Natural genetic adaptation allows flexible reproductive behaviour: the case of wild carrot (Daucus carota L. subsp. carota) vs cultivated carrot (Daucus carota L. subsp. sativus)
Asharp Godwin
A
B
Abstract
Comparing the life cycles of wild and cultivated carrots is vital for identifying any overlapping flowering periods, as wild carrots have the potential to compromise the genetic purity of commercial carrot seeds via pollen flow. However, little information is known about how juvenility, vernalization, and their interactions impact the flowering pattern of wild and cultivated carrots in New Zealand.
We evaluated the influence of different juvenile phases, and vernalization phases on floral characteristics and flowering behaviour of cultivated and wild carrots.
The study was a factorial randomized complete block design with four blocks of five plants per block, incorporating treatments of different carrot genotypes (G1, cultivated; G2, wild carrots), juvenile phases (J1, 12 weeks; J2, 8 weeks; J3, 4 weeks), and vernalization phases (V1, 12 weeks; V2, 4 weeks; V3, no vernalization). Flowering percentage, flowering time, percentage of overwinter survival, and floral traits, including number of umbels and branches, and height of floral stem were recorded and analyzed by ANOVA.
Cultivated carrots flowered only when exposed to 12 weeks vernalization, while wild carrots have shown 100% flowering across all treatment combinations. Wild carrots exhibited a higher overwintering survival rate (94.9–100%) than cultivated carrots (66.1–98.3%). Prolonged exposure to vernalization significantly affected the floral traits of wild carrots.
There is a high likelihood of overlapping flowering periods between wild and cultivated carrots, as wild carrots can survive as both winter and summer annuals.
To avoid undesirable pollen flow during overlapping flowering periods of wild and cultivated carrots, we recommend timely weed management strategies to control wild carrots.
Keywords: Daucus species, floral induction, life cycle, New Zealand, temperature, vernalization, umbels, weed management.
Introduction
Invasive weed species are alien plants that pose serious threats to natural ecosystems and result in substantial economic losses in agriculture (Wang et al. 2024). Due to their unique evolutionary mechanisms, invasive weeds have shown rapid adaptation to various climate change scenarios, often outperforming native species (Sun et al. 2023). Factors such as altered moisture regimes, rising atmospheric temperatures, and extreme climate events affect all plants, but invasive weeds can manipulate their life cycles and migrate to new regions more efficiently (Rathee et al. 2023). Consequently, weed infestations are considered a single major limitation to crop production (Datta et al. 2021; Ziska 2022), sometimes leading to yield losses of up to 100% in extreme cases (Damalas and Koutroubas 2022).
Domesticated and cultivated crops have naturally evolved from their weedy wild relatives (Kashyap et al. 2022). Hence, the invasion of wild populations adjacent to their domesticated close relatives has been a concern because of the gene flow between wild and cultivated species, which compromises the genetic purity of the seeds produced from cultivated commercial cultivars (Renzi et al. 2021). When both the spatial distribution and flowering are occurring simultaneously in the field, the instances of pollen flow between cultivated species and their wild relatives may be very high (Yook et al. 2021). This issue has been broadly observed throughout the world in commercial carrot seed production where cultivated carrots (Daucus carota subsp. sativus) can readily hybridise with wild carrots (Daucus carota subsp. carota) since both are sexually compatible (Mandel and Brunet 2019).
Wild carrot is considered an invasive weed, causing a significant threat to commercial carrot seed production (Hauser and Bjørn 2001; Rome and Lucero 2019). Wild carrots are particularly common along road edges where it grows as a roadside plant. It is also found in waste areas, gardens, cultivated lands, and ungrazed or unmown paddocks (James et al. 2012; Rooney 2016). Under ideal environmental conditions, wild carrots can grow to a height of 1 m (Soltani et al. 2018). In addition, wild carrot may produce a vast quantity of flowers, approximately 14,000 per plant in the field, which indicates the importance of controlling the wild carrots before their flowering (Koul et al. 1989). Both wild and cultivated carrots attract a wide variety of possible pollinators such as leafcutting bees, honeybees, and various other wild bees (Davidson et al. 2010; Howlett et al. 2015; Palmieri et al. 2019). Furthermore, higher outcrossing incidences (around 96%) and long-lasting pollen viability (up to 10 days) of wild carrots result in a significant incidence of pollen flow to cultivated carrots (Umehara et al. 2005; Rong et al. 2010). Pollen flow (introgression) from cultivated to wild carrots can enhance the weediness and invasiveness of wild carrots. This occurs due to the escape of beneficial alleles from cultivated carrots to wild carrots, potentially increasing their adaptability and competitiveness (Hernández et al. 2023; Vercellino et al. 2023). Furthermore, pollen transmission from wild to cultivated carrots can reduce the genetic purity of commercial carrot seeds due to undesirable outcrossing (Yahyaa et al. 2018; Mandel and Brunet 2019). Therefore, it is important to understand the flowering pattern of wild and cultivated carrots to preserve quality carrot seed production.
The bolting and flowering of cultivated carrots are affected by several factors such as cardinal temperature, juvenility (juvenility refers to the developmental stage during which carrot plants are not sensitive to vernalization signals), and vernalization (exposure to cold necessary for flowering in some cultivars). However, these requirements differ from cultivar to cultivar (Atherton et al. 1990). Carrot seedlings are receptive to vernalization signals only after a minimal period of development known as the juvenile phase (Villeneuve and Latour 2017). When carrot plants have 8–12 leaves and storage roots that are greater than 4–8 mm in diameter, the juvenile period is considered completed (Atherton et al. 1990). Complex vernalization signaling networks have developed in carrots to detect variations in temperature (cold temperature, 5°C) and photoperiod (short-day condition), which in turn regulates flowering time (Wohlfeiler et al. 2022). Moreover, the length of vernalization required is determined by the growth and size of the plants attained during the juvenile phase, with more developed plants requiring a shorter period of vernalization (Samuolienė et al. 2008). During the transition from vegetative to reproductive phase via vernalization, the vegetative apical meristem converts into an elongated reproductive conical meristem, followed by the elongation of the inflorescence apex (Borthwick 1931).
Cultivated carrots can be classified as annuals and biennials depending on the vernalization requirement, which is a genetically and geographically controllable trait. Generally, annuals require a shorter vernalization period (5–30 days) than biennials (11–12 weeks) (Atherton et al. 1990; Dias Tagliacozzo and Valio 1994; Wohlfeiler et al. 2021). According to Alessandro et al. (2013), annuality in cultivated carrots is regulated by a single dominant gene known as vrn1. Although annuals and biennials have different vernalization phases, both cultivars have the same juvenile period (Alessandro and Galmarini 2007). There is little information published regarding the juvenility and vernalization requirement of wild carrots, despite numerous studies on these requirements related to cultivated carrots. Due to the wide genetic diversity of wild carrots (Rong et al. 2010), their length of juvenility might vary more than cultivated carrots depending on the geographic locality (Nemeth 1999). Moreover, wild carrots have low vernalization requirements, which is a key strategy for producing a large amount of seeds before extreme winter temperatures (Wohlfeiler et al. 2019). These observations indicate the importance of studying the life cycle of wild carrots via understanding the juvenility and vernalization requirements.
Wild carrot populations have shown a wide variety of life history behaviour such as annuals, biennials, and perennials (de Jong et al. 2016; Mandel and Brunet 2019). Furthermore, the geographical location and climate conditions are the key determinants of the wild carrot’s life cycle. This highlights the significance of researching wild carrot behaviour at the regional level (Wijnheijmer et al. 1989; Kumarasamy et al. 2005; Wohlfeiler et al. 2019). Furthermore, timely research of the life cycle of wild carrots is important for understanding plant adaptation and evolution under climate change. Also, studying the life cycle of wild carrots in a timely manner is crucial to comprehending how these invasive weeds evolve and adapt to changing climatic conditions. Meanwhile, the flowering synchrony of wild and cultivated carrots can be predicted when the life history of wild carrots is known at a particular climatic condition. This could help in avoiding undesirable hybridisation between wild and cultivated carrots through implementation of timely weed management strategies, such as early detection and removal, and managing wild carrots prior to the flowering to avoid synchronisation with the flowering of cultivated carrots (Hauser and Shim 2007). This emphasises the importance of studying the life cycle of wild carrots in the context of designing weed management techniques to reduce its spread (Van Etten and Brunet 2017).
New Zealand supplies around 50% of the world’s supply of carrot seeds, which is predominantly hybrid seed (Bhatia 2023; Preece 2023). Hybrid carrot seed production comprises the controlled crossing between the selected male and female lines to obtain seeds with desirable traits, such as high yield, resistance to pests and diseases, and homogeneity (Broussard et al. 2017). This hybridisation process mostly uses cytoplasmic male sterile (CMS) lines to avoid self-pollination in female plants, ensuring that only pollen from the selected male line contributes to their fertilisation (Simon 2021). Furthermore, pollinators, such as honey bees, hoverflies, nectar scarabs, muscoid flies, and European Blue Blow Flies facilitate the pollen transfer from male to female line (Howlett 2012; Gaffney et al. 2018). However, nearby wild carrot populations can act as competing pollen donors, compromising the purity of commercial seed through undesirable hybridisation (Broussard et al. 2017; Mandel and Brunet 2019). As a result, no wild carrot plants should be within sufficient proximity of commercial carrot seed production sites to avoid pollen transfer to the cultivated carrot crop (Godwin et al. 2024).
Moreover, there is a major research gap in studies related to the flowering behaviour of wild carrots in New Zealand. Furthermore, several investigations on the juvenility and vernalization responsiveness of cultivated carrots have been undertaken (Atherton et al. 1990; Wohlfeiler et al. 2021). However, no research has been done to study the interaction effect between different juvenility and vernalization of wild and cultivated carrots in New Zealand and internationally. Thus, this study’s objectives are to: (1) determine the interaction effects between juvenility and vernalization on flowering and floral characteristics of wild and cultivated carrots; and (2) investigate the life cycle and floral morphology of wild carrots in New Zealand. This study will help to understand how the flowering pattern of wild and cultivated carrots varies under different juvenile and vernalization requirements. This study also presents essential information to the policymakers, researchers, seed industrialists and seed-producing farmers for producing high-quality and quantity carrot seeds in New Zealand and other carrot seed-producing countries across the world via managing the wild carrots in a timely manner.
Materials and methods
Plant materials and study site
A pot experiment was conducted at the Plant Growth Unit (PGU), Massey University in Palmerston North, New Zealand (40°22′41.1″S, 175°36′49.3″E) for a calendar year starting from 26 July 2022 to investigate the flowering phenology of wild (Daucus carota L. subsp. carota) and cultivated carrots (Daucus carota L. subsp. sativus) in terms of the need for juvenility and vernalization requirements. The key reason for choosing male carrot lines and wild carrots for this study is that both can act as pollen donors to the female plants. Seeds of cultivated carrots (male lines No. 27) were obtained from South Pacific Seeds Ltd. Wild carrot seeds were collected from Dairy One, a farm at Massey University, Palmerston North, New Zealand (40°22′31.0″S, 175°36′21.3″E) in May 2022. Seeds were air-dried under ambient conditions for 5 days, and debris was removed from the seed lot. The male line seeds and sorted wild carrot seeds were sealed in separate plastic boxes and stored at 5°C and 50% relative humidity as recommended by Zhao et al. (2022).
Crop establishment and husbandry
Wild and cultivated carrot seedlings were established in PVC pipes (Marley OPTIM DWV Pipe SN6) with a 50-cm height and a 10-cm diameter, filled with potting mixture (Wohlfeiler et al. 2021). The potting mixture consisted of a 3:1 ratio of soil and sand, constituting 80% sand, 12% silt, and 8% clay (Yadav et al. 2021). Each pot was subsequently filled with 4 L of potting mixture and slow-release fertiliser ‘Osmocot Exact Standard’ at the rate of 4 g L−1. This fertiliser provides a consistent supply of nutrients (15N-3.9P-9.1K + 1.2 Mg + Trace elements) over an extended period (around 8–9 months). Garden nets were placed across the bottom of each pot to enhance the drainage of excess water after irrigation. The wild and cultivated carrot seeds were sown at a rate of three seeds per pot. Additional seedlings were sown separately and transferred as needed to pots at the three-unfolded true leaf stage to retain the plant density as one plant per pot (Wohlfeiler et al. 2022). Initially, manual irrigation was practised by using a spray lance with a seedling nozzle until seedling emergence. Thereafter, plants were irrigated at a rate of 4 mL day−1 per plant until they were moved to growth chambers. A watering can was used to irrigate the plants inside the growth chamber. Weekly manual weeding was carried out throughout the study period. Pesticides were sprayed in response to the pest and disease infestations, with no fixed frequency for spraying. Mit‘e mec (Milbemectin) and Mavrik (Tau-fluvalinate) were used to control the mite attack, and when infestation level (such as discolouration and curling leaves) required Attack (Pirimiphos-methyl; Permethrin) and Carbendazim were sprayed to manage thrips and fungal infestation, respectively.
Experimental design and treatment combinations
For the study, a Randomized Complete Block Design (RCBD) in a three-way factorial arrangement with four (n = 4) replications along with five plants (r = 5) in each replication was used. The first factor was genotype (G) with two levels (wild carrot and cultivated carrot). The second factor was the juvenile period (J) with three levels (12 weeks, 8 weeks, and 4 weeks). The third factor was vernalization (V) with three levels (12 weeks, 4 weeks, and no vernalization). Therefore, 18 treatment combinations (two levels of G × three levels of J × three levels of V) were generated for this experiment. Thus, 360 plants (18 treatment combinations × four replicates × five plants per replicate), comprising 180 wild and 180 cultivated types, were established in total. The schematic diagram of the experimental schedule and treatment combinations are in Fig. 1.
Schematic diagram of treatment combinations and experimental schedule. PV, post-vernalization.
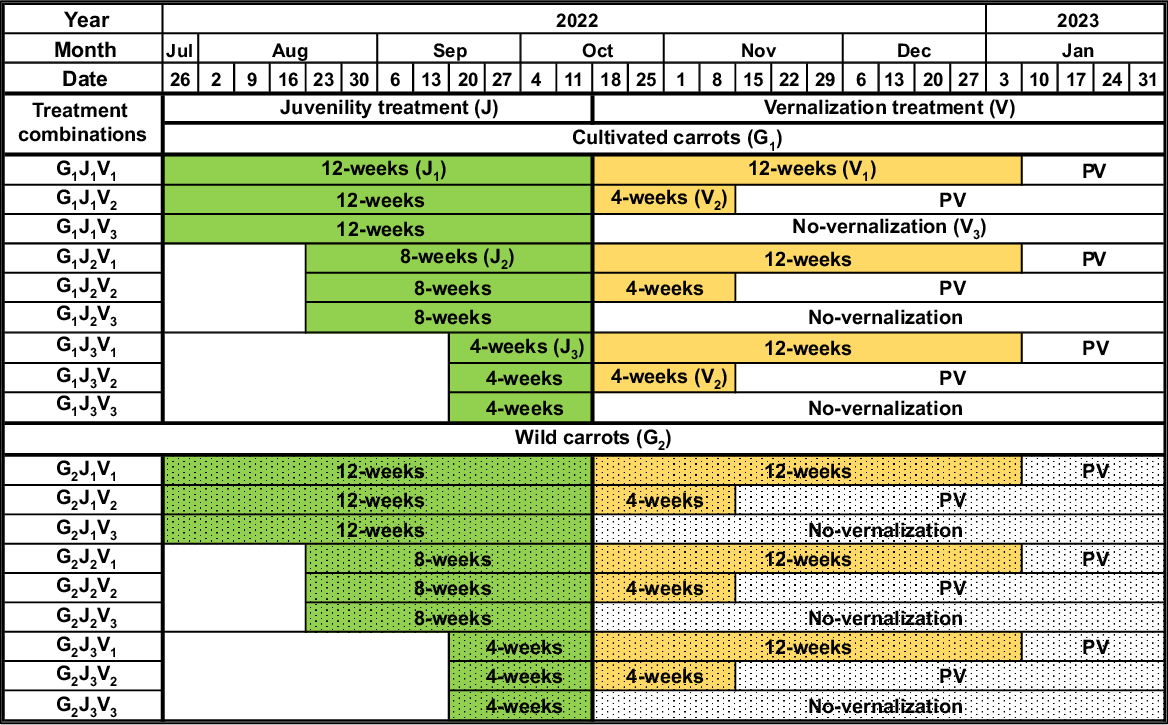
Generally, the initiation of flowering can be influenced by the age when plants are shifted to floral inductive conditions (juvenility) (Cave et al. 2011). In this study, the length of the juvenile phase was divided into three levels to determine how the age of both wild and cultivated carrots has influenced their flowering behaviour and phenology (Atherton et al. 1990). In order to achieve the 12-week (J1), 8-week (J2), and 4-week (J3) juvenile phases, wild and cultivated carrot seeds were sown in a glasshouse on 26 July, 23 August, and 20 September 2022, respectively (Fig. 1). The important reason for using a glasshouse in this experiment was to provide suitable controlled microclimate, including the temperature and photoperiod, for the growth and development of plants during their juvenile phase. The plants were established at an average temperature of 20.25°C in the glasshouse. Cool daylight fluorescent lights (Philips TLD 58w/865) were used in the glasshouse to provide the seedlings with a long-day photoperiod (16 h of light and 8 h of darkness) with the light intensities ranging from 140 to 150 μmol m−2 s−1 (Table 1).
Growth stages and treatments | Growth conditions (Wohlfeiler et al. 2022) | ||||||||
---|---|---|---|---|---|---|---|---|---|
Tmin (°C)A | Tmax (°C)A | Tavg (°C)A | CVt %B | PhotoperiodC | Light intensityD | Location | |||
Juvenility treatment | J1 | 14.00 | 28.5 | 20.26 | 4.84% | Long-day | 140–150 μmol m−2 s−1 | Glasshouse | |
J2 | |||||||||
J3 | |||||||||
Vernalization treatment | V1 | 5.13 | 12.11 | 6.00 | 25.23% | Short-day | Growth chamber | ||
V2 | 5.13 | 12.11 | 7.05 | 33.23% | |||||
V3 | 14.37 | 18.84 | 17.29 | 13.81% | Long-day | ||||
Post-vernalization | 13.15 | 19.13 | 17.90 | 14.51% | Long-day |
Vernalization is an important process in the induction of flowering in carrots, occurring after the juvenile phase (Liu et al. 2020). Therefore, the vernalization treatments (V1, V2, and V3) were undertaken from 19 October 2022 following the juvenility treatments (Fig. 1). Both the wild and cultivated plants from each juvenility treatment (J1, J2, and J3) were categorised into three different groups to implement the vernalization treatment (V1, V2, and V3). Thereafter, all the plants were transferred from the glasshouse to the growth chambers to provide different vernalization conditions for the plants. The plants belonging to the V1 and V2 treatments were maintained under low temperature (V1 = 6.00°C, mean temperature over 12 weeks; V2 = 7.05°C, mean temperature over 4 weeks) and short-day photoperiod (8 h light/16 h dark; light intensity 140–150 μmol m−2 s−1) condition (Table 1) for 12 weeks and 4 weeks, respectively, in cold growth chambers (Table 1). Following the completion of the required vernalization, plants belonging to V1 and V2 treatments were gradually exposed to post-vernalization conditions with a mean daily temperature of 17.90°C under long-day photoperiod (Table 1) from 10 January 2023 and 15 November 2022, respectively, until the end of the study. The plants belonging to V3 (no-vernalization, control treatment) were maintained at a mean temperature of 17.29°C under a long-day photoperiod, 16 h light (light intensity: 140 to 150 μmol m−2 s−1), and 8 h dark in separate growth chambers for the whole trial from 19 October 2022.
Sampling and measurements
Data on flowering percentage, days taken to flowering, overwinter survival percentage, height of floral stalk at flowering, total number of branches, and total number of umbels were collected in the glasshouse and growth chambers.
As suggested by Alessandro and Galmarini (2007), the elongation of the first floral stem internode was used as an indicator to determine the flowered plants. Flowering was recorded on a daily basis throughout the trial. The percentage of flowered plants was calculated by Eqn 1 and the flowering time from seed sowing was calculated. The survival percentage of plants during the vernalization treatment was calculated by using Eqn 2. When the plants reached their end stage of flowering, the parameters such as the maximum height of floral stem (from base to tip of the primary umbel), and the total number of branches were recorded for all plants (Noor et al. 2020). Moreover, the number of umbels per plant was recorded throughout the study to determine the total number of umbels at the end of the study. Plants without initiation of floral stem during the study, in the juvenile, vernalization, and post-vernalization phases, were identified as non-flowering. Consequently, there were no data related to floral traits gathered from non-flowering plants during the study period.
Statistical analysis
The three-way Analysis of variance (ANOVA) was conducted with RStudio (ver. 2022.07.2 + 576) to assess the effects of the genotype (G), juvenility (J), vernalization (V), and their interactions ((G × J), (G × V), and (G × J × V)) on collected parameters. Tukey’s post hoc tests were carried out at a 5% significance level (P < 0.05) to determine differences among the means for the various treatments considered.
Results and discussion
Flowering responses of wild and cultivated carrots to juvenility and vernalization period
The timing and success of flowering in D. carota species, including both cultivated and wild carrots, are substantially influenced by juvenility and vernalization requirements (Wohlfeiler et al. 2022). The interaction effect between genotype, juvenile period, and vernalization period was significant (P < 0.05) for the flowering percentage and time taken for flowering from seed sowing (Fig. 2).
(a) Flowering percentage and (b) time taken for flowering of wild and cultivated carrots under different vernalization and juvenile period treatments. Data presented are means. Means with different lowercase letters are significantly different at P < 0.05 (Tukey’s post hoc tests). G, J, and V represents the different genotype, juvenility periods, and vernalization periods, respectively.
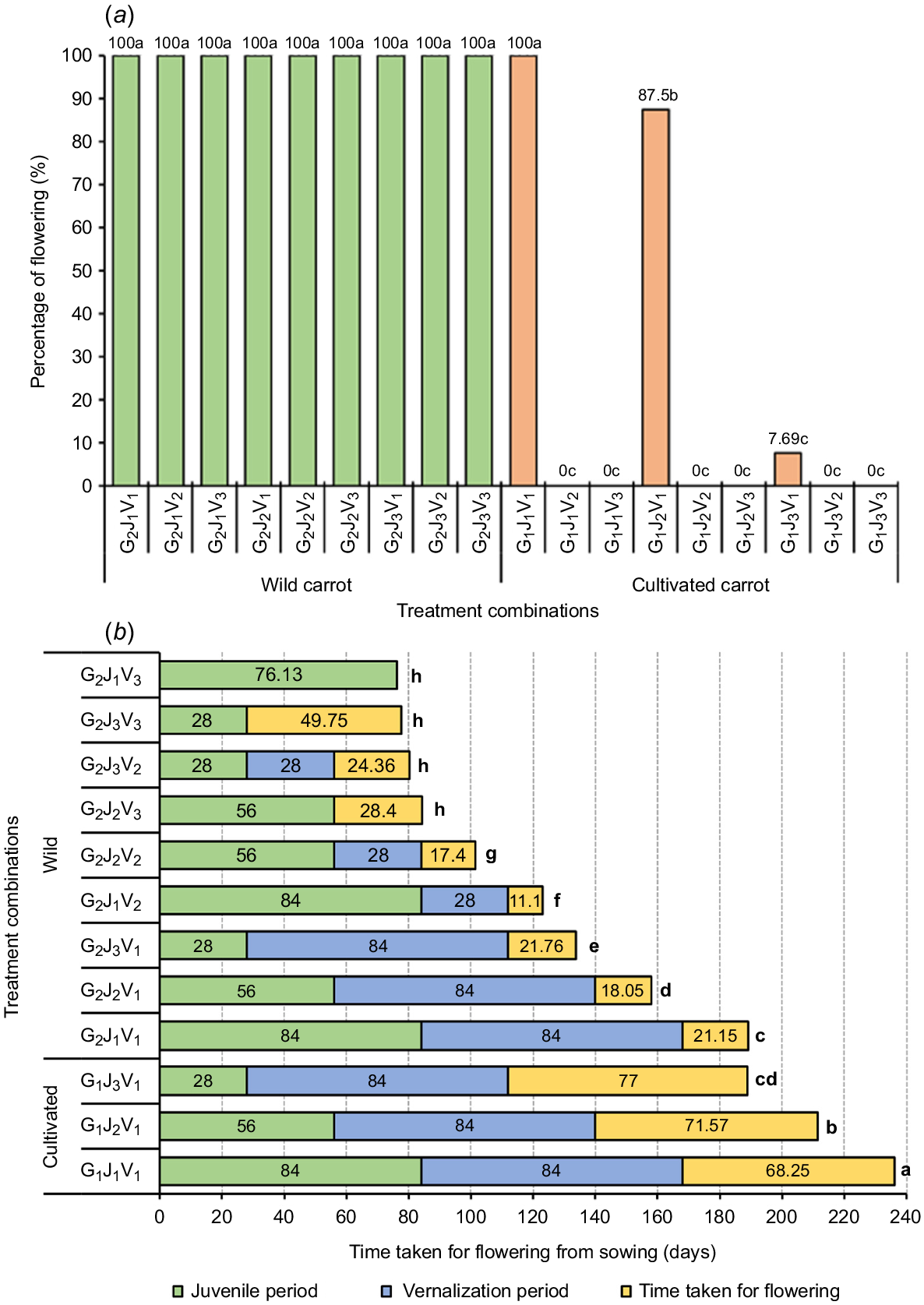
Flowering percentage is an important indicator of a carrot’s reproductive capacity and broader adaptability to various environmental conditions (Loarca et al. 2024). In our study, the flowering percentage varied significantly (P < 0.05) among the genotype groups, juvenile, and vernalization treatments and all their interactions. For cultivated carrot, no plants flowered in the treatments V2 (4 weeks of vernalization) and V3 (no vernalization). Furthermore, cultivated carrots that were exposed to 12 weeks of vernalization treatment (V1) at 12 weeks (G1J1V1), 8 weeks (G1J2V1), and 4 weeks (G1J3V1) of juvenile phase showed 100, 87.5, and 7.69% of flowering, respectively (Fig. 2a). However, the 4-week juvenile treatment that result in a lower flowering percentage (7.69%) cannot be considered in the context of commercial carrot seed production. As the juvenile age of cultivated carrots extended from 4 weeks to 12 weeks, the flowering percentage increased by around 92% due to an increased response. This clearly suggests that the vernalization response is enhanced with the increasing juvenile age of cultivated carrots, achieving its peak at 12 weeks of plant age. These findings were further supported by Atherton et al. (1990), who stated that the cultivated carrots (cv. Chantenay Red Cored) younger than 6 weeks did not respond to vernalization; for plants aged up to 10 weeks, there was an increase in vernalization response and all of the plants flowered due to the vernalization stimuli.
In addition, the findings from this study rationalise why cultivated carrots grown in New Zealand are biennial or late-flowering type, requiring around 12 weeks of vernalization at 5°C to induce flowering (Alessandro and Galmarini 2007). Similar findings were observed by Wohlfeiler et al. (2021), who found that the biennial carrots required lengthy cold exposure (11–12 weeks) compared with annuals (1–4 weeks) in order to induce flowering. Furthermore, bienniality is a domestication trait, which allow carrot plants to accumulate biomass in their roots. This trait enables the plants to store energy in their roots during the first year, which they then use to flower and produce seeds in the second year (Coe et al. 2023). The response to vernalization mainly relies on a range of genetic and environmental factors, as evidenced by different flowering responses of wild and cultivated genotypes of carrots to various chilling periods (12 weeks, 4 weeks, and no vernalization). Moreover, Wohlfeiler et al. (2019) indicated that the Vrn-A and Vrn-B genes are important regulators in controlling the transition from vegetative stage to flowering in response to vernalization in carrot genotypes.
Despite different juvenile and vernalization treatments, wild carrots (G2) flowered 100% in all the treatment combinations (Fig. 2a). Furthermore, it is clear that the wild carrots in New Zealand have the ability to flower without vernalization (G2J1V3, G2J2V3, and G2J3V3) emphasising the annual behaviour of wild carrots (Wohlfeiler et al. 2019). When the wild carrots entered (G2J1V1, G2J2V1, G2J3V1, G2J1V2, G2J2V2, and G2J3V2) into the overwintering phase (vernalization) as rosettes, they flower and set seed during the post-vernalization stage. This finding was further validated by Mandel and Brunet (2019), who noted that the wild carrots that survived the winter flowered during the following summer. Based on this, it is clear that the age of the plant when entering vernalization influences its life cycle, with the earlier establishment of wild carrots, especially prior to the winter, leading to a strong possibility of annual growth behaviour (Geoffriau et al. 2019).
Understanding the flowering pattern and flowering time of wild and cultivated carrots is important in investigating the phenology of both subspecies of Daucus carota since flowering is one of the key growth stages that are closely related to the overall life cycle (Loarca et al. 2024). Our results showed that flowering time varied significantly (P < 0.05) among treatments. The longest time to flowering was recorded as ~236 days for the G1J1V1 treatment combination (Fig. 2b), which is the standard practice (12 weeks for juvenile phase and 12 weeks for vernalization) followed by most of the commercial carrot seed producers in New Zealand. Furthermore, wild carrots with a long juvenile phase but no vernalization (G2J1V3) were the fastest to flower (~76 days) followed by wild carrots with, in addition, a short juvenile phase (G2J3V3, with approximately ~78 days). The findings clearly indicated that the wild carrots require less time than the cultivated carrots for flowering, both in vernalization and in juvenile phases. This might be mainly due to the different life history strategies of wild and cultivated carrots in New Zealand, where the wild and cultivated carrots exhibit annual and biennial behaviour, respectively. The different responses in life history strategies of wild and cultivated carrots are genetically controlled, specifically genes vrn-A and vrn-B, which regulate the vernalization requirement, and environmental factors, such as latitude and habitat’s resource quality (Mandel and Brunet 2019; Wohlfeiler et al. 2019). However, when wild carrots are exposed to vernalization conditions for long periods (chilling the plants for 12 weeks at 5°C), the floral induction is halted and only resumes after the completion of vernalization. As a result, flowering time is prolonged in some of the treatment combinations, such as G2J1V1 (~189 days), G2J2V1 (~158 days), and G2J3V1 (~133 days) compared with wild carrots without vernalization treatment, such as G2J1V3 (~76 days), G2J2V3 (~84 days), and G2J3V3 (~77 days) (Fig. 2b). The rosette of wild carrots presents in treatment combinations G2J1V1, G2J2V1 and G2J3V1 flowered post-winter conditions (vernalization), which indicated their behaviour as a winter annual. Conversely, wild carrots also flowered in the non-vernalized treatments such as G2J1V3, G2J2V3, and G2J3V3 indicating under some environmental conditions wild carrots can behave as a summer annual. In short, wild carrots in New Zealand can be classified as winter annuals (seed germinates in autumn, rosette survives in winter, and flowering occurs in summer of the first year) and summer annuals (seed germinate in spring and flowering occurs in summer of the first year) (Mandel and Brunet 2019). Similar findings were reported by de Jong et al. (2016), who revealed that most of the wild carrots in the Netherlands have exhibited winter annual (38.9%) and summer annual (36.8%) behaviour. The winter or summer annual life-histories may be due to genetic differences of phenotypic plasticity. When the vegetative phase of wild carrots overlaps with winter, reproductive growth can be prolonged due to undesirable climatic conditions for flowering, resulting in the establishment of a winter annual. In contrast, if flowering happens in spring, it leads to the formation of a summer annual (Mandel and Brunet 2019).
Fig. 3 illustrates the relationship between the flowering percentage and the time taken for flowering from sowing. For cultivated carrots belonging to the treatment combination G1J3V1, only 7.69% of the cultivated carrots flowered at 189 days after the seed sowing (or at 77 days after the vernalization treatment), when exposed to cold temperature for 12 weeks (Fig. 3a). Furthermore, wild carrots had the highest flowering percentage (100%) in all the treatment combinations studied, with the time taken for flowering increasing with the length of juvenile and vernalization treatment. For instance, wild carrots that did not receive vernalization reached 100% flowering at around 90 days, whereas wild carrots that received 12 weeks of vernalization achieved 100% flowering between 150 and 230 days from seed sowing (Fig. 3b).
Relationship between percentage of flowering and time taken for flowering from sowing in genotypes of (a) cultivated and (b) wild carrots with different vernalization (12 weeks, 4 weeks and 0 weeks) and different juvenile periods (4 weeks, 8 weeks, and 12 weeks), G, J, and V represent the different genotype, juvenility periods, and vernalization periods, respectively.
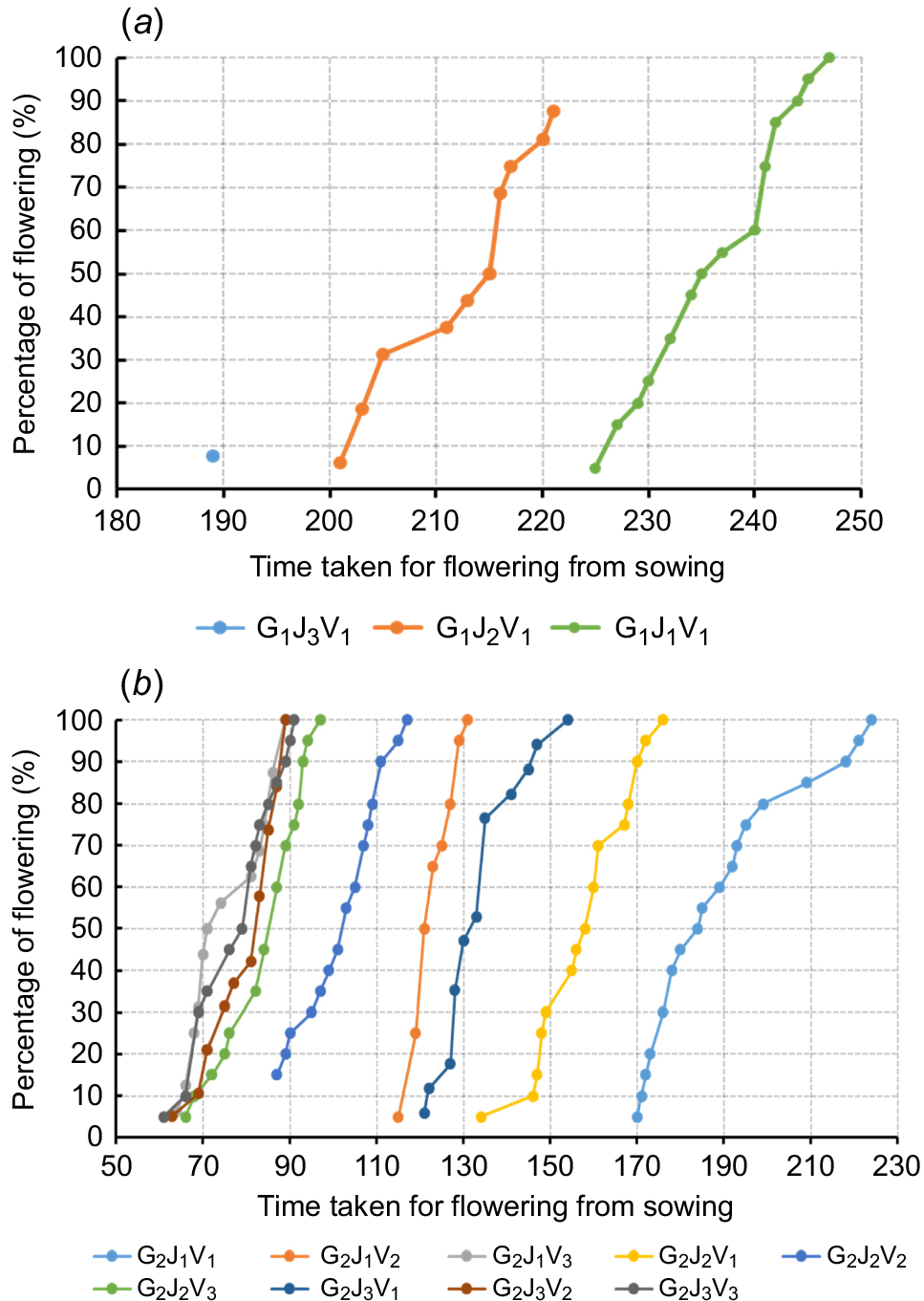
From the findings of this study, it is clear there is a high probability of overlapping flowering periods of wild and cultivated carrots in New Zealand. Since the wild carrots in New Zealand can behave as winter annuals and summer annuals, the flowering phase of cultivated carrots is likely to coincide with the flowering period of wild carrots. Consequently, there could be a significant threat of contamination of commercial carrot seeds via pollen flow, when the wild carrots are established adjacent to the carrot seed crops (Godwin et al. 2024). Given the diverse flowering pattern of wild carrots, it is critical to manage the wild carrots prior to flowering to avoid undesirable pollen flows and ensure the production of genetically pure carrot seeds (Rome and Lucero 2019).
Survival of wild and cultivated carrots during vernalization treatments
Controlling wild carrots prior to the overwintering stage is important, as they start to flower and proliferate, making them increasingly problematic (Van Etten and Brunet 2017). Therefore, understanding the effect of different juvenile phases and vernalization conditions on the survival of wild and cultivated carrots is key to assessing their ability to survive over the winter and formulating weed management strategies based on their survival nature. A significant interaction effect between genotype and juvenility period (P < 0.05) was detected in relation to survival percentage during vernalization (overwinter survival). Comparatively, wild carrots demonstrated a higher rate (94.9–100%) of overwinter survival than cultivated carrots (66.1–98.3%) in New Zealand (Fig. 4). This implies that wild carrots are better adapted to winter conditions. According to the results, 12-week-old wild and cultivated carrot plants had higher winter survival rates, compared with 4-week-old seedlings by 5.1% (wild) and 32.2% (cultivated), respectively (Fig. 4). Furthermore, the results of this study indicate that the winter survival percentage of wild carrots was higher when the plant’s juvenile age was higher at the time of transitioning into the overwintering phase. This implies that older plants (12 weeks old) are more resilient to winter conditions than younger plants (4 weeks old). This could be due to the underdeveloped physiological parts and root systems of younger plants compared with older plants (Lubbe et al. 2021). Moreover, winter temperatures will probably rise with global warming, which could speed up the spread of wild carrots due to their strong survival nature over the winter (Van Etten and Brunet 2017). As a consequence of climate change, winters are becoming gradually warmer in New Zealand (Ministry for the Environment, Stats NZ 2020). As a result of higher overwinter survival rates and the favourable conditions caused by warmer winters the threat wild carrots pose for carrot seed production, in New Zealand in the future, will increase.
Floral characteristics of wild and cultivated carrots
Identifying the floral characteristics of wild and cultivated (especially male lines) is important for understanding how these traits influence competitive advantages in regards to the attraction of pollinators since the wild and cultivated carrots have the capacity to attract the same pollinators. Understanding these floral traits helps in assessing the potential for cross-pollination, which is vital for maintaining the genetic purity of commercial carrot seeds. Additionally, the differences in floral traits can provide insights into the reproductive strategies and ecological adaptability of wild carrots, which are essential for developing effective weed management practices (Lamborn and Ollerton 2000; Howlett et al. 2015; Mandel and Brunet 2019). The findings related to number of umbels, height of floral stalk, and number of branches are presented in Fig. 5.
(a) Number of umbels, (b) height of floral stalk, and (c) number of branches of wild and cultivated carrots under different treatment combinations. Data presented are means. Means with different lowercase and uppercase letters are significantly different at P < 0.05 (Tukey’s post hoc tests).
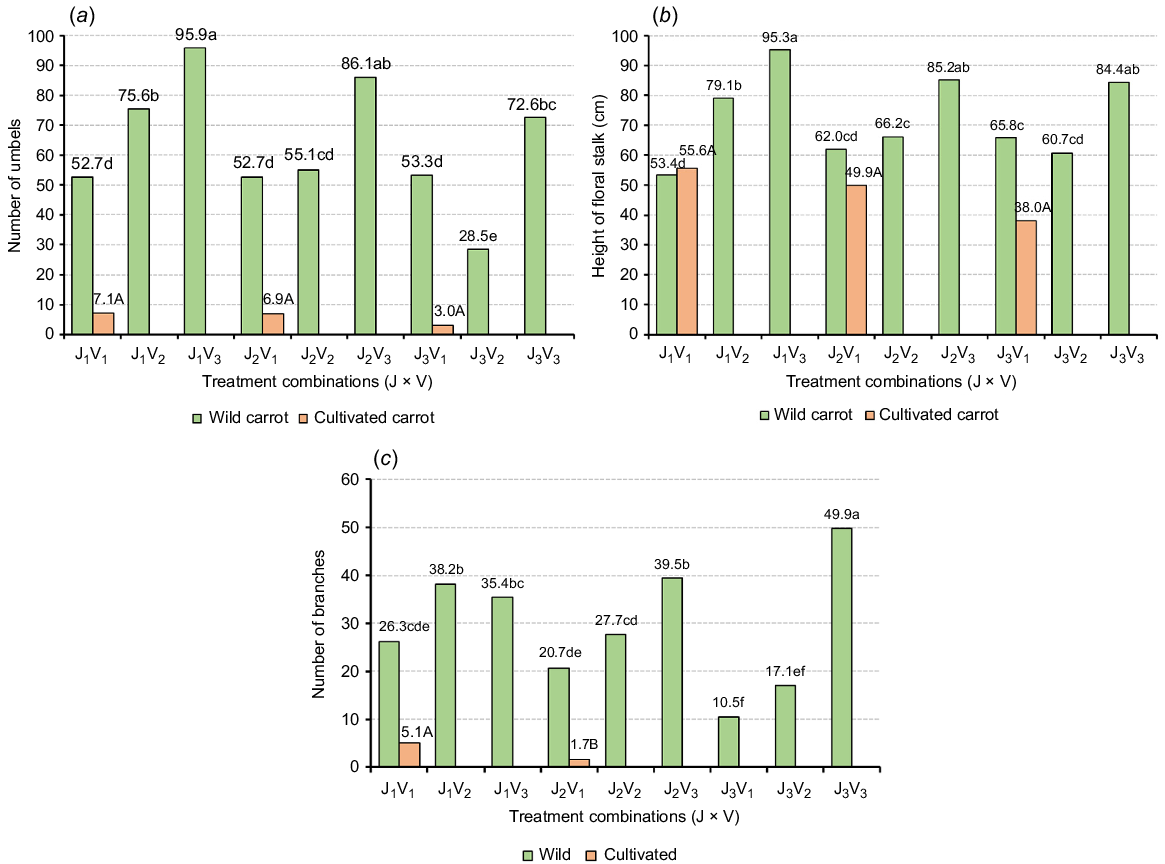
The interaction effect between juvenility and vernalization was significant (P < 0.05) for all the floral traits, including number of umbels, height of floral stalk, and number of branches. Therefore, the floral traits of wild and cultivated carrots were assessed separately with genotype-specific Tukey’s tests represented by upper (cultivated) and lower case (wild) letters in Fig. 5. The findings indicated that the wild carrots not exposed to vernalization (V3) produced taller floral stalks and more umbels and branches than wild carrots grown under other treatment combinations (juvenility × vernalization) except for the number of branches produced under J1V3. Moreover, increasing the length of cold exposure from 4 to 12 weeks significantly (P < 0.05) affected the floral traits of wild carrots that were exposed to vernalization at the age of 12 weeks (J1) and 8 weeks (J2) by reducing the number of umbels, height of the floral stalk, and number of branches. This indicates that prolonged cold exposure might affect the reproductive growth of wild carrots. Based on these observations, as temperatures rise wild carrots showing summer annual behaviour may become more problematic than those with winter annual behaviour in the context of weed management and attraction of pollinators in New Zealand. Comparatively, wild carrots have produced a higher number of umbels and branches, and taller floral stalks than male lines of cultivated carrots (except floral stalk height in J1V1, which can stimulate the attraction of pollinators (Fig. 5). Similar findings were reported by Koul et al. (1989), who noted that the wild carrots produced more umbel orders and flowers than cultivated carrots. In general, higher umbel counts attract pollinators to forage plants more frequently due to the increased floral density (Thomson 1988). In addition, Gonzalez et al. (2018) observed that the height of floral stalk (umbel height) of wild carrots has a significant influence on the attractiveness of the pollinators. Similar findings were reported by Fornoff et al. (2017), who stated that flower height is positively correlated with the diversity of species and frequency of insect visitations.
Conclusion
The duration of the juvenile stage, vernalization, and their interactions had a significant effect on the flowering responses of wild and cultivated carrots. In regard to commercial carrot seed production in New Zealand, the requirement of 12 weeks for vernalization emphasises the biennial nature of the plant. In contrast, wild carrots can flower all conditions, posing challenges due to their adaptability and higher overwintering survival rates. This flexibility allows wild carrots to thrive as winter or summer annuals, threatening the genetic purity of commercial seeds. In addition, the projected effect of climate change, especially warmer winters, will further facilitate the overwinter survival of wild carrots in New Zealand, necessitating more aggressive and timely weed management strategies. Meanwhile, wild carrots have a more competitive advantage over male lines of cultivated carrots in attracting and rewarding pollinators due to the development of more umbels, branches and taller floral stalks. In the context of commercial carrot seed production, to prevent overlapping flowering periods between wild and cultivated carrots, wild carrots must be controlled immediately after the winter. The findings of this study significantly enhance the understanding of the life history strategies of wild and cultivated carrots. These insights are important for designing timely weed management practices to ensure the production of genetically pure commercial carrot seeds in New Zealand and other seed-producing regions.
Conflicts of interest
The authors declare that they have no known competing financial interests or personal relationships that could have appeared to influence the work reported in this paper.
Declaration of funding
The authors express their appreciation to the Accelerating Higher Education Expansion and Development (AHEAD) Project (launched by the Sri Lankan Government under the World Bank fund), Seed Industry Research Centre (SIRC), AsureQuality Ltd., and School of Agriculture and Environment, Massey University, New Zealand for scholarship and research grant.
Acknowledgement
The authors thank South Pacific Seeds New Zealand Ltd. and Richard van Garderen who provided commercial carrot seeds for this study. We also thank the technical staff at the Plant Growth Unit, Massey University for their support with the project. Finally, we thank reviewers for their valuable time and constructive feedback on improving the quality of the article.
References
Alessandro MS, Galmarini CR (2007) Inheritance of vernalization requirement in carrot. Journal of the American Society for Horticultural Science 132(4), 525-529.
| Crossref | Google Scholar |
Alessandro MS, Galmarini CR, Iorizzo M, Simon PW (2013) Molecular mapping of vernalization requirement and fertility restoration genes in carrot. Theoretical and Applied Genetics 126, 415-423.
| Crossref | Google Scholar |
Atherton JG, Craigon J, Basher EA (1990) Flowering and bolting in carrot. I. Juvenility, cardinal temperatures and thermal times for vernalization. Journal of Horticultural Science 65(4), 423-429.
| Crossref | Google Scholar |
Bhatia SS (2023) Hyperspectral remote sensing for early detection of wild carrot in Carrot (Daucus carota) seed production – a feasibility study. Master of Science Thesis, Massey University, Manawatū, New Zealand. Available at http://hdl.handle.net/10179/20061
Borthwick HA (1931) Development of the macrogametophyte and embryo of Daucus carota. Botanical Gazette 92(1), 23-44.
| Crossref | Google Scholar |
Broussard MA, Mas F, Howlett B, Pattemore D, Tylianakis JM (2017) Possible mechanisms of pollination failure in hybrid carrot seed and implications for industry in a changing climate. PLoS ONE 12(6), e0180215.
| Crossref | Google Scholar |
Cave RL, Birch CJ, Hammer GL, Erwin JE, Johnston ME (2011) Juvenility and flowering of Brunonia australis (Goodeniaceae) and Calandrinia sp. (Portulacaceae) in relation to vernalization and daylength. Annals of Botany 108(1), 215-220.
| Crossref | Google Scholar |
Coe K, Bostan H, Rolling W, Turner-Hissong S, Macko-Podgórni A, Senalik D, Liu S, Seth R, Curaba J, Mengist MF, Grzebelus D, Van Deynze A, Dawson J, Ellison S, Simon P, Iorizzo M (2023) Population genomics identifies genetic signatures of carrot domestication and improvement and uncovers the origin of high-carotenoid orange carrots. Nature Plants 9(10), 1643-1658.
| Crossref | Google Scholar | PubMed |
Damalas CA, Koutroubas SD (2022) Weed competition effects on growth and yield of spring-sown white lupine. Horticulturae 8(5), 430.
| Crossref | Google Scholar |
Datta A, Maharaj S, Prabhu GN, Bhowmik D, Marino A, Akbari V, Rupavatharam S, Sujeetha JARP, Anantrao GG, Poduvattil VK, Kumar S, Kleczkowski A (2021) Monitoring the spread of water hyacinth (Pontederia crassipes): challenges and future developments. Frontiers in Ecology and Evolution 9, 631338.
| Crossref | Google Scholar |
Davidson MM, Butler RC, Howlett BG (2010) Apis mellifera and Megachile rotundata: a comparison of behaviour and seed yield in a hybrid carrot seed crop. New Zealand Journal of Crop and Horticultural Science 38(2), 113-117.
| Crossref | Google Scholar |
de Jong TJ, Grebenstein C, Tamis WLM (2016) Demography and life history of Daucus carota L. populations in the Netherlands. Flora 224, 154-158.
| Crossref | Google Scholar |
Dias Tagliacozzo GM, Valio IFM (1994) Effect of vernalization on flowering of Daucus carota (Cvs Nantes and Brasilia). Revista Brasileira de Fisiologia Vegetal 6(1), 71-73.
| Google Scholar |
Fornoff F, Klein A-M, Hartig F, Benadi G, Venjakob C, Schaefer HM, Ebeling A (2017) Functional flower traits and their diversity drive pollinator visitation. Oikos 126(7), 1020-1030.
| Crossref | Google Scholar |
Gaffney A, Bohman B, Quarrell SR, Brown PH, Allen GR (2018) Frequent insect visitors are not always pollen carriers in hybrid carrot pollination. Insects 9(2), 61.
| Crossref | Google Scholar |
Geoffriau E, Charpentier T, Huet S, Hägnefelt A, Lopes V, Nothnagel T, Lohwasser U, Mallor Gimenez C, Allender C (2019) CarrotDiverse: understanding variation in a wild relative of carrot. Acta Horticulturae 1264, 151-156.
| Crossref | Google Scholar |
Godwin A, Pieralli S, Sofkova-Bobcheva S, Ward A, McGill C (2024) Pollen-mediated gene flow from wild carrots (Daucus carota L. subsp. carota) affects the production of commercial carrot seeds (Daucus carota L. subsp. sativus) internationally and in New Zealand in the context of climate change: a systematic review. Science of The Total Environment 933, 173269.
| Crossref | Google Scholar |
Gonzalez VH, Cruz P, Folks N, Anderson S, Travis D, Hranitz J, Barthell J (2018) Attractiveness of the dark central floret in wild carrots: do umbel size and height matter? Journal of Pollination Ecology 23, 98-101.
| Crossref | Google Scholar |
Hauser TP, Bjørn GK (2001) Hybrids between wild and cultivated carrots in Danish carrotfields. Genetic Resources and Crop Evolution 48(5), 499-506.
| Crossref | Google Scholar |
Hauser TP, Shim SI (2007) Survival and flowering of hybrids between cultivated and wild carrots (Daucus carota) in Danish grasslands. Environmental Biosafety Research 6(4), 237-247.
| Crossref | Google Scholar |
Hernández F, Palmieri L, Brunet J (2023) Introgression and persistence of cultivar alleles in wild carrot (Daucus carota) populations in the United States. American Journal of Botany 110(11), e16242.
| Crossref | Google Scholar |
Howlett BG (2012) Hybrid carrot seed crop pollination by the fly Calliphora vicina (Diptera: Calliphoridae). Journal of Applied Entomology 136(6), 421-430.
| Crossref | Google Scholar |
Howlett BG, Lankin-Vega GO, Pattemore DE (2015) Native and introduced bee abundances on carrot seed crops in New Zealand. New Zealand Plant Protection 68, 373-379.
| Crossref | Google Scholar |
Kashyap A, Garg P, Tanwar K, Sharma J, Gupta NC, Ha PTT, Bhattacharya RC, Mason AS, Rao M (2022) Strategies for utilization of crop wild relatives in plant breeding programs. Theoretical and Applied Genetics 135(12), 4151-4167.
| Crossref | Google Scholar |
Koul P, Koul AK, Hamal IA (1989) Reproductive biology of wild and cultivated carrot (Daucus carota L.). New Phytologist 112(3), 437-443.
| Crossref | Google Scholar |
Kumarasamy Y, Nahar L, Byres M, Delazar A, Sarker SD (2005) The assessment of biological activities associated with the major constituents of the methanol extract of ‘wild carrot’ (Daucus carota L.) seeds. Journal of Herbal Pharmacotherapy 5(1), 61-72.
| Crossref | Google Scholar |
Lamborn E, Ollerton J (2000) Experimental assessment of the functional morphology of inflorescences of Daucus carota (Apiaceae): testing the ‘fly catcher effect’. Functional Ecology [4]14 [4] 445-454.
| Crossref | Google Scholar |
Liu L, Ou C, Chen S, Shen Q, Liu B, Li M, Zhao Z, Kong X, Yan X, Zhuang F (2020) The response of COL and FT homologues to photoperiodic regulation in carrot (Daucus carota L.). Scientific Reports 10(1), 9984.
| Crossref | Google Scholar |
Loarca J, Liou M, Dawson JC, Simon PW (2024) Advancing utilization of diverse global carrot (Daucus carota L.) germplasm with flowering habit trait ontology. Frontiers in Plant Science 15, 1342513.
| Crossref | Google Scholar |
Lubbe FC, Klimešová J, Henry HAL (2021) Winter belowground: changing winters and the perennating organs of herbaceous plants. Functional Ecology 35(8), 1627-1639.
| Crossref | Google Scholar |
Mandel JR, Brunet J (2019) Gene Flow in Carrot. In ‘The carrot genome. compendium of plant genomes’. (Eds P Simon, M Iorizzo, D Grzebelus, R Baranski) (Springer: Cham, Switzerland) 10.1007/978-3-030-03389-7_4
Ministry for the Environment, Stats NZ (2020) Our atmosphere and climate 2020, New Zealand’s environmental reporting series. Ministry for the Environment and Stats NZ. Available at https://www.mfe.govt.nz
Nemeth E (1999) ‘Caraway: the genus Carum.’ (CRC Press) 10.1201/9780203303672
Noor A, Ziaf K, Amjad M, Ahmad I (2020) Synthetic auxins concentration and application time modulates seed yield and quality of carrot by altering the umbel order. Scientia Horticulturae 262, 109066.
| Crossref | Google Scholar |
Palmieri L, Ellison SL, Senalik D, Simon PW, Brunet J (2019) Genetic markers to detect introgression of cultivar genes in wild carrot populations. Acta Horticulturae 1264, 165-174.
| Crossref | Google Scholar |
Preece D (2023) NZ’s world-leading seed industry just keeps growing. 1News. Available at https://www.1news.co.nz/2023/01/15/nzs-world-leading-seed-industry-just-keeps-growing/
Rathee S, Ahmad M, Sharma P, Batish DR, Singh HP (2023) Invasive plants in india: their adaptability, impact, and response to changing climate. In ‘Plant invasions and global climate change’. (Eds S Tripathi, R Bhadouria, P Srivastava, R Singh, DR Batish) pp. 173–198. (Springer Nature: Singapore) 10.1007/978-981-99-5910-5_8
Renzi JP, Reinoso O, Quintana M, Smýkal P (2021) spontaneous gene flow between cultivated and naturalized Vicia villosa roth populations increases the physical dormancy seed in a semiarid agroecosystem. Agronomy 11(5), 955.
| Crossref | Google Scholar |
Rome C, Lucero C (2019) Wild carrot (Daucus carota) management in the dungeness valley, Washington, United States: the power of citizen scientists to leverage policy change. Citizen Science: Theory and Practice 4(1), 36.
| Crossref | Google Scholar |
Rong J, Janson S, Umehara M, Ono M, Vrieling K (2010) Historical and contemporary gene dispersal in wild carrot (Daucus carota ssp. carota) populations. Annals of Botany 106(2), 285-296.
| Crossref | Google Scholar |
Rooney D (2016) Foster those weeds for a little help from your friends. New Zealand Garden Journal 19(2), 5-9.
| Google Scholar |
Samuolienė G, Urbonavičiūtė A, Šabajevienė G, Duchovskis P (2008) Flowering initiation in carrot and caraway. Sodininkystė Ir Daržininkystė 27(2), 17-25.
| Google Scholar |
Simon PW (2021) Carrot (Daucus carota L.) breeding. In ‘Advances in plant breeding strategies: vegetable crops’. (Eds JM Al-Khayri, SM Jain, DV Johnson) pp. 213–238. (Springer International Publishing) 10.1007/978-3-030-66965-2_5
Soltani N, Shropshire C, Sikkema PH (2018) Control of wild carrot (Daucus carota L.) in corn, soybean, and winter wheat. Canadian Journal of Plant Science 98(2), 425-431.
| Crossref | Google Scholar |
Sun J, Khattak WA, Abbas A, Nawaz M, Hameed R, Javed Q, Bo Y, Khan KA, Du D (2023) Ecological adaptability of invasive weeds under environmental pollutants: a review. Environmental and Experimental Botany 215, 105492.
| Crossref | Google Scholar |
Thomson JD (1988) Effects of variation in inflorescence size and floral rewards on the visitation rates of traplining pollinators of Aralia hispida. Evolutionary Ecology 2(1), 65-76.
| Crossref | Google Scholar |
Umehara M, Eguchi I, Kaneko D, Ono M, Kamada H (2005) Evaluation of gene flow and its environmental effects in the field. Plant Biotechnology 22(5), 497-504.
| Crossref | Google Scholar |
Van Etten ML, Brunet J (2017) Using population matrix models to reduce the spread of wild carrot. International Symposium on Carrot and Other Apiaceae 1153, 273-278.
| Crossref | Google Scholar |
Vercellino RB, Hernández F, Pandolfo C, Ureta S, Presotto A (2023) Agricultural weeds: the contribution of domesticated species to the origin and evolution of feral weeds. Pest Management Science 79(3), 922-934.
| Crossref | Google Scholar | PubMed |
Villeneuve F, Latour F (2017) Influence of sowing time and chilling exposure on flower induction in carrot (Daucus carota L.). Acta Horticulturae 1153, 47-54.
| Crossref | Google Scholar |
Wang K, Jin M, Li J, Ren Y, Li Z, Ren X, Huang C, Wan F, Qian W, Liu B (2024) The evolution and diurnal expression patterns of photosynthetic pathway genes of the invasive alien weed, Mikania micrantha. Journal of Integrative Agriculture 23(2), 590-604.
| Crossref | Google Scholar |
Wijnheijmer EHM, Brandenburg WA, Ter Borg SJ (1989) Interactions between wild and cultivated carrots (Daucus carota L.) in the Netherlands. Euphytica 40(1), 147-154.
| Crossref | Google Scholar |
Wohlfeiler J, Alessandro MS, Cavagnaro PF, Galmarini CR (2019) Multiallelic digenic control of vernalization requirement in carrot (Daucus carota L.). Euphytica 215, 37.
| Crossref | Google Scholar |
Wohlfeiler J, Alessandro MS, Cavagnaro PF, Galmarini CR (2021) Gradient of vernalization requirement in carrot cultivars from diverse geographical origins. Crop Science 61(5), 3373-3381.
| Crossref | Google Scholar |
Wohlfeiler J, Alessandro MS, Morales A, Cavagnaro PF, Galmarini CR (2022) Vernalization requirement, but not post-vernalization day length, conditions flowering in carrot (Daucus carota L.). Plants 11(8), 1075.
| Crossref | Google Scholar |
Yadav VK, Krishna Jha R, Kaushik P, Altalayan FH, Al Balawi T, Alam P (2021) Traversing arbuscular mycorrhizal fungi and Pseudomonas fluorescens for carrot production under salinity. Saudi Journal of Biological Sciences 28(8), 4217-4223.
| Crossref | Google Scholar |
Yahyaa M, Ibdah M, Marzouk S, Ibdah M (2018) Profiling of the terpene metabolome in carrot fruits of wild (Daucus carota L. ssp. carota) accessions and characterization of a geraniol synthase. Journal of Agricultural and Food Chemistry 66(10), 2378-2386.
| Crossref | Google Scholar |
Yook M-J, Park H-R, Zhang C-J, Lim S-H, Jeong S-C, Chung YS, Kim D-S (2021) Environmental risk assessment of glufosinate-resistant soybean by pollen-mediated gene flow under field conditions in the region of the genetic origin. Science of The Total Environment 762, 143073.
| Crossref | Google Scholar |
Zhao S, Zou H, Jia Y, Pan X, Huang D (2022) Carrot (Daucus carota L.) seed germination was promoted by hydro-electro hybrid priming through regulating the accumulation of proteins involved in carbohydrate and protein metabolism. Frontiers in Plant Science 13, 824439.
| Crossref | Google Scholar |
Ziska LH (2022) Plant invasions, rising CO2, and global climate change. In ‘Global plant invasions’. (Eds DR Clements, MK Upadhyaya, S Joshi, A Shrestha) pp. 71–87. (Springer) 10.1007/978-3-030-89684-3_4