Role of plant bioactive compounds in improving ruminant resilience to heat stress challenge
Farhad Ahmadi
A
B
Abstract
This review presents the reader with a comprehensive overview of the impact of phytogenic compounds on the productivity and health of lactating cows and growing ruminants, including calves and sheep, during heat stress challenge. Exposure to heat stress may result in reduced feed intake, compromised immune function, oxidative stress and productivity losses. The positive impacts of phytogenic compounds on the health and productivity of farm animals is well established, and recent evidence suggests that they may serve as an effective nutritional strategy to mitigate the adverse effects of heat stress on animals. More information is available on lactating cows than calves, particularly during the pre-weaning period. Although their specific mode of action is not clearly understood, some phytogenic compounds stimulate feed consumption in animals experiencing heat stress, which is associated with improved productivity (milk production or growth) compared with non-supplemented animals. Phytogenics may also play a role in improving immune and endocrine function, and alleviate hyperthermia-induced oxidative stress. Certain phytogenics (e.g. capsaicin) may regulate body temperature by expanding the blood vessels in the skin and facilitating heat exchange in heat-loaded animals. Phytogenic compounds may play a regulatory role in insulin secretion and sensitivity, promoting energy partitioning for productive purposes and increasing the resilience of animals to heat stress challenges. Still, it is a largely unexplored research area. Additional research is required at varying degrees of heat stress intensity and duration to confirm the potential effects of phytogenic compounds and expand upon these encouraging findings.
Keywords: botanical, endocrine function, inflammation, oxidative stress, phytonutrients, plant secondary metabolites, thermal stress, thermoregulation.
Introduction
Increased environmental temperature may disrupt the thermoregulatory mechanisms of animals, impairing their ability to dissipate heat efficiently and leading to increased heat load on the body (Chauhan et al. 2021; Rebez et al. 2023). This can negatively impact ruminants, as they tend to consume less feed when exposed to heat stress. This decreased feed intake, coupled with elevated energy expenditure for heat dissipation and physiological adaptations, compromises health and productivity (Baumgard and Rhoads 2013; Thornton et al. 2022). Consequently, heat stress jeopardises the profitability and sustainability of livestock production systems (Lemal et al. 2023).
Hyperthermia promotes excessive production of free radicals and reactive oxygen species (ROS), disrupting the balance between antioxidants and oxidants, and impairing normal metabolic processes (Chauhan et al. 2014c, 2021; Martemucci et al. 2022). When ROS level exceeds the neutralising capacity of antioxidative mechanisms, oxidative stress may develop, leading to inflammation, particularly within the gastrointestinal tract (Abeyta et al. 2023). The gastrointestinal tract operates as an energy-intensive organ, utilising a significant quantity of oxygen (Dunshea et al. 2017). Hyperthermia redirects the blood flow from the internal organs towards peripheral surfaces, resulting in a diminished supply of oxygen and nutrients to the rumen, and gastrointestinal tract tissues, potentially causing damage to the cells lining these tissues. Nutrient absorption is compromised, and under extreme circumstances, intestinal hypoxia and enterocyte damage may develop, accelerating the formation of ROS, and impairing cellular structures and membrane integrity (Tang et al. 2023). Systemic inflammation resulting from the dysfunctional intestinal barrier, and infiltration of bacterial lipopolysaccharides and other antigens activate the immune system, an energetically expensive process diverting nutrients from productive purposes (Baumgard and Rhoads 2013; Koch et al. 2019). Heat stress may also impair immune system function, leaving animals vulnerable to infectious diseases (Gupta et al. 2023; Lemal et al. 2023).
Substantial investments are needed to adapt livestock production to climate change, particularly rising temperatures and frequent heat waves, while maintaining animal health and productivity (Thornton et al. 2022). Among nutritional interventions investigated, the use of phytogenic compounds in animal diets has emerged as a promising approach to reducing oxidative stress and improving heat stress resilience in young and adult ruminant animals (Rochfort et al. 2008; Ahmadi et al. 2023). These compounds provide a range of benefits through their immunomodulatory, anti-inflammatory, antioxidant and antimicrobial effects. The increasing discouragement of antibiotics in animal production, driven by consumer preferences, has further attracted increased interest in the use of phytogenic compounds as natural alternatives to enhance the productivity and health of farm animals.
Studies have shown that phytogenic compounds can mitigate heat stress in monogastric animals (Abdel-Moneim et al. 2021; Papatsiros et al. 2022). Plenty of information also exists on the efficacy of phytogenic compounds in supporting the health and productivity of adult ruminants and young calves. For an in-depth review, readers are invited to refer to the review papers by Olagaray and Bradford (2019) and Reddy et al. (2020). However, the effectiveness of phytogenic compounds in heat-stressed ruminant animals, particularly growing calves, remains to be extensively investigated. Therefore, this review provides a thorough overview of the current literature investigating the effects of phytogenic compounds on the productivity and health of dairy cows and growing ruminant animals under heat stress challenge, and attempts to identify gaps in knowledge and suggest directions for future research.
Methodology
This review followed a systematic approach to identify existing literature on the impact of feeding phytogenic compounds on health and productivity of adult ruminant and young calves under heat stress challenge. The literature search was restricted to identifying peer-reviewed documents published between 2014 and 2024 accessed through the Web of Science, PubMed and ScienceDirect databases. The search strategy utilised keywords, including antioxidant activity, bioactive compound, calves, dairy cow, heat stress, oxidative stress, phytochemical, phytogenic, plant extract, ruminant, sheep and weaning. Inclusion criteria included original research articles published in English, with clearly specified dosage and supplementation durations of phytogenics, and clearly defined heat stress indicators, such as mean temperature or temperature–humidity index. Studies were excluded if they were available only in abstract form or classified as a thesis, book or book chapter, review, meta-analysis, conference proceeding, letter, or commentary. The data presented in Tables 1–3 includes a total of 39 studies: 22 on dairy cows, eight on calves and nine on sheep. The data collected from these studies includes animal type, physiological stage (e.g. days in milk for dairy cows or pre- and post-weaning classifications for calves), the supplementation details, heat stress indicator and major outcomes, mainly including feed intake, productivity, oxidative stress markers or immune responses. The results of these studies are summarised in Tables 1–3.
Effects of phytogenic compounds on farm animals under heat stress
Based on the studies identified in this review, the effects of phytogenic compounds on ruminant animals exposed to heat stress are discussed in the following sections, categorized by species (dairy cows, calves, and sheep). To support this discussion, Fig. 1 illustrates an overview of key ways by which phytogenic compounds may help to alleviate the physiological impacts of heat stress in different ruminant species.
Graphical representation highlighting the mechanisms of heat stress abatement by using plant bioactives. The figure was created using BioRender (https://biorender.com/ accessed on 27 August 2023).
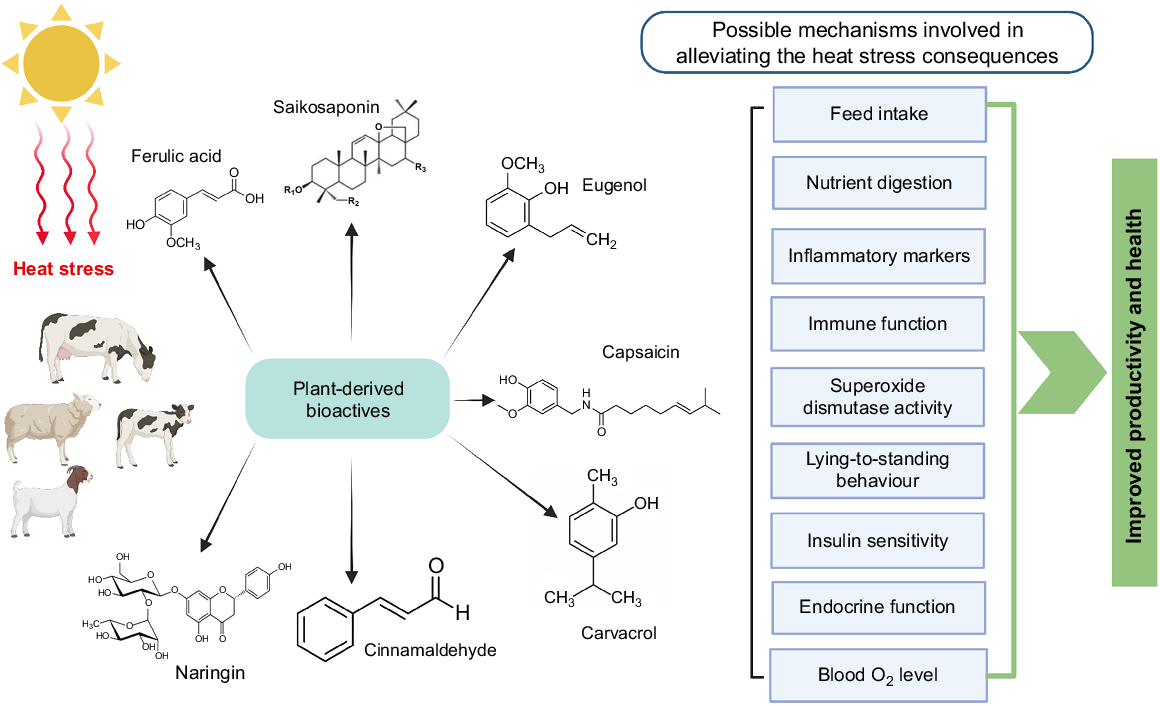
Dairy cows
Lactating cows experiencing heat stress commonly show depressed feed intake, increased inflammation, compromised gut health and decreased milk production (Becker et al. 2020). Glucose is prioritised for fuelling the activated immune system instead of being utilised for milk synthesis. For example, lactating cows with a hyperactivated immune system consumed >1 kg of glucose over 12 h (Kvidera et al. 2017). Despite implementing heat stress-abating strategies at commercial farms, the dairy industry continues to face substantial challenges caused by heat stress. Nutritional interventions, such as using antioxidants, have mitigated some of the adverse effects caused by heat stress (Dunshea et al. 2022). Table 1 presents a comprehensive overview of the literature about the impacts of feeding phytogenic compounds on productivity and health of lactating dairy cows under heat stress challenge.
Animal information | Mean THI or temperature | Plant/antioxidant | Major bioactive compound | Dosage | Duration | Major outcomes | References | |
---|---|---|---|---|---|---|---|---|
Holsteins (150 DIM) | 83.9 | Capsicum oleoresin | Capsaicin | 0.02, 0.04 or 0.08 g/kg DM | 30 days |
| An et al. (2022) | |
Holsteins (34 DIM) | 91.4 | Capsaicin | Capsaicin | 0.02, 0.04, 0.06 or 0.08 g/kg DM | 30 days |
| Abulaiti et al. (2021) | |
Holsteins (181 DIM) | 70.8 | A microencapsulated blend | Capsaicin, cinnamaldehyde, carvacrol and eugenol | 0.15 g/kg DM | 56 days |
| da Silva et al. (2020) | |
Mid-lactation Mediterranean buffaloes | 79.9 | Capsicum oleoresin | Capsaicin | 0.01, 0.02 or 0.04 g/kg DM | 21 days |
| An et al. (2023) | |
Holsteins (139 DIM) | A | A proprietary antioxidant blend | Ethoxyquin, tertiary butyl hydroquinone and citric acid | 10 g/day | 7 days |
| Abeyta et al. (2023) | |
Holsteins (105 DIM) | 74.6 | Lonicera japonica | Polyphenols | 14, 28 or 56 g/day | 70 days |
| Ma et al. (2020) | |
Holsteins (75 DIM) | 78.8 | Radix Bupleuri | Saikosaponins | 0.25, 0.5 or 1.0 g/kg DM | 70 days |
| Pan et al. (2014), Cheng et al. (2018) | |
Murrah buffalos (90 DIM) | 82 | Mulberry leaf | Flavones and flavonols | 15, 30 or 45 g/day | 45 days |
| Li et al. (2020) | |
Holsteins (230 DIM) | 74.5 | A proprietary fermented blend of 18 phytogenics | – | 25, 50, or 100 g/day | 42 days |
| Shan et al. (2018), Zhao et al. (2022) | |
Early-lactation Holstein-Friesians | MT = 35°C | A proprietary blend B | Alkaloids, curcumin and menthol | 50 or 100 g/head/day |
| Saleh et al. (2023) | ||
Holsteins (208 DIM) | 78 | Organic acid + pure botanicals | Thymol and vanillin | 0.075 g/kg BW | 14 days |
| Fontoura et al. (2022) | |
Holsteins (75 DIM) | C | Citrus extract | Ascorbic acid | 4 g/head/day | 28 days D |
| Havlin and Robinson (2015) | |
Holsteins (91 DIM) | 79 | A phytogenic blend | Carvacrol, limonene and anethole | 15 g/head/day | 35 days |
| Bagheri Varzaneh (2022) | |
Postparturient Holsteins | 80 | Pomegranate by-product silage | Phenols | 60, 120 or 180 g/kg DM | 63 days |
| Khorsandi et al. (2019) | |
Holstein-Friesians (118 DIM) | 73.8 | Garlic + pinecone oil + brown seaweed (1:1:1 ratio) | Alliin, γ-terpinene, 2-β-pinene, dl-limonene and isolongifolene | 0.16 g/kg DM | 40 days |
| Lee et al. (2020) | |
Holstein Friesian × Tharparkar cows (130 DIM) | 82.2 | Chlorophytum borivilianum | Alkaloids, saponins, flavonoids and polyphenols | 0.04 or 0.08 g/kg BW | 90 days |
| Devi et al. (2021a, 2021b) | |
Holsteins (94 DIM) | MT = 32.3°C | Propolis extract | Phenols and flavonoids | 32 or 64 mL/day | 17 days E |
| Varela et al. (2023) | |
Holsteins (180 DIM) | 80.1 (1st period), 78.4 (2nd period) | Plant polyphenol extract | Green tea, fenugreek, capsicum and electrolytes | 100 or 150 g/day | 56 days |
| Daddam et al. (2023) | |
Holsteins (20 DIM) | 91.0 | A fermented herbal preparation | Flavonoids and polyphenols | 0.5, 0.75 and 1 kg/cow/day | 30 days |
| Abulaiti et al. (2024) |
DIM, days in milk; IgA, immunoglobulin A; IgG, immunoglobulin G; THI, temperature-humidity index; MT, mean temperature; MY, milk yield; RT, rectal temperature; RR, respiratory rate.
Havlin and Robinson (2015) reported that feeding a citrus extract at a daily rate of 4 g/head in the diet of high-producing Holsteins exposed to heat stress did not benefit productivity. However, their findings demonstrated a positive impact on mammary health, as evidenced by lower somatic cell count, an indicator of the inflammation intensity in the mammary gland (Rainard et al. 2018). Somatic cell counts exhibit seasonal fluctuations, and lactating cows experiencing heat stress often peak in somatic cell count, often coinciding to an increased mastitis infection incidence during a hot summer (Archer et al. 2013). Lower somatic cell counts in cows receiving citrus extract may be attributed to the role of vitamin C in supporting a favourable balance between antioxidants and pro-oxidants that helped minimise oxidative damage and promote healthier mammary glands. Dairy cattle can synthesise enough vitamin C from glucose in their liver, which is necessary to support and sustain the healthy functioning of mammary glands. However, cows experiencing heat or immunological stress, particularly high-yielding cows in early lactation, may be unable to synthesise sufficient amounts of vitamin C, and supplemental vitamin C may help to counteract the oxidative stress (Matsui 2012). Additionally, this study revealed that the lying-to-standing proportion increased in cows receiving citrus extract. The length and frequency of lying behaviour are important signs of cow comfort, and provide valuable information into the cow–environment interaction (Tucker et al. 2021). Cows experiencing heat stress tend to reduce the time they spend lying, while spending more time standing to maximise their body surface area for heat dissipation, a behavioral adaptation aimed at minimising exposure to direct sunlight and ground radiation (Gaughan et al. 2019). The increased standing time can lead to increased energy expenditure and increased maintenance requirements (Becker et al. 2020).
Ma et al. (2020) investigated the effects of dietary Lonicera japonica extract, a rich source of polyphenolic compounds with potent antioxidant properties, on dairy cows maintained under elevated ambient temperatures. Cows receiving 28 g/day of Lonicera japonica extract showed improved antioxidant status and immune function. Cows consuming Lonicera japonica extract showed higher circulating concentrations of triiodothyronine and thyroxine, indicating a positive impact of the extract on endocrine regulation. Increased core body temperature due to exposure to heat stress may have an inhibitory effect on thyroid cell proliferation and secretion. The decreased concentration of thyroxine and triiodothyronine may represent an adaptive mechanism to limit metabolic heat production (Sejian et al. 2018).
Certain phytogenic compounds may promote blood vessel dilation and improve heat dissipation through skin evaporation. Radix Bupleuri is widely recognised for its potent antibacterial, antioxidant and anti-inflammatory properties, mainly attributed to the high concentration of saikosaponin, the primary active compound in Radix Bupleuri (Yuan et al. 2017). Holstein cows experiencing thermal stress and receiving Radix Bupleuri extract at a concentration of 0.25 or 0.5 g/kg exhibited a lower respiratory rate and rectal temperature (Pan et al. 2014). Rectal temperature is a reliable proxy for core body temperature, and its elevation during heat stress may indicate a diminished capacity to maintain thermal homeostasis (Sejian et al. 2018). The supplementation improved cow comfort, resulting in increased feed intake and milk production. During heat stress, animals may reduce their feed intake as an adaptive mechanism to counteract metabolic heat production (Gonzalez-Rivas et al. 2020). In another study, capsicum oleoresin, with capsaicin as the primary active component, was top-dressed at 0.02, 0.04 and 0.08 g/kg DM in the diet of lactating Holsteins under heat stress (An et al. 2022). Rectal temperature was the lowest in cows consuming capsicum oleoresin at a daily dose of 0.02 g/kg.
In a 56-day lactation study, a blend of capsaicin, carvacrol, eugenol and cinnamaldehyde was microencapsulated and top-dressed at 0.15 g/kg DM to the ration of dairy cows, assumed to be experiencing a mild degree of heat stress (da Silva et al. 2020). The blend increased feed intake and milk production, and lowered the frequency of high rectal temperature, likely via the vasodilation mechanism. Research on humans suggest that capsaicin may have a thermoregulatory function by expanding the blood vessels in the skin and facilitating heat exchange (Adaszek et al. 2019). The transient receptor potential vanilloid 1 receptor in the preoptic-anterior area of the hypothalamus is activated by capsaicin, stimulating the warm-sensitive neurons and preventing the activation of cold-sensitive neurons (Szolcsányi 2015). In contrast, feeding heat-stressed dairy cows an encapsulated pepper (rich in capsaicin) administered at 0.75 or 1.5 g/day increased feed intake and fat-corrected milk production, but did not affect the skin and rectal temperature, and respiration rate (Vittorazzi et al. 2022).
A proprietary blend of tertiary butyl hydroquinone, ethoxyquin and citric acid top-dressed at a rate of 10 g/day onto the ration of heat-stressed lactating dairy cows did not alter the inflammatory profile or productivity, but the supplemented cows tended to have lower blood urea nitrogen levels compared with those not receiving the supplementary blend (Abeyta et al. 2023). Immune system activation induced by hyperthermia demands substantial energy, promoting the degradation of skeletal muscle to supply amino acids for acute-phase protein synthesis and gluconeogenesis. Markers of muscle breakdown, such as blood urea nitrogen and creatine, usually increase in animals suffering from heat stress (Baumgard and Rhoads, 2013). Feeding a blend of organic acid and plant extracts in pure form (1.7% thymol and 1.0% vanillin) to heat-stressed mid-lactation Holsteins at a rate of 0.075 g/kg of bodyweight increased feed intake and nitrogen efficiency, demonstrated by increased milk protein and less nitrogen excretion. Hyperthermia may inhibit milk protein synthesis in the mammary gland, likely through the downregulation of major genes involved in milk protein and heat stress-induced mitochondrial dysfunction (Guo et al. 2021). Increased milk protein in the supplemented dairy cows exposed to heat stress was attributed to the greater energy intake and elevated circulating insulin that likely promoted protein synthesis in the mammary glands.
In early lactation, the body activates insulin resistance (or insensitivity) as a homeostatic mechanism to decrease glucose utilisation in adipose and muscle tissues. Instead, glucose is directed towards immune cells and the mammary glands to support the increased demand for milk synthesis (Piantoni and VandeHaar 2022). The function of inflammatory immune cells is modulated by insulin, which enhances their ability to use glucose as a metabolic fuel (Kvidera et al. 2017). Insulin insensitivity may disturb the mechanisms responsible for regulating body temperature, causing challenges in dealing with the negative impacts of heat stress in animals (Dunshea et al. 2013). Despite the depressed feed consumption and weight loss, dairy cows under heat stress may exhibit an elevation in circulating insulin, promoting a faster glucose clearance rate. Lactating cows under heat stress tend to mobilise less body fat because of increased insulin sensitivity, likely as a part of their adaptive response to heat stress (Baumgard and Rhoads 2013).
Phytogenic compounds may regulate insulin secretion and sensitivity in ruminants (Oh et al. 2017). This regulation may increase glucose accessibility to the mammary gland and potentially increase milk synthesis in lactating cows. In an in vitro heat stress model, cells derived from bovine subcutaneous adipose tissue were treated with 100 μM resveratrol, a natural polyphenol antioxidant. Resveratrol promoted adipose lipolysis and decreased lipogenesis (Kra et al. 2021), which may increase energy availability. A phytogenic preparation containing turmeric, rosemary, clove bud and cinnamon bark improved insulin sensitivity in dairy cows kept in thermoneutral conditions (Hashemzadeh-Cigari et al. 2015). Gohlke et al. (2013) reported that flavonoid quercetin administered intraduodenally at 36 mg/day/kg bodyweight over 4 weeks positively affected insulin secretion and sensitivity in high-producing cows in early lactation. This demonstrates the contribution of quercetin to the adjustment of glucose metabolism and promoting metabolic adaptation in early-lactation cows. More recently, Nicolás-López et al. (2023) reported that a 40-day ferulic acid supplementation (0.25 g/kg diet) of heat-stressed ewes improved insulin sensitivity, as demonstrated by an increase in the insulin:glucose ratio.
Phytogenic compounds and heat stress may have an additive effect in increasing insulin sensitivity during episodes of heat stress, and increase the resilience of high-yielding cows. Limited information is available on this topic, and more research is necessary to better understand the potential mechanisms of action by which phytogenic compounds affect insulin sensitivity under a heat stress challenge. Assessing the impact of varying durations and intensities of heat stress on insulin sensitivity at tissue-specific and whole-body levels in response to feeding phytogenic compounds would also provide valuable insights into improving metabolic resilience in ruminant animals facing heat stress challenges.
Periparturient dairy cows experience substantial metabolic stress, even under normal conditions, resulting in elevated formation of ROS. To support the production, immune function and metabolism of transition dairy cows, it is important to minimise additional external stressors, such as heat stress, which could exacerbate adverse health and productivity outcomes. Heat stress during the prepartum period can have persistent negative impacts on postpartum cows, such as impaired innate immune function and increased susceptibility to uterine diseases (Molinari et al. 2023). Exposure of peripartum cows to high temperatures may have adverse carryover effects on cell-mediated and passive immune function, and metabolic function of the neonate, with potential long-term implications for calf health and development (Abuelo et al. 2019; Tao et al. 2019). Prenatal heat stress has impaired the transfer of immunoglobulins through colostrum, which is vital for calf health and survivability (Tao et al. 2012). These effects on the neonate highlight the prioritisation of heat stress mitigation strategies for prepartum lactating cows.
During hot weather conditions, transition dairy cows are particularly susceptible to metabolic disorders, such as ketosis and fatty liver, most likely because of increased maintenance requirements for thermoregulation and reduced feed intake (Bernabucci et al. 2010). A summer study investigated the effects of supplementing postpartum dairy cow diets with pomegranate by-product silage (49 g polyphenols/kg DM) at 120 g/kg of diet DM. Supplementation increased milk yield, but reduced milk protein and lactose concentrations, and resulted in improved comfort and welfare, as supplemented cows spent more time lying down (Khorsandi et al. 2019).
Phytogenic compounds have shown varying resistance to ruminal degradation. For example, quercetin is highly susceptible to degradation by ruminal microorganisms (Berger et al. 2015), but it still remains readily available for absorption and utilisation by pre-ruminant calves (Maciej et al. 2016). In a continuous culture study, carvacrol, menthone and thymol had a recovery rate of approximately 60% after a 24-h ruminal fermentation (Franz et al. 2010). Some phytogenic compounds may negatively affect the fermentative processes in the rumen, and potentially affect feed efficiency and productivity. This signifies the necessity of a comprehensive evaluation of phytogenic compounds on ruminal fermentative patterns, and their degradation in the rumen before being administered into the ruminant diet.
Plant bioactive compounds, once absorbed from the small intestine, can be transported to the mammary glands and subsequently excreted into milk (Lejonklev et al. 2013; Lejonklev et al. 2016). The transfer of bioactive compounds to milk can provide nutritional and health benefits for consumers. De Paris et al. (2020) investigated the effects of a daily supplementation of oregano extract (10 g) and green tea extract (5 g) from 21 days prepartum to 21 days postpartum. This supplementation positively affected redox status biomarkers in both the cows and their calves, the latter consuming 2 L/day of their dam milk from Days 6 to 60 of life.
Colostrum contains several bioactive components with antioxidant properties (Costa et al. 2023). Antioxidants in colostrum may shield newborn calves from ROS and stimulate immune responses. A porcine model study demonstrated that supplementing hyperprolific sows with phytogenic compounds during gestation and lactation resulted in increased colostrum protein content and enhanced antioxidant status in piglets (Reyes-Camacho et al. 2020). The authors reported that the phytogenic compounds increased colostrum protein content and were transferred to piglets. Furthermore, the supplementation positively affected the expression of genes associated with gut health in piglets. These findings highlight the potential benefits of using phytogenic compounds to enhance maternal and offspring health in pig production. Incorporation of phytogenic additives into the diet of transition cows may improve the antioxidant capacity of colostrum, and benefit the antioxidant status of newborn calves. Current knowledge on this topic is limited, particularly in relation to the heat stress challenge, which has received less attention in previous research.
Neonatal bovine calf
The long-term viability and productivity of a dairy operation are intricately linked to maintaining calf growth and health (Soberon et al. 2012a). In mammals, the birth process is accompanied by a rise in ROS formation, primarily because of the abrupt transition from the low-oxygen intrauterine environment to the higher-oxygen concentration of the external environment (Przybylska et al. 2007). Calves immediately after birth and before colostrum ingestion were shown to have approximately 30% more ROS compared with their dams (Gaál et al. 2006). This vulnerability is further exacerbated by the underdeveloped antioxidant defense mechanisms in neonatal calves. Oxidative stress may be initiated in pre-ruminant calves during various stressor conditions, such as the weaning process (Majlesi et al. 2021), the transition from whole milk (richer in antioxidants) to milk replacer (Soberon et al. 2012b) or exposure to elevated environmental temperature (Wickramasinghe et al. 2023). Interventions that primarily enhance the antioxidant pool in newborn calves may mitigate the negative consequences of oxidative stress, ultimately promoting the development of healthier and more productive animals.
Although young calves are generally more tolerant to heat stress than adult ruminants, recent evidence emphasises the adverse effects of heat stress on calf physiology and metabolism, which is associated with increased morbidity and mortality (Roland et al. 2016; Wang et al. 2020). Heat stress burdens growing animals by reducing feed intake while activating energy-intensive thermoregulatory mechanisms to maintain core body temperature (Fontoura et al. 2023). Energy diversion towards thermoregulation for physiological homeostasis and immune system activation may limit the energy available for growth. Calves exposed to heat stress grew slower than those managed with proper heat-stress abatement measures or those born during the winter (López et al. 2018). Dado-Senn et al. (2020) demonstrated that prenatal heat stress abatement improved immunoglobulin transfer and calf growth, whereas postnatal heat stress abatement improved thermoregulatory responses, increased feed intake and promoted better health.
Administration of phytogenic compounds to newborn calves is an emerging field of research, driven by evidence demonstrating their potential in reducing pathogenic load within the gastrointestinal tract, boosting immune function, and promoting starter intake and growth (Supplementary Table S1). These studies were primarily conducted under thermoneutral conditions, but less information is available on the potential benefits of phytogenic compounds for calves reared under heat stress. Table 2 presents an overview of the available literature on the effects of feeding phytogenic compounds to calves (via liquid and solid diet) facing heat stress, covering both the pre- and post-weaning periods.
Animal information | Mean THI | Plant/antioxidant | Major bioactive compound | Dosage | Route of delivery (duration) | Major outcomes | References | |
---|---|---|---|---|---|---|---|---|
Pre-weaning period | ||||||||
Holstein female calves | 80.3 | Grape seed extract | Catechin, gallic acid, epicatechin, procyanidin dimers, kaempferol and protocatechuic acid | 0.025, 0.05 and 0.1 g/kg BW | Milk (Day 4 to 63) | Urkmez and Biricik (2022a , 2022b) | ||
Holstein bull and heifer calves | 79 | Organic acids + pure botanicalsA | Thymol and vanillin | 0.075 or 0.15 g/kg BW | Oesophagus bolus (Week 1 through 13) | Fontoura et al. (2023) | ||
Post-weaning period | ||||||||
5–6-month-old Holstein and Jersey heifers | 85 | A proprietary blend of vanilla, clove, cinnamon, licorice and caraway | Vanillin, eugenol, cinnamaldehyde, glycyrrhizin, limonene and carvone | 0.25 g/head/day | DietB | Wickramasinghe et al. (2023) | ||
8-month-old Holstein heifers | 79.2 | Fermented herbal tea residue | Flavones and alkaloids | 50 and 100 g/kg, replacing corn silage | Diet (31 days) | Xie et al. (2020) | ||
10-month-old Jinjiang beef | >79 | Proprietary herbal blend | Baicalin, hesperidin and berberine | 10 g/kg DM | Diet (60 days) | Song et al. (2014) | ||
14-month-old Angus beef | >72 | Allium mongolicum Regel powder | Flavonoid and polyphenol | 0, 10, 15 and 20 g/calf/day | Diet (120 days) | Liu et al. (2024) | ||
1.5-month-old Jinjiang beef | 81.8 | Radix Puerarin extract | Puerarin | 0.2, 0.4 and 0.8 g/kg concentrate mix | Diet (60 days) | Li et al. (2021) |
Grape seed extract at 0.05 and 0.1 g/day/kg bodyweight, administered through milk to heat-stressed young calves, resulted in reduced plasma concentrations of malondialdehyde, interferon-γ and tumour necrosis factor-α, key biomarkers of oxidative stress and inflammation. Grape seed extract supplementation also increased the calf antioxidant defence system, demonstrated by increased superoxide dismutase activity and total antioxidant capacity (Urkmez and Biricik 2022a). Superoxide dismutase is a vital initial defence mechanism against ROS, and its role is to catalyse the conversion of superoxide radicals into hydrogen peroxide and oxygen (Islam et al. 2022). The observed positive effects on antioxidant and anti-inflammatory activities were useful in mitigating the negative consequences of heat stress, and improving calf growth and health (Urkmez and Biricik 2022a, 2022b).
Fontoura et al. (2023) fed heat-stressed calves a blend of organic acid (25% citric acid and 16.7% sorbic acid) and a pure plant extract (1.7% thymol and 1.0% vanillin) administered through the oesophagus from Week 1 to 13 of calf life, at a dosage of 0.075 or 0.15 g/kg bodyweight. This supplementation improved feed intake during acute heat stress episodes, but did not impact growth or inflammatory marker responses (Fontoura et al. 2023). The authors suggested that the moderate level of heat stress experienced by the calves may not have been sufficient to trigger significant inflammatory responses. This highlights the need to examine further the impact of this blend on calves subjected to severe heat stress. Contrary to this finding, administering a similar blend at 75 mg/kg bodyweight increased feed consumption in heat-stressed dairy cows (Fontoura et al. 2022). This discrepancy in findings likely reflects the different nutritional, physiological and metabolic demands of growing calves compared with lactating dairy cows.
More recently, a proprietary phytogenic blend consisting of vanilla, clove, cinnamon, licorice and caraway was top-dressed at 0.25 g/head/day into the diet of weaned heifer calves subjected to a diurnal heat stress bout (Wickramasinghe et al. 2023). Feeding the blend during a 7-day cyclic heat stress period, followed by a 4-day post-heat stress period, increased blood oxygen level, alleviated oxidative stress as shown by lower protein carbonyl and thiobarbituric acid, and lowered inflammation (as characterised by lower blood haptoglobin and lipopolysaccharide-binding protein). However, calf growth remained unaffected, most likely because of the limited duration of phytogenic blend supplementation. Overall, the impact of feeding phytogenic compounds on heat-stressed growing calves, particularly during the pre-weaning phase, has yet to be extensively investigated.
Diarrhoea remains the primary factor responsible for morbidity and mortality in newborn calves, resulting in substantial economic losses (Carter et al. 2021). The aetiology of calf diarrhoea is multifaceted, with climate and the housing systems playing important roles. New research shows that early-life administration of certain phytogenic compounds can decrease the frequency and severity of neonatal calf diarrhoea. This positive effect is likely attributed to their antimicrobial and antiparasitic properties, helping to control pathogenic microorganisms and supporting the establishment of a healthy gut microbiota. Establishing a healthier gut environment, characterised by a lower parasite load, has shown promise in alleviating the intensity and duration of diarrhoeal episodes in newborn calves. Condensed tannins have particularly positive effects in this regard. They reduce the viability of larval stages of parasites and disrupt the hatching of eggs, impeding their life cycle and reducing their impact on calf health (Tong et al. 2022).
Calves, during the initial days of their life, are highly susceptible to intestinal disturbances caused primarily by enteric parasitic and bacterial infections. This susceptibility is exacerbated by exposure to elevated temperatures. The combination of these stressors can trigger proinflammatory reactions, jeopardising the health and development of neonatal calves. According to information provided in Supplementary Table S1, certain phytogenics may reduce pathogenic loads in the gut environment, boost intestinal barrier integrity, improve immune function and suppress inflammatory cytokines. However, their effectiveness in heat-stressed newborn calves remains largely unexplored. Currently, limited information is available on the efficacy of phytogenic compounds in alleviating neonatal calf diarrhoea incidence and improving gut health under heat-stress conditions. This a largely unexplored area of research that needs further investigation.
Administering phytogenic compounds to calves through liquid or solid feed has several advantages, such as practicality and ease of integration into existing farm routines, as compared with labour-intensive administration methods, such as direct oral dosing or injections. This is especially important for dairy operations with limited labour resources. However, the route of administration may influence the stability, bioavailability and efficacy of phytogenic compounds. During the production of pelleted calf starters, the inclusion of phytogenic compounds in the formulation may be impacted by the heat and pressure of pelleting that can alter their chemical structure or functionality, which may result in altered bioavailability (Turek and Stintzing, 2013). However, the addition of phytogenics into a liquid diet (milk or milk replacer) may avoid the potential degradation, and preserve their quality and functionality.
The reflex closure of the oesophageal groove in calves facilitates the direct flow of milk to the abomasum and small intestine, bypassing the reticulorumen (Dehority 2002). This mechanism minimises exposure to rumen microorganisms, which could otherwise degrade sensitive phytogenic compounds. Despite being pre-ruminant animals, microbial colonisation begins in the rumen shortly after birth, and some degree of ruminal degradation may occur when phytochemicals are delivered via the starter feed. For a substance to exert postabsorptive effects in vivo, it must be absorbed into the circulation and distributed throughout the body. As a pre-ruminant calf develops, intestinal permeability, absorptive capacity, rumen development and microbiome colonisation undergo significant alterations (Olagaray and Bradford 2019). These changes potentially impact the absorption of phytogenics into calf circulation. The dynamicity of the gastrointestinal system in the growing calf during this developmental phase influences how phytogenic compounds are processed and utilised, and affects their availability and effectiveness to exert a physiological effect.
Although there is encouraging evidence suggesting the beneficial effects of phytogenic compounds on calf health and development, it is important to exercise caution when introducing plant-derived phytogenics into the diet of neonatal calves. This is because limited information is available on the safety and toxicity of these compounds in young calves. Wieland et al. (2015) observed that feeding newborn calves dietary chestnut extract at a dosage of 0.02% of their bodyweight for 5 days led to the development of acute hepatic injury, as evidenced by the increased activity of aspartate aminotransferase and glutamate dehydrogenase. Glutamate dehydrogenase is an enzyme primarily found in the liver, and its activity is often used as a biomarker to assess liver function in young and adult ruminants (Hunter et al. 2013). In cases of acute liver injury, glutamate dehydrogenase is released into the bloodstream. The high tannin content in the chestnut extract is believed to be the underlying cause of the observed hepatotoxic effect in newborn calves. Contrary to adult ruminants, the pre-ruminant calf has an underdeveloped rumen incapable of effective tannin biotransformation. The consumption of phytochemicals at pharmacological concentrations has been documented to have toxic effects in monogastric species. These effects include gastrointestinal and hepatic toxicity, DNA damage and leukemogenicity induction (Lambert et al. 2007).
Sheep
Historically, heat stress management in sheep has received less attention compared with other livestock species, primarily due to their relatively minor contribution to global milk production, the lower emphasis on selective breeding for productivity and their inherent adaptability to hot climates compared with larger ruminant species. Nevertheless, the combination of physiological and behavioural alterations induced by high temperatures poses a challenge in maintaining the productivity and health of small ruminants (Joy et al. 2020). Table 3 presents a literature summary focused on the effects of incorporating phytogenic compounds into the diet of sheep during periods of heat stress.
Animal information | Mean THI or temperature | Plant/antioxidant | Major bioactive compound | Dosage | Duration | Major outcomes | References | |
---|---|---|---|---|---|---|---|---|
4-month-old Awassi lambs | MT = 35.5°C | Naringin | Naringin | 7 or 14 g/week/head A | 60 days |
| Alhidary and Abdelrahman (2016) | |
3-month-old Bamei lambs | MT = 29°C | Tomato extract | Lycopene | 0.05, 0.1 or 0.2 g/kg intake | 120 days |
| Jiang et al. (2015) | |
3-month-old Ujumqin lambs | 29.3 | Chestnut tannin | Tannin | 5 or 10 g/kg DM | 56 days |
| Liu et al. (2016) | |
3-month-old Han × Inner Mongolia lamb | MT = 29.2°C | Herbal blend (4 herbs) | Magnolol, emodin, baicalin | 5, 10, or 15 g/kg DM | 35 days |
| Su et al. (2024) | |
4–5-month-old Dalagh × Romanov lambs | 27.3 | Curcumin nano-micelle | Curcumin | 0.02, 0.04 or 0.08 g/day | 37 days |
| Bokharaeian et al. (2023) | |
4-month-old Katahdin × Dorper lambs | 30.4 | Ferulic acid | Ferulic acid | 0.25 g/kg DM | 55 days |
| Valadez-García et al. (2021) | |
11–12-month-old Afshari × Chaal lambs | 25.9 | A blend of rosemary leaf, clove buds, cinnamon bark and turmeric root | Carnosic acid and carnosol, eugenol cinnamaldehyde and curcumin | 10 or 20 g/kg DM | 48 days |
| Hashemzadeh et al. (2022) | |
Pelibuey × Katahdin ewes | MT = 31.3°C | Macleaya cordata extract B | Protopine and quaternary benzophenanthridine alkaloids | 0.5 g/head/day | 70 days |
| Estrada-Angulo et al. (2016) | |
4-month-old Katahdin × Dorper ewes | 30.4 | Ferulic acid | Ferulic acid | 0.25 g/kg DM | 40 days |
| Nicolás-López et al. (2023) |
THI in sheep was calculated according to Marai et al. (2007). Moderate heat stress: 22.2 ≤ THI ≤ 23.3; severe heat stress: 23.3 ≤ THI ≤ 25.6; extremely severe heat stress: THI ≥ 25.6.
THI, temperature-humidity index; MT, mean temperature; FE, feed efficiency.
A phytogenic blend of cinnamon, rosemary, clove bud and turmeric was included in the diet of heat-stressed lambs for 48 days at a dosage of 20 g/kg diet (Hashemzadeh et al. 2022). The results suggested increased blood and liver antioxidant status that accelerated growth. Experiments involving dietary antioxidants conducted using sheep under heat stress demonstrated that lycopene carotenoid improved feed intake, and tended to improve the growth and muscular development of Bamei lambs (Jiang et al. 2015). The flavonoid naringin administered orally at 7 or 14 g/week was evaluated in heat-stressed Awassi lambs. Feeding at 7 g/week increased growth and feed efficiency, but naringin administered at 14 g/week resulted in greater improvement in blood antioxidant status, as indicated by a higher concentration of glutathione peroxidase (Alhidary and Abdelrahman 2016). Chestnut tannins administered at 5 or 10 g/day in the diet of heat-stressed Ujumqin lambs versus those kept under thermoneutral conditions were associated with positive effects on feedlot performance, meat quality and antioxidant status, as indicated by increased superoxide dismutase and glutathione peroxidase activity (Liu et al. 2016). Exposure to heat stress may result in the depletion of antioxidant enzymes, including superoxide dismutase, glutathione peroxidase and glutathione, resulting in heat stress-induced cytotoxicity and cellular apoptosis (Tang et al. 2023). Promising results were observed in heat-stressed dairy sheep consuming a diet containing 20 g/kg grape residue flour (Alba et al. 2019). Grape residue flour, containing significant amounts of quercetin and resveratrol as active components, modulated anti-inflammatory responses. This dietary supplementation positively impacted milk quality, and reduced lipid peroxidation and somatic cell count, an indication of the healthy functioning of the mammary gland, which was further reinforced by a decrease in the number of globulins and lymphocytes.
Previous studies on heat-stressed sheep have shown promising results, with higher-than-normal dietary levels of antioxidants, such as vitamin E and selenium. These outcomes included a decrease in respiration rate and consistent feed intake (Chauhan et al. 2014b), modulation of the expression of inflammatory genes and heat shock proteins in skeletal muscle, resulting in lower rectal temperature (Chauhan et al. 2014a), and increased carcass weight and improved meat quality, as indicated by less lipid oxidation (Chauhan et al. 2020).
Challenges in application
Incorporating phytogenic compounds as feed additives in animal diets may present some obstacles. These include the risk for toxicity, undesirable taste or odour, which can negatively impact the organoleptic properties of the diet, and concerns regarding the stability of these compounds during feed processing and storage (Yang et al. 2015). Importantly, there is a need to find ways to protect these bioactive compounds from degradation, prevent undesirable tastes or odours and ensure that they retain their functional properties, even when interacting with other dietary components (Yang et al. 2015). This requires developing robust protection technologies that protect these components from degradation in the rumen and ensure maximum bioavailability at the intestinal level. Encapsulation has emerged as an effective and practical delivery method for phytogenic compounds, enabling the precise delivery of the appropriate and effective dosage of phytogenic compounds to the small intestine. The coating technologies enhance the cost-effectiveness of phytogenic feed additives (lower doses required), and help prevent excessive supplementation of phytogenics in young animals, which may result in potential toxicity (Yang et al. 2015; Reddy et al. 2020). The presence of specific active components in aromatic plants and essential oils, which may have an unpleasant odour or smell, can cause palatability concerns. This is especially critical for heat-stressed animals, as heat stress usually lowers their feed intake.
In dairy cows, increasing oregano leaf supplementation from 250 to 750 g/cow/day resulted in a linear decrease in DM intake by 6% (Hristov et al. 2013). This reduction may be attributed to carvacrol, the primary constituent of oregano, known for its distinct pungent odour that is likely unpleasant to ruminants. However, commercially available carvacrol-based phytogenic feed supplements have not typically shown a negative impact on feed intake in monogastric species (e.g. in piglets (Wei et al. 2017)). An encapsulated blend of carvacrol, cinnamaldehyde and thymol, added to the diet of post-weaned calves at 2.7 mg/kg bodyweight, resulted in comparable feed intake and growth to a control diet containing ionophore and non-ionophore antibiotics (Molosse et al. 2022).
Conclusions
Although the effects of feeding phytogenic compounds to both dairy cows and growing ruminant animals have been extensively studied, their effectiveness under heat stress, especially in preweaning calves, remains relatively less explored. The available data in lactating cows suggested that certain phytogenic compounds can potentially increase DM intake and improve milk production during heat stress, as compared with non-supplemented animals. Some studies have also demonstrated the positive effect of phytogenics on immune function and antioxidant status. Similarly, the data on growing ruminant animals (calves and sheep) suggest that feeding the phytogenics may promote feed intake and improve growth (and feed efficiency). However, the consistency of these positive effects varied across studies, with some reporting limited or no impact. Further investigation is needed under different heat stress severity and duration, and a larger number of animals to validate and build upon these emerging findings.
Data availability
The data that support this study are available in the article and accompanying online supplementary material.
Conflicts of interest
F. Dunshea is an Associate Editor for Animal Production Science. But did not at any stage have editor-level access to this manuscript during peer review. The authors have no further conflicts of interest to declare.
Declaration of funding
Farhad Ahmadi is a recipient of the McKenzie Postdoctoral Fellowship funded by The University of Melbourne, Australia, Grant No. UoM-23/26.
Author contributions
F.A.: Funding acquisition, conceptualization, project management and writing – original draft; H.A.R.S.: conceptualization and writing – review and editing; F.R.D.: conceptualization, supervision and writing – review and editing. All authors have read and agreed to the published version of the manuscript.
References
Abdel-Moneim A-ME, Shehata AM, Khidr RE, Paswan VK, Ibrahim NS, El-Ghoul AA, Aldhumri SA, Gabr SA, Mesalam NM, Elbaz AM, Elsayed MA, Wakwak MM, Ebeid TA (2021) Nutritional manipulation to combat heat stress in poultry – a comprehensive review. Journal of Thermal Biology 98, 102915.
| Crossref | Google Scholar | PubMed |
Abeyta MA, Al-Qaisi M, Horst EA, Mayorga EJ, Rodriguez-Jimenez S, Goetz BM, Carta S, Tucker H, Baumgard LH (2023) Effects of dietary antioxidant supplementation on metabolism and inflammatory biomarkers in heat-stressed dairy cows. Journal of Dairy Science 106(2), 1441-1452.
| Crossref | Google Scholar | PubMed |
Abuelo A, Hernández J, Benedito JL, Castillo C (2019) Redox biology in transition periods of dairy cattle: role in the health of periparturient and neonatal animals. Antioxidants 8(1), 20.
| Crossref | Google Scholar | PubMed |
Abulaiti A, Ahmed Z, Naseer Z, El-Qaliouby HS, Iqbal MF, Hua GH, Yang LG (2021) Effect of capsaicin supplementation on lactational and reproductive performance of Holstein cows during summer. Animal Production Science 61(13), 1321-1328.
| Crossref | Google Scholar |
Abulaiti A, Ahsan U, Naseer Z, Ahmed Z, Liu W, Ruan C, Pang X, Wang S (2024) Effect of dietary Chinese herbal preparation on dry matter intake, milk yield and milk composition, serum biochemistry, hematological profile, and reproductive efficiency of Holstein dairy cows in early postpartum period. Frontiers in Veterinary Science 11, 1434548.
| Crossref | Google Scholar | PubMed |
Adaszek Ł, Gadomska D, Mazurek Ł, Łyp P, Madany J, Winiarczyk S (2019) Properties of capsaicin and its utility in veterinary and human medicine. Research in Veterinary Science 123, 14-19.
| Crossref | Google Scholar | PubMed |
Ahmadi F, Suleria HAR, Tunkala B, Prathap P, Chauhan SS, Dunshea FR (2023) Potential of plant-derived bioactives and polyphenols to abate enteric methane emission and heat stress in ruminants. In ‘Proceedings of Cornell Nutrition Conference’, Cornell University, New York. pp. 31–42. (Cornell University)
Alba DF, Campigotto G, Cazarotto CJ, dos Santos DS, Gebert RR, Reis JH, Souza CF, Baldissera MD, Gindri AL, Kempka AP, Palmer EA, Vedovatto M, Da Silva AS (2019) Use of grape residue flour in lactating dairy sheep in heat stress: Effects on health, milk production and quality. Journal of Thermal Biology 82, 197-205.
| Crossref | Google Scholar | PubMed |
Alhidary IA, Abdelrahman MM (2016) Effects of naringin supplementation on productive performance, antioxidant status and immune response in heat-stressed lambs. Small Ruminant Research 138, 31-36.
| Crossref | Google Scholar |
An Z, Zhang X, Gao S, Zhou D, Riaz U, Abdelrahman M, Hua G, Yang L (2022) Effects of Capsicum Oleoresin supplementation on lactation performance, plasma metabolites, and nutrient digestibility of heat stressed dairy cow. Animals 12(6), 797.
| Crossref | Google Scholar | PubMed |
An Z, Zhao J, Zhang X, Gao S, Chen C, Niu K, Nie P, Yao Z, Wei K, Riaz U (2023) Effects of Capsicum oleoresin inclusion on rumen fermentation and lactation performance in Buffaloes (Bubalus bubalis) during summer: in vitro and in vivo studies. Fermentation 9(3), 232.
| Crossref | Google Scholar |
Archer SC, Mc Coy F, Wapenaar W, Green MJ (2013) Association of season and herd size with somatic cell count for cows in Irish, English, and Welsh dairy herds. The Veterinary Journal 196(3), 515-521.
| Crossref | Google Scholar | PubMed |
Bagheri Varzaneh M (2022) Effects of a commercial blend of phytogenic compounds and prebiotic on the performance of mid-lactation dairy cows exposed to heat-stress. Iranian Journal of Applied Animal Science 12(3), 489-495.
| Google Scholar |
Baumgard LH, Rhoads RP (2013) Effects of heat stress on postabsorptive metabolism and energetics. Annual Review of Animal Biosciences 1(1), 311-337.
| Crossref | Google Scholar |
Becker CA, Collier RJ, Stone AE (2020) Invited review: Physiological and behavioral effects of heat stress in dairy cows. Journal of Dairy Science 103(8), 6751-6770.
| Crossref | Google Scholar | PubMed |
Berger LM, Blank R, Zorn F, Wein S, Metges CC, Wolffram S (2015) Ruminal degradation of quercetin and its influence on fermentation in ruminants. Journal of Dairy Science 98(8), 5688-5698.
| Crossref | Google Scholar | PubMed |
Bernabucci U, Lacetera N, Baumgard LH, Rhoads RP, Ronchi B, Nardone A (2010) Metabolic and hormonal acclimation to heat stress in domesticated ruminants. Animal 4(7), 1167-1183.
| Crossref | Google Scholar | PubMed |
Bokharaeian M, Toghdory A, Ghoorchi T (2023) Effects of dietary curcumin nano-micelles on growth performance, blood metabolites, antioxidant status, immune and physiological responses of fattening lambs under heat-stress conditions. Journal of Thermal Biology 114, 103585.
| Crossref | Google Scholar | PubMed |
Carter HSM, Renaud DL, Steele MA, Fischer-Tlustos AJ, Costa JHC (2021) A narrative review on the unexplored potential of colostrum as a preventative treatment and therapy for diarrhea in neonatal dairy calves. Animals 11(8), 2221.
| Crossref | Google Scholar | PubMed |
Chauhan SS, Celi P, Fahri FT, Leury BJ, Dunshea FR (2014a) Dietary antioxidants at supranutritional doses modulate skeletal muscle heat shock protein and inflammatory gene expression in sheep exposed to heat stress. Journal of Animal Science 92(11), 4897-4908.
| Crossref | Google Scholar | PubMed |
Chauhan SS, Celi P, Leury BJ, Clarke IJ, Dunshea FR (2014b) Dietary antioxidants at supranutritional doses improve oxidative status and reduce the negative effects of heat stress in sheep. Journal of Animal Science 92(8), 3364-3374.
| Crossref | Google Scholar | PubMed |
Chauhan SS, Celi P, Ponnampalam EN, Leury BJ, Liu F, Dunshea FR (2014c) Antioxidant dynamics in the live animal and implications for ruminant health and product (meat/milk) quality: role of vitamin E and selenium. Animal Production Science 54(10), 1525-1536.
| Crossref | Google Scholar |
Chauhan SS, Dunshea FR, Plozza TE, Hopkins DL, Ponnampalam EN (2020) The impact of antioxidant supplementation and heat stress on carcass characteristics, muscle nutritional profile and functionality of lamb meat. Animals 10(8), 1286.
| Crossref | Google Scholar | PubMed |
Chauhan SS, Rashamol VP, Bagath M, Sejian V, Dunshea FR (2021) Impacts of heat stress on immune responses and oxidative stress in farm animals and nutritional strategies for amelioration. International Journal of Biometeorology 65, 1231-1244.
| Crossref | Google Scholar | PubMed |
Cheng J-B, Fan C-Y, Sun X-Z, Wang J-Q, Zheng N, Zhang X-K, Qin J-J, Wang X-M (2018) Effects of Bupleurum extract on blood metabolism, antioxidant status and immune function in heat-stressed dairy cows. Journal of Integrative Agriculture 17(3), 657-663.
| Crossref | Google Scholar |
Costa A, Sneddon NW, Goi A, Visentin G, Mammi LME, Savarino EV, Zingone F, Formigoni A, Penasa M, De Marchi M (2023) Invited review: Bovine colostrum, a promising ingredient for humans and animals—Properties, processing technologies, and uses. Journal of Dairy Science 106(8), 5197-5217.
| Crossref | Google Scholar | PubMed |
da Silva RB, Pereira MN, de Araujo RC, de Rezende Silva W, Pereira RAN (2020) A blend of essential oils improved feed efficiency and affected ruminal and systemic variables of dairy cows. Translational Animal Science 4(1), 182-193.
| Crossref | Google Scholar | PubMed |
Daddam JR, Daniel D, Kra G, Pelech I, Portnick Y, Moallem U, Lavon Y, Zachut M (2023) Plant polyphenol extract supplementation affects performance, welfare, and the Nrf2-oxidative stress response in adipose tissue of heat-stressed dairy cows. Journal of Dairy Science 106(12), 9807-9821.
| Crossref | Google Scholar | PubMed |
Dado-Senn B, Vega Acosta L, Torres Rivera M, Field SL, Marrero MG, Davidson BD, Tao S, Fabris TF, Ortiz-Colón GE, Dahl G, Laporta J (2020) Pre- and postnatal heat stress abatement affects dairy calf thermoregulation and performance. Journal of Dairy Science 103(5), 4822-4837.
| Crossref | Google Scholar | PubMed |
De Paris M, Stivanin S, Klein C, Vizzotto E, Passos L, Angelo I, Zanela M, Stone V, Matté C, Heisler G (2020) Calves fed with milk from cows receiving plant extracts improved redox status. Livestock Science 242, 104272.
| Crossref | Google Scholar |
Devi P, Singh M, Mehla R, Ajithakumar HM (2021a) Effect of Chlorophytum borivilianum supplementation on milk production, composition and fatty acid profile in crossbred cows during hot-humid season. Tropical Animal Health and Production 53(2), 300.
| Crossref | Google Scholar | PubMed |
Devi P, Singh M, Somagond YM, Aggarwal A (2021b) Alleviation of heat stress by Chlorophytum borivilianum: impact on stress markers, antioxidant, and immune status in crossbred cows. Tropical Animal Health and Production 53(3), 351.
| Crossref | Google Scholar | PubMed |
Dehority BA (2002) Gastrointestinal tracts of herbivores, particularly the ruminant: anatomy, physiology and microbial digestion of plants. Journal of Applied Animal Research 21(2), 145-160.
| Crossref | Google Scholar |
Dunshea FR, Leury BJ, Fahri F, DiGiacomo K, Hung A, Chauhan S, Clarke IJ, Collier R, Little S, Baumgard L (2013) Amelioration of thermal stress impacts in dairy cows. Animal Production Science 53(9), 965-975.
| Crossref | Google Scholar |
Dunshea FR, Gonzalez-Rivas PA, Hung AT, DiGiacomo K, Chauhan SS, Leury BJ, Celi PP, Ponnampalam EN, Cottrell JJ (2017) Nutritional strategies to alleviate heat stress in sheep. In ‘Sheep production adapting to climate change’. (Eds V Sejian, R Bhatta, J Gaughan, P Malik, S Naqvi, R Lal) pp. 371–388. (Springer: Singapore) 10.1007/978-981-10-4714-5_18
Dunshea FR, Leury BJ, DiGiacomo K, Cottrell JJ, Chauhan SS (2022) Nutritional amelioration of thermal stress impacts in dairy cows. In ‘Climate change and livestock production: recent advances and future perspectives’. (Eds V Sejian, SS Chauhan, C Devaraj, PK Malik, R Bhatta) pp. 141–150. (Springer: Singapore). 10.1007/978-981-16-9836-1_12
Estrada-Angulo A, Aguilar-Hernández A, Osuna-Pérez M, Núñez-Benítez VH, Castro-Pérez BI, Silva-Hidalgo G, Contreras-Pérez G, Barreras A, Plascencia A, Zinn RA (2016) Influence of quaternary benzophenantridine and protopine alkaloids on growth performance, dietary energy, carcass traits, visceral mass, and rumen health in finishing ewes under conditions of severe temperature-humidity index. Asian-Australasian Journal of Animal Sciences 29(5), 652.
| Crossref | Google Scholar | PubMed |
Fontoura ABP, Javaid A, Sáinz de la Maza-Escolà V, Salandy NS, Fubini SL, Grilli E, McFadden JW (2022) Heat stress develops with increased total-tract gut permeability, and dietary organic acid and pure botanical supplementation partly restores lactation performance in Holstein dairy cows. Journal of Dairy Science 105(9), 7842-7860.
| Crossref | Google Scholar | PubMed |
Fontoura ABP, Sáinz de la Maza-Escolà VS, Richards AT, Tate BN, Van Amburgh ME, Grilli E, McFadden JW (2023) Effects of dietary organic acid and pure botanical supplementation on growth performance and circulating measures of metabolic health in Holstein calves challenged by heat stress. Journal of Dairy Science 106(4), 2904-2918.
| Crossref | Google Scholar | PubMed |
Franz C, Baser KHC, Windisch W (2010) Essential oils and aromatic plants in animal feeding – a European perspective. A review. Flavour and Fragrance Journal 25(5), 327-340.
| Crossref | Google Scholar |
Gaál T, Ribiczeyné-Szabó P, Stadler K, Jakus J, Reiczigel J, Kövér P, Mezes M, Sümeghy L (2006) Free radicals, lipid peroxidation and the antioxidant system in the blood of cows and newborn calves around calving. Comparative Biochemistry and Physiology Part B: Biochemistry and Molecular Biology 143(4), 391-396.
| Crossref | Google Scholar |
Gaughan JB, Sejian V, Mader TL, Dunshea FR (2019) Adaptation strategies: ruminants. Animal Frontiers 9(1), 47-53.
| Crossref | Google Scholar | PubMed |
Gohlke A, Ingelmann C, Nürnberg G, Weitzel J, Hammon H, Görs S, Starke A, Wolffram S, Metges C (2013) Influence of 4-week intraduodenal supplementation of quercetin on performance, glucose metabolism, and mRNA abundance of genes related to glucose metabolism and antioxidative status in dairy cows. Journal of Dairy Science 96(11), 6986-7000.
| Crossref | Google Scholar | PubMed |
Gonzalez-Rivas PA, Chauhan SS, Ha M, Fegan N, Dunshea FR, Warner RD (2020) Effects of heat stress on animal physiology, metabolism, and meat quality: a review. Meat Science 162, 108025.
| Crossref | Google Scholar | PubMed |
Guo Z, Gao S, Ouyang J, Ma L, Bu D (2021) Impacts of heat stress-induced oxidative stress on the milk protein biosynthesis of dairy cows. Animals 11(3), 726.
| Crossref | Google Scholar | PubMed |
Gupta S, Sharma A, Joy A, Dunshea FR, Chauhan SS (2023) The impact of heat stress on immune status of dairy cattle and strategies to ameliorate the negative effects. Animals 13(1), 107.
| Crossref | Google Scholar |
Hashemzadeh-Cigari F, Ghorbani GR, Khorvash M, Riasi A, Taghizadeh A, Zebeli Q (2015) Supplementation of herbal plants differently modulated metabolic profile, insulin sensitivity, and oxidative stress in transition dairy cows fed various extruded oil seeds. Preventive Veterinary Medicine 118(1), 45-55.
| Crossref | Google Scholar | PubMed |
Hashemzadeh F, Rafeie F, Hadipour A, Rezadoust MH (2022) Supplementing a phytogenic-rich herbal mixture to heat-stressed lambs: growth performance, carcass yield, and muscle and liver antioxidant status. Small Ruminant Research 206, 106596.
| Crossref | Google Scholar |
Havlin JM, Robinson PH (2015) Intake, milk production and heat stress of dairy cows fed a citrus extract during summer heat. Animal Feed Science and Technology 208, 23-32.
| Crossref | Google Scholar |
Hristov AN, Lee C, Cassidy T, Heyler K, Tekippe JA, Varga GA, Corl B, Brandt RC (2013) Effect of Origanum vulgare L. leaves on rumen fermentation, production, and milk fatty acid composition in lactating dairy cows. Journal of Dairy Science 96(2), 1189-1202.
| Crossref | Google Scholar | PubMed |
Hunter A, Suttle N, Martineau H, Spence M, Thomson J, Macrae A, Brown S (2013) Mortality, hepatopathy and liver copper concentrations in artificially reared Jersey calves before and after reductions in copper supplementation. Veterinary Record 172(2), 46.
| Crossref | Google Scholar | PubMed |
Islam MN, Rauf A, Fahad FI, Emran TB, Mitra S, Olatunde A, Shariati MA, Rebezov M, Rengasamy KRR, Mubarak MS (2022) Superoxide dismutase: an updated review on its health benefits and industrial applications. Critical Reviews in Food Science and Nutrition 62(26), 7282-7300.
| Crossref | Google Scholar | PubMed |
Jiang H, Wang Z, Ma Y, Qu Y, Lu X, Guo H, Luo H (2015) Effect of dietary lycopene supplementation on growth performance, meat quality, fatty acid profile and meat lipid oxidation in lambs in summer conditions. Small Ruminant Research 131, 99-106.
| Crossref | Google Scholar |
Joy A, Dunshea FR, Leury BJ, Clarke IJ, DiGiacomo K, Chauhan SS (2020) Resilience of small ruminants to climate change and increased environmental temperature: A review. Animals 10(5), 867.
| Crossref | Google Scholar | PubMed |
Khorsandi S, Riasi A, Khorvash M, Hashemzadeh F (2019) Nutrients digestibility, metabolic parameters and milk production in postpartum Holstein cows fed pomegranate (Punica granatum L.) by-products silage under heat stress condition. Animal Feed Science and Technology 255, 114213.
| Crossref | Google Scholar |
Koch F, Thom U, Albrecht E, Weikard R, Nolte W, Kuhla B, Kuehn C (2019) Heat stress directly impairs gut integrity and recruits distinct immune cell populations into the bovine intestine. Proceedings of the National Academy of Sciences 116(21), 10333-10338.
| Crossref | Google Scholar |
Kra G, Daddam JR, Gabay H, Yosefi S, Zachut M (2021) Antioxidant resveratrol increases lipolytic and reduces lipogenic gene expression under in vitro heat stress conditions in dedifferentiated adipocyte-derived progeny cells from dairy cows. Antioxidants 10(6), 905.
| Crossref | Google Scholar | PubMed |
Kvidera SK, Horst EA, Abuajamieh M, Mayorga EJ, Sanz Fernandez MV, Baumgard LH (2017) Glucose requirements of an activated immune system in lactating Holstein cows. Journal of Dairy Science 100(3), 2360-2374.
| Crossref | Google Scholar | PubMed |
Lambert JD, Sang S, Yang CS (2007) Possible controversy over dietary polyphenols: benefits vs risks. Chemical Research in Toxicology 20(4), 583-585.
| Crossref | Google Scholar | PubMed |
Lee J-S, Kang S, Kim M-J, Han S-G, Lee H-G (2020) Dietary supplementation with combined extracts from garlic (Allium sativum), brown seaweed (Undaria pinnatifida), and pinecone (Pinus koraiensis) improves milk production in Holstein cows under heat stress conditions. Asian-Australasian Journal of Animal Sciences 33(1), 111.
| Crossref | Google Scholar | PubMed |
Lejonklev J, Løkke M, Larsen M, Mortensen G, Petersen M, Weisbjerg M (2013) Transfer of terpenes from essential oils into cow milk. Journal of Dairy Science 96(7), 4235-4241.
| Crossref | Google Scholar | PubMed |
Lejonklev J, Kidmose U, Jensen S, Petersen MA, Helwing ALF, Mortensen G, Weisbjerg MR, Larsen MK (2016) Effect of oregano and caraway essential oils on the production and flavor of cow milk. Journal of Dairy Science 99(10), 7898-7903.
| Crossref | Google Scholar | PubMed |
Lemal P, May K, König S, Schroyen M, Gengler N (2023) Invited review: From heat stress to disease—Immune response and candidate genes involved in cattle thermotolerance. Journal of Dairy Science 106(7), 4471-4488.
| Crossref | Google Scholar | PubMed |
Li M, Hassan F, Tang Z, Peng L, Liang X, Li L, Peng K, Xie F, Yang C (2020) Mulberry leaf flavonoids improve milk production, antioxidant, and metabolic status of water buffaloes. Frontiers in Veterinary Science 7, 599.
| Crossref | Google Scholar | PubMed |
Li Y, Shang H, Zhao X, Qu M, Peng T, Guo B, Hu Y, Song X (2021) Radix Puerarin extract (Puerarin) could improve meat quality of heat-stressed beef cattle through changing muscle antioxidant ability and fiber characteristics. Frontiers in Veterinary Science 7, 615086.
| Crossref | Google Scholar | PubMed |
Liu H, Li K, Mingbin L, Zhao J, Xiong B (2016) Effects of chestnut tannins on the meat quality, welfare, and antioxidant status of heat-stressed lambs. Meat Science 116, 236-242.
| Crossref | Google Scholar | PubMed |
Liu W, Gao H, He J, Yu A, Sun C, Xie Y, Yao H, Wang H, Duan Y, Hu J, Tang D, Ran T, Lei Z (2024) Effects of dietary Allium mongolicum Regel powder supplementation on the growth performance, meat quality, antioxidant capacity and muscle fibre characteristics of fattening Angus calves under heat stress conditions. Food Chemistry 453, 139539.
| Crossref | Google Scholar | PubMed |
López E, Mellado M, Martínez AM, Véliz FG, García JE, de Santiago A, Carrillo E (2018) Stress-related hormonal alterations, growth and pelleted starter intake in pre-weaning Holstein calves in response to thermal stress. International Journal of Biometeorology 62(4), 493-500.
| Crossref | Google Scholar | PubMed |
Ma FT, Shan Q, Jin YH, Gao D, Li HY, Chang MN, Sun P (2020) Effect of Lonicera japonica extract on lactation performance, antioxidant status, and endocrine and immune function in heat-stressed mid-lactation dairy cows. Journal of Dairy Science 103(11), 10074-10082.
| Crossref | Google Scholar | PubMed |
Maciej J, Schäff CT, Kanitz E, Tuchscherer A, Bruckmaier RM, Wolffram S, Hammon HM (2016) Effects of oral flavonoid supplementation on the metabolic and antioxidative status of newborn dairy calves. Journal of Dairy Science 99(1), 805-811.
| Crossref | Google Scholar | PubMed |
Majlesi A, Yasini SP, Azimpour S, Mottaghian P (2021) Evaluation of oxidative and antioxidant status in dairy calves before and after weaning. Bulgarian Journal of Veterinary Medicine 24(2), 184-190.
| Crossref | Google Scholar |
Marai IFM, El-Darawany AA, Fadiel A, Abdel-Hafez MAM (2007) Physiological traits as affected by heat stress in sheep—a review. Small Ruminant Research 71(1–3), 1-12.
| Crossref | Google Scholar |
Martemucci G, Costagliola C, Mariano M, D’andrea L, Napolitano P, D’Alessandro AG (2022) Free radical properties, source and targets, antioxidant consumption and health. Oxygen 2(2), 48-78.
| Crossref | Google Scholar |
Matsui T (2012) Vitamin C nutrition in cattle. Asian-Australasian Journal of Animal Sciences 25(5), 597-605.
| Crossref | Google Scholar | PubMed |
Molinari PCC, Davidson BD, Laporta J, Dahl GE, Sheldon IM, Bromfield JJ (2023) Prepartum heat stress in dairy cows increases postpartum inflammatory responses in blood of lactating dairy cows. Journal of Dairy Science 106(2), 1464-1474.
| Crossref | Google Scholar | PubMed |
Molosse VL, Deolindo GL, Glombosky P, Pereira WAB, Carvalho RA, Zotti CA, Solivo G, Vedovato M, Fracasso M, Silva AD, Morsch VM, Schafer da Silva A (2022) Curcumin or microencapsulated phytogenic blend to replace ionophore and non-ionophore antibiotics in weaned calves: effects on growth performance and health. Livestock Science 263, 105029.
| Crossref | Google Scholar |
Nicolás-López P, Macías-Cruz U, Avendaño-Reyes L, Valadez-García KM, Mellado M, Meza-Herrera CA, Díaz-Molina R, Castañeda VJ, Vicente-Pérez R, Luna-Palomera C (2023) Ferulic acid supplementation for 40 days in hair ewe lambs experiencing seasonal heat stress: short-term effects on physiological responses, growth, metabolism, and hematological profile. Environmental Science and Pollution Research 30(5), 11562-11571.
| Crossref | Google Scholar | PubMed |
Oh J, Wall EH, Bravo DM, Hristov AN (2017) Host-mediated effects of phytonutrients in ruminants: a review. Journal of Dairy Science 100(7), 5974-5983.
| Crossref | Google Scholar | PubMed |
Olagaray KE, Bradford BJ (2019) Plant flavonoids to improve productivity of ruminants – A review. Animal Feed Science and Technology 251, 21-36.
| Crossref | Google Scholar |
Pan L, Bu DP, Wang JQ, Cheng JB, Sun XZ, Zhou LY, Qin JJ, Zhang XK, Yuan YM (2014) Effects of Radix Bupleuri extract supplementation on lactation performance and rumen fermentation in heat-stressed lactating Holstein cows. Animal Feed Science and Technology 187, 1-8.
| Crossref | Google Scholar |
Papatsiros VG, Katsogiannou EG, Papakonstantinou GI, Michel A, Petrotos K, Athanasiou LV (2022) Effects of phenolic phytogenic feed additives on certain oxidative damage biomarkers and the performance of primiparous sows exposed to heat stress under field conditions. Antioxidants 11(3), 593.
| Crossref | Google Scholar | PubMed |
Piantoni P, VandeHaar MJ (2022) Symposium review: The impact of absorbed nutrients on energy partitioning throughout lactation. Journal of Dairy Science 106(3), 2167-2180.
| Crossref | Google Scholar | PubMed |
Przybylska J, Albera E, Kankofer M (2007) Antioxidants in bovine colostrum. Reproduction in Domestic Animals 42(4), 402-409.
| Crossref | Google Scholar | PubMed |
Rainard P, Foucras G, Boichard D, Rupp R (2018) Invited review: Low milk somatic cell count and susceptibility to mastitis. Journal of Dairy Science 101(8), 6703-6714.
| Crossref | Google Scholar | PubMed |
Rebez EB, Sejian V, Silpa MV, Dunshea FR (2023) Heat stress and histopathological changes of vital organs: a novel approach to assess climate resilience in farm animals. Sustainability 15(2), 1242.
| Crossref | Google Scholar |
Reddy PRK, Elghandour M, Salem A, Yasaswini D, Reddy PPR, Reddy AN, Hyder I (2020) Plant secondary metabolites as feed additives in calves for antimicrobial stewardship. Animal Feed Science and Technology 264, 114469.
| Crossref | Google Scholar |
Reyes-Camacho D, Vinyeta E, Pérez JF, Aumiller T, Criado L, Palade LM, Taranu I, Folch JM, Calvo MA, Van der Klis JD, Solà-Oriol D (2020) Phytogenic actives supplemented in hyperprolific sows: effects on maternal transfer of phytogenic compounds, colostrum and milk features, performance and antioxidant status of sows and their offspring, and piglet intestinal gene expression. Journal of Animal Science 98(1), skz390.
| Crossref | Google Scholar |
Rochfort S, Parker AJ, Dunshea FR (2008) Plant bioactives for ruminant health and productivity. Phytochemistry 69(2), 299-322.
| Crossref | Google Scholar | PubMed |
Roland L, Drillich M, Klein-Jöbstl D, Iwersen M (2016) Invited review: Influence of climatic conditions on the development, performance, and health of calves. Journal of Dairy Science 99(4), 2438-2452.
| Crossref | Google Scholar | PubMed |
Saleh AA, Soliman MM, Yousef MF, Eweedah NM, El-Sawy HB, Shukry M, Wadaan MAM, Kim IH, Cho S, Eltahan HM (2023) Effects of herbal supplements on milk production quality and specific blood parameters in heat-stressed early lactating cows. Frontiers in Veterinary Science 10, 1180539.
| Crossref | Google Scholar | PubMed |
Sejian V, Bhatta R, Gaughan JB, Dunshea FR, Lacetera N (2018) Review: Adaptation of animals to heat stress. Animal 12(s2), s431-s444.
| Crossref | Google Scholar | PubMed |
Shan C-H, Guo J, Sun X, Li N, Yang X, Gao Y, Qiu D, Li X, Wang Y, Feng M, Wang C, Zhao JJ (2018) Effects of fermented Chinese herbal medicines on milk performance and immune function in late-lactation cows under heat stress conditions. Journal of Animal Science 96(10), 4444-4457.
| Crossref | Google Scholar | PubMed |
Soberon F, Raffrenato E, Everett RW, Van Amburgh ME (2012a) Preweaning milk replacer intake and effects on long-term productivity of dairy calves. Journal of Dairy Science 95(2), 783-793.
| Crossref | Google Scholar | PubMed |
Soberon MA, Liu RH, Cherney DJR (2012b) Antioxidant activity of calf milk replacers. Journal of Dairy Science 95(5), 2703-2706.
| Crossref | Google Scholar | PubMed |
Song X, Luo J, Fu D, Zhao X, Bunlue K, Xu Z, Qu M (2014) Traditional Chinese medicine prescriptions enhance growth performance of heat stressed beef cattle by relieving heat stress responses and increasing apparent nutrient digestibility. Asian-Australasian Journal of Animal Sciences 27(10), 1513-1520.
| Crossref | Google Scholar | PubMed |
Su D, Song L, Dong Q, Zhang A, Zhang L, Wang Y, Feng M, Li X, Li F, Sun X, Gao Y (2024) Effects of herbal formula on growth performance, apparent digestibility, antioxidant capacity, and rumen microbiome in fattening lambs under heat stress. Environmental Science and Pollution Research 31(39), 51364-51380.
| Crossref | Google Scholar | PubMed |
Szolcsányi J (2015) Effect of capsaicin on thermoregulation: an update with new aspects. Temperature 2(2), 277-296.
| Crossref | Google Scholar |
Tang Z, Yang Y, Wu Z, Ji Y (2023) Heat stress-induced intestinal barrier impairment: current insights into the aspects of oxidative stress and endoplasmic reticulum stress. Journal of Agricultural and Food Chemistry 71(14), 5438-5449.
| Crossref | Google Scholar | PubMed |
Tao S, Monteiro APA, Thompson IM, Hayen MJ, Dahl GE (2012) Effect of late-gestation maternal heat stress on growth and immune function of dairy calves. Journal of Dairy Science 95(12), 7128-7136.
| Crossref | Google Scholar | PubMed |
Tao S, Dahl GE, Laporta J, Bernard JK, Orellana Rivas RM, Marins TN (2019) Physiology symposium: effects of heat stress during late gestation on the dam and its calf. Journal of Animal Science 97(5), 2245-2257.
| Crossref | Google Scholar | PubMed |
Thornton P, Nelson G, Mayberry D, Herrero M (2022) Impacts of heat stress on global cattle production during the 21st century: a modelling study. The Lancet Planetary Health 6(3), e192-e201.
| Crossref | Google Scholar | PubMed |
Tong Z, He W, Fan X, Guo A (2022) Biological function of plant tannin and its application in animal health. Frontiers in Veterinary Science 8, 803657.
| Crossref | Google Scholar | PubMed |
Tucker CB, Jensen MB, de Passillé AM, Hänninen L, Rushen J (2021) Invited review: Lying time and the welfare of dairy cows. Journal of Dairy Science 104(1), 20-46.
| Crossref | Google Scholar | PubMed |
Turek C, Stintzing FC (2013) Stability of essential oils: a review. Comprehensive Reviews in Food Science and Food Safety 12(1), 40-53.
| Crossref | Google Scholar |
Urkmez E, Biricik H (2022a) Grape seed extract supplementation in heat-stressed preweaning dairy calves: I. Effects on antioxidant status, inflammatory response, hematological and physiological parameters. Animal Feed Science and Technology 292, 115421.
| Crossref | Google Scholar |
Urkmez E, Biricik H (2022b) Grape seed extract supplementation in heat-stressed preweaning dairy calves: II. Effects on growth performance, blood metabolites, hormonal responses, and fecal fermentation parameters. Animal Feed Science and Technology 292, 115422.
| Crossref | Google Scholar |
Valadez-García KM, Avendaño-Reyes L, Díaz-Molina R, Mellado M, Meza-Herrera CA, Correa-Calderón A, Macías-Cruz U (2021) Free ferulic acid supplementation of heat-stressed hair ewe lambs: oxidative status, feedlot performance, carcass traits and meat quality. Meat Science 173, 108395.
| Crossref | Google Scholar | PubMed |
Varela AMG, de Lima Junior DM, de Araújo TLAC, de Souza Junior JBF, de Macedo Costa LL, Pereira MWF, Batista NV, de Lima Melo VL, de Oliveira Lima P (2023) The effect of propolis extract on milk production and composition, serum biochemistry, and physiological parameters of heat-stressed dairy cows. Tropical Animal Health and Production 55(4), 244.
| Crossref | Google Scholar | PubMed |
Vittorazzi PC, Jr, Takiya CS, Nunes AT, Chesini RG, Bugoni M, Silva GG, Silva TBP, Dias MSS, Grigoletto NTS, Rennó FP (2022) Feeding encapsulated pepper to dairy cows during the hot season improves performance without affecting core and skin temperature. Journal of Dairy Science 105(12), 9542-9551.
| Crossref | Google Scholar | PubMed |
Wang J, Li J, Wang F, Xiao J, Wang Y, Yang H, Li S, Cao Z (2020) Heat stress on calves and heifers: a review. Journal of Animal Science and Biotechnology 11(1), 79.
| Crossref | Google Scholar |
Wei H-K, Xue H-X, Zhou ZX, Peng J (2017) A carvacrol–thymol blend decreased intestinal oxidative stress and influenced selected microbes without changing the messenger RNA levels of tight junction proteins in jejunal mucosa of weaning piglets. Animal 11(2), 193-201.
| Crossref | Google Scholar | PubMed |
Wickramasinghe HKJP, Stepanchenko N, Oconitrillo MJ, Goetz BM, Abeyta MA, Gorden PJ, Baumgard LH, Appuhamy JADRN (2023) Effects of a phytogenic feed additive on weaned dairy heifer calves subjected to a diurnal heat stress bout. Journal of Dairy Science 106(9), 6114-6127.
| Crossref | Google Scholar | PubMed |
Wieland M, Weber BK, Hafner-Marx A, Sauter-Louis C, Bauer J, Knubben-Schweizer G, Metzner M (2015) A controlled trial on the effect of feeding dietary chestnut extract and glycerol monolaurate on liver function in newborn calves. Journal of Animal Physiology and Animal Nutrition 99(1), 190-200.
| Crossref | Google Scholar | PubMed |
Xie Y, Chen Z, Wang D, Chen G, Sun X, He Q, Luo J, Chen T, Xi Q, Zhang Y (2020) Effects of fermented herbal tea residues on the intestinal microbiota characteristics of Holstein heifers under heat stress. Frontiers in Microbiology 11, 1014.
| Crossref | Google Scholar | PubMed |
Yang C, Chowdhury MA, Hou Y, Gong J (2015) Phytogenic compounds as alternatives to in-feed antibiotics: potentials and challenges in application. Pathogens 4(1), 137-156.
| Crossref | Google Scholar | PubMed |
Yuan B, Yang R, Ma Y, Zhou S, Zhang X, Liu Y (2017) A systematic review of the active saikosaponins and extracts isolated from Radix Bupleuri and their applications. Pharmaceutical Biology 55(1), 620-635.
| Crossref | Google Scholar | PubMed |
Zhao S, Shan C, Wu Z, Feng M, Song L, Wang Y, Gao Y, Guo J, Sun X (2022) Fermented Chinese herbal preparation: impacts on milk production, nutrient digestibility, blood biochemistry, and antioxidant capacity of late-lactation cows under heat stress. Animal Feed Science and Technology 292, 115448.
| Crossref | Google Scholar |