Heat-dried sclerotia of Sclerotinia sclerotiorum myceliogenically germinate in water and are able to infect Brassica napus
D. W. Lane A , L. G. Kamphuis A , M. C. Derbyshire A and M. Denton-Giles A BA Centre for Crop and Disease Management, Curtin University, Kent Street, Bentley, Perth, WA 6102, Australia.
B Corresponding author. Email: matthew.denton-giles@curtin.edu.au
Crop and Pasture Science 69(8) 765-774 https://doi.org/10.1071/CP18109
Submitted: 23 February 2018 Accepted: 4 July 2018 Published: 24 July 2018
Journal compilation © CSIRO 2018 Open Access CC BY-NC-ND
Abstract
The phytopathogenic fungus Sclerotinia sclerotiorum forms dormant structures (termed sclerotia) that germinate myceliogenically under certain environmental conditions. During myceliogenic germination, sclerotia produce hyphae, which can infect leaves or stems of host plants directly from the ground; this is termed basal infection. This study determined which abiotic conditions were most important for promoting myceliogenic germination of sclerotia in vitro. A high sclerotium hydration level and low incubation temperature (15°C) improved mycelial growth in the presence of a nutrient source. Sclerotia incubated without a nutrient source on moist sand, vigorously myceliogenically germinated most frequently (63%) when they had been previously imbibed and then conditioned at −20°C. By far the most consistent amount of vigorous myceliogenic germination (>75%) was produced when sclerotia were heat-dried before being submerged in water. The hyphae of these sclerotia were shown to infect and proliferate on leaves of intact Brassica napus plants. This research provides a better understanding of the abiotic conditions that are likely to increase the risk of basal infection by S. sclerotiorum.
Additional keywords: basal infection, canola, dryland agriculture, Sclerotinia stem rot.
Introduction
The fungus Sclerotinia sclerotiorum (Lib) de Bary (1884) is a widespread phytopathogen that infects many plant species (Purdy 1979; Boland and Hall 1994; Saharan and Mehta 2008; Sharma et al. 2015). It causes disease in numerous economically important crops, including Brassica napus (oilseed rape, canola) in which the disease is termed Sclerotinia stem rot (SSR) (Twengström et al. 1998).
Oilseed rape has the second highest oilseed production weight in the world with an estimated 73.8 million tonnes produced from 36 million hectares grown in 2014 (FAOSTAT 2017). In Australia, oilseed rape is the most important oilseed crop and SSR in this country causes an estimated loss of $10.1 million (AUD) annually (Murray and Brennan 2012).
During infection, S. sclerotiorum forms hard black structures termed sclerotia (Sharma et al. 2015). These become incorporated into the soil where they can remain dormant for up to five years (Adams and Ayers 1979). Each sclerotium has a melanin rind, which provides protection from environmental stresses (Coley-Smith and Cooke 1971).
The sclerotia of S. sclerotiorum can germinate carpogenically or myceliogenically under optimum environmental conditions (Hind-Lanoiselet and Lewington 2004). During carpogenic germination, sclerotia produce apothecia, which generate ascospores that are wind dispersed (Stelfox et al. 1978; Sharma et al. 2015). Ascospores generally initiate infection of B. napus stems via petals lodged in leaf axils. Sclerotinia sclerotiorum requires these petals as an initial substrate when proliferating via carpogenic germination (Purdy 1979; Hind-Lanoiselet and Lewington 2004; Turkington and Morrall 1993; Koch et al. 2007; Derbyshire and Denton‐Giles 2016). The disease SSR is characterised by lesions on the plant stem caused by mycelial growth from the senescent petals. However, lesions can also form on branches and seed pods (Hind-Lanoiselet and Lewington 2004).
During myceliogenic germination, sclerotia produce hyphae as opposed to apothecia. These hyphae initiate infection of nearby plants by contact with the stem or lower leaves, a process known as ‘basal infection’ (McQuilken et al. 1994; Hind-Lanoiselet and Lewington 2004). Because this form of infection does not require petals, it can occur before flowering of B. napus (Purdy 1979; Finlayson et al. 1989; Hind-Lanoiselet and Lewington 2004). Basal infection is thought to be the dominant contributor to preflowering infections in B. napus (McQuilken et al. 1994). It is the most common type of infection in the related pathogen S. minor (Purdy 1979), but is also a significant problem arising from S. sclerotiorum (McQuilken et al. 1994). Basal infection in B. napus is characterised by patchy white mycelium at the base of the stem (Bolton et al. 2006). Most research on S. sclerotiorum germination has focused on the carpogenic form, since it is most commonly associated with disease (Huang and Dueck 1980; Garg et al. 2010; Khangura and Beard 2015). However, under certain environmental conditions basal infection of oilseed rape can be more damaging (Šaroun and Říha 2008).
Several abiotic conditions influence myceliogenic germination in S. sclerotiorum. The effects of many of these conditions may be linked to how they change the physiological state of the rind surrounding the sclerotium. Huang (1985) discovered that myceliogenic germination could be induced in moist conditions through rind injury (scarring) or by selecting immature sclerotia that had not attained complete rind melanisation (Huang 1985; Huang and Kozub 1994). Furthermore, Huang (1991) determined that conditioning sclerotia at sub-freezing temperatures induces vigorous myceliogenic germination in moist conditions. In a subsequent study, Huang et al. (1998) revealed that relatively high temperatures (20 and 25°C) during sclerotial development, in combination with sclerotial desiccation, led to vigorous myceliogenic germination under moist incubation conditions. It was proposed that the drying process again caused injury to the rind, which subsequently allowed the leakage of nutrients that were able to support vigorous hyphal growth (Huang et al. 1998). Further research is required to confirm the effect of sclerotial desiccation and drying before myceliogenic germination.
Moisture is a key factor influencing the survival of S. sclerotiorum sclerotia. In flooded soils, sclerotia have a lower survival rate compared with dry soils (Coley-Smith and Cooke 1971; Matheron and Porchas 2005). Although sclerotia are degraded in high moisture-content soils, this is offset by the production of secondary sclerotia, where a balance of destruction and formation is achieved in completely saturated soil (Williams and Western 1965). Al-Hamdani and Cooke (1987) found that decreasing the water potential of a nutritional medium decreased the radial growth of S. sclerotiorum, which led to a decrease in sclerotia production.
Little is known about the ability of myceliogenically germinating S. sclerotiorum to infect B. napus. Basal infection is more commonly associated with other host crops such as sunflower (Huang and Dueck 1980; Sedun and Brown 1989). However, a comprehensive analysis of the optimal abiotic conditions leading to basal infection of B. napus is required. Li et al. (2007) observed basal infection of B. napus from myceliogenically germinating sclerotia in a field study and assumed that infection occurred directly at the stem’s base. Basal infection occurring via direct sclerotium contact with the stem was demonstrated by Huang and Dueck (1980); however, only low frequencies of infection were observed in B. napus. In a field plot experiment, McQuilken et al. (1994) found that abundant early mycelial infection occurred through senescent and frost-damaged leaves close to the soil surface. It is expected that infection of spring cultivars would occur less often as they are less likely to incur frost damage. Further study is required in controlled conditions to determine whether hyphae from myceliogenically germinating sclerotia can infect viable and intact B. napus tissue.
Most research on myceliogenic germination of S. sclerotiorum has used sclerotia collected from regions in Canada, where sub-freezing temperatures routinely occur during winter (Huang and Dueck 1980; Huang 1985, 1991; Huang et al. 1998). However, it has been reported that basal infection also occurs in dryland agricultural regions of the world with a Mediterranean climate (Khangura and Beard 2015). The aim of this study was to determine the influence of abiotic factors on myceliogenic germination of a S. sclerotiorum isolate that originates from cropping regions that do not routinely experience sub-zero temperatures. A further aim was to determine the required conditions for basal infection of living B. napus tissue. Experiments were designed to (1) confirm the influence of previously published abiotic stresses (such as scarring, temperature conditioning and drying) on myceliogenic germination of S. sclerotiorum sampled from a dryland agriculture cropping region (Western Australia), (2) identify key abiotic stresses likely to induce myceliogenic germination in the absence of seasonal sub-zero temperatures and (3) demonstrate a potential basal infection pathway of B. napus by infecting whole plants with myceliogenically germinating sclerotia. Here we report on abiotic stresses that cause high levels of myceliogenic germination of S. sclerotiorum and the important role of water availability in this process. In addition, we describe a consistent method that promotes myceliogenic germination of S. sclerotiorum sclerotia and show that the hyphae from these sclerotia are capable of infecting intact B. napus tissue.
Materials and methods
Fungal growth and culture conditions
The fungal isolate CU11.19 (isolated from a canola crop in Geraldton, Western Australia, in 2014), was identified as S. sclerotiorum by sequencing the conserved internal transcribed spacer sequence and through whole genome sequencing (Clarkson et al. 2017; MC Derbyshire, M Denton-Giles, JK Hane, S Chang, M Mousavi-Derazmahalleh, S Raffaele, L Buchwaldt, LG Kamphuis, unpubl. data).
Sclerotia were produced in vitro using the following method. A single, sterile sclerotium was cut in half using a sterile scalpel blade. Each half was incubated on a 9-cm diameter Petri dish containing 39 g/L potato dextrose agar (PDA) (Becton Dickinson) at 17°C in darkness for 5–7 days. After incubation, the actively growing mycelium from each sclerotium half was sub-cultured onto 5–10 PDA plates, depending on the quantity of sclerotia required for downstream experiments. The PDA plates were incubated at 17°C in darkness for 14 days, until mature sclerotia had formed. Mature sclerotia were then air-dried for 7 days and passed through a sieve to selectively obtain those of 2–3 mm in diameter. The selected sclerotia were evenly separated into 12 × 10-mL Falcon tubes (multiple sclerotia per tube). Each Falcon tube was termed a ‘wash replicate’. The sclerotia from each wash replicate were surface sterilised with 70% ethanol for 90 s, washed three times with sterile autoclaved de-ionised water (ADW) and blot-dried on sterile tissue paper. For each experimental variable tested (multiple variables were tested per experiment), sclerotia were sourced from 12 independent wash replicates.
To evaluate the effect of sclerotium origin on the frequency of myceliogenic germination and vigorous myceliogenic germination, PDA-grown sclerotia were compared with sclerotia collected from B. napus plants. The B. napus-derived sclerotia were extracted from primary stems of mature plants that were artificially inoculated with PDA plugs containing mycelium of isolate CU11.19. The PDA-derived sclerotia were stored for 133 days at room temperature before use. The plant-derived sclerotia were stored for ~10 months at room temperature before use. Each group was subjected to the sterilisation and wash procedure described above.
Abiotic factors
Various abiotic factors were applied to each set of replicate sclerotia sequentially (n = 12, one from each wash replicate). Abiotic factors were always applied to sclerotia in the following order: sclerotium hydration, conditioning temperature, scarring, water availability and incubation temperature. The number of sclerotia required for each experiment was dependent on the number of abiotic factors included in each experiment (e.g. 2 hydration treatments × 4 conditioning temperatures × 2 incubation temperatures × 12 replicate sclerotia per treatment = 192 individual sclerotia).
Hydration of sclerotia was manipulated through four different treatments: imbibing, not imbibing, drying or heat-drying. Imbibing consisted of immersing sclerotia in 15 mL of ADW for two days in a Petri dish sealed with Parafilm; hydrated sclerotia were blot-dried on sterile tissue paper before use. Not imbibing consisted of incubating sclerotia for two days at 25°C in an empty Petri dish sealed with Parafilm. Drying consisted of placing sclerotia in an unsealed Petri dish in a laminar flow cabinet for two days. Heat-drying consisted of incubating sclerotia in an unsealed Petri dish at 37°C for two days.
Sclerotia were temperature conditioned at –20, 4, 17 or 37°C for 14 days in sealed Petri dishes that were wrapped in aluminium foil. Scarring of sclerotia was performed after temperature conditioning for some experiments, by removing ~1 mm from either end of a sclerotium using a sterile scalpel blade. Sclerotia were incubated at 15 or 25°C on 12.5% PDA (4.875 g/L PDA + 15 g/L agar), and on sterile moist sand. An additional incubation temperature of 37°C was used for heat-dried, submerged sclerotia. Moist sand was prepared by combining 6 mL of sterile silica quartz (Sigma-Aldrich) with 2.2 mL of ADW for each sub-divided Petri dish (halves or thirds). Moist sand was also prepared in the wells of a 24-well tray by combining 1 mL of sterile silica quartz with 0.36 mL of ADW. Sclerotia were submerged in water by placing each sclerotium in a single well of a 24-well tray and adding 0 (not submerged), 0.2 (partially submerged) or 0.4 mL (fully submerged) of ADW. All Petri dishes and trays were sealed with Parafilm and placed in darkness for the duration of the incubation period.
Measurements of mycelium growth, myceliogenic and vigorous myceliogenic germination from sclerotia
For mycelium growth measurements on PDA, photographs were taken of each inoculated PDA plate at multiple time points and colony diameter measurements taken at 8, 11 and 14 days post inoculation (DPI) (Fig. 1). Colony diameter measurements were made through the centre of each sclerotium and were measured in centimetres using ImageJ (v1.50i) (Schneider et al. 2012). The area under the curve (AUC) was calculated using the colony diameter measurements (CD1, 2, 3) from each time-point (T1, 2, 3).
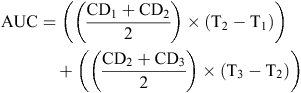
The presence of pigment on PDA was characterised by the darkening of colony colour after 11 DPI. Pigment presence was recorded as a presence or absence phenotype which was modelled using logistic regression. Pigment observations were made to complement the results from the different abiotic factors, as pigment accumulation has been reported as an abiotic stress response in other fungi (Hagiwara et al. 2017).
Myceliogenic germination of sclerotia on PDA was also assessed after 11 DPI (Table 1). This was distinct from mycelium growth and was defined as the presence of dense mycelium on the sclerotium rind or scar.
Myceliogenic germination of sclerotia placed on moist sand was categorised after 21 DPI (Table 1) and defined as the presence of mycelium either on or around the sclerotium. The amount of mycelium resulting from myceliogenic germination on moist sand was highly variable, so in order to simplify the statistical analyses, all the phenotypes were grouped into two categories: vigorous (recorded as 1) and non-vigorous myceliogenic germination (recorded as 0). Vigorous myceliogenic germination phenotypes included sparse radial mycelium (>1 cm diameter), production of secondary sclerotia or initials, dense radial mycelium and dense white mycelium growing from the sclerotium rind or scar. Non-vigorous myceliogenic germination phenotypes included no myceliogenic germination, sparse mycelium growing from the sclerotium rind or scar and sparse radial growth (<1 cm diameter).
For heat-dried, submerged sclerotia, myceliogenic germination was recorded after 5 DPI and was defined as either vigorous or non-vigorous myceliogenic germination based on phenotypes described for moist sand. The difference being that radial growth was observed across the water surface instead of across moist sand and sparse radial growth was not observed.
Host infection assay
Rapid-cycling B. napus plants (Musgrave 2000) were grown for 35 days at 18°C in 90% relative humidity at 200 µmol/m2·s of light. Non-vigorous, myceliogenically germinating sclerotia (unconditioned sclerotia) or sclerotia undergoing vigorous myceliogenic germination (conditioned by heat-drying then submergence in water), were individually placed in re-sealable plastic bags (75 mm × 100 mm). Water availability in the bag was varied by either gently blotting the sclerotia on tissue paper to dry them completely or by adding 0.4 mL of ADW to the bag. To establish infection, bags were sealed over the first fully formed rapid-cycling B. napus leaf, and lesion length was measured after four days. The sclerotia were visually inspected to ensure contact with the leaf for the duration of the infection period. Leaf lesion length measurements (cm) were made from the tip of the leaf to the highest point that the lesion reached.
Statistical analyses
All statistical tests were performed using R version 3.4.0 (R Core Team 2014). Full-factorial analysis of variance (ANOVA) was used for the AUC data and the leaf lesion length data (Figs 1 and 3). Tukey’s Honest Significant Difference (HSD) post-hoc test was used to determine which specific variables and variable interactions significantly differed from one another (P < 0.05). Data were transformed to meet the ANOVA assumptions of normality of residuals and homoscedasticity. Normality of residuals was assessed using Shapiro–Wilk’s test and homoscedasticity was assessed using Cochran’s test for outlying variance. In some cases, only partial homoscedasticity and normality were achieved. However, ANOVA is robust against moderate deviations from normality of residuals (Schmider et al. 2010) and heteroscedasticity (Rogan and Keselman 1977). There were many zero values for leaf lesion length, and the data were analysed using the Kruskal–Wallis test, as they were not normally distributed and were heteroscedastic.
Phenotype categories were analysed using logistic regression. In each case, the outcome of the experiment was treated as binary, e.g. myceliogenic germination = 1, no myceliogenic germination = 0. The model used a logit link function:

where Ln is the natural logarithm, P is the probability that the dependent variable equals one, β0 is the intercept of the regression equation and βnXn is any of the independent variables Xn in the optimised model multiplied by its coefficient βn. This regression coefficient is the estimated increase in the log odds of the dependent variable per unit increase in the independent variable. Additionally, in some models, interactions between independent variables were included and formulated as βnXn × βnXn. Variables and their interactions were included when they were required to better explain the model. Both incubation and conditioning temperature were assessed as continuous variables. All others were considered factors with discrete levels. A likelihood ratio test was used to determine if removing a variable improved the model fit, and this selection was aided by comparing the model to the null model using a chi-square test. The best model was confirmed by the model’s relative quality using the Akaike information criterion (AIC) test (Akaike 1973). The variables and variable interactions included in the final optimised logistic regression model were tested to determine if they significantly explained (P < 0.05) the model using a Wald test, as implemented in the R core function ‘glm’. The significance reported for these variables and variable interactions indicate that they significantly contributed to the model that best described the observed data (Table 1). The odds ratios reported are a measure of association between an abiotic variable (exposure) and the germination phenotype (outcome) (Szumillas 2010). A higher odds ratio for an abiotic variable suggests that the specific germination phenotype is more likely to occur when a sclerotium is exposed to that specific abiotic treatment.
Results
Sclerotium water content and incubation temperature influence mycelium growth and myceliogenic germination in the presence of exogenous nutrients
Experiments were initially designed to include an incubation step on PDA. This step was intended as a positive control, as healthy sclerotia are known to germinate and produce mycelium on PDA plates. However, we noticed differences in growth on PDA plates caused by prior exposure to different abiotic conditions. Therefore we recorded these. We found that imbibed sclerotia incubated at 15°C had a significantly greater log(AUC) value than imbibed sclerotia incubated at 25°C and dried sclerotia incubated at 15 or 25°C (Fig. 1a). There was no significant difference in log(AUC) between dried sclerotia incubated at either 15 or 25°C.
The same interaction was significant for sclerotia that had been scarred. Imbibed, scarred sclerotia incubated at 15°C had a significantly greater AUC than imbibed, scarred sclerotia incubated at 25°C and dried, scarred sclerotia incubated at 15 or 25°C (Fig. 1b). These observations showed that incubation of imbibed sclerotia at a lower temperature promoted mycelium growth on PDA.
Conditioning temperature also had a significant effect on mycelium growth in the presence of nutrients. Sclerotia conditioned at 4°C consistently produced the highest average AUC, which was significantly greater than sclerotia conditioned at 37°C (Fig. 1c).
In addition to colony growth, there were some obvious phenotypes, including the production of pigment (Fig. 1d–e) and the induction of myceliogenic germination on the surface of the sclerotium (Fig. 2a). The presence of pigment was significantly explained by incubation temperature (P < 1 × 10−11).
The highest frequency of sclerotia with myceliogenic germination on PDA was found after imbibing sclerotia and conditioning them at −20°C (55%). Sclerotia exhibiting myceliogenic germination produced dense mycelium directly on the sclerotium surface (Fig. 2a), clearly noticeable in comparison to the control (Fig. 2b). Based on logistic regression analyses, myceliogenic germination on PDA was significantly explained by sclerotium hydration and by the interaction between sclerotium hydration and conditioning temperature (Table 1). These results suggest that conditioning of imbibed sclerotia at −20°C promoted myceliogenic growth on the surface of the sclerotium.
Myceliogenic germination on moist sand is influenced by sclerotium hydration, temperature and scarring
Sclerotia of S. sclerotiorum can undergo myceliogenic germination on substrates that do not contain any nutrients, such as moist sand (Huang 1991). Various abiotic factors have been shown to cause this phenotype but never for an isolate sampled from a dryland agricultural system. We therefore evaluated the effects of a range of abiotic conditions on myceliogenic germination on moist sand. A high frequency of myceliogenic germination was observed when sclerotia were both imbibed and incubated at 25°C (88%). Imbibed sclerotia displayed a non-monotonic trend with respect to conditioning temperature, with the frequency of myceliogenic germination greatest at 37 and −20°C. These results demonstrate that the conditioning temperature extremes of –20 and 37°C significantly influenced the likelihood of sclerotia myceliogenically germinating in the absence of exogenous nutrients.
Imbibed, scarred sclerotia produced a higher frequency of myceliogenic germination compared with imbibed, unscarred sclerotia for all conditioning temperatures. However, compared with imbibed, scarred sclerotia, the dried, scarred sclerotia exhibited greater odds of myceliogenic germination as the conditioning temperature increased, with the greatest frequency of myceliogenic germination following 37°C conditioning (83%). However, of all factor combinations, the highest level of myceliogenic germination was for imbibed, scarred sclerotia conditioned at −20°C (97%).
The logistic regression models that best supported these two sets of data (myceliogenic germination for sclerotia on moist sand with and without a scarring treatment) were significantly explained by sclerotium hydration, incubation temperature, scarring and the interaction between sclerotium hydration and conditioning temperature (Table 1).
Myceliogenic germination can be vigorous or non-vigorous in the absence of nutrients
Among sclerotia that myceliogenically germinated on moist sand, a range of phenotypes were observed. Previous attempts to capture this diversity categorised myceliogenic germination as non-vigorous (‘germination’) and vigorous (Huang et al. 1998). To simplify statistical analyses, we used similar categories, but defined them based on the range of subtly different phenotypes we observed (see Materials and methods for definitions). Sclerotia in the vigorous myceliogenic germination category mostly exhibited dense hyphal growth from the sclerotium rind (Fig. 2c) or scar (Fig. 2d). Overall, 74% of vigorously germinating sclerotia had this phenotype and 46% of sclerotia with this phenotype also exhibited dense radial hyphal growth (Fig. 2e–g). In total, 10% of sclerotia that exhibited vigorous myceliogenic germination produced at least one secondary sclerotium or sclerotium initial (Fig. 2h) and this phenotype was always observed in combination with another vigorous myceliogenic germination phenotype. Only 29% of scarred sclerotia were categorised as having undergone vigorous myceliogenic germination. Non-vigorous myceliogenic germination phenotypes included sparse hyphal growth erupting from the rind (Fig. 2i) and no myceliogenic germination (absence of visible mycelium) (Fig. 2j). Sclerotia with no visible mycelium represented 54% of the sclerotia in the non-vigorous myceliogenic germination category. The data were re-analysed based on these categories to determine the effects of abiotic conditions on the frequency of vigorous myceliogenic germination.
Vigorous myceliogenic germination is promoted by sclerotial hydration, conditioning temperature and incubation temperature
After defining which sclerotia had undergone vigorous myceliogenic germination using the previously mentioned criteria, we tested which abiotic factors contributed to this phenotype in the same way that we did for myceliogenic germination. The highest percentage of vigorous germination occurred when sclerotia were imbibed and conditioned at −20°C (63%). In contrast, dried, scarred sclerotia were more likely to vigorously germinate compared with imbibed, scarred sclerotia at a conditioning temperature of 37°C (29%). Based on logistic regression analyses, sclerotium hydration, incubation temperature, the interaction between sclerotium hydration and conditioning temperature and the interaction between incubation temperature and conditioning temperature significantly explained the models (Table 1). Scarring imbibed sclerotia did not significantly explain the model. In summary, these results show that significant levels of vigorous myceliogenic germination can be achieved by imbibing sclerotia and conditioning them at −20°C, or drying scarred sclerotia and conditioning them at 37°C.
Heat-drying followed by submergence in water promotes vigorous myceliogenic germination in the absence of a nutrient source
Results on moist sand indicated that high conditioning temperatures (37°C) could induce both myceliogenic and vigorous myceliogenic germination of sclerotia. Sclerotia present in dryland agricultural regions like Australia are likely to be exposed to high temperatures over summer, followed by the sudden availability of water during autumn. Therefore, we were particularly interested in the ability of high conditioning temperatures to prime sclerotia for myceliogenic germination. To investigate this, sclerotia were heat-dried at 37°C in an incubator (a more severe form of drying), then submerged in water. Submerging heat-dried sclerotia in 0.2 mL of ADW at 25°C resulted in 100% myceliogenic germination. However, like sclerotia on moist sand, submerged sclerotia also produced variable amounts of mycelium, therefore myceliogenic germination phenotypes were classified as either vigorous or non-vigorous.
Both heat-drying and hydration influence vigorous myceliogenic germination. A total of 75% of heat-dried sclerotia underwent vigorous myceliogenic germination compared with 8% when sclerotia were imbibed and not heat-dried (Fig. 2k). Sclerotia that were heat-dried but not submerged in water did not germinate (Fig. 2l). Sclerotium hydration before heat-drying also influenced the frequency of vigorous myceliogenic germination. A total of 67% of not imbibed, heat-dried sclerotia underwent vigorous myceliogenic germination compared with 17% of imbibed, heat-dried sclerotia. Based on logistic regression analyses, both sclerotium hydration before submergence in water and sclerotium hydration before heat-drying significantly contributed to the model (Table 2). Scarring and incubation temperature did not significantly contribute to the model. Interestingly, no myceliogenic germination was observed among sclerotia incubated at 37°C, suggesting that incubation at this temperature, following abiotic stress, reduced sclerotium viability.
To determine whether sclerotium origin influenced myceliogenic germination of sclerotia, PDA- and plant-derived sclerotia were compared. Sclerotia from both origins were heat-dried, subsequently partially submerged in 0.2 mL of ADW and incubated at 25°C. Sclerotia originating from both sources exhibited high levels of vigorous myceliogenic germination, with 92% of PDA-derived and 100% of plant-derived sclerotia vigorously germinating. Thus, sclerotium origin did not significantly influence the observed frequency of vigorous myceliogenic germination.
Vigorously myceliogenically germinating sclerotia infect intact living host tissue
Heat-drying followed by submergence of sclerotia in water, generated consistently high levels of vigorous myceliogenic germination. Therefore, this method was used to generate vigorously myceliogenically germinating sclerotia and test their ability to infect B. napus leaves. Preconditioned, vigorous myceliogenically germinating sclerotia (heat-dried and submerged in ADW) produced a significantly greater leaf lesion length than sclerotia that were unconditioned, by 4 DPI (Fig. 3a). In a separate experiment, there was no significant difference between lesion lengths caused by vigorously myceliogenically germinating sclerotia that were either blot-dried or fully submerged (0.4 mL of ADW) before inoculation (Fig. 3b). Images of the infection over time showed the development of a water-soaked, brown leaf lesion developing in proximity to a sclerotium undergoing vigorous myceliogenic germination (Fig. 3c–e). These results suggest that infection of B. napus by vigorously germinated sclerotia occurred at a faster rate than with sclerotia that were not vigorously germinating.
Discussion
Water availability significantly contributed to the development of mycelia from sclerotia. Sclerotia that were imbibed before incubation showed increased growth on a nutrient-containing medium and a higher percentage of myceliogenic germination on moist sand. Furthermore, when they were submerged in water, all sclerotia underwent myceliogenic germination and at least 75% vigorously myceliogenically germinated when sclerotia were heat-dried before submergence in water. This indicates that free water had a major impact on the propensity of sclerotia to undergo vigorous myceliogenic germination. Sclerotia that were half or fully submerged in water exhibited the same high percentage of vigorous myceliogenic germination. This may be because the level of sclerotial saturation required for this form of germination was reached without full submergence.
Submergence in water was previously shown to reduce the viability of sclerotia in soil (Moore 1949). In a study by Matheron and Porchas (2005), sclerotium viability was reduced to ~20% after two weeks in flooded soil. In the current study, myceliogenic germination occurred after a minimum of three days of submergence in water. By five days of submergence, 100% of sclerotia exhibited myceliogenic germination. Those that vigorously germinated were able to infect intact B. napus plants. Therefore, loss in viability due to flooding for two weeks in the field could be offset by infection of lower plant parts by myceliogenically germinating sclerotia, if a suitable host is present.
Because all experiments were conducted under sterile conditions, the effects of soil microbiota on myceliogenic germination were not assessed. It was shown previously that increasing soil moisture under non-sterile conditions increases the rate of sclerotium degradation by soil-borne microbes (Williams and Western 1965). Additionally, nutrient leakage from sclerotia encourages their microbial degradation (Smith 1972). Thus, further research is required to determine whether the abiotic factors assessed in this study are the same under non-sterile conditions.
Among several abiotic factors, Huang (1985) concluded that sclerotial scarring had the biggest impact on myceliogenic germination. However, we found that it had a minimal impact on myceliogenic germination and vigorous myceliogenic germination when sclerotia were submerged in water or placed on moist sand. It is not possible to pinpoint why this might be the case given the current data. However, it is possible that the isolates used in the different studies exhibit different adaptations to their local environments (North America vs Australia).
Temperature had a major influence on myceliogenic germination. The two conditioning temperature extremes of –20 and 37°C induced the highest frequencies of both myceliogenic and vigorous myceliogenic germination. Although application of either temperature has a different outcome, i.e. −20°C leads to freezing of sclerotia and 37°C leads to drying, both may cause internal and external sclerotium injury, leading to myceliogenic germination (Huang 1991; Huang et al. 1998). In the current study, sclerotia had to first be imbibed before exposure to sub-zero temperatures to elicit the same response. This may explain why, in a subsequent study to Huang (1991), Foley et al. (2016) were unable to demonstrate myceliogenic germination after exposure to sub-zero temperatures, as sclerotia were taken directly from the growth medium with no initial period of imbibition.
Al-Hamdani and Cooke (1987) and Huang et al. (1998) demonstrated a greater amount of myceliogenic germination in desiccated sclerotia that were subsequently incubated on a moist substrate. However, in these experiments the sclerotia were not heat-dried or completely submerged in water.
The current study showed that a 37°C heat exposure followed by full submergence in water, consistently induced very high levels of vigorous myceliogenic germination. Although it is not possible to safely extrapolate from this observation because only a single isolate was used in this study, further research could be conducted to determine the frequency of myceliogenic germination in sclerotia isolated from dryland agricultural systems. Because conditions that routinely arise in such regions consistently promoted myceliogenic germination in vitro, we speculate that basal infection occurs more frequently in these regions than in temperate regions. Despite overall high levels of vigorous myceliogenic germination among heat-dried then submerged sclerotia, no growth occurred from these sclerotia when they were incubated at 37°C. This is not surprising, as S. sclerotiorum ascospores were found to be unable to germinate at 30°C or above in planta (Young et al. 2004). Temperature also affected radial growth of mycelium on PDA, which was less vigorous and often pigmented at 25°C compared with 15°C. Indeed 25°C was shown to be at the top end of the optimum growth range for S. sclerotiorum (Young et al. 2004).
Of all combinations of abiotic factors tested, heat-drying followed by submergence in water caused the highest frequency of vigorous myceliogenic germination among sclerotia. Therefore, we chose this method for preparing sclerotia for host infection assays. Although several studies have used infection assays based on ascospore sprays or agar plugs (Finlayson et al. 1989; Li et al. 2007; Zhao et al. 2009), none assessed the ability of myceliogenically germinating sclerotia to infect plants. The only attempt so far at direct inoculation of plants with sclerotia was by Huang and Dueck (1980) – this study demonstrated very low frequencies of infection in B. napus plants after inoculation with unconditioned sclerotia. In the current study, all vigorously myceliogenically germinating sclerotia produced infection in intact living B. napus plants. This contradicts a previous hypothesis that basal infection only occurs in oilseed rape after mechanical damage or senescence of plant tissue (McQuilken et al. 1994).
The simple protocol developed in this study for inducing myceliogenic germination of S. sclerotiorum could be applied to infection assays on other major host species that are more prone to basal infection by myceliogenic germination of sclerotia such as lettuce, tomato, sunflower and carrot (Letham et al. 1976; Huang and Hoes 1980; Finlayson et al. 1989; Subbarao 1998). Vigorous myceliogenic germination can be consistently induced within seven days using the method described in the current study. This relatively short amount of time lends itself to high throughput screening of large germplasm collections to identify resistance to S. sclerotiorum basal infection. Furthermore, infection by germinating sclerotia may be closer to what is observed in the field for some species than infection with an inoculated agar plug.
In summary, this study highlighted some of the major abiotic factors that cause myceliogenic germination of S. sclerotiorum. In particular, we demonstrated consistent high levels of vigorous myceliogenic germination among sclerotia that were heat-dried and subsequently submerged in water. The findings from this research led to the development of a timely protocol for production of myceliogenically germinating sclerotia, which can be used to induce basal infection in B. napus. The impact of basal infection has not been well documented in the field, although it has been reported in dryland agriculture regions of Australia (Khangura and Beard 2015). We believe that this body of research increases our understanding of the abiotic factors that are likely responsible for the induction of myceliogenic germination of S. sclerotiorum and subsequent basal infection of host plants.
Conflicts of interest
The authors declare no conflicts of interest.
Acknowledgements
The authors would like to acknowledge Dr John Golz for kindly providing rapid-cycling B. napus seed. This project was supported by a student stipend from the Centre for Crop and Disease Management which is in turn supported by Curtin University and the GRDC (CUR00023 Program 6).
References
Adams P, Ayers W (1979) Ecology of Sclerotinia species. Phytopathology 69, 896–899.| Ecology of Sclerotinia species.Crossref | GoogleScholarGoogle Scholar |
Akaike H (1973) Information theory and an extension of the maximum likelihood principle. In ‘Proceedings of the 2nd International Symposium on Information Theory’. (Eds BN Petrov, F Csaki) pp. 267–281. (Akademiai Kiado: Budapest)
Al-Hamdani AM, Cooke RC (1987) Effects of water potential on accumulation and exudation of carbohydrates and glycerol during sclerotium formation and myceliogenic germination in Sclerotinia sclerotiorum. Transactions of the British Mycological Society 89, 51–60.
| Effects of water potential on accumulation and exudation of carbohydrates and glycerol during sclerotium formation and myceliogenic germination in Sclerotinia sclerotiorum.Crossref | GoogleScholarGoogle Scholar |
Boland GJ, Hall R (1994) Index of plant hosts of Sclerotinia sclerotiorum. Canadian Journal of Plant Pathology 16, 93–108.
| Index of plant hosts of Sclerotinia sclerotiorum.Crossref | GoogleScholarGoogle Scholar |
Bolton MD, Thomma BP, Nelson BD (2006) Sclerotinia sclerotiorum (Lib.) de Bary: biology and molecular traits of a cosmopolitan pathogen. Molecular Plant Pathology 7, 1–16.
| Sclerotinia sclerotiorum (Lib.) de Bary: biology and molecular traits of a cosmopolitan pathogen.Crossref | GoogleScholarGoogle Scholar |
Clarkson J, Warmington R, Walley P, Denton-Giles M, Barbetti M, Brodal G, Nordskog B (2017) Population structure of Sclerotinia subarctica and Sclerotinia sclerotiorum in England, Scotland and Norway. Frontiers in Microbiology 8, 490
| Population structure of Sclerotinia subarctica and Sclerotinia sclerotiorum in England, Scotland and Norway.Crossref | GoogleScholarGoogle Scholar |
Coley-Smith J, Cooke R (1971) Survival and germination of fungal sclerotia. Annual Review of Phytopathology 9, 65–92.
| Survival and germination of fungal sclerotia.Crossref | GoogleScholarGoogle Scholar |
Derbyshire MC, Denton-Giles M (2016) The control of sclerotinia stem rot on oilseed rape (Brassica napus): current practices and future opportunities. Plant Pathology 65, 859–877.
FAOSTAT (2017) Data, crops: rapeseed. Available at: http://www.fao.org/faostat/en/#data/QC (accessed 15 August 2017).
Finlayson JE, Rimmer SR, Pritchard MK (1989) Infection of carrots by Sclerotinia sclerotiorum. Canadian Journal of Plant Pathology 11, 242–246.
| Infection of carrots by Sclerotinia sclerotiorum.Crossref | GoogleScholarGoogle Scholar |
Foley ME, Doğramacı M, West M, Underwood WR (2016) Environmental factors for germination of Sclerotinia sclerotiorum sclerotia. Journal of Plant Pathology & Microbiology 7, 379–383.
| Environmental factors for germination of Sclerotinia sclerotiorum sclerotia.Crossref | GoogleScholarGoogle Scholar |
Garg H, Sivasithamparam K, Barbetti MJ (2010) Scarification and environmental factors that enhance carpogenic germination of sclerotia of Sclerotinia sclerotiorum. Plant Disease 94, 1041–1047.
| Scarification and environmental factors that enhance carpogenic germination of sclerotia of Sclerotinia sclerotiorum.Crossref | GoogleScholarGoogle Scholar |
Hagiwara D, Sakai K, Suzuki S, Umemura M, Nogawa T, Kato N, Osada H, Watanabe A, Kawamoto S, Gonoi T, Kamei K (2017) Temperature during conidiation affects stress tolerance, pigmentation, and trypacidin accumulation in the conidia of the airborne pathogen Aspergillus fumigatus. PLoS One 12, e0177050
| Temperature during conidiation affects stress tolerance, pigmentation, and trypacidin accumulation in the conidia of the airborne pathogen Aspergillus fumigatus.Crossref | GoogleScholarGoogle Scholar |
Hind-Lanoiselet T, Lewington F (2004) Canola concepts: managing sclerotinia. New South Wales Agriculture Agnote DPI-490. NSW Department of Primary Industries, Wagga Wagga, NSW.
Huang HC (1985) Factors affecting myceliogenic germination of sclerotia of Sclerotinia sclerotiorum. Phytopathology 75, 433–437.
| Factors affecting myceliogenic germination of sclerotia of Sclerotinia sclerotiorum.Crossref | GoogleScholarGoogle Scholar |
Huang HC (1991) Induction of myceliogenic germination of sclerotia of Sclerotinia sclerotiorum by exposure to sub-freezing temperatures. Plant Pathology 40, 621–625.
| Induction of myceliogenic germination of sclerotia of Sclerotinia sclerotiorum by exposure to sub-freezing temperatures.Crossref | GoogleScholarGoogle Scholar |
Huang HC, Dueck J (1980) Wilt of sunflower from infection by mycelial-germinating sclerotia of Sclerotinia sclerotiorum. Canadian Journal of Plant Pathology 2, 47–52.
| Wilt of sunflower from infection by mycelial-germinating sclerotia of Sclerotinia sclerotiorum.Crossref | GoogleScholarGoogle Scholar |
Huang HC, Hoes JA (1980) Importance of plant spacing and sclerotial position to development of Sclerotinia wilt of sunflower. Plant Disease 64, 81–84.
| Importance of plant spacing and sclerotial position to development of Sclerotinia wilt of sunflower.Crossref | GoogleScholarGoogle Scholar |
Huang HC, Kozub GC (1994) Germination of immature and mature sclerotia of Sclerotinia sclerotiorum. Botanical Bulletin - Academia Sinica Taipei 35, 243–247.
Huang HC, Chang C, Kozub GC (1998) Effect of temperature during sclerotial formation, sclerotial dryness, and relative humidity on myceliogenic germination of sclerotia of Sclerotinia sclerotiorum. Canadian Journal of Botany 76, 494–499.
| Effect of temperature during sclerotial formation, sclerotial dryness, and relative humidity on myceliogenic germination of sclerotia of Sclerotinia sclerotiorum.Crossref | GoogleScholarGoogle Scholar |
Khangura R, Beard C (2015) Managing sclerotinia stem rot in canola. Department of Agriculture and Food. Western Australian Government. Available at: https://www.agric.wa.gov.au/canola/managing-sclerotinia-stem-rot-canola (accessed 10 June 2017).
Koch S, Dunker S, Kleinhenz B, Röhrig M, Tiedemann AV (2007) A crop loss-related forecasting model for Sclerotinia stem rot in winter oilseed rape. Phytopathology 97, 1186–1194.
| A crop loss-related forecasting model for Sclerotinia stem rot in winter oilseed rape.Crossref | GoogleScholarGoogle Scholar |
Letham DB, Huett DO, Trimboli DS (1976) Biology and control of Sclerotinia sclerotiorum in cauliflower and tomato crops in coastal New South Wales. Plant Disease Reporter 60, 286–289.
Li CX, Li H, Siddique AB, Sivasithamparam K, Salisbury P, Banga SS, Banga S, Chattopadhyay C, Kumar A, Singh R, Singh D, Agnihotri A, Liu SY, Li YC, Tu J, Fu TD, Wang YF, Barbetti MJ (2007) The importance of the type and time of inoculation and assessment in the determination of resistance in Brassica napus and B. juncea to Sclerotinia sclerotiorum. Crop & Pasture Science 58, 1198–1203.
| The importance of the type and time of inoculation and assessment in the determination of resistance in Brassica napus and B. juncea to Sclerotinia sclerotiorum.Crossref | GoogleScholarGoogle Scholar |
Matheron M, Porchas M (2005) Influence of soil temperature and moisture on eruptive germination and viability of sclerotia of Sclerotinia minor and S. sclerotiorum. Plant Disease 89, 50–54.
| Influence of soil temperature and moisture on eruptive germination and viability of sclerotia of Sclerotinia minor and S. sclerotiorum.Crossref | GoogleScholarGoogle Scholar |
McQuilken M, Mitchell S, Archer S (1994) Origin of early attacks of Sclerotinia stem rot on winter oilseed rape (Brassica napus sub. sp. oleifera var. biensis) in the UK. Journal of Phytopathology 140, 179–186.
| Origin of early attacks of Sclerotinia stem rot on winter oilseed rape (Brassica napus sub. sp. oleifera var. biensis) in the UK.Crossref | GoogleScholarGoogle Scholar |
Moore W (1949) Flooding as a means of destroying the sclerotia of Sclerotinia sclerotiorum. Phytopathology 39, 920–927.
Murray G, Brennan JP (2012) The current and potential costs from diseases of oilseed crops in Australia. Grains Research & Development Corporation, Kingston, ACT, Australia. https://www.grdc.com.au/ Resources/Publications/2012/06/The-Current-and-Potential-Costs-fromDiseases-of-Oilseed-Crops-in-Australia (accessed 21 July 2017).
Musgrave ME (2000) Realizing the potential of rapid-cycling Brassica as a model system for use in plant biology research. Journal of Plant Growth Regulation 19, 314–325.
| Realizing the potential of rapid-cycling Brassica as a model system for use in plant biology research.Crossref | GoogleScholarGoogle Scholar |
Purdy LH (1979) Sclerotinia sclerotiorum: history, diseases and symptomatology, host range, geographic distribution, and impact. Phytopathology 69, 875–880.
| Sclerotinia sclerotiorum: history, diseases and symptomatology, host range, geographic distribution, and impact.Crossref | GoogleScholarGoogle Scholar |
R Core Team (2014) R: A language and environment for statistical computing. R Foundation for Statistical Computing Vienna, Austria. http://www.R-project.org/
Rogan JC, Keselman H (1977) Is the ANOVA F-test robust to variance heterogeneity when sample sizes are equal? An investigation via a coefficient of variation. American Educational Research Journal 14, 493–498.
| Is the ANOVA F-test robust to variance heterogeneity when sample sizes are equal? An investigation via a coefficient of variation.Crossref | GoogleScholarGoogle Scholar |
Saharan GS, Mehta N (2008) ‘Sclerotinia diseases of crop plants: biology, ecology and disease management.’ (Springer: Dordrecht, The Netherlands)
Šaroun J, Říha K (2008) Možné příčiny poklesu výnosu řepky v ročníku 2007–2008 (Possible causes of decline in yield of rapeseed 2007–2008.). In ‘Sborník referátů 25 vyhodnocovacího semináře, Hluk 2008’. pp. 296–304 (SPZO: Praha)
Schmider E, Ziegler M, Danay E, Beyer L, Bühner M (2010) Is it really robust? Reinvestigating the robustness of ANOVA against violations of the normal distribution assumption. Methodology 6, 147–151.
| Is it really robust? Reinvestigating the robustness of ANOVA against violations of the normal distribution assumption.Crossref | GoogleScholarGoogle Scholar |
Schneider CA, Rasband WS, Eliceiri KW (2012) NIH Image to ImageJ: 25 years of image analysis. Nature Methods 9, 671–675.
| NIH Image to ImageJ: 25 years of image analysis.Crossref | GoogleScholarGoogle Scholar |
Sedun F, Brown J (1989) Comparison of three methods to assess resistance in sunflower to basal stem rot caused by Sclerotinia sclerotiorum and S. minor. Plant Disease 73, 52–55.
| Comparison of three methods to assess resistance in sunflower to basal stem rot caused by Sclerotinia sclerotiorum and S. minor.Crossref | GoogleScholarGoogle Scholar |
Sharma P, Meena PD, Verma PR, Saharan GS, Mehta N, Singh D, Kumar A (2015) Sclerotinia sclerotiorum (Lib) de Bary causing Sclerotinia rot in oilseed Brassicas: a review. Journal of Oilseed Brassica 6, 1–44.
Smith AM (1972) Biological control of fungal sclerotia in soil. Soil Biology & Biochemistry 4, 131–132.
| Biological control of fungal sclerotia in soil.Crossref | GoogleScholarGoogle Scholar |
Stelfox D, Williams JR, Soehngen U, Topping RC (1978) Transport of Sclerotinia sclerotiorum ascospores by rapeseed pollen in Alberta. Plant Disease Reporter 62, 576–579.
Subbarao KV (1998) Progress toward integrated management of lettuce drop. Plant Disease 82, 1068–1078.
| Progress toward integrated management of lettuce drop.Crossref | GoogleScholarGoogle Scholar |
Szumillas M (2010) Explaining odds ratios. Journal of the Canadian Academy of Child and Adolescent Psychiatry 19, 227–229.
Turkington T, Morrall R (1993) Use of petal infestation to forecast Sclerotinia stem rot of canola: the influence of inoculum variation over the flowering period and canopy density. Phytopathology 83, 682–689.
| Use of petal infestation to forecast Sclerotinia stem rot of canola: the influence of inoculum variation over the flowering period and canopy density.Crossref | GoogleScholarGoogle Scholar |
Twengström E, Sigvald R, Svensson C, Yuen J (1998) Forecasting Sclerotinia stem rot in spring sown oilseed rape. Crop Protection 17, 405–411.
| Forecasting Sclerotinia stem rot in spring sown oilseed rape.Crossref | GoogleScholarGoogle Scholar |
Williams GH, Western JH (1965) The biology of Sclerotinia trifoliorum Erikss. and other species of sclerotium-forming fungi. Annals of Applied Biology 56, 261–268.
| The biology of Sclerotinia trifoliorum Erikss. and other species of sclerotium-forming fungi.Crossref | GoogleScholarGoogle Scholar |
Young C, Clarkson JP, Smith JA, Watling M, Phelps K, Whipps JM (2004) Environmental conditions influencing Sclerotinia sclerotiorum infection and disease development in lettuce. Plant Pathology 53, 387–397.
| Environmental conditions influencing Sclerotinia sclerotiorum infection and disease development in lettuce.Crossref | GoogleScholarGoogle Scholar |
Zhao J, Buchwaldt L, Rimmer SR, Sharpe A, McGregor L, Bekkaoui D, Hegedus D (2009) Patterns of differential gene expression in Brassica napus cultivars infected with Sclerotinia sclerotiorum. Molecular Plant Pathology 10, 635–649.
| Patterns of differential gene expression in Brassica napus cultivars infected with Sclerotinia sclerotiorum.Crossref | GoogleScholarGoogle Scholar |