Phenotypic characterisation and evaluation of resistance to Fusarium ear rot, fumonisin contamination and agronomic traits in a collection of maize landraces
Lorenzo Stagnati A B # , Alessandra Lanubile
A
B
C
Handling Editor: Zed Rengel
Abstract
Maize is a major crop in Italy and is constantly affected by the fungus Fusarium verticillioides, producing ear rot and grain contamination by fumonisins. Finding new genotypes resistant to Fusarium infection is an important goal for the improvement of maize cultivation.
The objective of this work was to test a collection of 33 traditional landraces from the Emilia-Romagna (Italy) region for Fusarium ear rot (FER) severity, fumonisin content, and their agronomic performance.
Primary ears were artificially inoculated with a toxigenic strain of F. verticillioides in a 2-year experimental trial. The landrace ‘Nostrano di Storo’ and a commercial hybrid of FAO maturity class 300 were also included and used as comparisons representing a well-known and highly valued landrace and a modern flint hybrid, respectively.
The collection showed great phenotypic variability for all the agronomic traits assessed and responded differently to the Fusarium infection with percentages of FER ranging from 6.6% to 49.3%, and fumonisins from 4.3 mg/kg to 34.5 mg/kg. Thirteen and six landraces displayed FER percentages and fumonisin content very similar to the hybrid, respectively. Moreover, eight landraces exhibited grain yield values comparable to the hybrid. Interestingly, Va221, Va227 and EMR03 showed the best combination among these three traits.
This local material can be considered suitable for breeding purposes targeting the development of FER and fumonisin resistant germplasm.
The collection may represent a resource for future research aimed at evaluating the response to multiple pathogens and their associated mycotoxins.
Keywords: agrobiodiversity, ear morphology, Emilia-Romagna region, fumonisins, Fusarium verticillioides, grain yield, kernel type, maize germplasm.
Introduction
In the first decades of the 16th century, maize spread from the Iberian Peninsula to southern France, northern Italy, and the Balkans (Brandolini and Brandolini 2009). In Italy, numerous new landraces were originated and adapted to different agroecological situations and diversified uses of maize grains among the several cultures spread in the peninsula to the point that Italy can be defined as a secondary centre of maize diversification (Brandolini and Brandolini 2006, 2009; Eschholz et al. 2010; Cömertpay et al. 2012; Ardenghi et al. 2018). Traditional landraces dominated the agricultural landscape until the end of the second World War, when they were quickly substituted by better performing hybrids deriving from USA. Fortunately, the same institutions involved in the introduction of high-yielding materials understood the necessity of preserving traditional materials for future generations. At the beginning of the ‘50s, a comprehensive sampling survey took place and ended in the collection of 562 maize samples from all Italy (Brandolini and Brandolini 2009). Several traditional genotypes were also sampled in the last few years, especially in mountain areas, supporting the interest of local farmers in preserving these resources (Bertolini et al. 2002; Cassani et al. 2017; Ardenghi et al. 2018; Stagnati et al. 2022).
Nowadays, maize cultivation is based on first filial generation (F1) hybrids deriving from breeding plans that rely on a few elite inbred lines. It is estimated that only 2–5% of maize biodiversity is utilised for breeding programs, leaving the remaining genetic variability hidden in differences within and between landraces (Ignjatovic-Micic et al. 2008; Devi et al. 2013). The narrow genetic base of the parental lines of commercial hybrids highlights the necessity of conservation and characterisation of these local genetic resources for future breeding programs. Several studies can be enumerated focused on the genetic characterisation of traditional maize varieties (Barcaccia et al. 2003; Hartings et al. 2008; Qi-Lun et al. 2008; Eschholz et al. 2010; Cömertpay et al. 2012; Oppong et al. 2014; Palumbo et al. 2017; Stagnati et al. 2021) or on their morphological evaluation (Ruiz de Galarreta and Alvarez 2001; Brandolini and Brandolini 2009; Ardenghi et al. 2018), but little is available concerning agronomic traits, including the evaluation of the resistance to fungal pathogens and their associated mycotoxins (Lucchin et al. 2003; Djemel et al. 2012; Devi et al. 2013; Torri et al. 2015; Bernardi et al. 2018; Stagnati et al. 2021). Nowadays, worldwide agriculture is under pressure from many challenges such as drought, heat and cold stress. Moreover, new pathogen outbreaks, among which the occurrence of ear rot diseases caused by several fungal species such as Fusarium verticillioides, F. graminearum, Aspergillus flavus, and their derived mycotoxins, like fumonisins, deoxynivalenol, zearalenone and aflatoxins, represent still unsolved issues of modern agriculture (Lanubile et al. 2014; Logrieco et al. 2021). Regarding the resistance to ear rots and mycotoxin contamination, breeding programs focused on the development of adequate screening methods (Ju et al. 2017; Maschietto et al. 2017; Septiani et al. 2019; Stagnati et al. 2019, 2020a), on the research of resistant germplasm (Lanubile et al. 2011; Ouko et al. 2020; Stagnati et al. 2020b), and on the identification of genetic markers (Zila et al. 2013, 2014; Lanubile et al. 2017; Gaikpa and Miedaner 2019). However, despite efforts and advances in the selection process, currently grown materials are not highly resistant.
In this framework, the identification of new sources of resistance is crucial to broaden the genetic bases of maize and introduce new resistant alleles. Potential sources of resistance may be detected in germplasm collections in areas where the pathogen is endemic and different maize host populations are exposed to natural infections (Presello et al. 2004). Traditional maize germplasm experienced centuries of adaptation to local conditions and may have a greater possibility to harbour interesting alleles for both agronomic and resistance traits (Presello et al. 2004; Djemel et al. 2012; Gaikpa et al. 2021). Despite the effort of germplasm banks to collect and maintain maize landraces, the use of such genetic resources is limited. Many factors could contribute to germplasm underutilisation, starting from a lack of documentation of the collections, the absence of a proper phenotypic description and agronomic evaluation, as well as the frequent inadequate seed availability (Bonciarelli 1961; Nass and Paterniani 2000; Bertolini et al. 2002, 2005; Lucchin et al. 2003; Centro di Riferimento per l’Agricoltura Biologica (CRAB) 2004; Giupponi et al. 2021). In this regard, the present work investigated the agronomic performance of a collection of 33 traditional landraces deriving from the Emilia-Romagna (Italy) region in a 2-year experimental trial. Artificial inoculation with F. verticillioides was performed and the evaluation of Fusarium ear rot (FER) disease along with the fumonisin content was carried out to identify possible resistance sources useful to plant breeders.
Materials and methods
Germplasm
A set of 33 traditional maize landraces from the Emilia-Romagna (Italy) region was evaluated under field condition (Table 1). The landraces presented different origins: the ‘Va’ series was retrieved from the germplasm bank of CREA-Cereal and Industrial Crops (CREA-CI) in Bergamo, and was collected during the 1954 census; the ‘EMR’ series was provided by the Plant Germplasm Bank of the Department of Earth and Environmental Sciences at Università degli Studi di Pavia, Pavia, Italy, and is of recent sampling; five additional landraces (Quarantina Genovese-Q_G, Mais da pipe-M_P, Ustnenina gialla-U_G, Ustneina arancione-U_A, and Ustneina rossa-U_R) derived from the germplasm collection maintained at Department of Sustainable Crop Production at Università Cattolica del Sacro Cuore, Piacenza, Italy. The landrace ‘Mais Nostrano di Storo’ (N_S), grown in Valli Giudicarie, Trento, Italy, and a commercial hybrid of the FAO maturity class 300 were also included in this study and used as comparisons representing a well-known and valorised landrace and a modern flint hybrid, respectively (Table 1).
Landrace | Denomination | Collection year | Sampling location | Altitude (m) | Ear morphology | Kernel type | |
---|---|---|---|---|---|---|---|
Va211 | Dente di cavallo nostrano | 1954 | San Lorenzo-Riccione (FC) | 12 | Conical | Dent | |
Va212 | Ottofile | 1954 | Ricò-Meldola (FC) | 86 | Conical | Intermediate (Flint-like) | |
Va213 | Cinquantino | 1954 | Ravaldino in Monte (FC) | 192 | Conical | Flint | |
Va214 | Locale Rocca di San Casciano | 1954 | Rocca San Casciano (FC) | 133 | Slightly conical | Flint | |
Va215 | Giallo nostrano | 1954 | Predappio (FC) | 133 | Conical | Intermediate (Flint-like) | |
Va216 | Giallo comune | 1954 | Santa Sofia (FC) | 257 | Conical | Dent | |
Va217 | Spinato | 1954 | Cesena (FC) | 42 | Cylindrical | Dent | |
Va218 | Nostrano | 1954 | Sogliano sul Rubicone (FC) | 362 | Conical | Dent | |
Va219 | Nostrano o Locale | 1954 | Modena (MO) | 34 | Conical | Dent | |
Va220 | Cinquantino bianco | 1954 | Modena (MO) | 34 | Cylindrical | Flint | |
Va221 | Turco | 1954 | Borgo Val di Taro (PR) | 411 | Conical | Intermediate (Flint-like) | |
Va222 | Ferragostano | 1954 | Albareto (PR) | 512 | Conical | Intermediate (Flint-like) | |
Va223 | Piacentino o Nostrano | 1954 | Salsomaggiore (PR) | 157 | Conical | Dent | |
Va224 | Nostrano | 1954 | Talignano di Sala (PR) | 269 | Conical | Flint | |
Va225 | Nano precoce | 1954 | Ottone (PC) | 510 | Conical | Intermediate (Flint-like) | |
Va226 | Agostano | 1954 | Cerignole (PC) | 725 | Conical | Intermediate (Flint-like) | |
Va227 | Agostano 16 file | 1954 | Bobbio (PC) | 272 | Conical | Intermediate (Flint-like) | |
Va228 | Ottofile | 1954 | Bobbio (PC) | 272 | Conical | Intermediate (Flint and Dent) | |
Va229 | Piacentino | 1954 | Bobbio (PC) | 272 | Cylindrical | Flint | |
Va230 | Nostrano | 1954 | Gramizzola-Ottone (PC) | 645 | Conical | Intermediate (Flint-like) | |
Va231 | Nostrano ottofile | 1954 | Carignole-Bobbio (PC) | 725 | Cylindrical | Flint | |
EMR01 | Tagliolino di Vetto | / | Vetto (RE) | 447 | Slightly conical | Dent (Dent-like) | |
EMR03 | Cinquantino rosso di Ramiseto | / | Frazione Ramiseto, Ventasso (RE) | 865 | Slightly conical | Intermediate (Flint-like) | |
EMR04 | Rosso di Rasora | / | Frazione Rasora, Castiglione dei Pepoli (BO) | 775 | Slightly conical | Intermediate | |
EMR06 | Mais da scoppio | / | Casola Valsenio (RA) | 195 | Cylindrical | Flint (Pop) | |
EMR07 | Santa Sofia Romualdi | / | Santa Sofia (FC) | 303 | Conical | Intermediate (Intermediate and Dent-like) | |
EMR10 | Principe di Scavolino | / | Pennabilli (RN) | 762 | Slightly conical | Intermediate (Flint-like) | |
EMR13 | Piacentino di Coli | / | Frazione Fontana, Coli (PC) | 724 | Slightly conical | Flint (Flint and Flint-like) | |
Q_G | Quarantina Genovese | 2018 | Santo Stefano D’Aveto (GE) | 1012 | Conical | Intermediate | |
M_P | Mais da pipe | 1980–90 | Alta Val Trebbia/Nure | 1107 | Conical | Flint | |
U_A | Ustneina arancione | 2016 | Val Tidone (PC) | 192 | Cylindrical | Flint | |
U_G | Ustneina gialla | 2016 | Val Tidone (PC) | 192 | Cylindrical | Flint | |
U_R | Ustneina rossa | 2016 | Val Tidone (PC) | 192 | Cylindrical | Flint | |
N_S | Nostrano di Storo | / | Storo (TN) | 409 | Cylindrical | Intermediate (Flint-like) | |
FAO 300 | Commercial hybrid | / | Po plain | 50 | Cylindrical | Flint |
If the current kernel type classification was different from the original one (Centro di Riferimento per l’Agricoltura Biologica (CRAB) 2004; Bertolini et al. 2005), this is reported in parentheses.
Field management
Field trials were carried out in 2 years, 2019 and 2020, at Centro Ricerche Zootecniche (45.005066° N, 9.704206° E, San Bonico, Piacenza, Italy). In 2019, fields were sown on 17 April, and in 2020, on 15 April. Each experimental field represented a completely randomised design with four replications originated by the blockdesign package (Edmondson 2019) of the R software (R Core Team 2017). Each plot consisted of 4 rows of 5 m long, spaced 0.8 m apart, and separated by a 1 m aisle, and 25 seeds were planted for each row. The central rows of each plot were used in order to assess agronomic traits relying on the indications of the UPOV-CPVO TP/2/3 protocol as follows: tasselling (50% of plants shedding pollen), silking (50% of plants with visible silks) and physiological maturity (presence of the black layer on mid-ear kernels). Plant height (tassel included), primary ear height and ear/plant insertion ratio were measured on five plants per plot at 3 weeks after pollination, and the number of plants per plot and the percentage of lodged plants were recorded over the total plot as well. At maturity all ears of the central rows were hand harvested and shelled. Grain moisture (GM; %) and hectolitre weight (kg/hL) were measured with a GAC® 2100-AGRI grain moisture analyser (DICKEY-john, Auburn, IL, USA). Yield potential was calculated at 14% GM and adjusted to a final density of 70,000 plants per hectare.
Artificial inoculation, Fusarium ear rot severity and fumonisin content analysis
Artificial inoculation was performed according to the pin-bar inoculation method at 15 days after pollination (DAP) (Stagnati et al. 2020b; Guche et al. 2022). Briefly, the primary ear of five plants per plot was inoculated with a spore suspension of F. verticillioides MPVP 294 (ITEM 10027). This strain is maintained in the culture collection of the Department of Sustainable Crop Production, Università Cattolica del Sacro Cuore of Piacenza, Italy. The inoculum was produced as described in Lanubile et al. (2013, 2021) to a final concentration of 1 × 106 conidia/mL. Inoculated ears were hand harvested at maturity and air dried in a barn.
Fusarium ear rot (FER) severity was visually evaluated, assessing the percentage of the rotted surface of the ear, using a seven-point severity grid and assigning 1 for the absence of symptoms, 2 for 1–3% infection, 3 for 4–10% infection, 4 for 11–25% infection, 5 for 26–50% infection, 6 for 51–75% infection, and 7 for 76–100% infection (Fig. 1; Stagnati et al. 2020b; Guche et al. 2022).
Rating scale of Fusarium ear rot severity and spread of the infection (%) on the F. verticillioides inoculated maize ears. Class 1 = no symptoms; Class 2 = 1–3% infection; Class 3 = 4–10% infection; Class 4 = 11–25% infection; Class 5 = 26–50% infection; Class 6 = 51–75% infection; Class 7 = 76–100% infection.
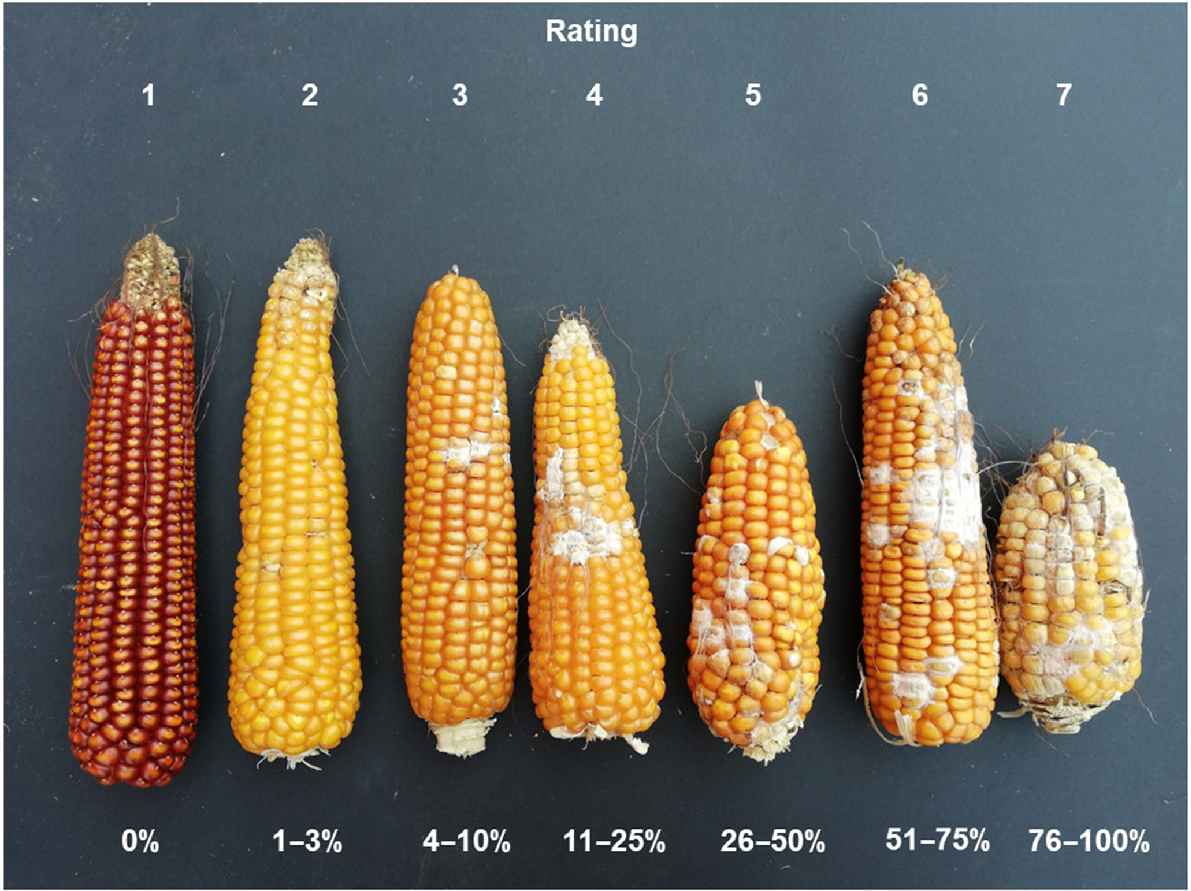
For the mycotoxin analysis, grains derived from the inoculated ears belonging to the same plot were bulked and further dried for 2 days at 65°C. A random subsample of 150–200 g was milled with a laboratory mill (Cyclotec™ 1093 Sample Mill, FOSS, Hilleroed, Denmark) using a 1 mm mesh. To avoid cross-contamination of kernels showing different disease levels special attention was taken in the milling procedure. Total fumonisins (B1 + B2 + B3) were determined in kernels using the VICAM Fumo-V AQUA strips (VICAM, Watertown, MA, USA), a fluorometric-immunocapture assay having a limit of detection of 0.2 mg/kg and a quantitation range from 0 to 100 mg/kg (Guche et al. 2022). Values of fumonisins given in the text are expressed in mg/kg.
Statistical analysis
Data manipulation and visualisation was carried out using the software R (R Core Team 2017). Before proceeding with ANOVA analysis all data were transformed using the Box–Cox function (Morales et al. 2018; Stagnati et al. 2020b) using the MASS (Venables and Ripley 2002) and rcompanion packages (Mangiafico 2018). λ coefficients for transformations were: −5.2 for days to tasselling, −3.2 for days to silking, 3.3 for days to physiological maturity, 1.2 for plant height, 1 for ear height, 1.3 for ear/plant insertion ratio, 0.1 for percentage of lodged plants, 0.5 for yield potential, −0.6 for grain moisture, 0.1 for both FER and fumonisin content. A +1 constant was added for calculating the percentage of lodged plants to correct for a 0 score, in the case of plots with no lodging.
Two-way ANOVA and Fisher’s least significant difference (l.s.d.) test with the Bonferroni correction were performed using the car (Fox and Weisberg 2011) and agricolae (de Mendiburu 2017) R packages.
Correlation between different traits and years were investigated by the chart.correlation function available in the PerformanceAnalytics (Peterson and Carl 2018) package.
Results
Evaluation of Fusarium ear rot severity and fumonisin accumulation
Significant differences in the expression of FER symptoms were revealed among landraces and years (P = 8.5 × 10−6 and 1.0 × 10−7, respectively), but not for the landrace × year interaction (P = 4.1 × 10−1) (Table 2). Mean values of FER severity were 27.2 and 17.2 in 2019 and 2020, respectively (Supplementary Table S1). In 2019, U_G and Va229 showed the lowest and highest FER percentage (6.9% and 69.6%, respectively), whereas in 2020, EMR07 was the most resistant landrace (6.2% of FER), followed immediately after by U_G (6.4%), and EMR06 was the most susceptible (56.0% of FER).
Landrace | FER (%) | FUM (mg/kg) | T (days) | S (days) | PM (days) | PH (cm) | EH (cm) | E/P_IR (%) | L (%) | Y (t/ha) | GM (%) | |
---|---|---|---|---|---|---|---|---|---|---|---|---|
Va211 | 19.0abc | 4.3a | 70.8abcdefg | 75.0bcde | 123.9bcd | 216.5cdefgh | 103.0abc | 47.5b | 26.7ab | 6.9a | 18.3a | |
Va212 | 16.2abc | 9.3ab | 68.6bcdefg | 72.9bcde | 125.8b | 215.4cdefghi | 93.2abcde | 43.2bcde | 18.4abcd | 5.7abc | 17.3ab | |
Va213 | 24.2abc | 34.5ab | 70.0bcdefg | 73.9bcde | 124.3bc | 211.9defghij | 85.9bcdefgh | 40.5bcdefg | 9.3abcdefg | 5.1abcd | 16.3ab | |
Va214 | 14.4bc | 14.5ab | 67.6cdefg | 70.9bcde | 121.9bcde | 193.6hij | 76.2efghij | 39.2bcdefgh | 18.7abc | 5.3abc | 16.1ab | |
Va215 | 32.0ab | 12.3ab | 69.1bcdefg | 74.3bcde | 123.8bcd | 216.7cdefgh | 89.1bcdefg | 41.1bcdefg | 15.7abcd | 5.2abcd | 16.1ab | |
Va216 | 31.1ab | 11.9ab | 68.9bcdefg | 74.9bcde | 122.0bcde | 213.0defghij | 82.8cdefghij | 38.8cdefgh | 16.2abcd | 4.6bcd | 16.3ab | |
Va217 | 15.8abc | 29.5ab | 79.1a | 85.3a | 130.5a | 209.8efghij | 114.6a | 56.4a | 7.2cdefg | 7.5a | 18.3a | |
Va218 | 23.4abc | 5.8ab | 71.0abcdefg | 75.3abcde | 123.1bcde | 218.7cdefg | 86.5bcdefgh | 39.6bcdefgh | 28.1a | 7.0ab | 16.3ab | |
Va219 | 29.1abc | 6.0ab | 70.0bcdefg | 74.1bcde | 121.9bcde | 228.4abcd | 89.8bcdefg | 39.1bcdefgh | 13.2abcd | 5.6abc | 17.3ab | |
Va220 | 25.9abc | 15.3ab | 66.9defg | 70.9bcde | 115.1gh | 189.7j | 71.2ghijk | 37.5cdefghi | 2.1defg | 3.2de | 15.1ab | |
Va221 | 11.5bc | 7.7ab | 70.4bcdefg | 73.3bcde | 124.0bcd | 223.3abcdef | 84.4bcdefghi | 36.4defghi | 9.3abcdefg | 5.0abcd | 15.1ab | |
Va222 | 22.2abc | 8.7ab | 73.6abcde | 77.6abc | 123.9bcd | 237.9abc | 85.7bcdefghi | 36.0defghi | 13.1abcde | 6.5ab | 17.2ab | |
Va223 | 37.4ab | 21.4b | 70.9abcdefg | 74.3bcde | 124.3bc | 216.4cdefgh | 99.0abcd | 45.6bc | 6.1bcdefg | 5.5abc | 15.5ab | |
Va224 | 17.7abc | 16.6ab | 71.0abcdefg | 74.4bcde | 122.5bcde | 217.7cdefg | 87.2bcdefgh | 39.9bcdefg | 12.5abcdef | 4.8abcd | 15.8ab | |
Va225 | 24.2abc | 9.2ab | 68.3cdefg | 72.9bcde | 124.0bcd | 210.9defghij | 88.2bcdefgh | 37.7cdefgh | 8.7abcdefg | 6.3abc | 16.4ab | |
Va226 | 27.9abc | 7.3ab | 68.6bcdefg | 72.3bcde | 125.0b | 211.2defghij | 85.1bcdefghi | 40.4bcdefg | 6.0cdefg | 5.5abc | 16.0ab | |
Va227 | 17.3abc | 4.8ab | 72.3abcde | 77.3ab | 123.1bcde | 218.1cdefg | 84.5bcdefghi | 38.8bcdefgh | 12.5abcde | 6.3abc | 17.5ab | |
Va228 | 21.4abc | 10.7ab | 67.5cdefg | 70.6bcde | 123.3bcde | 200.1ghij | 65.0ijk | 32.5ghi | 8.3abcdefg | 5.0abcd | 15.5ab | |
Va229 | 41.6abc | 7.0ab | 74.1abc | 77.4ab | 125.3b | 241.5ab | 99.5abcd | 41.1bcdefg | 19.6abc | 5.5abc | 15.2ab | |
Va230 | 18.7abc | 6.7ab | 68.5bcdefg | 72.1bcde | 120.6cdef | 208.4defghij | 79.0defghij | 37.6cdefghi | 10.7abcdefg | 5.0abcd | 15.7ab | |
Va231 | 20.5abc | 22.9ab | 72.0abcde | 76.6abc | 125.1b | 220.7bcdefg | 81.3defghij | 36.8defghi | 5.0cdefg | 4.5bcd | 16.8ab | |
EMR01 | 20.1abc | 15.9ab | 72.5abcd | 74.8bcde | 125.1b | 210.3defghij | 96.4abcde | 45.6bc | 0.1fg | 6.0ab | 14.8ab | |
EMR03 | 11.0bc | 7.1ab | 70.1abcdefg | 72.5bcde | 123.0bcde | 228.2abcd | 97.9abcd | 42.6bcde | 7.7bcdefg | 5.9abc | 16.5ab | |
EMR04 | 17.5abc | 12.9ab | 72.4abcde | 76.9abc | 125.6b | 222.8abcdefg | 93.0abcdef | 41.7bcdef | 1.5efg | 6.6a | 15.6ab | |
EMR06 | 49.3a | 21.5ab | 75.1ab | 77.0ab | 123.6bcde | 191.5j | 82.1defghij | 42.8bcde | 3.2defg | 2.3ef | 15.3ab | |
EMR07 | 12.5bc | 15.7ab | 68.5cdefg | 72.3bcde | 119.4efg | 202.2fghij | 67.5hijk | 33.1fghi | 7.7bcdefg | 5.8abc | 15.1ab | |
EMR10 | 20.1abc | 8.0ab | 66.6fg | 69.5e | 123.0bcde | 209.6defghij | 72.2fghij | 28.1i | 21.1abc | 5.9abc | 16.2ab | |
EMR13 | 23.8abc | 7.6ab | 72.3abcdef | 76.0abcde | 124.1bcd | 211.6defghij | 83.2bcdefghij | 39.4bcdefgh | 5.2cdefg | 5.0abcd | 13.6ab | |
Q_G | 19.5abc | 6.0ab | 69.3cdefg | 72.0bcde | 115.1gh | 192.7ij | 63.1jk | 32.9fghi | 24.6abc | 5.1abcd | 17.8ab | |
M_P | 20.2abc | 7.5ab | 66.0g | 70.4cde | 111.1h | 164.6k | 50.6k | 30.0hi | 20.3ab | 0.5f | 20.0a | |
U_A | 9.7bc | 6.3ab | 68.0cdefg | 69.5de | 117.3fg | 206.6defghij | 71.4ghijk | 34.2efghi | 7.4bcdefg | 4.4bcd | 15.6ab | |
U_G | 6.6c | 18.9ab | 66.6efg | 71.0bcde | 119.8def | 215.2cdefghi | 79.5defghij | 36.7defghi | 10.7abcdefg | 5.1abc | 15.1ab | |
U_R | 30.3ab | 11.1ab | 69.8abcdef | 73.8abcd | 122.9bcde | 228.6abcde | 90.9bcdefgh | 38.3cdefgh | 11.4abcdefg | 3.9cde | 15.6ab | |
N_S | 26.9abc | 8.8ab | 74.0abcde | 77.4bcde | 124.4bc | 220.1bcdefg | 94.7abcd | 44.2bcd | 1.5efg | 5.0abcd | 15.3ab | |
FAO 300 | 19.0abc | 6.5ab | 72.3abc | 75.9abc | 125.5b | 245.3a | 104.0ab | 42.3bcde | 0.4g | 5.9ab | 16.3ab | |
Mean | 22.2 | 12.0 | 70.4 | 74.1 | 122.7 | 213.4 | 85.1 | 39.4 | 11.2 | 5.2 | 16.2 | |
Landrace | 8.5 × 10−6 | 3.2 × 10−2 | 6.7 × 10−16 | 1.9 × 10−14 | <2.0 × 10−16 | <2.0 × 10−16 | <2.0 × 10−16 | <2.0 × 10−16 | <2.0 × 10−16 | <2.0 × 10−16 | 3.0 × 10−2 | |
Year | 1.0 × 10−7 | <2.0 × 10−16 | 5.5 × 10−4 | 1.4 × 10−5 | <2.0 × 10−16 | <2.0 × 10−16 | <2.0 × 10−16 | 9.1 × 10−3 | 1.6 × 10−8 | <2.0 × 10−16 | <2.0 × 10−16 | |
Landrace × Year | 4.1 × 10−1 | 1.3 × 10−2 | 1.3 × 10−1 | 2.9 × 10−1 | 3.9 × 10−13 | 4.8 × 10−7 | 2.9 × 10−1 | 2.9 × 10−6 | 2.5 × 10−1 | 4.4 × 10−2 | 1.5 × 10−1 |
The following traits were considered: Fusarium ear rot (FER, %), fumonisin (B1 + B2 + B3) content (FUM, mg/kg), days to tasselling (T, days), days to silking (S, days), days to physiological maturity (PM, days), plant height (PH, cm), ear height (EH, cm), ear/plant insertion ratio (E/P_IR, %), percentage of lodged plants (L, %), grain yield (Y, t/ha), and grain moisture (GM, %).
Means followed by the same letter in the column do not differ significantly at the 0.05 probability level based on Bonferroni test.
Q_G, Quarantina Genovese; M_P, Mais da pipe; U_A, Ustneina arancione; U_G, Ustneina gialla; U_R, Ustneina rossa; N_S, Nostrano di Storo.
Artificially inoculated landraces were also analysed for the content of total fumonisins (B1 + B2 + B3) in kernels and significant differences were observed among landraces, years and landrace × year interaction, with the strongest trait effect due to the year (P < 2.0 × 10−16) (Table 2). Fumonisin levels ranged from 0.7 (Va221) to 12.0 (Va223) mg/kg in 2019 (mean value = 3.7 mg/kg), and from 4.2 (Va219) to 65.5 (Va213) mg/kg in 2020 (mean value = 20.3 mg/kg) (Table S1). Va211 and Va223 were found to be contrasting landraces considering an α = 0.07 (Table 2).
A good correlation (r = 0.66, P ≤ 0.001) was observed between FER severity and fumonisin accumulation in 2019, and between FER severity of both years (r = 0.40, P ≤ 0.05) (Fig. 2), whereas no correlation was observed between the two traits when both years were analysed together (Fig. 3).
Correlation between Fusarium ear rot (FER, %) and fumonisin (B1 + B2 + B3) content (FUM, mg/kg) traits measured in 33 traditional maize landraces, Mais Nostrano di Storo and the commercial hybrid FAO 300 during 2019 and 2020 field trials. The distribution of each trait is shown on the diagonal. On the bottom of the diagonal the bivariate scatter plots with a fitted line are displayed. On the top of the diagonal the value of the correlation plus the significance level are reported as stars (*** P ≤ 0.001; * P ≤ 0.05).
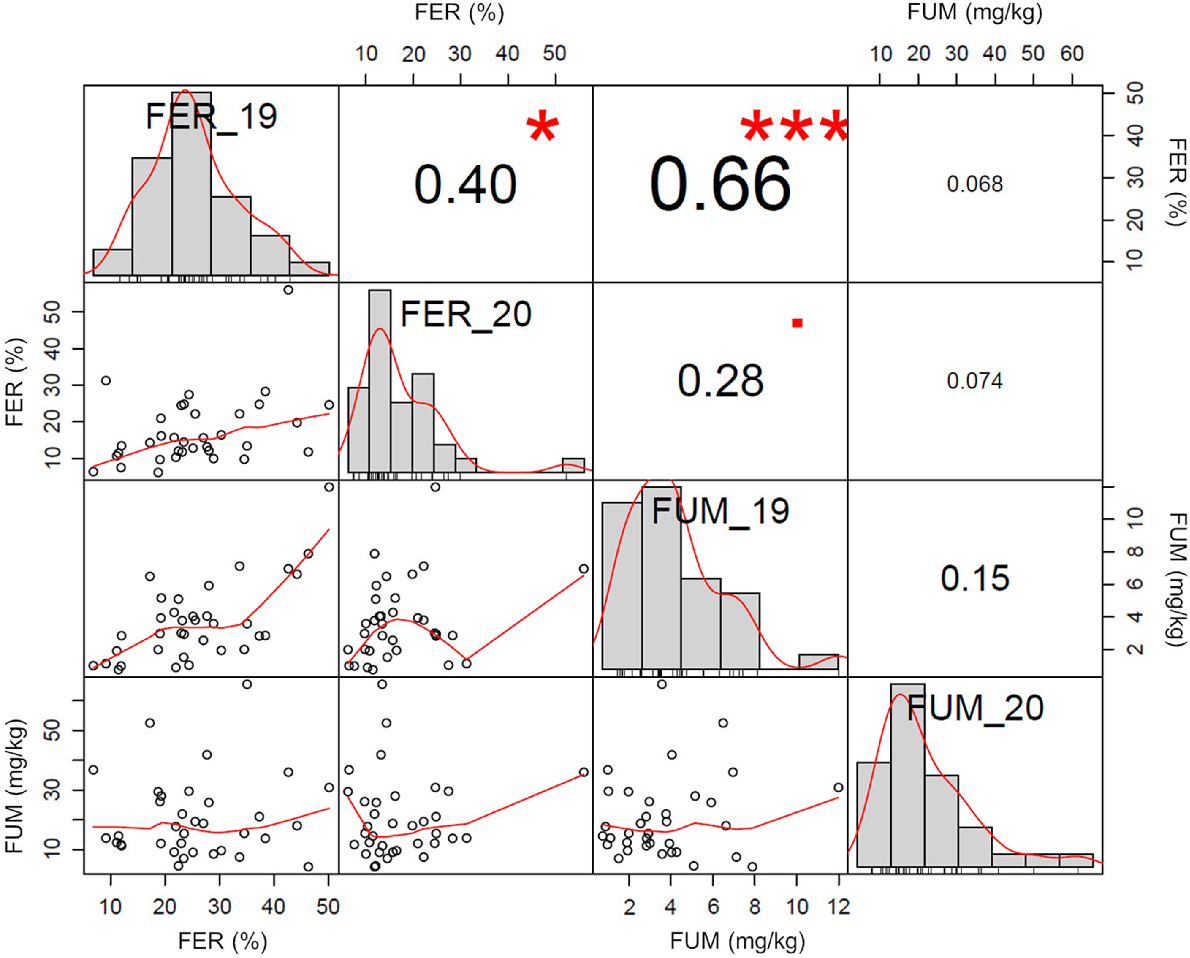
Correlation among Fusarium ear rot (FER, %), fumonisin (B1 + B2 + B3) content (FUM, mg/kg), days to tasselling (T, days), days to silking (S, days), days to physiological maturity (PM, days), plant height (PH, cm), ear height (EH, cm), ear/plant insertion ratio (E/P_IR, %), percentage of lodged plants (L, %), grain yield (Y, t/ha), and grain moisture (GM, %) traits measured in 33 traditional maize landraces, Mais Nostrano di Storo and the commercial hybrid FAO 300 during 2019 and 2020 field trials. The distribution of each trait is shown on the diagonal. On the bottom of the diagonal the bivariate scatter plots with a fitted line are displayed. On the top of the diagonal the value of the correlation plus the significance level are reported as stars (*** P ≤ 0.001; * P ≤ 0.05).
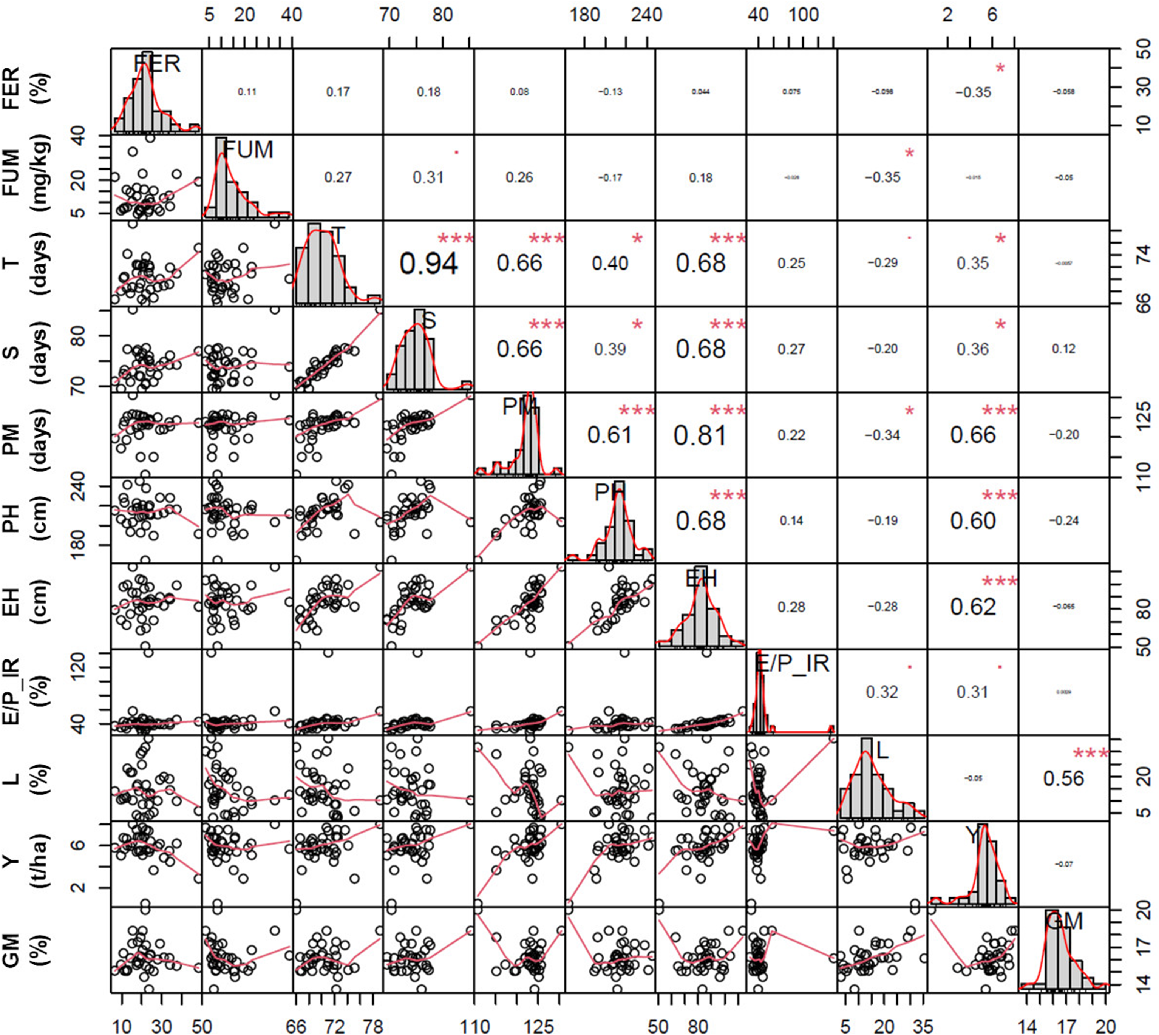
Evaluation of agronomic performance
The agronomic parameters of the maize landrace collection are described in Tables 2 and S1. In general, great phenotypic variability was observed for all agronomic traits measured in 2019 and 2020.
Significant differences were detected among the 33 maize landraces, N_S and the commercial hybrid for the traits associated with flowering, days to tasselling and days to silking, at both landrace (P = 6.7 × 10−16 and 1.9 × 10−14) and year (P = 5.5 × 10−4 and 1.4 × 10−5) level, whereas the landrace × year interaction was not significant (P = 1.3 × 10−1 and 2.9 × 10−1) (Table 2). During 2019, the earliest and latest landraces were U_G and Va217, and the mean values were 70.9 and 75.0 days to tasselling and silking, respectively (Table S1). During 2020, Va217 was confirmed as the latest landrace, whereas the earliest anthesis and silking were recorded for M_P and EMR10, respectively. On average, anthesis was reached at 69.8 days and silking at 73.3 days (Table S1).
Cycle length is determined at the appearance of the so called ‘black layer’ at the kernel pedicel. This trait significantly differed among landraces (P = 2.0 × 10−16), years (P = 2.0 × 10−16), and landrace × year interaction (P = 3.9 × 10−13) (Table 2). For both years, the earliest landrace was M_P, which reached maturity at 108.5 and 113.7 days, whereas the latest was confirmed as Va217, maturing at 135.5 and 125.5 days for 2019 and 2020, respectively (Table S1). Considering the entire germplasm collection, physiological maturity occurred at approximately 123 days (Table 2). The commercial hybrid reached maturity at 127.5 and 123.5 days in the 2 years 2019 and 2020, respectively; this is classified in the early maturity class FAO300 and suggests that the maize collection from Emilia-Romagna is characterised by a widespread presence of early landraces.
As expected, cycle traits (days to tasselling and silking, and physiologic maturity) were positively and significantly correlated with each other (P ≤ 0.001), and the highest correlation was observed for anthesis and silking traits (r = 0.94) facilitating the proper pollination of the collection (Fig. 3).
The mean values of plant and ear height in 2019 and 2020 varied significantly among landraces at both genotype and year level (P = 2.0 × 10−16), by contrast a significant landrace × year interaction was found only for plant height (P = 4.8 × 10−7), as reported in Table 2. The average of both traits was higher in 2020 than 2019, with mean values of 199.2 cm and 77.3 cm in 2019, and 227.5 cm and 92.8 cm in 2020 for plant and ear height, respectively (Table S1). The shortest variety was M_P for both traits in both years, whereas the hybrid was found to be the tallest genotype (230.9 cm and 259.7 cm in 2019 and 2020, respectively). The most elevated ear height was recorded for Va217 (108.6 cm and 120.7 cm in 2019 and 2020, respectively) (Tables 2 and S1). Plant and ear height were found to be significantly and positively correlated each other (r = 0.68; P ≤ 0.001) and with cycle duration traits (Fig. 3), illustrating how early landraces were generally characterised by reduced height.
From plant and ear insertion height it was possible to compute an index of plant architecture that is the ear/plant insertion ratio. This trait was influenced by landrace (P = 2.0 × 10−16), year (P = 9.1 × 10−3) and landrace × year interaction (P = 2.9 × 10−6), as shown in Table 2. Mean values of the collection were similar during the 2 years (Table S1), with EMR10 and Va217 being the two most distant landraces for this trait (Table 2). Moreover, ear insertion height and ear/plant insertion ratio showed significant associations with the different kernel types (dent, intermediate and flint) of landraces (P = 0.038 and 0.0068, respectively) (Fig. 4a, b).
Relationship between: (a) ear height (EH, cm) and kernel type (dent, intermediate, and flint); (b) ear/plant insertion ratio (E/P_IR, %) and kernel type; (c) percentage of lodged plants (L, %) and ear morphology; (d) grain yield (Y, t/ha) and kernel type; (e) altitude (ALT, m) and kernel type; (f) altitude (ALT, m) and ear morphology. Differences among groups were detected by a pairwise-t-test. The used bowblot convention was 1.5 IQR.
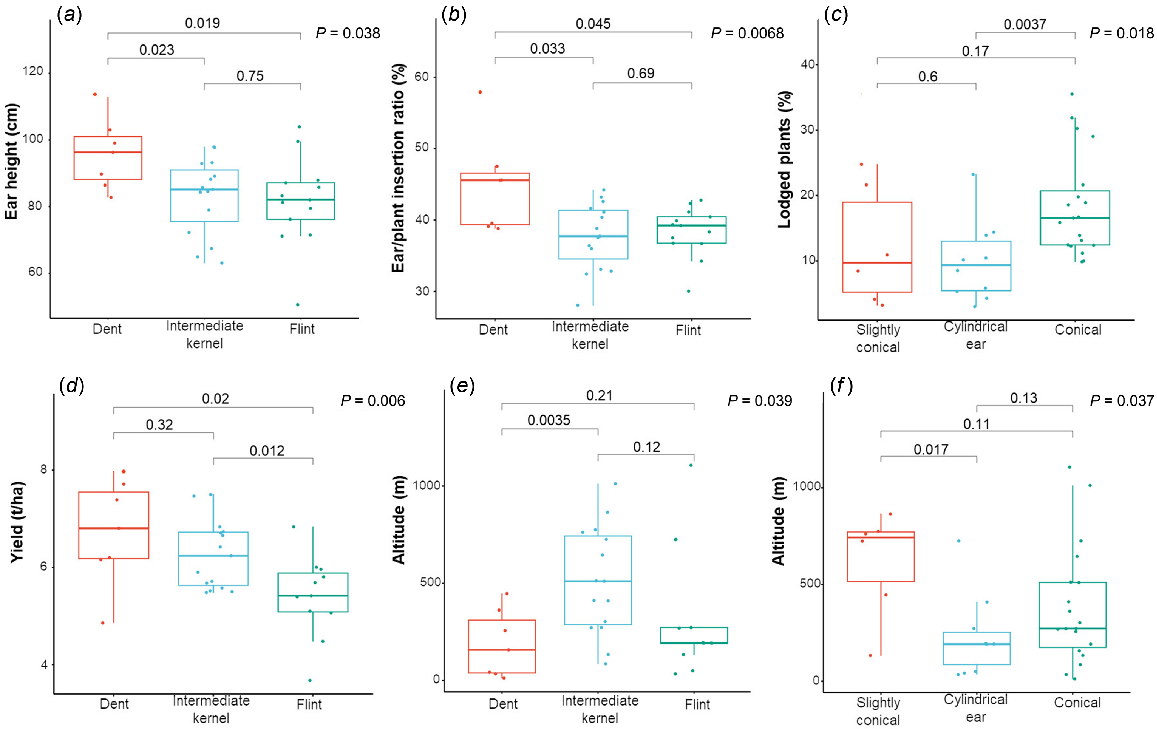
A further agronomic trait related to plant architecture is the susceptibility to lodging. In both years, a moderate percentage of lodging was recorded (Table 2). In 2019, lodging percentage varied from a minimum of 0.3% for EMR01 to 40.0% for Va218 with a mean percentage of 14.6% (Table S1). During 2020, the percentage of plant lodging was lower, varying from 0% for Va220, EMR01, EMR04, EMR07, N_S and the commercial hybrid, to 21.7% for Va211, with a mean percentage of 7.8% (Table S1). Significant differences were observed at both landrace (P = 2.0 × 10−16) and year (P = 1.6 × 10−8) level, whereas landrace × year interaction was not significant (Table 2). The higher incidence of lodging during 2019 was probably due to an adverse meteorological event like strong wind and heavy rains that occurred after flowering. Significant moderately negative correlations were found between lodging and time to tasselling (r = −0.29; P ≤ 0.05), and lodging and physiological maturity (r = −0.34; P ≤ 0.05) (Fig. 3). It was previously reported that early root lodging occurred during wind and rainstorm events around flowering time when corn was in an awkward (top-heavy) stage, and this phenomenon differed depending on the years and localities (Troyer 2000).
Lodging percentage was related to ear shape of landraces and significant differences among the three different ear morphologies (slightly conical, conical and cylindrical; P = 0.018) were observed (Fig. 4c). Conversely, kernel types and sampling location did not affect this trait (data not shown). Landraces with conical ears were more prone to lodging (Fig. 4c), and this could be due to a modification of the centre of gravity of the plants, mainly in those with a tall architecture.
Grain yield represents the driving factor for plant breeding and one of the key elements to take into account for landrace exploitation. Average production was about 4.7 t/ha in 2019, and 6.0 t/ha in 2020 (Table S1). The best performing landrace was EMR04 (6.3 t/ha) and Va217 (8.9 t/ha) in 2019 and 2020, respectively. Landraces and years were significantly different (P = 2.0 × 10−16), whereas landrace × year interaction was not significant (Table 2). Significant positive correlations were observed between yield and days to tasselling (r = 0.35; P ≤ 0.05), silking (r = 0.36; P ≤ 0.05), and physiological maturity (r = 0.66; P ≤ 0.001), as well as between yield and plant and ear heights (r = 0.60 and 0.62, P ≤ 0.001, respectively) (Fig. 3). Similar findings have been already reported (Cömertpay et al. 2012; Stagnati et al. 2020b), highlighting how a longer vegetative phase allows bigger plants and higher grain yields. A significant moderately negative correlation (r = −0.35; P ≤ 0.05) was detected between yield and FER disease, in line with Stagnati et al. (2020b) results. To understand the potential of the landrace collection as source of genetic variability without any detrimental effect on grain yield, the commercial hybrid characterised by a short cycle was used as comparison. Nearly all landraces showed average productions comparable to that of the hybrid, and some landraces displayed yield values higher than the hybrid, such as Va211, Va217, Va218, Va222, Va225, Va227, EMR01 and EMR04 (Table 2). Even though a few of them still present some morphological defects such as open leaf angle, lodging susceptibility and big tassels, these landraces represent good sources of genetic diversity and may be used to extract new inbred lines or improved ‘per se’ through mass selection.
Differences in yield values were found among landraces characterised by different kernel types (P = 0.006), as reported in Fig. 4d. Dent and intermediate landraces were characterised by a similar yield, whereas the flint ones had reduced yields. Further considerations are necessary for M_P and EMR06 landraces, which were removed from the correlation analysis. M_P showed very bad agronomic performance, explained by the presence of silk-cut and popped kernel symptoms and a low seed set under hot climate conditions. Even including these landraces in the analysis, yield distribution results according to kernel types were not affected (P = 0.0024), and the comparison between dent and intermediate kernel type landraces still remained not significant (data not shown). Interestingly, altitude of landrace sampling locations was significantly related to kernel types (P = 0.039) (Fig. 4e).
Average grain moisture was 14.8% in 2019 and 17.6% in 2020, with an effect of the year (P = 2.0 × 10−16) higher than that of landrace (P = 3.0 × 10−2) (Tables 2 and S1). Interestingly, in both years the landrace with the highest moisture content was M_P, an accession characterised by a strongly conical ear morphology. Therefore, a significant relationship between ear morphology and altitude of sampling location was displayed (P = 0.037) (Fig. 4f). Landraces characterised by the presence of conical ears were the most abundant and sampled from plain to high mountains (over 1000 m above the sea), landraces with slightly conical ears were few and generally sampled in areas between 500 and 700 m above the sea, whereas maize with cylindrical ears were localised mainly in the plain or hills (altitude less than 500 m).
Discussion
The present research evaluated for the first time the effect of artificial inoculation with F. verticillioides and fumonisin content on a maize landrace collection from the Emilia-Romagna region. Data were based only on pin-bar inoculation technique. This method is very suited to differentiating genotypes for kernel resistance by inserting pins previously soaked in the fungal suspension into the middle of the ear through husk leaves (Lanubile et al. 2014). Previous studies found that F. verticillioides inoculation by the pin-bar method gave a much higher infection severity and toxin contamination than silk channel inoculation, and it seems that kernel resistance testing has an enhanced stability and reproducibility (Robertson et al. 2006; Lanubile et al. 2014).
The presence of FER and fumonisins was found in all landraces in 2019 and 2020. The collection has never been utilised in breeding programs for these two traits. It could be assumed that, throughout history, the occurrence of ear rots in Italian maize landraces was not considered particularly relevant. Instead, at the beginning of the 20th century, the most devastating maize disease was the common smut caused by Ustilago maydis (DC.) Cda. To constrain the spread of common smut disease some agronomy indications were provided by Zapparoli (1930), whereas Bresaola (1930) supplied information on the selection of resistant genotypes. Concerns regarding common smut disease were considered of such importance that Bonciarelli (1961), in his agronomic comparisons of landraces from Umbria region (Italy), evaluated only this disease among the many affecting maize.
It is interesting to note that specific breeding programs led in Canada and aimed at developing Fusarium resistant genotypes, also selected for common smut, as reported by Reid et al. (2009), thus suggesting common resistance pathways. Moreover, Italian landraces are usually characterised by flint or flint-like kernels, for example U_G, which was one of the best landraces for the resistance to FER. It was reported that flint maize showed higher resistance to fungal infection and fumonisin contamination than dent maize, and this phenotypic trait was attributed to the presence of a hard outer endosperm layer and high amylase content typical of these kernels (Santiago et al. 2015).
Relationships between disease development and mycotoxin content were not always uniform across both years. Contrasting results were previously reported in literature. Several works found relatively high correlations between resistance to FER and the presence of fumonisins (Robertson et al. 2006; Hung and Holland 2012; Maschietto et al. 2017; Czembor et al. 2019; Stagnati et al. 2020b). However, differences in fumonisin content for genotypes with the same levels of disease severity were reported as well (Clements et al. 2003; Mesterházy et al. 2012; Torri et al. 2015).
The agronomic performance of the collection was evaluated as well. The general earliness observed in the landraces was consistent with the available historical and geographic information. In many areas on the south side of the Po plain, irrigation was difficult due to the absence of lakes and the tendency of many rivers to dry during summer. Therefore, crops had to rely on rainfall for water supply and varieties with short cycles were preferred to avoid summer drought. In fields where the irrigation was possible, many short cycle varieties were cultivated as a secondary crop after the wheat harvest. These materials, generally called ‘Quarantini’ or ‘Cinquantini’, were able to grow in a short season with high grain yield (Brandolini and Brandolini 2009). Moreover, several landraces of the collection were sampled in localities at high altitude or in mountain valleys where the favourable season for maize growth is usually shorter than in the Po plain, thus explaining the general earliness of the material object of this study. In the evaluation of maize landraces from Umbria, Bonciarelli (1961) reported that early material performed better under unfavourable conditions and the selection for earliness was strongly supported at the beginning of the 20th century by Zapparoli (1930). The latest landrace, Va217, can be considered an outlier of the Emilia-Romagna collection. The plant is tall, with abundant leaves, and the ear presents yellow dent kernels on a red cob. These traits are quite uncommon in the Italian maize germplasm and in addition, phylogenetic analysis revealed that Va217 was not related to the other landraces from the same area, probably having been introduced later along with improved dent materials from USA (Stagnati et al. 2022).
Reduced heights were generally observed in early landraces. Traditionally, maize cultivation was intercropped with other species, both herbaceous and woody, and to avoid excessive competition and shading among species, maize varieties with limited growth, such as ‘Quarantini’ and ‘Cinquantini’ were preferred. Moreover, excessively tall landraces were usually discarded because they were more susceptible to wind damage and displayed higher water demand and excessively long growth cycles (Zapparoli 1930; Cömertpay et al. 2012).
In the present study, many landraces showed very low values for ear/plant insertion ratio, particularly landraces with intermediate and flint kernels. Ideal values of this trait are around 46–50%, and during selection progenies with ears inserted too low or too high on the stalk are usually not retained. At the harvesting time, in many Italian areas devoted to maize cultivation, it was a common agronomic practice to top maize by cutting the stalk just above the leaf bearing the ear. This practice was carried out soon after flowering and with the aim of producing green forage for animals. Zapparoli (1930) considered this habit very detrimental for grain yield, but small mountain farmers still assume it as necessary. It could be speculated that farmers who used to follow this practice may have unintentionally selected for low ear insertion ratios by preferring a greater amount of green forage for animals. Moreover, empiric crop improvement was performed using as selection criteria the lowering of ear insertion. This was done to reduce plant height, increase earliness and improve lodging resistance (Zapparoli 1930).
Moderate percentages of lodging were displayed by the landraces in both years. During inbred selection, stalk lodging was one of the main agronomic traits to be assessed and improved in order to facilitate the mechanical harvesting (Troyer 2000; Gerke et al. 2015). Current hybrids are generally resistant to lodging (Stagnati et al. 2020b), whereas lodging may be severe in varieties not subject to breeding (Bonciarelli 1961; Lucchin et al. 2003; Bernardi et al. 2018). After flowering, the competition between stalk and ears as photosynthate sinks can lead to stalk breakage if the photosynthetic apparatus is not adequate to ensure supply for both ear and stalk (Verderio et al. 1989). It could be hypothesised that late varieties have a higher leaf surface and time to accumulate sugars in vegetative organs, thus ensuring a better resistance to lodging.
As concerns grain yield, near all landraces exhibited average productions similar to the hybrid considered in this study. EMR06 and M_P represented an exception, the later one also showing popped kernel symptoms. Generally, popped kernels are regarded as an oddity that rarely occurs in commercial hybrids, but it may be present in inbred lines. The injury may arise when during grain filling very dry weather conditions are followed by rainfall or irrigation, causing the endosperm to outgrow the pericarp (White 1999). EMR06 is the only popcorn landrace available in the collection of traditional landraces from Emilia-Romagna region. Popcorns are characterised by small ears and little grains; even though a single plant may produce multiple ears by tillering, the production is lower than flint or dent corns. Nonetheless, considering the particular landrace type, the fact that plants and tillers were completely developed and seed set was adequate, the production of EMR06 could be considered as acceptable, though lower than other landraces (Table 2). Popcorns were traditionally considered horticultural crops not field crops.
Different kernel types and ear morphology characterised the collection. In this regard, kernel types were significantly influenced by altitude of landrace growing locations. Dent landraces were almost always grown in the plains and low hills, whereas intermediate and flint landraces were cultivated from plains to mountains. Some dent corn landraces were introduced from USA at the beginning of the 20th century with the aim to increase yield in the most favourable areas, the plains and low hills (Fig. 4e). Dent corn was used to produce intervarietal crosses with the traditional flint corn (Zapparoli 1930; Brandolini and Brandolini 2006). It cannot be excluded that a certain level of unwanted intercrossing occurred among different landraces, producing intermediate strains of ‘high yielding corns’. The presence of extensive admixture of traditional landraces grown in the same area was previously reported for this collection (Stagnati et al. 2022), even if landraces continued to maintain their distinctive traits. This is because traditional growers focused their selection on few traits (i.e. ear morphology and kernel type) along with high production (Zapparoli 1930; Arteaga et al. 2016). The primary purpose of corn was for human nutrition through the production of polenta, for the preparation of which kernels from flint or flint-like landraces were the most suited, whereas dent corns were generally used for livestock feeding. The wide distribution of flint or intermediate landraces is consistent with the main use of corn in the 1950s and with the fact that flint or flint-like landraces were considered the most suitable to be conserved during the long, cold, and wet winter of the Italian peninsula (Brandolini and Brandolini 2006, 2009).
As regards ear morphology, the greatest grain moisture was observed in a conical ear landrace. The racial complex of conical maize is characterised by landraces suited to non-irrigated environments. The big conical cob, which is rich in parenchyma, is an adaptation to low-moisture conditions, as the distinctive structure helps the creation of a water reservoir for kernel development, which is particularly useful in stressful environments or under drought pressure (Bonciarelli 1961; Brandolini and Brandolini 2006, 2009). These preliminary observations may require further investigations to determine the potential of conical ear landraces as source of resistance/tolerance to drought.
It could be speculated that landraces with cylindrical ears were selected in the most favourable environments at lower altitudes, conversely conical maize landraces, characterised by a short growth cycle, were adapted either to high altitudes or as rainfed crops in the plains (Bonciarelli 1961; Brandolini and Brandolini 2006, 2009).
Conclusion
The 33 maize landraces from the Emilia-Romana region evaluated in the present study showed great phenotypic variability for all the agronomic traits assessed in 2019 and 2020 and provide some appealing sources of resistance to FER disease and fumonisin accumulation. Overall, the collection was characterised by a general earliness and thirteen and six landraces displayed FER percentages and fumonisin content very similar to a commercial hybrid, respectively. Moreover, eight landraces exhibited grain yield values comparable to the hybrid. Interestingly, Va221, Va227 and EMR03 showed the best combination among these three traits and therefore, can be considered suitable for breeding purposes targeting the development of FER and fumonisin-resistant germplasm. Furthermore, this material may represent a resource for future research aimed at the evaluation of the response to additional mycotoxigenic fungal pathogens, as F. graminearum and Aspergillus flavus, and their associated mycotoxins.
Declaration of funding
This research has been carried out in the framework of the PSR2014-2020 project ‘RICOLMA’ with the financial support of Regione Emilia-Romagna, Italy, project number 5015253, and is part of the project NODES, which has received funding from the MUR – M4C2 1.5 of PNRR with grant agreement no. ECS00000036.
Acknowledgements
The authors thank Paola Battilani for kindly providing Fusarium verticillioides strain.
References
Ardenghi NMG, Rossi G, Guzzon F (2018) Back to beaked: Zea mays subsp. mays Rostrata Group in northern Italy, refugia and revival of open-pollinated maize landraces in an intensive cropping system. PeerJ 6, e5123.
| Crossref | Google Scholar | PubMed |
Arteaga MC, Moreno-Letelier A, Mastretta-Yanes A, Vázquez-Lobo A, Breña-Ochoa A, Moreno-Estrada A, Eguiarte LE, Piñero D (2016) Genomic variation in recently collected maize landraces from Mexico. Genomics Data 7, 38-45.
| Crossref | Google Scholar | PubMed |
Barcaccia G, Lucchin M, Parrini P (2003) Characterization of a flint maize (Zea mays var. indurata) Italian landrace, II. Genetic diversity and relatedness assessed by SSR and Inter-SSR molecular markers. Genetic Resources and Crop Evolution 50, 253-271.
| Crossref | Google Scholar |
Bernardi J, Stagnati L, Lucini L, Rocchetti G, Lanubile A, Cortellini C, De Poli G, Busconi M, Marocco A (2018) Phenolic profile and susceptibility to Fusarium infection of pigmented maize cultivars. Frontiers in Plant Science 9, 1189.
| Crossref | Google Scholar | PubMed |
Bonciarelli F (1961) Studio agronomico comparato delle popolazioni umbre di mais. Maydica 6, 35-61.
| Google Scholar |
Brandolini A, Brandolini A (2009) Maize introduction, evolution and diffusion in Italy. Maydica 54, 233-242.
| Google Scholar |
Cassani E, Puglisi D, Cantaluppi E, Landoni M, Giupponi L, Giorgi A, Pilu R (2017) Genetic studies regarding the control of seed pigmentation of an ancient European pointed maize (Zea mays L.) rich in phlobaphenes: the “Nero Spinoso” from the Camonica valley. Genetic Resources and Crop Evolution 64, 761-773.
| Crossref | Google Scholar |
Centro di Riferimento per l’Agricoltura Biologica (CRAB) (2004) ‘Gli antichi mais del Piemonte.’ (Graffio Press: Borgone di Susa, TO, Italy) Available at http://www.antichimaispiemontesi.it/files/mais-piemontesi.pdf
Clements MJ, Kleinschmidt CE, Maragos CM, Pataky JK, White DG (2003) Evaluation of inoculation techniques for Fusarium ear rot and fumonisin contamination of corn. Plant Disease 87, 147-153.
| Crossref | Google Scholar | PubMed |
Cömertpay G, Baloch FS, Kilian B, Ülger AC, Özkan H (2012) Diversity assessment of Turkish maize landraces based on fluorescent labelled SSR markers. Plant Molecular Biology Reporter 30, 261-274.
| Crossref | Google Scholar |
Czembor E, Waskiewicz A, Piechota U, Puchta M, Czembor JH, Stepień Ł (2019) Differences in ear rot resistance and Fusarium verticillioides-produced fumonisin contamination between Polish currently and historically used maize inbred lines. Frontiers in Microbiology 10, 449.
| Crossref | Google Scholar | PubMed |
de Mendiburu F (2017) Agricolae: statistical procedures for agricultural research. R package version 1.2.8. Available at https://cran.r-project.org/web/packages/agricolae/index.html
Devi HN, Devi KN, Singh NB, Singh TR, Jyotsna N, Paul A (2013) Phenotypic characterization, genetic variability and correlation studies among maize landraces of Manipur. International Journal of Bio-resource and Stress Management 4, 352-355.
| Google Scholar |
Djemel A, Revilla P, Hanifi-Mekliche L, Malvar RA, Álvarez A, Khelifi L (2012) Maize (Zea mays L.) from the Saharan oasis: adaptation to temperate areas and agronomic performance. Genetic Resources and Crop Evolution 59, 1493-1504.
| Crossref | Google Scholar |
Edmondson RN (2019) blocksdesign: nested and crossed block designs for factorial, fractional factorial and unstructured treatment sets. R package version 3.4. Available at https://CRAN.R-project.org/package=blocksdesign
Eschholz TW, Stamp P, Peter R, Leipner J, Hund A (2010) Genetic structure and history of Swiss maize (Zea mays L. ssp. mays) landraces. Genetic Resources and Crop Evolution 57, 71-84.
| Crossref | Google Scholar |
Gaikpa DS, Miedaner T (2019) Genomics-assisted breeding for ear rot resistances and reduced mycotoxin contamination in maize: methods, advances and prospects. Theoretical and Applied Genetics 132, 2721-2739.
| Crossref | Google Scholar | PubMed |
Gaikpa DS, Kessel B, Presterl T, Ouzunova M, Galiano-Carneiro AL, Mayer M, Melchinger AE, Schön C-C, Miedaner T (2021) Exploiting genetic diversity in two European maize landraces for improving Gibberella ear rot resistance using genomic tools. Theoretical and Applied Genetics 134, 793-805.
| Crossref | Google Scholar | PubMed |
Gerke JP, Edwards JW, Guill KE, Ross-Ibarra J, McMullen MD (2015) The genomic impacts of drift and selection for hybrid performance in maize. Genetics 201, 1201-1211.
| Crossref | Google Scholar | PubMed |
Giupponi L, Pedrali D, Leoni V, Rodari A, Giorgi A (2021) The analysis of Italian plant agrobiodiversity databases reveals that hilly and sub-mountain areas are hotspots of herbaceous landraces. Diversity 13, 70.
| Crossref | Google Scholar |
Guche MD, Pilati S, Trenti F, Dalla Costa L, Giorni P, Guella G, Marocco A, Lanubile A (2022) Functional study of lipoxygenase-mediated resistance against Fusarium verticillioides and Aspergillus flavus infection in maize. International Journal of Molecular Sciences 23, 10894.
| Crossref | Google Scholar | PubMed |
Hartings H, Berardo N, Mazzinelli GF, Valoti P, Verderio A, Motto M (2008) Assessment of genetic diversity and relationships among maize (Zea mays L.) Italian landraces by morphological traits and AFLP profiling. Theoretical and Applied Genetics 117, 831-842.
| Crossref | Google Scholar | PubMed |
Hung H-Y, Holland JB (2012) Diallel analysis of resistance to Fusarium ear rot and fumonisin contamination in maize. Crop Science 52, 2173-2181.
| Crossref | Google Scholar |
Ignjatovic-Micic D, Drinic SM, Nikolic A, Lazic-Jancic V (2008) SSR analysis for genetic structure and diversity determination of maize local populations from former Yugoslavia territories. Genetika 44, 1517-1524.
| Google Scholar | PubMed |
Ju M, Zhou Z, Mu C, Zhang X, Gao J, Liang Y, Chen J, Wu Y, Li X, Wang S, Wen J, Yang L, Wu J (2017) Dissecting the genetic architecture of Fusarium verticillioides seed rot resistance in maize by combining QTL mapping and genome-wide association analysis. Scientific Reports 7, 46446.
| Crossref | Google Scholar | PubMed |
Lanubile A, Pasini L, Lo Pinto M, Battilani P, Prandini A, Marocco A (2011) Evaluation of broad spectrum sources of resistance to Fusarium verticillioides and advanced maize breeding lines. World Mycotoxin Journal 4, 43-51.
| Crossref | Google Scholar |
Lanubile A, Logrieco A, Battilani P, Proctor RH, Marocco A (2013) Transcriptional changes in developing maize kernels in response to fumonisin-producing and nonproducing strains of Fusarium verticillioides. Plant Science 210, 183-192.
| Crossref | Google Scholar | PubMed |
Lanubile A, Maschietto V, Borrelli VM, Stagnati L, Logrieco AF, Marocco A (2017) Molecular basis of resistance to Fusarium ear rot in maize. Frontiers in Plant Science 8, 1774.
| Crossref | Google Scholar | PubMed |
Lanubile A, Giorni P, Bertuzzi T, Marocco A, Battilani P (2021) Fusarium verticillioides and Aspergillus flavus co-occurrence influences plant and fungal transcriptional profiles in maize kernels and in vitro. Toxins 13, 680.
| Crossref | Google Scholar | PubMed |
Logrieco A, Battilani P, Leggieri MC, Jiang Y, Haesaert G, Lanubile A, Mahuku G, Mesterhazy A, Ortega-Beltran A, Pasti M, Smeu I, Torres A, Xu J, Munkvold G (2021) Perspectives on global mycotoxin issues and management from the MycoKey Maize Working Group. Plant Disease 105, 525-537.
| Crossref | Google Scholar | PubMed |
Lucchin M, Barcaccia G, Parrini P (2003) Characterization of a flint maize (Zea mays L. convar. mays) Italian landrace: I. Morphophenological and agronomic traits. Genetic Resources and Crop Evolution 50, 315-327.
| Crossref | Google Scholar |
Mangiafico S (2018) rcompanion: functions to support extension education program evaluation. R package version 1.11.3. Available at https://CRAN.R-project.org/package=rcompanion
Maschietto V, Colombi C, Pirona R, Pea G, Strozzi F, Marocco A, Rossini L, Lanubile A (2017) QTL mapping and candidate genes for resistance to Fusarium ear rot and fumonisin contamination in maize. BMC Plant Biology 17, 20.
| Crossref | Google Scholar | PubMed |
Mesterházy Á, Lemmens M, Reid LM (2012) Breeding for resistance to ear rots caused by Fusarium spp. in maize – a review. Plant Breeding 131, 1-19.
| Crossref | Google Scholar |
Morales L, Marino TP, Wenndt AJ, Fouts JQ, Holland JB, Nelson RJ (2018) Dissecting symptomatology and fumonisin contamination produced by Fusarium verticillioides in maize ears. Phytopathology 108, 1475-1485.
| Crossref | Google Scholar | PubMed |
Nass LL, Paterniani E (2000) Pre-breeding: a link between genetic resources and maize breeding. Scientia Agricola 57, 581-587.
| Crossref | Google Scholar |
Oppong A, Bedoya CA, Ewool MB, Asante MD, Thompson R, Adu-Dapaah H, Lamptey JNL, Ofori K, Offei SK, Warburton ML (2014) Bulk genetic characterization of Ghanaian maize landraces using microsatellite markers. Maydica 59, 1-8.
| Google Scholar |
Ouko A, Okoth S, Netshifhefhe NEL, Viljoen A, Rose LJ (2020) Tolerance to Fusarium verticillioides infection and fumonisin accumulation in maize F1 hybrids and subsequent F2 populations. Agronomy Journal 112, 2432-2444.
| Crossref | Google Scholar |
Palumbo F, Galla G, Martínez-Bello L, Barcaccia G (2017) Venetian local corn (Zea mays L.) germplasm: disclosing the genetic anatomy of old landraces suited for typical cornmeal mush production. Diversity 9, 32.
| Crossref | Google Scholar |
Peterson BG, Carl P (2018) PerformanceAnalytics: econometric tools for performance and risk analysis. R package version 1.5.2. Available at https://CRAN.Rproject.org/package=PerformanceAnalytics
Presello DA, Reid LM, Mather DE (2004) Resistance of Argentine maize germplasm to Gibberella and Fusarium ear rots. Maydica 49, 73-81.
| Google Scholar |
Qi-Lun Y, Ping F, Ke-Cheng K, Guang-Tang P (2008) Genetic diversity based on SSR markers in maize (Zea mays L.) landraces from Wuling mountain region in China. Journal of Genetics 87, 287-291.
| Crossref | Google Scholar | PubMed |
R Core Team (2017) ‘R: a language and environment for statistical computing.’ (R Foundation for Statistical Computing: Vienna, Austria) Available at https://www.R-project.org/
Reid LM, Zhu X, Parker A, Yan W (2009) Increased resistance to Ustilago zeae and Fusarium verticilliodes in maize inbred lines bred for Fusarium graminearum resistance. Euphytica 165, 567-578.
| Crossref | Google Scholar |
Robertson LA, Kleinschmidt CE, White DG, Payne GA, Maragos CM, Holland JB (2006) Heritabilities and correlations of Fusarium ear rot resistance and fumonisin contamination resistance in two maize populations. Crop Science 46, 353-361.
| Crossref | Google Scholar |
Ruiz de Galarreta JI, Alvarez A (2001) Morphological classification of maize landraces from northern Spain. Genetic Resources and Crop Evolution 48, 391-400.
| Crossref | Google Scholar |
Santiago R, Cao A, Butrón A (2015) Genetic factors involved in fumonisin accumulation in maize kernels and their implications in maize agronomic management and breeding. Toxins 7, 3267-3296.
| Crossref | Google Scholar | PubMed |
Septiani P, Lanubile A, Stagnati L, Busconi M, Nelissen H, Pè ME, Dell’Acqua M, Marocco A (2019) Unravelling the genetic basis of Fusarium seedling rot resistance in the MAGIC maize population: novel targets for breeding. Scientific Reports 9, 5665.
| Crossref | Google Scholar | PubMed |
Stagnati L, Lanubile A, Samayoa LF, Bragalanti M, Giorni P, Busconi M, Holland JB, Marocco A (2019) A genome wide association study reveals markers and genes associated with resistance to Fusarium verticillioides infection of seedlings in a maize diversity panel. G3 Genes|Genomes|Genetics 9, 571-579.
| Crossref | Google Scholar | PubMed |
Stagnati L, Rahjoo V, Samayoa LF, Holland JB, Borrelli VMG, Busconi M, Lanubile A, Marocco A (2020a) A genome-wide association study to understand the effect of Fusarium verticillioides infection on seedlings of a maize diversity panel. G3 Genes|Genomes|Genetics 10, 1685-1696.
| Crossref | Google Scholar | PubMed |
Stagnati L, Martino M, Battilani P, Busconi M, Lanubile A, Marocco A (2020b) Development of early maturity maize hybrids for resistance to Fusarium and Aspergillus ear rots and their associated mycotoxins. World Mycotoxin Journal 13, 459-471.
| Crossref | Google Scholar |
Stagnati L, Martino M, Soffritti G, Lanubile A, Ravasio A, Marocco A, Rossi G, Busconi M (2021) Microsatellite and morphological characterization of three Rostrato di Val Chiavenna (Sondrio, Italy) maize (Zea mays L.) accessions. Genetic Resources and Crop Evolution 68, 3025-3038.
| Crossref | Google Scholar |
Stagnati L, Soffritti G, Desiderio F, Lanubile A, Zambianchi S, Marocco A, Rossi G, Busconi M (2022) The rediscovery of traditional maize agrobiodiversity: a study case from northern Italy. Sustainability 14, 12110.
| Crossref | Google Scholar |
Torri A, Lanzanova C, Locatelli S, Valoti P, Balconi C (2015) Screening of local Italian maize varieties for resistance to Fusarium verticillioides. Maydica 60, 1-8.
| Google Scholar |
Verderio A, Bosio M, Introzzi F, Livini C, Motto M (1989) Relazioni tra prolungata persistenza fogliare e componenti della produzione in ibridi di mais (Zea mays L.). Agronomia 23, 173-177.
| Google Scholar |
Zila CT, Samayoa LF, Santiago R, Butrón A, Holland JB (2013) A genome-wide association study reveals genes associated with Fusarium ear rot resistance in a maize core diversity panel. G3 Genes|Genomes|Genetics 3, 2095-2104.
| Crossref | Google Scholar | PubMed |
Zila CT, Ogut F, Romay MC, Gardner CA, Buckler ES, Holland JB (2014) Genome-wide association study of Fusarium ear rot disease in the U.S.A. maize inbred line collection. BMC Plant Biology 14, 372.
| Crossref | Google Scholar | PubMed |