Gigault J, Halle AT, Baudrimont M, Pascal P-Y, Gauffre F, Phi T-L, El Hadri H, Grassl B, Reynaud S (2018) Current opinion: what is a nanoplastic? Environmental Pollution 235, 1030-1034.
| Crossref | Google Scholar | PubMed |
Grillo R, Rosa AH, Fraceto LF (2015) Engineered nanoparticles and organic matter: a review of the state-of-the-art. Chemosphere 119, 608-619.
| Crossref | Google Scholar | PubMed |
Gualtieri M, Andrioletti M, Vismara C, Milani M, Camatini M (2005) Toxicity of tire debris leachates. Environment International 31(5), 723-730.
| Crossref | Google Scholar | PubMed |
Halle LL, Palmqvist A, Kampmann K, Khan FR (2020) Ecotoxicology of micronized tire rubber: past, present and future considerations. Science of The Total Environment 706, 135694.
| Crossref | Google Scholar | PubMed |
Halle LL, Palmqvist A, Kampmann K, Jensen A, Hansen T, Khan FR (2021) Tire wear particle and leachate exposures from a pristine and road-worn tire to Hyalella azteca: comparison of chemical content and biological effects. Aquatic Toxicology 232, 105769.
| Crossref | Google Scholar | PubMed |
Halsband C, Sørensen L, Booth AM, Herzke D (2020) Car tire crumb rubber: does leaching produce a toxic chemical cocktail in coastal marine systems? Frontiers in Environmental Science 8, 125.
| Crossref | Google Scholar |
Hazeem LJ, Yesilay G, Bououdina M, Perna S, Cetin D, Suludere Z, Barras A, Boukherroub R (2020) Investigation of the toxic effects of different polystyrene micro-and nanoplastics on microalgae Chlorella vulgaris by analysis of cell viability, pigment content, oxidative stress and ultrastructural changes. Marine Pollution Bulletin 156, 111278.
| Crossref | Google Scholar | PubMed |
He X, Wang W-X (2008) Stoichiometric regulation of carbon and phosphorus in P‐deficient Daphnia magna. Limnology and Oceanography 53(1), 244-254.
| Crossref | Google Scholar |
Jemec Kokalj A, Dolar A, Titova J, Visnapuu M, Škrlep L, Drobne D, Vija H, Kisand V, Heinlaan M (2021) Long term exposure to virgin and recycled LDPE microplastics induced minor effects in the freshwater and terrestrial crustaceans Daphnia magna and Porcellio scaber. Polymers 13(5), 771.
| Crossref | Google Scholar | PubMed |
Jeong Y, Lee S, Woo S-H (2022) Chemical leaching from tire wear particles with various treadwear ratings. International Journal of Environmental Research and Public Health 19(10), 6006.
| Crossref | Google Scholar | PubMed |
Khan FR, Halle LL, Palmqvist A (2019) Acute and long-term toxicity of micronized car tire wear particles to Hyalella azteca. Aquatic Toxicology 213, 105216.
| Crossref | Google Scholar | PubMed |
Khan FR, Rødland ES, Kole PJ, Van Belleghem FG, Jaén-Gil A, Hansen SF, Gomiero A (2024) An overview of the key topics related to the study of tire particles and their chemical leachates: from problems to solutions. TrAC Trends in Analytical Chemistry 172, 117563.
| Crossref | Google Scholar |
Kole PJ, Löhr AJ, Van Belleghem F, Ragas A (2017) Wear and tear of tyres: a stealthy source of microplastics in the environment. International Journal of Environmental Research and Public Health 14(10), 1265.
| Crossref | Google Scholar | PubMed |
Kukutschová J, Moravec P, Tomášek V, Matějka V, Smolík J, Schwarz J, Seidlerová J, Safářová K, Filip P (2011) On airborne nano/micro-sized wear particles released from low-metallic automotive brakes. Environmental Pollution 159(4), 998-1006.
| Crossref | Google Scholar | PubMed |
LaPlaca SB, van den Hurk P (2020) Toxicological effects of micronized tire crumb rubber on mummichog (Fundulus heteroclitus) and fathead minnow (Pimephales promelas). Ecotoxicology 29(5), 524-534.
| Crossref | Google Scholar | PubMed |
LaPlaca SB, Rice CD, van den Hurk P (2022) Chronic toxicity of tire crumb rubber particles to mummichog (Fundulus heteroclitus) in episodic exposures. Science of The Total Environment 846, 157447.
| Crossref | Google Scholar | PubMed |
Lee K-W, Shim WJ, Kwon OY, Kang J-H (2013) Size-dependent effects of micro polystyrene particles in the marine copepod Tigriopus japonicus. Environmental Science & Technology 47(19), 11278-11283.
| Crossref | Google Scholar | PubMed |
Liu Z, Cai M, Yu P, Chen M, Wu D, Zhang M, Zhao Y (2018) Age-dependent survival, stress defense, and AMPK in Daphnia pulex after short-term exposure to a polystyrene nanoplastic. Aquatic Toxicology 204, 1-8.
| Crossref | Google Scholar | PubMed |
Liu Z, Cai M, Wu D, Yu P, Jiao Y, Jiang Q, Zhao Y (2020) Effects of nanoplastics at predicted environmental concentration on Daphnia pulex after exposure through multiple generations. Environmental Pollution 256, 113506.
| Crossref | Google Scholar |
Llompart M, Sanchez-Prado L, Lamas JP, Garcia-Jares C, Roca E, Dagnac T (2013) Hazardous organic chemicals in rubber recycled tire playgrounds and pavers. Chemosphere 90(2), 423-431.
| Crossref | Google Scholar | PubMed |
Lu F, Su Y, Ji Y, Ji R (2021) Release of zinc and polycyclic aromatic hydrocarbons from tire crumb rubber and toxicity of leachate to Daphnia magna: effects of tire source and photoaging. Bulletin of Environmental Contamination and Toxicology 107(4), 651-656.
| Crossref | Google Scholar | PubMed |
Luo Z, Zhou X, Su Y, Wang H, Yu R, Zhou S, Xu EG, Xing B (2021) Environmental occurrence, fate, impact, and potential solution of tire microplastics: Similarities and differences with tire wear particles. Science of The Total Environment 795, 148902.
| Crossref | Google Scholar |
Magni S, Tediosi E, Maggioni D, Sbarberi R, Noé F, Rossetti F, Fornai D, Persici V, Neri MC (2022) Ecological impact of end-of-life-tire (ELT)-derived rubbers: acute and chronic effects at organism and population levels. Toxics 10(5), 201.
| Crossref | Google Scholar | PubMed |
Marwood C, McAtee B, Kreider M, Ogle RS, Finley B, Sweet L, Panko J (2011) Acute aquatic toxicity of tire and road wear particles to alga, daphnid, and fish. Ecotoxicology 20(8), 2079-2089.
| Crossref | Google Scholar | PubMed |
Mayer PM, Moran KD, Miller EL, Brander SM, Harper S, Garcia-Jaramillo M, Carrasco-Navarro V, Ho KT, Burgess RM, Hampton LMT (2024) Where the rubber meets the road: emerging environmental impacts of tire wear particles and their chemical cocktails. Science of The Total Environment 927, 171153.
| Crossref | Google Scholar | PubMed |
Mayer‐Pinto M, Ledet J, Crowe TP, Johnston EL (2020) Sublethal effects of contaminants on marine habitat‐forming species: a review and meta‐analysis. Biological Reviews 95(6), 1554-1573.
| Crossref | Google Scholar | PubMed |
Müller K, Hübner D, Huppertsberg S, Knepper TP, Zahn D (2022) Probing the chemical complexity of tires: Identification of potential tire-borne water contaminants with high-resolution mass spectrometry. Science of The Total Environment 802, 149799.
| Crossref | Google Scholar | PubMed |
Murphy M, Warner GR (2022) Health impacts of artificial turf: toxicity studies, challenges, and future directions. Environmental Pollution 310, 119841.
| Crossref | Google Scholar | PubMed |
Nogueira DJ, da Silva ACO, da Silva MLN, Vicentini DS, Matias WG (2022) Individual and combined multigenerational effects induced by polystyrene nanoplastic and glyphosate in Daphnia magna (Strauss, 1820). Science of The Total Environment 811, 151360.
| Crossref | Google Scholar | PubMed |
Organisation for Economic Co-operation and Development (2012) Test number 211: Daphnia magna reproduction test. In ‘OECD Guidelines for the Testing of Chemicals, Section 2’. (OECD Publishing: Paris, France) 10.1787/9789264185203-en
Panko JM, Kreider ML, McAtee BL, Marwood C (2013) Chronic toxicity of tire and road wear particles to water-and sediment-dwelling organisms. Ecotoxicology 22(1), 13-21.
| Crossref | Google Scholar | PubMed |
Pikuda O, Dumont ER, Matthews S, Xu EG, Berk D, Tufenkji N (2022) Sub-lethal effects of nanoplastics upon chronic exposure to Daphnia magna. Journal of Hazardous Materials Advances 7, 100136.
| Crossref | Google Scholar |
Pochron ST, Fiorenza A, Sperl C, Ledda B, Patterson CL, Tucker CC, Tucker W, Ho YL, Panico N (2017) The response of earthworms (Eisenia fetida) and soil microbes to the crumb rubber material used in artificial turf fields. Chemosphere 173, 557-562.
| Crossref | Google Scholar | PubMed |
Redondo-Hasselerharm PE, de Ruijter VN, Mintenig SM, Verschoor A, Koelmans AA (2018) Ingestion and chronic effects of car tire tread particles on freshwater benthic macroinvertebrates. Environmental Science & Technology 52(23), 13986-13994.
| Crossref | Google Scholar | PubMed |
Rhodes EP, Ren Z, Mays DC (2012) Zinc leaching from tire crumb rubber. Environmental Science & Technology 46(23), 12856-12863.
| Crossref | Google Scholar | PubMed |
Ritz C, Baty F, Streibig JC, Gerhard D (2015) Dose-response analysis using R. PloS ONE 10(12), e0146021.
| Crossref | Google Scholar | PubMed |
Sakwińska O (2004) Persistent maternal identity effects on life history traits in Daphnia. Oecologia 138(3), 379-386.
| Crossref | Google Scholar | PubMed |
Schmid S, Rundberget JT, Song Y, Tollefsen KE (2023) Age and synchronization of Daphnia magna affect sensitivity to teflubenzuron in acute standardized toxicity tests. Environmental Toxicology and Chemistry 42(8), 1806-1815.
| Crossref | Google Scholar | PubMed |
Selbes M, Yilmaz O, Khan AA, Karanfil T (2015) Leaching of DOC, DN, and inorganic constituents from scrap tires. Chemosphere 139, 617-623.
| Crossref | Google Scholar | PubMed |
Seyoum A, Pradhan A (2019) Effect of phthalates on development, reproduction, fat metabolism and lifespan in Daphnia magna. Science of The Total Environment 654, 969-977.
| Crossref | Google Scholar | PubMed |
Siddiqui S, Dickens JM, Cunningham BE, Hutton SJ, Pedersen EI, Harper B, Harper S, Brander SM (2022) Internalization, reduced growth, and behavioral effects following exposure to micro and nano tire particles in two estuarine indicator species. Chemosphere 296, 133934.
| Crossref | Google Scholar | PubMed |
Sieber R, Kawecki D, Nowack B (2020) Dynamic probabilistic material flow analysis of rubber release from tires into the environment. Environmental Pollution 258, 113573.
| Crossref | Google Scholar | PubMed |
Stack M, Hollman K, Mladenov N, Harper B, Pinongcos F, Sant K, Rochman C, Richardot W, Dodder N, Hoh E (2023) Micron-size tire tread particles leach organic compounds at higher rates than centimeter-size particles: compound identification and profile comparison. Environmental Pollution 334, 122116.
| Crossref | Google Scholar | PubMed |
Straub S, Hirsch PE, Burkhardt-Holm P (2017) Biodegradable and petroleum-based microplastics do not differ in their ingestion and excretion but in their biological effects in a freshwater invertebrate Gammarus fossarum. International Journal of Environmental Research and Public Health 14(7), 774.
| Crossref | Google Scholar | PubMed |
Sugiura M, Takada H, Takada N, Mizukawa K, Tsuyuki S, Furumai H (2021) Microplastics in urban wastewater and estuarine water: importance of street runoff. Environmental Monitoring and Contaminants Research 1, 54-65.
| Crossref | Google Scholar |
Tallec K, Huvet A, Yeuc’h V, Le Goïc N, Paul-Pont I (2022) Chemical effects of different types of rubber-based products on early life stages of Pacific oyster, Crassostrea gigas. Journal of Hazardous Materials 427, 127883.
| Crossref | Google Scholar | PubMed |
Tatarazako N, Oda S (2007) The water flea Daphnia magna (Crustacea, Cladocera) as a test species for screening and evaluation of chemicals with endocrine disrupting effects on crustaceans. Ecotoxicology 16(1), 197-203.
| Crossref | Google Scholar | PubMed |
Thomas K, Irvin-Barnwell E, Guiseppi-Elie A, Ragin-Wilson A, Zambrana J (2019) Synthetic turf field recycled tire crumb rubber research under the Federal Research Action Plan: final report part 1 – tire crumb rubber characterization, volume 1. EPA/600/R-19/051.2, US Environmental Protection Agency, Washington, DC, USA.
Tian Z, Gonzalez M, Rideout CA, Zhao HN, Hu X, Wetzel J, Mudrock E, James CA, McIntyre JK, Kolodziej EP (2022) 6PPD-quinone: revised toxicity assessment and quantification with a commercial standard. Environmental Science & Technology Letters 9(2), 140-146.
| Google Scholar |
Traudt EM, Ranville JF, Meyer JS (2017) Effect of age on acute toxicity of cadmium, copper, nickel, and zinc in individual‐metal exposures to Daphnia magna neonates. Environmental Toxicology and Chemistry 36(1), 113-119.
| Crossref | Google Scholar | PubMed |
Turner A, Rice L (2010) Toxicity of tire wear particle leachate to the marine macroalga, Ulva lactuca. Environmental Pollution 158(12), 3650-3654.
| Crossref | Google Scholar | PubMed |
Urabe J, Sterner R (2001) Contrasting effects of different types of resource depletion on life‐history traits in Daphnia. Functional Ecology 15(2), 165-174.
| Crossref | Google Scholar |
Varshney S, Gora AH, Siriyappagouder P, Kiron V, Olsvik PA (2022) Toxicological effects of 6PPD and 6PPD quinone in zebrafish larvae. Journal of Hazardous Materials 424, 127623.
| Crossref | Google Scholar | PubMed |
Verschoor AJ, Van Gelderen A, Hofstra U (2021) Fate of recycled tyre granulate used on artificial turf. Environmental Sciences Europe 33(1), 1-15.
| Crossref | Google Scholar |
Wagner S, Hüffer T, Klöckner P, Wehrhahn M, Hofmann T, Reemtsma T (2018) Tire wear particles in the aquatic environment – a review on generation, analysis, occurrence, fate and effects. Water research 139, 83-100.
| Crossref | Google Scholar | PubMed |
Wang T, Li B, Zou X, Wang Y, Li Y, Xu Y, Mao L, Zhang C, Yu W (2019) Emission of primary microplastics in mainland China: invisible but not negligible. Water Research 162, 214-224.
| Crossref | Google Scholar | PubMed |
Werbowski LM, Gilbreath AN, Munno K, Zhu X, Grbic J, Wu T, Sutton R, Sedlak MD, Deshpande AD, Rochman CM (2021) Urban stormwater runoff: a major pathway for anthropogenic particles, black rubbery fragments, and other types of microplastics to urban receiving waters. ACS ES&T Water 1(6), 1420-1428.
| Crossref | Google Scholar |
Weyrauch S, Seiwert B, Voll M, Wagner S, Reemtsma T (2023) Accelerated aging of tire and road wear particles by elevated temperature, artificial sunlight and mechanical stress—A laboratory study on particle properties, extractables and leachables. Science of The Total Environment 904, 166679.
| Crossref | Google Scholar | PubMed |
Wik A, Dave G (2005) Environmental labeling of car tires – toxicity to Daphnia magna can be used as a screening method. Chemosphere 58(5), 645-651.
| Crossref | Google Scholar | PubMed |
Wik A, Dave G (2006) Acute toxicity of leachates of tire wear material to Daphnia magna – variability and toxic components. Chemosphere 64(10), 1777-1784.
| Crossref | Google Scholar | PubMed |
Wik A, Dave G (2009) Occurrence and effects of tire wear particles in the environment – a critical review and an initial risk assessment. Environmental Pollution 157(1), 1-11.
| Crossref | Google Scholar | PubMed |
Wik A, Nilsson E, Källqvist T, Tobiesen A, Dave G (2009) Toxicity assessment of sequential leachates of tire powder using a battery of toxicity tests and toxicity identification evaluations. Chemosphere 77(7), 922-927.
| Crossref | Google Scholar | PubMed |
Xu EG, Cheong RS, Liu L, Hernandez LM, Azimzada A, Bayen S, Tufenkji N (2020) Primary and secondary plastic particles exhibit limited acute toxicity but chronic effects on Daphnia magna. Environmental Science & Technology 54(11), 6859-6868.
| Crossref | Google Scholar | PubMed |
Ziajahromi S, Drapper D, Hornbuckle A, Rintoul L, Leusch FD (2020) Microplastic pollution in a stormwater floating treatment wetland: detection of tyre particles in sediment. Science of The Total Environment 713, 136356.
| Crossref | Google Scholar | PubMed |
Zimmermann L, Göttlich S, Oehlmann J, Wagner M, Völker C (2020) What are the drivers of microplastic toxicity? Comparing the toxicity of plastic chemicals and particles to Daphnia magna. Environmental Pollution 267, 115392.
| Crossref | Google Scholar | PubMed |