Age determination in the platypus (Ornithorhynchus anatinus) using spur sheath and spur developmental stages: a review
Tom Grant A * , Melody Serena B , Geoff A. Williams B and Peter Temple-Smith CA
B
C
Abstract
Reliable techniques and criteria for identifying sex and age class are important to understand the population biology of species in the course of wildlife research and monitoring. In platypuses, males have a keratinous spur on the inner surface of the ankle on each hind leg that undergoes characteristic age-related morphological changes within the first 3 years of life. Females, in contrast, do not develop a spur but instead develop a vestigial spur sheath that is lost toward the end of their first year. Spur developmental stages can be used to assign male platypuses to three age categories: ‘juveniles’ (≤12 months old), ‘sub-adults’ (13–24 months old) and ‘adults’ (>2 years of age), which are capable of breeding. Male spurs grow from a mean length of 12.4 (±0.5) mm after emergence from the nesting burrow to 18.6 (±0.6) mm by the end of the first year of life in Victoria. Platypus age categories have at times been inaccurately assigned. Here, we present an annotated pictorial guide of these age categories, suitable for use by wildlife rescuers and researchers in a field setting, and in veterinary surgeries, to facilitate accurate identification by those not familiar with this aspect of platypus biology.
Keywords: age, age estimation guide, juvenile male spur length, monotreme, Ornithorhynchus anatinus, Platypus, sexual dimorphism, spur development, spur morphology.
Introduction
In behavioural, physiological and ecological field studies, it is often important to be able to readily determine sex and age structure of a study population. Sexual dimorphism in the platypus, Ornithorhynchus anatinus, relates both to body size, with males generally being larger than females (Grant and Temple-Smith 1983; Grant 2007; Furlan et al. 2011; Gust and Griffiths 2011; Connolly et al. 1998, 2016) and the presence of calcaneal spurs in males. Females only develop a vestigial spur sheath, which is typically lost by the end of their first year of life (Temple-Smith 1973; Grant 2007; Williams et al. 2013).
Mature male platypuses have a functional venom system, which appears to have evolved for use in competition between males in the breeding season (Carrick et al. 2023). The morphological development of the spur and its associated structures, including the venom gland, was first studied in detail by Temple-Smith (1973). The spurs in males are hollow bilateral structures located in the inner ankle region, made of keratin and connected by a duct to a venom gland. The spur sits on a male-specific sesamoid bone (os calcaris) that has evolved with spur development in males, and is supported by muscles which can contract to erect it away from the ankle during its use in defence or attack. The venom glands rest under the skin over the dorsal surface of the pelvis, surrounded by muscles of the gluteal region and the body walls. From the gland, the duct conveying the venom (predominantly produced during the breeding season) passes between the muscles of the thigh to join a reservoir at the base of the spur (Temple-Smith 1973; Grant 2007).
Using the months in which his animals were caught, Temple-Smith (1973) assigned approximate ages to his captures in their early years of life. In collaboration with Temple-Smith, Grant (1984, 1989, 1995, 2007) refined these age estimates to better describe how spur morphology changes after emergence from the nesting burrow. Multiple captures of marked individuals from streams in Victoria and New South Wales (NSW) enabled Williams et al. (2013) to further improve spur/age estimates, by assessing variation within known-age cohorts and estimating the frequency of errors in classifying age categories. These reported errors for platypuses in Victoria and NSW were found to be low (<5%), indicating that early age classes can be accurately assigned to most individuals using spur morphology (Williams et al. 2013).
However, despite the above publications detailing how the size and appearance of platypus spur sheath and spurs change with age, it is our experience that many people handling platypuses still have difficulty in applying the published age criteria accurately, particularly to distinguish sub-adult males from older or younger individuals. This has been exacerbated by incorrect figure labelling in a chapter of a textbook, often used as a reference by veterinarians and members of animal rescue organisations, in which no photograph of a sub-adult spur is presented (Booth and Connolly 2008). Here, we provide a guide, featuring annotated photographs and age class-specific details, which should permit a range of workers to identify platypus age classes in either living or dead animals. To fill a gap in published reference values, information on the length of juvenile male spurs by month in Victorian platypuses is also presented.
Methods
We used information published in a doctoral thesis (Temple-Smith 1973), books (Grant 1984, 1989, 1995, 2007) and scientific journals (Williams et al. 2013), along with our own unpublished data and field experience, to relate the developmental stages, chronology and age of young platypuses to spur morphology. In referring to platypus age classes, we rejected the use of the term ‘puggle’ to designate a young platypus (as recently adopted in some popular literature, e.g. https://news.unsw.edu.au/en/platypuses-in-the-park; https://wwf.org.au/blogs/9-interesting-platypus-facts/), instead using ‘nestling’, ‘juvenile’, ‘sub-adult’ and ‘adult’. These terms are tied to defined developmental stages and have a well-established history of use in both the popular and scientific literature. In the case of females, reproduction has been confirmed to occur as early as an animal’s second breeding season after hatching, although some females fail to breed before their fourth breeding season (Grant et al. 2004). In the case of males, histological study of testis development indicates that spermatogenesis is likely to be initiated at the age of approximately 2 years, but sperm production is apparently both less and begins later in the breeding season as compared to older males (Temple-Smith 1973). Second-year males have reportedly fathered offspring after being translocated as juveniles to Warrawong Sanctuary in South Australia (T. G., unpubl. data) and Cardinia Creek in Victoria (M. S. and G. A. W., unpubl. data). However, research is needed to determine the extent to which second-year males contribute to breeding (if at all) in established wild populations containing older males.
Spur length (measured from tip to base in a straight line along the outer aspect) of juvenile males from streams in Victoria was measured by two of the authors (M. S. and G. A. W.) using hand-held dial calipers (accuracy = 0.1 mm). Left and right spur measurements were averaged to provide a mean estimated length for a given individual on a given date.
Results
Male spur/age categories
Platypus nestlings (comprising 1–3 individuals per litter; Burrell 1927) are confined to the nesting burrow, being dependent on milk from their mothers, for 3–4 months after hatching (Grant et al. 2004; Hawkins and Battaglia 2009; Thomas et al. 2020). Small spur sheaths are located on each side of the rear ankle in nestlings of both sexes (Temple-Smith 1973).
When the male nestling emerges from the nesting burrow (thereby qualifying as a ‘juvenile’), the spur sheath, inside which the spur continues to grow, is a hard conical structure, consisting of an outer horny sheath approximately 0.5 mm thick, enclosing a thicker inner fibrous layer which tapers from a basal thickness of 2–3 mm to little more than a veneer in the apical region of the spur (Temple-Smith 1973). The outer layer of the sheath may look fibrous or smooth and is usually cream or ivory white in colour. This layer and the fibrous inner sheath progressively flake away in the months following emergence, beginning at the apex, to expose the spur itself (Figs 1 and 2). The mean length of the spur sheath in February, around the time that a male in Victoria first emerges from a nesting burrow, is 12.4 ± 0.5 mm (mean ± SEM), but with considerable individual variation (range = 9.9–16.1 mm) (Table 1). Although at least the tip of the true spur has been exposed in >80% of juvenile male spurs in Victoria and NSW by May, entirely intact spur sheaths are occasionally observed as late as July, possibly related to the nature of the habitat, including the foraging substrate (Williams et al. 2013). The spur sheath completely degenerates (termed 'exsheathment' by Temple-Smith 1973) in most individuals by the end of their first year of life, with spur length in Victorian males increasing to a mean of 18.6 ± 0.6 mm by November, although again with considerable variation in length evident among individuals (range = 17.1–20.5 mm).
Juvenile male (first-year) spurs. The (a) hard smooth or (b) fibrous sheath encloses the spur. Spur sheath degeneration (exsheathment) typically begins within 3–4 months after the young first emerges from the nesting burrow. Exsheathment progresses from the tip (c) and is usually completed by approximately 10 months after emergence (see also Fig. 2).
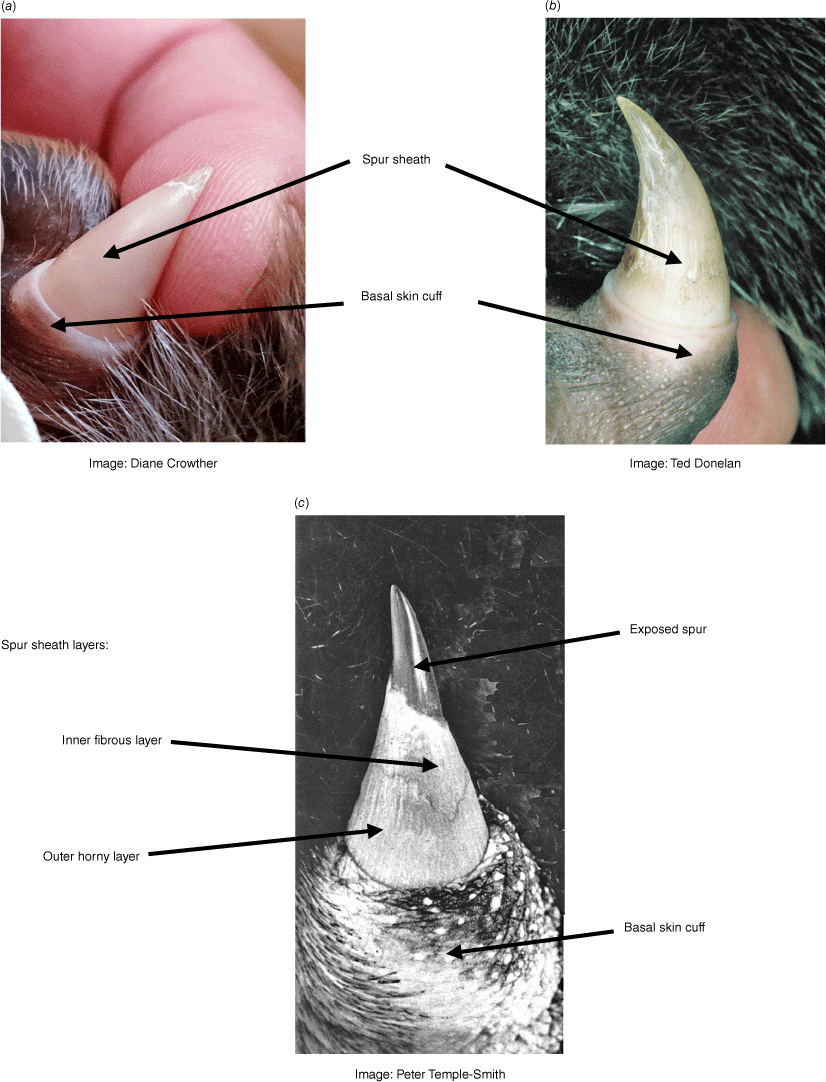
Progressive degeneration of the spur sheath (exsheathment) of a newly emerged juvenile male. This animal was picked up alongside a road on the NSW Central Coast on 26 January 2010 when approximately 3 months old. Sequence: (a) 1 month, (b) 3 months and (c) 5 months after emergence from the nesting burrow. Approximate ages from hatching (birth) and photo dates are shown in the figure. Unpublished information and photographs – Lynette Fletcher.
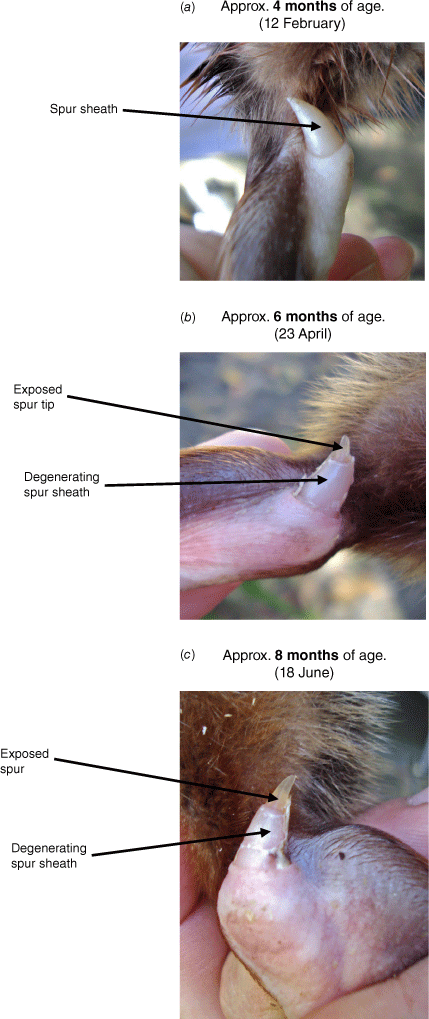
Month | n | Spur length (mm) | ||
---|---|---|---|---|
Mean ± s.e. | Range | |||
Feb | 13 | 12.4 ± 0.5 | 9.9–16.1 | |
Mar | 39 | 14.1 ± 0.3 | 8.9–17.2 | |
Apr | 32 | 15.5 ± 0.3 | 11.7–18.1 | |
May | 10 | 15.6 ± 0.8 | 11.1–19.1 | |
June | 4 | 16.7 ± 0.5 | 15.6–18.1 | |
July | 2 | 16.6 ± 0.0 | 16.5–16.6 | |
Aug | 2 | 16.8 ± 0.7 | 16.1–17.5 | |
Sept | 21 | 18.1 ± 0.3 | 16.2–22.2 | |
Oct | 24 | 18.2 ± 0.4 | 11.2–21.6 | |
Nov | 5 | 18.6 ± 0.6 | 17.1–20.5 |
Despite there being considerable variation within populations and geographical areas, there is an overall clinal increase in the body size of platypuses (weight and length in both sexes) from Tasmania in the south to north Queensland (Connolly and Obendorf 1998; Kolomyjec 2010; Furlan et al. 2011) and across the Great Dividing Range (Grant and Temple-Smith 1983; Furlan et al. 2011). There are no published data relating to how male spur dimensions change with growth in Tasmania or Queensland. However, Macgregor (2015) describes a similar pattern of change in spur and spur sheath morphology in Tasmanian platypuses in their first year of life, albeit out of phase with mainland animals, which breed around 2–3 months earlier in the year (Connolly and Obendorf 1998; Macgregor 2015; Roberts and Serena 2024).
Once the hard spur sheath breaks away completely in the male, a pink or pale-coloured collar of vascularised tissue is evident, termed the 'epidermal collar'. This initially extends approximately one-third of the height of the spur above its base, and is the key feature used to recognise a sub-adult. Occasionally, some of the spur sheath will persist into the second year of life, but this was found in <5% of known-age animals (Williams et al. 2013). Fig. 3a shows the position of the epidermal collar in a relatively young sub-adult (estimated to be ~15 months old), with the collar shown to have regressed further down the spur in an older sub-adult (Fig. 3b) estimated to be approximately 22 months old. These ages were calculated based on an assumed hatching date of 1 November of the previous year (Williams et al. 2013).
Sub-adult male (second-year) spurs. The spur sheath in both of these males has degenerated and been lost. The fleshy, vascularised epidermal collar, which is easily damaged but heals quickly, is most prominent in early sub-adults but less so later in their second year. Animal (a) is known to be approximately 15 months old and (b) approximately 22 months.
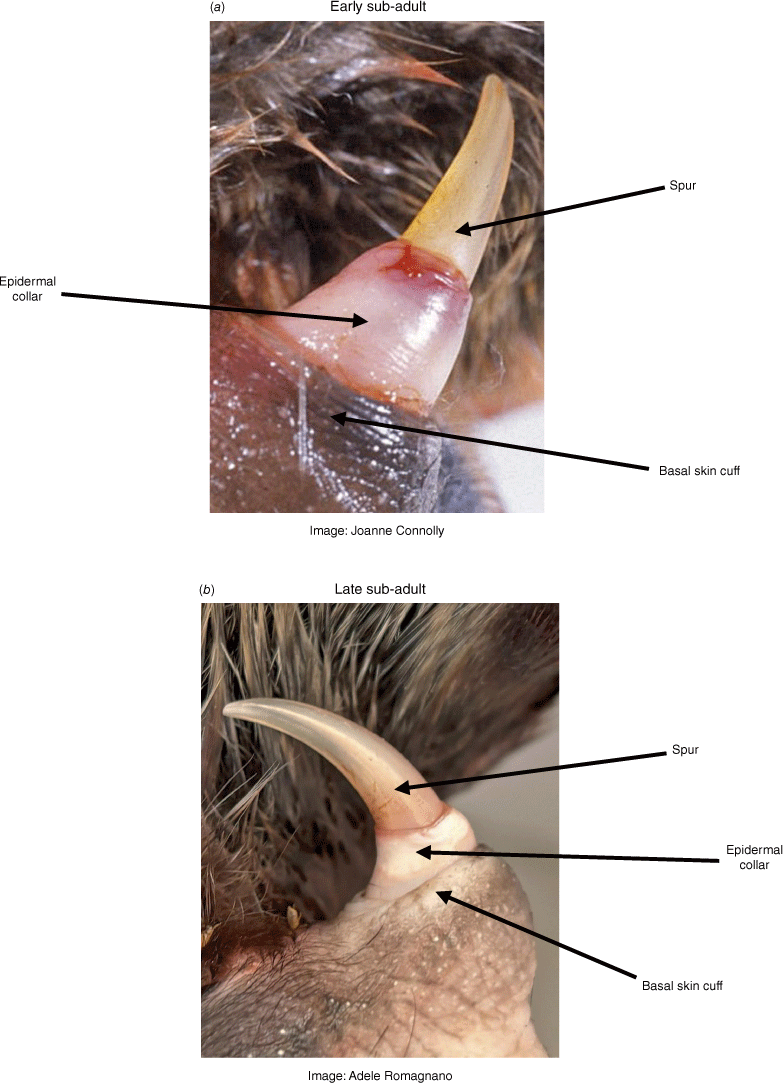
In a male’s third year of life, the epidermal collar retains some prominence for the first 3–4 months (Fig. 4a), but then recedes until it is just visible above the basal skin cuff (Fig. 4b). Beyond the third year, male age cannot be determined with any degree of precision. Some very old animals may have spurs which have worn down with blunt rather than pointed tips (Fig. 4c; Serena et al. 2024), are darkly pigmented and have the venom canal exiting more apically than in the case of younger males (Temple-Smith 1973). The maximum known age of a male platypus in the wild is 24 years (Serena et al. 2024), but many individuals probably have a shorter life span of up to 6–8 years (Grant 2004; Serena et al. 2014).
Adult male (third year or older) spurs. The spur is fully exposed and the epidermal collar shrunk down to the base of the spur. The loose basal skin cuff around the spur base moves with handling and may expose (a) more or (b) less of the remaining epidermal collar. Older male spurs may be rounded at the tip and be more darkly pigmented as in (c), a male known by recapture to be in its 23rd year (Serena et al. 2024).
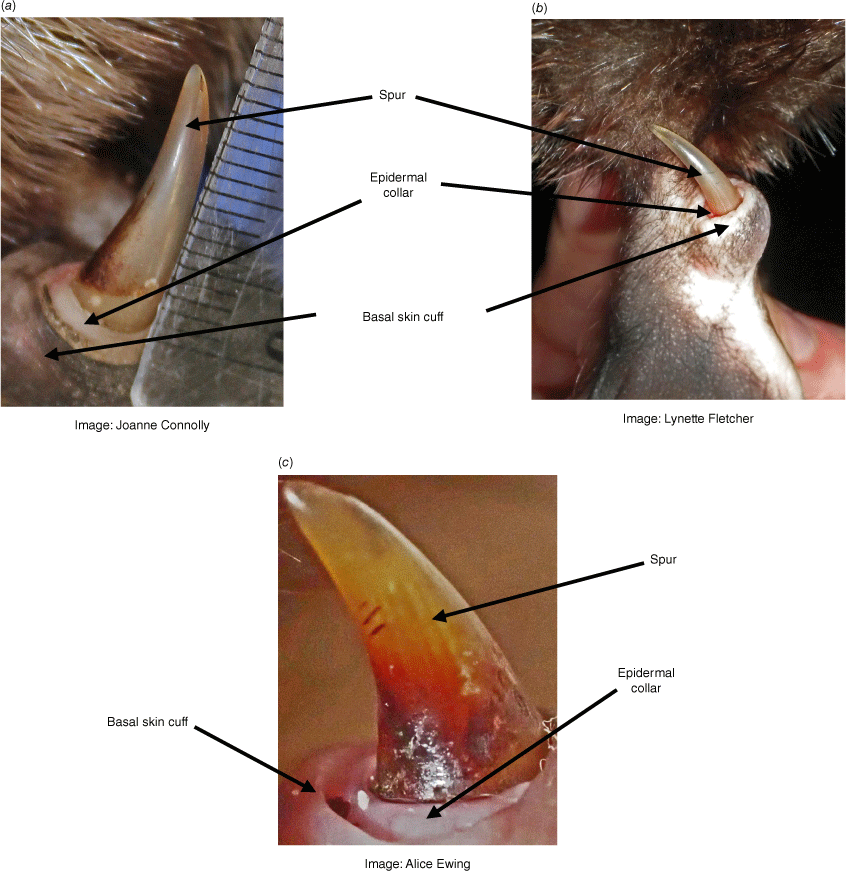
Female spur sheath/age categories
A true spur does not develop in the female platypus. However, a vestigial spur sheath measuring around 1–4 mm in length and whitish to brown in colour is present in the ankle region (at the same site where male spurs are found; Fig. 5a, b) in the first year of life. These structures are most typically lost by wild females at the age of 8–10 months, although sometimes earlier in captive individuals (Grant 2007; Williams et al. 2013). In some wild animals, the sheath on one hind limb may persist longer than on the other, with ≤2% of wild juvenile females estimated to have at least a remnant of the spur sheath visible beyond the age of 12 months (Williams et al. 2013). After the spur sheath is lost, a shallow, narrow depression in the skin of the inner ankle remains in all females (Fig. 5c). In short, a female with any remnant of a spur sheath can be identified as a juvenile from the immediate past breeding season, but after both spur sheaths are completely lost, the age of a female platypus cannot be assigned unless it has been previously marked as a juvenile. The maximum recorded female longevity in the wild is 21 years (Grant 2004), although most individuals have a shorter life span (Grant 2004; Serena et al. 2014).
Juvenile female (first year) spur sheath. These can be (a) brown or (b) light-coloured and prominent, although the ankle may need to be gently bent back to evert the sheath. (c) Sub-adult/adult female (indeterminate age). The spur sheath has been lost, leaving only the spur sheath recess, which occurs in all sub-adult/adult females. Metal leg bands were used to identify individuals until the early 1990s prior to the use of microchips (Grant and Whittington 1991).
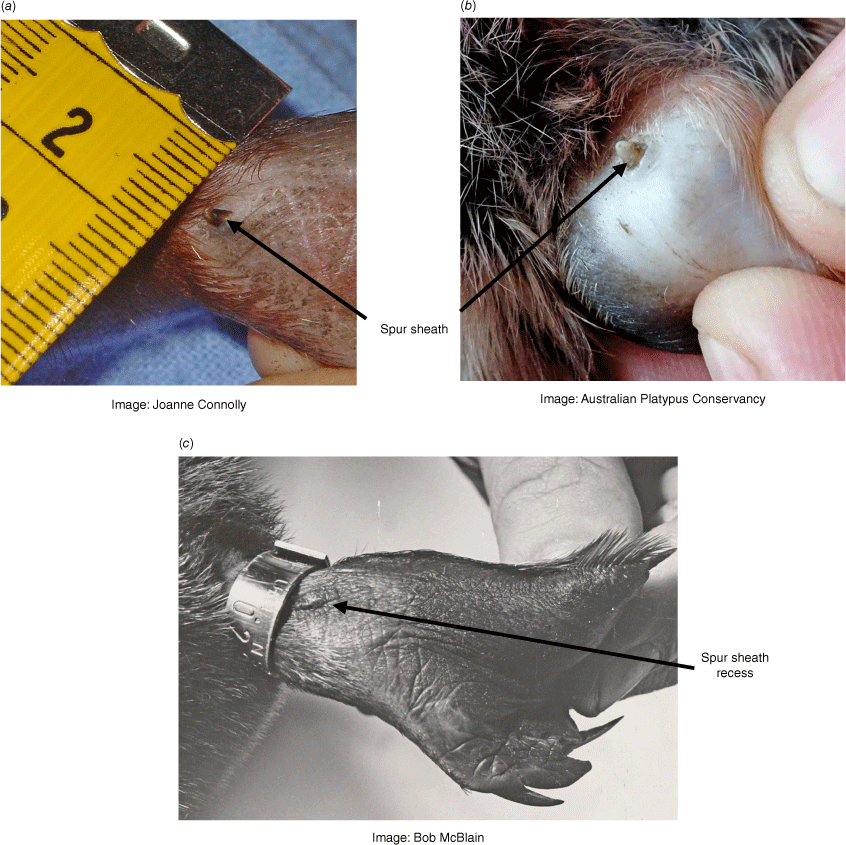
Discussion
Numbers of wild platypuses of both sexes marked as juveniles and recaptured later in life are low, so that the long-term age structure of wild populations is often not known, making a comprehensive understanding of the dynamics of those populations difficult. However, sampling a population by captures during the period when juveniles have recently left their mothers’ nesting burrows, prior to later dispersal (especially juvenile males; Grant 1989, 2004; Serena et al. 2014; Bino et al. 2015), can provide an estimate of reproductive success in that population as an indicator of its resilience and persistence. In south-eastern mainland Australia (Victoria, NSW) juvenile platypuses begin leaving the nesting burrows from late January and remain in their natal area until at least March to May (Grant 1989, 2004; Grant et al. 2004; Serena and Williams 2012), whereas in Tasmania juveniles generally emerge in April to June, namely 2–3 months later than on the mainland (Connolly and Obendorf 1998; Munks et al. 2002; Macgregor 2015). However, using this indicator of reproductive success depends on accurately identifying first-year males and females in the captured sample.
As an example, a long-term study of platypuses in the upper Shoalhaven River on the Southern Tablelands of NSW showed varying numbers of new juveniles entered the population in different years, but indicated successful breeding in almost all years, even in the severe drought years of 1982–1983 and during the first 4 years of the Millennium Drought from 1997 to 2001 (http://www.bom.gov.au/climate/drought/knowledge-centre/previous-droughts.shtml), when the river was at times reduced to a series of unconnected pools. Accurate identification of juvenile, sub-adult and adult male and female platypuses also enabled the development of a long-term modelling study indicating that the upper Shoalhaven River population was stable, with the likelihood of extinction within 100 years estimated to be only 4% (Bino et al. 2015).
Similarly, recovery of a dead juvenile male in July 2021 (when the animal was ~8–9 months of age) from the Wingecarribee River in NSW confirmed that there had been at least one successful breeding event in that part of the river, even following 3 years of extreme drought conditions that reduced the river to unconnected pools and most tributary streams had stopped flowing (T. G., unpub. data). This important observation was only possible due to the identification of the age of this animal by one of the authors (T. G.).
Capture of a young juvenile female (spur sheath intact) and a somewhat older juvenile female (spur sheath remnants only) and analysis of video footage showing a juvenile male (distinct spur sheath present) indicated that at least one successful breeding event occurred in each of three consecutive breeding seasons in the upper Jenolan River in the Jenolan Karst Conservation Reserve in central western NSW. This was in spite of major instream habitat disturbance occurring during major river desedimentation works, again illustrating the value of being able to accurately distinguish early age classes using spur sheath/spur morphology (Musser et al. 2024).
A recent publication reported the presence of perfluorooctane sulfonate (PFOS) contaminants in the livers of eight necropsied wild platypuses from a number of areas in NSW (Warwick et al. 2024). PFOS was detected at varying levels in all eight specimens, but the third highest level was from the previously discussed juvenile male from the Wingecarribee River. It seems of concern that this juvenile male had accumulated PFOS contaminants in its liver after exposure to the external environment for only around 5 months, after being previously fed on milk in the nesting burrow during the 3–4 months preceding its emergence. The second highest level of PFOS recorded was in a female from Ourimbah Creek on the NSW Central Coast and the highest in a platypus from the Hunter River in the Hunter Region of NSW. While the authors refer to using the criteria from Williams et al (2013) to assign age to their specimens, they incorrectly listed the Wingecarribee River platypus as an adult male, the one from Ourimbah Creek as a sub-adult female (an age category that could not have been assigned unless it had been previously captured and marked) and the most contaminated specimen from the Hunter River as a juvenile male. This example again illustrates the importance of being able to accurately assign platypuses to their correct early age classes.
Accurate identification of the age categories of wild caught, rescued or dead platypuses requires those involved to have a sound understanding of specific age identification criteria. The five figures in this publication are presented to facilitate reliable age determination, even by those with little prior knowledge or experience with the platypus and without necessarily having to refer to the main text of our paper.
Data availability
The juvenile spur length data contributing to this study will be shared upon reasonable request to M. Serena or G. A. Williams.
Declaration of funding
Fieldwork contributing to the juvenile male spur dataset was funded by the Cities of Banyule, Knox and Manningham, Coliban Water, Corangamite CMA, Earthwatch Australia, Glenelg Hopkins CMA, Melbourne Water, Nillumbik Shire, Parks Victoria, Primelife Corporation, Project Platypus, Sara Halvedene Foundation, Stewart Park Management Committee, Upper Maribyrnong Catchment Landcare, VicRoads, Victorian Department of Energy, Environment and Climate Action, Werribee River Association, Wimmera CMA and Yarra Ranges Council.
Acknowledgements
We thank the many volunteers (too numerous to name individually) who have assisted us over the years with platypus live-trapping studies, and the landholders who provided access to river and creek frontages, allowed us to camp on their properties and often gave other assistance during field visits. Details of permits/licences and ethics approvals are given in the publications listed in the Reference section, which also include funding, permit/licence and ethics approval details of the field investigations resulting in the previously published material used in the development of this review. Platypus live-trapping and handling activities during the collection of spur length data were authorised by DEECA Wildlife Research Permits 95-208 to 10004761 and Victorian Fisheries Authority Fisheries Permit RP 553, with annual oversight and approval kindly provided by the Australian Platypus Conservancy AEEC (Project 95/1). Patrick Tegart, Environmental Projects Officer Wingecarribee Shire Council and Jess Whinfield, then from Taronga Zoo, kindly provided photographs of the dead platypus from the Wingecarribee River. We also thank Ted Donelan, Adele Romagnano, Diane Crowther/Arthur Rylah Institute, Joanne Connolly/Charles Sturt University, Wagga Wagga, Lynette Fletcher/University of Newcastle, Ourimbah, Alice Ewing/Ecology Australia and Bob McBlain, for generously allowing us to reproduce their images noted in Figs 1–5.
References
Bino, G., Grant, T. R., and Kingsford, R. T. (2015). Life history and dynamics of a platypus (Ornithorhynchus anatinus) population: four decades of mark-recapture surveys. Scientific Reports 5, 16073.
| Crossref | Google Scholar | PubMed |
Connolly, J. H., and Obendorf, D. L. (1998). Distribution, captures and physical characteristics of the platypus (Ornithorhynchus anatinus) in Tasmania. Australian Mammalogy 20, 231-237.
| Crossref | Google Scholar |
Connolly, J. H., Claridge, T., Cordell, S. M., Nielsen, S., and Dutton, G. F. (2016). Distribution and characteristics of the platypus (Ornithorhynchus anatinus) in the Murrumbidgee catchment. Australian Mammalogy 38, 58-67.
| Crossref | Google Scholar |
Furlan, E., Griffiths, J., Gust, N., Armistead, R., Mitrovski, P., Handasyde, K., Serena, M., Hoffmann, A. A., and Weeks, A. R. (2011). Is body size variation in the platypus (Ornithorhynchus anatinus) associated with environmental variables?. Australian Journal of Zoology 59, 201-215.
| Crossref | Google Scholar |
Grant, T. R. (2004). Captures, capture mortality, age and sex ratios of platypuses, Ornithorhynchus anatinus, during studies over 30 years in the upper Shoalhaven River in New South Wales. Proceedings of the Linnean Society of NSW 125, 217-226.
| Google Scholar |
Grant, T. R., and Temple-Smith, P. D. (1983). Size, seasonal weight change and growth in platypuses, Ornithorhynchus anatinus, in lakes and rivers of New South Wales. Australian Mammalogy 6, 51-60.
| Crossref | Google Scholar |
Grant, T. R., and Whittington, R. J. (1991). The use of freeze-branding and implanted transponder tags as a permanent marking method for platypuses, Ornithorhynchus anatinus (Monotremata: Ornithorhynchidae). Australian Mammalogy 14, 147-150.
| Crossref | Google Scholar |
Grant, T. R., Griffiths, M., and Temple-Smith, P. D. (2004). Breeding in a free-ranging population of platypuses, Ornithorhynchus anatinus, in the upper Shoalhaven River in New South Wales - a 27 year study. Proceedings of the Linnean Society of NSW 125, 227-234.
| Google Scholar |
Gust, N., and Griffiths, J. (2011). Platypus (Ornithorhynchus anatinus) body size, condition and population structure in Tasmanian river catchments: variability and potential mucormycosis impacts. Wildlife Research 38, 271-289.
| Crossref | Google Scholar |
Hawkins, M., and Battaglia, A. (2009). Breeding behaviour of the platypus (Ornithorhynchus anatinus) in captivity. Australian Journal of Zoology 57, 283-293.
| Crossref | Google Scholar |
Munks, S. A., Otley, H. M., Bethge, P., and Jackson, J. (2002). Reproduction, diet and daily energy expenditure of the platypus in a sub-alpine Tasmanian lake. Australian Mammalogy 21, 260-261.
| Google Scholar |
Musser, A., Grant, T., and Turak, E. (2024). Movements of platypuses around and through instream structures and natural barriers in the Jenolan Karst Conservation Reserve. Australian Mammalogy 46, AM23031.
| Crossref | Google Scholar |
Roberts, S., and Serena, M. (2024). Use of consolidated time-lapse camera imagery to detect and monitor platypus (Ornithorhynchus anatinus) activity. Australian Mammalogy 46, AM23045.
| Crossref | Google Scholar |
Serena, M., and Williams, G. A. (2012). Effect of sex and age on temporal variation in the frequency and direction of platypus (Ornithorhynchus anatinus) captures in fyke nets. Australian Mammalogy 34, 75-82.
| Crossref | Google Scholar |
Serena, M., Williams, G. A., Weeks, A., and Griffiths, J. (2014). Variation in platypus (Ornithorhynchus anatinus) life-history attributes and population trajectories in urban streams. Australian Journal of Zoology 62, 223-234.
| Crossref | Google Scholar |
Serena, M., Snowball, G., Thomas, J. L., Williams, G. A., and Danger, A. (2024). Platypus longevity: a new record in the wild and information on captive life span. Australian Mammalogy 46, AM23048.
| Crossref | Google Scholar |
Thomas, J. L., Parrott, M. L., Handasyde, K. A., and Temple-Smith, P. D. (2020). Maternal care of platypus nestlings (Ornithorhynchus anatinus). Australian Mammalogy 42, 283-292.
| Crossref | Google Scholar |
Warwick, K. G., Wright, I. A., Whinfield, J., Reynolds, J. K., and Ryan, M. M. (2024). First report of accumulation of perfluorooctane sulfonate (PFOS) in platypuses (Ornithorhynchus anatinus) in New South Wales, Australia. Environmental Science and Pollution Research International 31, 51037-51042.
| Crossref | Google Scholar | PubMed |
Williams, G. A., Serena, M., and Grant, T. R. (2013). Age-related change in spurs and spur sheaths of the platypus (Ornithorhynchus anatinus). Australian Mammalogy 35, 107-114.
| Crossref | Google Scholar |