Malnutrition of pregnant beef cows and the impact on passive immunity transfer to calves
L. F. P. Silva

A The University of Queensland, Queensland Alliance for Agriculture and Food Innovation, Centre for Animal Science, Gatton, Qld 4343, Australia.
B School of Environmental and Rural Science, The University of New England, Armidale, NSW, Australia.
Abstract
Nutritional management in rangeland beef cattle systems prioritises optimal body condition scores at calving for improved fertility and reproductive success. However, this focus often overlooks short-term dietary deficiencies before calving, which can lead to adverse outcomes for neonatal calves. This review explores the effects of beef cow malnutrition during the periparturient period on colostrum production, lactation onset, and passive immunity transfer to calves. Additionally, it discusses the long-term impact of such malnutrition on the offspring. By understanding how nutritional interventions affect the transition from gestation to lactation, it becomes possible to enhance calf health and survival in arid tropical environments. Commonly occurring short-term dietary restrictions, particularly protein deficiencies, can disrupt the hormonal equilibrium, resulting in reduced colostrum volume and quality, hindering calf growth and increasing mortality risks. Furthermore, dietary restrictions during this period affect critical physiological processes such as mammary gland blood flow and fetal small-intestine development. The review explores how these constraints influence colostrum production and immunoglobulin absorption by neonatal calves. Additionally, it highlights the significance of addressing other common nutrient deficiencies such as phosphorus and water and investigates the potential benefits of supplementing microbial products to enhance rumen function and protect cows from inflammation. Ultimately, addressing malnutrition during pregnancy is essential to prevent negative impacts on offspring performance, including alterations in carcass composition and muscle marbling. Consequently, cattle producers who aim for superior muscle marbling in the carcass by using costly genetics should give priority to enhancing nutritional programs for late-pregnant cows. In conclusion, a comprehensive understanding of the effects of malnutrition during the periparturient period on colostrum production, passive immunity transfer, and overall calf health is crucial for developing effective nutritional interventions that improve colostrum production, passive immunity transfer, and overall calf health in rangeland beef cattle systems.
Keywords: beef cattle, calf loss, colostrum, maternal nutrition, passive immunity, pregnancy, progesterone, rangeland.
Introduction
During evolution, mammals developed survival mechanisms to adapt to changes in environmental conditions. One such crucial adaptation is the ability to sense the abundance of nutrients and regulate reproduction to minimise herd mortality. Thin cows, with body condition scores (BCS) of <3 (1–5 scale), have lower fertility and wean lighter calves than do those with greater body reserves (D’Occhio et al. 2019). In the dry tropics, especially in northern Australia with one of the most variable climates on the planet (King et al. 2020), the continuous and unpredictable cycle of wet and dry years creates a unique challenge to beef-cattle producers and nutritionists: how to maintain a cost-effective nutritional program to ensure high reproductive efficiency and the survival of healthy calves?
The two fundamental steps to developing a sound nutritional program are knowing the nutrient supply throughout the year and the requirement of the animals. Both are very difficult to predict in extensive rangeland systems with a highly variable climate. The difficulty in predicting nutrient supply is easily understood, because pasture availability and quality will depend on the climate conditions, mostly rainfall. A subtler problem is the definition of nutrient requirements for rangeland beef cows. Serial slaughter studies can determine the nutrient requirements of the growing fetus and monitoring the growth of calves can determine the requirements of lactating cows.
These methods work well in nutritional programs in which a diet will be formulated to supply all nutrients required by the cows, which is usually not the case in rangeland beef. For example, the available literature indicates that pregnant Brahman cows during the last month of gestation require 963 g of crude protein (CP), 70 MJ of metabolisable energy (ME) and 15 g of phosphorus (P) per day (NASEM 2016). However, given that it is almost certainly not cost-effective to supply these amounts in rangeland systems, the important question is as follows: what is the impact on lifetime productivity of supplying less than the calculated requirements? If we cannot answer this question, beef cattle producers will understandably be reluctant to invest in improving the nutritional programs.
The benefits of improved nutrition are most commonly evaluated by the impact on fertility and reproductive performance of cows (D’Occhio et al. 2019). Usually, cows with good BCS around calving (BCS ≥ 3) are able to maintain high fertility, independently of the diet conditions around calving, as long as they can replenish body reserves during the year (Morrison et al. 1999; Carvalho et al. 2022). However, malnutrition during late gestation can potentially affect colostrum secretion and milk delivery to calves, leading to impaired transfer of passive immunity, high mortality rates and reduced calf growth early in life (McGee and Earley 2019). These effects are not well understood.
In contrast to other mammals, such as humans, the cotyledonary placenta of bovine has three maternal and fetal layers, impeding the transfer of maternal immunoglobulin to the fetus in the uterus (Weaver et al. 2000). Therefore, calves are born with agammaglobulinemia (Weaver et al. 2000; Barrington and Parish 2001; Castro et al. 2011) and are largely dependent on the passive immunity in the colostrum to protect them from infectious diseases before their immunity is fully developed (Franklin et al. 2003; Bragg et al. 2020). The current rates of calf mortality in north Australia and the dry tropics, which is >10%, are not acceptable for sustainable livestock systems and better nutritional programs are an important part of the solution (McGowan et al. 2014). Our aim with this review is to discuss the current knowledge on the impact of prepartum malnutrition on the neonatal transfer of passive immunity in beef calves, with the implicit objective to motivate the pursuit of management solutions to improve the nutrition of pregnant cows and the health and growth of calves.
The endocrine shift from gestation to lactation
Before discussing the effects of malnutrition during late pregnancy, it is important to note that this is a period of drastic and rapid changes in the animal’s metabolism and consequently is a time when a cow is more susceptible to nutritional and environmental stressors (Abuelo et al. 2019). The pregnant cow needs not only to go through the metabolic changes necessary to terminate gestation and deliver the fetus but also to prepare the mammary gland and the physiological apparatus to initiate lactation as soon as the calf is born (Drackley 1999). Recently, the high energetic cost of excess inflammation and immune activation during the transition period has become evident (Bradford et al. 2015; Sordillo 2016; Horst et al. 2021). Other reviews have described in detail the physiological mechanism of both parturition and lactogenesis in cattle (Drackley et al. 2005). Our intent here is to present the process of how the transfer of immunoglobulins from the maternal circulation into mammary secretion occurs during prepartum, as a basis for discussing the nutritional modulation of passive immunity transfer.
The process of colostrum formation, i.e. the transfer of immunoglobulins from the maternal circulation into mammary secretions during the last weeks of gestation is called colostrogenesis, or Stage I lactogenesis (Neville et al. 2002). The onset and conclusion of colostrum production have been observed to occur approximately 14 days before calving, with the rate of colostrogenesis gradually accelerating until peaking 24–48 h before calving (Sasaki et al. 1976; Baumrucker and Bruckmaier 2014). In cows, the main component of immunoglobulins is immunoglobulin G1 (IgG1), representing 90% of the total concentration of immunoglobulins (Butler 1974; McGee et al. 2005, 2006).
The transfer of IgG1 occurs via transcytosis, which involves a distinct receptor (Mayer et al. 2005; Baumrucker and Bruckmaier 2014). The functioning of these receptors is influenced by elevated oestrogens concentrations and reduced progesterone concentrations, leading to their activation and enhancement (Winger et al. 1995; Barrington et al. 2001). The transfer of IgG1 ceases during parturition, coinciding with a rapid increase in prolactin concentration in the blood, the commencement of Stage II lactogenesis, and the onset of copious milk secretion (Barrington et al. 2001; Akers 2006). Elevated oestrogen and reduced progesterone concentrations are also crucial for initiating lactation, by stimulating the release of prolactin from the pituitary gland and increasing the density of prolactin receptors in mammary cells (Tucker 2000).
Apart from IgG1, calcium (Ca) is a vital component of colostrum, and its availability can affect colostrum production. Cow’s colostrum typically contains approximately 2.1 g of Ca per litre, and the secretion of colostrum represents a significant withdrawal of blood Ca (Kume and Tanabe 1993). In healthy adult cows, approximately 3 g of Ca are present in the plasma, and the abrupt transfer of Ca to the mammary gland during the onset of lactation, particularly in high-producing cows, can lead to a substantial decrease in blood Ca concentration, resulting in hypocalcaemia (Goff 2000). Increased bone Ca mobilisation and renal reabsorption are physiological mechanisms activated during hypocalcaemia (Goff 2000).
Stimulation of Ca mobilisation prior to calving through low dietary cation–anion-difference (low-DCAD) diets has been demonstrated to increase overall colostrum yield and total IgG production without altering colostrum IgG concentrations (Graef et al. 2021). Two hormones primarily regulate Ca mobilisation around calving, namely, parathyroid hormone (PTH) and 1,25-dihydroxycholecalciferol, which is a metabolite of vitamin D. The concentration of 1,25-dihydroxycholecalciferol in circulation increases during late gestation and early lactation in response to elevated PTH concentration. Both hormones function to raise blood Ca concentrations through enhanced absorption in the intestines and kidneys, as well as the release of Ca from bones (Goff 2000). Because Ca and phosphorus (P) work in conjunction to form hydroxyapatite, a crucial component of bone mineralisation, prolonged exposure of rangeland beef cows to diets deficient in P or Ca can deplete bone reserves (Call et al. 1986) and affect the availability of Ca during the onset of lactation.
Effect of prepartum nutrition on the transfer of passive immunity
One of the consistent risk factors causing high rates of beef calf mortality in the dry tropics is malnutrition of cows during the prepartum period (Fordyce et al. 2023) and it is believed to be associated with reduced milk delivery and dehydration of neonatal calves (Fordyce et al. 2015). In addition to the dehydration effect, low colostrum intake can cause the failure of passive immunity transfer to neonatal calves, resulting in increased morbidity and mortality rates (McGuirk and Collins 2004; Raboisson et al. 2016). Thus, beef calves must ingest a minimum of 3 L of high-quality colostrum within the initial hours after birth. This colostrum should have IgG concentrations exceeding 100 g/L to ensure a plasma IgG concentration of at least 24 g/L, which signifies successful passive transfer (Dewell et al. 2006; McGee et al. 2006; Drikic et al. 2018). In general, beef cows have higher colostrum IgG concentrations at first milking than do dairy cows, but there is a significant variation among cows (Guy et al. 1994). In contrast to dairy cows, there was no variation in colostrum IgG concentrations between multiparous and primiparous beef cows, but there was variation in the immune status of their calves, reflecting the lower colostrum yield in first-calving heifers (McGee et al. 2006).
Protein and energy malnutrition often occurs during the immediate prepartum period for beef cows in the dry tropics. In general, late gestation and colostrogenesis fall during the late dry season (1 September until the season break; Bortolussi et al. 2005) and there is a significant disparity between nutrients supplied by pasture and the cow’s CP and energy requirements (Fig. 1). This is particularly problematic for CP, which is generally the first limiting nutrient for beef cows grazing pasture in northern Australia (Poppi et al. 2018; Dixon et al. 2022). The seasonal variation in nutrient supply from the pasture is also usually greater for CP (4.5–9.5% DM) than for energy (7–9 MJ/kg), as illustrated in Fig. 1.
Protein and energy requirements of a Bos indicus cow during the annual cycle. Assuming a mature weight of 480 kg, calving on 1 November and weaning on 1 June. The same range (2–14) is used in the protein and energy Y-axes to illustrate the greater relative variation in protein requirement and supply during the cycle.
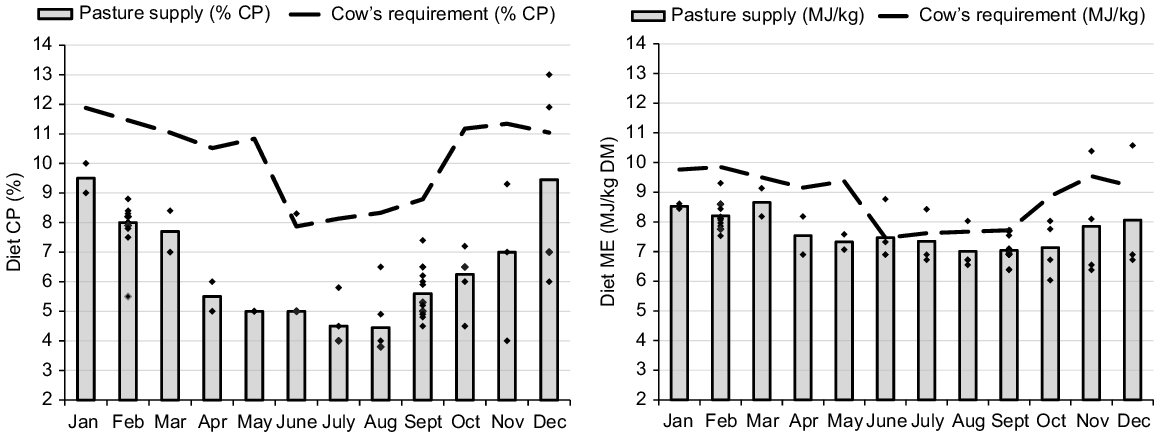
The lower supply of protein and energy from pasture to prepartum cows can impair fetal growth and metabolism (Funston et al. 2010; Blair et al. 2021), and this can be expressed through lower weight at birth (Larson et al. 2009; Chen et al. 2022). The same condition may also reduce the IgG1 content in the colostrum. In sheep, a more drastic dietary restriction for the last 38 days of gestation decreased colostrum yield by 52% (Fig. 2; Nørgaard et al. 2008), even considering the excellent BCS of the ewes at the start of the restriction period (BCS = 4.5 in a 1–5 scale). McGee et al. (2006) changed the diet of multiparous cows from grass silage to straw for only the last 15 days of gestation and reported an 18% reduction in total IgG1 mass in the colostrum (Fig. 2). The impact of short-term dietary restrictions on milk delivery to newborn calves has also been recently validated by measuring calf growth for the first 2 weeks and IgG1 concentration in calf plasma as indicators of colostrum intake (Muller et al. 2022; Silva et al. 2022). These studies suggested that even temporary deficiencies during the time of calving can significantly diminish colostrum production and hinder passive transfer. Furthermore, it is noteworthy that the impact of malnutrition is not contingent on the BCS of the dam.
Effect of prepartum diet restrictions on total colostrum yield. Data from Nørgaard et al. (2008) and McGee et al. (2006).
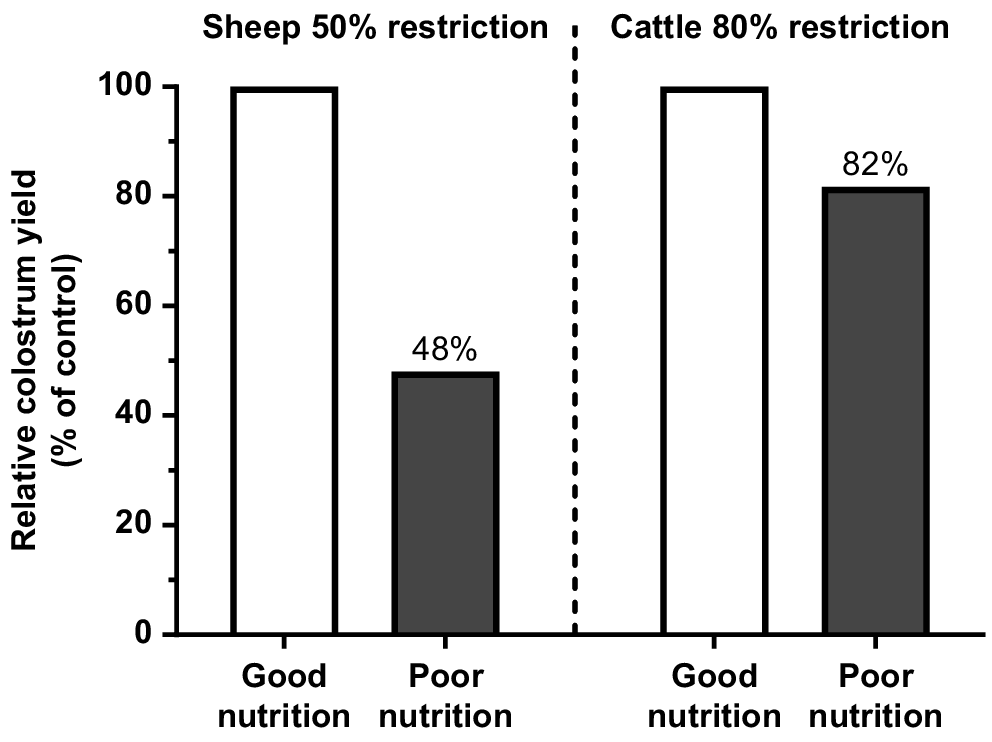
The mechanism by which prepartum malnutrition can restrict the transfer of IgG1 into mammary secretions during colostrogenesis is unclear (McGee et al. 2006). However, it is likely to be associated with alterations in progesterone and oestrogen concentrations in the week leading up to calving (Silva et al. 2022). Insufficient prepartum nutrition can delay the decline in progesterone concentrations before calving (O’Doherty and Crosby 1996; Silva et al. 2022), which has been found to inhibit the transfer of IgG1 into colostrum and impede milk production (Baumrucker and Bruckmaier 2014; Gross et al. 2014).
Severe nutrient restriction during the prepartum may also influence the mammary gland blood flow and supply of nutrients. Kennedy et al. (2019) found that when late gestational beef cows were provided a low-protein diet (5.1% CP, ad libitum access to corn stover and corn silage), the ipsilateral mammary gland blood flow was significantly lower than in cows that were supplemented with corn dried-distiller’s grains plus solubles at a rate of 0.30% of their bodyweight (1.76 ± 0.30 L/min vs 2.76 ± 0.30 L/min). Milk yield was also lower in the protein-restricted-diet cows than in supplemented cows (10.2 kg/day vs 13.5 ± 1.2 kg/day). Similar results on reduced blood flow to the mammary gland were reported in a recent study with Hereford–SimAngus heifers receiving 70% of calculated requirements (Redifer et al. 2022).
In the dry tropics, the prepartum period also coincides with elevated ambient temperatures. Reduced blood flow to internal organs is a well known consequence of heat stress in cattle (Sejian et al. 2018). In dairy cows, heat stress during the dry period can reduce mammary cell proliferation (Tao et al. 2012) and also reduce feed intake and milk production (Cowley et al. 2015). In beef cows, because their average threshold of temperature–humidity index is higher, and can decrease the body temperature through decreasing feed intake, increasing water intake, panting and urination, the thermoneutral zone is higher than in dairy cows (Summer et al. 2019). Brody (1956) reported that the milk yield of Brahman cows decreases by approximately 1 kg/day when the ambient temperatures increase from 35°C to 43°C. Thus, the combination of heat stress and nutrient restriction during the late dry system may reduce milk production via a reduction in the supply of nutrients for milk synthesis as well as a reduced uptake and transfer of IgG1 by the mammary gland (Davis and Collier 1985; Wall and McFadden 2012).
The successful transfer of passive immunity relies not only on the quantity and quality of colostrum produced by the cow but also on the calf’s ability to ingest colostrum and the intestinal capacity of newborn calves to absorb IgG1 (Weaver et al. 2000). Insufficient maternal nutrition during late gestation can affect both factors and often leads to lower birth weights, which can result in reduced calf vigour and delayed initiation of suckling (Kim et al. 1988; Riley et al. 2004; Hogan et al. 2022). Once colostrum is ingested, the enterocytes in the small intestine absorb IgG1 through pinocytosis and transfer it across the intestinal barrier into lymphatics via exocytosis (Broughton and Lecce 1970; Staley et al. 1972). Subsequently, IgG1 enters the bloodstream through the thoracic duct (Weaver et al. 2000).
The closure of IgG1 absorption occurs when the small intestine can no longer absorb macromolecules and transfer them into the bloodstream (Lecce and Morgan 1962), usually about 24 h after birth (Godden et al. 2019; McGee and Earley 2019). The gut-closure mechanism is unclear (Weaver et al. 2000). However, this process seems to be associated with the depletion of the pinocytotic activity, or the enterocytes being replaced with mature epithelial cells (Lopez and Heinrichs 2022). The absorption and transfer capacity of the small intestine in neonatal calves may also be associated with the development and maturation of the small intestine during the intrauterine stage.
Prepartum nutrition restriction can impair intestinal development of the fetus (Duarte et al. 2013) and reduce the ability of newborn calves to absorb IgG1 (Blecha et al. 1981). In sheep, low protein content in the diets during pre-lambing decreased the lamb’s efficiency to absorb IgG during the first 18 h of age (O’Doherty and Crosby 1997). Blecha et al. (1981) found that although there was no effect of increased CP consumption on circulating IgG in maternal serum or colostrum, CP intake below 0.61 kg/day (1.6 g/kg liveweight, LW) can reduce the ability of calves to absorb IgG. All the calves in this study were removed from the dam and fed commercial colostrum, so this was a direct effect of the lack of protein on gut development and function, rather than an interaction between colostrum and the calf.
Alterations in the plasma cortisol concentration of neonatal calves may also affect the precocious closure of the small intestine to macromolecules and reduce IgG1 absorption. Cortisol and other glucocorticoids stimulate tight junction and mucosal formation in neonatal calves (Hulbert and Moisá 2016) and increase brush border enzymes such as sucrase, alkaline phosphatase and cell-membrane transporters such as Na+/K+ATPase (Guiraldes et al. 1981). Cortisol produced by the fetus increases as the fetal hypothalamic–pituitary–adrenal axis matures in the last 3–7 days before calving (Taverne et al. 1988; Schuler et al. 2018) and the cortisol peak about the time of calving seems to be beneficial to enhance the initial rate of IgG1 absorption (Hough et al. 1990a). In contrast to this beneficial effect of elevated cortisol, prepartum dietary restrictions increase plasma cortisol and reduce triiodothyronine (T3) concentrations in newborn calves, and reduce the absorption efficiency and the plasma IgG1 concentration (Burton et al. 1984; Hough et al. 1990b; LeMaster et al. 2017). Likely, the lower IgG1 absorption efficiency in the small intestine of calves born from nutrient-restricted cows is related to lower birth weight and immaturity of the intestine rather than a direct inhibitory effect of elevated cortisol concentrations.
From the discussions above, it is apparent that prepartum malnutrition can cause severe impacts on the calf’s growth and survival (Fig. 3). One possible mechanism involves reduced nutrient supply, which is likely to be linked to decreased blood flow to both the uterus and the mammary gland (Kennedy et al. 2016, 2019). The lower nutrient supply can result in reduced birth weight and diminished colostrum production. The second potential mechanism is characterised by elevated progesterone concentrations and decreased oestrogen concentrations in the blood in the weeks leading up to calving. As a result, the transfer of IgG1 into mammary secretions is hindered, leading to reduced colostrum quality and potential failure in the transfer of passive immunity. Additionally, as a third potential mechanism, an increase in cortisol concentrations in both the cow and newborn calf can diminish the ability of the small intestine to absorb IgG1 and delay milk production and delivery.
Phosphorus
Because of low soil fertility, mineral deficiencies, especially P, are common across the dry tropics (McCosker and Winks 1994). Therefore, the cattle industry has invested considerable resources in determining cattle P requirements and the most cost-effective supplementation strategies (Dixon et al. 2020). Nonetheless, there is limited information on the effect of P supplementation on colostrum production and the transfer of passive immunity. To our knowledge, no study has evaluated the direct effect of P deficiency on the transfer of passive immunity in grazing cattle.
There is evidence that calf mortality is increased when cattle are in P-deficient pastures, as measured by faecal P (Fordyce et al. 2023), which could be linked to delayed colostrogenesis leading to dehydration and death of the calf (Muller et al. 2022). However, a recent experiment in northern Australia with long-term P deficiency in grazing cows did not find statistically significant differences in calf mortality between supplemented and non-supplemented cows (17% vs 22% of calf loss, P > 0.05), despite large differences observed in cow liveweight and weaning weight of calves (188 kg vs 155 kg LW at weaning for the supplemented and non-supplemented group respectively; Schatz et al. 2023). Transfer of passive immunity was not evaluated.
It has been well established that P-deficient cattle have lower DM and energy intake, leading to cows with lower LW and BCS at calving (Benvenutti et al. 2015; Dixon et al. 2016). Also, a lower BCS at calving and lower DM intake reduce colostrum and total mass of IgG yields (Odde 1988; Shearer et al. 1992; McGee et al. 2006). Therefore, it is reasonable to expect that prepartum P supplementation would increase colostrum production and transfer of passive immunity in P-deficient cattle, especially when considering the significant increase in P requirements during late gestation and early lactation (CSIRO 2007). However, mature cows can mobilise bone P stocks to supply the deficit during the prepartum period and maintain similar colostrum and milk production (Valk et al. 2002; Dixon et al. 2020). It is only in extreme situations when body P reserves are depleted, that a lack of P can result in lighter birth weight of calves (Read et al. 1986a), lower milk yield and lower calf growth (Read et al. 1986b; Schatz et al. 2023), potentially increasing the risk of calf mortality. Therefore, in most situations, the short-term supply of P supplements about the time of calving is expected to have minimal impacts on colostrogenesis and the transfer of passive immunity.
Microbial products
The health status of pregnant cows during the calving period can have a significant impact on colostrogenesis and milk yield, because inflammatory processes during this time may divert energy away from colostrum and milk synthesis (Horst et al. 2021). The metabolic status of prepartum dairy cows has been shown to influence colostrum quality, with cows under higher metabolic stress, as measured by higher lameness score and higher serum activity of glutamate dehydrogenase (GLDH), having lower colostrum quality (Immler et al. 2021). Therefore, microbial products, including prebiotics, probiotics, and postbiotics, have been used in an attempt to modulate the immune response of cows during the periparturient period (Barreto et al. 2021).
These microbial products contain antioxidants, B vitamins, nucleotides, amino acids, soluble fibre, and other bioactive compounds that can serve as direct nutrient sources, growth promoters for rumen microflora, and protect the gut wall (Zaworski et al. 2014). Certain components of yeast, such as beta-glucan, can agglutinate harmful bacteria, protecting the host from infection and the need for an immune response (Zanello et al. 2011).
In ewes, supplementation with live yeast during the last month of gestation increased colostrum IgG and other bioactive molecules (Dunière et al. 2022). This improvement in colostrum composition translated into higher serum IgG in lambs born from supplemented ewes and kept with their mothers for only 12 h, while no effect of supplementation was observed in the lamb group that stayed with the dams (Dunière et al. 2022). In dairy cows, supplementation with a yeast fermentation product during the transition period enhanced mucosal immunity and humoral immune response, without a positive effect on colostrum IgG (Yuan et al. 2015). In another similar study, the inclusion of yeast-fermentation products during the transition period decreased serum cortisol on the first day after calving, increased milk production, and decreased milk somatic cell count (Zaworski et al. 2014). The same beneficial effect on milk somatic cell count, reflecting the immune modulation, was reported by Yuan et al. (2015).
There is limited information on the effects of microbial products on the inflammatory response of grazing beef cows. The inclusion of yeast-fermentation products in the protein supplement has been shown to enhance transfer of passive immunity in Bos indicus cows (Silva et al. 2022) fed with low-quality hay. Taken together, these studies suggested that although protein and energy are the limiting nutrients during the prepartum period, the addition of microbial products can assist in the transfer of passive immunity by either improving rumen efficiency, protecting the cow from the negative effects of inflammation, or both.
Nonetheless, for microbial products to be useful as additives in cattle supplements, they need not only to promote nutritional and health benefits and be cost-effective but also to maintain activity during the supplementation period. Variations in moisture and temperature, as well as mixing with other toxic compounds (e.g. copper), can cause cell death and reduce the viability of active dry microbes (Sullivan and Bradford 2011). Micro-encapsulation and drying technologies and the use of yeast cultures instead of live cells have emerged as solutions to improve the stability of microbial products (Chaucheyras-Durand and Dunière 2020).
Water-restriction impact on milk delivery to neonatal calves
It is not uncommon for cattle in extensive grazing systems to experience some degree of water deprivation as water is not freely available, and cattle may drink only once daily or every other day (Squires 1981; Williams et al. 2019). Because of the high cost of installing water points in large paddocks, the frequency and distribution of water points are determined on the basis of the manipulation of grazing distribution and, in some regions, such as the northern forest in Australia, distances between water points greater than 3 km are not uncommon (Hunt et al. 2014; Cowley et al. 2020). Therefore, in inadequately managed paddocks, the malnutrition of late-pregnant cows is usually associated with large distances between water points, creating a compounding risk effect for impaired milk production and transfer of passive immunity to calves.
There is a scarcity of studies evaluating the direct impact of water restriction on colostrum yield and the transfer of passive immunity in ruminants, and almost no information on the impact on rangeland beef cows. It has been recognised that severe water deprivation reduces milk production in other lactating ruminants, such as dairy cows (Golher et al. 2021), sheep (Chedid et al. 2014; Casamassima et al. 2018) and goats (Aganga 1992), and that the reduced milk yield is part of the survival mechanism, which is likely to be caused by a combined effect of lower DM intake and changes in blood osmolarity (Olsson 2005; Ocak Yetisgin and Şen 2020). In dairy cows, a decline of 50% in voluntary water intake resulted in a 26% reduction in milk yield (Little et al. 1980).
Only one study was identified that quantified impacts of water restriction during late pregnancy on colostrum secretion in either sheep or cattle. Mleil et al. (2012) reported that giving water only every 3 days during the last 50 days of pregnancy and the first 60 days of lactation increased the bodyweight loss of postpartum Barbarine ewes (a breed native to arid lands) by 19% and reduced by 26% the milk yield on Day 10 of lactation (first measurement after birth). The secretion of colostrum during the first 24 h was numerically lower by 25% (627 g/h compared with 832 ± 350 g/h); however, despite being biologically relevant, this difference was not statistically significant (P > 0.05) and the growth rate of lambs was not affected by the water restriction.
A similar effect of water restriction in magnifying the problems of malnutrition during late pregnancy was observed by Nicholson (1987) in southern Ethiopia. Water restriction depressed birth weights of calves by 2–5 kg, and the weight and condition of lactating cows declined more rapidly during the dry season than did those of cows given daily access to water, likely reflecting lower intake during the prepartum period. Water restriction reduced the milk intake of calves by 15%, as measured by the 210-day weaning weight (Nicholson 1987). Despite the observed negative effects of water restriction on late-pregnant cows, because less frequent drinking did not affect calving rates or the growth of steers, Nicholson (1987) concluded that giving water only every 3 days can be undertaken indefinitely with all classes of stock.
The conclusion of the Nicholson (1987) study highlighted the complexities involved in evaluating the impact of inadequate nutrition during pregnancy on long-term productivity. Previous experiments with beef cattle have consistently demonstrated that restricting water access from daily to every second or third day reduces both total water and feed intake, elevates the risk of LW loss, and is likely to decrease milk production (Williams et al. 2017), although the effect on milk-solid yield is unknown. However, the effect of water restriction on cattle LW production remains unclear and inconclusive, precluding producers from making informed financial decisions regarding investments in water-infrastructure improvements.
It is evident that pregnancy and lactation increase the water requirements of cows, and so does high ambient temperature (Winchester and Morris 1956; NRC 1981). During the last trimester of pregnancy, the combined effect of increased intake, fetal growth, and high ambient temperatures elevates daily water requirements from approximately 32–50 L (Fig. 4). Therefore, it is reasonable to expect that improving water infrastructure can increase milk delivery and neonatal calf health (Bell and Sangster 2023; Fordyce et al. 2023). However, this hypothesis is yet to be tested.
Water requirements during the annual cycle of a Bos indicus cow in three locations with different annual average temperatures. Assuming a mature weight of 480 kg, calving on 1 November and weaning on 1 June. Estimated using equations from Winchester and Morris (1956).
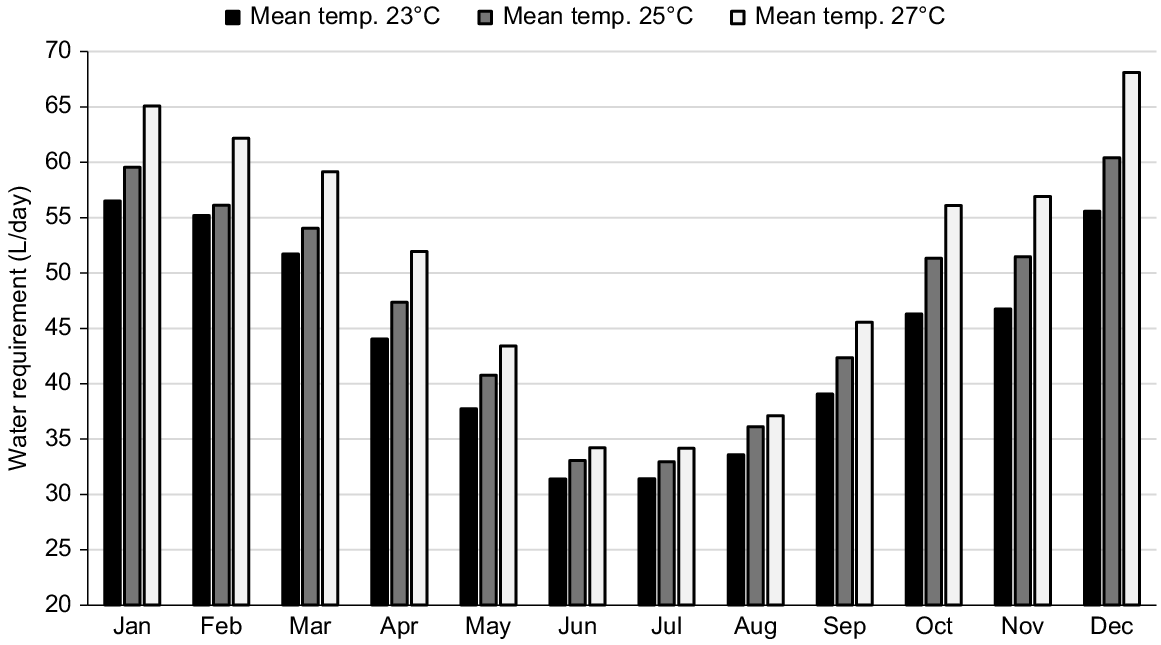
Long-term effects of prepartum malnutrition
In northern Australia, Brazil and other tropical regions, Wagyu and other B. taurus genetics are becoming increasingly incorporated into the beef herd and, with their comparatively higher growth and marbling potential, the impact of maternal nutrition may persist into later life (Greenwood 2021). Thus, it is important to understand how restricted maternal energy or protein intake will affect offspring growth and meat quality. As discussed, impairments in milk delivery and the transfer of passive immunity can have long-term effects on offspring performance (Fischer-Tlustos et al. 2021). In addition, lack of nutrients during pregnancy can affect fetal programming and promote epigenetic changes, also associated with reduced offspring performance, including a compromised immune system, increased fat deposition, slower growth rates, reduced meat quality, reduced muscle mass and lower reproductive outcomes (Robinson et al. 2013; Bell and Greenwood 2016). Thus, it is difficult to separate the effects of overall maternal lactational performance, failure of passive immunity transfer, fetal programming and true epigenetic changes.
Prepartum malnutrition that results in intrauterine growth retardation results in calves that are lighter than their peers in the same cohort at any given age (Greenwood and Cafe 2007). Restricting energy and protein supply during the 2nd and 3rd trimesters of Angus cows carrying calves with either Piedmontese or Wagyu sires resulted in a 35% reduction in birth weight (Greenwood et al. 2005, 2006). Even once adequate levels of nutrition were restored, the growth rates from birth to weaning were 8.7% lower than those with adequate prenatal nutrition (Cafe et al. 2006). This could be explained by carry-over effects on the dam’s lactational performance or a reduction in the capacity of the progeny to achieve compensatory growth. At feedlot entry (26 months) steers were still significantly lighter than their high birth-weight counterparts and had lower average daily gains until feedlot exit at 30 months (Fig. 5). Carcass weights at 30 months were reduced and retail yield was 7% lower for low birth-weight steers (Greenwood et al. 2006). When adjusted for differences in carcass weight, carcass traits between the two groups, including marbling, did not vary, other than rump fat cover (P8 fat), which was significantly increased in low birth-weight steers when slaughtered at 30 months (Fig. 5).
Summary of results from the Australian Beef Co-operative Research Centre, showing the impacts of reduced nutrition during the 2nd and 3rd trimesters on growth and carcass parameters of steers with Piedmontese or Wagyu sires entering the feedlot at 26 months, Cafe et al. (2006), (2009); Greenwood et al. (2006).
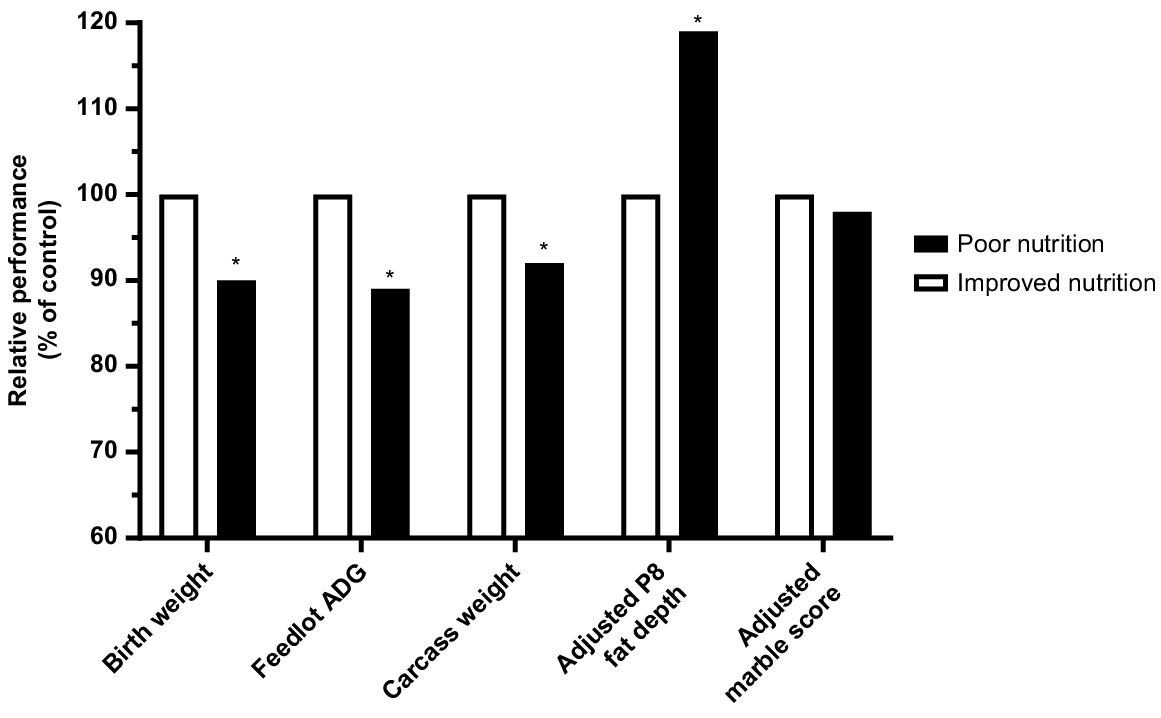
Other studies have reported that supplementing cows in the last trimester of gestation with either protein or energy can improve the carcass quality of steers, even if there are no effects on LW or feed efficiency. Larson et al. (2009) found that protein supplementation significantly improved marbling scores and carcass quality. Supplementing dams with an average of 0.45 kg/day of a 28% CP supplement (0.13 kg CP/day) resulted in an 11% improvement in marbling grade and more carcasses qualifying for higher USDA quality grades. Variability in marbling can be attributed to the variation in both the number and size of intramuscular adipocytes. Administration of targeted supplements during crucial stages of adipocyte development in dams can lead to an improvement in marbling scores (Ladeira et al. 2018).
The timing of the supplementation appears to have an impact on how fetal programming occurs. A shorter, more intensive period of supplementation in late gestation, when the fetus is at a critical growth point, seems to be more effective than supplementing at a lower level of intake over the entire year or pregnancy (Palmer et al. 2022a). Concentrating dried distiller-grain supplementation into the first part of the third trimester improved marbling scores when compared with lower levels of supplementation over the whole third trimester (Palmer et al. 2022b).
Malnutrition during all stages of pregnancy can negatively affect growth and carcass traits. Targeted supplementation of pregnant cows during late gestation, when fetal muscle fibre hypertrophy and adipocyte proliferation are occurring (Du et al. 2010), can increase the feedlot performance of their progeny (Cafe et al. 2006; Greenwood et al. 2006; Palmer et al. 2022a). These effects have not been tested on the B. indicus x B. taurus genotypes that make up the majority of the northern Australian beef industry.
Conclusions
In northern Australia and the dry tropics, late-pregnant beef cows are often under nutritional and environmental stress. The success of the nutritional program is usually evaluated by the reproductive performance of the cows, with few studies evaluating the impact on the newborn calf. Current research demonstrated that severe diet restrictions about the time of calving can cause endocrinal changes and impair colostrogenesis and the transfer of passive immunity to calves. Moreover, inadequate nutrition and higher cortisol concentrations during the periparturient period can impair fetal intestine development and reduce immunoglobulin absorption in newborn calves. Thus, malnutrition during late pregnancy and early life can have long-lasting effects on calf performance, including changes in carcass composition and muscle marbling.
Despite these advances in the current knowledge on the nutritional modulation of colostrogenesis in grazing beef cows, crucial areas for improvement remain largely unknown. Specifically, the impact of restricting specific dietary components such as water, energy, protein, lipids, minerals, and vitamins is not well understood, hindering the development of targeted supplementation strategies and infrastructure investments. How improved nutrition and the use of active compounds can protect cows from costly inflammatory responses is another area of active research. Nonetheless, the increased concern for the wellbeing of livestock is motivating the industry to find solutions to reduce the nutritional and environmental stress currently faced by pregnant beef cows.
References
Abuelo A, Havrlant P, Wood N, Hernandez-Jover M (2019) An investigation of dairy calf management practices, colostrum quality, failure of transfer of passive immunity, and occurrence of enteropathogens among Australian dairy farms. Journal of Dairy Science 102, 8352-8366.
| Crossref | Google Scholar | PubMed |
Aganga A (1992) Water utilization by sheep and goats in northern Nigeria. World Animal Review 73, 9-14.
| Google Scholar |
Akers RM (2006) Major advances associated with hormone and growth factor regulation of mammary growth and lactation in dairy cows. Journal of Dairy Science 89, 1222-1234.
| Crossref | Google Scholar | PubMed |
Barreto MO, Soust M, Moore RJ, Olchowy TWJ, Alawneh JI (2021) Systematic review and meta-analysis of probiotic use on inflammatory biomarkers and disease prevention in cattle. Preventive Veterinary Medicine 194, 105433.
| Crossref | Google Scholar | PubMed |
Barrington GM, Parish SM (2001) Bovine neonatal immunology. Veterinary Clinics of North America: Food Animal Practice 17, 463-476.
| Crossref | Google Scholar | PubMed |
Barrington GM, McFadden TB, Huyler MT, Besser TE (2001) Regulation of colostrogenesis in cattle. Livestock Production Science 70, 95-104.
| Crossref | Google Scholar |
Baumrucker CR, Bruckmaier RM (2014) Colostrogenesis: IgG1 transcytosis mechanisms. Journal of Mammary Gland Biology and Neoplasia 19, 103-117.
| Crossref | Google Scholar | PubMed |
Bell AW, Greenwood PL (2016) Prenatal origins of postnatal variation in growth, development and productivity of ruminants. Animal Production Science 56, 1217.
| Crossref | Google Scholar |
Bell A, Sangster N (2023) Research, development and adoption for the north Australian beef cattle breeding industry: an analysis of needs and gaps. Animal Production Science 63, 1-40.
| Crossref | Google Scholar |
Benvenutti M, Dixon RM, Goodwin KL, Kidd L, Mayer R, Fletcher MT, Anderson S (2015) Responses of Bos indicus cows in early lactation to dietary phosphorus deficiency. In ‘Recent advances in animal nutrition in Australia. Vol. 2015’. (Ed. R Swick) pp. 47–48. (School of Environmental and Rural Science, University of New England: Armidale, NSW, Australia)
Blair AD, Gubbels ER, Block JJ, Olson KC, Grubbs JK, Underwood KR (2021) Maternal nutrition and meat quality of progeny. Meat and Muscle Biology 5, 1-9.
| Crossref | Google Scholar |
Blecha F, Bull RC, Olson DP, Ross RH, Curtis S (1981) Effects of prepartum protein restriction in the beef cow on immunoglobin content in blood and colostral whey and subsequent immunoglobin absorption by the neonatal calf. Journal of Animal Science 53, 1174-1180.
| Crossref | Google Scholar | PubMed |
Bortolussi G, McIvor JG, Hodgkinson JJ, Coffey SG, Holmes CR (2005) The northern Australian beef industry, a snapshot. 2. Breeding herd performance and management. Australian Journal of Experimental Agriculture 45, 1075-1091.
| Crossref | Google Scholar |
Bradford BJ, Yuan K, Farney JK, Mamedova LK, Carpenter AJ (2015) Invited review: Inflammation during the transition to lactation: new adventures with an old flame. Journal of Dairy Science 98, 6631-6650.
| Crossref | Google Scholar | PubMed |
Bragg R, Macrae A, Lycett S, Burrough E, Russell G, Corbishley A (2020) Prevalence and risk factors associated with failure of transfer of passive immunity in spring born beef suckler calves in Great Britain. Preventive Veterinary Medicine 181, 105059.
| Crossref | Google Scholar | PubMed |
Brody S (1956) Climatic physiology of cattle. Journal of Dairy Science 39, 715-725.
| Crossref | Google Scholar |
Broughton CW, Lecce JG (1970) Electron-microscopic studies of the jejunal epithelium from neonatal pigs fed different diets. The Journal of Nutrition 100, 445-449.
| Crossref | Google Scholar | PubMed |
Burton JH, Hosein AA, McMillan I, Grieve DG, Wilkie BN (1984) Immunoglobulin absorption in calves as influenced by dietary protein intakes of their dams. Canadian Journal of Animal Science 64, 185-186.
| Crossref | Google Scholar |
Butler JE (1974) Immunoglobulins of the mammary secretions. In ‘Lactation: a comprehensive treatise’. (Eds BL Larson, V Smith) pp. 217–255. (Academic Press: New York, NY, USA) doi:10.1016/b978-0-12-436703-6.50011-9
Cafe LM, Hennessy DW, Hearnshaw H, Morris SG, Greenwood PL (2006) Influences of nutrition during pregnancy and lactation on birth weights and growth to weaning of calves sired by Piedmontese or Wagyu bulls. Australian Journal of Experimental Agriculture 46, 245-255.
| Crossref | Google Scholar |
Cafe LM, Hennessy DW, Hearnshaw H, Morris SG, Greenwood PL (2009) Consequences of prenatal and preweaning growth for feedlot growth, intake and efficiency of Piedmontese- and Wagyu-Sired cattle. Animal Production Science 49, 491-467.
| Crossref | Google Scholar |
Call JW, Butcher JE, Shupe JL, Blake JT, Olson AE (1986) Dietary phosphorus for beef cows. American Journal of Veterinary Research 47, 475-481.
| Google Scholar | PubMed |
Carvalho RS, Cooke RF, Cappellozza BI, Peres RFG, Pohler KG, Vasconcelos JLM (2022) Influence of body condition score and its change after parturition on pregnancy rates to fixed-timed artificial insemination in Bos indicus beef cows. Animal Reproduction Science 243, 107028.
| Crossref | Google Scholar | PubMed |
Casamassima D, Palazzo M, Nardoia M, D’Alessandro AG, Vizzarri F (2018) Effect of water restriction on milk yield and quality in Lacaune breed ewes. Journal of Animal Physiology and Animal Nutrition 102, e677-e685.
| Crossref | Google Scholar | PubMed |
Castro N, Capote J, Bruckmaier RM, Argüello A (2011) Management effects on colostrogenesis in small ruminants: a review. Journal of Applied Animal Research 39, 85-93.
| Crossref | Google Scholar |
Chaucheyras-Durand F, Dunière L (2020) The use of probiotics as supplements for ruminants. In ‘Improving rumen function’. (Eds C McSweeney, R Mackie) pp. 775–818. (Burleigh Dodds Science Publishing: London, UK) doi:10.1201/9781003047841
Chedid M, Jaber LS, Giger-Reverdin S, Duvaux-Ponter C, Hamadeh SK (2014) Review: water stress in sheep raised under arid conditions. Canadian Journal of Animal Science 94, 243-257.
| Crossref | Google Scholar |
Chen H, Wang C, Huasai S, Chen A (2022) Effect of prepartum dietary energy density on beef cow energy metabolites, and birth weight and antioxidative capabilities of neonatal calves. Scientific Reports 12, 4828.
| Crossref | Google Scholar | PubMed |
Cowley FC, Barber DG, Houlihan AV, Poppi DP (2015) Immediate and residual effects of heat stress and restricted intake on milk protein and casein composition and energy metabolism. Journal of Dairy Science 98, 2356-2368.
| Crossref | Google Scholar | PubMed |
Davis SR, Collier RJ (1985) Mammary blood flow and regulation of substrate supply for milk synthesis. Journal of Dairy Science 68, 1041-1058.
| Crossref | Google Scholar | PubMed |
Dewell RD, Hungerford LL, Keen JE, Laegreid WW, Griffin DD, Rupp GP, Grotelueschen DM (2006) Association of neonatal serum immunoglobulin G1 concentration with health and performance in beef calves. Journal of the American Veterinary Medical Association 228, 914-921.
| Crossref | Google Scholar | PubMed |
Dixon RM, Castells L, Kidd L, Goodwin KL, Mayer R, McNeill DM, Fletcher MT, Anderson ST (2016) Responses of deficient breeder cows to additional diet phosphorus. Proceedings of the Australian Society of Animal Production 31, 91.
| Google Scholar |
Dixon RM, Anderson ST, Kidd LJ, Fletcher MT (2020) Management of phosphorus nutrition of beef cattle grazing seasonally dry rangelands: a review. Animal Production Science 60, 863-879.
| Crossref | Google Scholar |
Dixon RM, Sullivan MT, O’Connor SN, Mayer RJ (2022) Diet quality, liveweight change and responses to N supplements by cattle grazing. The Rangeland Journal 44, 97-113.
| Crossref | Google Scholar |
Drackley JK (1999) Biology of dairy cows during the transition period: the final frontier? Journal of Dairy Science 82, 2259-2273.
| Crossref | Google Scholar | PubMed |
Drackley JK, Dann HM, Douglas N, Guretzky NAJ, Litherland NB, Underwood JP, Loor JJ (2005) Physiological and pathological adaptations in dairy cows that may increase susceptibility to periparturient diseases and disorders. Italian Journal of Animal Science 4, 323-344.
| Crossref | Google Scholar |
Drikic M, Windeyer C, Olsen S, Fu Y, Doepel L, De Buck J (2018) Determining the IgG concentrations in bovine colostrum and calf sera with a novel enzymatic assay. Journal of Animal Science and Biotechnology 9, 69.
| Crossref | Google Scholar |
Du M, Tong J, Zhao J, Underwood KR, Zhu M, Ford SP, Nathanielsz PW (2010) Fetal programming of skeletal muscle development in ruminant animals. Journal of Animal Science 88, E51-E60.
| Crossref | Google Scholar | PubMed |
Duarte MS, Gionbelli MP, Paulino PVR, Serão NVL, Martins TS, Tótaro PIS, Neves CA, Valadares Filho SC, Dodson MV, Zhu M, Du M (2013) Effects of maternal nutrition on development of gastrointestinal tract of bovine fetus at different stages of gestation. Livestock Science 153, 60-65.
| Crossref | Google Scholar |
Dunière L, Renaud JB, Steele MA, Achard CS, Forano E, Chaucheyras-Durand F (2022) A live yeast supplementation to gestating ewes improves bioactive molecules composition in colostrum with no impact on its bacterial composition and beneficially affects immune status of the offspring. Journal of Nutritional Science 11, E5.
| Crossref | Google Scholar |
D’Occhio MJ, Baruselli PS, Campanile G (2019) Influence of nutrition, body condition, and metabolic status on reproduction in female beef cattle: a review. Theriogenology 125, 277-284.
| Crossref | Google Scholar |
Fischer-Tlustos AJ, Lopez A, Hare KS, Wood KM, Steele MA (2021) Effects of colostrum management on transfer of passive immunity and the potential role of colostral bioactive components on neonatal calf development and metabolism. Canadian Journal of Animal Science 101, 405-426.
| Crossref | Google Scholar |
Fordyce G, Olchowy TWJ, Anderson A (2015) Hydration in non-suckling neonatal Brahman-cross calves. Australian Veterinary Journal 93, 214-220.
| Crossref | Google Scholar | PubMed |
Fordyce G, McCosker KD, Barnes TS, Perkins NR, O’Rourke PK, McGowan MR (2023) Reproductive performance of northern Australia beef herds. 6. Risk factors associated with reproductive losses between confirmed pregnancy and weaning. Animal Production Science 63, 365-377.
| Crossref | Google Scholar |
Franklin ST, Amaral-Phillips DM, Jackson JA, Campbell AA (2003) Health and performance of Holstein calves that suckled or were hand-fed colostrum and were fed one of three physical forms of starter. Journal of Dairy Science 86, 2145-2153.
| Crossref | Google Scholar | PubMed |
Funston RN, Larson DM, Vonnahme KA (2010) Effects of maternal nutrition on conceptus growth and offspring performance: implications for beef cattle production. Journal of Animal Science 88, E205-E215.
| Crossref | Google Scholar | PubMed |
Godden SM, Lombard JE, Woolums AR (2019) Colostrum management for dairy calves. Veterinary Clinics of North America: Food Animal Practice 35, 535-556.
| Crossref | Google Scholar | PubMed |
Goff JP (2000) Pathophysiology of calcium and phosphorus disorders. Veterinary Clinics of North America: Food Animal Practice 16, 319-337.
| Crossref | Google Scholar | PubMed |
Golher DM, Patel BHM, Bhoite SH, Syed MI, Panchbhai GJ, Thirumurugan P (2021) Factors influencing water intake in dairy cows: a review. International Journal of Biometeorology 65, 617-625.
| Crossref | Google Scholar |
Graef GM, Kerwin AL, Ferro LN, Ordaz-Puga S, Ryan CM, Westhoff TA, Barbano DM, Glosson KM, Zanzalari KP, Chapman JD, Overton TR (2021) Effect of prepartum DCAD strategy and level of dietary calcium on postpartum calcium status and performance of multiparous holstein cows. In ‘Cornell Nutrition Conference’, Ithaca, NY, USA. pp. 64–72. (Cornell University)
Greenwood PL (2021) An overview of beef production from pasture and feedlot globally, as demand for beef and the need for sustainable practices increase. Animal 15, 100295.
| Crossref | Google Scholar | PubMed |
Greenwood PL, Cafe LM (2007) Prenatal and pre-weaning growth and nutrition of cattle: long-term consequences for beef production. Animal 1, 1283-1296.
| Crossref | Google Scholar | PubMed |
Greenwood PL, Cafe LM, Hearnshaw H, Hennessy DW, Thompson JM, Morris SG (2006) Long-term consequences of birth weight and growth to weaning on carcass, yield and beef quality characteristics of Piedmontese- and Wagyu-sired cattle. Australian Journal of Experimental Agriculture 46, 257-269.
| Crossref | Google Scholar |
Gross JJ, Kessler EC, Bjerre-Harpoth V, Dechow C, Baumrucker CR, Bruckmaier RM (2014) Peripartal progesterone and prolactin have little effect on the rapid transport of immunoglobulin G into colostrum of dairy cows. Journal of Dairy Science 97, 2923-2931.
| Crossref | Google Scholar | PubMed |
Guiraldes E, Gall DG, Hamilton JR (1981) Effect of cortisone on postnatal development of ion transport in rabbit small intestine. Pediatric Research 15, 1530-1532.
| Crossref | Google Scholar | PubMed |
Guy MA, McFadden TB, Cockrell DC, Besser TE (1994) Regulation of colostrum formation in beef and dairy cows. Journal of Dairy Science 77, 3002-3007.
| Crossref | Google Scholar | PubMed |
Hogan LA, McGowan MR, Johnston SD, Lisle AT, Schooley K (2022) Suckling behaviour of beef calves during the first five days postpartum. Ruminants 2, 321-340.
| Crossref | Google Scholar |
Horst EA, Kvidera SK, Baumgard LH (2021) Invited review: the influence of immune activation on transition cow health and performance: a critical evaluation of traditional dogmas. Journal of Dairy Science 104, 8380-8410.
| Crossref | Google Scholar | PubMed |
Hough RL, McCarthy FD, Thatcher CD, Kent HD, Eversole DE (1990a) Influence of glucocorticoid on macromolecular absorption and passive immunity in neonatal lambs. Journal of Animal Science 68, 2459-2464.
| Crossref | Google Scholar | PubMed |
Hough RL, McCarthy FD, Kent HD, Eversole DE, Wahlberg ML (1990b) Influence of nutritional restriction during late gestation on production measures and passive immunity in beef cattle. Journal of Animal Science 68, 2622-2627.
| Crossref | Google Scholar | PubMed |
Hulbert LE, Moisá SJ (2016) Stress, immunity, and the management of calves. Journal of Dairy Science 99, 3199-3216.
| Crossref | Google Scholar | PubMed |
Hunt LP, McIvor JG, Grice AC, Bray SG (2014) Principles and guidelines for managing cattle grazing in the grazing lands of northern Australia: stocking rates, pasture resting, prescribed fire, paddock size and water points – a review. The Rangeland Journal 36, 105-119.
| Crossref | Google Scholar |
Immler M, Failing K, Gärtner T, Wehrend A, Donat K (2021) Associations between the metabolic status of the cow and colostrum quality as determined by Brix refractometry. Journal of Dairy Science 104, 10131-10142.
| Crossref | Google Scholar | PubMed |
Kennedy VC, Mordhorst BR, Gaspers JJ, Bauer ML, Swanson KC, Lemley CO, Vonnahme KA (2016) Supplementation of corn dried distillers’ grains plus solubles to gestating beef cows fed low-quality forage: II. Impacts on uterine blood flow, circulating estradiol-17β and progesterone, and hepatic steroid metabolizing enzyme activity. Journal of Animal Science 94, 4619-4628.
| Crossref | Google Scholar | PubMed |
Kennedy VC, Gaspers JJ, Mordhorst BR, Stokka GL, Swanson KC, Bauer ML, Vonnahme KA (2019) Late gestation supplementation of corn dried distiller’s grains plus solubles to beef cows fed a low-quality forage: III. Effects on mammary gland blood flow, colostrum and milk production, and calf body weights. Journal of Animal Science 97, 3337-3347.
| Crossref | Google Scholar | PubMed |
Kim HN, Godke RA, Hugh-Jones M, Olcott BM, Strain GM (1988) The ‘dummy calf’ syndrome in neonatal beef calves. Agri-Practice 9, 23-29.
| Google Scholar |
King AD, Pitman AJ, Henley BJ, Ukkola AM, Brown JR (2020) The role of climate variability in Australian drought. Nature Climate Change 10, 177-179.
| Crossref | Google Scholar |
Kume S-I, Tanabe S (1993) Effect of parity on colostral mineral concentrations of Holstein cows and value of colostrum as a mineral source for newborn calves. Journal of Dairy Science 76, 1654-1660.
| Crossref | Google Scholar | PubMed |
Ladeira MM, Schoonmaker JP, Swanson KC, Duckett SK, Gionbelli MP, Rodrigues LM, Teixeira PD (2018) Review: nutrigenomics of marbling and fatty acid profile in ruminant meat. Animal 12, s282-s294.
| Crossref | Google Scholar | PubMed |
Larson DM, Martin JL, Adams DC, Funston RN (2009) Winter grazing system and supplementation during late gestation influence performance of beef cows and steer progeny. Journal of Animal Science 87, 1147-1155.
| Crossref | Google Scholar | PubMed |
Lecce JG, Morgan DO (1962) Effect of dietary regimen on cessation of intestinal absorption of large molecules (closure) in the neonatal pig and lamb. The Journal of Nutrition 78, 263-268.
| Crossref | Google Scholar | PubMed |
LeMaster CT, Taylor RK, Ricks RE, Long NM (2017) The effects of late gestation maternal nutrient restriction with or without protein supplementation on endocrine regulation of newborn and postnatal beef calves. Theriogenology 87, 64-71.
| Crossref | Google Scholar | PubMed |
Little W, Collis KA, Gleed PT, Sansom BF, Allen WM, Quick AJ (1980) Effect of reduced water intake by lactating dairy cows on behaviour, milk yield and blood composition. The Veterinary Record 106, 547-551.
| Crossref | Google Scholar | PubMed |
Lopez AJ, Heinrichs AJ (2022) Invited review: the importance of colostrum in the newborn dairy calf. Journal of Dairy Science 105, 2733-2749.
| Crossref | Google Scholar | PubMed |
Mayer B, Doleschall M, Bender B, Bartyik J, Bősze Z, Frenyó LV, Kacskovics I (2005) Expression of the neonatal Fc receptor (FcRn) in the bovine mammary gland. Journal of Dairy Research 72, 107-112.
| Crossref | Google Scholar | PubMed |
McGee M, Earley B (2019) Review: passive immunity in beef-suckler calves. Animal 13, 810-825.
| Crossref | Google Scholar | PubMed |
McGee M, Drennan MJ, Caffrey PJ (2005) Effect of suckler cow genotype on milk yield and pre-weaning calf performance. Irish Journal of Agricultural and Food Research 44, 185-194.
| Google Scholar |
McGee M, Drennan MJ, Caffrey PJ (2006) Effect of age and nutrient restriction pre partum on beef suckler cow serum immunoglobulin concentrations, colostrum yield, composition and immunoglobulin concentration and immune status of their progeny. Irish Journal of Agricultural and Food Research 45, 157-171.
| Google Scholar |
McGuirk SM, Collins M (2004) Managing the production, storage, and delivery of colostrum. Veterinary Clinics of North America: Food Animal Practice 20, 593-603.
| Crossref | Google Scholar | PubMed |
Mleil S, Lassoued N, Salem HB, Rekik M (2012) Effect of water deprivation during pregnancy and lactation of Barbarine ewes on mammary secretions and lamb’s growth. In ‘Animal farming and environmental interactions in the Mediterranean region’. (Eds S Mleil, N Lassoued, H Ben Salem, M Rekik) pp. 115–120. (Wageningen Academic Publishers: Wageningen, Netherlands) doi:10.3920/978-90-8686-741-7_14
Morrison DG, Spitzer JC, Perkins JL (1999) Influence of prepartum body condition score change on reproduction in multiparous beef cows calving in moderate body condition. Journal of Animal Science 77, 1048-1054.
| Crossref | Google Scholar | PubMed |
Muller J, Prada e Silva L, Fordyce G (2022) High frequency of delayed milk delivery to neonates in tropical beef herds. Reproduction in Domestic Animals 57, 1176-1186.
| Crossref | Google Scholar | PubMed |
Neville MC, McFadden TB, Forsyth I (2002) Hormonal regulation of mammary differentiation and milk secretion. Journal of Mammary Gland Biology and Neoplasia 7, 49-66.
| Crossref | Google Scholar | PubMed |
Nicholson MJ (1987) The effect of drinking frequency on some aspects of the productivity of zebu cattle. The Journal of Agricultural Science 108, 119-128.
| Crossref | Google Scholar |
Nørgaard JV, Nielsen MO, Theil PK, Sørensen MT, Safayi S, Sejrsen K (2008) Development of mammary glands of fat sheep submitted to restricted feeding during late pregnancy. Small Ruminant Research 76, 155-165.
| Crossref | Google Scholar |
Ocak Yetisgin S, Şen U (2020) Resilience to drought in semi-desert sheep: effects of water restriction during pregnancy on placental efficiency in the Awassi breed. Animal Science Journal 91, e13494.
| Crossref | Google Scholar | PubMed |
Odde KG (1988) Survival of the neonatal calf. Veterinary Clinics of North America: Food Animal Practice 4, 501-508.
| Crossref | Google Scholar | PubMed |
Olsson K (2005) Fluid balance in ruminants: adaptation to external and internal challenges. Annals of the New York Academy of Sciences 1040, 156-161.
| Crossref | Google Scholar | PubMed |
O’Doherty JV, Crosby TF (1996) The effect of diet in late pregnancy on progesterone concentration and colostrum yield in ewes. Theriogenology 46, 233-241.
| Crossref | Google Scholar | PubMed |
O’Doherty JV, Crosby TF (1997) The effect of diet in late pregnancy on colostrum production and immunoglobulin absorption in sheep. Animal Science 64, 87-96.
| Crossref | Google Scholar |
Palmer EA, Vedovatto M, Oliveira RA, Ranches J, Vendramini JMB, Poore MH, Martins T, Binelli M, Arthington JD, Moriel P (2022a) Timing of maternal supplementation of dried distillers grains during late gestation influences postnatal growth, immunocompetence, and carcass characteristics of Bos indicus-influenced beef calves. Journal of Animal Science 100, skac022.
| Crossref | Google Scholar |
Palmer EA, Vedovatto M, Oliveira RA, Ranches J, Vendramini JMB, Poore MH, Martins T, Binelli M, Arthington JD, Moriel P (2022b) Effects of maternal winter vs. year-round supplementation of protein and energy on postnatal growth, immune function, and carcass characteristics of Bos indicus-influenced beef offspring. Journal of Animal Science 100, skac003.
| Crossref | Google Scholar |
Poppi DP, Quigley SP, Silva TACCd, McLennan SR (2018) Challenges of beef cattle production from tropical pastures. Revista Brasileira de Zootecnia 47, e20160419.
| Crossref | Google Scholar |
Raboisson D, Trillat P, Cahuzac C (2016) Failure of passive immune transfer in calves: a meta-analysis on the consequences and assessment of the economic impact. PLoS ONE 11, e0150452.
| Crossref | Google Scholar | PubMed |
Read MVP, Engels EAN, Smith WA (1986a) Phosphorus and the grazing ruminant. 3. Rib bone samples as an indicator of the P status of cattle. South African Journal of Animal Science 16, 13-17.
| Google Scholar |
Read MVP, Engels EAN, Smith WA (1986b) Phosphorus and the grazing ruminant. 2. The effects of supplementary P on cattle at Glen and Armoedsvlakte. South African Journal of Animal Science 16, 7-12.
| Google Scholar |
Redifer CA, Wichman LG, Meyer AM (2022) 68 late gestational nutrient restriction decreases blood flow to the mammary gland in lactating primiparous beef females. Journal of Animal Science 100, 26.
| Crossref | Google Scholar |
Riley DG, Chase CC, Jr, Olson TA, Coleman SW, Hammond AC (2004) Genetic and nongenetic influences on vigor at birth and preweaning mortality of purebred and high percentage Brahman calves. Journal of Animal Science 82, 1581-1588.
| Crossref | Google Scholar | PubMed |
Robinson DL, Cafe LM, Greenwood PL (2013) Meat science and muscle biology symposium: developmental programming in cattle: consequences for growth, efficiency, carcass, muscle, and beef quality characteristics. Journal of Animal Science 91, 1428-1442.
| Crossref | Google Scholar | PubMed |
Sasaki M, Davis CL, Larson BL (1976) Production and turnover of IgG1 and IgG2 immunoglobulins in the bovine around parturition. Journal of Dairy Science 59, 2046-2055.
| Crossref | Google Scholar | PubMed |
Schatz TJ, McCosker KD, Heeb C (2023) Phosphorus supplementation improves the growth and reproductive performance of female Brahman cattle grazing phosphorus-deficient pastures in the Victoria River District, Northern Territory, Australia. Animal Production Science 63, 544-559.
| Crossref | Google Scholar |
Schuler G, Fürbass R, Klisch K (2018) Placental contribution to the endocrinology of gestation and parturition. Animal Reproduction 15, 822-842.
| Crossref | Google Scholar | PubMed |
Sejian V, Bhatta R, Gaughan JB, Dunshea FR, Lacetera N (2018) Adaptation of animals to heat stress. Animal 12, s431-s444.
| Crossref | Google Scholar | PubMed |
Shearer J, Mohammed HO, Brenneman JS, Tran TQ (1992) Factors associated with concentrations of immunoglobulins in colostrum at the first milking post-calving. Preventive Veterinary Medicine 14, 143-154.
| Crossref | Google Scholar |
Silva LFP, Muller J, Cavalieri J, Fordyce G (2022) Immediate prepartum supplementation accelerates progesterone decline, boosting passive immunity transfer in tropically adapted beef cattle. Animal Production Science 62, 983-992.
| Crossref | Google Scholar |
Sordillo LM (2016) Nutritional strategies to optimize dairy cattle immunity. Journal of Dairy Science 99, 4967-4982.
| Crossref | Google Scholar | PubMed |
Staley TE, Corley LD, Bush LJ, Wynn Jones E (1972) The ultrastructure of neonatal calf intestine and absorption of heterologous proteins. The Anatomical Record 172, 559-579.
| Crossref | Google Scholar | PubMed |
Sullivan ML, Bradford BJ (2011) Viable cell yield from active dry yeast products and effects of storage temperature and diluent on yeast cell viability. Journal of Dairy Science 94, 526-531.
| Crossref | Google Scholar |
Summer A, Lora I, Formaggioni P, Gottardo F (2019) Impact of heat stress on milk and meat production. Animal Frontiers 9, 39-46.
| Crossref | Google Scholar | PubMed |
Tao S, Monteiro APA, Thompson IM, Hayen MJ, Dahl GE (2012) Effect of late-gestation maternal heat stress on growth and immune function of dairy calves. Journal of Dairy Science 95, 7128-7136.
| Crossref | Google Scholar | PubMed |
Taverne MAM, Bevers MM, Van der Weyden GC, Dieleman SJ, Fontijne P (1988) Concentration of growth hormone, prolactin and cortisol in fetal and maternal blood and amniotic fluid during late pregnancy and parturition in cows with cannulated fetuses. Animal Reproduction Science 17, 51-59.
| Crossref | Google Scholar |
Tucker HA (2000) Hormones, mammary growth, and lactation: a 41-year perspective. Journal of Dairy Science 83, 874-884.
| Crossref | Google Scholar | PubMed |
Valk H, Šebek LBJ, Beynen AC (2002) Influence of phosphorus intake on excretion and blood plasma and saliva concentrations of phosphorus in dairy cows. Journal of Dairy Science 85, 2642-2649.
| Crossref | Google Scholar | PubMed |
Wall E, McFadden T (2012) Regulation of mammary development as it relates to changes in milk production efficiency. In ‘Milk production-an up-to-date overview of animal nutrition, management and health’. (Ed. N Chaiyabutr) pp. 258–288. (IntechOpen) doi:10.5772/50777
Weaver DM, Tyler JW, VanMetre DC, Hostetler DE, Barrington GM (2000) Passive transfer of colostral immunoglobulins in calves. Journal of Veterinary Internal Medicine 14, 569-577.
| Crossref | Google Scholar | PubMed |
Williams LR, Jackson EL, Bishop-Hurley GJ, Swain DL (2017) Drinking frequency effects on the performance of cattle: a systematic review. Journal of Animal Physiology and Animal Nutrition 101, 1076-1092.
| Crossref | Google Scholar | PubMed |
Williams LR, Fox DR, Bishop-Hurley GJ, Swain DL (2019) Use of radio frequency identification (RFID) technology to record grazing beef cattle water point use. Computers and Electronics in Agriculture 156, 193-202.
| Crossref | Google Scholar |
Winchester CF, Morris MJ (1956) Water intake rates of cattle. Journal of Animal Science 15, 722-740.
| Crossref | Google Scholar |
Winger K, Gay CC, Besser TE (1995) Immunoglobulin G1 transfer into induced mammary secretions: the effect of dexamethasone. Journal of Dairy Science 78, 1306-1309.
| Crossref | Google Scholar | PubMed |
Yuan K, Mendonça LGD, Hulbert LE, Mamedova LK, Muckey MB, Shen Y, Elrod CC, Bradford BJ (2015) Yeast product supplementation modulated humoral and mucosal immunity and uterine inflammatory signals in transition dairy cows. Journal of Dairy Science 98, 3236-3246.
| Crossref | Google Scholar | PubMed |
Zanello G, Meurens F, Berri M, Chevaleyre C, Melo S, Auclair E, Salmon H (2011) Saccharomyces cerevisiae decreases inflammatory responses induced by F4+ enterotoxigenic Escherichia coli in porcine intestinal epithelial cells. Veterinary Immunology and Immunopathology 141, 133-138.
| Crossref | Google Scholar | PubMed |
Zaworski EM, Shriver-Munsch CM, Fadden NA, Sanchez WK, Yoon I, Bobe G (2014) Effects of feeding various dosages of Saccharomyces cerevisiae fermentation product in transition dairy cows. Journal of Dairy Science 97, 3081-3098.
| Crossref | Google Scholar | PubMed |