Probiotics effects on the growth performance and meat quality of broiler chickens
Nazerke Begdildayeva

A
B
C
D
E
F
G
Abstract
In the limited field of research on Kazakhstan’s poultry, this study explores a new area by investigating the lack of studies on the impact of probiotics from camel milk and mare’s milk. This study provides valuable knowledge about an important aspect of poultry production in the region.
The purpose of the study was to investigate the effect of probiotics isolated from traditional fermented dairy products, fermented camel milk (shubat) and fermented mare’s milk (koumiss) on the growth performance and meat quality of broiler chickens.
In total, 240 1-day-old Ross 308 chicks were randomly separated into four treatment groups of three replicates (20 birds per replicate). Three experimental groups were fed by basal diet plus drinking water containing 0.25 mL, 0.5 mL, and 1.0 mL of probiotics per bird (Pro1, Pro2, and Pro3 respectively). The control group was fed by basal diet plus drinking water. The carcass characteristics included the weights of the body and carcass rate. Organoleptic analysis, proximate composition, pH, drip loss, cook loss and were used to determine meat-quality properties.
According to the study results isolated probiotics Lacticaseibacillus paracasei subsp. paracasei SH1, Enterococcus faecalis SH6, and Kazachstania unispora Y 2.2 had a significant effect on body and carcass weight (P < 0.05). Average daily gain and feed conversion ratio were also improved in probiotics groups (P < 0.05). Additionally, Pro 2 group had increased meat protein contents (P < 0.05). No significant differences in organoleptic quality between the control and experimental groups fed with the probiotics were determined.
Traditional fermented dairy products could become a source of new effective probiotics useful to improve broiler production.
The readily available shubat and koumiss in Kazakhstan present a promising opportunity for establishing localised probiotic production for poultry, indicating a viable path for promoting sustainable and tailored approaches to poultry farming in the region.
Keywords: broiler chickens, fermented dairy products, growth performance, koumiss, meat quality, poultry, probiotics, shubat.
Introduction
Poultry production is expected to see a significant increase in the coming decades, with global production of poultry meat projected to rise by over 181 million megagrams by 2050 (Tavaniello et al. 2018). Moreover, the proposed ascent of Kazakhstan to the 58th position as a global contributor to poultry meat production by 2026 is supported by available data, which suggest a consistent annual growth rate of 1.8% and a previous increase of 1.6% per year since 2017 (‘Kazakhstan Poultry Meat Industry Outlook 2022–2026’). The increase in demand for poultry in industrialised nations can be attributed to its healthy image, affordability, and valuable processed options (Petracci et al. 2009). In response to the ban on antibiotic growth promoters in many countries, the poultry industry has been searching for alternative solutions to support the health and growth of broiler chickens (Abou-Kassem et al. 2021). One potential solution is the use of probiotic microorganisms that can provide health benefits when consumed in appropriate amounts (FAO 2001).
Probiotics have been investigated as diet additives (Fallah et al. 2013), or alternatives to antibiotics for improving production (Al-Khalaifa et al. 2019), and ultimately improving the quality of chicken meat (Mohammed et al. 2021). The most common microorganisms used as probiotics in animal husbandry are lactic acid bacteria (LAB) and yeasts. Representatives of the genera Lactobacillus, Streptococcus, Pediococcus, Enterococcus, and Weissella are most commonly used in poultry farming (Begdildayeva et al. 2022). They boost animal health and productivity without leaving any lingering odour or taste in the final product (Patterson and Burkholder 2003). Yeast (Saccharomyces cerevisiae) and yeast-derived products have been shown to have several positive effects on broiler chickens, including increased growth performance (Gao et al. 2008), moderation of gut pH (Afsharmanesh et al. 2010), and modulation of gut microbes (Ahiwe et al. 2021). In addition to potential benefits for animal health, the use of probiotics may affect the meat quality. Kim et al. (2016) reported that dietary probiotic supplements could improve chicken meat quality attributes, such as water-holding capacity (WHC), tenderness, sensory properties, and microbial safety, whereas Zhou et al. (2010) did not find a synergistic effect of probiotics on chicken meat quality. However, a recent study by Jeni et al. (2021) suggested that these subtle and limited effects may not include all of the criteria desired for optimal health or be detectable in standard production metrics such as bodyweight gain, feed conversion ratio (FCR), or meat quality.
According to the literature, raw camel milk and its fermented products could be a potential source of beneficial probiotic strains (Konuspayeva et al. 2023). Furthermore, camel milk and shubat microflora possess a wide range of therapeutic and antimicrobial properties (Rehman et al. 2020). Additional research has also found that probiotics isolated from koumiss demonstrate impressive probiotic characteristics (Zhou et al. 2010; Wang et al. 2013), cholesterol-lowering potentials (Guo et al. 2015), and antioxidant properties (Hou et al. 2019).
Studies investigating the effect of probiotics isolated from camel milk and mare milk on poultry are extremely rare. Furthermore, the availability of shubat and koumiss in Kazakhstan could be a good opportunity to organise manufacture of local probiotic preparation for poultry in the future. The purpose of this research was to investigate the effect of dietary probiotic supplementation on the performance, pH, drip loss, cook loss, composition, and sensory characteristics of chicken meat.
Materials and methods
Potential probiotics
Probiotic strains were obtained from the culture bank of LLP Research and Production Enterprise ‘Antigen’ (Almaty, Kazakhstan), which were stored at −80°C. Lactic acid bacteria (LAB) L. paracasei SH1 (GenBank Accession no. OQ411023) and E. faecalis SH6 (Genbank Accession no. OQ415517.1) were used in this study as the potential probiotic microorganisms isolated from fermented camel milk (shubat) and K. unispora Y 2.2 (Genbank Accession no. OP984721) isolated from fermented mare’s milk (koumiss). Strains were isolated from milk by using serial dilution and plating on selective media (Mithun et al. 2015): De Man Rogosa and Sharpe (MRS) media (TM Media, Titan Biotech, India) for LAB and Sabouraud media (TM Media, Titan Biotech, India) for yeasts. The strains were cultivated in a culture-media incubator at 30°C (for yeast) and 37°C (for LAB). The cells were harvested by centrifugation (3000g) for 15 min at 4°C after incubation, washed three times with phosphate-buffered saline (pH 7.2), and resuspended in the same buffer until use.
Experimental design
The present study was conducted at the accredited testing centre and Department of Microbiology, LLP Research and Production Enterprise ‘Antigen’ (Almaty, Kazakhstan). The experimental procedures were conducted by the guidelines of the Department of Microbiology, LLP ‘Research and Production Enterprise ‘Antigen’.
Animal material and diets
In total, 240 1-day-old Ross 308 chicks were randomly allotted to four treatment groups of three replicates (20 birds per replicate). The control groups were fed a basal diet (Table 1) without any probiotic and the three treatment groups were fed diets consisting of L. paracasei SH1, E. faecalis SH6 and K. unispora Y 2.2 at initial concentrations of 1.0 × 109 colony-forming units (CFU)/mL, 5.0 × 108 CFU/mL, and 2.5 × 108 CFU/mL per bird (Groups Pro1, Pro2, and Pro3 respectively), for 42 days. The strains were gradually introduced to the water, mixing in portions in a water container, to produce these final concentrations. To determine and modify the number of probiotic bacteria in each water container, agar-plate count was performed every half an hour until the water ran out.
Item | Starter | Grower | Finisher | |
---|---|---|---|---|
0–14 days | 15–28 days | 29–42 days | ||
Ingredient (g/kg) | ||||
Soybean meal | 32.5 | 28.20 | 25.33 | |
Soybean oil | 2.10 | 1.33 | 1.80 | |
Wheat | 26.73 | 29.19 | 30.66 | |
Plant oil | – | 2.00 | 2.50 | |
Salt | 0.30 | 0.30 | 0.28 | |
Maize | 30.00 | 30.00 | 30.00 | |
Mel stern | 1.09 | 0.95 | 0.85 | |
Canola seeds | 5.00 | 6.00 | 7.00 | |
Vitamin–mineral premix A | 0.50 | 0.50 | 0.50 | |
Nutrient composition | ||||
ME (kcal/kg) | 2975 | 3050 | 3100 | |
Crude protein (%) B | 23.00 | 21.50 | 19.50 | |
Lysine (%) B | 1.32 | 1.18 | 1.08 | |
Methionine (%) B | 0.55 | 0.51 | 0.48 | |
Threonine (%) B | 0.88 | 0.79 | 0.72 | |
Calcium (%) B | 0.95 | 0.75 | 0.65 | |
Available P (%) B | 0.50 | 0.42 | 0.36 |
Management
During the experiment, all groups were kept in suitable conditions. Each replicate is housed in a separate battery cage (200 × 50 × 90 cm). Broiler chicks were kept on continuous light until the end of the first 7 days of life, after which they received 22 h of light per day. The room temperature was kept at 33°C for the initial 3 days and then adjusted to a range of 32–30°C from Day 4 to Day 7. Subsequently, it was gradually decreased by 23°C per week until it stabilised within the range of 22–24°C. The brooder battery came with two white fluorescent lamps. All of the birds were kept in the same sanitary, environmental, and management conditions. Feed was provided ad libitum, and freshwater was available at all times during the experiment. Drinkers and feeding troughs were cleaned daily.
Growth performance
To determine the bodyweight, the birds were individually weighed with an accuracy of 0.1 g at 1, 21, and 42 days of age. The feed intake of the birds, based on the pen they were in, was measured at 1, 21, and 42 days of the experiment. These measurements were used to calculate the average daily gain (ADG) and FCR. Throughout the experiment, the mortality rate of the Ross 308 broiler chicks was recorded daily, and it was calculated for each specific period of the study, namely, grower period (1–21 days), finisher period (22–42 days), and overall period (1–42 days).
Carcass yield
At the end of the experiment, randomly 13 chickens from each group were selected for slaughter to investigate the carcass characteristics. The liveweight (g) was recorded as the weight of each bird after undergoing the fasting process before slaughter at the designated facility. Following the slaughter, the feathers, head, feet, and giblets were removed, and the carcass weight (g) was measured, enabling the calculation of the carcass rate (%).
Proximate composition
Moisture content was determined by using the air oven-drying method of the AOAC (Official Method 990.19, AOAC 2016). The measurement was performed by drying the samples in a Binder ED-115 Oven (Binder, Germany) at 105°C overnight. The dish was then cooled in a desiccator and weighed and the process was repeated until constant weight was reached.
Kjeldahl method was used to determine protein content on the basis of the standard procedure of the AOAC (Official Method 973.48, AOAC 2016). This method is performed with an automated Kjeltec instrument UDK 142 (VELP, Italy) to determine protein content in meat samples.
Fat content was determined by the Soxhlet extraction method, by using the AOAC Official Method 960.39 (AOAC 2016). Instead, fat content was measured by weight loss of the sample or by the weight of the fat removed.
The ash content of the samples was determined using the dry-ashing method of the AOAC (Official Method 999.11, AOAC 2016). Ashing of the meat sample (10 g) was performed in a SNOL 8,2/1100 LSM (AB ‘Umega’, Lithuania) muffle furnace at 550°C. Before ashing, the crucible was dried in an oven at 105°C for 3 h.
The amino acid content of the meat was determined by chromatography method of the AOAC (Official Method 982.30, AOAC 2016).
Sensory characteristics
Meat samples were subjected to boiling in water until their internal temperature reached 70°C for assessment. The temperature verification was conducted through the placement of a thermometer within the central geometric region of the meat specimen. A sensory panel of 10 experienced evaluators investigated the sensory qualities after the slaughter. The evaluation was conducted utilising a nine-point rating scale to gauge various quality aspects, including colour (ranging from 1 (very light/pale) to 9 (reddish-black)), flavour (ranging from 1 (very poor) to 9 (excellent)), tenderness (ranging from 1 (tough) to 9 (very soft/tender)), taste (ranging from 1 (low) to 9 (high)), and juiciness (ranging from 1 (very dry) to 9 (succulent)) (Standards of Kazakhstan 2014).
pH measurement
The pH of all broiler breast muscles was measured in triplicate by using an insertion-type pH meter (Seven Compact pH/Ion meter S220-Std-Kit) 24 and 192 h postmortem after slaughter.
Drip-loss measurement
Drip loss was measured in breast fillets, beginning 24 and 192 h after slaughter. The breast filets were hung on hooks through their thickest part. To reduce evaporation, the filets were packed in plastic bags and sealed with rubber bands, with the hooks extending out of the bags. The filets in bags were then hung from a grate for 24 h in a 4°C incubator. The samples were weighed before and after hanging. Drip loss (DL) was calculated as the difference in weight, corrected for mass, and expressed as a percentage:
where DL is drip loss (%), m1 is mass before hanging, and m2 is mass after hanging (Albrecht et al. 2017).
Cook-loss measurement
For cook-loss measurements, about 80 g of fillet sample was vacuum-packed and cooked at 80°C to a core temperature of 75°C. During cooking, the core temperature was recorded using a portable needle-tipped thermometer (GT-309, Giltron, New Taipei, Taiwan). The samples were then equilibrated to room temperature and re-weighed. Cook loss (CL) was determined by calculating the weight loss during cooking as a percentage of the weight before cooking and was expressed as follows (Lee et al. 2022):
where CL is cook loss (%), m1 is mass final weight, and m2 is mass initial weight.
Microbiological analyses
Microbiological analysis was performed 24 and 192 h after slaughter to determine the total number of viable cells (TVC) as well as the amount of Pseudomonas spp. (Pse), a specific microorganism that causes spoilage of fresh, in aerobically packed poultry. For the microbiological analysis, 25 g of surface meat tissue measuring 4 × 7 × 0.5 cm was taken aseptically with a sterile scalpel. The sample was transferred to a sterile filtered gastric bag and filled with 225 mL of physiological solution–peptone diluent (0.85% NaCl with 0.1% peptone). The samples were stirred for 60 s and 10-fold dilutions of the homogenate were prepared in the saline–peptone diluent. TVC was determined using a pour-plate method on a countable amount of agar in the plate. The plates were incubated at 30°C for 72 h. Pseudomonas spp. (Pse) was identified by plating on Pseudomonas agar plates with a cetrimide–fucidin–cephalosporin selective additive. The plates were incubated at 25°C for 48 h.
Statistical analyses
The statistical analysis of all the data was conducted using the one-way ANOVA and principal-component analysis (PCA, using Pearson (n–1)) procedures provided by the XLSTAT-Student, ver. 2023 (Lumivero, USA, https://www.xlstat.com/). Subsequently, the Fisher’s test was applied to identify significant (P < 0.05) differences whenever they were observed. The determination of significance was based on a threshold of P < 0.05. The data are presented as the means ± standard errors of the mean (s.e.m.).
Ethics approval and consent to participate
The study adhered to the principles of good laboratory practice outlined in the State Standard of the Republic of Kazakhstan, along with the main provisions of ST RK 1613-2017. Ethical approval for the study was granted by the Local Committee on Animal Research Ethics of the LLP Research and Production Enterprise ‘Antigen’ under Protocol number 39, dated 28 August 2022, in Almaty, Kazakhstan.
Results
The potential effects of probiotics on broiler chicken growth at different phases are demonstrated in Table 2. Between Days 1 and 21, Pro3 group showed a significant (P < 0.01) improvement in their ADG. Additionally, on the 21st day of the experiment, Group Pro3 exhibited an average bodyweight significantly (P < 0.01) different from that of the other treatment groups. However, from Day 21 to Day 42, the difference in ADG among the groups was not statistically significant (P > 0.05). During the entire trial period (Days 1–42) both Pro2 and Pro3 groups had a considerably higher (P < 0.05) increase in ADG and a significantly (P < 0.01) lower FCR than did the control group. Furthermore, in comparison to the control group, the Pro2 and Pro3 groups had significantly higher final bodyweights at the end of the experiment (P < 0.01) higher final bodyweights at the end of the experiment.
Item | Dietary treatment | P-value | s.e.m. | ||||
---|---|---|---|---|---|---|---|
Control | Pro1 | Pro2 | Pro3 | ||||
1–21 days | |||||||
Day-1 BW (g) | 44.6 | 45.4 | 44.7 | 44.7 | 0.31 | 0.48 | |
ADG (g/bird) | 38.54b | 39.46b | 37.76b | 41.69a | <0.01 | 0.43 | |
FCR (g/g) | 1.32 | 1.24 | 1.69 | 1.30 | 0.86 | 0.03 | |
Day-21 BW (g) | 856.23b | 874.15b | 839.15b | 922.15a | <0.01 | 9.02 | |
22–42 days | |||||||
ADG (g/bird) | 75.69 | 77.61 | 82.54 | 79.85 | 0.08 | 1.00 | |
FCR (g/g) | 1.18 | 1.15 | 1.01 | 1.10 | 0.09 | 0.02 | |
Day-42 BW (g) | 2445.46a | 2505.08ab | 2573.85a | 2597.92a | <0.01 | 18.79 | |
1–42 days | |||||||
ADG (g/bird) | 57.15b | 58.53ab | 60.31a | 60.85a | <0.01 | 0.45 | |
FCR (g/g) | 1.60a | 1.45ab | 1.40b | 1.52b | <0.01 | 0.01 |
Control: basal diet; Pro1, Pro2 and Pro3 groups, basal diet with addition of 0.25, 0.5, and 1.0 mL of probiotics respectively.
s.e.m., standard error of the mean (n = 13); ADG, average daily weight gain; FCR, feed conversion ratio; BW, bodyweight.
Carcass weight and carcass rate of broilers treated with varied amounts of potential probiotics are shown in Table 3. All groups fed probiotics showed a significant increase in carcass weight (P < 0.01), whereas carcass rate did not differ among treatments (P > 0.05).
Trait | Treatment | P-value | s.e.m. | ||||
---|---|---|---|---|---|---|---|
Control | Pro1 | Pro2 | Pro3 | ||||
Carcass weight (g) | 2096b | 2221a | 2242a | 2241.25a | <0.01 | 17.50 | |
Carcass rate (%) | 85.77 | 88.69 | 87.12 | 86.24 | 0.06 | 0.42 |
Control: basal diet; Pro1, Pro2 and Pro3 groups, basal diet with addition of 0.25, 0.5, and 1.0 mL of probiotics respectively.
s.e.m., standard error of the mean (n = 13).
The protein content, fat content, ash, and moisture content of chicken meat are presented in Fig. 1. The protein content in chicken meat displayed significant (P < 0.03) differences between the Pro2 treatment and the control group (Fig. 1a). The chicken meat from Pro2 group demonstrated a lower fat content, on average, than did that from both the control group and the other treatment groups (Fig. 1c), but the difference was not significant (P > 0.05). There were no substantial variations observed in moisture and ash contents between the control group and the experimental groups (Fig. 1b, d).
Variations in (a) protein, (b) moisture, (c) fat, and (d) ash contents in broiler meat between the control and experimental groups (Pro1, Pro2, Pro3).
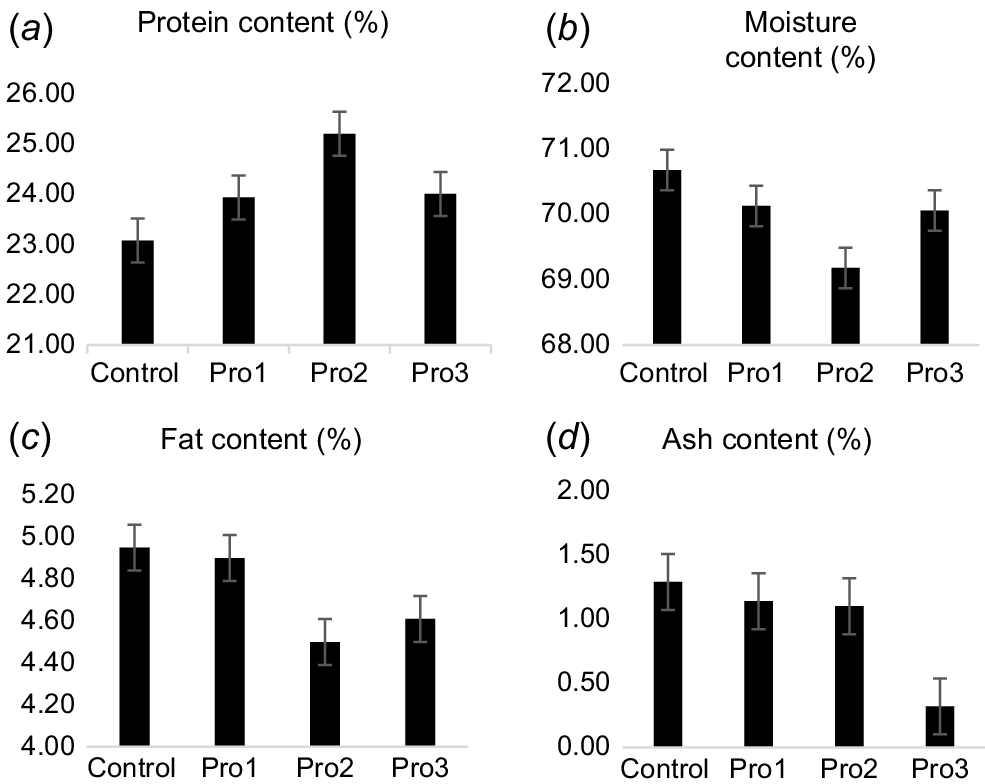
The amino acid composition of broiler meat is shown in Table 4. The method used allowed an accurate measurement of eight essential amino acids and four non-essential amino acids. The most abundant amino acids in broiler meat were arginine, followed by lysine, alanine, leucine/isoleucine, valine and threonine. Among the essential amino acids, there were no significant differences among treatments for threonine, methionine, phenylalanine, and histidine (P > 0.05). In contrast, compared to the control, valine showed a substantial rise in Pro1, Pro2, and Pro3 treatments (P < 0.01). Additionally, experimental groups had significantly (P < 0.009) greater leucine/isoleucine contents than did the control group. Lysine and arginine did not show significant (P > 0.05 for both) differences among treatments. Non-essential amino acids serine, glycine, and tyrosine showed no significant (P > 0.05) differences across treatments. In contrast, alanine contents in Pro1, Pro2, and Pro3 groups were significantly (P < 0.004) higher than those in the control group.
Trait (%) | Dietary treatment | P-value | s.e.m. | ||||
---|---|---|---|---|---|---|---|
Control | Pro1 | Pro2 | Pro3 | ||||
Total amino acids | 12.57 | 16.14 | 18.14 | 13.31 | |||
Essential amino acids (EAA) | |||||||
Threonine | 0.95 | 1.10 | 1.20 | 0.87 | 0.16 | 0.06 | |
Methionine | 0.70 | 0.80 | 0.89 | 0.69 | 0.27 | 0.04 | |
Valine | 0.96c | 1.48ab | 1.58a | 1.14bc | <0.01 | 0.08 | |
Phenylalanine | 0.81 | 0.99 | 1.06 | 0.94 | 0.06 | 0.03 | |
Histidine | 0.72 | 0.74 | 0.82 | 0.62 | 0.6 | 0.05 | |
Leucine/isoL | 1.14c | 1.63ab | 1.83a | 1.38bc | <0.009 | 0.08 | |
Lysine | 1.75 | 2.32 | 2.59 | 1.87 | 0.06 | 0.13 | |
Arginine | 1.58b | 2.32b | 3.19a | 2.16b | <0.003 | 0.16 | |
Non-essential amino acids (NEAA) | |||||||
Serine | 0.92 | 1.04 | 1.08 | 0.99 | 0.56 | 0.04 | |
Alanine | 1.33bc | 1.73ab | 1.81a | 1.11b | <0.004 | 0.08 | |
Glycine | 0.92 | 1.10 | 1.18 | 0.90 | 0.18 | 0.05 | |
Tyrosine | 0.80 | 0.89 | 0.9 | 0.70 | 0.1 | 0.04 |
Control: basal diet; Pro1, Pro2 and Pro3 groups, basal diet with addition of 0.25, 0.5, and 1.0 mL of probiotics respectively.
s.e.m., standard error of the mean (n = 13).
The main factors of the PCA explained 33% of the variance (Fig. 2). The results showed that better separation is achieved due to the group’s proximity to the centre of the PCA with Factors 1 and 2. An increase in amino acid concentrations was observed when probiotics were administered at 0.25 mL and 0.5 mL per bird. However, when the probiotic dose per bird increased up to 1 mL, amino acid amount decreased in the analysed samples.
PCA analysis of amino acid composition in broiler meat of control and experimental groups (Pro1, Pro2, Pro3).
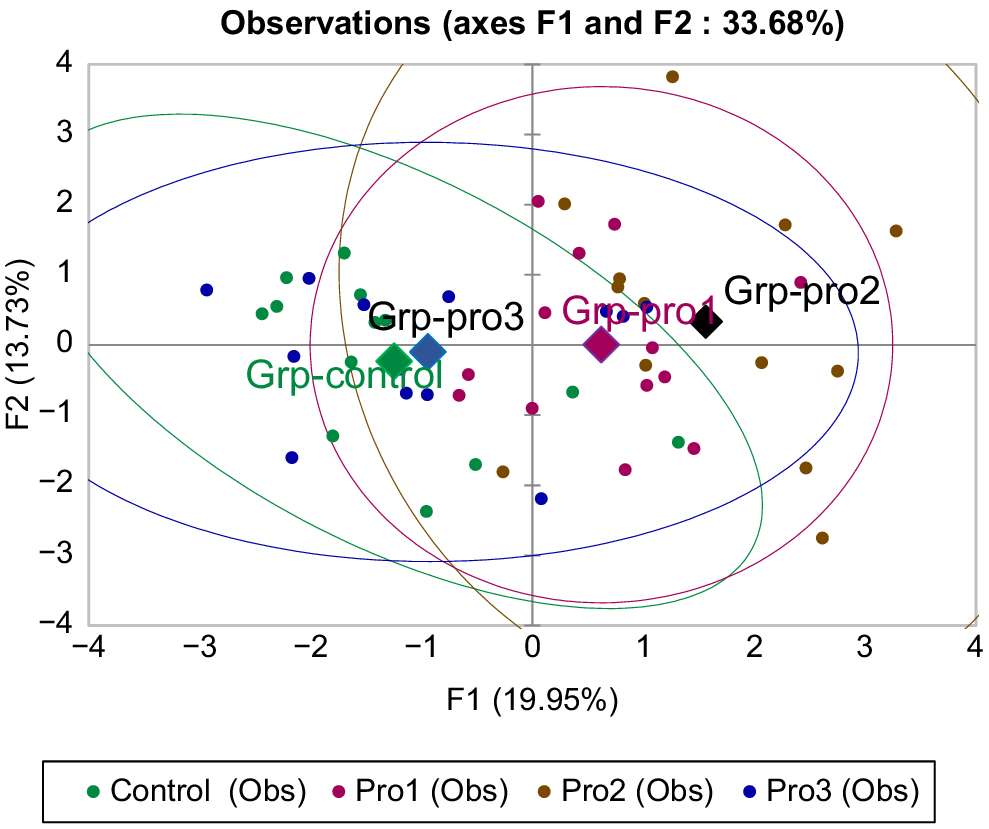
The effects of probiotic supplements on pH, DL, heating loss, TVC, and Pse of chicken meat at 24 and 192 h after slaughter are showed in Table 5. There were no significant differences in drip-loss, and cook-loss values between the groups after 1 day (P > 0.05). The meat pH after 24 h was substantially higher in the control group (P < 0.03) than in the probiotics groups. The Pro2 group had the greatest DL after 192 h among the groups. But the difference was not significant (P > 0.05). The average TVC of all samples 24 h after slaughtering was 6.55–6.85 log CFU/g, whereas the mean initial bacterial count of Pseudomonas spp. was 1.35–1.67 log CFU/g. At the start of storage, there was a significant difference in Pseudomonas counts in treatment groups, indicating that all fillets had equivalent initial contamination by microbes (P < 0.004). After 192 h, the mean TVC of the supplemented groups and the control group ranged between 6.14 and 6.49 log CFU/g, with the supplemented groups having higher TVC (P < 0.005). At the last stage of storage (after 192 h), the mean counts for Pseudomonas spp. varied between 5.39 and 6.28 log CFU/g. The count of Pseudomonas spp. was substantially different in the Pro2 group after 192 h (P < 0.005) than the counts in the control and other experimental groups.
Item | Dietary treatment | P-value | s.e.m. | ||||
---|---|---|---|---|---|---|---|
Control | Pro1 | Pro2 | Pro3 | ||||
pH24 | 6.56a | 6.18b | 6.17b | 6.22b | <0.03 | 0.06 | |
pH192 | 5.73 | 5.76 | 5.81 | 5.56 | 0.59 | 0.06 | |
Drip loss24 | 0.7 | 0.79 | 0.84 | 0.85 | 0.35 | 0.03 | |
Drip loss192 | 0.9b | 0.81b | 1.29a | 0.93b | <0.005 | 0.06 | |
Cook loss24 | 21.08 | 21.47 | 20.69 | 22.05 | 0.06 | 0.20 | |
Cook loss192 | 23.17 | 23.01 | 21.74 | 22.56 | 0.10 | 0.23 | |
TVC24 | 6.2b | 6.14b | 6.49a | 6.31a | <0.004 | 0.04 | |
TVC192 | 6.85a | 6.83a | 6.55c | 6.69b | <0.005 | 0.04 | |
Pse24 | 1.6a | 1.61a | 1.35b | 1.42b | <0.005 | 0.04 | |
Pse192 | 5.77c | 6.39a | 5.43d | 6.28b | <0.005 | 0.12 |
Means within the same row with no common letter differ significantly (P < 0.05).
Control: basal diet; Pro1, Pro2 and Pro3 groups, basal diet with addition of 0.25, 0.5, and 1.0 mL of probiotics respectively.
s.e.m., standard error of the mean (n = 3); TVC, total number of viable cells; Pse, Pseudomonas spp.
The comparison of meat attributes, including colour, smell, taste, tenderness, and juiciness, among the control group and three groups supplemented with probiotics is illustrated in Fig. 3. No significant differences were detected among the treatments for the remaining sensory characteristics (P > 0.05), indicating that the aroma strength and texture of chicken meat might not be influenced by the effects of L. paracasei SH1, E. faecalis SH6, and K. unispora Y 2.2.
Discussion
Fermented milk products may be viewed as more beneficial probiotics by poultry producers (Ghasemi-Sadabadi et al. 2019; Sjofjan et al. 2021). A meta-analysis highlights the improved performance observed in broilers after supplementation with probiotics, notably LAB and yeasts (Sjofjan et al. 2021). Furthermore, probiotics have the potential to improve the quality of broiler meat in a variety of ways, including pH, colour, water-holding capacity, chemical composition, fatty acid profile, and oxidative stability (Popova 2017).
Growth performance and carcass rate
Rahmani Alizadeh et al. (2023) showed that probiotics isolated from camel milk had a good effect on broiler growth rate and FCR. The bodyweights of Ross 308 chicks on Days 21 and 42 were comparable to the findings of Martínez and Valdivié (2021). The literature data on the effect of probiotics on the bodyweight and carcass parameters yield inconsistent results. Kalavathy et al. (2003) discovered that probiotic-fed chicks acquired considerably more weight from Day 22 to Day 42, although there was no difference between groups for the first 21 days. Rehman et al. (2020) observed considerable weight growth during the first 21 days, but no significant difference over the entire trial period (0–35 days). They also found that probiotics did not influence carcass properties. In line with our findings, Liu et al. (2017) found that the experimental group receiving 1 mL probiotics achieved a statistically significant improvement in daily weight gain at 4 and 6 weeks of age. However, Lee et al. (2010) found that dietary probiotics had little or no influence on broiler chicken growth performance. These discrepancies in outcomes were caused by probiotic strains, administration dosage, preparation methods, bird age, meat composition, and hygiene state (Zhang et al. 2012).
Proximate composition
Zhou et al. (2010) reported that the application of probiotics had no discernible impact on the levels of moisture, protein, crude fat, and crude ash in the musculature of chicken breast and thigh meat. In contrast, Aziz et al. (2020) found a noteworthy augmentation in the protein content within the breast and thigh meat of broiler chickens. An independent investigation undertaken by Abdulla et al. (2017) unveiled a significant reduction in the fat composition of broiler chicken breast muscles when a probiotic formulation containing Bacillus subtilis was used. The substantial reduction in adipose tissue content in poultry meat contributes positively to the nutritional value of the meat, aligning with the preferences of a majority of global consumers. Conversely, an increased fat content augments the sensory attributes encompassing meat taste and tenderness, as emphasised by Chartrin et al. (2006). In another study, Ivanovic (2012) showed a marked increase in moisture content, coupled with a notable decline in fat and protein constituents, in the drumstick meat harvested from 42-day-old Arbor Acres broilers fed diverse probiotics, including Lactiplantibacillus plantarum, E. faecium, Bacillus cereus IP5832, or a combination of Bacillus CH200 and Bacillus CH201. Similar results were obtained by Sharipova et al. (2017), who also observed a decrease in moisture content when the animals were fed with probiotics, which was explained by probiotics stimulating protein synthesis that results in a decrease in moisture content as a percentage of the overall weight. Consistent with our findings, the use of probiotics led to an increase in the crude protein content of the meat. High moisture content indicates low quality of meat (Freitas et al. 2018). Consequently, probiotics, by lowering the moisture content, can improve meat quality.
Meat amino acid composition
The quality and flavour of broiler chicken meat are greatly influenced by the amino acid profile of the meat. Whereas specific amino acids that contribute to flavour, such as alanine, glycine, glutamine, and serine, particularly glutamine, significantly influence the overall flavour of meat, essential amino acids have a significant impact on the quality of muscle proteins (Tang et al. 2021). Although limited research has been conducted on the influence of probiotics on the amino acid composition of chicken meat, several notable studies have examined this relationship (Guldas 2016; Popova 2017). Comparing control and experimental groups, it was found that the control group had greater levels of the majority of essential amino acids, such as lysine, leucine, threonine, valine, isoleucine, and phenylalanine. Similarly, Liu et al. (2017) demonstrated that adding probiotic Lactobacillus johnsonii to broiler chicken meals at low and high levels had no discernible effect on the meat amino acid composition. Sharipova et al. (2017) did not find any beneficial effects of B. subtilis supplementation on the amino acid content of broiler chicken meat. However, the results of the present study showed that the contents of a few amino acids were the highest in the experimental group. In addition, the means of valine, leucine/isoleucine, arginine and alanine were the highest in the group with diet of 0.5 mL of probiotics per bird. Intriguingly, chickens fed a diet containing a low dose of probiotics showed significantly higher concentrations of most amino acids than in the control group. Our results are consistent with those made by Podolian (2017), who noted that different dietary doses of a probiotic product made up of Lactobacillus bulgaricus and E. faecium resulted in appreciable increases in the amounts of lysine, valine, methionine, and phenylalanine. Contrary to the findings of our investigation, Sharipova et al. (2017) did not find any beneficial effects of B. subtilis supplementation on the amino acid content of broiler chicken meat. These collective findings underscore the intricate relationship between probiotics and amino acid composition in broiler chicken meat, suggesting that various factors including probiotic type and dietary application level play a role in influencing these outcomes.
pH, DL, CL
According to the obtained results, after 1 and 24 h of broiler meat storage, there was a substantial pH change between the control and probiotic groups. However, there was no difference by the end of storage at 192 h. No difference in DL was noticed after 24 h of storage. However, after 192 h, DL was significantly higher in the Pro2 group. No difference was observed in CL among the studied groups. Zheng et al. (2015) found that the pH of chicken breast meat in the groups treated with E. faecium was higher, resulting in a reduction in DL and cooking loss. DL and cooking loss are important in determining meat quality because some nutrients are rapidly lost owing to water loss during exudation (Bai et al. 2017), and DL is a growing problem in the meat industry, affecting both meat yield and quality (Abdullah et al. 2014). Furthermore, Xiong et al. (2015) found that pH was significantly and adversely linked with DL in pig meat after 24 h. However, the results of our current study indicated that pH was insignificantly connected with DL. pH results are consistent with Mohammed et al. (2021), who found that supplementing probiotic B. subtilis decreased the pH of meat 0.5 and 5 h after slaughter. Ivanovic (2012) reported meat pH increase with supplementation of B. cereus, whereas supplementation of E. faecium decreased the pH of meat. Dietary probiotics were beneficial for meat quality by improving the pH, tenderness, and colour (Pelicano et al. 2003).
Microbiology
Pseudomonas spp. have been identified as a possible spoiling factor in poultry meat (Rouger et al. 2017). According to Carrizosa et al. (2017), spoilage and off-flavour can result from the breakdown of amino acids when the concentration of putrefactive bacteria exceeds 8 log CFU/g. Furthermore, Pseudomonas spp. demonstrated a microbiological rejection level of 7.5 log CFU/g (Bruckner et al. 2012). In the present study, it was observed that after 192 h, all samples belonging to both the control and experimental groups did not reach the microbiological rejection limit. The higher level of moisture, drip loss, and pH value suggested by the Pro1 and Pro3 groups in comparison with the Pro2 and control groups could explain the differences in microbial count development between the control and supplemented groups. However, Lee et al. (2017) identified that after 120 h of bacterial growth in chicken meat increased over 8.3 log CFU/g, and Pseudomonas spp. was the most common genus responsible for the spoiling of chicken meat. The maximum acceptable level for the TVC in fresh meat, as indicated by Lázaro et al. (2015), is 7 log CFU/g. In our study, we observed that the control group exhibited a significantly higher TVC level than did the experimental groups, with an average of 6.8 log CFU/g over 192 h. These findings align with the results of Chmiel et al. (2022), who also reported an increase in TVC to approximately 6.5 log CFU/g on Day 9.
Sensory characteristics
The findings derived from our experimental study suggest that the addition of probiotics containing L. paracasei SH1, E. faecalis SH6, and K. unispora Y 2.2 in the water did not provide any statistically significant impact on the texture and preference of breast muscles obtained from Ross 308 broiler chickens at 42 days of age. According to Kabir (2009), probiotics have been found to have a positive impact on enhancing the sensory attributes of cooked broiler meat. In a study conducted by Alfaig et al. (2013), it was observed that the addition of probiotics to broiler cooked meat resulted in enhanced juiciness and tenderness. In contrast to the findings of Loddi et al. (2000), no statistically significant impact on various sensory attributes such as aroma intensity, flavour, tenderness, juiciness, acceptability, and overall appearance of breast muscles in 42-day-old Ross chickens was observed when administered with probiotics containing E. faecium, avoparcin probiotic, or a combination of probiotics and antibiotics, as compared with the control group. In a separate investigation conducted by Abdulla et al. (2017), it was shown that the treatment of probiotics containing B. subtilis did not have any statistically significant impact on the tenderness of breast muscles in 42-day-old Cobb 500 chickens.
Conclusions
In summary, the study demonstrated that the probiotics Lacticaseibacillus paracasei subsp. paracasei SH1, Enterococcus faecalis SH6, and Kazachstania unispora Y 2.2 positively affected bodyweight, carcass weight, and meat protein content in chickens. Average daily gain and feed conversion ratio were also significantly improved in the probiotic groups. No significant differences were found in the organoleptic quality between the control and experimental groups.
Overall, this study showed that probiotics can improve the growth performance, meat quality, and specific amino acid profiles of broiler chickens. These results suggest that camel and mare’s milk probiotics have potential to be a promising growth promoter that can contribute to improved poultry production and meat quality.
Data availability
The data that support the findings of this study are available from the corresponding author upon reasonable request.
Declaration of funding
This research has been funded by the Science Committee of the Ministry of Education and Science of the Republic of Kazakhstan (Grant no. AP22685916).
Acknowledgements
The authors extend their sincere appreciation to Professor Bernard Faye for his invaluable guidance and support during the statistical analysis phase of this research paper.
References
Abdulla NR, Zamri ANM, Sabow AB, Kareem KY, Nurhazirah S, Ling FH, Sazili AQ, Loh TC (2017) Physico-chemical properties of breast muscle in broiler chickens fed probiotics, antibiotics or antibiotic-probiotic mix. Journal of Applied Animal Research 45, 64-70.
| Crossref | Google Scholar |
Abdullah BM, Cullen JD, Korostynska O, Mason A, Al-Shamma’a AI (2014) Assessing water-holding capacity (WHC) of meat using microwave spectroscopy. In ‘Smart sensors, measurement and instrumentation’. (Eds A Mason, S Mukhopadhyay, K Jayasundera, N Bhattacharyya) pp. 117–140. (Springer) doi:10.1007/978-3-319-02318-2_7
Abou-Kassem DE, Elsadek MF, Abdel-Moneim AE, Mahgoub SA, Elaraby GM, Taha AE, Elshafie MM, Alkhawtani DM, Abd El-Hack ME, Ashour EA (2021) Growth, carcass characteristics, meat quality, and microbial aspects of growing quail fed diets enriched with two different types of probiotics (Bacillus toyonensis and Bifidobacterium bifidum). Poultry Science 100, 84-93.
| Crossref | Google Scholar | PubMed |
Afsharmanesh M, Barani M, Silversides FG (2010) Evaluation of wet-feeding wheat-based diets containing Saccharomyces cerevisiae to broiler chickens. British Poultry Science 51, 776-783.
| Crossref | Google Scholar |
Ahiwe EU, Tedeschi Dos Santos TT, Graham H, Iji PA (2021) Can probiotic or prebiotic yeast (Saccharomyces cerevisiae) serve as alternatives to in-feed antibiotics for healthy or disease-challenged broiler chickens? A review. Journal of Applied Poultry Research 30, 100164.
| Crossref | Google Scholar |
Al-Khalaifa H, Al-Nasser A, Al-Surayee T, Al-Kandari S, Al-Enzi N, Al-Sharrah T, Ragheb G, Al-Qalaf S, Mohammed A (2019) Effect of dietary probiotics and prebiotics on the performance of broiler chickens. Poultry Science 98, 4465-4479.
| Crossref | Google Scholar | PubMed |
Albrecht A, Herbert U, Miskel D, Heinemann C, Braun C, Dohlen S, Zeitz JO, Eder K, Saremi B, Kreyenschmidt J (2017) Effect of methionine supplementation in chicken feed on the quality and shelf life of fresh poultry meat. Poultry Science 96, 2853-2861.
| Crossref | Google Scholar |
Alfaig E, Angelovicova M, Kral M, Vietoris V, Zidek R (2013) Effect of probiotics and thyme essential oil on the texture of cooked chicken breast meat. Acta Scientiarum Polonorum, Technologia Alimentaria 12, 379-384.
| Google Scholar |
Aziz N, Khidhir Z, Hama Z, Mustafa N (2020) Influence of probiotic (miaclost) supplementation on carcass yield, chemical composition and meat quality of broiler chick. Journal of Animal and Poultry Production 11, 9-12.
| Crossref | Google Scholar |
Bai K, Huang Q, Zhang J, He J, Zhang L, Wang T (2017) Supplemental effects of probiotic Bacillus subtilis fmbJ on growth performance, antioxidant capacity, and meat quality of broiler chickens. Poultry Science 96, 74-82.
| Crossref | Google Scholar |
Begdildayeva NZ, Kudaibergenova AK, Nurgazina AS, Akhmetsadykova SN, Akhmetsadykov NN (2022) Fermented camel milk as a probiotics source for poultry farming. Food Science and Technology 42, e53122.
| Crossref | Google Scholar |
Bruckner S, Albrecht A, Petersen B, Kreyenschmidt J (2012) Characterization and comparison of spoilage processes in fresh pork and poultry. Journal of Food Quality 35, 372-382.
| Crossref | Google Scholar |
Carrizosa E, Benito MJ, Ruiz-Moyano S, Hernández A, Villalobos MdC, Martín A, Córdoba MdG (2017) Bacterial communities of fresh goat meat packaged in modified atmosphere. Food Microbiology 65, 57-63.
| Crossref | Google Scholar |
Chartrin P, Méteau K, Juin H, Bernadet MD, Guy G, Larzul C, Rémignon H, Mourot J, Duclos MJ, Baéza E (2006) Effects of intramuscular fat levels on sensory characteristics of duck breast meat. Poultry Science 85, 914-922.
| Crossref | Google Scholar |
Chmiel M, Roszko M, Hać-Szymańczuk E, Cegiełka A, Adamczak L, Florowski T, Pietrzak D, Bryła M, Świder O (2022) Changes in the microbiological quality and content of biogenic amines in chicken fillets packed using various techniques and stored under different conditions. Food Microbiology 102, 103920.
| Crossref | Google Scholar |
Fallah R, Kiani A, Azarfar A (2013) A review of the role of five kinds of alternatives to in-feed antibiotics in broiler production. Journal of Veterinary Medicine and Animal Health Review 5, 317-321.
| Crossref | Google Scholar |
Freitas AS, Carvalho LM, Soares AL, Madruga MS, Neto ACS, Carvalho RH, Ida EI, Estevez M, Shimokomaki M (2018) Further insight into the role of Ca2+ in broiler pale, soft and exudative-like (PSE) meat through the analysis of moisture by TGA and strong cation elements by ICP–OES. Journal of Food Science and Technology 55, 3181-3187.
| Crossref | Google Scholar |
Gao J, Zhang HJ, Yu SH, Wu SG, Yoon I, Quigley J, Gao YP, Qi GH (2008) Effects of yeast culture in broiler diets on performance and immunomodulatory functions. Poultry Science 87, 1377-1384.
| Crossref | Google Scholar |
Ghasemi-Sadabadi M, Ebrahimnezhad Y, Shaddel-Tili A, Bannapour-Ghaffari V, Kozehgari H, Didehvar M (2019) The effects of fermented milk products (kefir and yogurt) and probiotic on performance, carcass characteristics, blood parameters, and gut microbial population in broiler chickens. Archives Animal Breeding 62, 361-374.
| Crossref | Google Scholar |
Guldas M (2016) Effects of royal jelly and bee pollen on the growth of selected probiotic bacteria (Bf. animalis spp. Lactis, L. acidophilus and L. casei). Journal of Apicultural Science 60, 129-140.
| Crossref | Google Scholar |
Guo C-F, Zhang S, Yuan Y-H, Yue T-L, Li J-Y (2015) Comparison of lactobacilli isolated from Chinese suan-tsai and koumiss for their probiotic and functional properties. Journal of Functional Foods 12, 294-302.
| Crossref | Google Scholar |
Hou Q, Li C, Liu Y, Li W, Chen Y, Siqinbateer , Bao Y, Saqila W, Zhang H, Menghe B, Sun Z (2019) Koumiss consumption modulates gut microbiota, increases plasma high density cholesterol, decreases immunoglobulin G and albumin. Journal of Functional Foods 52, 469-478.
| Crossref | Google Scholar |
Ivanovic S (2012) The effect of different probiotics on broiler meat quality. African Journal of Microbiology Research 6, 937-943.
| Crossref | Google Scholar |
Jeni RE, Dittoe DK, Olson EG, Lourenco J, Corcionivoschi N, Ricke SC, Callaway TR (2021) Probiotics and potential applications for alternative poultry production systems. Poultry Science 100, 101156.
| Crossref | Google Scholar |
Kabir SML (2009) The role of probiotics in the poultry industry. International Journal of Molecular Sciences 10, 3531-3546.
| Crossref | Google Scholar |
Kalavathy R, Abdullah N, Jalaludin S, Ho YW (2003) Effects of Lactobacillus cultures on growth performance, abdominal fat deposition, serum lipids and weight of organs of broiler chickens. British Poultry Science 44, 139-144.
| Crossref | Google Scholar |
Kim HW, Yan FF, Hu JY, Cheng HW, Kim YHB (2016) Effects of probiotics feeding on meat quality of chicken breast during postmortem storage. Poultry Science 95, 1457-1464.
| Crossref | Google Scholar | PubMed |
Konuspayeva G, Baubekova A, Akhmetsadykova S, Faye B (2023) Traditional dairy fermented products in central Asia. International Dairy Journal 137, 105514.
| Crossref | Google Scholar |
Lázaro CA, Conte-Júnior CA, Canto AC, Monteiro MLG, Costa-Lima B, Cruz AGd, Mársico ET, Franco RM (2015) Biogenic amines as bacterial quality indicators in different poultry meat species. LWT 60, 15-21.
| Crossref | Google Scholar |
Lee KW, Lee SH, Lillehoj HS, Li GX, Jang SI, Babu US, Park MS, Kim DK, Lillehoj EP, Neumann AP, Rehberger TG, Siragusa GR (2010) Effects of direct-fed microbials on growth performance, gut morphometry, and immune characteristics in broiler chickens. Poultry Science 89, 203-216.
| Crossref | Google Scholar |
Lee HS, Kwon M, Heo S, Kim MG, Kim G-B (2017) Characterization of the biodiversity of the spoilage microbiota in chicken meat using next generation sequencing and culture dependent approach. Korean Journal for Food Science of Animal Resources 37, 535-541.
| Crossref | Google Scholar |
Lee S-K, Chon J-W, Yun Y-K, Lee J-C, Jo C, Song K-Y, Kim D-H, Bae D, Kim H, Moon J-S, Seo K-H (2022) Properties of broiler breast meat with pale color and a new approach for evaluating meat freshness in poultry processing plants. Poultry Science 101, 101627.
| Crossref | Google Scholar |
Liu L, Ni X, Zeng D, Wang H, Jing B, Yin Z, Pan K (2017) Effect of a dietary probiotic, Lactobacillus johnsonii BS15, on growth performance, quality traits, antioxidant ability, and nutritional and flavour substances of chicken meat. Animal Production Science 57, 920-926.
| Crossref | Google Scholar |
Loddi MM, Gonzales E, Takita TS, Mendes AA, Roca RD (2000) Effect of the use of probiotic and antibiotic on the performance, yield and carcass quality of broilers. Revista Brasileira De Zootecnia – Brazilian Journal of Animal Science 29, 1124-1131.
| Google Scholar |
Martínez Y, Valdivié M (2021) Efficiency of Ross 308 broilers under different nutritional requirements. Journal of Applied Poultry Research 30, 100140.
| Crossref | Google Scholar |
Mithun S, Dipak V, Sheela S (2015) Isolation and identification of lactobacilli from raw milk samples obtained from Aarey Milk Colony. International Journal of Scientific and Research Publications 5, 1-5.
| Google Scholar |
Mohammed AA, Zaki RS, Negm EA, Mahmoud MA, Cheng HW (2021) Effects of dietary supplementation of a probiotic (Bacillus subtilis) on bone mass and meat quality of broiler chickens. Poultry Science 100, 100906.
| Crossref | Google Scholar |
Patterson JA, Burkholder KM (2003) Application of prebiotics and probiotics in poultry production. Poultry Science 82, 627-631.
| Crossref | Google Scholar |
Pelicano ERL, Souza PAd, Souza HBAd, Oba A, Norkus EA, Kodawara LM, Lima TMAd (2003) Effect of different probiotics on broiler carcass and meat quality. Revista Brasileira de Ciência Avícola 5, 207-214.
| Crossref | Google Scholar |
Petracci M, Bianchi M, Cavani C (2009) The European perspective on pale, soft, exudative conditions in poultry. Poultry Science 88, 1518-1523.
| Crossref | Google Scholar | PubMed |
Podolian JN (2017) Effect of probiotics on the chemical, mineral, and amino acid composition of broiler chicken meat. Ukrainian Journal of Ecology 7, 61-65.
| Crossref | Google Scholar |
Popova T (2017) Effect of probiotics in poultry for improving meat quality. Current Opinion in Food Science 14, 72-77.
| Crossref | Google Scholar |
Rahmani Alizadeh M, Aliakbarpour H-R, Hashemi Karouei SM (2023) Effect of dietary supplementation of Iranian multi-strain probiotic or P. acidilactici of camel milk isolate on broilers performance, blood parameters, intestinal histology, and microbiota. Italian Journal of Animal Science 22, 660-665.
| Crossref | Google Scholar |
Rehman A, Arif M, Sajjad N, Al-Ghadi MQ, Alagawany M, Abd El-Hack ME, Alhimaidi AR, Elnesr SS, Almutairi BO, Amran RA, Hussein EOS, Swelum AA (2020) Dietary effect of probiotics and prebiotics on broiler performance, carcass, and immunity. Poultry Science 99, 6946-6953.
| Crossref | Google Scholar |
Rouger A, Tresse O, Zagorec M (2017) Bacterial contaminants of poultry meat: sources, species, and dynamics. Microorganisms 5, 50.
| Crossref | Google Scholar |
Sharipova A, Khaziev D, Kanareikina S, Kanareikin V, Rebezov M, Kazanina M, Andreeva A, Okuskhanova E, Yessimbekov Z, Bykova O (2017) The effects of a probiotic dietary supplementation on the amino acid and mineral composition of broilers meat. Annual Research & Review in Biology 21, 1-7.
| Crossref | Google Scholar |
Sjofjan O, Adli DN, Harahap RP, Jayanegara A, Utama DT, Seruni AP (2021) The effects of lactic acid bacteria and yeasts as probiotics on the growth performance, relative organ weight, blood parameters, and immune responses of broiler: a meta-analysis. F1000Research 10, 183.
| Crossref | Google Scholar |
Tang X, Liu X, Liu H (2021) Effects of dietary probiotic (Bacillus subtilis) supplementation on carcass traits, meat quality, amino acid, and fatty acid profile of broiler chickens. Frontiers in Veterinary Science 8, 767802.
| Crossref | Google Scholar |
Tavaniello S, Maiorano G, Stadnicka K, Mucci R, Bogucka J, Bednarczyk M (2018) Prebiotics offered to broiler chicken exert positive effect on meat quality traits irrespective of delivery route. Poultry Science 97, 2979-2987.
| Crossref | Google Scholar |
Wang Y, Li Y, Xie J, Zhang Y, Wang J, Sun X, Zhang H (2013) Protective effects of probiotic Lactobacillus casei Zhang against endotoxin- and d-galactosamine-induced liver injury in rats via anti-oxidative and anti-inflammatory capacities. International Immunopharmacology 15, 30-37.
| Crossref | Google Scholar |
Xiong X, Liu X, Zhou L, Yang J, Yang B, Ma H, Xie X, Huang Y, Fang S, Xiao S, Ren J, Chen C, Ma J, Huang L (2015) Genome-wide association analysis reveals genetic loci and candidate genes for meat quality traits in Chinese Laiwu pigs. Mammalian Genome 26, 181-190.
| Crossref | Google Scholar |
Zhang ZF, Zhou TX, Ao X, Kim IH (2012) Effects of β-glucan and Bacillus subtilis on growth performance, blood profiles, relative organ weight and meat quality in broilers fed maize–soybean meal based diets. Livestock Science 150, 419-424.
| Crossref | Google Scholar |
Zheng W, Zhang Y, Lu H-M, Li D-T, Zhang Z-L, Tang Z-X, Shi L-E (2015) Antimicrobial activity and safety evaluation of Enterococcus faecium KQ 2.6 isolated from peacock feces. BMC Biotechnology 15, 30.
| Crossref | Google Scholar |
Zhou X, Wang Y, Gu Q, Li W (2010) Effect of dietary probiotic, Bacillus coagulans, on growth performance, chemical composition, and meat quality of Guangxi Yellow chicken. Poultry Science 89, 588-593.
| Crossref | Google Scholar | PubMed |