Effects of heading date and Epichloë endophyte on persistence of diploid perennial ryegrass (Lolium perenne). 1. Heading date
Katherine N. Tozer

A
B
Abstract
Data are lacking on the effects of heading date of perennial ryegrass (Lolium perenne L.) on ryegrass persistence.
We aimed to determine the impact of heading date on perennial ryegrass persistence.
Two mid-heading (Samson, Bronsyn) and two late-heading (One50, Rohan) cultivars, infected with Epichloë endophytes (AR37, nea2/6 or standard toxic), were established in a replicated plot study grazed by cattle in a subtropical environment of the upper North Island of New Zealand. Persistence characteristics were quantified at least five times per annum, over 4 years.
Late-heading cultivars had higher yield, nutritive value, perennial ryegrass content in pasture dry matter, ground cover and tiller density than mid-heading cultivars. There were large seasonal impacts on all ryegrass characteristics, with a major decline over summer and recovery during late autumn into early spring, with less recovery in the final year. Overall, there was a strong linear decline in ryegrass content measured during spring (2018–21).
The two late-heading cultivars were more persistent than the two mid-heading cultivars, although persistence declined over 4 years for all cultivars.
Results support industry recommendations of choosing late-heading cultivars for improved pasture quality and persistence.
Keywords: flowering date, herbage production, maturity date, nutritive value, pasture persistence, perennial pastures, resilience, tiller populations.
Introduction
There is increasing focus on the benefits of later heading cultivars of perennial ryegrass (Lolium perenne L.) in perennial-ryegrass-based pastures, given the potential of these cultivars to maintain pasture quality into spring through delayed onset of reproductive development and senescence (Donaghy et al. 2021). Heading date is defined as the date when 50% of perennial ryegrass tillers have an emerging inflorescence (Lee et al. 2012; Wims et al. 2014). In New Zealand, the heading dates of perennial ryegrass cultivars are defined relative to day 0, which is typically 22 October in Canterbury, South Island of New Zealand (Lee et al. 2012; Wims et al. 2014). Later heading cultivars remain vegetative for a longer period during spring than earlier heading cultivars; the onset of stem elongation and reproductive development and associated decline in nutritive values is delayed. This has benefits for pasture quality, dry matter intake and livestock performance (Gowen et al. 2003; O’Donovan and Delaby 2005; Hendriks et al. 2017).
Traditionally, pastures are grazed during spring to minimise flowering and the production of low-quality reproductive stem (Edwards and Chapman 2011). However, after flowering there is a flush of new tiller growth from the base of the reproductive tillers (Laidlaw 2004). These new daughter tillers have a higher probability of surviving dry summers than those produced from the base of vegetative tillers (Waller and Sale 2001). Therefore, allowing ryegrass to flower and produce daughter tillers can have a positive impact on tiller survival over summer (Matthew et al. 1991), which could presumably improve pasture persistence.
One unintended consequence of the use of later heading perennial ryegrass cultivars may be that tiller survival over summer is compromised, leading to a decline in pasture persistence. In later heading cultivars, the flush of tiller growth after flowering occurs later in spring and may coincide with the onset of hotter temperatures and soil-moisture-deficit stress, potentially compromising (daughter) tiller survival (Matthew and Sackville Hamilton 2011). By contrast, earlier heading cultivars will undergo flowering and daughter tiller production earlier in spring when rainfall may be more reliable. For this reason, Leddin (2017) recommended that earlier heading cultivars be sown to increase persistence of perennial-ryegrass-based pastures in south-western Victoria, Australia; early spring rainfall was generally sufficient for ryegrass growth but later spring rainfall was more variable and sometimes inadequate for growth.
There is some evidence for natural selection of earlier heading ryegrass ecotypes in hill-country pastures. Forde and Suckling (1980) found that perennial ryegrass plants collected from old pastures with a low phosphorus (P) input were more vigorous and earlier heading than plants collected from well-fertilised or oversown sites, in high-rainfall New Zealand hill country. They found little correlation between heading date and vegetative characteristics such as plant height and leafiness, although the more persistent populations produced large amounts of seed.
There are no robust published data investigating the impact of heading date on perennial ryegrass persistence. Data are available concerning cultivars that vary in heading date and are managed in common and defoliated on the same date (e.g. Griffiths et al. 2020). This approach provides valuable information on the comparative morphology and nutritive value of cultivars varying in heading date. However, it does not take into account the effect of the timing of grazing relative to the reproductive development stage and how this may affect the survival of defoliated tillers. Compared with vegetative tillers, in which the apical meristem is below grazing height, elongating or reproductive tillers are more likely to die when grazed owing to removal of the elevated apical meristem. It follows that, during spring, differences in the reproductive development stage of cultivars with different heading dates will affect the extent of tiller regrowth after grazing. To address this, swards would need to be defoliated at an equivalent reproductive stage, such as on their estimated heading date, rather than on the same calendar date.
Commercially available perennial ryegrass cultivars sold in New Zealand are typically infected with selected Epichloë fungal endophytes (Caradus et al. 2021). The ryegrass–endophyte association produces alkaloids that confer protection against invertebrate pest damage over and above equivalent endophyte-free ryegrass.
To our knowledge, no studies are available that investigate the effects of heading date and endophyte strain on perennial ryegrass persistence, with defoliation management based on reproductive stage rather than calendar date. Therefore, we designed a study to compare the persistence over 4 years of two mid-heading diploid cultivars and two late-heading diploid cultivars infected with a range of endophytes. The hypothesis was that the mid-heading cultivars would be more persistent than the late-heading cultivars, regardless of endophyte strain, with greater persistence characterised by higher tiller populations, herbage production, and perennial ryegrass ground cover and content, and less weed ingress over time. Here, in the first of this two-part series, we focus on heading date, and in the second paper, on endophyte effects and interactions with heading date.
In order to eliminate the confounding effects of heading date and defoliation date, a long spring rotation was imposed for all treatments. The rotation length was based on the commercially defined heading dates to allow a similar level of reproductive development for the mid-and late-heading cultivars. Reproductive development has traditionally been discouraged because of the decline in pasture quality that occurs with stem elongation and seedhead development (Waghorn and Clark 2004); however, allowing anthesis to occur prior to grazing, followed by defoliation of reproductive tillers, can increase post-anthesis tillering, facilitate root development and improve pasture persistence (Soper 1958; Da Silva et al. 1994).
Materials and methods
Site establishment
The study was undertaken between March 2018 and May 2022. The 0.1-ha site was established in March 2018 on the Ruakura Research Station, Hamilton, upper North Island of New Zealand (37°46′15″S, 175°18′35″E; 20 m a.s.l.), on a Typic Orthic Gley (Hewitt 1998) with a pH of 5.6 and Olsen P of 36 mg L−1 (Table 1). Rainfall was below average in spring 2018, summer 2018–19, autumn 2019, summer 2019–20, spring 2020, summer 2020–21, autumn 2021 and summer 2021–22 (Fig. 1). Average monthly temperatures ranged from approximately 5°C in winter to 25°C in summer (Fig. 1).
Sampling time | pH | Olsen P | K | Ca | Mg | Na | Sulfate-S | Total C | Total N | |
---|---|---|---|---|---|---|---|---|---|---|
(mg L−1) | (cmolc kg−1) | (mg kg−1) | (%) | |||||||
March 2018 | 5.6 | 36 | 1.00 | 12.9 | 1.40 | 0.19 | 26 | 12.8 | 0.91 | |
March 2019 | 5.6 | 37 | 0.86 | 8.9 | 0.75 | 0.14 | 20 | 12.5 | 0.91 | |
April 2021 | 5.7 | 30 | 0.27 | 14.7 | 0.78 | 0.12 | 10 | 12.5 | 0.74 | |
May 2022 | 5.8 | 23 | 0.49 | 15.1 | 0.84 | 0.17 | 35 | 13.0 | 0.87 |
Long-term (30-year, 1986–2015) average monthly rainfall, and monthly rainfall and average maximum and minimum air temperatures from January 2018 to June 2022. Data were collected from the electronic weather station (EWS) at the experimental site (Hamilton Ruakura 2EWS, NIWA agent number 26117).
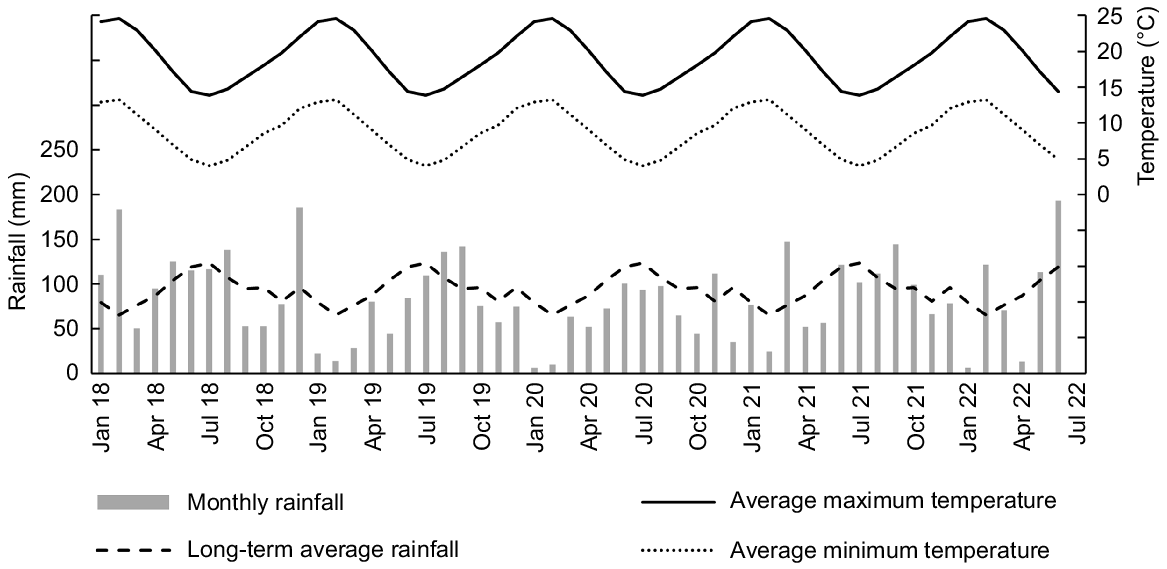
The existing perennial-ryegrass-based pasture was removed by applying the herbicide CRUCIAL (600 g L−1, a.i. glyphosate @ 2400 g ha−1; Nufarm, Auckland, New Zealand) on 3 March 2018. The site was then ploughed on 23 March and power-harrowed on 29 March.
On 24 April 2018, plots were drilled to a depth of 1 cm with 20 kg ha−1 of seed from one of the four treatment cultivars that was coated with Superstrike (insecticide; PGG Wrightson Seeds), using a precision plot seeder with press wheels. Buffer areas surrounding the plots were then hand-sown with 20 kg ha−1 of Superstrike-coated seed of perennial ryegrass cv. One50 infected with AR37 endophyte, followed by hand raking. After sowing, 150 kg ha−1 of diammonium phosphate (18% N, 46% P; Ballance Agri-Nutrients, Tauranga, New Zealand) and 8 kg ha−1 of Slugout (a.i. metaldehyde @ 18 g kg−1; Nufarm) were hand-broadcast across the site.
For weed control, pre-emergent applications of Weedmaster TS540 (2.7 L ha−1, a.i. glyphosate @ 540 g L−1; Nufarm) and Nortron (3 L ha−1, a.i. ethofumesate @ 500 g L−1; Bayer CropScience, Auckland, New Zealand) were applied on 26 April, and a post-emergent application of Tropotox Ultra (4.5 L ha−1, a.i. MCPB @ 375 g L−1 and MCPA @ 25 g L−1; Nufarm) was applied on 1 June 2018.
Experimental design and treatments
The design was a randomised complete block with 11 treatments and four replicates. Treatments were an incomplete factorial combination of four perennial ryegrass cultivars, comprising two mid-season heading (‘mid-heading’) and two late-season heading (‘late-heading’) cultivars, infected with one of three strains of endophyte (Epichloë festucae var. lolii or Epichloë sp. LpTG-3) (Table 2). The two mid-heading cultivars were Bronsyn (heading date = day 0) and Grasslands Samson (hereafter termed Samson; +3 days), and the two late-heading cultivars were Rohan (+18 days) and One50 (+20 days) (Lee et al. 2012), with a germination percentage of 89% (±3.4%, standard error of difference) averaged over all cultivars. The endophytes were standard toxic endophyte (SE), and the selected endophytes AR37 and nea2/6. The combination One50–nea2/6 was not available.
Endophyte strain | Heading date type and cultivar | ||||
---|---|---|---|---|---|
Mid-heading | Late-heading | ||||
Bronsyn (day 0) | Samson (+3 days) | Rohan (+18 days) | One50 (+20 days) | ||
SE (Epichloë festucae var. lolii) | ✓ | ✓ | ✓ | ✓ | |
AR37 (Epichloë sp. LpTG-3) | ✓ | ✓ | ✓ | ✓ | |
nea2/6 (E. festucae var. lolii) (50:50 nea2:nea6) | ✓ | ✓ | ✓ | – |
Days relate to the number of days after the standard heading date of 22 October (Lee et al. 2012). –, not available.
Plot size was 3 m by 7 m. A 1.5-m buffer separated parallel replicate rows and surrounded the periphery, and a 0.5-m transverse buffer bisected the site near the longitudinal mid-point.
Fertiliser and weed management
Fertiliser application was based on soil test results (Table 1). This included applications of 2 t ha−1 of lime in July 2019, August 2019 and September 2020; a combination of 22 kg Mg ha−1 (as MgSO4, 9.9% Mg) and 73 kg K ha−1 (as K2SO4, 43% K) applied after every grazing from May 2021; and 25 kg N ha−1 after every grazing from September 2018 (SustaiN, 46% N; Ballance Agri-Nutrients). No herbicide was applied after site establishment.
Defoliation management
The site was first grazed on 28 August 2018 by 150 heifers aged 12 months, and thereafter, by mixed-breed and mixed-age dairy heifers, with other stock classes used occasionally (e.g. dairy calves or beef heifers). Mob sizes varied. Treatments were grazed or mown on four occasions in 2018 between October and December; and on 10 occasions in 2019, 10 in 2020, 10 in 2021, and four in 2022 between January and May, when the experiment ended. Grazing was delayed if required to ensure that there were at least two leaves present at the time of grazing. Between January and September, when perennial ryegrass was vegetative, the site was grazed only when there were at least two new leaves since the last grazing (Fulkerson and Donaghy 2001). Grazing occurred over ~6 h and stock were removed when there was a residual herbage mass of ~1500 kg dry matter (DM) ha−1. On several occasions, grazing was required over 2 days to achieve this residual mass, in which case stock were removed from the study site overnight to prevent pugging damage. Occasionally, the site was mown to a residual height of 5 cm if it had been grazed unevenly.
Between October and December, when perennial ryegrass was undergoing reproductive development, livestock were excluded and treatments were mown to a height of 7 cm (Masport President 3000 mower, set at cutting height 5; Masport, Auckland, New Zealand). Defoliation decision rules were based on perennial ryegrass phenology with a defoliation interval to allow anthesis. This entailed a long spring rotation of ~7 weeks for both treatments, to allow perennial ryegrass reproductive development (Fig. 2, Tables 3 and 4). The timing of the long spring rotation depended on the pasture growth, which varied among years. In general, all treatments were defoliated in early October. The long spring rotation occurred between early October and mid-November for mid-heading cultivars, and late October and mid-December for late-heading cultivars, simulating a ‘split-grazing’ (Fig. 2). All treatments were defoliated in mid-December.
Approximate defoliation dates and percentage of reproductive tillers for the mid- and late-season heading cultivars during spring (October–December) 2018–2021.
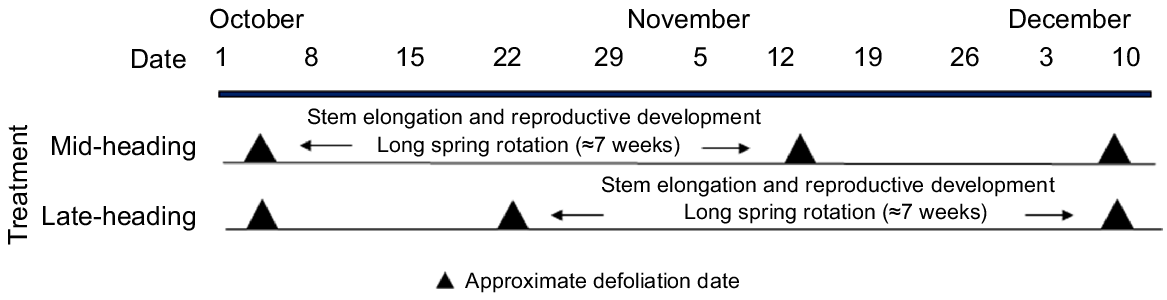
2018 | 2019 | 2020 | 2021 | |||
---|---|---|---|---|---|---|
Duration of long spring rotation (days) | ||||||
Mid-heading | 48 | 47 | 47 | 47 | ||
Late-heading | 47 | 47 | 46 | 48 | ||
Percentage of reproductive tillers | ||||||
Mid-heading | Start | 0 | 0 | 0 | 0 | |
End | 35 | 53 | 45 | 32 | ||
Late-heading | Start | 0 | 1 | 0 | 0 | |
End | 35 | 45 | 62 | 22 |
Year | Development stage | Late September/early October | Late October | Mid-November | Mid-December | |||||||||||||
---|---|---|---|---|---|---|---|---|---|---|---|---|---|---|---|---|---|---|
Mid | Late | s.e.d. | P-value | Mid | Late | s.e.d. | P-value | Mid | Late | s.e.d. | P-value | Mid | Late | s.e.d. | P-value | |||
3 October | 31 October | 20 November | 17 December | |||||||||||||||
2018 | Vegetative | 87 | 88 | 2.9 | 0.575 | 67 | 77 | 4.1 | 0.013 | 53 | 78 | 3.1 | <0.001 | 99 | 65 | 2.8 | <0.001 | |
Elongating | 13 | 12 | 2.9 | 0.575 | 30 | 23 | 4.3 | 0.082 | 12 | 20 | 2.6 | 0.003 | 0 | 3 | 1.0 | 0.001 | ||
Reproductive | 0 | 0 | – | – | 3 | 0 | 1.1 | 0.004 | 35 | 2 | 2.2 | <0.001 | 1 | 35 | 4.5 | <0.001 | ||
3 October | 30 October | 19 November | 16 December | |||||||||||||||
2019 | Vegetative | 99 | 98 | 0.9 | 0.095 | 75 | 88 | 3.1 | <0.001 | 38 | 78 | 2.9 | <0.001 | 94 | 53 | 2.9 | <0.001 | |
Elongating | 1 | 3 | 0.9 | 0.095 | 21 | 12 | 2.7 | 0.002 | 9 | 21 | 1.7 | <0.001 | 0 | 2 | 0.9 | 0.024 | ||
Reproductive | 0 | 0 | – | – | 4 | 1 | 1.0 | <0.001 | 53 | 1 | 3.2 | <0.001 | 6 | 45 | 3.0 | <0.001 | ||
23 September | 22 October | 10 November | 7 December | |||||||||||||||
2020 | Vegetative | 100 | 99 | – | – | 70 | 88 | 2.8 | <0.001 | 49 | 75 | 3.3 | <0.001 | – | 36 | – | – | |
Elongating | 0 | 1 | – | – | 27 | 12 | 2.24 | <0.001 | 5 | 14 | 2.4 | <0.001 | – | 2 | – | – | ||
Reproductive | 0 | 0 | – | – | 3 | 0 | 1.0 | 0.002 | 45 | 11 | 3.4 | <0.001 | – | 62 | – | – | ||
29 September | 26 October | 15 November | 13 December | |||||||||||||||
2021 | Vegetative | 99 | 98 | 0.7 | 0.048 | 71 | 76 | 3.8 | 0.097 | 57 | 80 | 2.9 | <0.001 | 99 | 76 | 2.1 | <0.001 | |
Elongating | 1 | 2 | 0.7 | 0.048 | 28 | 24 | 3.7 | 0.132 | 10 | 16 | 1.7 | 0.021 | 0 | 2 | 0.6 | 0.044 | ||
Reproductive | 0 | 0 | – | – | 0 | 0 | – | – | 32 | 4 | 3.3 | <0.001 | 1 | 22 | 2.0 | <0.001 |
In December 2020, data were collected for late-heading cultivars only. –, data either insufficient for statistical analyses (October 2018 and 2019, September 2020 and 2021) or not collected (December 2020).
Number and timing of measurements
Herbage mass was assessed in all treatments before and after grazing, or before and after mowing (Table 5). Pre-grazing leaf stage, ground cover and herbage mass, and post-grazing residual herbage mass, were assessed for up to 11 grazings/mowings per annum (Table 5). In addition, reproductive development, tiller density, botanical composition and nutritive values were assessed once before grazing in early and late spring, mid–late summer, autumn and winter, for all treatments (Table 5). Reproductive development was also assessed for the split-grazing in October/November.
Measurement | 2018 | 2019 | 2020 | 2021 | 2022 | |
---|---|---|---|---|---|---|
Herbage mass (RPM) | 4 | 11 | 11 | 11 | 3 | |
Ground cover | 2 | 9 | 9 | 9 | 3 | |
Reproductive development | 4 | 4 | 4 | 4 | 0 | |
Leaf stage | 2 | 9 | 9 | 9 | 3 | |
Tiller density | 2 | 5 | 4 | 6 | 2 | |
Botanical composition | 2 | 5 | 4 | 6 | 2 | |
Nutritive values | 2 | 5 | 4 | 6 | 2 | |
Alkaloid content | 2 | 5 | 4 | 6 | 2 | |
Soil nutrient status | 1 | 1 | 1 | 1 | ||
Endophyte infection frequency | 2 | 1 | 1 | 1 | ||
Invertebrate abundance | 1 | 1 |
Summer measurements occurred in December for all years except 2020, when they occurred in mid-January owing to the timing of the grazing round; this resulted in four measurements in 2020 but six in 2021.
RPM, rising plate meter; mower cuts were also used to assess herbage mass on six occasions each year.
Reproductive development was assessed between September and December; before September, all tillers were vegetative. Leaf stage was assessed before grazing or mowing, including split-harvests.
Pasture and soil measurements
Ground cover was visually assessed using a score of 1–10, with 1 equating to perennial ryegrass ground cover of ≤10%, and 10 equating to ≥91%. Scoring was done by the same operator for the duration of the experiment.
Perennial ryegrass tiller density in each plot was determined in five quadrats of 10 cm by 15 cm, positioned perpendicular to drill rows. During the second and third summers, 10 quadrats were used per plot owing to the low abundance and patchiness of perennial ryegrass. Vegetation within each quadrat was cut to ground level, bulked for each plot and weighed. A subsample (containing ~150 whole adult perennial ryegrass tillers) was removed and sorted into perennial ryegrass and ‘other vegetation’, the components were weighed, and the number of adult perennial ryegrass tillers was counted. Each component was oven-dried at 65°C to a constant weight over ~24 h, weighed, and the tiller mass of perennial ryegrass estimated. Perennial ryegrass tiller number, and percentage of the DM in the subsample and whole sample, were used to estimate the number of perennial ryegrass tillers in the whole sample. This was scaled to estimate the tiller number per square metre.
Herbage mass was assessed before and after each grazing between sowing and August 2018, using a rising plate meter (RPM: model EC-10; Jenquip, Feilding, New Zealand) randomly positioned in 20 locations per plot. The RPM calibration equation was: pasture mass = compressed pasture height × 140 + 500. The RPM calibration was verified for spring 2018 (September–November 2018) by plating four adjacent positions within each of 10 quadrats of 100 cm by 23 cm within the buffer zone on 3 October 2018. Quadrat locations spanned the range in available herbage. The average pasture mass was recorded for each quadrat, and the herbage was cut to ground level and oven-dried at 65°C to a constant weight. Pasture mass from the RPM was regressed on oven-dried herbage mass. The RPM calibration was similar to the estimates obtained from herbage cuts.
Herbage mass was also measured in each plot by mowing a 50-cm-wide strip to 7 cm above ground level, after RPM measurements. Strip length was recorded, the cut herbage weighed, and a minimum 300-g subsample oven-dried at 65°C for 48 h to determine the percentage DM and the DM yield of each mowing strip. Results were scaled to determine the yield on a per hectare basis. There were four adjacent mowing strip locations in each plot, and a different strip was mown each time on rotation. Herbage was harvested using a mower, six times per year for each cultivar. Seasonal herbage production was calculated using the daily rate of growth between measurements, scaled to the number of days in the season.
After grazing, herbage mass was assessed in each plot by using the RPM. On eight additional occasions, herbage mass was assessed in 10 random positions in the mower strip in each plot: 3 October 2018, 3 October 2019, 23 September 2020, 13 January 2021, 14 April 2021, 2 August 2021, 29 September 2021 and 28 February 2022. This was done to determine whether the mower cutting height was similar to the average post-grazing herbage mass and, therefore, provided a robust estimate of the herbage removed by the grazing livestock.
Nutritive values were determined by taking 20 random handfuls of pasture cut to ground level, bulking for each plot and subsampling using the quartering method (Cayley and Bird 1996). Subsamples were frozen at −20°C, freeze-dried (FD80 freeze dryer; Cuddon, Blenheim, New Zealand), and ground to a fine powder with a 1-mm sieve (Cyclone Sample Mill; UDY Corporation, Fort Collins, CO, USA) for feed profile analyses by Hill Laboratories (Hamilton, New Zealand), using near-infrared spectroscopy (Corson et al. 1999). Values were estimated for metabolisable energy (ME), digestibility of organic DM, crude protein (CP), soluble sugars, neutral detergent fibre (NDF) and ash.
For botanical composition assessment, a second subsample, using the same sampling method, was sorted into perennial ryegrass; ‘other grass’ – predominantly the temperate C3 species soft brome (Bromus hordeaceus L.), annual poa (Poa annua L.), browntop (Agrostis capillaris L.) and Yorkshire fog (Holcus lanatus L.), and the subtropical C4 species summer grass (Digitaria sanguinalis (L.) Scop.); white clover (Trifolium repens L.); other clovers; broadleaf weed; and dead vegetation. Reproductive development and leaf stage were assessed on the perennial ryegrass component from the botanical composition subsample. Thirty randomly selected perennial ryegrass tillers were separated into vegetative, elongating and reproductive tillers for the purpose of defining the extent of reproductive development (Moore et al. 1991) during the extended spring rotation. Tillers were classed as ‘elongating’ from the onset of stem elongation until inflorescence emergence, and ‘reproductive’ from inflorescence emergence until anthesis and fertilisation (Moore et al. 1991). The percentage of mid- and late-heading reproductive tillers in each of the five reproductive substages, as defined by Moore et al. (1991), was also recorded. To assess leaf stage, 10 vegetative tillers were randomly selected and the number of new leaves was counted for each tiller. Following this, all botanical composition vegetation components (perennial ryegrass, other grass, white clover, other clovers, broadleaf weed, dead vegetation) were oven-dried at 65°C to a constant weight to determine their contribution to total DM.
Leaf stage of perennial ryegrass was also assessed up to 1 week before grazing, to ensure that there were two or more new leaves present at the time of grazing. Ten randomly selected perennial ryegrass tillers were collected from the same eight plots throughout the study, which ranged in cultivar and endophyte strain, and their leaf stage was determined. These interim data are not reported; only leaf stage data collected from all plots at the time of grazing are reported. Soil nutrient status was assessed annually in autumn: in March 2018, March 2019, April 2021 and May 2022. Forty-four cores (one from each plot) were randomly sampled to a depth of 7.5 cm across the experimental site. Samples were bulked and analysed by Hill Laboratories (Hamilton, New Zealand) for pH, Olsen P, K, Ca, Mg, Na, sulfate-S, total C and total N, using standard commercial laboratory procedures.
Endophyte presence
The Epichloë endophyte tiller infection frequency was tested for sown seed and was tested in field plots in autumn 2018, spring 2018, and thereafter in autumn from 2019 to 2021, using the tissue print immunoblot assay of Simpson et al. (2012). Between 30 and 60 (depending on the year) randomly selected vegetative ryegrass tillers, each from a different plant, were cut at ground level from each plot. In the laboratory, tillers were recut and the moist tiller end was placed on nitrocellulose blotting paper. Subsequent antibody and colour development enabled presence/absence of endophyte to be determined and the frequency of endophyte infection estimated.
Statistical analyses
All data were analysed using Genstat 21st edition (VSN International, Hemel Hempstead, UK). Because grass cultivar and heading date were confounded, the data were analysed for grass cultivar and heading date separately. The design was unbalanced, and so data were analysed by residual maximum likelihood (Patterson and Thompson 1971) with grass cultivar or heading date, endophyte and their interaction as fixed effects, and replicate and row as random effects. Seasonal herbage production was calculated using the daily rate of growth between measurements, scaled to the number of days in the season. Post-grazing and post-mowing RPM measurements were compared for the eight dates when both were recorded, using a split-plot ANOVA fitting plot and harvest within plot. Because all data met the normality assumptions of the analyses, no transformations of the data were performed.
Heading date effects are reported in this paper, and endophyte effects and the small number of interactions are reported in the companion paper (Tozer et al. 2024); nearly all interactions between heading date and endophyte were for alkaloid concentrations. Cultivar effects are also reported when significant (P ≤ 0.05) differences occurred between the two cultivars within each heading date category.
Leaf stage, ground cover, tiller density, botanical composition and nutritive values from the split-harvests are not reported in the Results. This is because mid- and late-heading cultivars were subjected to different defoliation regimes during the mid–late-spring period, which affects these persistence characteristics. Our focus was on measuring heading date and endophyte effects, not defoliation effects.
Results
Establishment plant densities
The density of perennial ryegrass plants was higher for late-heading than mid-heading cultivars in May 2018 (133 vs 120 plants m−2, P = 0.001). Densities were similar for the two mid-heading cultivars (P = 0.106) and for the two late-heading cultivars (P = 0.743).
Heading date
There was negligible effect of heading treatment on the percentage of vegetative, elongating or reproductive tillers at the beginning of the long spring rotation in late September/early October (Table 4, Supplementary Table S1), with nearly all tillers being vegetative. This had changed considerably at the late October and mid-November measurements, with mid-heading cultivars having a lower percentage of vegetative tillers and a higher percentage of reproductive tillers than late-heading cultivars in most years. By mid-December, the opposite was the case, with late-heading cultivars having a higher percentage of elongating/reproductive tillers and a lower percentage of vegetative tillers.
The percentages of tillers in each of the three categories were similar (P > 0.05) for the two mid-heading cultivars, and the two late-heading cultivars, on nearly all occasions. In the few instances where there were significant (P < 0.05) differences between cultivars within a heading date category, no consistent trends were observed (Table S2).
Endophyte infection frequency
Infection frequency of viable endophyte in sown seed averaged 95% and did not differ (P > 0.05) between heading date treatments. Endophyte infection frequency of perennial ryegrass tillers was high throughout the experiment, averaging ≥91% at each of the five measurements, with no change over time (October 2018–May 2021, P = 0.076).
Endophyte infection frequency was higher in late-heading than mid-heading cultivars in October 2018 (95% vs 91%, P = 0.011) and May 2021 (96% vs 93%, P = 0.003), but there was no effect (P > 0.05) of heading date in May 2018 (mean 91%), May 2019 (mean 94%) or May 2020 (mean 93%). Infection frequency was also higher in mid-heading than late-heading cultivars (95% vs 93%, P = 0.030) based on a repeated measures analyses (October 2018–May 2021).
Endophyte infection frequency was higher (P < 0.05) for Bronsyn than for Samson on three occasions and higher (P < 0.05) for Rohan than for One50 on two occasions (Table S2). On average, it was also higher (P < 0.01) for Bronsyn than Samson (97% vs 94%) and for Rohan than One50 (94% vs 90%), based on a repeated measures analysis.
Leaf stage
Swards were grazed or mown when there were 2.5 new leaves since the last defoliation event, averaged over all 32 occasions (Fig. 3). The number of new leaves was greater (P < 0.05) for mid-heading than late-heading cultivars on one occasion, and greater (P < 0.05) for late-heading than mid-heading cultivars on five occasions, with most differences occurring in late spring/summer.
Effect of heading date on the number of new leaves per tiller at the time of each harvest date, where ‘leaf stage’ is defined as the number of new leaves since the last grazing/mowing. Mid, mid-season heading cultivars; Late, late-season heading cultivars. Error bars represent the standard error of the mean. *P < 0.05; **P < 0.01.
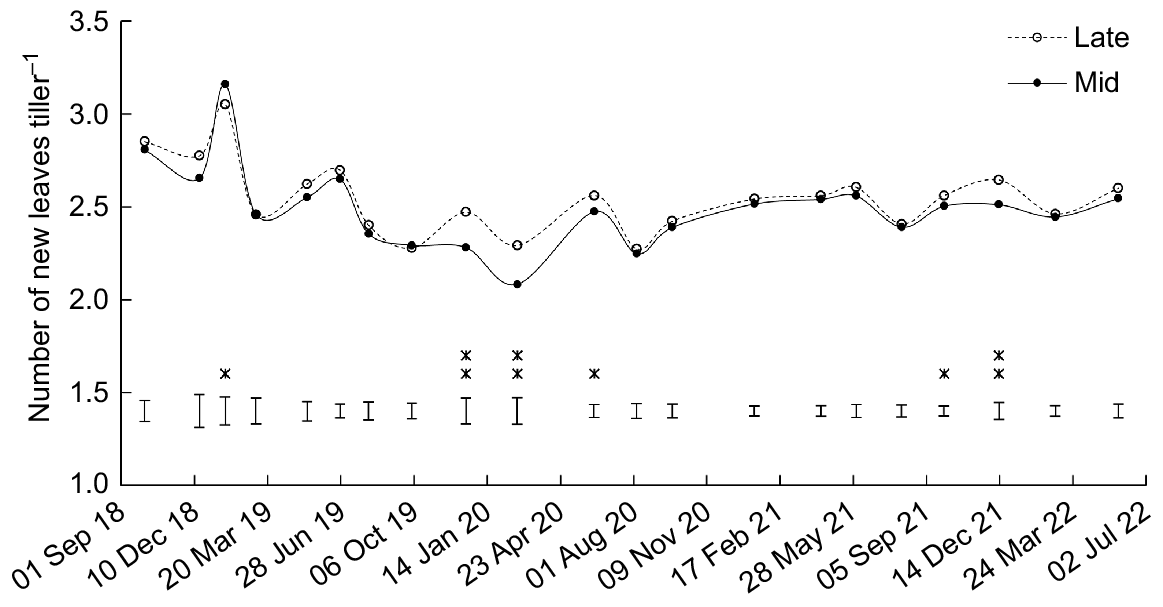
Between mid-spring and mid-summer, the number of new leaves was greater (P < 0.05) for Rohan than One50 on five occasions (Table S2).
Perennial ryegrass ground cover
There was an annual cycle of a decline in ground cover over summer followed by an autumn recovery (Fig. 4a). In late summer/early autumn 2021, ground cover score fell to <2.5 in late-heading treatment, after which it recovered. In 2022, there was a similar drop, but no recovery on the last measurement occasion in May 2022.
Effect of heading date on perennial ryegrass (a) ground cover and (b) tiller density. Mid, mid-season heading cultivars; Late, late-season heading cultivars. Error bars represent the standard error of the mean. *P < 0.05; **P < 0.01; ***P < 0.001.
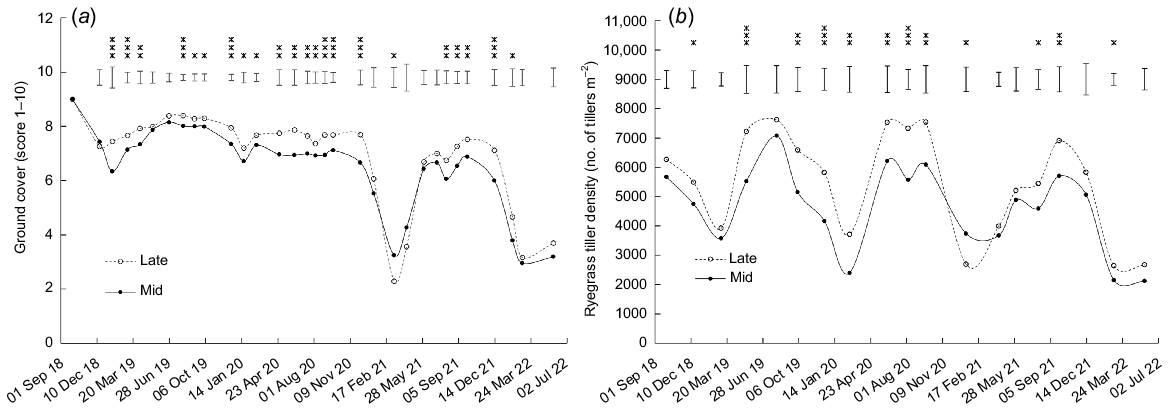
Ground cover of perennial ryegrass was higher (P < 0.05) in late-heading than mid-heading cultivars on 21 of 32 occasions and higher (P = 0.028) in mid-heading than late-heading cultivars on one occasion (Fig. 4a). Ground cover was higher (P < 0.05) for Bronsyn than Samson on 15 occasions, and higher (P < 0.05) for One50 than Rohan on three occasions (Table S2).
Tiller density
Tiller density followed an annual cyclical trend for all treatments, with maximums in early spring and minimums in late summer/early autumn. Tiller density was higher (P < 0.05) for late-heading than mid-heading cultivars on 11 of the 19 occasions (Fig. 4b). Bronsyn had a higher (P < 0.01) density than Samson on three occasions, and Rohan had a higher (P < 0.05) tiller density than One50 on six occasions (Table S2).
Herbage production
For the 16 seasons of measurement, herbage production was higher (P < 0.05) for late-heading than mid-heading cultivars for seven seasons but lower (P < 0.05) for late-heading than mid-heading cultivars for three seasons, all of which were in spring (Fig. 5).
Effect of heading date on seasonal herbage production. Data from winter 2018 were obtained from a rising plate meter to avoid damaging the establishing sward, and data thereafter were from from mower cuts. Mid, mid-season heading cultivars; Late, late-season heading cultivars. Error bars represent the standard error of the mean. *P < 0.05; **P < 0.01; ***P < 0.001.
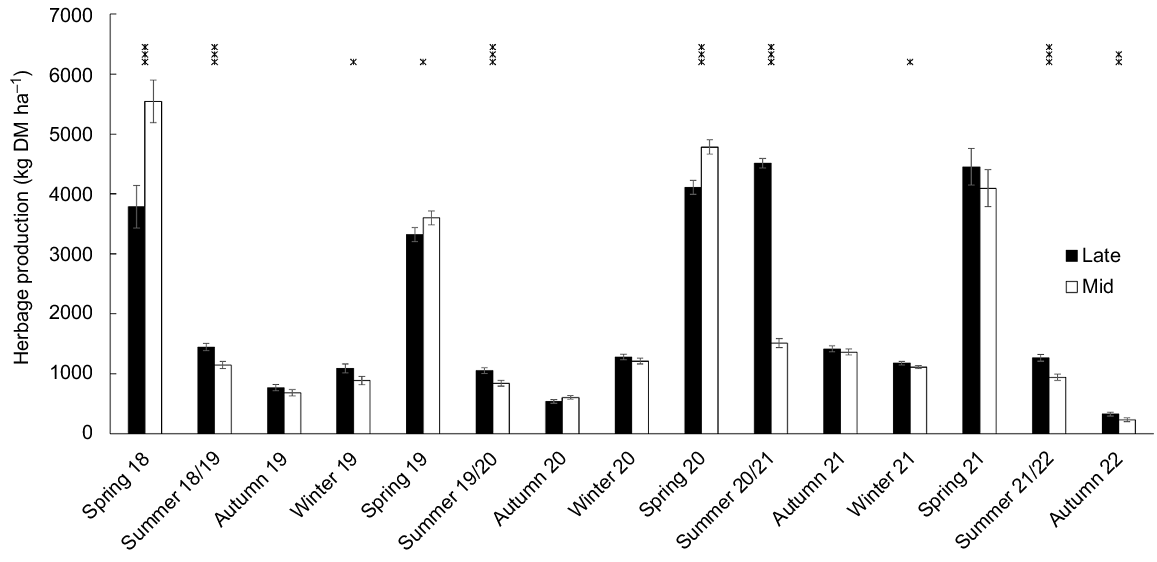
Total annual herbage production was higher (P < 0.05) for late-heading than mid-heading cultivars in the third year and final year, and for the mean of all years (Table 6). Seasonal herbage production, meaned over the 4 years, was also higher (P < 0.05) for late-heading than mid-heading cultivars in summer and autumn, but lower (P < 0.05) for late-heading than mid-heading cultivars in spring Table 6).
Year/season | Herbage production (kg DM ha−1) | P-value | ||||
---|---|---|---|---|---|---|
Mid | Late | s.e.d. | ||||
Total annual | 2018–19 | 11,470 | 10,570 | 661 | 0.121 | |
2019–20 | 5930 | 6000 | 199 | 0.613 | ||
2020–21 | 8870 | 11,320 | 223 | <0.001 | ||
2021–22 | 6380 | 7220 | 332 | 0.013 | ||
Seasonal means | Winter | 1830 | 2030 | 128 | 0.129 | |
Spring | 4510 | 3910 | 128 | <0.001 | ||
Summer | 1110 | 2070 | 33 | <0.001 | ||
Autumn | 720 | 760 | 24 | 0.031 | ||
Overall total | Annual | 8170 | 8710 | 208 | 0.014 |
A mower was used to harvest the pasture for estimates of herbage mass, except during winter 2018, when a rising plate meter was used to avoid mower damage of the newly established sward.
s.e.d., standard error of difference.
Herbage production was higher for Bronsyn than Samson in winter 2021 (P = 0.019), and in winter and spring when averaged over 4 years (P < 0.05, Table S2). The residual herbage mass was higher in the grazed sward than in the mowing strips (2.81 kg vs 2.67 kg DM plot−1, P < 0.001 (plot size 21 m2)).
Botanical composition
The content of perennial ryegrass was higher (P < 0.05) in swards sown with late-heading than with mid-heading cultivars on five of 19 occasions (Fig. 6a). The perennial ryegrass content was higher (P < 0.05) for swards sown with Bronsyn than with Samson on five occasions (Table S2). There was an increase in the unsown species content (% of DM) in spring between 2018 and 2021 (R2 = 0.92, P < 0.001, Fig. 7).
Effect of heading date on the content of (a) perennial ryegrass, (b) other grass, (c) legumes, (d) broadleaf weed, and (e) dead vegetation. Mid, mid-season heading cultivars; Late, late-season heading cultivars. Error bars represent the standard error of the mean. *P < 0.05; **P < 0.01; ***P < 0.001.
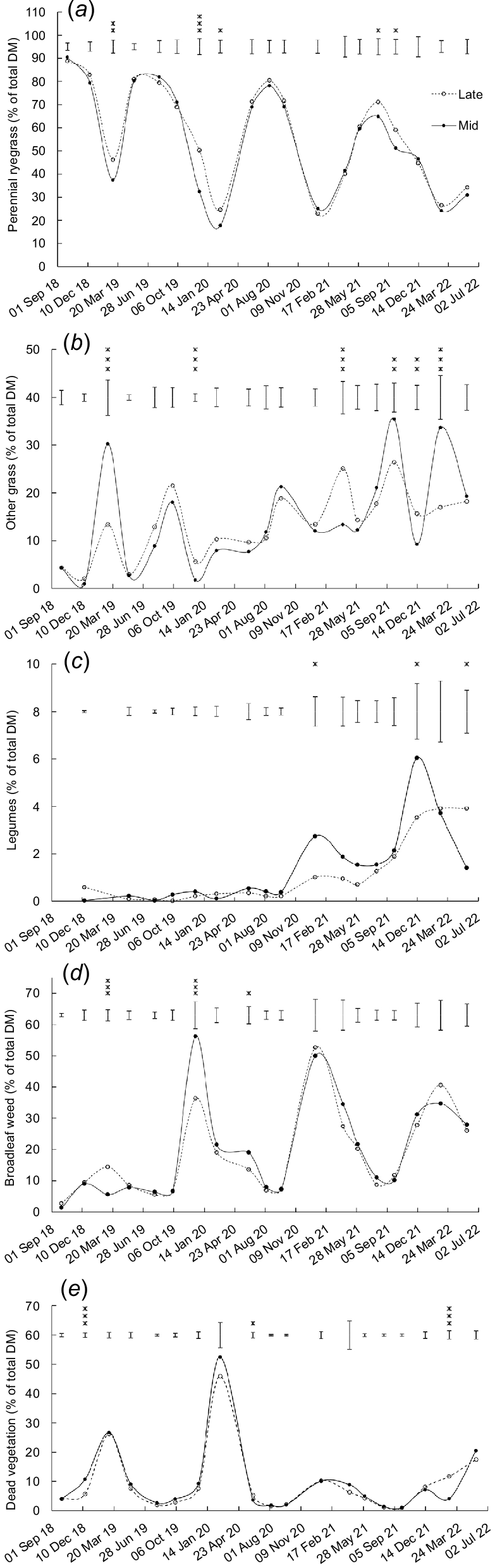
Increase in unsown species content (% in total DM) in spring (2018–21; linear regression R2 = 0.92, P < 0.001). Data are averages of heading date (mid, late) and endophyte strain (SE, AR37 and nea2/6) treatments.
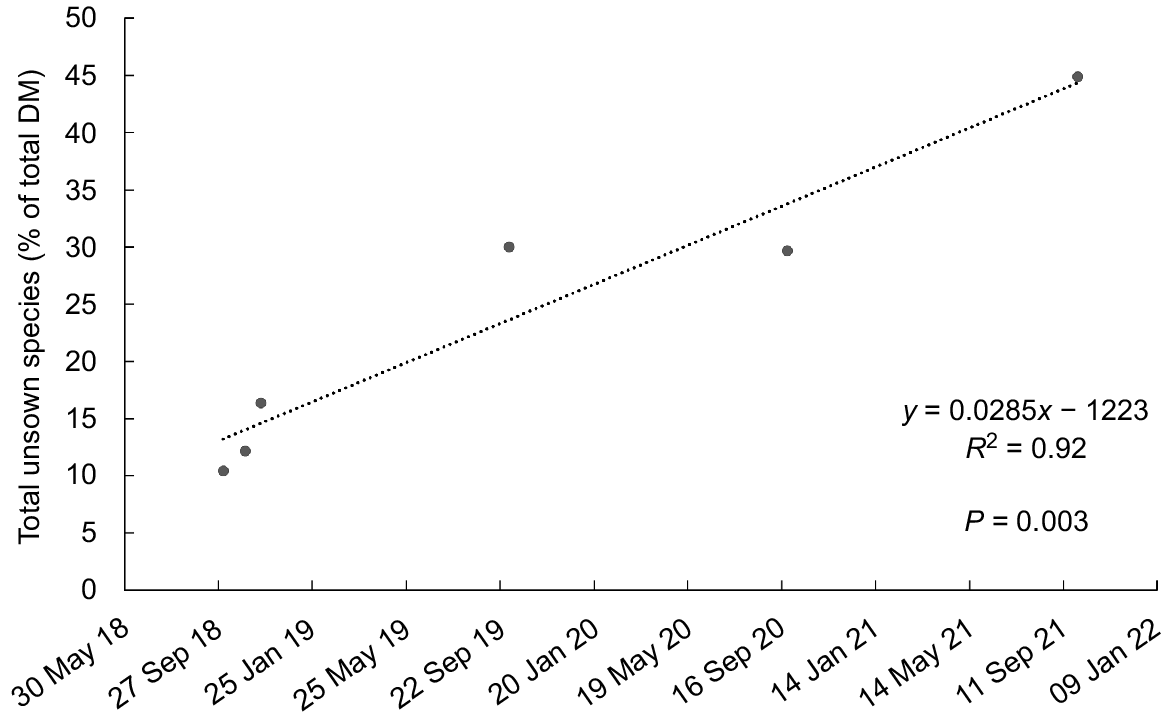
There was a significant (P < 0.01) effect of heading date on the content of other grass on six occasions, but the differences followed no clear trend (Fig. 6b). The content of other grass was higher (P = 0.017) for swards sown with Bronsyn than with Samson on one occasion (Table S2). There were also differences (P < 0.05) between One50 and Rohan in the content of other grass on two occasions, with no clear trends (Table S2).
The legume content mainly comprised white clover, and it remained <7% of total DM throughout the study. The legume content was lower (P < 0.05) in swards sown with late-heading than mid-heading cultivars on two occasions and higher (P < 0.05) on one occasion (Fig. 6c). The legume content was higher (P = 0.003) for swards sown with Samson than with Bronsyn on one occasion (Table S2).
The content of broadleaf weed was lower (P < 0.05) in swards sown with late-heading than mid-heading cultivars on two occasions and higher (P < 0.05) on one occasion (Fig. 6d). Broadleaf weed content was higher (P < 0.05) for Samson than for Bronsyn on four occasions (Table S2).
The content of dead vegetation was higher (P < 0.05) for swards sown with late-heading than mid-heading cultivars on two occasions and lower (P < 0.05) for swards sown with late-heading than mid-heading cultivars on one occasion (Fig. 6e).
Nutritive values
Metabolisable energy content was higher (P < 0.05) in swards sown with late-heading than mid-heading cultivars on three occasions, between mid-summer and early autumn (March 2019, January 2021, February 2020) (Fig. 8a). On seven other occasions, between April and December, the ME content was higher (P < 0.05) in swards sown with mid-heading than late-heading cultivars (Fig. 8a). ME content was higher (P < 0.05) in swards sown with Samson than Bronsyn on three occasions and higher (P = 0.005) with Rohan than One50 on one occasion (Table S2).
Effect of heading date on (a) metabolisable energy, (b) crude protein, (c) neutral detergent fibre (NDF), and (d) ash. Mid, mid-season heading cultivars; Late, late-season heading cultivars. Error bars represent the standard error of the mean. *P < 0.05; **P < 0.01; ***P < 0.001.
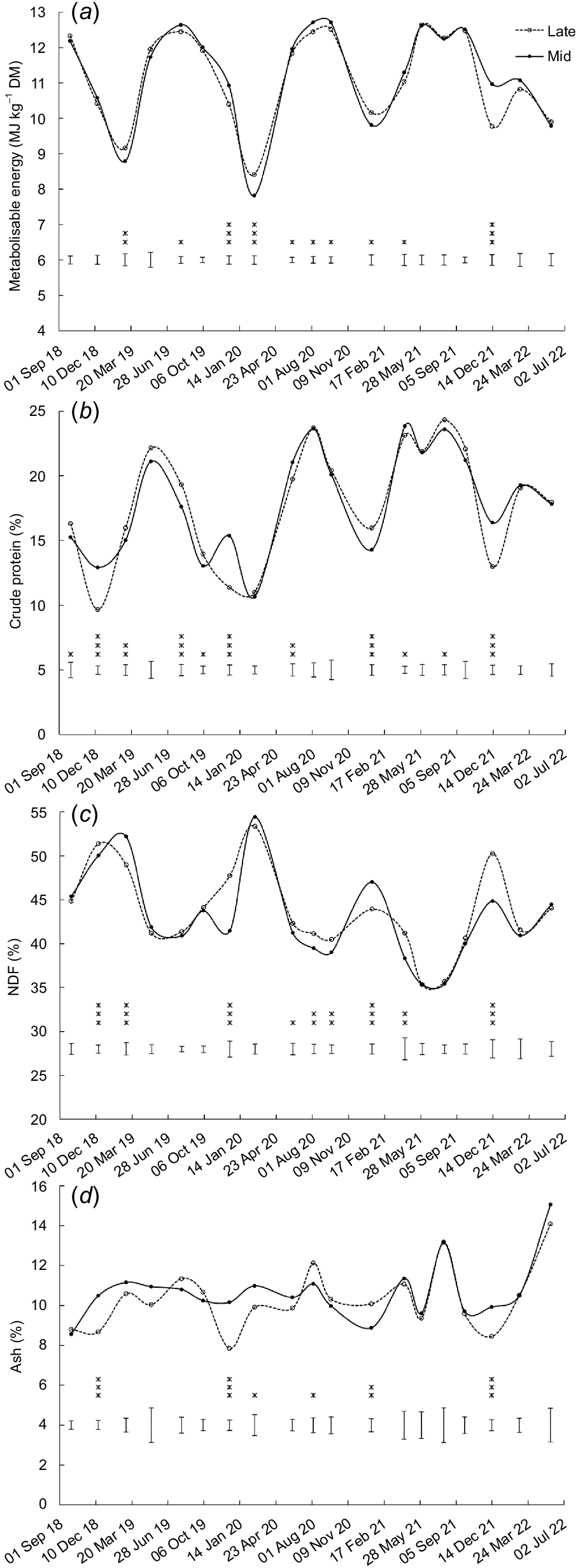
There were no consistent effects of heading date on CP content. The CP content was higher (P < 0.05) in swards sown with late-heading than mid-heading cultivars on five occasions, and lower (P < 0.05) in swards sown with late-heading than mid-heading cultivars on another five occasions (Fig. 8b). The CP content was higher (P < 0.05) for swards sown with Samson than Bronsyn on four occasions and higher (P < 0.05) with Rohan than One50 on three occasions (Table S2).
Values of NDF were higher (P < 0.05) for swards sown with late-heading than mid-heading cultivars on seven occasions and lower (P < 0.001) in swards sown with late-heading than mid-heading cultivars on two occasions (Fig. 8c). Values of NDF were higher (P < 0.05) for swards sown with Bronsyn than Samson on four occasions; they were also higher (P < 0.05) for swards sown with One50 than Rohan on seven occasions, and with Rohan than One50 on one occasion (Table S2).
Ash content was higher (P < 0.05) for swards sown with late-heading than with mid-heading cultivars on two occasions and lower (P < 0.05) for swards sown with late-heading than mid-heading cultivars on four occasions (all of which were during summer (December–February, Fig. 8d)). The ash content was higher (P < 0.05) in swards sown with Bronsyn than Samson on three occasions, and with Samson than Bronsyn on one occasion (Table S2).
Discussion
Establishment of treatments and general trends
All treatments established well, with high levels of endophyte infection, and perennial ryegrass ground cover and content (all >90%), and tiller densities of ~6000 m−2 in the first spring after establishment. Reproductive growth, as defined by Moore et al. (1991), began earlier for mid-heading than late-heading cultivars in all years, as expected, although there was variation in the percentage of tillers that became reproductive (32–53% for mid-heading cultivars and 22–62% for late-heading cultivars at the end of the long spring rotation, Tables 3 and 4). These differences may be explained, in part, by variability among years for spring temperatures, because the rate of reproductive growth is influenced by temperature (Keatinge et al. 1979). Temperatures in early spring have a strong influence on heading date of early-heading cultivars, while the heading date of later heading cultivars is more dependent on temperatures during stem elongation (Camlin 1975).
Given the establishment success for all cultivars, and differences in heading date between the mid- and late-heading cultivars, the experimental swards enabled a robust test of the hypothesis that the two mid-heading cultivars would be more persistent than the two late-heading cultivars.
There was a strong seasonal trend in perennial ryegrass performance and a loss of ryegrass persistence characterised by weed ingress, regardless of heading date. This is consistent with research in the upper North Island of New Zealand, in which perennial ryegrass populations declined and the abundance of weedy species increased as pastures aged (Tozer et al. 2014; Dodd et al. 2018; Beukes et al. 2021).
Heading date effects
Contrary to our hypothesis, later heading cultivars were more persistent than earlier heading cultivars. This was demonstrated by higher seasonal and annual herbage production, perennial ryegrass ground cover and content in total DM, and tiller densities, and lower content of broadleaf weed and legumes, for late-heading than mid-heading cultivars on many occasions. There were some consistent exceptions, such as in spring, when mid-heading cultivars generally produced more herbage than late-heading cultivars. This trend reflects the earlier timing of reproductive development of the mid-heading cultivars and associated higher levels of biomass accumulation during the reproductive period (Laidlaw 2005).
As expected, the ME contents of late-heading cultivars were generally higher than those of mid-heading cultivars during summer. This most likely reflected the extended period of vegetative growth and later reproductive development of late-heading cultivars (Laidlaw 2004). Results were more variable for levels of CP, NDF and ash, with no consistent trends.
The persistence and quality advantage for the two late-heading cultivars occurred from establishment, based on ryegrass ground cover, tiller density and ryegrass content in total DM, and this advantage was maintained thereafter. This poses the question of whether their greater persistence was purely due to greater establishment success rather than superior agronomic performance per se. This was not the case. Late-heading cultivars were more severely affected than mid-heading cultivars by the summer drought in 2020–21, with a greater decline in tiller densities and ground cover. However, their recovery from this low point was also greater, suggesting that the late-heading cultivars were better able to recover from stresses than the mid-heading cultivars (e.g. Fig. 4).
A possible reason for greater persistence and resilience of late-heading cultivars may be related to their tillering patterns during spring. Compared with mid-heading cultivars, late heading cultivars have a longer period during spring to produce vegetative tillers and their tiller densities were higher on numerous occasions. A similar trend occurred in a British study, where ‘intermediate maturing’ cultivars had higher tiller densities than ‘early maturing’ cultivars (Laidlaw 2004). Having a higher tiller population prior to drought stress over summer may ensure that more tillers per unit area survive the drought, which could assist in the recovery of tiller populations after drought, as also suggested by Thom et al. (2014).
An alternative reason for the greater persistence of the late-heading cultivars may be related to their genetics. Although concerns have been expressed that newer cultivars are less persistent than older cultivars, evidence for this is lacking (Easton et al. 2011; Faville et al. 2020a; Chapman et al. 2023a). It is estimated that plant breeding has led to increases in genetic gain for a variety of traits of >1% per year in New Zealand, with recent breeding focusing on the production of late-heading cultivars as well as early- and mid-heading cultivars (Woodfield and Easton 2004; Lee et al. 2012). Improvements in traits include increases in DM production and nutritive value (Chapman et al. 2023b). These improvements are consistent with the greater persistence of the two late-heading cultivars in our study, both of which are more recent than the two mid-heading cultivars. Regardless of the mechanism(s), our results lend support to using late-heading cultivars in preference to mid-heading cultivars to improve pasture productivity, persistence and livestock performance.
Cultivar effects
A premise of this study was that there would be greater variation between the heading date categories than between the two cultivars within each category. This was critical to enable the effects of heading date on pasture persistence to be quantified. Given the number and magnitude of the heading date main effects, this premise was sound, despite differences occurring between cultivars within each heading date category.
Differences between the two late-heading cultivars, One50 and Rohan, were variable, with higher values for One50 for some production and persistence attributes (e.g. ground cover) but higher values for Rohan for others (e.g. leaf emergence and tiller density). Differences may be due in part to their genetics. Rohan was released as a commercial cultivar in the 2010s (Nichols et al. 2016) and One50 was released in the 2000s (Lee et al. 2012), originating from a cross between New Zealand and Spanish breeding lines (Faville et al. 2020b). Rohan was bred for high tiller densities (Nichols et al. 2016) and a spreading habit, which is consistent with its high tiller densities in our study, although there was no difference between the two late-heading cultivars in ground cover.
Of the two mid-heading cultivars, Bronsyn was more persistent than Samson, with higher ground cover, tiller densities, seasonal herbage production, perennial ryegrass content, and concentrations of ergovaline and peramine (see companion paper), and a lower broadleaf weed content, on at least three measurement occasions. The greater productivity and persistence of cv. Bronsyn than Samson is consistent with other research (Lee et al. 2012). Although both cultivars were released in the 1990s, Bronsyn was bred from the Mangere ecotype from the upper North Island of New Zealand, whereas cultivar Samson was derived from a combination of Mangere and Hawkes Bay ecotypes (Stewart 2006; Caradus et al. 2023).
Limitations
This study compared two mid-heading and two late-heading cultivar–endophyte combinations in the upper North Island of New Zealand. Further studies in different years with more cultivars would be required to be confident of the validity of these results, because there are many factors affecting the persistence of each of the cultivar–endophyte combinations. Factors include year of sowing, the climate and soils, pest pressure, management, and their interactions with different cultivars and endophytes (Thom et al. 1996; Hume et al. 2010; Tozer et al. 2014; Cosgrove et al. 2020; Caradus et al. 2021). This has led to the creation of national field trials and forage value indexes at a range of locations across New Zealand, Australia and Ireland to obtain robust data on the productivity, persistence and profitability of different cultivar–endophyte combinations from multiple sites and years (e.g. Grogan and Gilliland 2011; Chapman et al. 2017; O’Donovan et al. 2017; Leddin et al. 2018, 2022; Caradus et al. 2021). Such trials demonstrate strong genotype × environment interactions, with differences between regions and years in the relative performance of perennial ryegrass cultivars (Chapman et al. 2017). There could also be variation between seedlots in their relative performance. Therefore, although results are suggestive of a strong heading-date effect, further research, using multiple cultivars in different regions, will be of value in validating our results.
Plot studies enable many variables to be controlled and are useful for increasing our understanding of ecological and biochemical mechanisms related to persistence. Results can inform future plant-breeding objectives to improve ryegrass resilience (Easton et al. 2011). However, care must be taken when extrapolating results of plot-scale studies because benefits may not be realised on-farm. For example, in a review of plot-scale and dairy-farmlet-scale research that involved heading date, yield advantages of late-heading cultivars from plot studies did not translate to greater pasture productivity, milk production or profitability (Hendriks et al. 2017). There were advantages of later heading perennial ryegrass cultivars, but advantages were due to improved pasture quality in spring and greater feed utilisation efficiency rather than differences in yield (Hendriks et al. 2017).
Conclusion
Results were contrary to the hypothesis that late-heading cultivars would be less persistent than mid-heading cultivars. Late-heading cultivars were more productive and persistent based on yield, nutritive values, and perennial ryegrass content, tiller densities and ground cover, on multiple occasions over the 4-year study. The higher tiller densities of the late-heading than mid-heading cultivars prior to the onset of summer drought may have contributed to improved persistence, although persistence declined over the 4 years for all cultivars. The greatest variation in perennial ryegrass productivity was due to seasonal effects, with large declines in productivity over summer and subsequent recovery during late autumn and into spring. Results support the use of the late-heading cultivars rather than the mid-heading cultivars tested in this study, given the greater persistence of the two late-heading cultivars.
Conflicts of interest
D. E. Hume is among the inventors of AR37. The authors have no other conflicts of interest to declare.
Declaration of funding
This project was funded from the AgResearch Strategic Science Investment Fund.
Acknowledgements
We thank Libby North, Bridget Wise, Jan Sprosen, Won Hong and Yulia Morozova of AgResearch for technical assistance; Tim Hale and Lee Falini of AgResearch and Shane Hill of Tainui Group Holdings for stock management; Agricom and Barenbrug for supplying seed; and Agricom for sowing the experiment. We also thank John Caradus (Grasslanz Technology), Jim Crush (AgResearch), Alan Stewart and Michael Norriss (PGG Wrightson Seeds), Graham Kerr (Barenbrug), and David Chapman (DairyNZ) for their useful comments that helped improve this manuscript.
References
Beukes P, Babylon A, Griffiths W, Woodward S, Kalaugher E, Sood A, Chapman D (2021) Modelling perennial ryegrass (Lolium perenne) persistence and productivity for the Upper North Island under current and future climate. NZGA: Research and Practice Series 17, 297-306.
| Crossref | Google Scholar |
Camlin MS (1975) Variation in heading dates of perennial ryegrass cultivars as influenced by spring and early summer temperatures. Grass and Forage Science 30, 289-294.
| Crossref | Google Scholar |
Caradus J, Chapman D, Cookson T, Cotching B, Deighton M, Donnelly L, Ferguson J, Finch S, Gard S, Hume D, Johnson L, Kerr G, Norriss M, Peddie K, Popay A (2021) Epichloë endophytes–new perspectives on a key ingredient for resilient perennial grass pastures. NZGA:Research and Practice Series 17, 347-360.
| Crossref | Google Scholar |
Caradus JR, Duder R, Kerr GA, Stewart AV, Thom ER (2023) Mangere to mainstream – the story of a perennial ryegrass ecotype, a determined farmer, and a progressive seed company. New Zealand Journal of Agricultural Research 1-17.
| Crossref | Google Scholar |
Chapman DF, Bryant JR, Olayemi ME, Edwards GR, Thorrold BS, McMillan WH, Kerr GA, Judson G, Cookson T, Moorhead A, Norriss M (2017) An economically based evaluation index for perennial and short-term ryegrasses in New Zealand dairy farm systems. Grass and Forage Science 72, 1-21.
| Crossref | Google Scholar |
Chapman DF, Wims CM, Ludemann CI, Kuhn-Sherlock B (2023a) The contribution of perennial ryegrass (Lolium perenne L.) breeding to whole pasture productivity under dairy cattle grazing in New Zealand. 1. Variation in yield, nutritive value and persistence-related traits. Grass and Forage Science 78, 64-84.
| Crossref | Google Scholar |
Chapman DF, Ludemann CI, Wims CM, Kuhn-Sherlock B (2023b) The contribution of perennial ryegrass (Lolium perenne L.) breeding to whole pasture productivity under dairy cattle grazing in New Zealand. 2. Rates of gain in production traits and economic value. Grass and Forage Science 78, 85-100.
| Crossref | Google Scholar |
Corson DC, Waghorn GC, Ulyatt MJ, Lee J (1999) NIRS: forage analysis and livestock feeding. Proceedings of the New Zealand Grassland Association 61, 127-132.
| Crossref | Google Scholar |
Cosgrove GP, Trolove MR, Staincliffe MR, Tozer KN (2020) Persistence of perennial ryegrass, tall fescue and cocksfoot following sequential annual sowings: pasture yield, composition and density in three establishment years under cattle grazing in Waikato. Journal of New Zealand Grasslands 82, 149-159.
| Crossref | Google Scholar |
Da Silva SC, Hodgson J, Matthews PNP, Matthew C, Holmes CW (1994) Effect of contrasting spring grazing management on summer-autumn pasture and milk production of mixed ryegrass-clover dairy swards. Proceedings of the New Zealand Society of Animal Production 54, 79-82.
| Google Scholar |
Dodd MB, Chapman DF, Ludemann CI, Griffiths W, Tozer KN, Donnelly L (2018) The measurement of perennial ryegrass persistence. Journal of New Zealand Grasslands 80, 161-168.
| Crossref | Google Scholar |
Donaghy D, Bryant R, Cranston L, Egan M, Griffiths W, Kay J, Pembleton K, Tozer K (2021) Will current rotational grazing management recommendations suit future intensive pastoral systems? NZGA: Research and Practice Series 17, 225-242.
| Crossref | Google Scholar |
Easton HS, Stewart AV, Kerr GA (2011) Ryegrass in pastures – breeding for resilience. NZGA: Research and Practice Series 15, 139-147.
| Crossref | Google Scholar |
Edwards GR, Chapman DF (2011) Plant responses to defoliation and relationships with pasture persistence. NZGA: Research and Practice Series 15, 39-46.
| Crossref | Google Scholar |
Faville MJ, Crush JR, Hong W, Phillips H, Lee JM, Chapman D (2020a) Effects of pasture age on the genotype and phenotype of perennial ryegrass. Grass and Forage Science 75, 135-144.
| Crossref | Google Scholar |
Faville MJ, Griffiths AG, Baten A, Cao M, Ashby R, Ghamkhar K, Hong W, Larking A, Williamson M, Webber Z (2020b) Genomic assessment of white clover and perennial ryegrass genetic resources. Journal of New Zealand Grasslands 82, 27-34.
| Crossref | Google Scholar |
Forde MB, Suckling FET (1980) Genetic resources in high-rainfall hill pastures of New Zealand: II. Description of the ryegrass collection. New Zealand Journal of Agricultural Research 23, 179-189.
| Crossref | Google Scholar |
Fulkerson WJ, Donaghy DJ (2001) Plant-soluble carbohydrate reserves and senescence – key criteria for developing an effective grazing management system for ryegrass-based pastures: a review. Australian Journal of Experimental Agriculture 41, 261-275.
| Crossref | Google Scholar |
Gowen N, O’Donovan M, Casey I, Rath M, Delaby L, Stakelum G (2003) The effect of grass cultivars differing in heading date and ploidy on the performance and dry matter intake of spring calving dairy cows at pasture. Animal Research 52, 321-336.
| Crossref | Google Scholar |
Griffiths WM, Roach CG, Chapman DF (2020) Should forage evaluation indices include a feeding value trait? Grass and Forage Science 75, 242-252.
| Crossref | Google Scholar |
Grogan D, Gilliland TJ (2011) A review of perennial ryegrass variety evaluation in Ireland. Irish Journal of Agricultural and Food Research 50, 65-81.
| Crossref | Google Scholar |
Hendriks SJ, Donaghy DJ, Cranston LM, Edwards GR, Chapman DF (2017) Perennial ryegrass breeding and the scaling issue: a review of system experiments investigating milk production and profit differences among cultivars. Animal Production Science 57, 1289-1296.
| Crossref | Google Scholar |
Hume DE, Hickey MJ, Lyons TB, Baird DB (2010) Agronomic performance and water-soluble carbohydrate expression of selected ryegrasses at two locations in New Zealand. New Zealand Journal of Agricultural Research 53, 37-57.
| Crossref | Google Scholar |
Keatinge JDH, Camlin MS, Stewart RH (1979) The influence of climatic factors on physiological development in perennial ryegrass. Grass and Forage Science 34, 55-59.
| Crossref | Google Scholar |
Laidlaw AS (2004) Effect of heading date of perennial ryegrass cultivars on tillering and tiller development in spring and summer. Grass and Forage Science 59, 240-249.
| Crossref | Google Scholar |
Laidlaw AS (2005) The relationship between tiller appearance in spring and contribution to dry-matter yield in perennial ryegrass (Lolium perenne L.) cultivars differing in heading date. Grass and Forage Science 60, 200-209.
| Crossref | Google Scholar |
Leddin CM, Jacobs JL, Smith KF, Giri K, Malcolm B, Ho CKM (2018) Development of a system to rank perennial ryegrass cultivars according to their economic value to dairy farm businesses in south-eastern Australia. Animal Production Science 58, 1552-1558.
| Crossref | Google Scholar |
Leddin C, Giri K, Smith K (2022) Variation in the nutritive characteristics of modern perennial ryegrass cultivars in South-Eastern Australian dairy environments and prospects for inclusion in the Australian Forage Value Index (FVI). Agronomy 12, 136.
| Crossref | Google Scholar |
Lee JM, Matthew C, Thom ER, Chapman DF (2012) Perennial ryegrass breeding in New Zealand: a dairy industry perspective. Crop & Pasture Science 63, 107-127.
| Crossref | Google Scholar |
Matthew C, Sackville Hamilton NR (2011) Analysing persistence of grass swards in terms of tiller birth and death. NZGA: Research and Practice Series 15, 63-68.
| Crossref | Google Scholar |
Matthew C, Chu ACP, Hodgson J, Mackay AD (1991) Early summer pasture control: what suits the plant? Proceedings of the New Zealand Grassland Association 53, 73-77.
| Crossref | Google Scholar |
Moore KJ, Moser LE, Vogel KP, Waller SS, Johnson BE, Pedersen JF (1991) Describing and quantifying growth stages of perennial forage grasses. Agronomy Journal 83, 1073-1077.
| Crossref | Google Scholar |
Nichols S, Crush J, Eady C, Faville M, Ghamkhar K, Woodfield D (2016) Future forage plants for hill country systems. NZGA: Research and Practice Series 16, 233-242.
| Crossref | Google Scholar |
O’Donovan M, Delaby L (2005) A comparison of perennial ryegrass cultivars differing in heading date and grass ploidy with spring calving dairy cows grazed at two different stocking rates. Animal Research 54, 337-350.
| Crossref | Google Scholar |
O’Donovan M, McHugh N, McEvoy M, Grogan D, Shalloo L (2017) Combining seasonal yield, silage dry matter yield, quality and persistency in an economic index to assist perennial ryegrass variety selection. The Journal of Agricultural Science 155, 556-568.
| Crossref | Google Scholar |
Patterson HD, Thompson R (1971) Recovery of inter-block information when block sizes are unequal. Biometrika 58, 545-554.
| Crossref | Google Scholar |
Simpson WR, Schmid J, Singh J, Faville MJ, Johnson RD (2012) A morphological change in the fungal symbiont Neotyphodium lolii induces dwarfing in its host plant Lolium perenne. Fungal Biology 116, 234-240.
| Crossref | Google Scholar |
Soper K (1958) Effects of flowering on the root system and summer survival of ryegrass. New Zealand Journal of Agricultural Research 1, 329-340.
| Crossref | Google Scholar |
Stewart A (2006) Genetic origins of perennial ryegrass (Lolium perenne) for New Zealand pastures. NZGA: Research and Practice Series 12, 55-61.
| Crossref | Google Scholar |
Thom ER, Van Vught VT, McCabe RJ (1996) Growth and persistence of perennial ryegrass lines with different tolerances to ‘pulling’ during grazing. Proceedings of the New Zealand Grassland Association 58, 67-72.
| Crossref | Google Scholar |
Thom ER, Popay AJ, Waugh CD, Minneé EMK (2014) Impact of novel endophytes in perennial ryegrass on herbage production and insect pests from pastures under dairy cow grazing in northern New Zealand. Grass and Forage Science 69, 191-204.
| Crossref | Google Scholar |
Tozer KN, Chapman DF, Bell NL, Crush JR, King WM, Rennie GM, Wilson DJ, Mapp NR, Rossi L, Aalders LT, Cameron CA (2014) Botanical survey of perennial ryegrass-based dairy pastures in three regions of New Zealand: implications for ryegrass persistence. New Zealand Journal of Agricultural Research 57, 14-29.
| Crossref | Google Scholar |
Tozer KN, Hume DE, Cameron C, Greenfield R, Dale T, Mace WJ, Craven T, Faville MJ (2024) Effects of heading date and Epichloë endophyte on persistence of diploid perennial ryegrass (Lolium perenne). 2. Endophyte strain and interactions with heading date. Crop & Pasture Science 75, CP24101.
| Crossref | Google Scholar |
Waghorn GC, Clark DA (2004) Feeding value of pastures for ruminants. New Zealand Veterinary Journal 52, 320-331.
| Crossref | Google Scholar | PubMed |
Waller RA, Sale PWG (2001) Persistence and productivity of perennial ryegrass in sheep pastures in south-western Victoria: a review. Australian Journal of Experimental Agriculture 41, 117-144.
| Crossref | Google Scholar |
Wims CM, Lee JM, Rossi L, Chapman DF (2014) Variation in the reproductive development of perennial ryegrass (Lolium perenne L.) cultivars. Proceedings of the New Zealand Grassland Association 76, 189-192.
| Crossref | Google Scholar |
Woodfield DR, Easton HS (2004) Advances in pasture plant breeding for animal productivity and health. New Zealand Veterinary Journal 52, 300-310.
| Crossref | Google Scholar | PubMed |