Platypus fyke-netting methods: a review of recommended field protocols and options for assessing population abundance
Melody Serena A * , Geoff A. Williams A , Chris Bloink B and Dana Dekkers CA
B
C
Abstract
Fyke nets are the preferred type of equipment used for platypus (Ornithorhynchus anatinus) live-trapping surveys in shallow watercourses. However, numerous factors other than abundance may affect the frequency of platypus captures, including when, where and how nets are set, stream discharge and entry into nets by rakali (Hydromys chrysogaster), which create exit holes that are then also available to a platypus. A threefold difference in the mean monthly frequency of platypus captures in fyke nets is also evident across the year. To limit net evasion, it makes sense to reduce net visibility and minimise opportunities for animals to squeeze under, climb over or walk around nets. Time-lapse cameras can also be deployed at fyke-netting sites to estimate how many individuals evade capture. To protect platypus welfare and the welfare of non-target species held in fyke nets, it is important to ensure that captured animals have access to air, to check nets frequently throughout the time they are set and to release bycatch when first encountered. Fyke-netting studies typically generate sparse platypus population data, so care is needed when selecting and interpreting platypus abundance metrics generated through mark–recapture modelling or use of indices.
Keywords: animal welfare, aquatic wildlife survey methods, CPUE, monotreme, net avoidance behaviour, Ornithorhynchidae, platypus population monitoring, population abundance index, time-lapse cameras, trap shyness.
Introduction
Reliable live-capture techniques for mammals are often needed to enable tissue samples to be obtained for veterinary, genetic or physiological studies, fit tracking devices, mark animals to assess population size and turnover, or assess demographic attributes. In the case of the platypus (Ornithorhynchus anatinus), fyke nets are the type of equipment mainly used to capture animals for research or monitoring purposes in relatively shallow water bodies such as creeks or along the margins of lakes or backwaters (Grant 2012; Serena and Williams 2012).
By definition, fyke nets have a long cylindrical body fabricated from netting and supported by a series of metal rings. Distally-pointing funnels and/or sleeves are located within the cylinder to prevent an animal from travelling back towards the entrance, and one or more vertical panels of netting (known as wings or leaders) extend out from the entrance to direct target organisms inside (Portt et al. 2006; He et al. 2021). Though easily transported, fyke nets are fixed in position while deployed; as passive sampling equipment, their effectiveness varies with how often target animals encounter and enter a net and how frequently they escape (Portt et al. 2006).
Fyke nets are routinely used to characterise abundance, body size, demographic attributes or movement of freshwater fish, especially eels (Anguilla spp.) (e.g. Jellyman and Graynoth 2005; Beentjes et al. 2006; Jones and O’Connor 2017; MacNamara et al. 2017). Caveats on the use and interpretation of fish population data derived from fyke-netting studies include the fact that capture frequencies are sometimes low and often variable (contributing to low statistical power), and may be influenced by diverse environmental factors (e.g. ambient temperature, lunar phase, channel morphology, flow) as well as fish size, net avoidance behaviour and migration (Jellyman 1991; Krueger et al. 1998; Jellyman and Graynoth 2005; Portt et al. 2006; Bevacqua et al. 2009).
Similarly, the frequency of platypus captures in fyke nets is predicted to vary with how, where and when nets are set (Serena and Williams 2012), and may be affected by net-avoidance behaviour (Griffiths et al. 2013) or behaviour of other species, for example, rakali or Australian water-rat (Hydromys chrysogaster) (Grant 2012). Platypus population density is typically only 1–4 animals km−1 in small lotic systems (Serena 1994; Gardner and Serena 1995b; Serena and Williams 1997; Serena et al. 2014), thereby limiting the potential number of captures in fyke nets and placing a premium on adopting protocols to maximise netting success. Sparse capture data also mean that analytical methods must be applied carefully to ensure that population estimates are statistically valid. Platypus fyke-netting studies (and those involving other species in platypus habitats) must also consider welfare issues arising from the platypus’s air-breathing and homeothermic nature, along with its ability to climb over or walk around nets.
This review aims to contribute to improved platypus fyke-netting outcomes by (1) considering how various factors may affect how often a platypus encounters, enters and/or escapes from a fyke net, (2) identifying issues potentially affecting platypus welfare (and that of bycatch) in fyke nets and (3) discussing the options currently available for estimating platypus abundance from fyke-netting data. Along with information garnered from the scientific literature, some new behavioural findings concerning platypus behaviour recorded by cameras deployed at fyke-netting sites are presented.
Identifying platypus behaviours used to evade fyke nets
Two fyke nets (respectively facing upstream and downstream) are typically deployed per site in platypus surveys (Serena 1994; Gardner and Serena 1995b; Serena and Pettigrove 2005; Gust and Griffiths 2011; Serena and Williams 2012; Serena et al. 2023), though other configurations are sometimes utilised (Bino et al. 2021; Hawke et al. 2021a). To discourage platypus movement under, over or around nets, heavy objects (rocks, bricks) are positioned to eliminate gaps between the channel bed and netting, sticks (sourced mainly on-site) are used to hold the top margins of wings well above the water, and the distal end of wings are either stretched laterally along shallow banks for ≥1 m beyond the water’s edge or pinned firmly to vertical banks (Serena and Williams 2012) (Fig. 1).
A pair of fyke nets set with wings stretched laterally onto land to reduce the likelihood that a platypus walks around them. Note how the main body of each net is angled across the channel to help direct animals into the facing net.
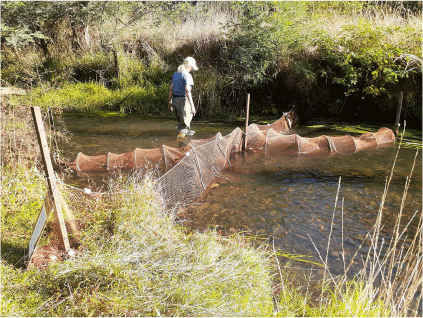
To test whether the measures described above are sufficient to deter a platypus from bypassing fyke nets and (if not) characterise behaviours contributing to net evasion, the authors placed time-lapse cameras at four of five sites where platypus fyke nets were set in Monbulk Creek (Belgrave, Victoria) on 25–26 September 2023 and all four sites where platypus fyke nets were set in the Steavenson/Little Steavenson Rivers (Buxton, Victoria) on 10–11 April 2024. Protocols otherwise followed those of a normal platypus fyke-netting survey; for example, nets were checked at regular intervals through the night and standard procedures were used to remove, handle and mark/identify captured animals (Serena et al. 2022). Three camera models were deployed in 2023 (Brinno MAC200DN, GardePro E8P, Nextech QC 8043 1080p) and one model in 2024 (X-Trail 4CR 24MP HD). Cameras (1–2 per site) were attached to stakes on the banks and recorded images at 4-s intervals (GardePro E8P) or 5-s intervals (other models) from dusk to dawn, with nocturnal lighting provided by IR spotlights (850 nm, Brinno MAC200DN) or built-in flashes (940 nm, other models). Platypus fyke-netting sessions had previously been conducted at Belgrave on 46 occasions (from February 1996 to April 2023), but never in the Steavenson/Little Steavenson system.
Factors affecting platypus fyke-netting results
Temporal variation in platypus activity
Although platypus activity tends to be mainly nocturnal, animals sometimes forage during the day (Grigg et al. 1992; Serena 1994; Gust and Handasyde 1995; Gardner and Serena 1995b; Otley et al. 2000; Bethge et al. 2009; Bino et al. 2018; Hawke et al. 2021b). After setting fyke nets in the afternoon at 616 sites sampled in 403 live-trapping sessions from January through December, Serena and Williams (2012) reported that only 0.2% of captures occurred before sunset, perhaps because nets are readily visible in daylight. Based on the same data set, a threefold difference was evident in the mean monthly frequency of adults, subadults and juveniles captured in fyke nets across the year (Fig. 2). A disproportionately high frequency of captures also occurred in the first half of the night (juveniles, 73%; older animals, 63%), similar to the pattern reported for platypus captures in rectangular mesh (or gill) nets set overnight (Grant 1992). This could potentially be due to a range of factors, including reduced platypus activity in the second half of the night (Serena and Williams 2012).
Mean monthly platypus captures (N of captures site−1 night−1) for adult/subadult males (black), adult/subadult females (grey) and juveniles (white) in Victoria. Juveniles are assumed to hatch on 1 Nov. and become subadults when 1 year old (Williams et al. 2013). See text for sampling effort.
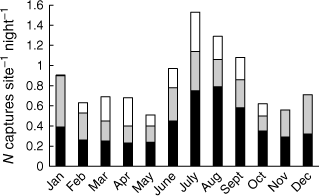
Distance between consecutive netting sites and number of sites
Platypus capture frequency is predicted to be sensitive to the distance between consecutive fyke-netting sites. Although reducing this distance will increase the likelihood that all animals in a given area encounter nets on a given night, it will also reduce the length of area sampled unless the number of sites is increased. To optimise both the proportion of a population that encounters nets and (for a given number of sites) the length of area surveyed, spacing between consecutive sites should in theory be a little less than the average distance that a platypus is expected to travel overnight. In practice, platypus home ranges typically encompass several 100 m to several kilometres of stream or river channel when assessed over a few weeks (Serena 1994; Gust and Handasyde 1995; Gardner and Serena 1995b; Serena et al. 1998, 2001; Bino et al. 2018; Thomas et al. 2019), with 26–58% (McLeod 1993) or 24–70% (Serena 1994) of a home range used on any given day. However, both the number of netting sites and their spacing (e.g. 5–9 sites set at intervals of 0.8–1.5 km in urban streams: Serena and Pettigrove 2005) will also typically be constrained by the distribution of access points along a water course and the time needed to set nets carefully and check them overnight (see below).
Stream discharge
Noting that fewer platypus captures were recorded in fyke nets set in exceptionally wet or dry years, Serena et al. (2014) postulated that this could be due to changes in habitat use or behaviour (e.g. heightened territorial defence). However, it could also reflect variation in fyke-netting efficiency: high discharge will tend to displace anchoring rocks, cause internal sleeves to be held open (especially if nets are not set at an angle to the current) and carry leafy debris that accumulates along the net, making it more visible. Conversely, low discharge will likely make internal sleeves and funnels more evident to a platypus as it approaches and passes through them, expose more netting above the water (again contributing to visibility) and increase opportunities for a platypus to stand upright in the channel before climbing over netting.
Activity of non-target vertebrates
After entering a fyke net, a rakali will characteristically chew a hole in netting to escape, which can then be used for the same purpose by a platypus. Attempts to intercept rakali before they damage nets by setting baited metal cage traps on the banks have met with limited success (Grant 2012). However, camera images suggest that rakali may sometimes be lured into nets by the presence of fish or crustaceans, both of which are common dietary items (Woollard et al. 1978) (Fig. 3). Releasing bycatch in a timely manner may, therefore, reduce the number of holes made by rakali.
Platypus net evasion
Active avoidance of gill nets has been posited to contribute to fewer platypus captures occurring at sites subject to greater netting effort (Connolly and Obendorf 1998). In the case of fyke nets, a 10-year study in which two annual survey sessions were typically conducted in creeks, with nets set at mean intervals of 0.8–1.2 km, resulted in 58–65% of marked adults being captured on average per year (Serena et al. 2014). Though some animals may have failed to encounter nets in some years, others are likely to have evaded capture by avoiding or bypassing nets. Platypus fyke nets are unbaited (see below) and hence no reward accrues to an animal to offset negative aspects of the experience of being held in a net and handled. An adult male fitted with an acoustic transmitter has been recorded bypassing three pairs of fyke nets while travelling both upstream and downstream (Griffiths et al. 2013).
Behaviours contributing to platypus net evasion were documented by cameras deployed in both the Steavenson/Little Steavenson Rivers (where platypus fyke nets had never previously been set) and Monbulk Creek (where they had). In the Steavenson system, one animal halted after entering the front chamber of a net and eventually escaped through the entrance, requiring just over 30 min to find the inset opening (Fig. 4). Other sequences recorded a platypus climbing over a netting wing set in very shallow water (Fig. 5) and another escaping through a hole previously created by a rakali. At Monbulk Creek, animals were filmed entering a net and then leaving through the entrance on at least two occasions (taking as little as 18 s to do so, suggesting prior knowledge of the net’s design). These same sequences depicted an animal repeatedly trying to push under a netting wing (Fig. 6) or climbing the bank to investigate part of the wing (within 1 m of the water’s edge) before returning to the channel. Other sequences showed a platypus turning around as it approached (and presumably saw) a net and then backtracking down the channel (Fig. 7) or escaping through a hole made by a rakali. Based on when and where animals were detected and their direction of travel, three individuals are presumed to have evaded nets in the Steavenson system, with three being captured. At least two animals evaded nets along Monbulk Creek, with four being captured. Recommended actions to reduce (though not eliminate) platypus evasion of fyke nets are listed in Table 1.
A platypus (circled in red) seeks to escape from the first internal chamber of a fyke net set in the Steavenson River.
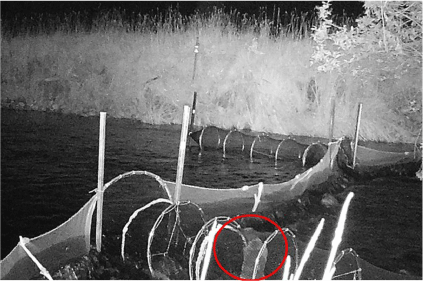
A platypus (circled in red) stands upright in the channel with its bill tip over the top of a wing. It presumably scrambled over successfully, as its image was not subsequently recorded. Note camera on far bank (circled in blue).
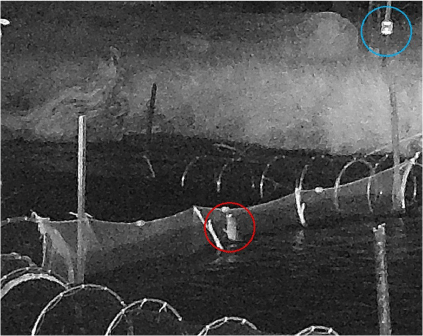
A platypus (circled in red) tries unsuccessfully to push under the wing of a fyke net set in Monbulk Creek. Note floating leafy material accumulated along the wing at the water surface.
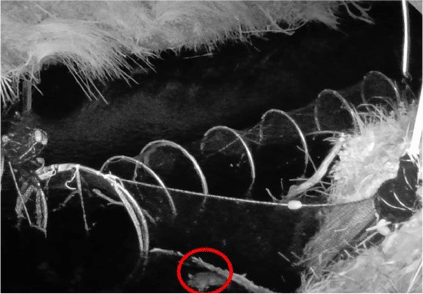
A platypus (circled in red) backtracks down Monbulk Creek after reaching but not entering a fyke net.
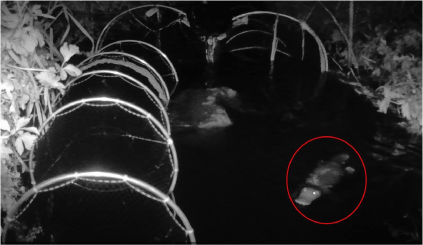
Behaviour | Action | |
---|---|---|
Find or create gaps by pushing under the frame or netting. | Position sizable rocks, bricks, etc. in at least one continuous line (ideally two) to block all possible gaps below a net and prevent the frame and netting from shifting position. | |
Travel around the distal end of a net. | Avoid sites with undercut banks; stretch net wings laterally across land for ≥1 m or pin them firmly to vertical banks. | |
Climb over netting or frame. | Set nets at sites where water is too deep for a platypus to stand up readily in the channel; ensure netting is upright. | |
Stop in first net chamber and exit through entrance. | Ensure that internal funnels and sleeves are submerged deeply enough that animals swim through with their eyes closed. | |
Backtrack down channel before entering net. | Reduce visibility of nets, e.g. set where water is reasonably deep, remove leaf litter from wings over the period that nets are set, avoid setting nets on strongly moonlit nights or at sites where extensive frothy bubbles build up behind nets. | |
Escape through rakali hole. | Release rakali prey species (fish, decapod crustaceans) when first encountered; inspect nets for holes and make running repairs with precut lengths of twine. |
Fyke net design
Fyke nets may vary with respect to their length, mesh size, the dimensions and spacing of internal funnels and sleeves, supporting ring shape, and so on. Though it is quite possible that elements of net design can affect platypus netting success, this hypothesis currently remains untested.
Risks to platypus welfare in fyke nets
Fyke-netting surveys entail a range of potential risks to platypus welfare, as described below. Recommended measures to mitigate platypus risks are listed in Table 2.
Risk | Recommended action | |
---|---|---|
Drowning | Securely suspend the end chamber of nets (ideally all chambers) partly above the water surface; place the end chamber of nets in relatively slow-moving water and/or at an angle to the main current; remove nets from water if water levels begin to rise. | |
Hunger | Release a platypus back to the wild ASAP (recommended maximum cumulative holding time in a net and then in a bag = 5 h, though also see below). | |
Stress | Release a platypus back to the wild ASAP (recommended maximum cumulative holding time in a net and then in a bag = 5 h, though also see below). | |
Litter | Check animals carefully for possible litter entanglement (using finger tips to probe for fishing line); carry small scissors and forceps to remove litter items before releasing the animal back to the wild ASAP. | |
Hypothermia | Check nets at intervals of ≤2 h if water temperature at dusk is <15°C, i.e. the presumed lower limit of the platypus’s thermoneutral zone. | |
Hyperthermia | Do not hold captured animals in cloth bags at air temperatures >28°C or in direct sunlight. | |
Male aggression | Check nets at intervals of ≤2 h during the main platypus breeding season. | |
Disrupted nest attendance | Check nets at intervals of ≤2 h during and soon after the main platypus breeding season; release adult females back to the wild ASAP. | |
Predation | Do not stretch the cylindrical body of a net across land or very shallow water. In waters supporting large aquatic predators, check nets at intervals of ≤2 h in months when small juveniles may be trapped. | |
Misadventure | Visit all nets routinely before dark to check for signs of human interference. |
Drowning
An active platypus can hold its breath for only 2.3 min (McLeod 1993; Bethge et al. 2003) or 2.6 min (Grant et al. 2004b). The risk that a platypus will drown in a completely submerged fyke net is correspondingly high – for example, if water levels rise after nets have been set due to increased outflow from a dam or unexpected rainfall, especially if the latter occurs in an urban catchment (Shuster et al. 2005).
Hunger and reduced food intake
A platypus typically feeds for 8–16 h a day (e.g. see papers cited in Serena and Williams 2012), with a gut transit time (stomach to colon) of just 5 h (Booth and Connolly 2008). Because the platypus diet mainly comprises live benthic insects (Faragher et al. 1979; McLachlan-Troup et al. 2010; Marchant and Grant 2015; Hawke et al. 2022), no technique has yet been developed to bait fyke nets or otherwise feed a platypus while it is confined in a net or capture bag.
Physiological stress
Plasma concentrations of glucocorticoids (adrenaline and noradrenaline) rise sharply in the first 30 min after a platypus is captured in a net, eventually increasing by more than an order of magnitude by ~12 h after capture (McDonald et al. 1992; Handasyde et al. 2003). Baseline glucocorticoid concentrations and the magnitude of post-capture glucocorticoid response are both greater during the breeding season compared to other months (Handasyde et al. 2003).
Litter entanglement
Plastic, rubber or metal litter items are often found encircling a captured platypus’s neck or torso, frequently in association with abraded skin or deeper lesions (Serena and Williams 1998, 2022). Though litter entanglement is not caused by netting, it plausibly exacerbates the stress experienced by captured animals.
Hypothermia and hyperthermia
The platypus is normally a competent homeotherm with a body temperature close to 32°C (Grant and Dawson 1978a; Grant 1983; Grigg et al. 1992). However, extreme hypothermia (resulting in near immobility) can develop quite rapidly when two or more animals occupy the same fyke net in water <15°C (the presumed lower limit of the platypus’s thermoneutral zone: Bethge et al. 2001) (MS, GAW and CB, pers. obs.). This likely happens when the insulating air layer in platypus underfur is disrupted as animals interact closely in nets (Grant and Dawson 1978a).
A platypus may overheat when held in a capture bag due to its naturally low body temperature and limited ability to cool itself by sweating (Grant and Dawson 1978b). Noting that animals become ‘noticeably lethargic’ in captivity at ambient temperatures above 29°C, with death sometimes eventuating above 34°C, Booth and Connolly (2008) recommend that this species should not be kept at air temperatures >27°C.
Inter-male aggression
Agonistic male interactions are probably rarely fatal in the wild, but mortality may ensue in captivity (Grant 2007). A similar outcome could arise if two adult males occupy the same net, especially during the platypus breeding season when androgens and venom production reach their annual peak (Temple-Smith 1973). This is most likely to occur in late winter and spring on the Australian mainland (De-La-Warr and Serena 1999; Temple-Smith and Grant 2001; Easton et al. 2008) or late spring and early summer in Tasmania (Temple-Smith and Grant 2001; Roberts and Serena 2024).
Disruption of egg and early brood attendance by females
Adult females spend minimal time outside the burrow while incubating eggs and only gradually increase their foraging hours after eggs hatch, resuming normal activity ~28–36 days after egg-laying, or ~44–52 days after mating (Thomas et al. 2020). Holding captured adult females for a lengthy period during incubation or soon after eggs hatch may, therefore, greatly increase the risk of reproductive failure.
Misadventure and predation
A platypus reportedly drowned in a fyke net after an unknown visitor untied the distal end from its supporting stake and failed to refasten it properly (R. Armistead, Cesar Australia, pers. comm.). While contained in a net, a platypus may potentially be attacked by rakali or other sizable aquatic predators such as long-finned eels (Anguilla reinhardtii) or by terrestrial predators such as foxes (Vulpes vulpes) (particularly if the section of a fyke net that contains a platypus is stretched across very shallow water or dry land).
Risks to welfare of non-target vertebrates held in fyke nets
Non-target vertebrates likely to enter platypus fyke nets include rakali, waterbirds, fish and (more rarely) turtles. Associated welfare risks are described below, with recommended mitigating actions listed in Table 3.
Taxon | Risk | Recommended action | |
---|---|---|---|
Rakali, waterbird, turtle | Drowning | Securely suspend the end chamber of nets (ideally all chambers) partly above the water surface. | |
Waterbird, fish, decapod crustacean | Predation | Check all nets routinely before dark; release from nets whenever nets are checked. | |
Fish, decapod crustacean | Become wedged or tangled in netting | Release by gently disentangling or pushing individuals through netting. | |
Fish | Interference with migratory movement | Avoid setting nets in streams of known importance for migratory fish (particularly threatened species) if flow has recently risen in a period when movement is otherwise likely; remove nets from water if a substantial migratory or spawning event by any species appears to be in progress. |
Rakali/water-rat
Like the platypus, rakali are at risk of drowning in submerged fyke nets (Beumer et al. 1981; Williams and Serena 2018), though they can (and generally do) escape from partly elevated nets by chewing through netting. Rakali can also become hypothermic in winter, with body temperature dropping ~8°C within 1–3 h of entering 15°C water (Fanning and Dawson 1980). However, only small juveniles typically remain in fyke nets for any length of time, and litters mainly appear in spring and summer in temperate regions (McNally 1960; Gardner and Serena 1995a; Leigh and Breed 2020). The actual risk that a rakali becomes hypothermic in a fyke net is, therefore, low.
Waterbirds
Ducklings often enter platypus fyke nets, sometimes with their mother (who invariably remains nearby) (MS, GAW and CB, pers. obs.). Ducklings may be killed in nets by rakali, large predatory fish or even large freshwater crayfish (e.g. Euastacus kershawi: MS and GAW, pers. obs.). Rakali are capable of killing unconfined adult waterbirds up to the size of a musk duck (Biziura lobata) (Woollard et al. 1978), and have been known to kill an adult pacific black duck (Anas superciliosa) in a fyke net (CB, pers. obs.).
Fish and decapod crustaceans
Fish are generally unharmed inside a fyke net (Krueger et al. 1998; Portt et al. 2006) but, like crustaceans, may be killed by rakali, predatory fish or (rarely) a male platypus, or sustain injuries after becoming wedged or tangled in netting (Portt et al. 2006). Because platypus fyke nets aim to block the entire channel, they can potentially interrupt migratory movements by fish that move substantial distances to spawn or otherwise complete their life cycle. However, such movements are often (though not invariably) triggered by increased flow (O’Connor et al. 2005; Reinfelds et al. 2013; Amtstaetter et al. 2016), which also typically provides suboptimal conditions for platypus fyke-netting (see above).
Inferring platypus abundance from fyke-netting data
Spatial or temporal variation in platypus populations may be evaluated without estimating population size, as exemplified by Coleman et al. (2022). We focus here on the narrower topic of what methods are currently available to assess platypus abundance (either in absolute terms or by generating a reliable index) from fyke-netting data.
Population (mark–recapture) modelling
Statistical population-estimation models using mark–recapture data are likely to yield relatively unbiased abundance estimates if models accurately reflect population attributes (McKelvey and Pearson 2001). This can be difficult to achieve when data are sparse, though problems arising from low sample size may sometimes be corrected by combining sparse data (White 2005). As a rule of thumb, McKelvey and Pearson (2001) have suggested that statistical estimation procedures should ideally utilise ≥100 capture records, though also noting that this may vary with the reliability of model-selection algorithms. Alternatively, sampling efficiency and statistical power may be improved (both in model-based studies of abundance and the index-based approaches described below) by applying techniques to assess sampling bias and optimise study design (Gwinn et al. 2011, 2016). Imperfect detection in platypus fyke-netting studies may also be addressed by adopting additional sampling methodologies, such as eDNA assays (Lugg et al. 2018) or time-lapse cameras (Roberts and Serena 2024). However, care is then needed to discriminate between findings from occupancy modelling (using data sets in which individuals are not identified, such as those generated by eDNA and most camera studies) and population estimation models: the relationship between occupancy and abundance is positive but not necessarily linear (Steenweg et al. 2018), and occupancy and abundance models can yield different predictive outcomes for a given system (Dibner et al. 2017).
Statistical approaches that have been applied to platypus fyke-netting data include conditional likelihood closed population models and updated Jolly–Seber open population models (Furlan et al. 2012), the Chao open population estimator developed and tested by Huggins et al. (2018), albeit using data derived from a population deemed to be closed (Furlan et al. 2013), and maximum likelihood closed population models (Hawke et al. 2021a). In practice, these studies all relied on samples comprising <100 records and generally yielded abundance estimates characterised by relatively low precision as inferred from 95% confidence limits or the magnitude of standard deviations relative to estimated values (Table 4). The only study to evaluate a procedure’s performance concluded that the Chao open population estimator was prone to negative bias and sensitive to population variation over a time scale corresponding to three consecutive sampling periods (Huggins et al. 2018).
Study | Duration | N captures | N individuals | Abundance | CL | |
---|---|---|---|---|---|---|
Furlan et al. (2012) | 21 months | 40 (F), 52 (M) | 20 (F), 24 (M) | 24 (F), 32 (M) | 18–30 (F), 20–45 (M) | |
Huggins et al. (2018) | 18 years | 82 | 28 | ~8–16 annually | ~2–8 (lower) to ~24–50 (upper) | |
Hawke et al. (2021a) | 5 months | 20 | 18 | 20 ± 12 A, 11 ± 2 B | N/A |
Catch per unit effort
Catch per unit effort (CPUE) is an easily calculated index of population abundance in which the number of captures is divided by associated trapping effort. In the case of fish studies, CPUE is usually expressed as the mean number of captures recorded per net deployed in a given time period (Portt et al. 2006). This approach implicitly assumes that nets act independently of each other. In contrast, platypus fyke-netting typically aims to block the channel by using two nets as an integrated sampling unit; adding nets to a given set may increase captures but is not expected to do so in a directly proportional manner. Accordingly, most platypus fyke-netting studies have reported CPUE as the mean number of captures site−1 night−1 (Serena and Pettigrove 2005; Serena and Williams 2012; Bino et al. 2021; Serena et al. 2023) or mean number of captures site−1 h−1 (Koch et al. 2006), excluding recaptures of a given individual occurring in a given night after it is released partway through a survey session. The decision to include captures of first-year juveniles when calculating platypus CPUE will partly depend on a study’s temporal design, given that juveniles emerge as a cohort and cannot (especially in the case of young females) be reliably identified in all months (Williams et al. 2013). Furthermore, given that relatively few juveniles (<25%) typically settle in their natal population and survive to adulthood (Grant 2004; Grant et al. 2004a; Serena et al. 2014), first-year juveniles should normally be excluded if CPUE aims to assess resident population size or platypus carrying capacity. Lastly, given that platypus reproductive success is known to be affected by discharge (Serena et al. 2014; Serena and Grant 2017), lumping juveniles with older animals may cause CPUE to vary with rainfall or seasonal flow even if the number of resident animals remains unchanged.
CPUE is subject to bias unless the target species is uniformly distributed and equally detectable across space and time, thereby maintaining a constant and proportional relationship between CPUE and true abundance (Maunder et al. 2006; Wiewel et al. 2009; Watkins et al. 2010; Gwinn et al. 2016). In practice, these assumptions are unlikely to be met given that numerous factors can influence platypus catchability (this paper) and/or affect the spatial distribution of platypus activity (e.g. Serena et al. 2001). Even small changes in detectability may lead to very misleading findings concerning the extent to which abundance is deemed to vary temporally (Hangsleben et al. 2013). Although the predictive utility of CPUE can sometimes be improved by increased sampling (Wiewel et al. 2009), caution is warranted before augmenting platypus sample size by amalgamating data from different areas, given that platypus numbers may vary asynchronously in populations inhabiting neighbouring watersheds (Serena et al. 2014). If platypus fyke-netting cannot generate robust data at the desired spatial scale due to factors such as high cost or trap-avoidance, it may be best to accept that associated CPUE values are at best imprecise, and simply assign populations to two or more abundance classes (Serena and Pettigrove 2005).
Counts
The number of unique captured individuals can potentially serve as a valid abundance metric when sparse data hinders application of more sophisticated techniques (Slade and Blair 2000; McKelvey and Pearson 2001). Although counts may fail to enumerate every member of a population and therefore have a known negative bias (Slade and Blair 2000), they also yield abundance estimates that are necessarily bounded by zero and true population size, sometimes reducing their sensitivity to sampling variance as compared to model-based approaches (McKelvey and Pearson 2001). When used to support improved platypus management by government agencies or community groups, count data are both easily interpreted and readily mapped to describe platypus dispersion. Fyke-netting count data have also been used to calculate platypus population density (Serena 1994; Gardner and Serena 1995b; Serena and Williams 1997), subject to the caveat that density will be underestimated if some resident animals remain uncaptured and overestimated if home ranges of any captured individuals extend beyond the bounds of the sampled area (Serena 1994). Studies are needed to investigate how platypus density estimates vary with sampling intensity and spatial scale, with explicit attention paid to bias caused by home ranges being truncated at the upstream and downstream ends of survey areas (Efford 2004).
Minimum number alive
Minimum Number Alive (MNA) analysis generates modified count data, calculated as the number of animals captured in a given survey period plus the number of individuals that were not captured but deemed to be present given that they were encountered on previous and subsequent occasions (Krebs 1966). MNA is most likely to mirror true abundance in studies adhering to a fixed protocol within a spatially well-defined study area (Slade and Blair 2000). Furthermore, because MNA relies on information obtained in earlier and later surveys, it tends to underestimate population size in relatively brief longitudinal studies (Byrne et al. 2012) or in the initial and final stages of longer studies (Pocock et al. 2004), especially if capture probability is low (Byrne et al. 2012). In practice, after sacrificing data obtained in both the early and late years of long-term platypus mark–recapture studies, MNA has been found to yield platypus population estimates that are similar to (and more precise than) those generated by the Chao population estimator (Serena et al. 2014; Huggins et al. 2018) and Cormack–Jolly–Seber modelling (Bino et al. 2015; Serena and Grant 2017).
Conclusions and implications for future research
The results of camera-based platypus monitoring indicate that some individuals will evade capture in fyke nets even when encountering them for the first time. Animals may try to climb over, squeeze under or walk around nets, or backtrack through the entrance. It follows that variation in netting protocols (e.g. with respect to how well nets are anchored or how far netting wings are stretched onto land or above the water) may well contribute to variation in platypus fyke-netting results. Although steps can be taken to increase the proportion of a population that is likely to be captured, studies involving co-ordinated use of time-lapse cameras are needed to test the efficacy of specific protocols in limiting platypus net evasion. Research is also required to determine how factors such as surface discharge or channel morphology may influence fyke-netting outcomes independently of how they affect platypus population abundance.
The precision and accuracy of platypus abundance estimates may also be improved by routinely deploying cameras at (and potentially between) platypus fyke-netting sites. Although individual animals are unlikely to be identifiable in camera images, information on when and where they are filmed and their direction of travel can be used to help establish upper and lower bounds for the number of animals active in a given area.
The most pressing risks to platypus welfare in fyke nets include drowning (if nets become submerged) and development of hypothermia (in cold conditions). These are most readily addressed by ensuring that the end chamber of fyke nets remains partly elevated above the waterline and that nets are checked at regular intervals starting just before dusk and continuing through the night. Adopting these measures will also reduce welfare risks to bycatch, particularly if fish, crustaceans and air-breathing vertebrates are routinely released when first encountered.
Data availability
Results of the Steavenson/Little Steavenson Rivers platypus fyke-netting session will be shared upon reasonable request to the corresponding author. Results of the Monbulk Creek fyke-netting session have previously been summarised in a report to Melbourne Water (project number 1808CR24/23-012, report dated 16 April 2024).
Declaration of funding
We thank Melbourne Water for funding the Monbulk Creek platypus fyke-netting survey. Participation in this project by DD was funded by ARC Linkage Program LP 200200107; Open Access publication was made possible by a generous donation by Chocolatier Australia to the Australian Platypus Conservancy.
Acknowledgements
We thank Jason Van Weenen and Liberty Olds (Green Adelaide) for their help with setting and checking fyke nets in the Steavenson River system, and Alice Ewing and Matthew Linn (Ecology Australia) for conducting the fyke-netting session along Monbulk Creek. Platypus-related fieldwork was authorised by the Victorian Department of Environment, Land, Water and Planning (now Department of Energy, Environment and Climate Action) wildlife research permit 10010423, Victorian Department of Jobs, Precincts and Regions license SPFL20097 and Victorian Fisheries Authority research permit RP1142. Photographs are courtesy of Australian Platypus Conservancy/Geoff Williams (Figs 1, 3, 4 and 5) and Dana Dekkers (Figs 6 and 7).
References
Amstaetter, F., O’Connor, J., and Pickworth, A. (2016). Environmental flow releases trigger spawning migrations by Australian grayling Protroctes maraena, a threatened, diadromous fish. Aquatic Conservation: Marine and Freshwater Ecosystems 26, 35-43.
| Crossref | Google Scholar |
Beentjes, M. P., Jellyman, D. J., and Kim, S. W. (2006). Changing population structure of eels (Anguilla dieffenbachii and A. australis) from southern New Zealand. Ecology of Freshwater Fish 15, 428-440.
| Crossref | Google Scholar |
Bethge, P., Munks, S., and Nicol, S. (2001). Energetics of foraging and locomotion in the platypus Ornithorhynchus anatinus. Journal of Comparative Physiology B: Biochemical, Systemic, and Environmental Physiology 171(6), 497-506.
| Crossref | Google Scholar |
Bethge, P., Munks, S., Otley, H., and Nicol, S. (2003). Diving behaviour, dive cycles and aerobic dive limit in the platypus Ornithorhynchus anatinus. Comparative Biochemistry and Physiology. Part A, Molecular & Integrative Physiology 136, 799-809.
| Crossref | Google Scholar | PubMed |
Bethge, P., Munks, S., Otley, H., and Nicol, S. (2009). Activity patterns and sharing of time and space of platypuses, Ornithorhynchus anatinus, in a subalpine Tasmanian lake. Journal of Mammalogy 90, 1350-1356.
| Crossref | Google Scholar |
Beumer, J. P., Burbury, M. E., and Harrington, D. J. (1981). The capture of fauna other than fishes in eel and mesh nets. Wildlife Research 8, 673-677.
| Crossref | Google Scholar |
Bevacqua, D., De Leo, G. A., Gatto, M., and Melià, P. (2009). Size selectivity of fyke nets for European eel Anguilla anguilla. Journal of Fish Biology 74, 2178-2186.
| Crossref | Google Scholar | PubMed |
Bino, G., Grant, T. R., and Kingsford, R. T. (2015). Life history and dynamics of a platypus (Ornithorhynchus anatinus) population: four decades of mark-recapture surveys. Scientific Reports 5, 16073.
| Crossref | Google Scholar | PubMed |
Bino, G., Kingsford, R. T., Grant, T., Taylor, M. D., and Vogelnest, L. (2018). Use of implanted acoustic tags to assess platypus movement behaviour across spatial and temporal scales. Scientific Reports 8, 5117.
| Crossref | Google Scholar | PubMed |
Bino, G., Hawke, T., and Kingsford, R. T. (2021). Synergistic effects of a severe drought and fire on platypuses. Science of The Total Environment 777, 146137.
| Crossref | Google Scholar | PubMed |
Byrne, A. W., O’Keefe, J., Green, S., Sleeman, D. P., Corner, L. A. L., Gormley, E., Murphy, D., Martin, S. W., and Davenport, J. (2012). Population estimation and trappability of the European badger (Meles meles): implications for tuberculosis management. PLoS One 7, e50807.
| Crossref | Google Scholar | PubMed |
Coleman, R. A., Chee, Y. E., Bond, N. R., Weeks, A., Griffiths, J., Serena, M., Williams, G. A., and Walsh, C. J. (2022). Understanding and managing the interactive impacts of growth in urban land use and climate change on freshwater biota: a case study using the platypus (Ornithorhynchus anatinus). Global Change Biology 28, 1287-1300.
| Crossref | Google Scholar | PubMed |
Connolly, J. H., and Obendorf, D. L. (1998). Distribution, captures and physical characteristics of the platypus (Ornithorhynchus anatinus) in Tasmania. Australian Mammalogy 20, 231-237.
| Crossref | Google Scholar |
De-La-Warr, M., and Serena, M. (1999). Observations of platypus Ornithorhynchus anatinus mating behaviour. The Victorian Naturalist 116, 172-174.
| Google Scholar |
Dibner, R. R., Doak, D. F., and Murphy, M. (2017). Discrepancies in occupancy and abundance approaches to identifying and protecting habitat for an at-risk species. Ecology and Evolution 7, 5692-5702.
| Crossref | Google Scholar | PubMed |
Easton, L., Williams, G., and Serena, M. (2008). Monthly variation in observed activity of the platypus Ornithorhynchus anatinus. The Victorian Naturalist 125, 104-109.
| Google Scholar |
Efford, M. (2004). Density estimation in live-trapping studies. Oikos 106, 598-610.
| Crossref | Google Scholar |
Fanning, F. D., and Dawson, T. J. (1980). Body temperature variability in the Australian water rat, Hydromys chrysogaster, in air and water. Australian Journal of Zoology 28, 229-238.
| Crossref | Google Scholar |
Faragher, R. A., Grant, T. R., and Carrick, F. N. (1979). Food of the platypus (Ornithorhynchus anatinus) with notes on the food of brown trout (Salmo trutta) in the Shoalhaven River, NSW. Australian Journal of Ecology 4, 171-179.
| Crossref | Google Scholar |
Furlan, E. M., Griffiths, J., Gust, N., Handasyde, K. A., Grant, T. R., Gruber, B., and Weeks, A. R. (2013). Dispersal patterns and population structuring among platypuses, Ornithorhynchus anatinus, throughout southeastern Australia. Conservation Genetics 14, 837-853.
| Crossref | Google Scholar |
Furlan, E., Stoklosa, J., Griffiths, J., Gust, N., Ellis, R., Huggins, R. M., and Weeks, A. R. (2012). Small population size and extremely low levels of genetic diversity in island populations of the platypus, Ornithorhynchus anatinus. Ecology and Evolution 2, 844-857.
| Crossref | Google Scholar | PubMed |
Gardner, J. L., and Serena, M. (1995a). Observations on activity patterns, population and den characteristics of the water rat Hydromys chrysogaster along Badger Creek, Victoria. Australian Mammalogy 18, 710-75.
| Crossref | Google Scholar |
Gardner, J. L., and Serena, M. (1995b). Spatial organisation and movement patterns of adult male platypus, Ornithorhynchus anatinus (Monotremata: Ornithorhynchidae). Australian Journal of Zoology 43, 91-103.
| Crossref | Google Scholar |
Grant, T. R. (1983). Body temperatures of free-ranging platypuses, Ornithorhynchus anatinus (Monotremata), with observations on their use of burrows. Australian Journal of Zoology 31, 117-122.
| Crossref | Google Scholar |
Grant, T. (2004). Captures, capture mortality, age and sex ratios of platypuses, Ornithorhynchus anatinus, during studies over 30 years in the upper Shoalhaven River in New South Wales. Proceedings of the Linnean Society of New South Wales 125, 217-226.
| Crossref | Google Scholar |
Grant, T. R., and Dawson, T. J. (1978a). Temperature regulation in the platypus, Ornithorhynchus anatinus: maintenance of body temperature in air and water. Physiological Zoology 51, 1-6.
| Crossref | Google Scholar |
Grant, T. R., and Dawson, T. J. (1978b). Temperature regulation in the platypus, Ornithorhynchus anatinus: production and loss of metabolic heat in air and water. Physiological Zoology 51, 315-332.
| Crossref | Google Scholar |
Grant, T. R., Griffiths, M., and Temple-Smith, P. D. (2004a). Breeding in a free-ranging population of platypuses, Ornithorhynchus anatinus, in the upper Shoalhaven River, New South Wales – a 27 year study. Proceedings of the Linnean Society of New South Wales 125, 227-234.
| Crossref | Google Scholar |
Grant, T. R., Lowry, M. B., Pease, B., Walford, T. R., and Graham, K. (2004b). Reducing the by-catch of platypuses (Ornithorhynchus anatinus) in commercial and recreational fishing gear in New South Wales. Proceedings of the Linnean Society of New South Wales 125, 259-272.
| Crossref | Google Scholar |
Griffiths, J., Kelly, T., and Weeks, A. (2013). Net-avoidance behaviour in platypuses. Australian Mammalogy 35, 245-247.
| Crossref | Google Scholar |
Grigg, G., Beard, L., Grant, T., and Augee, M. (1992). Body temperature and diurnal activity patterns in the platypus (Ornithorhynchus anatinus) during winter. Australian Journal of Zoology 40, 135-142.
| Crossref | Google Scholar |
Gust, N., and Griffiths, J. (2011). Platypus (Ornithorhynchus anatinus) body size, condition and population structure in Tasmanian river catchments: variability and potential mucormycosis impacts. Wildlife Research 38, 271-289.
| Crossref | Google Scholar |
Gust, N., and Handasyde, K. (1995). Seasonal variation in the ranging behaviour of the platypus (Orithorhynchus anatinus) on the Goulburn River, Victoria. Australian Journal of Zoology 43, 193-208.
| Crossref | Google Scholar |
Gwinn, D. C., Brown, P., Tetzlaff, J. C., and Allen, M. S. (2011). Evaluating mark-recapture sampling designs for fish in an open riverine system. Marine and Freshwater Research 62, 835-840.
| Crossref | Google Scholar |
Gwinn, D., Beesley, L., Close, P., Gawne, B., and Davies, P. (2016). Imperfect detection and the determination of environmental flows for fish: challenges, implications and solutions. Freshwater Biology 61, 172-180.
| Crossref | Google Scholar |
Handasyde, K. A., McDonald, I. R., and Evans, B. K. (2003). Plasma glucocorticoid concentrations in free-ranging platypuses (Ornithorhynchus anatinus): response to capture and patterns in relation to reproduction. Comparative Biochemistry and Physiology. Part A, Molecular & Integrative Physiology 136, 895-902.
| Crossref | Google Scholar | PubMed |
Hangsleben, M. A., Allen, M. S., and Gwinn, D. C. (2013). Evaluation of electrofishing catch per unit effort for indexing fish abundance in Florida lakes. Transactions of the American Fisheries Society 142, 247-256.
| Crossref | Google Scholar |
Hawke, T., Bino, G., and Kingsford, R. T. (2021a). Damming insights: variable impacts and implications of river regulation on platypus populations. Aquatic Conservation: Marine and Freshwater Ecosystems 31, 504-519.
| Crossref | Google Scholar |
Hawke, T., Bino, G., Kingsford, R. T., Iervasi, D., Iervasi, K., and Taylor, M. D. (2021b). Long-term movements and activity patterns of platypus on regulated rivers. Scientific Reports 11, 3590.
| Crossref | Google Scholar |
Hawke, T., Bino, G., Shackleton, M. E., Ross, A. K., and Kingsford, R. T. (2022). Using DNA metabarcoding as a novel approach for analysis of platypus diet. Scientific Reports 12, 2247.
| Crossref | Google Scholar | PubMed |
He, P., Chopin, F., Suuronen, P., Ferro, R. S. T., and Lansley, J. (2021). Classification and illustrated definition of fishing gears. Technical Paper No. 672. (Food and Agriculture Organization of the United Nations, FAO Fisheries and Aquaculture: Rome, Italy) 10.4060/cb4966en
Huggins, R., Stoklosa, J., Roach, C., and Yip, P. (2018). Estimating the size of an open population using sparse capture-recapture data. Biometrics 74, 280-288.
| Crossref | Google Scholar | PubMed |
Jellyman, D. J. (1991). Factors affecting the activity of two species of eels (Anguilla spp.) in a small New Zealand lake. Journal of Fish Biology 39, 7-14.
| Crossref | Google Scholar |
Jellyman, D. J., and Graynoth, E. (2005). The use of fyke nets as a quantitative capture technique for freshwater eels (Anguilla spp.) in rivers. Fisheries Management and Ecology 12, 237-247.
| Crossref | Google Scholar |
Koch, N., Munks, S. A., Utesch, M., Davies, P. E., and McIntosh, P. D. (2006). The platypus Ornithorhynchus anatinus in headwater streams, and effects of pre-Code forest clearfelling, in the South Esk River catchment, Tasmania, Australia. Australian Zoologist 33, 458-473.
| Crossref | Google Scholar |
Krebs, C. J. (1966). Demographic changes in fluctuating populations of Microtus californicus. Ecological Monographs 36, 239-273.
| Crossref | Google Scholar |
Krueger, K. L., Hubert, W. A., and Price, R. M. (1998). Tandem-set fyke nets for sampling benthic fishes in lakes. North American Journal of Fisheries Management 18, 154-160.
| Crossref | Google Scholar |
Leigh, C. M., and Breed, W. G. (2020). A demographic study of the water-rat (Hydromys chrysogaster) on the River Torrens in Adelaide, South Australia. Australian Mammalogy 42, 277-282.
| Crossref | Google Scholar |
Lugg, W. H., Griffiths, J., van Rooyen, A. R., and Weeks, A. R. (2018). Optimal survey designs for environmental DNA sampling. Methods in Ecology and Evolution 9, 1049-1059.
| Crossref | Google Scholar |
MacNamara, R., McCarthy, T. K., and Barry, J. (2017). Estimation of production and long-term changes in the population of European eel Anguilla anguilla (L.) in an Irish lake. Ecology of Freshwater Fish 26, 602-608.
| Crossref | Google Scholar |
Marchant, R., and Grant, T. R. (2015). The productivity of the macroinvertebrate prey of the platypus in the upper Shoalhaven River, New South Wales. Marine and Freshwater Research 66, 1128-1137.
| Crossref | Google Scholar |
Maunder, M. N., Sibert, J. R., Fonteneau, A., Hampton, J., Kleiber, P., and Harley, S. J. (2006). Interpreting catch per unit effort data to assess the status of individual stocks and communities. ICES Journal of Marine Science 63, 1373-1385.
| Crossref | Google Scholar |
McKelvey, K. S., and Pearson, D. E. (2001). Population estimation with sparse data: the role of estimators versus indices revisited. Canadian Journal of Zoology 79, 1754-1765.
| Crossref | Google Scholar |
McLachlan-Troup, T. A., Dickman, C. R., and Grant, T. R. (2010). Diet and dietary selectivity of the platypus in relation to season, sex and macroinvertebrate assemblages. Journal of Zoology 280, 237-246.
| Crossref | Google Scholar |
McNally, J. (1960). The biology of the water rat Hydromys chrysogaster Geoffroy (Muridae: Hydromyinae) in Victoria. Australian Journal of Zoology 8, 170-180.
| Crossref | Google Scholar |
O’Connor, J. P., O’Mahony, D. J., and O’Mahony, J. M. (2005). Movements of Macquaria ambigua, in the Murray River, south-eastern Australia. Journal of Fish Biology 66, 392-403.
| Crossref | Google Scholar |
Otley, H. M., Munks, S., and Hindell, M. A. (2000). Activity patterns, movements and burrows of platypuses (Ornithorhynchus anatinus) in a sub-alpine Tasmanian lake. Australian Journal of Zoology 48, 701-713.
| Crossref | Google Scholar |
Pocock, M. J. O., Frantz, A. C., Cowan, D. P., White, P. C. L., and Searle, J. B. (2004). Tapering bias inherent in minimum number alive (MNA) population indices. Journal of Mammalogy 85, 959-962.
| Crossref | Google Scholar |
Reinfelds, I. V., Walsh, C. T., Van der Meulen, D. E., Growns, I. O., and Gray, C. A. (2013). Magnitude, frequency and duration of instream flows to stimulate and facilitate catadromous fish migrations: Australian bass (Macquaria novemaculeata ). River Research and Applications 29, 512-517.
| Crossref | Google Scholar |
Roberts, S., and Serena, M. (2024). Use of consolidated time-lapse camera imagery to detect and monitor platypus (Ornithorhynchus anatinus) activity. Australian Mammalogy 46, AM23045.
| Crossref | Google Scholar |
Serena, M. (1994). Use of time and space by platypus (Ornithorhynchus anatinus: Monotremata) along a Victorian stream. Journal of Zoology 232, 117-131.
| Crossref | Google Scholar |
Serena, M., and Grant, T. R. (2017). Effect of flow on platypus (Ornithorhynchus anatinus) reproduction and related population processes in the upper Shoalhaven River. Australian Journal of Zoology 65, 130-139.
| Crossref | Google Scholar |
Serena, M., and Pettigrove, V. (2005). Relationship of sediment toxicants and water quality to the distribution of platypus populations in urban streams. Freshwater Science 24, 679-689.
| Crossref | Google Scholar |
Serena, M., and Williams, G. A. (1997). Population attributes of platypus (Ornithorhynchus anatinus) in Flinders Chase National Park, Kangaroo Island. The South Australian Naturalist 72, 28-34.
| Google Scholar |
Serena, M., and Williams, G. (1998). Rubber and plastic rubbish: a summary of the hazard posed to platypus Ornithorhynchus anatinus in suburban habitats. The Victorian Naturalist 115, 47-49.
| Google Scholar |
Serena, M., and Williams, G. A. (2012). Effect of sex and age on temporal variation in the frequency and direction of platypus (Ornithorhynchus anatinus) captures in fyke nets. Australian Mammalogy 34, 75-82.
| Crossref | Google Scholar |
Serena, M., and Williams, G. (2022). Factors affecting the frequency and outcome of platypus entanglement by human rubbish. Australian Mammalogy 44, 81-86.
| Crossref | Google Scholar |
Serena, M., Worley, M., Swinnerton, M., and Williams, G. A. (2001). Effect of food availability and habitat on the distribution of platypus (Ornithorhynchus anatinus) foraging activity. Australian Journal of Zoology 49, 263-277.
| Crossref | Google Scholar |
Serena, M., Thomas, J. L., Williams, G. A., and Officer, R. C. E. (1998). Use of stream and river habitats by the platypus, Ornithorhynchus anatinus, in an urban fringe environment. Australian Journal of Zoology 46, 267-282.
| Crossref | Google Scholar |
Serena, M., Lyon, J. P., Tonkin, Z. D., Lieschke, J., and Williams, G. A. (2023). Differential impacts of a wildfire and post-fire sedimentation event on platypus and fish populations in a Victorian upland river. Marine and Freshwater Research 74, 86-94.
| Crossref | Google Scholar |
Serena, M., Williams, G. A., Weeks, A. R., and Griffiths, J. (2014). Variation in platypus (Ornithorhynchus anatinus) life-history attributes and population trajectories in urban streams. Australian Journal of Zoology 62, 223-234.
| Crossref | Google Scholar |
Shuster, W. D., Bonta, J., Thurston, H., Warnemuende, E., and Smith, D. R. (2005). Impacts of impervious surface on watershed hydrology: a review. Urban Water Journal 2, 263-275.
| Crossref | Google Scholar |
Slade, N. A., and Blair, S. M. (2000). An empirical test of using counts of individuals captured as indices of population size. Journal of Mammalogy 81, 1035-1045.
| Crossref | Google Scholar |
Steenweg, R., Hebblewhite, M., Whittington, J., Lukacs, P., and McKelvey, K. (2018). Sampling scales define occupancy and underlying occupancy-abundance relationships in animals. Ecology 99, 172-183.
| Crossref | Google Scholar | PubMed |
Temple-Smith, P., and Grant, T. (2001). Uncertain breeding: a short history of reproduction in monotremes. Reproduction, Fertility and Development 13, 487-497.
| Crossref | Google Scholar | PubMed |
Thomas, J. L., Parrott, M. L., Handasyde, K. A., and Temple-Smith, P. (2019). Burrow use by juvenile platypuses (Ornithorhynchus anatinus) in their natal home range. Journal of Mammalogy 100, 1182-1190.
| Crossref | Google Scholar |
Thomas, J. L., Parrott, M. L., Handasyde, K. A., and Temple-Smith, P. (2020). Maternal care of platypus nestlings (Ornithorhynchus anatinus). Australian Mammalogy 42, 283-292.
| Crossref | Google Scholar |
Watkins, A. F., McWhirter, J. L., and King, C. M. (2010). Variable detectability in long-term population surveys of small mammals. European Journal of Wildlife Research 56, 261-274.
| Crossref | Google Scholar |
White, G. C. (2005). Correcting wildlife counts using detection probabilities. Wildlife Research 32, 211-216.
| Crossref | Google Scholar |
Wiewel, A. S., Yackel Adams, A. A., and Rodda, G. H. (2009). Evaluating abundance estimate precision and the assumptions of a count-based index for small mammals. Journal of Wildlife Management 73, 761-771.
| Crossref | Google Scholar |
Williams, G., and Serena, M. (2018). Distribution of the Australian water-rat Hydromys chrysogaster in Victoria: findings from community-based sightings and live-trapping surveys. The Victorian Naturalist 135, 71-83.
| Crossref | Google Scholar |
Williams, G. A., Serena, M., and Grant, T. R. (2013). Age-related change in spurs and spur sheaths of the platypus (Ornithorhynchus anatinus). Australian Mammalogy 35, 107-114.
| Crossref | Google Scholar |
Woollard, P., Vestjens, W. J. M., and MacLean, L. (1978). The ecology of the eastern water rat Hydromys chrysogaster at Griffiths, N.S.W.: food and feeding habits. Wildlife Research 5, 59-73.
| Crossref | Google Scholar |