Unpacking the recruitment potential of seeds in reconstructed soils and varying rainfall patterns
Todd E. Erickson




A Centre for Engineering Innovation: Agriculture and Ecological Restoration, School of Agriculture and Environment, The University of Western Australia, Crawley, WA 6009, Australia.
B Kings Park Science, Department of Biodiversity, Conservation and Attractions, Kings Park, WA 6005, Australia.
C The University of Queensland, School of Biological Sciences, St Lucia, Qld 4072, Australia.
D CSIRO Land and Water, Ecosciences Precinct, Dutton Park, Qld 4102, Australia.
E School of Biological Sciences, The University of Western Australia, Crawley 6009, WA, Australia.
F California Polytechnic State University, Department of Natural Resources and Environmental Management, San Luis Obispo, CA 93407, USA.
G Department of Plant Biology and Ecology, University of Seville, Seville, Spain.
H School of Biological, Earth and Environmental Sciences, University of New South Wales, Randwick, NSW 2052, Australia.
Australian Journal of Botany - https://doi.org/10.1071/BT22141
Submitted: 22 December 2022 Accepted: 24 April 2023 Published online: 5 June 2023
© 2023 The Author(s) (or their employer(s)). Published by CSIRO Publishing. This is an open access article distributed under the Creative Commons Attribution-NonCommercial-NoDerivatives 4.0 International License (CC BY-NC-ND)
Abstract
Context: Seeding is common practice for ecological restoration, but establishment rates can be low. For seeds to successfully establish they must transition through early life stages of germination, emergence, and (initial) survival. Examining these demographic processes for seeds sown under a range of abiotic conditions can identify failure points and inform techniques to improve seed use.
Aims: Here we quantified seed and seedling life-stage transitions in five reconstructed soils across four varying levels of simulated rainfall using five species (Acacia hilliana, Acacia inaequilatera, Indigofera monophylla, Triodia pungens, and Triodia wiseana) commonly seeded for mined land restoration of the Pilbara bioregion in north-west Western Australia.
Methods: Germination, emergence, and survival were measured over a 6 week period and transition probabilities between each life-stage transition were modelled for each treatment combination.
Key results: For four species, both rainfall amount and/or soil substrate significantly influenced germination probability. Rainfall was the more significant determinant, with germination greatest under the higher rainfall regimes of 120–280 mm, irrespective of soil type. Following germination, emergence of both Acacia spp. was positively influenced by soils containing topsoil, suggesting the microenvironment of soils containing topsoil was most favourable during this emergence phase. The effect of substrate was less clearcut for I. monophylla and Triodia spp, where emergence was most limited in substrates comprised solely of overburden waste material and the lowest rainfall regime exacerbated emergence failure, relative to germination success. When compared to the well-watered, 100% topsoil substrate, seedling survival of all species was most constrained in the 100% overburden waste, demonstrating these reconstructed mining substrates compromise seedling recruitment.
Conclusions: This study underscores that successful seedling recruitment in this ecosystem is dependent on frequent, and repeated, rainfall events above a certain threshold (≥120 mm) and highlights the beneficial effects of sowing seeds in a substrate containing topsoil.
Implications: Future seeding technologies should focus on improving the moisture relations of the microsite to offset the recruitment challenges experienced by seeds sown in hostile growing environments such as the overburden wastes tested here.
Keywords: arid, emergence, germination envelope, plant demography, rehabilitation, restoration, seed enhancement, seed traits, soil water potential, systems approach.
Introduction
The success of seed-based restoration in dryland ecosystems relies upon seeds and seedlings enduring and overcoming a raft of harsh environmental conditions (Shackelford et al. 2021). Interacting abiotic and biotic environmental factors, including extreme temperatures, low and sporadic rainfall, and degraded soil conditions, all challenge the ability of sown seeds to establish (Merino-Martín et al. 2017a; James et al. 2019; Masarei et al. 2020). Seeds require a specific, and sometimes narrow, set of environmental conditions for germination. The properties of the soil or substrate into which seeds are sown heavily influences the seedbed microenvironment (e.g. temperature/moisture dynamics) and its favourability to germination and seedling recruitment (James et al. 2013; Merino-Martín et al. 2017a). Yet, there is still a limited understanding of how soil–moisture–seed interactions ultimately govern recruitment in the many and varied restoration scenarios faced by practitioners.
Degraded soils are commonly encountered at sites designated for restoration (Chazdon 2008; Heneghan et al. 2008). Overburden ‘waste’ dumps associated with open pit mining, for example, comprise uneconomical rock and mineral products, often from deep underground, and form substrates vastly altered in chemical, physical, and biological properties in comparison with undisturbed soils (Lamb et al. 2015; Bateman et al. 2021). For mine site restoration, topsoil is a preferred medium as a potential source of seeds, nutrients, and microorganisms supporting plant establishment and growth (Koch 2007; Muñoz-Rojas et al. 2016a; Golos et al. 2019). But long periods of stockpiling degrade the quality of the topsoil and the seeds within, and topsoil is frequently in short supply relative to the size of the disturbance footprint requiring restoration (Lamb et al. 2015). Therefore, restoration substrates into which seeds are sown may comprise pure waste material (Gillespie et al. 2015), or alternatively blends of wastes and topsoil in an effort to remediate any negative abiotic properties of wastes (e.g. poor water retention, low water holding capacity and nutrient availability) and to improve biological function (e.g. increase soil microbial activity) (Muñoz-Rojas et al. 2016b; Merino-Martín et al. 2017a). Nonetheless these reconstructed substrates can still have altered hydrology and depleted organic matter, nutrients and microbial diversity as compared to undisturbed soils (Muñoz-Rojas et al. 2016a). How seeds and plants interact with these reconstructed soils, including plant–soil feedbacks over the longer term (Muñoz-Rojas 2018; Yang et al. 2022), will ultimately influence the success, or otherwise, of restoration (Heneghan et al. 2008).
Plant recruitment from seeds is inherently risky in dryland ecosystems. Periods of favourable conditions of temperature and moisture availability are usually brief and infrequent, limiting opportunities for seed germination and seedling establishment (Lewandrowski et al. 2017; Leger et al. 2019; Hardegree et al. 2020). Consequently, recruitment and survival tend to be naturally low and episodic (Noy-Meir 1973; Shackelford et al. 2021). Variation in recruitment events between and within sites has been linked to rainfall timing (Ackerman 1979; Williams et al. 2002; Knutson et al. 2014) and the extent of this rainfall a major factor in the year to year variation in the availability of suitable microsite conditions for germination (Hardegree et al. 2013). Seed traits that have been shown to increase the likelihood of successful establishment in dryland restoration efforts include size, with larger seeds generally outperforming smaller seeds, such as for seeds of Acacia (Shackelford et al. 2021). Species that produce seed populations that are less dormant or that possess a wider germination envelope have also been shown to emerge more readily following sowing (Erickson et al. 2017; Lewandrowski et al. 2017).
The early developmental life stages of plants, including seeds and seedlings, are the most vulnerable to environmental change (Walck et al. 2011). By examining the demographic processes of seed recruitment and the transition of seeds through dormancy, non-dormancy, germination, seedling emergence, and establishment and survival, previous studies have found failure points to occur at different life stages, depending on the species and ecosystems. In the drylands of the USA, for example, James et al. (2011) found in a number of grasses (Agropyron desertorum, Elymus elymoides, Pseudoroegneria spicata) the transition from a germinated seed to an emerged seedling to have the highest rate of mortality. Whereas, Lewandrowski et al. (2017) showed that recruitment of the northern Australian dryland grasses Triodia epactia and Triodia wiseana was most dependent upon seeds successfully transitioning from the dormant to non-dormant state. Similar bottlenecks in recruitment due to seed dormancy have been observed across various Acacia species (perennial shrubs) from more southern dryland systems of Australia (Commander et al. 2020).
The poor plant establishment from sown seeds (commonly <10%) and the variation in early plant life-stage transitions most influential to establishment across different species and dryland restoration scenarios motivates research to identify key failure points to improve future seeding efforts. Our study was conducted in the context of informing seed management practices of significance to ecological restoration of highly degraded mine sites in dryland regions of Australia. Our study region, the Pilbara biogeographical region of Western Australia, holds a diverse hummock grassland and shrubland flora of >1800 species (Erickson and Merritt 2016) and vast areas are subject to disturbance due to open-cut mining operations (Erickson et al. 2017). The landscapes subject to restoration are challenging, with vastly altered soil substrates and topography, and sporadic and spatially variable rainfall (mean annual rainfall of 290 mm) combined with extreme water loss demand (i.e. potential evaporation 8- to 20-fold higher than the mean annual rainfall) (McKenzie et al. 2009). We set out to understand how reconstructed soil types and varying water availability, as key components of the abiotic environment, influence seedling recruitment. Our aim was to model for our study species the probability of seed germination, seedling emergence, and survival, to quantify early life-stage transitions and define those most constraining seedling recruitment under varying abiotic environmental scenarios. We selected five species considered key to successful restoration and representing differing plant lifeform and seed traits (shrubs with seeds of large to moderate size versus small-seeded grasses) to: (1) understand the influence of abiotic conditions (water availability and substrate type) on recruitment; (2) determine the probability of sown seeds transitioning through each of the key demographic life stages under the varying abiotic conditions for each plant species; and (3) interpret the results in order to guide the development of scalable intervention strategies that increase seedling recruitment and survival in future restoration programs.
Materials and methods
Study species
Seeds of five commonly occurring shrub and grass species from the Pilbara bioregion of Western Australia were utilised in this study: Acacia inaequilatera Domin, Acacia hilliana Maiden, Indigofera monophylla DC., Triodia pungens R.Br., and T. wiseana C.A. Gardner (species nomenclature follows Western Australian Herbarium 1998–). Both Acacia species are large-seeded (A. inaequilatera 17 seeds g−1, and A. hilliana 180 seeds g−1), perennial shrubs that make up a key component of mature plant communities of the Pilbara. Indigofera monophylla is a common leguminous shrub with moderately-sized seeds (313 seeds g−1) that is frequently seen immediately post-fire and contributes to the lower-storey perennial plant composition. Both Triodia species are small-seeded (T. pungens 800 seeds g−1 and T. wiseana 1300 seeds g−1) grasses that form the dominant grassland ecosystem of the Pilbara and are therefore the primary target plant lifeform for re-instatement at restoration sites.
Seed collections were sourced from wild plant populations in October 2009 for A. hilliana, in February–March 2011 for T. pungens and T. wiseana, in October 2011 for A. inaequilatera, and in October 2014 for I. monophylla. Upon receipt, in December 2014 for T. wiseana, and December 2015 for all other species, seed collections were stored in a controlled environment room maintained at 15–20°C and 15–20% relative humidity at Kings Park and Botanic Garden (Perth, Western Australia). Collections were only removed when required for experimental purposes.
Seed attributes, quality optimisation, and dormancy-break
Seeds of Triodia species are dispersed in single-seeded indehiscent florets (i.e. each floret is comprised of a lemma and palea). Florets, however, commonly possess very low fill rates (i.e. don’t contain a viable seed; average fill rate of 30%). Further, when present, seeds encased within florets commonly exhibit deep physiological dormancy (Erickson et al. 2016a). These characteristics limit the germination potential of many Triodia collections under both laboratory and field conditions (Erickson et al. 2016a, 2017; Lewandrowski et al. 2017, 2018). Therefore, prior to experiments, seeds were extracted from their covering floret structures by rubbing the florets on a ribbed rubber mat (see methods outlined in Erickson et al. 2016b). Hereafter, all references to Triodia seeds relate to these extracted seeds.
Seeds of all five species were cleaned further to maximise the quality of each batch before experimentation. Non-seed material (e.g. chaff and other plant debris) was separated from seed material via multiple passes through suitably sized sieves and via vacuum aspiration (‘Zig Zag’ Selecta, Machinefabriek, BV, Netherlands). Seed viability was maximised by removing empty, partially filled and non-viable seeds via X-ray analysis (Faxitron MX-20 Digital X-ray Cabinet; Tucson, Arizona, USA). A seed was deemed filled and viable when the X-ray image showed no abnormalities and the embryo and endosperm appeared uniform white-grey in the imagery. These seed cleaning and quality assessments ensured >99% filled and viable material was used during experimentation, thereby removing seed quality as a potential contributing factor to regeneration performance.
To ensure the highest possible germination capacity, seed batches were pre-treated to alleviate dormancy using the most reliable dormancy break treatment known at the time of experimentation, following Erickson et al. (2016b) and Lewandrowski et al. (2017). Seeds of the two Acacia species and I. monophylla are known to possess physical seed dormancy, which was alleviated by immersing seeds in hot water prior to experimentation: A. hilliana, 90–95°C for 2 min; A. inaequilatera, 90°C for 2 min; I. monophylla, 90°C for 1 min (Erickson et al. 2016c). As seed batches of the two Triodia species were collected a number of years prior to the experiments and stored under dry conditions, any physiological dormancy imposed within the seed was likely alleviated during storage, via after-ripening (Erickson et al. 2016b). Therefore, the removal of the florets and cleaning to pure live seeds was sufficient to maximise the germination potential of the two Triodia accessions.
Seed germination experiments
To quantify the impacts of water availability upon germination capacity for each species, seeds were incubated under laboratory conditions across a range of water potentials (0, −0.25, −0.50, −0.75, −1.00, −1.25, and −1.50 MPa) at each of three temperatures (25, 30 and 35°C) considered optimal for germination of many species from the Pilbara (Erickson et al. 2016b, 2017). Prior to germination testing, four replicates of 25 seeds for each species were surface sterilised in a 2% calcium hypochlorite (Ca[OCl]2) solution for 30 min under vacuum (10 min on-off-on, at −70 kPA), and rinsed three times in sterile water. Water potential was manipulated using different concentrations of polyethylene glycol (PEG-8000; Sigma-Aldrich Pty Ltd, Sydney, NSW, Australia) following the methods described in Michel (1983). Seeds were placed in 90 mm Petri dishes that contained one filter paper (Advantec, Dublin, California, USA) and one of the target PEG solutions combined with 0.2% v/v PPM™ (Plant Preservation Mixture, Austratec Pty. Ltd., Bayswater, Victoria, Australia) and 0.1% v/v Previcur (Propamocarb, Mirco Bros Henderson, WA, Australia) to reduce fungal contamination. Petri dishes were sealed with cling wrap to prevent moisture loss and placed in incubators at each temperature under a 12 h alternating light/dark regime (cool white fluorescent tubes (30 W) with a photon flux density of 30 μ mol m−2 s−1, 400–700 nm). Germination, defined as radicle emergence greater than one-third of the seed length, was assessed daily for the first 14 days and then periodically for an additional 14 days (i.e. 28 days in total).
Rain exclusion shelter layout, design features, and soil physicochemical attributes
The impact of reconstructed soil type and moisture availability on seed germination, emergence and survival were studied in the field in a purpose-built rain exclusion shelter (35 m × 40 m) constructed of galvanised steel tubing lined with a 180 μm thick, clear greenhouse film (10–30% UV transmission, 300–390 nm, >80% light transmission and diffusion; Shanghai HiTec Plastics Co., Ltd) and located at the Mt Whaleback mine site in Newman, Western Australia (23°21′41.8″S, 119°42′26.1″E). A broad summary of the facility can be found in Erickson et al. (2017). Within the shelter, 32 above-ground planter boxes were constructed at 400 cm (width) × 400 cm (depth) × 30 cm (height). Each planter box was divided lengthways with a pine beam to create 64 experimental plots in total (i.e. each plot was 400 cm × 200 cm × 30 cm or 2.4 m3 in size; Fig. 1).
Experiments were carried out in a purpose-built rain exclusion shelter in the Pilbara (top left) to unpack the seed recruitment potential of five species (Acacia inaequilatera, A. hilliana, Indigofera monophylla, Triodia pungens, and T. wiseana) commonly used in mine restoration programs. Seeds were precision sown (3–5 mm) in five reconstructed soil types and subjected to four simulated rainfall regimes that mimicked near-natural summer rainfall conditions experienced in the region. Recruitment success for each life-stage transition of germination, emergence, and survival at 6 weeks were then assessed (top right). Seeds for each species were randomly sown in four replicate zones, within each soil plot (n = 3) prior to the commencement of simulated rainfall (n = 4) (bottom trial schematic). Topsoil, 100% stockpiled topsoil or ‘G1’; MWB Waste, 100% waste from the Mt Whaleback mine or ‘G2’; ER Waste, 100% waste from the Eastern Ridge mine or ‘G3’; TS/MWB Blend, a 50:50 blend of topsoil and MWB waste or ‘G4’; TS/ER Blend, a 50:50 blend of topsoil and ER waste or ‘G5’. W1, 280 mm (daily events of 40 mm for 7 days); W2, 160 mm (four events of 40 mm every second day, over 7 days); W3, 120 mm (four events of 30 mm every second day, over 7 days); W4, 80 mm (four events of 20 mm every second day, over 7 days). Seeding lines (L1–L5) within each seeding zone are indicated.
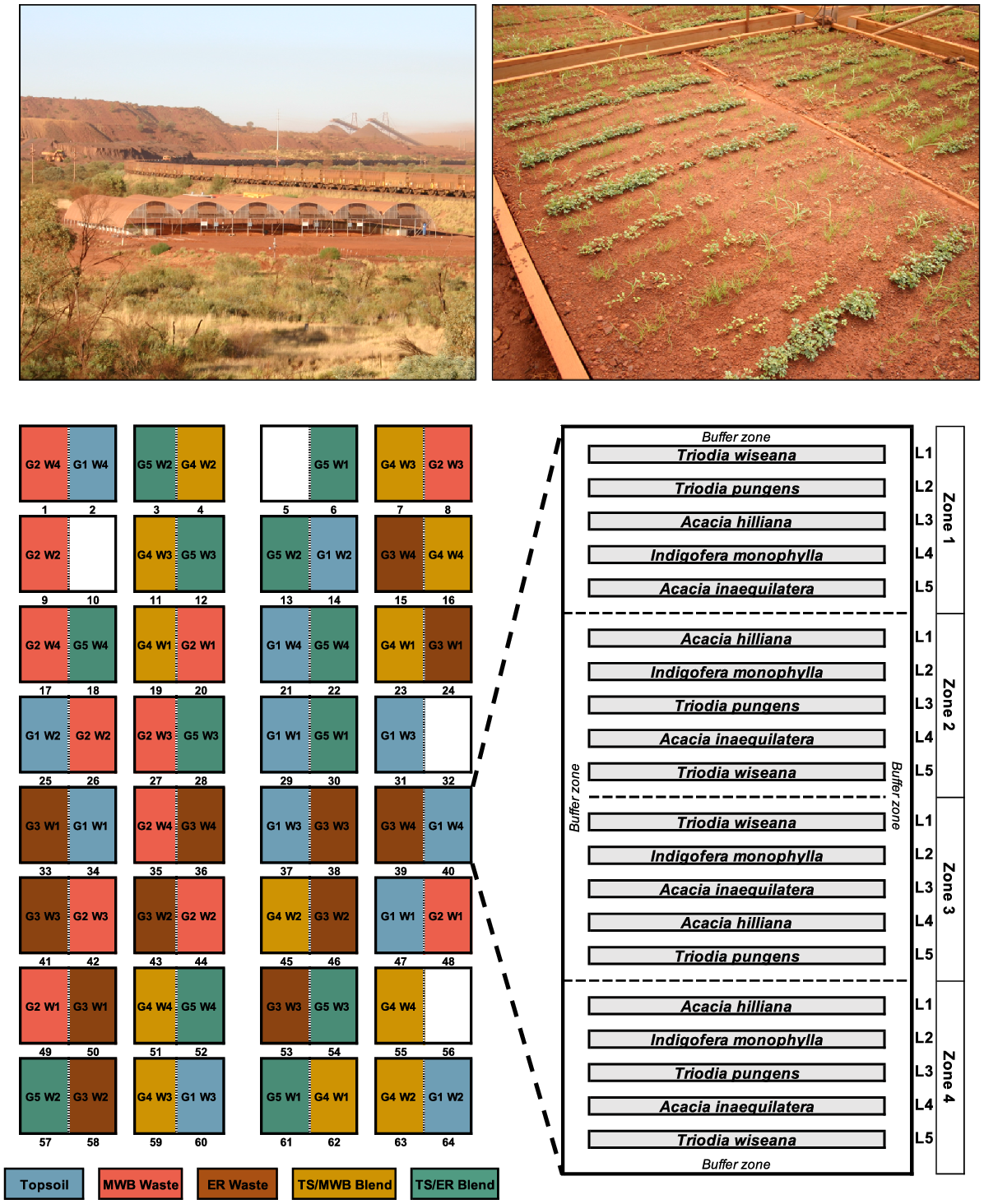
Five different reconstructed soil types commonly encountered in restoration programs in the region were each randomly allocated to 12 of the plots, resulting in a total of 60 experimental plots. These five reconstructed soil profiles comprised: (1) 100% stockpiled topsoil that was recovered prior to open-cut mining (‘TS’); (2) 100% overburden waste materials from the Mt Whaleback mine (‘MWB waste’); (3) 100% overburden waste materials from the Eastern Ridge mine (‘ER waste’); (4) a 50:50 blend of topsoil and MWB waste (‘TS/MWB Blend’); (5) a 50:50 blend of topsoil and ER waste (‘TS/ER Blend’).
Within the 12 plots containing the same reconstructed soil type, four simulated rainfall regimes were applied to three replicate plots (i.e. 3 × 4 = 12; described below). Irrigation was supplied to each planter box with a fully automated, pressurised watering system (up to 300 kPA) that simulated rainfall at a rate of 10 mm per h−1 (MP 2000 sprinkler heads; Hunter MP Rotator®, Hunter Industries, San Marcos, CA, USA). The environmental conditions within the shelter were monitored using remotely accessible, real-time data loggers (Hobo® RX3000 Remote Monitoring Station Data Logger; Onset Computer Corporation, Bourne, MA, USA) that logged soil moisture (EC-5 ECH2O Dielectric Aquameter; Decagon Devices, Inc.) and soil temperature at 5-min intervals. Soil moisture and temperature probes were installed ~50 mm below the soil surface in one planter box of each of the soil type × moisture combinations (i.e. five soil types × four rainfall scenarios; n = 20).
The soil physicochemical parameters for each of the five reconstructed soil types were obtained by taking three composite 500 g samples prior to the commencement of the experiment. To determine soil texture, the particle size of air-dried and sieved (<2 mm) samples was analysed by laser diffraction using a Mastersizer 2000 (Malvern Instruments, Malvern, England) after removing the organic matter with hydrogen peroxide (H2O2). Soil pH and electrical conductivity (EC) were measured in suspension using deionised water (1:5), with a pH meter (AD8000, Adwa, Hungary and pH Cube pH-ORP-Temp meter, TPS Pty Ltd, Australia). Total organic carbon was determined by dichromate oxidation using the Walkley–Black method (Walkley and Black 1934), and total nitrogen was determined using the Kjeldahl method (Bremner and Mulvaney 1982).
Seeding design
Each of the 60 plots were divided into four replicate seeding zones of 90 cm (width) × 160 cm (length). Each seeding zone was further sub-divided into five seeding lines that were 14 cm wide and 160 cm long, each separated with 4 cm buffer (Fig. 1). This resulted in 20 seeding lines per plot (i.e. four seeding zones × five seeding lines; n = 20). A buffer zone of 20 cm around the edge of the whole plot was retained to minimise potential edge effects. Seeds of each species were randomly assigned to one seeding line per zone at the following densities: A. hilliana (n = 450), A. inaequilatera (n = 500), I. monophylla (n = 500), T. pungens (n = 500), and T. wiseana (n = 800). The different sowing densities for each species reflected the varying levels of the germination capacity recorded in the initial laboratory assessments to ensure sufficient data (i.e. number of seedlings above ground) were available to quantify treatment effects on germination, emergence and seedling survival. Germination was assessed using the ‘burial bag’ method (James et al. 2011; Lewandrowski et al. 2017) whereby 40 seeds of each species were placed into fine-mesh nylon sachets (5 cm × 5 cm) and positioned half-way along their respective seeding line in zones 2 and 3 of each plot (germination bags were not included in zones 1 and 4). Once both the germination bag and seedling emergence lines were sown, all seeds were covered with up to 5 mm of the respective soil type for that plot, which was first sieved to achieve a particle size of <5 mm.
Application of irrigation to simulate rainfall regimes
The field trial commenced on the 31 December 2015 with irrigation supplied to the sown seeds to simulate the different rainfall regimes detailed below. The duration and frequency of irrigation was informed by findings of our previous research (Lewandrowski et al. 2017) which demonstrated that either a once-off, or two successive, rainfall events ranging between 12 mm (i.e. a summer thunderstorm) and 96 mm (i.e. a cyclonic rain system), failed to promote seedling emergence. We therefore varied the amount of irrigation applied for each event, while the frequency of the events were kept the same, as recommended by Lewandrowski et al. (2017). Three of the four simulated rainfall regimes comprised four discrete irrigation events of 20, 30 or 40 mm per day, applied on days 1, 3, 5, and 7, resulting in totals of 80, 120, and 160 mm, respectively, over a 7-day rainfall period. These events of between 20 and 40 mm per day of irrigation simulate the median through to the 75th percentile rainfall events over the Pilbara wet season (Lewandrowski et al. 2017). The fourth rainfall regime applied was designed for water to be ‘non-limiting’ and plots received a single irrigation event of 40 mm for each of the 7 days (i.e. a total of 280 mm).
Monitoring of seed germination, seedling emergence, and early seedling survival in field plots
Monitoring of the plots occurred 6 weeks after the commencement of the simulated rainfall regimes, in early February 2016. For each seeded line, individual plants were counted as ‘emerged’ if cotyledons or a coleoptile were visible above the soil surface. Emerged plants were further categorised as being alive or dead, whereby plants were deemed alive if some portion of the leaves was still green and pliable. The germination bags, which were placed in the centre of each seeding line in zones 2 and 3, were retrieved and transported to Kings Park and Botanic Garden, Perth, for inspection under a dissecting microscope. Seeds were evaluated and scored as (1) germinated, (2) predated or decayed (i.e. ‘dead’), or (3) filled and still viable, but not germinated.
Statistical analysis
All statistical analyses were performed using the R environment ver. 3.5.3 (R Core Team 2020) and implemented in RStudio ver. 1.3.1093 (RStudio Team 2020).
Base water potentials (Ψb50) of the germinable fraction of seeds from each species were determined using the ‘drc’ package in R (Ritz and Streibig 2005), as described in Lewandrowski et al. (2017). A model selection process using the function mselect() was used to compare a range of nonlinear, s-shaped curves (3- and 4- parameter log-logistic and Weibull functions), which were then ranked via the Akaike information criterion (AIC) and log-likelihood. Three-parameter log-logistic functions were most appropriate to describe the germination response across all species and were fitted in order to calculate the inflection point i.e. ‘e’; water potential at which germination was reduced by 50% (hereafter referred to as Ψb50) to reflect the germinable fraction of each species sown in the plots.
Germination, emergence and survival were modelled as separate but successive life-stage transitions. The models respectively provided estimates for each treatment combination of (1) the germination probability of viable seeds, (2) the emergence probability of seeds that germinated and (3) the survival probability (after 6 weeks) of seedlings that emerged. Each transition was modelled using a generalised linear mixed-effects model (GLMM) with binomial errors and logit link functions using the glmer() function in the ‘lme4’ package (Bates et al. 2015). In all models the fixed effects were soil type (five levels), rainfall regime (four levels) and their two-way interaction. Planter box and plot (nested in planter box) were included as random effects. For all models we calculated post hoc Dunnett’s contrasts using the emmeans() function in the ‘emmeans’ package (Lenth 2022). Specifically, we set the topsoil/280 mm rainfall treatment combination as the reference level (i.e. the most benign treatment combination) and compared all other treatment combinations to this level.
The germination dataset only included zones 2 and 3 from each plot and was therefore half the length of the emergence and survival datasets. The germination response variable was a concatenation of the number of viable seeds per bag that germinated and the number of viable seeds per bag that didn’t germinate. In some plots the germination proportion was lower than the proportion of sown seeds that emerged in the same line due to presumably the lower sample size of material available for germination combined with the vastly smaller spatial extent covered by the germination ‘burial bags’ (when compared to the sown emergence quantity and line length). In these instances, we made the conservative assumption that germination was equal to the proportion of sown seeds that emerged. To achieve this, we calculated the mean proportion of sown seeds that emerged for each plot half (zones 1 and 2 versus zones 3 and 4) and used these mean proportions to calculate the ‘corrected’ germination proportion for each bag (i.e. mean proportion of zones 1 and 2 was used to represent germination in zone 2). Finally, we expressed the mean proportion of sown seeds that emerged as a ‘corrected’ number of viable seeds that germinated in the corresponding germination bag for use in the GLMM.
The emergence model considered only the seeds that germinated. The response variable was a concatenation of the number of emerged seedlings and the number of seeds that germinated but didn’t emerge. The survival model considered only the seedlings that emerged. The response variable was a concatenation of the number of living seedlings after 6 weeks and the number of emerged seedlings.
To communicate the cumulative fate of seeds in each treatment combination, we used the model coefficient estimates and associated standard errors to generate probability distributions, each comprising 10 000 values, for each transition. We then simulated the fate of 1000 sown seeds in each treatment combination as they progressed through the life-stage transitions by multiplying 1000 by the successive probabilities of germinating, emerging and surviving. From the 10 000 resulting values we calculated the median and the 2.5th and 97.5th quantiles.
Results
Seed germination experiments
Germination in laboratory conditions in the absence of water stress (0 MPa) was high (>80%) for seeds of A. hilliana, A. inaequilatera and I. monophylla, and ranged between 35 and 57% for seeds of both Triodia species across the three incubation temperatures (Table 1). For seeds incubated at 25°C, modelled Ψb50 values were lower for both Acacia species (A. hilliana Ψb50 = −1.11 ± 0.025, A. inaequilatera Ψb50 = −1.04 ± 0.030 MPa) in comparison with seeds of T. pungens (Ψb50 = −0.61 ± 0.056 MPa), I. monophylla (Ψb50 = −0.54 ± 0.057 MPa), and T. wiseana (Ψb50 = −0.45 ± 0.059 MPa) (Table 1). Modelled Ψb50 values for each species became progressively higher as incubation temperature increased, and similar variation in Ψb50 between species was evident for each temperature, with Ψb50 of seeds of the Acacia species and T. pungens < I. monophylla and T. wiseana.
Species | 0 MPa (%) 25°C | Ψb50 25°C | 0 MPa (%) 30°C | Ψb50 30°C | 0 MPa (%) 35°C | Ψb50 35°C |
Acacia inaequilatera | 99 ± 0.01 | −1.04 ± 0.030 | 99 ± 0.01 | −0.82 ± 0.018 | 99 ± 0.01 | −0.68 ± 0.027 |
Acacia hilliana | 84 ± 0.04 | −1.11 ± 0.025 | 89 ± 0.04 | −1.08 ± 0.021 | 92 ± 0.04 | −0.79 ± 0.022 |
Indigofera monophylla | 84 ± 0.02 | −0.54 ± 0.057 | 82 ± 0.05 | −0.73 ± 0.025 | 89 ± 0.02 | −0.33 ± 0.024 |
Triodia pungens | 44 ± 0.06 | −0.61 ± 0.056 | 40 ± 0.03 | −0.93 ± 0.081 | 35 ± 0.09 | −0.69 ± 0.045 |
Triodia wiseana | 47 ± 0.03 | −0.45 ± 0.059 | 39 ± 0.1 | −0.32 ± 0.096 | 57 ± 0.03 | −0.37 ± 0.046 |
The analyses of soil physicochemical properties (Table 2) showed that the TS reconstructed substrate had the highest levels of C content (C = 2.2%) and the associated C to N ratio (C:N = 96.1) when compared to the TS/waste blends (C = 0.60–1.2%, C:N = 35.5–47.1), ER waste (C = 0.40%, C:N = 19.7) and MWB waste (C = 0.03%, C:N = 2.0). Particle size distributions for all five reconstructed soil types were similar, although, the ER waste presented higher levels of sand (82.4%), and therefore lower levels of silt (15.4%) and clay (2.2%). The combined clay and silt fine fractions were highest in the TS (35.6%) followed closely by all reconstructed soil types except the ER waste type (lowest clay-silt fine fraction of 17.6%). No abnormal values of EC (7.6–42.2 mS m−1) and pH (7.6–8.5) were observed.
Soil type | pH | EC | C (%) | N (%) | C:N | Clay (%) | Silt (%) | Sand (%) |
TS | 7.9 ± 0.1 | 42.2 ± 1.3 | 2.20 ± 0.1 | 0.03 ± 0.1 | 96.1 ± 25.6 | 4.7 ± 0.3 | 30.9 ± 1.9 | 64.4 ± 2.2 |
MWB | 7.8 ± 0.1 | 7.6 ± 0.3 | 0.03 ± 0.03 | 0.01 ± 0.1 | 2.0 ± 0.2 | 4.5 ± 0.5 | 28.7 ± 1.0 | 66.8 ± 1.0 |
ER | 7.6 ± 0.2 | 11.9 ± 2.6 | 0.40 ± 0.0 | 0.02 ± 0.1 | 19.7 ± 3.2 | 2.2 ± 0.4 | 15.4 ± 2.5 | 82.4 ± 2.9 |
MWB/TS | 8.5 ± 0.1 | 2.3 ± 0.5 | 0.60 ± 0.1 | 0.02 ± 0.1 | 35.5 ± 7.6 | 4.4 ± 0.7 | 29.4 ± 1.8 | 66.2 ± 2.1 |
ER/TS | 8.3 ± 0.1 | 30.5 ± 2.1 | 1.20 ± 0.1 | 0.03 ± 0.1 | 47.1 ± 3.2 | 4.0 ± 0.1 | 28.4 ± 0.7 | 67.6 ± 0.7 |
EC, electrical conductivity (mS m−1); C, total Carbon; N, total Nitrogen; C:N, Carbon to Nitrogen ratio. TS, 100% stockpiled topsoil; MWB, 100% waste from the Mt Whaleback mine; ER, 100% waste from the Eastern Ridge mine; MWB/TS, a 50:50 blend of topsoil and MWB waste; ER/TS, a 50:50 blend of topsoil and ER waste.
Seed germination in field plots
Across the five species and 20 treatment combinations (five soil types × four simulated rainfall regimes), the germination of sown seeds varied greatly between treatments and species (Figs 2, 3). Germination of seeds of the two Acacia species was significantly different due to the effect of simulated rainfall, irrespective of soil type or its interaction with rainfall treatment (P < 0.001; Table 3). For both Acacia species, the probability of germinating was highest at the non-limiting rainfall regime of 280 mm over 7 days (e.g. ≥0.61 across all soil types, and ≥0.94 in some TS and TS/waste combinations). When compared to the reference TS + 280 mm treatment, the probability of germination was similarly high across all soil types under the 160 mm and 120 mm rainfall regimes, but significantly lower at the 80 mm rainfall regime (Fig. 3).
Seed fate for each of the five species (Acacia inaequilatera, A. hilliana, Indigofera monophylla, Triodia pungens, and T. wiseana) was assessed by burying 40 seeds of each species in fine-mesh nylon sachets positioned within the their respective seeding line of each treatment combination (5 × reconstructed soils types, 4 × simulated moisture regimes/water treatments). After 6 weeks, the nylon bags were exhumed, evaluated under a dissecting microscope, and split into proportions defined as (1) ‘Dead’ = clearly predated or decayed, (2) ‘Viable’ = intact, firm, and filled upon dissection, or (3) ‘Germinated’ = evidence of radicle emergence. Topsoil, 100% stockpiled topsoil; MWB Waste, 100% waste from the Mt Whaleback mine; ER Waste, 100% waste from the Eastern Ridge mine; TS/MWB Blend, a 50:50 blend of topsoil and MWB waste; TS/ER Blend, a 50:50 blend of topsoil and ER waste. 280 mm, daily watering events of 40 mm for 7 days; 160 mm, four events of 40 mm every second day, over 7 days; 120 mm, four events of 30 mm every second day, over 7 days; 80 mm, four events of 20 mm every second day, over 7 days.
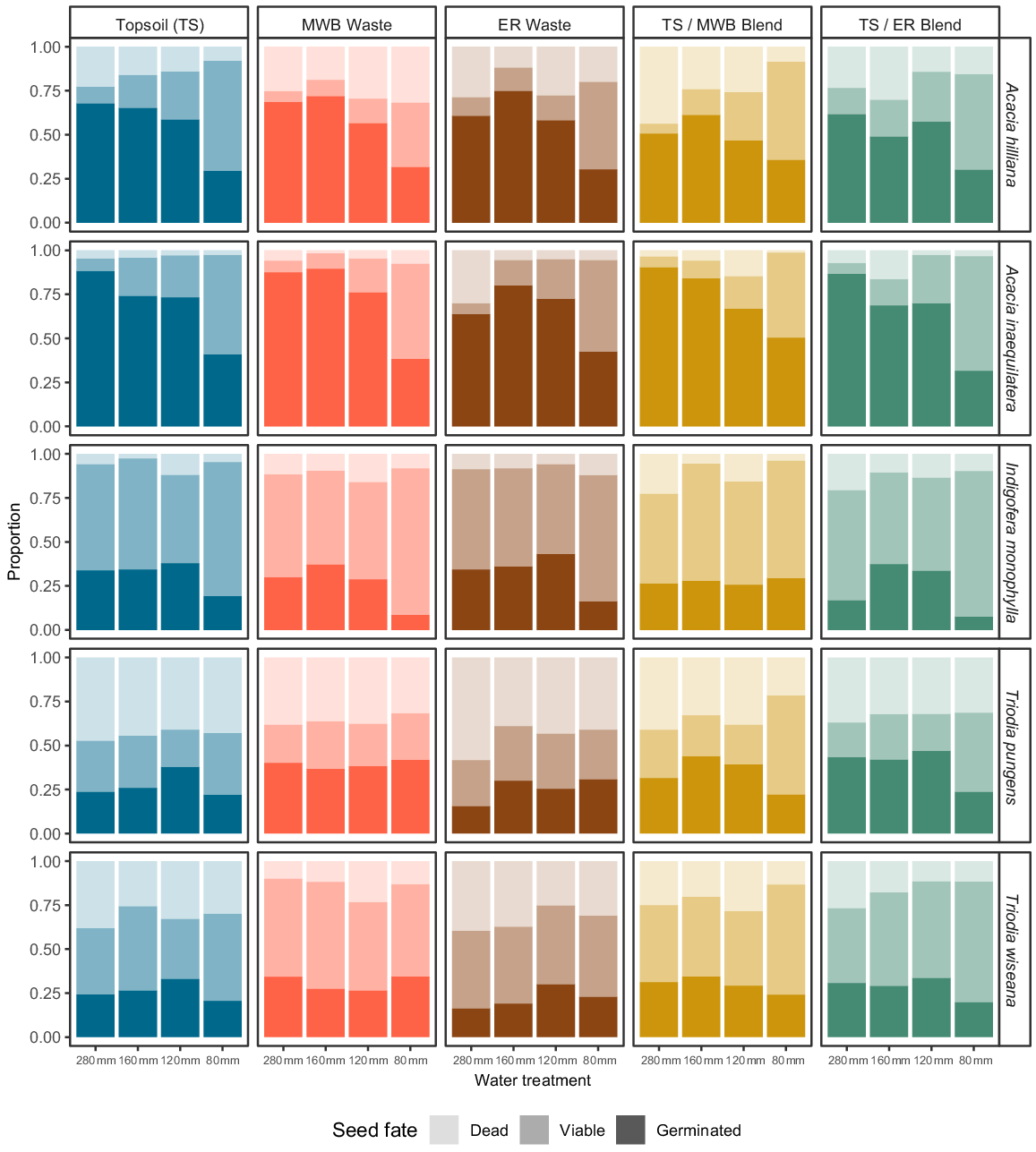
Probability of germinating and 95% confidence intervals for seeds of each of the five species (Acacia inaequilatera, A. hilliana, Indigofera monophylla, Triodia pungens, and T. wiseana) after being precision sown in one of 20 treatment combinations (5 × reconstructed soils types, 4 × simulated moisture regimes). Topsoil (TS), 100% stockpiled topsoil; MWB Waste, 100% waste from the Mt Whaleback mine; ER Waste, 100% waste from the Eastern Ridge mine; TS/MWB Blend, a 50:50 blend of topsoil and MWB waste; TS/ER Blend, a 50:50 blend of topsoil and ER waste. 280 mm, daily watering events of 40 mm for 7 days; 160 mm, four events of 40 mm every second day, over 7 days; 120 mm, four events of 30 mm every second day, over 7 days; 80 mm, four events of 20 mm every second day, over 7 days. Asterisks represent a significant difference when compared to the reference (Ref.) TS + 280 mm treatment.
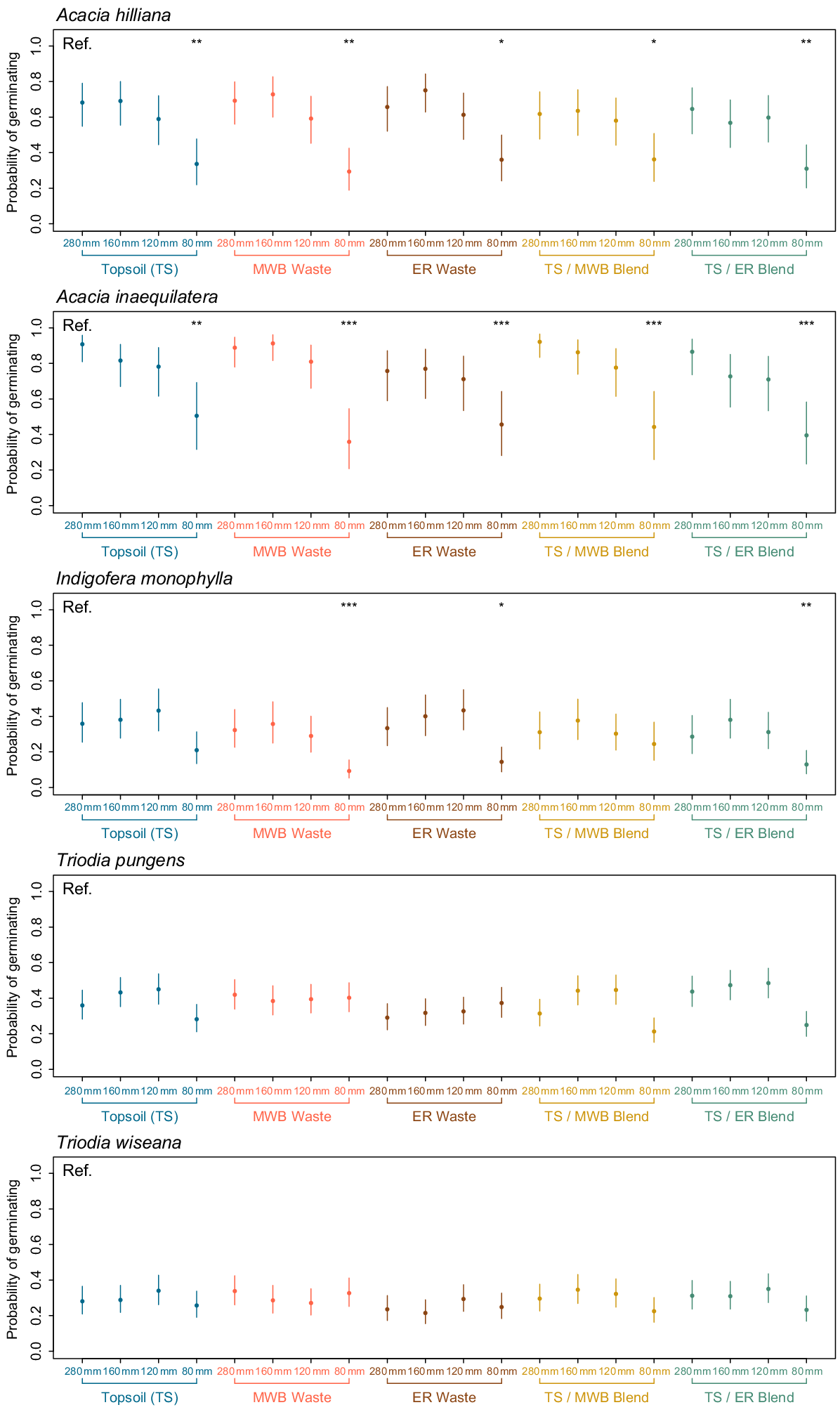
Model term | d.f. | F-ratio | P-value | |
Acacia hilliana | Rainfall | 3 | 17.467 | <0.001 |
Soil | 4 | 0.674 | 0.610 | |
Rainfall×Soil | 12 | 0.416 | 0.958 | |
Acacia inaequilatera | Rainfall | 3 | 17.920 | <0.001 |
Soil | 4 | 2.467 | 0.043 | |
Rainfall×Soil | 12 | 0.893 | 0.554 | |
Indigofera monophylla | Rainfall | 3 | 9.853 | <0.001 |
Soil | 4 | 2.793 | 0.025 | |
Rainfall×Soil | 12 | 1.153 | 0.311 | |
Triodia pungens | Rainfall | 3 | 5.358 | 0.001 |
Soil | 4 | 3.483 | 0.008 | |
Rainfall×Soil | 12 | 2.894 | 0.001 | |
Triodia wiseana | Rainfall | 3 | 1.084 | 0.354 |
Soil | 4 | 1.791 | 0.127 | |
Rainfall×Soil | 12 | 1.118 | 0.340 |
Unlike the Acacia species, the probability of seed germination of I. monophylla, T. pungens, and T. wiseana did not exceed 0.48 in any treatment and the effects of soil type, simulated rainfall, and their interaction, on germination was species specific. For seeds of I. monophylla and T. pungens, there were significant differences in germination probability due to water availability (P < 0.001 and P = 0.001, respectively) and soil type (P = 0.025 and P = 0.008, respectively), and also due the interaction of water availability and soil type for T. pungens (P = 0.001) (Table 3). As for seeds of the Acacia species, germination probability of I. monophyla seeds was significantly lower at the 80 mm rainfall regime across most soil types when compared against the TS + 280 mm reference treatment (Fig. 3). For seeds of T. wiseana no significant difference in germination probability was observed in any treatment.
For the Acacia seeds that did not germinate, more viable seeds tended to remain following the lower rainfall regime of 80 mm, irrespective of soil type, and for both species, 64% of retrieved seeds were viable in the TS + 80 mm rainfall treatment (Fig. 2). Seed death was less pronounced across both soil type and rainfall treatment for these Acacia seeds with only the occasional soil type + rainfall combination having moderate levels of loss (e.g. 43% ‘dead’ in the TS/MWB blend + 280 mm treatment, Fig. 2). Most of the ungerminated seeds of I. monophylla remained viable, regardless of soil type or rainfall regime (Fig. 2). For seeds of the two Triodia species, a larger proportion of seeds appeared to be lost from the soil seed bank via decay or predation (i.e. ‘dead’; Fig. 2).
Emergence outcomes
For the two Acacia species, the probability of successful emergence (pr) following germination was >0.61 across all treatment combinations (Fig. 4) and was significantly influenced by soil type (P < 0.001; Table 4), with emergence generally lower in the MWB waste and ER waste. When compared to the TS + 280 mm rainfall reference (pr = 0.94), the probability of successful emergence for seeds of A. inaequilatera was significantly lower in the MWB waste under the 80 mm (pr = 0.67, P < 0.01) and 120 mm rainfall regimes (pr = 0.69, P < 0.01), and in the ER waste receiving 160 mm (pr = 0.68, P < 0.01) and 280 mm (pr = 0.70, P < 0.01) of rainfall (Fig. 4). Conversely, for seeds of I. monophylla, rainfall regime, rather than soil type, was the factor that significantly influenced the likelihood of emergence (P = 0.003; Table 4) with emergence generally lower when less water was available.
Probability of emergence and 95% confidence intervals for seeds of each of the five species (Acacia inaequilatera, A. hilliana, Indigofera monophylla, Triodia pungens, and T. wiseana) after being precision sown in one of 20 treatment combinations (5 × reconstructed soils types, 4 × simulated moisture regimes). Topsoil (TS), 100% stockpiled topsoil; MWB Waste, 100% waste from the Mt Whaleback mine; ER Waste, 100% waste from the Eastern Ridge mine; TS/MWB Blend, a 50:50 blend of topsoil and MWB waste; TS/ER Blend, a 50:50 blend of topsoil and ER waste. 280 mm, daily watering events of 40 mm for 7 days; 160 mm, four events of 40 mm every second day, over 7 days; 120 mm, four events of 30 mm every second day, over 7 days; 80 mm, four events of 20 mm every second day, over 7 days. Asterisks represent a significant difference when compared to the reference (Ref.) TS + 280 mm treatment.
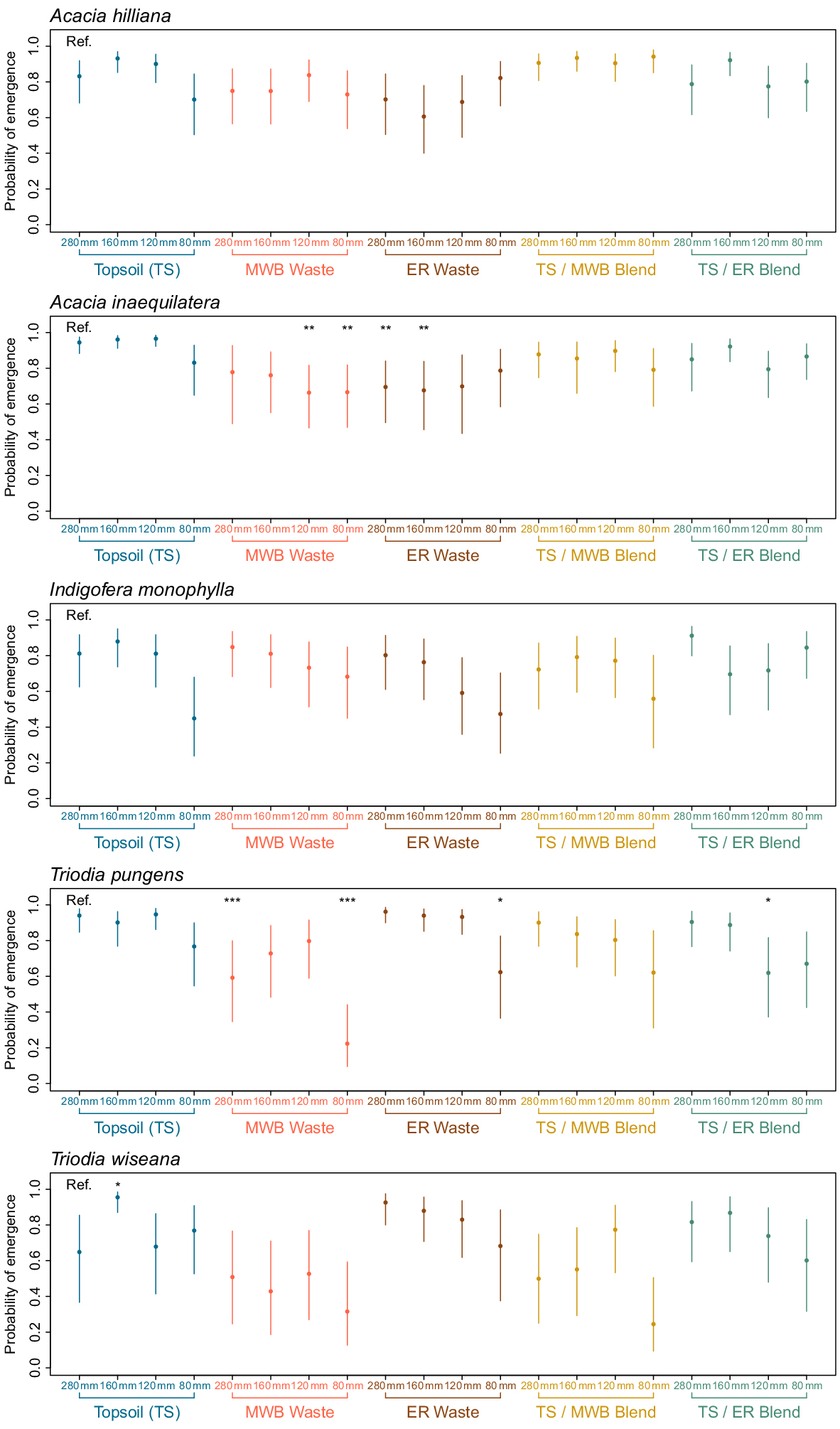
Model term | d.f. | F-ratio | P-value | |
Acacia hilliana | Rainfall | 3 | 0.917 | 0.432 |
Soil | 4 | 7.596 | <0.001 | |
Rainfall×Soil | 12 | 1.502 | 0.115 | |
Acacia inaequilatera | Rainfall | 3 | 1.102 | 0.347 |
Soil | 4 | 13.726 | <0.001 | |
Rainfall×Soil | 12 | 1.337 | 0.189 | |
Indigofera monophylla | Rainfall | 3 | 4.568 | 0.003 |
Soil | 4 | 1.348 | 0.249 | |
Rainfall×Soil | 12 | 1.236 | 0.251 | |
Triodia pungens | Rainfall | 3 | 4.709 | 0.003 |
Soil | 4 | 16.619 | <0.001 | |
Rainfall×Soil | 12 | 2.083 | 0.015 | |
Triodia wiseana | Rainfall | 3 | 3.778 | 0.010 |
Soil | 4 | 9.990 | <0.001 | |
Rainfall×Soil | 12 | 1.605 | 0.083 |
For the emergence of seeds of T. pungens there was a significant interaction between rainfall regime and soil type observed (P = 0.015; Table 4). Emergence probability was generally high (0.80–0.90) under the rainfall regimes ≥160 mm in most substrate types, but was reduced in the MWB waste under all rainfall regimes (Fig. 4), and significantly lower in the MWB and ES waste receiving 80 mm rainfall, and the TS/ER blend receiving 120 mm rainfall (Fig. 4). Similarly, the probability of successful emergence of seeds of T. wiseana was significantly influenced by both water availability (P = 0.010) and soil type (P < 0.001) (Table 4). For instance, the probability of successful emergence was highest for seeds in the well-watered (≥160 mm rainfall regimes) TS, ER waste and TS/ER blend substrates (pr ranging between 0.65 and 0.96), while the probability of emergence was lower for seeds in the MWB waste and the TS/MWB waste blend (ranging between 0.25 and 0.77) (Fig. 4). However, in comparison to the reference treatment, these effects were not statistically significant due to the large amount of variation observed between replicate treatments.
Survival outcomes
The probability of surviving after 6 weeks post-emergence for all species was influenced by the interaction of both water availability and soil type (P ≤ 0.004; Table 5). For seeds of A. hilliana, the likelihood of successful survival was ≤50%, and was significantly reduced in many of the MWB and ER waste substrates when compared to the TS + 280 mm reference (i.e. P < 0.01 for the majority of treatment combinations (Fig. 5). Similarly, seeds of A. inaequilatera were less likely to survive in the ER soil type across all rainfall treatments, along with the majority of the TS/ER blend and the MWB receiving 80 mm of rainfall, when compared to the TS + 280 mm reference (see asterisks in Fig. 5).
Model term | d.f. | F-ratio | P-value | |
Acacia hilliana | Rainfall | 3 | 4.008 | 0.007 |
Soil | 4 | 40.251 | <0.001 | |
Rainfall×Soil | 12 | 2.431 | 0.004 | |
Acacia inaequilatera | Rainfall | 3 | 2.133 | 0.094 |
Soil | 4 | 42.275 | <0.001 | |
Rainfall×Soil | 12 | 4.706 | <0.001 | |
Indigofera monophylla | Rainfall | 3 | 1.234 | 0.295 |
Soil | 4 | 57.577 | <0.001 | |
Rainfall×Soil | 12 | 2.434 | 0.004 | |
Triodia pungens | Rainfall | 3 | 19.27 | <0.001 |
Soil | 4 | 24.843 | <0.001 | |
Rainfall×Soil | 12 | 9.997 | <0.001 | |
Triodia wiseana | Rainfall | 3 | 16.009 | <0.001 |
Soil | 4 | 32.793 | <0.001 | |
Rainfall×Soil | 12 | 9.315 | <0.001 |
Probability of survival and 95% confidence intervals for seeds of each of the five species (Acacia inaequilatera, A. hilliana, Indigofera monophylla, Triodia pungens, and T. wiseana) after being precision sown in one of 20 treatment combinations (5 × reconstructed soils types, 4 × simulated moisture regimes). Topsoil (TS), 100% stockpiled topsoil; MWB Waste, 100% waste from the Mt Whaleback mine; ER Waste, 100% waste from the Eastern Ridge mine; TS/MWB Blend, a 50:50 blend of topsoil and MWB waste; TS/ER Blend, a 50:50 blend of topsoil and ER waste. 280 mm, daily watering events of 40 mm for 7 days; 160 mm, four events of 40 mm every second day, over 7 days; 120 mm, four events of 30 mm every second day, over 7 days; 80 mm, four events of 20 mm every second day, over 7 days. Asterisks represent a significant difference when compared to the reference (Ref.) TS + 280 mm treatment.
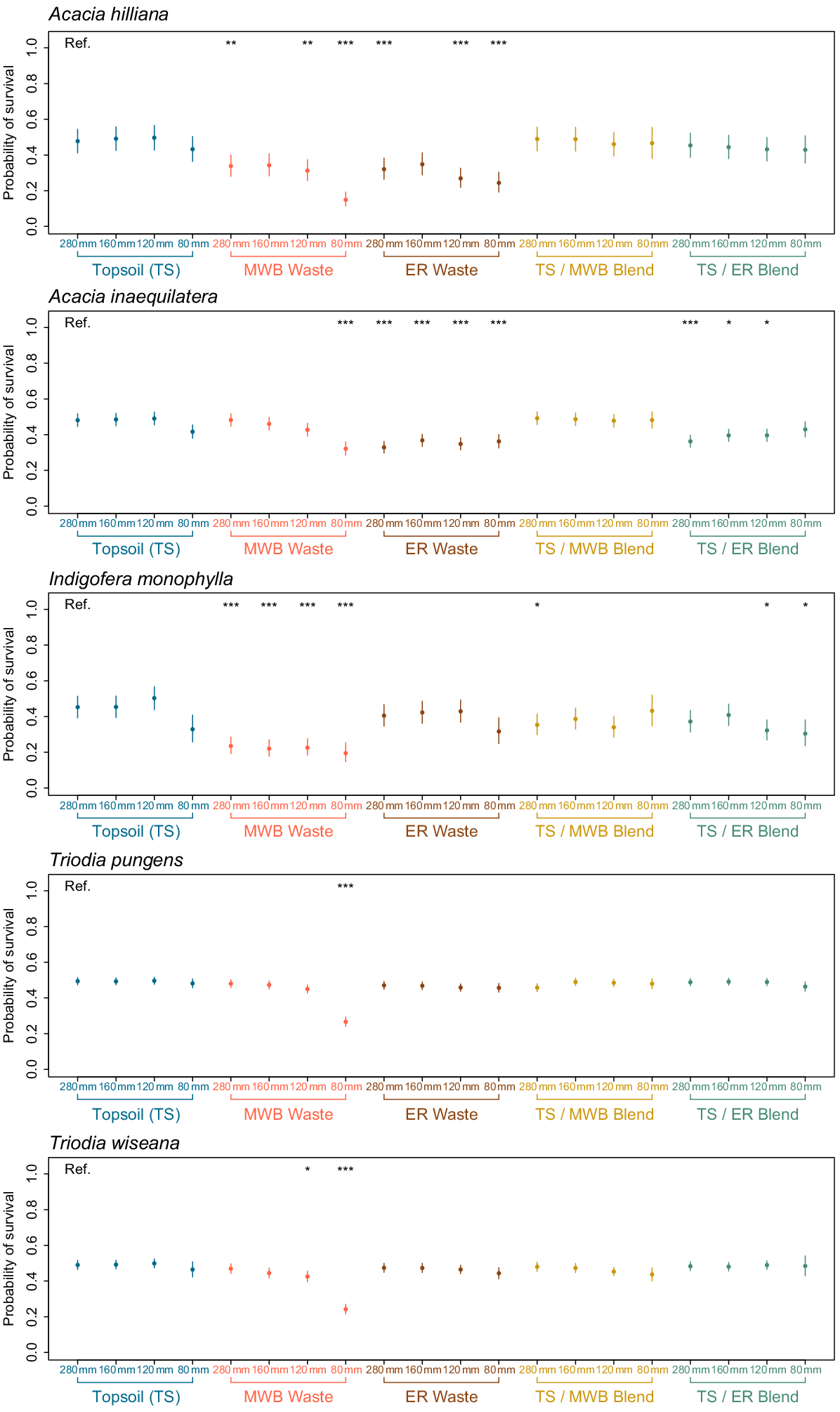
The probability of survival was most restricted for seeds of I. monophylla (pr ≤ 0.24) in the MWB waste, even under the high rainfall treatment (Fig. 5). Significant reductions in the probability of survival were also observed in the TS/MWB + 280 mm treatment and in the TS/ER + 80–120 mm treatments.
Once seedlings had successfully emerged for both T. pungens and T. wiseana, the probability of surviving was relatively constant (Fig. 5). However, the probability of survival was significantly lower for the MWB + 80 mm event for T. pungens, and significantly lower in the MWB waste + 120 and 80 mm events for T. wiseana, when compared to the TS + 280 mm reference (asterisks in Fig. 5).
Discussion
The successful establishment of seedlings from seeds sown in dryland restoration efforts is challenged by many abiotic (e.g. altered soil properties) and biotic (e.g. seed traits including dormancy-breaking and germination requirements) factors. In this study we quantified seed and seedling life-stage transitions in a range of reconstructed soils and across varying levels of simulated rainfall. For four of our species, we found both rainfall amount and/or soil substrate to significantly influence the probability of germination, with rainfall being the more significant determinant of germination success. For example, non-dormant seeds of A. inaequilatera and A. hilliana germinated readily, irrespective of soil type, under the higher simulated rainfall regimes of 120–280 mm. At the lowest rainfall amount (80 mm) germination was consistently reduced across all soil types. For seeds of I. monophylla and T. pungens, although germination was low to moderate, similar trends in reduced germination at the lowest 80 mm rainfall amount were observed. Soil moisture availability is commonly the factor most limiting to the germination of non-dormant seeds in restoration programs in dryland environments. For example, for a range of Acacia species in a dryland system nearby our study site, Merino-Martín et al. (2017b) have demonstrated a similar germination reduction when moisture was limited under both glasshouse and field trial conditions.
Our data reinforce that the initiation of germination in these dryland environments is linked to frequent and repeated rainfall events above a certain threshold amount. In a previous study on Triodia species, Lewandrowski et al. (2017) demonstrated the importance of frequent rainfall events to germination whereby four events of 24 mm (over a 7-day period) in respread topsoil promoted up to 35% germination for Triodia seeds (i.e. seeds cleaned out of florets; the least dormant state evaluated). Yet, a single, or two successive events, of rainfall ranging between 12 and 96 mm failed to elucidate germination (i.e. <5% germination recorded). The higher germination can be attributed to the frequent rainfall contributing to maintenance of soil water potentials above a certain threshold for a long enough period to allow the completion of germination (Rawlins et al. 2012; Lewandrowski et al. 2017). In the present study, for seeds of both Triodia species there was a less pronounced decrease in germination at the lower rainfall amount treatment (80 mm) and germination was instead similar across all rainfall regimes, suggesting that the moisture conditions experienced across all treatments was sufficient to promote germination in the non-dormant fraction of the sown material in all the soil types.
While germination is a critical step in plant recruitment, the first few days to weeks are also critical for the transition to an emergent seedling (Larson et al. 2020). The germinants must continue to develop their root systems as well as penetrate the soil surface to successfully complete the emergence transition. The success of this life-stage transition is largely dependent on continual access to plant available water and a soil microenvironment that supports these early growth stages. Soil crusting due to higher clay content or via the use of mine overburden, for instance, has previously been suggested to limit seedling emergence (Grigg et al. 2006; James et al. 2011; Madsen et al. 2012). Further, soils remade with overburden waste material lack structure and sufficient levels of organic matter and possess a reduced maximum water holding capacity (Spargo and Doley 2016). This translates into less rainfall infiltrating and being held in the soil profile and available for use by young seedlings (Merino-Martín et al. 2017b). For both Acacia species in this study, the highest proportion of successful emergence occurred in the reconstructed soil types that contained topsoil (i.e. >80%) suggesting the microenvironment provided by soils containing topsoil here was more favourable for seedling emergence when compared to the emergence performance of the substrates containing the mining overburden (Muñoz-Rojas et al. 2016b). However, for the seeds of Indigofera and the two Triodia species, the probability of emergence of germinated seeds was less clearcut, with respect to the influence of substrate type. Both the reconstructed soil types and water availability influenced the performance of these species and it appears emergence is most limited when seedlings are growing in the substrates comprised solely of overburden waste material (particularly the MWB waste), and less so the blends, with the lowest rainfall regime exacerbating emergence failure (relative to germination success). Waste types such as the MWB and ER, originate from deep within the mine pit and differ considerably in physicochemical characteristics (Grigg et al. 2006; Bateman et al. 2021) and, particularly when drying or dry, can form an impenetrable upper soil crust. Such crusting was observed in this trial for the MWB following irrigation whereby this reconstructed soil type possessed a very hard ‘cement’ layer that would not be favourable for emergence. The MWB also had the lowest levels of carbon, likely resulting in a less favourable water holding capacity.
Seedling survival was similarly influenced by both water availability, the growing environment, and their interactions. When compared to the well-watered, reconstructed soil type containing 100% topsoil, survival was most constrained for the soil types comprised of 100% overburden waste, providing further evidence that these reconstructed mining substrates compromise seedling recruitment. However, seedling survival of the Acacia spp. was relatively higher in the topsoil and waste blends, particularly when compared to the 100% MWB waste, highlighting that blending topsoil and waste may contribute to overcoming some of the detrimental impacts of waste material on recruitment. Whether lower concentrations of topsoil than used in this study, such as a 25:75 topsoil:waste blend, could be used remains to be evaluated, but this approach has shown promise under glasshouse conditions (Muñoz-Rojas et al. 2016b). Alternatively, the use of amendments such as mulch, biosolids, and leaf litter has also been shown to ameliorate some of the hostile properties of waste material (Grigg et al. 2006; Spargo and Doley 2016; Parry et al. 2022) by increasing water retention and improving substrate structure (Luna et al. 2018; Veblen et al. 2022). Nonetheless the impracticalities of scaling the use of either topsoil for blending with waste or the use of alternative amendments across mining operations such the one found in this Pilbara study system (disturbance footprint exceeds 300 000 ha), will likely restrict industry-wide adoption. More efficient spreading and use of limited topsoil resources is the more likely option for improving the growing conditions of waste material (Stock et al. 2020).
Considering the cumulative progression of seeds through all life stages (Fig. 6) provides some insights into future restoration strategies and focus areas for research. Germination remains a major limiting step for the smaller-seeded grass species, and to a lesser extent for I. monophylla (i.e. germination did not exceed 50%), despite our efforts to alleviate dormancy prior to sowing, but is less of a problem for the Acacia species. The majority of the sown seeds of Triodia failed to germinate under all watering regimes, including the ‘non-limiting’ 280-mm treatment. However, the ungerminated fraction of the sown seeds remained viable for the duration of the study, in all soil profiles (Fig. 2). It appears that (partially) unresolved physiological dormancy is still contributing to lower recruitment of Triodia spp., and dormancy remains the largest bottleneck for this keystone species, and for many other species in the region (Erickson et al. 2017). Pre-sowing treatment solutions need to be developed more to improve the reliability of dormancy alleviation for species with complex physiological dormancy. For example, recent studies have consistently produced up to 40% emergence of Triodia seedlings through removing the floret structures and priming the seeds with karrikinolide to increase germination potential (Erickson et al. 2019, 2021; Kildisheva 2019).
Representation of the cumulative life-stage transitions of germination, emergence and survival for seeds of each of the five species (Acacia inaequilatera, A. hilliana, Indigofera monophylla, Triodia pungens, and T. wiseana) after being precision sown in one of 20 treatment combinations (5 × reconstructed soils types, 4 × simulated moisture regimes). Topsoil (TS), 100% stockpiled topsoil; MWB Waste, 100% waste from the Mt Whaleback mine; ER Waste, 100% waste from the Eastern Ridge mine; TS/MWB Blend, a 50:50 blend of topsoil and MWB waste; TS/ER Blend, a 50:50 blend of topsoil and ER waste. 280 mm, daily watering events of 40 mm for 7 days; 160 mm, four events of 40 mm every second day, over 7 days; 120 mm, four events of 30 mm every second day, over 7 days; 80 mm, four events of 20 mm every second day, over 7 days. Maximum laboratory germination (and 95% confidence interval) at 25°C is depicted by the grey shading. The dotted errors bars represent the 95% confidence interval for each individual life-stage transition assessed.
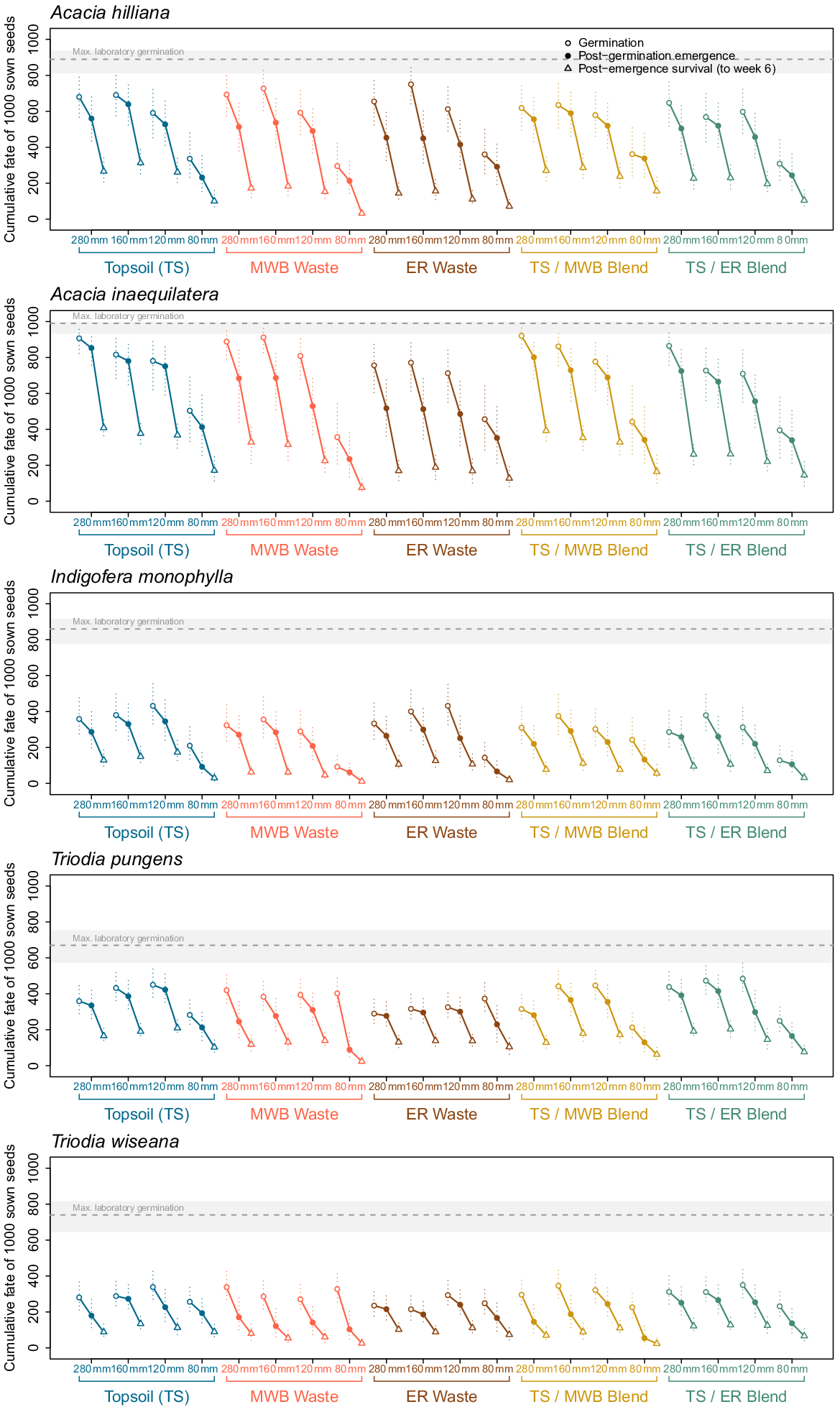
Coupled with this greater germination success from less dormant seed material, future research needs to focus on the possibility of discovering reoccurring ecological patterns to overcome these recruitment barriers. Larson et al. (2015), for instance, demonstrated that seed mass was a strong predictor of germination success in grasses, and therefore larger seeds were more likely to survive, in the drylands of the USA. Leger et al. (2019) also found for grasses that larger seeds produced larger roots in the first 10 days, and that seed size and early seedling root development are traits that are strong predictors of restoration success. Likewise, larger seeds, in general, have been shown to have a positive influence on species presence in restoration programs (Shackelford et al. 2021). It is notable in the present study that the two larger-seeded Acacia species could germinate under lower water potentials in the laboratory, as compared to seeds of the Indigofera and the two Triodia species (Table 1). This suggests that the Acacia seeds possessed a wider germination envelope and may respond to available soil moisture either much earlier, or for a longer period, than other the other species evaluated. Future efforts, however, should not rule out the ability to manipulate the recruitment potential of small seeds. For instance, Triodia seeds hydro-primed in a solution of karrikinolide Kildisheva (2019) demonstrated an increase in emergence potential and greater root growth performance in drier soils.
The data showing seedling emergence (following germination) generally proceeding under the conditions tested, suggests that the frequent rainfall events (i.e. four events over a 7-day period, ≥120 mm in total), was an important driver to kick-start recruitment processes for many focal species in this Pilbara ecosystem. For T. pungens the probability of emergence was influenced by a strong interaction between soil type and moisture availability (e.g. emergence was most constrained in the 100% waste types when moisture was most limiting). This significant soil type by moisture availability interaction was also evident for survival probabilities for all species.
Seeding technologies focussed on improving the moisture relations of the microsite may help to offset the recruitment challenges experience in both hostile growing environments (e.g. the MWB waste tested here) and when water is most limited (Golos et al. 2019). Stock et al. (2020), for instance, has demonstrated that seeds deployed inside extruded pellets containing a 1:1 blend of topsoil and mine waste can promote seedling emergence in T. pungens. This provides a new avenue to pursue in future seeding efforts whereby seed treatments can be combined with beneficial media that optimise seed–soil–moisture contact and inoculation with locally-sourced microbiota (Stock et al. 2020; Dadzie et al. 2022). If proven feasible, extruded pellets offer a solution to spread limited topsoil in a much more targeted manner and potentially >100-fold further than broadacre spreading (Stock et al. 2020).
Data availability
All data and R script is available via Figshare – https://doi.org/10.6084/m9.figshare.22790924.v1
Declaration of funding
TEE, ELD, MMR and DJM acknowledge the financial support provided by BHP Western Australia Iron Ore Community Development Project (contract no. 8 600 048 550) under the auspices of the Restoration Seedbank Initiative (2013–2018), a partnership between BHP Western Australia Iron Ore, The University of Western Australia, and the Botanic Gardens and Parks Authority.
Acknowledgements
TEE and DJM designed the study, JMD and ELD analysed the data, MMR and JJJ provided expert inputs to the findings, TEE, ELD, DJM drafted the manuscript, all authors contributed to the final version of the writing.
References
Ackerman TL (1979) Germination and survival of perennial plant species in the Mojave Desert. The Southwestern Naturalist 24, 399–408.| Germination and survival of perennial plant species in the Mojave Desert.Crossref | GoogleScholarGoogle Scholar |
Bateman AM, Erickson TE, Merritt DJ, Veneklaas EJ, Muñoz-Rojas M (2021) Native plant diversity is a stronger driver for soil quality than inorganic amendments in semi-arid post-mining rehabilitation. Geoderma 394, 115001
| Native plant diversity is a stronger driver for soil quality than inorganic amendments in semi-arid post-mining rehabilitation.Crossref | GoogleScholarGoogle Scholar |
Bates D, Mächler M, Bolker B, Walker S (2015) Fitting linear mixed-effects models using lme4. Journal of Statistical Software 67, 1–48.
| Fitting linear mixed-effects models using lme4.Crossref | GoogleScholarGoogle Scholar |
Bremner JM, Mulvaney CS (1982) Nitrogen-total. In ‘Methods of soil analysis. Part 2’. Agronomy Monographs 9. 2nd edn. (Eds AL Page, RH Miller) pp. 595–624. (ASA and SSSA: Madison, WI, USA)
Chazdon RL (2008) Beyond deforestation: restoring forests and ecosystem services on degraded lands. Science 320, 1458–1460.
| Beyond deforestation: restoring forests and ecosystem services on degraded lands.Crossref | GoogleScholarGoogle Scholar |
Commander LE, Merino-Martín L, Elliott CP, Miller BP, Dixon K, Stevens J (2020) Demographic, seed and microsite limitations to seedling recruitment in semi-arid mine site restoration. Plant and Soil 457, 113–129.
| Demographic, seed and microsite limitations to seedling recruitment in semi-arid mine site restoration.Crossref | GoogleScholarGoogle Scholar |
Dadzie FA, Moles AT, Erickson TE, Slavich E, Muñoz-Rojas M (2022) Native bacteria and cyanobacteria can influence seedling emergence and growth of native plants used in dryland restoration. Journal of Applied Ecology 59, 2983–2992.
| Native bacteria and cyanobacteria can influence seedling emergence and growth of native plants used in dryland restoration.Crossref | GoogleScholarGoogle Scholar |
Erickson TE, Merritt DJ (2016) Introduction to plant diversity of the Pilbara. In ‘Pilbara seed atlas and field guide: plant restoration in Australia’s arid northwest’. (Eds TE Erickson, RL Barrett, DJ Merritt, KW Dixon) pp. 1–6. (CSIRO Publishing: Dickson, ACT, Australia)
Erickson TE, Shackelford N, Dixon KW, Turner SR, Merritt DJ (2016a) Overcoming physiological dormancy in seeds of Triodia (Poaceae) to improve restoration in the arid zone. Restoration Ecology 24, S64–S76.
| Overcoming physiological dormancy in seeds of Triodia (Poaceae) to improve restoration in the arid zone.Crossref | GoogleScholarGoogle Scholar |
Erickson TE, Barrett RL, Symons DR, Turner SR, Merritt DJ (2016b) An atlas to the plants and seeds of the Pilbara region. In ‘Pilbara seed atlas and field guide: plant restoration in Australia’s arid northwest’. (Eds TE Erickson, RL Barrett, DJ Merritt, KW Dixon) pp. 43–256. (CSIRO Publishing: Dickson, ACT, Australia)
Erickson TE, Merritt DJ, Turner SR (2016c) Overcoming physical seed dormancy in priority native species for use in arid-zone restoration programs. Australian Journal of Botany 64, 401–416.
| Overcoming physical seed dormancy in priority native species for use in arid-zone restoration programs.Crossref | GoogleScholarGoogle Scholar |
Erickson TE, Munoz-Rojas M, Kildisheva OA, et al. (2017) Benefits of adopting seed-based technologies for rehabilitation in the mining sector: a Pilbara perspective. Australian Journal of Botany 65, 646–660.
| Benefits of adopting seed-based technologies for rehabilitation in the mining sector: a Pilbara perspective.Crossref | GoogleScholarGoogle Scholar |
Erickson TE, Munoz-Rojas M, Guzzomi AL, et al. (2019) A case study of seed-use technology development for Pilbara mine site rehabilitation. In ‘13th International conference on mine closure’. (Eds AB Fourie, M Tibbett) pp. 679–692. (Australian Centre for Geomechanics: Perth) Available at https://papers.acg.uwa.edu.au/p/1915_54_Erickson/
Erickson TE, Kildisheva OA, Baughman OW, Breed MF, Ruiz-Talonia L, Brown VS, Madsen MD, Merritt DJ, Ritchie AL (2021) FloraBank guidelines module 12 – seed enhancement technologies. In ‘FloraBank guidelines’. 2nd edn. (Ed. LE Commander). (FloraBank Consortium: Australia)
Gillespie M, Glenn V, Doley D (2015) Reconciling waste rock rehabilitation goals and practice for a phosphate mine in a semi-arid environment. Ecological Engineering 85, 1–12.
| Reconciling waste rock rehabilitation goals and practice for a phosphate mine in a semi-arid environment.Crossref | GoogleScholarGoogle Scholar |
Golos PJ, Commander LE, Dixon KW (2019) The addition of mine waste rock to topsoil improves microsite potential and seedling emergence from broadcast seeds in an arid environment. Plant and Soil 440, 71–84.
| The addition of mine waste rock to topsoil improves microsite potential and seedling emergence from broadcast seeds in an arid environment.Crossref | GoogleScholarGoogle Scholar |
Grigg AH, Sheridan GJ, Pearce AB, Mulligan DR (2006) The effect of organic mulch amendments on the physical and chemical properties and revegetation success of a saline-sodic minespoil from central Queensland, Australia. Soil Research 44, 97–105.
| The effect of organic mulch amendments on the physical and chemical properties and revegetation success of a saline-sodic minespoil from central Queensland, Australia.Crossref | GoogleScholarGoogle Scholar |
Hardegree SP, Moffet CA, Flerchinger GN, Cho J, Roundy BA, Jones TA, James JJ, Clark PE, Pierson FB (2013) Hydrothermal assessment of temporal variability in seedbed microclimate. Rangeland Ecology & Management 66, 127–135.
| Hydrothermal assessment of temporal variability in seedbed microclimate.Crossref | GoogleScholarGoogle Scholar |
Hardegree SP, Sheley RL, James JJ, Reeves PA, Richards CM, Walters CT, Boyd CS, Moffet CA, Flerchinger GN (2020) Germination syndromes and their relevance to rangeland seeding strategies in the intermountain western United States. Rangeland Ecology & Management 73, 334–341.
| Germination syndromes and their relevance to rangeland seeding strategies in the intermountain western United States.Crossref | GoogleScholarGoogle Scholar |
Heneghan L, Miller SP, Baer S, Callaham MA, Montgomery J, Pavao-Zuckerman M, Rhoades CC, Richardson S (2008) Integrating soil ecological knowledge into restoration management. Restoration Ecology 16, 608–617.
| Integrating soil ecological knowledge into restoration management.Crossref | GoogleScholarGoogle Scholar |
James JJ, Svejcar TJ, Rinella MJ (2011) Demographic processes limiting seedling recruitment in arid grassland restoration. Journal of Applied Ecology 48, 961–969.
| Demographic processes limiting seedling recruitment in arid grassland restoration.Crossref | GoogleScholarGoogle Scholar |
James JJ, Sheley RL, Erickson T, Rollins KS, Taylor MH, Dixon KW (2013) A systems approach to restoring degraded drylands. Journal of Applied Ecology 50, 730–739.
| A systems approach to restoring degraded drylands.Crossref | GoogleScholarGoogle Scholar |
James JJ, Sheley RL, Leger EA, Adler PB, Hardegree SP, Gornish ES, Rinella MJ (2019) Increased soil temperature and decreased precipitation during early life stages constrain grass seedling recruitment in cold desert restoration. Journal of Applied Ecology 56, 2609–2619.
| Increased soil temperature and decreased precipitation during early life stages constrain grass seedling recruitment in cold desert restoration.Crossref | GoogleScholarGoogle Scholar |
Kildisheva OA (2019) Improving the outcomes of seed-based restoration in cold and hot deserts: an investigation into seed dormancy, germination, and seed enhancement. PhD Thesis, University of Western Australia.
Knutson KC, Pyke DA, Wirth TA, Arkle RS, Pilliod DS, Brooks ML, Chambers JC, Grace JB (2014) Long-term effects of seeding after wildfire on vegetation in Great Basin shrubland ecosystems. Journal of Applied Ecology 51, 1414–1424.
| Long-term effects of seeding after wildfire on vegetation in Great Basin shrubland ecosystems.Crossref | GoogleScholarGoogle Scholar |
Koch JM (2007) Restoring a Jarrah Forest understorey vegetation after bauxite mining in Western Australia. Restoration Ecology 15, S26–S39.
| Restoring a Jarrah Forest understorey vegetation after bauxite mining in Western Australia.Crossref | GoogleScholarGoogle Scholar |
Lamb D, Erskine PD, Fletcher A (2015) Widening gap between expectations and practice in Australian minesite rehabilitation. Ecological Management & Restoration 16, 186–195.
| Widening gap between expectations and practice in Australian minesite rehabilitation.Crossref | GoogleScholarGoogle Scholar |
Larson JE, Sheley RL, Hardegree SP, Doescher PS, James JJ (2015) Seed and seedling traits affecting critical life stage transitions and recruitment outcomes in dryland grasses. Journal of Applied Ecology 52, 199–209.
| Seed and seedling traits affecting critical life stage transitions and recruitment outcomes in dryland grasses.Crossref | GoogleScholarGoogle Scholar |
Larson JE, Anacker BL, Wanous S, Funk JL (2020) Ecological strategies begin at germination: traits, plasticity and survival in the first 4 days of plant life. Functional Ecology 34, 968–979.
| Ecological strategies begin at germination: traits, plasticity and survival in the first 4 days of plant life.Crossref | GoogleScholarGoogle Scholar |
Leger EA, Atwater DZ, James JJ (2019) Seed and seedling traits have strong impacts on establishment of a perennial bunchgrass in invaded semi-arid systems. Journal of Applied Ecology 56, 1343–1354.
| Seed and seedling traits have strong impacts on establishment of a perennial bunchgrass in invaded semi-arid systems.Crossref | GoogleScholarGoogle Scholar |
Lenth R (2022) emmeans: estimated marginal means, aka least-squares means. R package version 1.8.2. 2020. Available at https://CRAN.R-project.org/package=emmeans
Lewandrowski W, Erickson TE, Dixon KW, Stevens JC (2017) Increasing the germination envelope under water stress improves seedling emergence in two dominant grass species across different pulse rainfall events. Journal of Applied Ecology 54, 997–1007.
| Increasing the germination envelope under water stress improves seedling emergence in two dominant grass species across different pulse rainfall events.Crossref | GoogleScholarGoogle Scholar |
Lewandrowski W, Erickson TE, Dalziell EL, Stevens JC (2018) Ecological niche and bet-hedging strategies for Triodia (R.Br.) seed germination. Annals of Botany 121, 367–375.
| Ecological niche and bet-hedging strategies for Triodia (R.Br.) seed germination.Crossref | GoogleScholarGoogle Scholar |
Luna L, Vignozzi N, Miralles I, Solé-Benet A (2018) Organic amendments and mulches modify soil porosity and infiltration in semiarid mine soils. Land Degradation & Development 29, 1019–1030.
| Organic amendments and mulches modify soil porosity and infiltration in semiarid mine soils.Crossref | GoogleScholarGoogle Scholar |
Madsen MD, Davies KW, Williams CJ, Svejcar TJ (2012) Agglomerating seeds to enhance native seedling emergence and growth. Journal of Applied Ecology 49, 431–438.
| Agglomerating seeds to enhance native seedling emergence and growth.Crossref | GoogleScholarGoogle Scholar |
Masarei M, Astfalck LC, Guzzomi AL, Merritt DJ, Erickson TE (2020) Soil rock content influences the maximum seedling emergence depth of a dominant arid zone grass. Plant and Soil 450, 497–509.
| Soil rock content influences the maximum seedling emergence depth of a dominant arid zone grass.Crossref | GoogleScholarGoogle Scholar |
McKenzie NL, van Leeuwen S, Pinder AM (2009) Introduction to the Pilbara biodiversity survey, 2002–2007. Records of the Western Australian Museum, Supplement 78, 3–89.
| Introduction to the Pilbara biodiversity survey, 2002–2007.Crossref | GoogleScholarGoogle Scholar |
Merino-Martín L, Commander L, Mao Z, Stevens JC, Miller BP, Golos PJ, Mayence CE, Dixon K (2017a) Overcoming topsoil deficits in restoration of semiarid lands: designing hydrologically favourable soil covers for seedling emergence. Ecological Engineering 105, 102–117.
| Overcoming topsoil deficits in restoration of semiarid lands: designing hydrologically favourable soil covers for seedling emergence.Crossref | GoogleScholarGoogle Scholar |
Merino-Martín L, Courtauld C, Commander L, Turner S, Lewandrowski W, Stevens J (2017b) Interactions between seed functional traits and burial depth regulate germination and seedling emergence under water stress in species from semi-arid environments. Journal of Arid Environments 147, 25–33.
| Interactions between seed functional traits and burial depth regulate germination and seedling emergence under water stress in species from semi-arid environments.Crossref | GoogleScholarGoogle Scholar |
Michel BE (1983) Evaluation of the water potentials of solutions of polyethylene glycol 8000 both in the absence and presence of other solutes. Plant Physiology 72, 66–70.
| Evaluation of the water potentials of solutions of polyethylene glycol 8000 both in the absence and presence of other solutes.Crossref | GoogleScholarGoogle Scholar |
Muñoz-Rojas M (2018) Soil quality indicators: critical tools in ecosystem restoration. Current Opinion in Environmental Science & Health 5, 47–52.
| Soil quality indicators: critical tools in ecosystem restoration.Crossref | GoogleScholarGoogle Scholar |
Muñoz-Rojas M, Erickson TE, Dixon KW, Merritt DJ (2016a) Soil quality indicators to assess functionality of restored soils in degraded semiarid ecosystems. Restoration Ecology 24, S43–S52.
| Soil quality indicators to assess functionality of restored soils in degraded semiarid ecosystems.Crossref | GoogleScholarGoogle Scholar |
Muñoz-Rojas M, Erickson TE, Martini DC, Dixon KW, Merritt DJ (2016b) Climate and soil factors influencing seedling recruitment of plant species used for dryland restoration. Soil 2, 287–298.
| Climate and soil factors influencing seedling recruitment of plant species used for dryland restoration.Crossref | GoogleScholarGoogle Scholar |
Noy-Meir I (1973) Desert ecosystems: environment and producers. Annual Review of Ecology and Systematics 4, 25–51.
| Desert ecosystems: environment and producers.Crossref | GoogleScholarGoogle Scholar |
Parry ML, Bellairs SM, Lu P (2022) Improved native understorey establishment in mine waste rock in Australia’s wet–dry tropics. Australian Journal of Botany 70, 248–262.
| Improved native understorey establishment in mine waste rock in Australia’s wet–dry tropics.Crossref | GoogleScholarGoogle Scholar |
R Core Team (2020) ‘R: a language and environment for statistical computing.’ (R Foundation for Statistical Computing: Vienna, Austria) Available at http://www.R-project.org/
Rawlins JK, Roundy BA, Egget D, Cline N (2012) Predicting germination in semi-arid wildland seedbeds II. Field validation of wet thermal-time models. Environmental and Experimental Botany 76, 68–73.
| Predicting germination in semi-arid wildland seedbeds II. Field validation of wet thermal-time models.Crossref | GoogleScholarGoogle Scholar |
Ritz C, Streibig JC (2005) Bioassay analysis using R. Journal of Statistical Software 12, 1–22.
| Bioassay analysis using R.Crossref | GoogleScholarGoogle Scholar |
RStudio Team (2020) ‘RStudio: integrated development for R (Version 1.3.1093).’ (RStudio Inc.: Boston, USA) Available at http://www.R-project.org/
Shackelford N, Paterno GB, Winkler DE, et al. (2021) Drivers of seedling establishment success in dryland restoration efforts. Nature Ecology & Evolution 5, 1283–1290.
| Drivers of seedling establishment success in dryland restoration efforts.Crossref | GoogleScholarGoogle Scholar |
Spargo A, Doley D (2016) Selective coal mine overburden treatment with topsoil and compost to optimise pasture or native vegetation establishment. Journal of Environmental Management 182, 342–350.
| Selective coal mine overburden treatment with topsoil and compost to optimise pasture or native vegetation establishment.Crossref | GoogleScholarGoogle Scholar |
Stock E, Standish RJ, Muñoz-Rojas M, Bell RW, Erickson TE (2020) Field-deployed extruded seed pellets show promise for perennial grass establishment in arid zone mine rehabilitation. Frontiers in Ecology and Evolution 8, 576125
| Field-deployed extruded seed pellets show promise for perennial grass establishment in arid zone mine rehabilitation.Crossref | GoogleScholarGoogle Scholar |
Veblen KE, Nehring KC, Duniway MC, et al. (2022) Soil depth and precipitation moderate soil textural effects on seedling survival of a foundation shrub species. Restoration Ecology 30, e13700
| Soil depth and precipitation moderate soil textural effects on seedling survival of a foundation shrub species.Crossref | GoogleScholarGoogle Scholar |
Walck JL, Hidayati SN, Dixon KW, Thompson K, Poschlod P (2011) Climate change and plant regeneration from seed. Global Change Biology 17, 2145–2161.
| Climate change and plant regeneration from seed.Crossref | GoogleScholarGoogle Scholar |
Walkley A, Black IA (1934) An examination of the Degtjareff method for determining soil organic matter, and a proposed modification of the chromic acid titration method. Soil Science 37, 29–38.
| An examination of the Degtjareff method for determining soil organic matter, and a proposed modification of the chromic acid titration method.Crossref | GoogleScholarGoogle Scholar |
Western Australian Herbarium (1998–) FloraBase – the Western Australian Flora. Vol. 2022. (Department of Biodiversity, Conservation and Attractions) Available at http://florabase.dpaw.wa.gov.au/
Williams MI, Schuman GE, Hild AL, Vicklund LE (2002) Wyoming big sagebrush density: effects of seeding rates and grass competition. Restoration Ecology 10, 385–391.
| Wyoming big sagebrush density: effects of seeding rates and grass competition.Crossref | GoogleScholarGoogle Scholar |
Yang B, Balazs KR, Butterfield BJ, Laushman KM, Munson SM, Gornish ES, Barberán A (2022) Does restoration of plant diversity trigger concomitant soil microbiome changes in dryland ecosystems? Journal of Applied Ecology 59, 560–573.
| Does restoration of plant diversity trigger concomitant soil microbiome changes in dryland ecosystems?Crossref | GoogleScholarGoogle Scholar |