Impact of extreme heatwaves and life-history traits on seed germination responses in Cumberland Plain Woodland native plant species
Philippa R. Alvarez
A School of Life Sciences, University of Technology Sydney, PO Box 123, Ultimo, NSW 2007, Australia.
B Australian PlantBank, Botanic Gardens of Sydney, Mount Annan, NSW, Australia.
Australian Journal of Botany - https://doi.org/10.1071/BT22117
Submitted: 13 October 2022 Accepted: 14 June 2023 Published online: 11 July 2023
© 2023 The Author(s) (or their employer(s)). Published by CSIRO Publishing. This is an open access article distributed under the Creative Commons Attribution-NonCommercial-NoDerivatives 4.0 International License (CC BY-NC-ND)
Abstract
Context: The Cumberland Plain Woodland (CPW) is a threatened ecological community that is expected to experience heatwaves of increasing intensity and frequency into the future. Given the central role that temperature plays in regulating seed germination, understanding the impacts of such heatwaves on key germination attributes is vital to identify potential impacts on plant community structure.
Aims: To determine the impacts of increased heatwave intensity and frequency treatments on seed germination within and across 15 native species of the CPW. We also examined the influence of interspecific variation in life-history traits on germination responses.
Methods: Seeds were exposed to seven heatwave treatments of increasing intensity and frequency. Life-history trait data were also collected for each species’ dormancy type, fire response traits, life form and seed mass.
Key results: There was evidence within the study species of a significant effect of heatwave treatment. Germination duration was prolonged for the two most extreme simulated heatwave events. Life-history traits could not explain germination responses after heatwave treatments, however, larger seeded species had quicker germination onset and shorter periods of germination over small-seeded species; and trees had lower proportions of germination than any other life form.
Conclusions: Increases in heatwave intensity and frequency have an impact on seed germination responses in native species of the CPW. Life-history traits could not explain germination responses across species along the heatwave gradient.
Implications: Projected increases in heatwave intensity and frequency are likely to impact seed germination, and thus community composition, in species from the CPW.
Keywords: biodiversity, climate change, heatwaves, life-history traits, plant communities, seed ecology, seed germination, woodland ecology.
Introduction
Plant communities are expected to face potentially devastating impacts of future climate-driven heatwaves (Dale et al. 2001; Mondoni et al. 2012; Wolkovich et al. 2012; Parmesan and Hanley 2015; Cochrane 2017; Maher et al. 2019). The most recent projections submitted by the Intergovernmental Panel on Climate Change (IPCC) predict an 85% increase in the frequency of Australasian heatwaves accompanying a 1.5–2.0°C increase in global temperatures, which rises to a four-fold increase in heatwaves if a 3°C increase occurs in global temperatures (Lawrence et al. 2022). Based on these predicted climatic scenarios, understanding the potential impacts of extreme heatwaves on the beginning of a plant’s lifecycle at the seed germination stage is crucial for providing critical insight into the future of Australia’s plant biodiversity (Read et al. 1997; Hulme 1998; Tang et al. 2003; Cochrane 2020).
Plant seeds are highly reliant on suitable temperatures for successful germination (Bewley and Black 1994; Bewley 1997; Baskin and Baskin 1998). The germination niche of species is characterised by an optimum temperature range in which seeds are most likely to succeed in germination (Ooi et al. 2014; Ruiz-Talonia et al. 2018). Outside their optimum temperature range, seeds are less likely to germinate and are less successful at establishing during the seedling stage (Ooi et al. 2014; Hatfield and Prueger 2015; Cochrane 2017). Previous research has demonstrated, for instance, that when seeds are exposed to high intensity temperatures that exceed the optimal temperature for a species, the proportion of seeds that successfully germinate can be markedly reduced (Hoyle et al. 2013; Cochrane et al. 2014; Ruiz-Talonia et al. 2018).
Although exposure to high intensity temperatures exceeding a species’ optimum seed germination may not necessarily lead to long-term impacts on plant communities, the likelihood of seeds being exposed to multiple heatwave events may have more consistent negative impacts on seed germination and thus plant community structure and function (Ahrens et al. 2021). However, the impacts of multiple heatwave events on seed germination have not received much attention. In one study, Italian alpine seeds experienced an autumn and spring heatwave, which increased seed germination proportions in 30% of species tested after the spring heatwave and in 50% of species after the autumn heatwave out of 51 species (Orsenigo et al. 2015). In another study, seedlings were shown to be highly resilient to multiple heatwaves, with chlorophyll fluorescence remaining steady after heatwave exposure (French et al. 2019). With so few studies, more research is needed to elucidate the impacts of multiple heatwaves on Australia’s plant communities.
One underlying source of inherent variation in seed germination is variation in plant life-history traits among species (Keith et al. 2014; Jiménez-Alfaro et al. 2016). For example, key life-history traits including seed mass (Gross and Smith 1991; Moles and Westoby 2004; Kahmen and Poschlod 2008; Norden et al. 2009; Barak et al. 2018), seed dormancy (Ooi et al. 2007, 2014), fire response traits (Bradstock et al. 1997) and life form (Grime et al. 1981; Bu et al. 2008) all have the potential to affect the proportion of seeds that germinate, the timing of germination and the duration of time over which germination occurs. Therefore, any study that seeks to identify the impacts of intense and frequent heatwaves on seed germination among species needs to account for life-history variation.
Projected increases in temperatures associated with climate change may shift habitats outside the germination niche that seeds typically need for productive germination. For many native plant species, this will have important implications for threatened ecological communities (Hoyle et al. 2013; Orsenigo et al. 2015). As such, there is potential for enormous change in the composition of plant communities as a result of temperature-driven reductions in seed germination due to climate change (Van der Veken et al. 2004; Shi et al. 2015). One such plant community, and the focus of this study, is the Cumberland Plain Woodland (CPW) in NSW, Australia. For the CPW, the effects of intense and frequent heatwaves may prove devastating for seedling establishment and growth (Chapin et al. 2000; Walther et al. 2002; Hatfield and Prueger 2015). With only 6–8% of the original area untouched by urbanisation (Tozer et al. 2015), understanding how future temperature shifts may impact seed germination responses and therefore plant community composition is vital.
In the present study, we examined the effects of intense and frequent heatwaves on seed germination responses in 15 native plant species of the CPW using laboratory-based experiments. We focused on three seed germination attributes including onset of germination, the duration of germination and the proportion of seeds that germinate. Our experiments examined patterns of seed germination within and across the 15 species along a gradient of increasing heatwave intensity and frequency. This gradient of experimental treatments included a 5-day exposure period to (1) a lab-based control, (2) an average temperature, (3) a recent average heatwave, (4) a projected 4°C hotter heatwave based on IPCC projections of an average global increase in temperature, (5) a post-fire heatwave simulating no canopy cover and exposed ground, and then a series of (6) three heatwaves, and (7) five heatwaves at the post-fire temperature (Table 1). We also examined the roles of interspecific variation in seed mass, dormancy, fire response traits and life form on germination responses.
Heatwave type | Treatment | Temperature (°C) | Gradient |
---|---|---|---|
Control | A | 22 | Least extreme |
Recent average – BOM | B | 29/17 | ![]() |
Recent heatwave – BOM | C | 39/21 | |
Projected heatwave – BOM + IPCC | D | 43/25 | |
One post-fire heatwave | E | 60/22 | |
Three post-fire heatwave heatwaves | F | 60/22 | |
Five post-fire heatwave heatwaves | G | 60/22 | Most extreme |
Temperatures are shown as maximum/minimum, and were based on: Bureau of Meteorology (BOM) for the control and recent heatwave; BOM and the IPCC increase of 4°C for the projected heatwave; information from a prior study based on datalogger data from the CPW for the three post-fire heatwave treatments. An arrow shows the direction of the increasing intensity and frequency of the treatment temperatures across a gradient.
Materials and methods
Study region
The CPW is a threatened ecological community (Environmental Protection and Biodiversity Conservation Act 1999; NSW Government 2016) that lies within the Sydney Basin in south-eastern Australia on Hawkesbury Sandstone. It encompasses the First Nations lands of the Dharug, Eora, Dharawal and Kuring-gai communities. Extending from Parramatta in the east to the Blue Mountains in the west, and from the Hornsby Plateau in the north to the Woronora Plateau in the south, this ecological community consists of both urbanised land and remnant woodland. The woodlands and forests of the CPW are dominated by Eucalyptus canopy species, a shrubby understorey consisting typically of species from families including Fabaceae, Pittosporaceae and Asteraceae, and a rich diversity of species from the Poaceae in the grassy understorey (Benson and Howell 2002). Between 2006 and 2019, average minimum and maximum summer temperatures were 16.5°C and 29.2°C and the lowest and highest summer temperatures were 11.1°C and 38.2°C respectively (Bureau of Meteorology station no. 68257). Rainfall mostly occurs in the wetter summer/autumn months, with annual rainfall averaging around 700 mm throughout the CPW.
Study species and seed collection
The selection of species for the germination experiments was based on ensuring representation of a diversity of life-history traits in the CPW bioregion. The species that were used in the experiments captured the broad taxonomic range found in the study region, which included 15 species from 13 genera spread across eight families (Table 2). Seeds for these species were sourced from either fresh field collections or from a seedbank collection. Seedbank collections were previously stored for a maximum 10 years at 15% relative humidity (RH) at 4°C in sealed, airtight packages. Seeds of four species were collected from stands of CPW at the Australian Botanic Garden Mount Annan (ABG) between February and March 2018 and seeds of 11 species from the same area were obtained from the ABG PlantBank storage facilities (Table 2).
Family | Species | Collection | Pre-treatment | Seed mass (g) | Life form | Fire response | Dormancy |
---|---|---|---|---|---|---|---|
Asteraceae | Calotis lappulacea | Field | N/A | 0.46 | Shrub | Resprouter | Dormant |
Cassinia aculeata | PlantBank | N/A | 0.05 | Herb | Obligate seeder | Dormant | |
Chenopodiaceae | Einadia nutans subsp. nutans | Field | N/A | 0.68 | Herb | Obligate seeder | Non-dormant |
Fabaceae | Acacia decurrens | PlantBank | Scarification | 12.99 | Tree | Obligate seeder | Dormant |
Acacia falcata | PlantBank | Scarification | 14.09 | Shrub | Obligate seeder | Dormant | |
Hardenbergia violacea | PlantBank | Scarification | 17.15 | Climber | Resprouter | Dormant | |
Indigofera australis | PlantBank | Scarification | 3.71 | Shrub | Obligate seeder | Dormant | |
Lamiaceae | Plectranthus parviflorus | Field | N/A | 0.24 | Herb | Obligate seeder | Non-dormant |
Myrtaceae | Eucalyptus crebra | PlantBank | N/A | 0.15 | Tree | Resprouter | Non-dormant |
Eucalyptus tereticornis | PlantBank | N/A | 0.29 | Tree | Resprouter | Non-dormant | |
Poaceae | Dichanthium sericeum | PlantBank | Floret removed | 0.61 | Grass | Resprouter | Dormant |
Microlaena stipoides | PlantBank | Floret removed | 5.03 | Grass | Resprouter | Dormant | |
Themeda triandra | PlantBank | Floret removed | 3.77 | Grass | Resprouter | Dormant | |
Ranunculaceae | Clematis glycinoides var. glycinoides | PlantBank | N/A | 1.94 | Climber | Resprouter | Dormant |
Sapindaceae | Dodonaea viscosa subsp. cuneata | PlantBank | Scarification | 3.20 | Shrub | Resprouter | Dormant |
Collection refers to either field collection of seeds or PlantBank accessed seeds. Pre-treatment refers to any standard treatments used to promote seed germination or break physical dormancy. Seed mass is the weight of 1000 seeds (g). Life form: grass, herb, shrub, tree, or climber. Fire response: obligate seeder or resprouter. Dormancy: dormant or non-dormant.
Experimental design
All seeds were initially left to acclimatise to room temperature and humidity for approximately one fortnight. In an oven (Labec G701; Marrickville, Australia), dry seeds were exposed to a variety of heatwave scenarios for a period of 5 days, this length of time being representative of a typical heatwave in this region (Bureau of Meteorology 2020), at a prescribed temperature for each treatment (Table 1). These treatments were used to create a heatwave gradient of the least extreme heatwave scenario, which was 29/17°C (maximum/minimum) for a 5 day period, to the most extreme heatwave scenario which was 60/22°C for a 5 day period repeated five times with a 6 day gap between each heatwave (French et al. 2019). A lab-based control without a diel temperature cycle was also used (22°C). All seeds were subjected to these dry heatwave conditions at the same time, regardless of replicate.
To establish the range of temperatures for the treatments, we gathered meteorological data from the Bureau of Meteorology for the summers between 2013 and 2018 inclusive (Bureau of Meteorology 2020) for four locations spanning the distribution of the CPW. Diel temperature data were obtained for the four sites. After initial on-site testing with temperature loggers (Onsolution Thermochron DS1921G; Baulkham Hills, Australia), it was established that there was little to no difference between topsoil temperature and ambient air temperature, therefore ambient temperatures could be used rather than temperatures collected from within the top 5 cm of soil. The definition of a heatwave was based on the Bureau of Meteorology’s description of three or more days of high minimum and maximum temperatures that are ‘unusually high’ for that location (Bureau of Meteorology 2020). The four sites were located near the boundary of the CPW and positioned more than 17 km from each other. The sites included the weather stations Richmond RAAF (067105; northern boundary, −33°36′S, 150°47′E), Camden airport (068192; southern boundary, −34°2′S, 150°41′E), Bankstown airport (066137; eastern boundary, −33°55′S, 150°59′E) and Penrith Lakes AWS (067113; western boundary, −33°43′S, 150°41′E). The projected heatwave is based on IPCC projections of a 4°C increase in global temperature by the end of the century (Pörtner et al. 2022). To establish the temperature needed for the post-fire heatwave treatment, a data logger was placed on recently burnt ground in the CPW woodland with no canopy cover present (von Richter, unpublished data).
Following each treatment, and once species had been pre-treated using appropriate germination requirements (such as physical scarification or removal of external coverings, Table 2), 10 seeds were placed on 9 cm petri dishes, each dish containing 8 g/L of agar. The control, recent heatwave and projected heatwave groups had five replicates of 10 seeds; one post-fire heatwave, three post-fire heatwaves and five post-fire heatwaves had six replicates of 10 seeds. After each treatment, dishes were placed into species-specific optimum temperatures for germination of each species (based on initial trials and information provided by the Society for Ecological Restoration International Network for Seed Based Restoration and Royal Botanic Gardens Kew (2023). This study aimed to assess the effect of a range of heatwave treatments on post-heatwave seed germination responses and so used optimum germination temperature to identify the impact of these extreme events. As such, some species were placed into incubators with diel temperature cycles (see Supplementary materials Table S1), others were placed in constant temperatures (20°C). The light settings for all incubators were set to a diel cycle with a 12 h/12 h light/dark rotation.
Germination checks commenced 2 days after seeds were placed into the incubators. No germination occurred before placement in the incubators. Each group was checked every second day for the first 14 days. From day 14 to day 30, the plates were checked every 4 days, and then weekly until no further germination was observed for 14 days in a row. Germination was defined as emergence of a radicle of more than 2 mm. After this time, a cut test was used to reveal the condition of those seeds that had not germinated (Ooi et al. 2004). Germinability was assessed to be the most ecologically relevant measure of seed germination success, not viability. In this study, germination total is represented by those seeds that germinated out of the total number of seeds with the potential to germinate, i.e. viable seeds, not seeds that were empty or destroyed by predation.
Collection of trait information for each species
Table 2 and Fig. 1 present summary trait information for each species. Seed mass was measured as the weight of 1000 seeds for each species using an analytical balance (Mettler Toledo AG204; Mumbai, India). Seeds were left to equilibrate at room temperature and humidity, and all dispersal structures were removed before weighing. Species were classified as dormant (species that require a germination stimulant if no germination occurs within 6 weeks under suitable conditions) or non-dormant (species that readily germinate within 6 weeks under suitable conditions) based on observations within the study region (noting that dormancy can vary within a species across geographic ranges; Baskin and Baskin 2004). As some dormancy classes had only one or two representatives among the species tested, the split between dormant and non-dormant was determined to be suitable for this study. Dormancy types of species were obtained from Chapter 7 in Hirst et al. 2021. Each species was classified as either an obligate seeder (species that die when exposed to fire and rely on the seedbank for regeneration) or a resprouter (species that regenerate after fire through epicormic shoots or lignotubers) based on observations within the study region (noting that fire response traits can also vary within a species across geographic ranges; Gill 1981; Gill and Bradstock 1992). Fire response trait data were obtained from fire ecology research performed in the Cumberland Plain Woodland (CPW) (Watson 2005; Penman et al. 2008; Kubiak 2009). Information on species’ life forms was sourced from PlantNET (The NSW Plant Information Network System) (2019), Tozer et al. (2015), Watson (2005) and (Fairley and Moore 2010). Each species was classified as either a tree (a single-stemmed woody plant >5 m), shrub (a multi-stemmed woody plant <5 m), herb a non-woody plant <2 m), climber (a small multi-branched woody plant <1 m) or grass (species in Poaceae).
Statistical analyses
First, to determine the effects of the heatwave treatment levels on germination responses within each species, we fitted generalised linear models (GLMs) to each species individually for onset of germination, germination duration (GLMs with Poisson error structure), and germination proportion (GLM with binomial error structure), using petri dishes as replicates and a single term for heat treatment (fixed categorical factor with seven categories). Following this, to quantify the size of the effects of the heat treatments across all species considered together, we fitted generalised linear mixed effects models (GLMMs) to onset of germination and germination duration (Poisson error structure), and a linear mixed effect for germination proportion (logit transformed). Here, to account for the multiple observations of species within levels of the heat treatment, we included a random term for species, nested within plant family. This approach allowed us to broadly account for phylogeny while using models that allow inclusion of multiple observations per species (Smith and Brown 2018). Finally, to test if plant life-history traits explained patterns in germination responses, separate mixed effects models for each plant life-history trait were fitted including a term for the heat treatment and the life-history trait of interest (seed mass [ln transformed], life form, dormancy and fire response traits). For the categorical life-history traits, random effects for plant species were nested within the life-history term of interest, which was in turn nested within plant family, and species nested within family for seed mass. This structure of random terms allowed us to control for species level effects within factor levels, and to account for any effects of plant family over and above those of species and life-history factors. We employed Poisson GLMMs for onset and duration of germination, and a linear mixed model for germination proportion (logit transformed). All analyses were performed in R 4.2.1 (R Core Team 2022) with the packages ape (Paradis and Schliep 2019), car (Fox et al. 2019), V.PhyloMaker (Jin and Qian 2019), emmeans (Lenth et al. 2021), and lme4 (Bates et al. 2015).
Results
Germination responses within species to the heatwave treatments
The majority of species showed significant differences in onset of germination among heat treatment levels (12 species; Fig. 2b), seven species in germination duration (Fig. 2c) and 10 species in germination proportion (Fig. 2d; full model outputs in Supplementary Table S2). Species that showed a significant heat treatment effect generally showed delayed germination onset in the more extreme heat treatments (e.g. Eucalyptus tereticornis and E. crebra, Acacia decurrens). Other species showed differing effects, such as Cassinia aculeata (with a pattern of decrease in time to germination across the treatments), Calotis lappulacea and Einadia nutans (with no directional trend related to the treatments emerging), or Clematis glycinoides, which showed a slowing in treatments C to E, and similar delays in treatments A and B compared to F to H. For germination duration, two species tended towards longer durations across the treatments, E. tereticornis and C. lappulacea, with the other species showing no directional trend, e.g. C. aculeata and Dodonaea viscosa. Germination proportion for A. decurrens, Hardenbergia violacea and Microlaena stipoides tended towards a decrease across the heat treatments, whereas other species again showed significant patterns with no clear directionality, e.g. Plectranthus parviflorus or D. viscosa. In contrast, C. aculeata appears to show an upward trend.
Plot of the phylogeny for the study species (a), onset of germination (b), germination duration (c) and germination proportion (d). Species values correspond to the tips of the phylogeny in plots (b–d). Values for the heat treatments A–G for each species are displayed from the top to bottom for each species and coloured blue to red. Where a significant effect of the heat treatment emerged for a given species this is indicated by a red asterisk on the right margin of the plot. Black lines on points indicate the 95% confidence interval (CI) for the means.
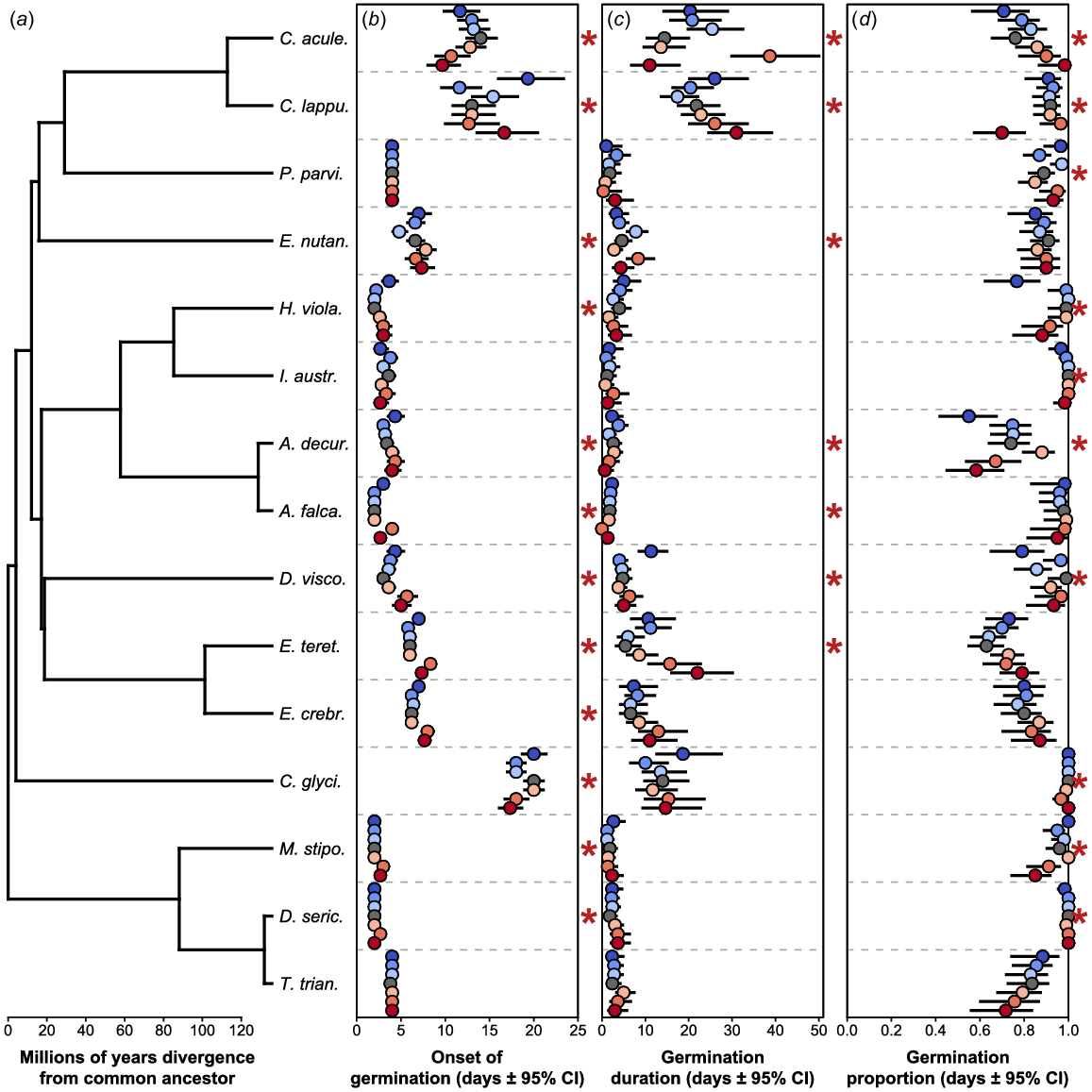
Germination responses among species to the heatwave treatments and relationship with life-history traits
Across species, no significant effect of heat treatment emerged for onset of germination (χ2 = 11.780, d.f. = 6, P = 0.07; Fig. 3a), whereas germination duration was significantly shorter in treatments B to E compared to A, F and G, with treatment F showing significantly longer duration relative to treatment A and G (χ2 = 142.850, d.f. = 6, P < 0.0001; Fig. 3b. For germination proportion, a significant effect of heat treatment was found (χ2 = 12.935, d.f. = 6, P = 0.0.44; Fig. 3c), Treatments F and G had lower germination proportions than the other treatments, but these were not shown to be non-significant after correction for multiple comparisons. Of the life-history traits, seed mass emerged as significant for onset of germination (χ2 = 7.067, d.f. = 1, P = 0.008; Fig. 4a), and also for germination duration (χ2 = 7.067, d.f. = 1, P = 0.008; Fig. 4b), with heavier seeds germinating faster over a shorter period. For germination proportion, only life form was significantly related to germination proportion (χ2 = 17.201, d.f. = 4, P = 0.002; Fig. 4c), with a higher proportion of climbers’ seeds germinating relative to tree species, with grasses, herbs and shrubs not differing significantly from either climbers or trees. In these models of the heat treatment including life-history traits, germination duration and germination proportion remained significant (all P-values < 0.05; Supplementary Table S3), indicating none of the life-history traits were sufficient to explain the patterns related to the heat treatment.
Plots of the heat treatments’ effects for onset of germination (a), germination duration (b) and germination proportion (c). Points are species means within the levels of the heat treatment. Black lines are means of treatments across species and shaded areas the 95% confidence intervals (CI) of the means. In (b) and (c), the red letters indicate the pattern of significant differences among the treatment levels from pairwise testing. For (c), no significant pairwise differences emerged after correction for multiple comparisons, so the patterns without correction are shown (denoted by the asterisks next to letters).
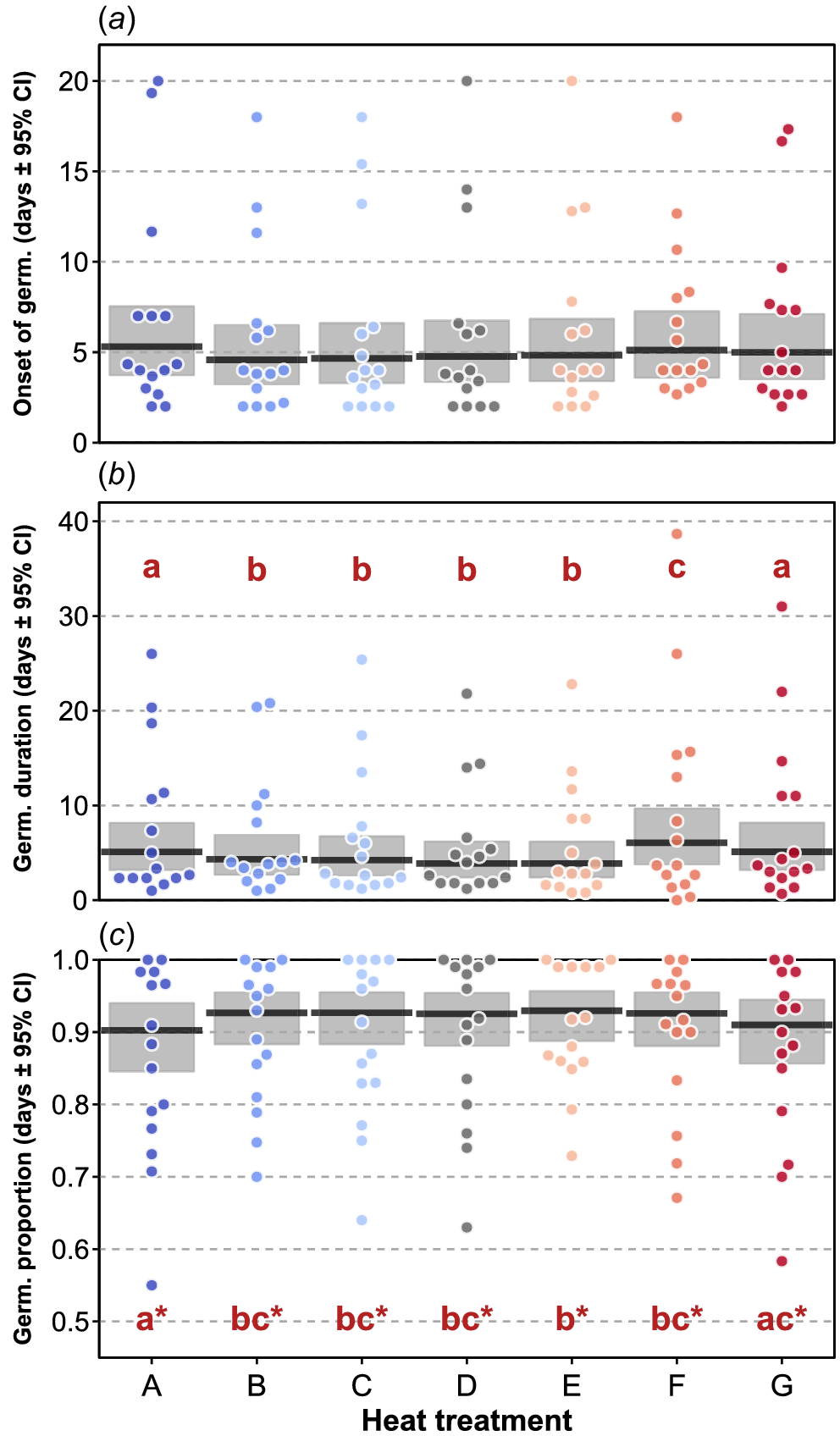
Plot of onset of germination (a) and germination duration (b) against ln seed mass. Points are the mean values for species across levels of the heat treatments. The red line is the line of best fit from the GLMMs (representing the average across factor combinations in the models). In (c) the germination proportions for plant life forms are shown. Black lines are means of treatments across species and shaded areas the 95% CI of the means. Red letters in (c) indicate the pattern of significant differences among factor levels.
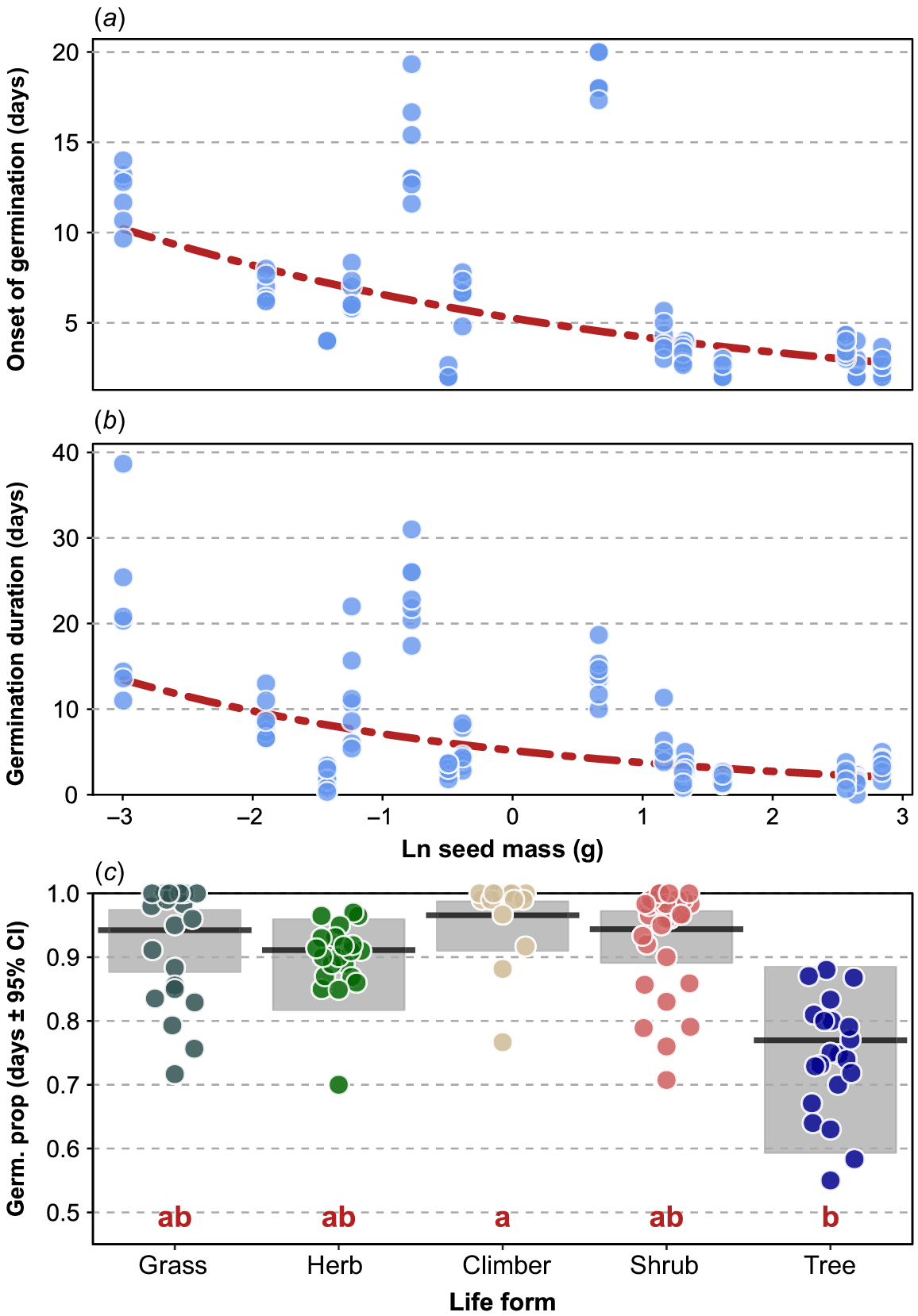
Discussion
The germination attributes of our study species were significantly affected by increases in the intensity and frequency of heatwaves. Most species responded to the increase with delayed germination onset, prolonged germination duration time, and a reduced proportion of germinated seeds. These broad findings support previous studies exploring the impacts of high temperatures on seed germination (Ellis et al. 1987; Baskin and Baskin 1988; Roberts 1998; Clarke and French 2005; Davies et al. 2018). The direction of influence of heatwaves on species attributes was variable, but the general trend was for longer germination duration and lower total germination proportion. However, the influence of heatwaves on germination onset was variable with no consistent directional trend. Interestingly, for germination duration the most prolonged germination period was found after three heatwaves and not after the expected five heatwaves. This may be a result of the drop in total proportion of germination with those species that experienced five heatwaves dying before any duration changes were observed. Treatment A, the control, had constant temperature exposure, which may have lowered the total germination proportion for species that require a diel temperature cycle for optimal germination, a well-known phenomenon from early studies (Thompson et al. 1977; Probert 2000).
In this study, the influences of key life-history traits on seed germination saw the emergence of underlying mechanisms governing seed germination attributes, though they did not explain the patterns associated with heatwave stress. There was also no apparent association between phylogenetic relationships among species and the germination responses post-heatwave exposure. Rather, life-history traits were the key drivers. Seed mass was significantly related to germination onset and duration. Heavier seeds were quicker to germinate than smaller seeds and had shorter germination periods. These results were similar to a few studies in the literature (Moles and Westoby 2004, 2006), but opposed other studies (Verdú and Traveset 2005; Norden et al. 2009; Barak et al. 2018). In terms of bet-hedging strategy, generally speaking, small-seeded species have greater long-term advantages over their lifespan that would outweigh the benefits gained by large-seeded species, which only seem to benefit in short-term quick germination onset (Moles and Westoby 2004). Our results show that although large-seeded species may have the initial advantages over small-seeded species with quicker germination, this may not be a useful strategy in the long term.
For total proportion of germination, the only significant life-history trait was life form. Climbers had the highest proportion of germination, trees had the lowest proportion, and everything else was in between. Although bet-hedging strategies provide some answers (Pianka 1970; Reznick et al. 2002; Olofsson et al. 2009), the seed mass of the trees in this experiment varied considerably, from some species with very small seeds to other species with comparatively large seeds. In future, this may mean a reduction in species from certain life form groups such as trees and an increase in more hardy life form types such as grasses. This would mean a fairly dramatic shift in species composition should the number of intense and frequent heatwaves increase over the next few decades and centuries, as they are expected to.
As for the other life traits, we expected to find an observable difference within the fire response traits, between the obligate seeders and resprouters. Throughout the literature, obligate seeders are recognised as more prolific germinators (Hansen et al. 1991; Bell et al. 1993; Hunter 2003) as they dedicate more resources to seeds than resprouters (Knox and Morrison 2005; Palmer et al. 2018). We also expected delayed germination onset for dormant vs non-dormant seeds. It has been argued that dormant seeds are more likely to survive higher temperatures than non-dormant seeds due to the physiological differences between dormant and non-dormant seeds (Ramos et al. 2016).
One other aspect of this study to consider is that dry seeds are naturally more adapted to survive than imbibed seeds (Woodstock 1988). Had the seeds in this experiment been imbibed, we known that the proportion of seed to germinate would have been greatly reduced (Tangney et al. 2019). Once imbibition occurs, the metabolic function within seeds begins and the effect of temperature becomes far more apparent in these germination attributes (Weitbrecht et al. 2011). Had the seeds in our study been at a resting 95% relative humidity, not quite at the point of imbibition, we may have seen contrasting results (Bewley and Black 2013).
Conclusion
Our study has identified the impact of intense and frequent heatwaves on the seed germination responses of species from the Cumberland Plain Woodland and the underlying influence of life-history traits on seed germination. Most affected species had delayed germination onset, prolonged germination duration time, and a reduced proportion of germinated seeds after exposure to more extreme heatwave conditions. We also recognised the importance of life-history traits like seed mass and life form on seed germination responses. These results provide much needed insight into the future prospects of threatened ecological communities such as the CPW.
Acknowledgements
The authors would like to thank the PlantBank facility at the Australian Institute of Botanical Sciences for use of their laboratory facilities and seed collections. Special thanks to Lotte von Richter for her continued help and advice regarding equipment and protocols over the course of this study. We thank Graeme Errington for his knowledge pertaining to the seed collection and for allowing us to conduct experiments on the seeds he and his team collected. The science team at PlantBank kindly provided on-going advice and tips throughout this research.
References
Ahrens CW, Challis A, Byrne M, Leigh A, Nicotra AB, Tissue D, Rymer P (2021) Repeated extreme heatwaves result in higher leaf thermal tolerances and greater safety margins. New Phytologist 232, 1212-1225.
| Crossref | Google Scholar |
Barak RS, Lichtenberger TM, Wellman-Houde A, Kramer AT, Larkin DJ (2018) Cracking the case: seed traits and phylogeny predict time to germination in prairie restoration species. Ecology and Evolution 8, 5551-5562.
| Crossref | Google Scholar |
Baskin CC, Baskin JM (1988) Germination ecophysiology of herbaceous plant species in a temperate region. American Journal of Botany 75, 286-305.
| Crossref | Google Scholar |
Baskin JM, Baskin CC (2004) A classification system for seed dormancy. Seed Science Research 14, 1-16.
| Crossref | Google Scholar |
Bates D, Mächler M, Bolker B, Walker S (2015) Fitting linear mixed-effects models using lme4. Journal of Statistical Software 67, 1-48.
| Crossref | Google Scholar |
Bell DT, Plummer JA, Taylor SK (1993) Seed germination ecology in southwestern Western Australia. The Botanical Review 59, 24-73.
| Crossref | Google Scholar |
Benson DH, Howell J (2002) Cumberland Plain Woodland ecology then and now: interpretations and implications from the work of Robert Brown and others. Cunninghamia 7, 631-650.
| Google Scholar |
Bewley JD (1997) Seed germination and dormancy. The Plant Cell 9, 1055-1066.
| Crossref | Google Scholar |
Bradstock RA, Tozer MG, Keith DA (1997) Effects of high frequency fire on floristic composition and abundance in a fire-prone heathland near Sydney. Australian Journal of Botany 45, 641-655.
| Crossref | Google Scholar |
Bu H, Du G, Chen X, Xu X, Liu K, Wen S (2008) Community-wide germination strategies in an alpine meadow on the eastern Qinghai-Tibet plateau: phylogenetic and life-history correlates. Plant Ecology 195, 87-98.
| Crossref | Google Scholar |
Bureau of Meteorology (2020) Understanding heatwaves. (Australian Government, Bureau of Meteorology) Available at http://www.bom.gov.au/australia/heatwave/knowledgecentre/understanding.shtml [Accessed 16 June 2020]
Chapin FS, III, Zavaleta ES, Eviner VT, Naylor RL, Vitousek PM, Reynolds HL, Hooper DU, Lavorel S, Sala OE, Hobbie SE, Mack MC, Díaz S (2000) Consequences of changing biodiversity. Nature 405, 234-242.
| Crossref | Google Scholar |
Clarke S, French K (2005) Germination response to heat and smoke of 22 Poaceae species from grassy woodlands. Australian Journal of Botany 53, 445-454.
| Crossref | Google Scholar |
Cochrane A (2017) Are we underestimating the impact of rising summer temperatures on dormancy loss in hard-seeded species? Australian Journal of Botany 65, 248-256.
| Crossref | Google Scholar |
Cochrane JA (2020) Thermal requirements underpinning germination allude to risk of species decline from climate warming. Plants 9, 796.
| Crossref | Google Scholar |
Cochrane A, Hoyle GL, Yates CJ, Wood J, Nicotra AB (2014) Predicting the impact of increasing temperatures on seed germination among populations of Western Australian Banksia (Proteaceae). Seed Science Research 24, 195-205.
| Crossref | Google Scholar |
Dale VH, Joyce LA, McNulty S, Neilson RP, Ayres MP, Flannigan MD, Hanson PJ, Irland LC, Lugo AE, Peterson CJ, Simberloff D, Swanson FJ, Stocks BJ, Wotton BM (2001) Climate change and forest disturbances: climate change can affect forests by altering the frequency, intensity, duration, and timing of fire, drought, introduced species, insect and pathogen outbreaks, hurricanes, windstorms, ice storms, or landslides. BioScience 51, 723-734.
| Crossref | Google Scholar |
Davies M, Ecroyd H, Robinson SA, French K (2018) Stress in native grasses under ecologically relevant heat waves. PLoS ONE 13, e0204906.
| Crossref | Google Scholar |
Ellis RH, Hong TD, Roberts EH (1987) Comparison of cumulative germination and rate of germination of dormant and aged barley seed lots at different constant temperatures. Seed Science and Technology 15, 717-727.
| Google Scholar |
Fox J, Weisberg S, Adler D, Bates D, Baud-Bovy G, Ellison S, Firth D, Friendly M, Gorjanc G, Graves S, Heiberger R (2019) Package ‘car’. p. 16. R Foundation for Statistical Computing, Vienna. Available at https://cran.r-project.org/web/packages/car/index.html
French K, Jansens IB, Ashcroft MB, Ecroyd H, Robinson SA (2019) High tolerance of repeated heatwaves in Australian native plants. Austral Ecology 44, 597-608.
| Crossref | Google Scholar |
Gill AM, Bradstock RA (1992) A national register for the fire responses of plant species. Cunninghamia 2, 653-660.
| Google Scholar |
Grime JP, Mason G, Curtis AV, Rodman J, Band SR (1981) A comparative study of germination characteristics in a local flora. The Journal of Ecology 69, 1017-1059.
| Crossref | Google Scholar |
Gross KL, Smith AD (1991) Seed mass and emergence time effects on performance of Panicum dichotomiflorum Michx. across environments. Oecologia 87, 270-278.
| Crossref | Google Scholar |
Hansen A, Pate JS, Hansen AP (1991) Growth and reproductive performance of a seeder and a resprouter species of Bossiaea as a function of plant age after fire. Annals of Botany 67, 497-509.
| Crossref | Google Scholar |
Hatfield JL, Prueger JH (2015) Temperature extremes: effect on plant growth and development. Weather and Climate Extremes 10, 4-10.
| Crossref | Google Scholar |
Hirst MJ, Commander LE, Emery NJ, Guja LK, Liyanage GS, Merritt DJ, Norton SL, Stevens A, Turner SR, Wood JA (2021) Chapter 7: Seed germination and dormancy. In ‘Plant germplasm conservation in Australia: strategies and guidelines for developing, managing and utilising ex situ collections’. 3rd edn. (Eds AJ Martyn Yenson, CA Offord, PF Meagher, T Auld, D Bush, DJ Coates, LE Commander, LK Guja, SL Norton, RO Makinson, R Stanley, N Walsh, D Wrigley, L Broadhurst) pp. 187–240. (Australian Network for Plant Conservation: Canberra, ACT)
Hoyle GL, Venn SE, Steadman KJ, Good RB, McAuliffe EJ, Williams ER, Nicotra AB (2013) Soil warming increases plant species richness but decreases germination from the alpine soil seed bank. Global Change Biology 19, 1549-1561.
| Crossref | Google Scholar |
Hulme PE (1998) Post-dispersal seed predation and seed bank persistence. Seed Science Research 8, 513-519.
| Crossref | Google Scholar |
Hunter JT (2003) Persistence on inselbergs: the role of obligate seeders and resprouters. Journal of Biogeography 30, 497-510.
| Crossref | Google Scholar |
Jiménez-Alfaro B, Silveira FAO, Fidelis A, Poschlod P, Commander LE (2016) Seed germination traits can contribute better to plant community ecology. Journal of Vegetation Science 27, 637-645.
| Crossref | Google Scholar |
Jin Y, Qian H (2019) V.PhyloMaker: an R package that can generate very large phylogenies for vascular plants. Ecography 42, 1353-1359.
| Crossref | Google Scholar |
Kahmen S, Poschlod P (2008) Does germination success differ with respect to seed mass and germination season? Experimental testing of plant functional trait responses to grassland management. Annals of Botany 101, 541-548.
| Crossref | Google Scholar |
Keith DA, Elith J, Simpson CC (2014) Predicting distribution changes of a mire ecosystem under future climates. Diversity and Distributions 20, 440-454.
| Crossref | Google Scholar |
Knox KJE, Morrison DA (2005) Effects of inter-fire intervals on the reproductive output of resprouters and obligate seeders in the Proteaceae. Austral Ecology 30, 407-413.
| Crossref | Google Scholar |
Kubiak PJ (2009) Fire responses of bushland plants after the January 1994 wildfires in northern Sydney. Cunninghamia 11, 131-165.
| Google Scholar |
Lawrence J, Mackey B, Chiew F, Costello MJ, Lansbury N, Hennessy K, Nidumolu U, Pecl G, Rickards L, Tapper N, Woodward A, Wreford A (2022) Chapter 11: Australasia. In ‘Working Group II: Impacts, adaptation and vulnerability, sixth assessment report’. (Eds H-O Pottner, D Roberts) pp. 1–151. (Intergovernmental Panel on Climate Change: Geneva, Switzerland)
Lenth R, Singmann H, Love J, Buerkner P, Herve M (2021) emmeans: estimated marginal means, aka least-squares means. R Package Version 1.7.0. Available at https://CRAN.R-project.org/package=emmeans
Maher T, Mirzaei M, Pascovici D, Wright IJ, Haynes PA, Gallagher RV (2019) Evidence from the proteome for local adaptation to extreme heat in a widespread tree species. Functional Ecology 33, 436-446.
| Crossref | Google Scholar |
Moles AT, Westoby M (2004) Seedling survival and seed size: a synthesis of the literature. Journal of Ecology 92, 372-383.
| Crossref | Google Scholar |
Moles AT, Westoby M (2006) Seed size and plant strategy across the whole life cycle. Oikos 113, 91-105.
| Crossref | Google Scholar |
Mondoni A, Rossi G, Orsenigo S, Probert RJ (2012) Climate warming could shift the timing of seed germination in alpine plants. Annals of Botany 110, 155-164.
| Crossref | Google Scholar |
Norden N, Daws MI, Antoine C, Gonzalez MA, Garwood NC, Chave J (2009) The relationship between seed mass and mean time to germination for 1037 tree species across five tropical forests. Functional Ecology 23, 203-210.
| Crossref | Google Scholar |
Olofsson H, Ripa J, Jonzén N (2009) Bet-hedging as an evolutionary game: the trade-off between egg size and number. Proceedings of the Royal Society B: Biological Sciences 276, 2963-2969.
| Google Scholar |
Ooi M, Auld T, Whelan R (2004) Comparison of the cut and tetrazolium tests for assessing seed viability: a study using Australian native Leucopogon species. Ecological Management & Restoration 5, 141-143.
| Crossref | Google Scholar |
Ooi MKJ, Auld TD, Whelan RJ (2007) Distinguishing between persistence and dormancy in soil seed banks of three shrub species from fire-prone southeastern Australia. Journal of Vegetation Science 18, 405-412.
| Crossref | Google Scholar |
Ooi MKJ, Denham AJ, Santana VM, Auld TD (2014) Temperature thresholds of physically dormant seeds and plant functional response to fire: variation among species and relative impact of climate change. Ecology and Evolution 4, 656-671.
| Crossref | Google Scholar |
Orsenigo S, Abeli T, Rossi G, Bonasoni P, Pasquaretta C, Gandini M, Mondoni A (2015) Effects of autumn and spring heat waves on seed germination of high mountain plants. PLoS ONE 10, e0133626.
| Crossref | Google Scholar |
Palmer HD, Denham AJ, Ooi MKJ (2018) Fire severity drives variation in post-fire recruitment and residual seed bank size of Acacia species. Plant Ecology 219, 527-537.
| Crossref | Google Scholar |
Paradis E, Schliep K (2019) ape 5.0: an environment for modern phylogenetics and evolutionary analyses in R. Bioinformatics 35, 526-528.
| Crossref | Google Scholar |
Parmesan C, Hanley ME (2015) Plants and climate change: complexities and surprises. Annals of Botany 116, 849-864.
| Crossref | Google Scholar |
Penman TD, Binns DL, Allen RM, Shiels RJ, Plummer SH (2008) Germination responses of a dry sclerophyll forest soil-stored seedbank to fire related cues. Cunninghamia 10, 547-555.
| Google Scholar |
Pianka ER (1970) On r- and K-selection. The American Naturalist 104, 592-597.
| Crossref | Google Scholar |
PlantNET (The NSW Plant Information Network System) (2019) Royal Botanic Gardens and Domain Trust, Sydney. Available at http://plantnet.rbgsyd.nsw.gov.au [Accessed 11 November 2019]
Ramos DM, Liaffa ABS, Diniz P, Munhoz CBR, Ooi MKJ, Borghetti F, Valls JFM (2016) Seed tolerance to heating is better predicted by seed dormancy than by habitat type in Neotropical savanna grasses. International Journal of Wildland Fire 25, 1273-1280.
| Crossref | Google Scholar |
R Core Team (2022) ‘R: a language and environment for statistical computing.’ (R Foundation for Statistical Computing: Vienna, Austria) Available at https://www.R-project.org/ [Accessed 12 August 2022]
Reznick D, Bryant MJ, Bashey F (2002) r- and k- selection revisited: the role of population regulation in life-history evolution. Ecology 83, 1509-1520.
| Crossref | Google Scholar |
Ruiz-Talonia L, Carr D, Smith R, Whalley RDB, Reid N (2018) Effect of temperature and light on germination of 10 species of Eucalyptus from north-western NSW. Australian Journal of Botany 66, 657-666.
| Crossref | Google Scholar |
Shi Z, Sherry R, Xu X, Hararuk O, Souza L, Jiang L, Xia J, Liang J, Luo Y (2015) Evidence for long-term shift in plant community composition under decadal experimental warming. Journal of Ecology 103, 1131-1140.
| Crossref | Google Scholar |
Smith SA, Brown JW (2018) Constructing a broadly inclusive seed plant phylogeny. American Journal of Botany 105, 302-314.
| Crossref | Google Scholar |
Society for Ecological Restoration, International Network for Seed Based Restoration and Royal Botanic Gardens Kew (2023) Seed Information Database (SID). Available at https://ser-sid.org/ [Accessed 20 February 2023]
Tang Y, Boulter SL, Kitching RL (2003) Heat and smoke effects on the germination of seeds from soil seed banks across forest edges between subtropical rainforest and eucalypt forest at Lamington National Park, south-eastern Queensland, Australia. Australian Journal of Botany 51, 227-237.
| Crossref | Google Scholar |
Tangney R, Merritt DJ, Fontaine JB, Miller BP (2019) Seed moisture content as a primary trait regulating the lethal temperature thresholds of seeds. Journal of Ecology 107, 1093-1105.
| Crossref | Google Scholar |
Thompson K, Grime JP, Mason G (1977) Seed germination in response to diurnal fluctuations of temperature. Nature 267, 147-149.
| Crossref | Google Scholar |
Tozer MG, Leishman MR, Auld TD (2015) Ecosystem risk assessment for Cumberland Plain Woodland, New South Wales, Australia. Austral Ecology 40, 400-410.
| Crossref | Google Scholar |
Van Der Veken S, Bossuyt B, Hermy M (2004) Climate gradients explain changes in plant community composition of the forest understory: an extrapolation after climate warming. Belgian Journal of Botany 137, 55-69.
| Google Scholar |
Verdú M, Traveset A (2005) Early emergence enhances plant fitness: a phylogenetically controlled meta-analysis. Ecology 86, 1385-1394.
| Crossref | Google Scholar |
Walther G-R, Post E, Convey P, Menzel A, Parmesan C, Beebee TJC, Fromentin J-M, Hoegh-Guldberg O, Bairlein F (2002) Ecological responses to recent climate change. Nature 416, 389-395.
| Crossref | Google Scholar |
Weitbrecht K, Müller K, Leubner-Metzger G (2011) First off the mark: early seed germination. Journal of Experimental Botany 62, 3289-3309.
| Crossref | Google Scholar |
Wolkovich EM, Cook BI, Allen JM, Crimmins TM, Betancourt JL, Travers SE, Pau S, Regetz J, Davies TJ, Kraft NJB, Ault TR, Bolmgren K, Mazer SJ, McCabe GJ, McGill BJ, Parmesan C, Salamin N, Schwartz MD, Cleland EE (2012) Warming experiments underpredict plant phenological responses to climate change. Nature 485, 494-497.
| Crossref | Google Scholar |
Woodstock LW (1988) Seed imbibition: a critical period for successful germination. Journal of Seed Technology 12, 1-15.
| Google Scholar |