Production of light-coloured, low heat-absorbing Holstein Friesian cattle by precise embryo-mediated genome editing
Jingwei Wei
A
B
C
D
Abstract
Genome editing enables the introduction of beneficial sequence variants into the genomes of animals with high genetic merit in a single generation. This can be achieved by introducing variants into primary cells followed by producing a live animal from these cells by somatic cell nuclear transfer cloning. The latter step is associated with low efficiencies and developmental problems due to incorrect reprogramming of the donor cells, causing animal welfare concerns. Direct editing of fertilised one-cell embryos could circumvent this issue and might better integrate with genetic improvement strategies implemented by the industry.
In vitro fertilised zygotes were injected with TALEN editors and repair template to introduce a known coat colour dilution mutation in the PMEL gene. Embryo biopsies of injected embryos were screened by polymerase chain reaction and sequencing for intended biallelic edits before transferring verified embryos into recipients for development to term. Calves were genotyped and their coats scanned with visible and hyperspectral cameras to assess thermal energy absorption.
Multiple non-mosaic calves with precision edited genotypes were produced, including calves from high genetic merit parents. Compared to controls, the edited calves showed a strong coat colour dilution which was associated with lower thermal energy absorbance.
Although biopsy screening was not absolutely accurate, non-mosaic, precisely edited calves can be readily produced by embryo-mediated editing. The lighter coat colouring caused by the PMEL mutation can lower radiative heat gain which might help to reduce heat stress.
The study validates putative causative sequence variants to rapidly adapt grazing cattle to changing environmental conditions.
Keywords: cattle, coat color dilution, embryo-mediated, genome editing, heat stress, homology-directed repair, microinjection, P. Leu18del, PMEL, pre-melanosome protein 17, TALEN.
Introduction
Today’s availability of whole genome sequence information for large numbers of individual animals, made possible by the rapid advances in sequencing technology, has greatly improved the identification of causative mutations (Georges et al. 2019). Genome editing has the potential to utilise the information for the genetic improvement of livestock by directly and rapidly introducing advantageous sequence variants into the genomes of animals with high genetic merit (Ruan et al. 2017; Bishop and Van Eenennaam 2020). Hence, it provides an opportunity for the required fast adaptation of grazing cattle to already warming temperatures.
Black coats have been identified to increase solar heat load as a potential contributor to heat stress (Hansen 1990; Gebremedhin et al. 2011). Many of the thermotolerant tropical cattle breeds are typically light-coloured with coats that reflect much of the incident solar radiation. A naturally occurring colour dilution variant in the premelanosome protein (PMEL) gene, first identified in Highland and Galloway cattle, was later found to also segregate in the Zimbabwean breed of Tuli cattle (Schmutz and Dreger 2013; Davis et al. 2017). Hence, this 3 bp deletion PMEL variant, deleting leucine 18 in the signal peptide (p.Leu18del), was a strong candidate towards improving the thermotolerance of temperate Holstein Friesian dairy cattle by genome editing. In a previous study, this PMEL sequence variant was introduced using cell-mediated genome editing and somatic cell nuclear transfer (SCNT) to produce a grey and white Holstein Friesian calf showing a strong colour dilution effect (Laible et al. 2021). Highlighting the inefficiencies of the cloning approach, the calf died after 4 weeks due to known cloning-related complications. The embryo-mediated approach avoids the inefficiencies associated with producing live calves by SCNT and could be implemented by adjusting contemporary embryo-based genomic selection strategies (Ponsart et al. 2013; McLean et al. 2020). In contrast to the cell-mediated approach, embryo-mediated genome editing provides limited control over editing outcomes, particularly for the less efficient introduction of precise edits by homology-directed repair (HDR) mechanisms (Park et al. 2020). This limitation could potentially be overcome by screening embryo biopsies to verify the introduction of the intended edits before live animals are produced from the edited embryos (Wei et al. 2022). However, the potential for generating mosaic embryos with multiple different edits might reduce the accuracy of predicting the correct genotypes of the animals produced from the biopsied embryos (Vilarino et al. 2018).
In the present study, we have used embryo-mediated genome editing in combination with biopsy screening to produce several calves, homozygous for the p.Leu18del mutation. The mutation caused colour-diluted coats which absorbed less thermal energy compared to non-edited-controls. This adaptation can lower solar heat gain in the colour-diluted cattle and might help to reduce heat stress.
Materials and methods
Experimental animals
All animal experiments were performed in accordance with the relevant guidelines and regulations with approvals from New Zealand’s Environmental Protection Authority (GMD100279) and the AgResearch Ruakura Animal Ethics Committee (15088, 15467, 0685).
Ovum pick up, in vitro maturation, in vitro fertilisation and embryo culture
Transvaginal ovum pick up (OPU) was performed on 14 elite dairy cows (ranging within the top 1–32% of the New Zealand dairy herd) once a week on four occasions (Bols et al. 1995). Other oocytes were aspirated from abattoir-sourced ovaries. Oocytes were in vitro matured and fertilised (IVF) with semen from an ordinary (for abattoir-derived embryos) or high breeding worth Holstein Friesian dairy bull (for OPU-derived embryos) according to described procedures (Wei et al. 2015).
Embryo-mediated editing, embryo culture, biopsy and vitrification
HDR editing in embryos for the p.Leu18del PMEL mutation, biopsy screening for editing outcomes and cryopreservation of biopsied embryos were essentially performed as described in our previous study (Wei et al. 2022). Briefly, zygotes were injected 5–8 h after IVF with PMEL-specific TALENs TL17 and TR217 delivered as plasmid (20 ng/μL each) or as mRNA (100 ng/μL each), together with the single-stranded oligodeoxynucleotide (ssODN) repair template 1288 (100 ng/μL). Embryos were then cultured for 7 days to the blastocyst stage and a ~15 cell biopsy sliced off the trophoblast. The biopsied embryos were vitrified and stored in liquid nitrogen prior to warming and individual transfer into synchronised recipients 7 days after oestrus (Wei et al. 2018). Pregnancy establishment and progression were monitored by ultrasonography and palpation with calving induced near full term (Brophy et al. 2003).
Cell clone derivation
A small biopsy from the ear was taken and after cleansing put into culture as previously described (Wagner et al. 2017). From outgrown primary fibroblasts, mitotic cells were manually picked with a glass capillary into a 96 well plate for expansion (Laible et al. 2021).
Genotyping
Embryo biopsies were lysed in 10 μL proteinase K (0.2 μg/μL) containing PCR buffer. The PMEL target site was preamplified for 17 cycles with primers 1249/1250 and 2 μL of the reaction were then used for the HDR-specific droplet digital PCR (ddPCR) assay (40 cycles) with primers 1283 and 1284, hybridisation probes 1285, 1321 and 1289 and gBlock® 1282 as positive control. All primers and probes are summarised in Supplementary Table S1.
For sequencing, 2 μL of the preamplified sample was further amplified for 36 cycles with primers 1249/1250 in a new reaction. Resulting PCR fragments were separated on a gel, isolated, and sent for Sanger sequencing. Mixed sequences were analysed by Tracking of Indels by Decomposition (TIDE) (Brinkman et al. 2014).
Genomic DNA from blood and ear fibroblasts was extracted with a Cytiva Nucleon BACC3 kit (Cytiva, Little Chalfont, UK) and 50 ng of the DNA used without preamplification for ddPCR (40 cycles) and PCR (36 cycles). PCR fragments were processed for sequence analysis as described above.
Cells from individual cell clones were trypsinised, washed in PBS and lysed in 20 μL proteinase K buffer. The crude lysate (2 μL) was used for PMEL target site amplification by ddPCR and PCR for identifying allelic contributions by the quantitative assay and sequencing of PCR fragments, respectively.
Genomic DNA (50 ng) of the calves was assessed for the potential integration of TALEN plasmids by PCR amplification (36 cycles) with the TALEN vector-specific primers 1607 and 1608 amplifying a 336 bp fragment of the plasmid sequence encoding the N-terminal TALE domain. Amplification (36 cycles) of a 444 bp fragment from the endogenous bovine alpha-lactalbumin gene (LALBA) with primers 211 and 212 served as a control for the presence of amplifiable DNA.
Parentage analysis
Whole genome DNA samples isolated from blood were profiled by Genomnz (www.genomnz.co.nz). Samples were analysed on an ultra-low density single nucleotide polymorphism (SNP) chip with approximately 1000 bovine SNPs using the Illumina iScan platform.
Thermal absorption
The left side trunk of the animals, aged five (calves 22, 24) and 7.5 (calves 14 and 16) months, was imaged in the visible spectrum (460–640 nm) and the near-infrared (NIR) spectrum (670–960 nm) with Ximea Snapshot VIS and Ximea Snapshot NIR cameras (Imech, Belgium), respectively, under illumination (LMR16503KAL, 50 W, 3000 K; Hugo Lighting, USA). Within the scanned images, three pixels were manually selected in a patch of the coat representing the lightest and darkest hues, the associated spectra extracted, and the average ratio light absorbed:incident light (average absorbance) compared at each wavelength (Matlab, Mathworks Inc, USA). For each animal, overall absorption scores were obtained by averaging the total absorbance for all pixels within the scanned trunk region on a representative spectral image (Fig. S1). As a reference point, we included an NIR reference tile (Labsphere Inc, USA) that reflects almost 100% of NIR energy (close to 0% absorbance) and reflects light brown in the visible range.
Results
We have previously edited a naturally occurring PMEL sequence variant in embryos, using biopsies to show that embryos can be fully converted into the precision edited genotype (Wei et al. 2022). As a next step, we now used embryo-mediated editing to produce HDR-edited cattle for the same p.Leu18del PMEL mutation.
Editing of IVF embryos produced with abattoir- and OPU-derived oocytes
Following on from our previous study, we first used abattoir-derived oocytes to generate IVF embryos for HDR editing (referred to as abattoir-derived). Zygotes classified as fertilised (n = 471) were injected with the PMEL-specific TALEN pair, delivered by plasmid or mRNA, and ssODN repair template (Table 1). Intact embryos were placed into culture (n = 465) with an overall development rate into morphological grade 1 and 2 blastocysts of 13% (62/471). Embryos of grade 1 and 2 quality were biopsied for the assessment of editing outcomes and vitrified, preserving the option of transfer if suitably edited. For the plasmid injected embryos, 33 of 35 biopsies could be analysed, and 16 embryos were classified as 100% edited for the intended 3 bp deletion mutation in the PMEL gene. The full conversion HDR efficiency was 48% (16/33) for plasmid-injected embryos. Only 16 biopsies from 27 mRNA-injected embryos could be analysed, with four embryos that were fully converted to the HDR genotype (4/16; 25%). For the samples that could not be analysed, the PCR amplification failed, probably due to loss or degradation of the sample.
Oocyte source | Editors | Injected | IVC | Cleavage A | G1–3 A | G1–2 A | 100% edited B | |
---|---|---|---|---|---|---|---|---|
Abattoir | Plasmid | 301 | 296 | 253 (85%) | 117 (40%) | 35 (12%) | 16/33 (48%) | |
Abattoir | mRNA | 170 | 169 | 156 (92%) | 59 (35%) | 27 (16%) | 4/16 (25%) | |
Abattoir | Total | 471 | 465 | 409 (88%) | 176 (38%) | 62 (13%) | 20/49 (41%) | |
OPU | Plasmid | 227 | 217 | 196 (90%) | 69 (32%) | 44 (20%) | 21/40 (53%) | |
OPU | mRNA | 193 | 185 | 165 (89%) | 62 (36%) | 26(14%) | 4/24 (17%) | |
OPU | Total | 420 | 402 | 361 (90%) | 131 (33%) | 70 (17%) | 25/64 (39%) |
Although oocytes can be readily and cost-effectively sourced from local abattoirs, they are lacking information on the genetic background of the donor cow, which can be of various breeds and genetic merit. To better control for the maternal contribution of the edited embryos, we next generated IVF embryos from oocytes derived from a cohort of 14 dairy donor cows with high genetic merit. A total of 446 oocytes were recovered from four separate OPU sessions (Table 1) and were fertilised in vitro with the semen from a New Zealand Holstein Friesian bull of high genetic merit. The fertilised zygotes (n = 420) were injected with the PMEL-specific TALEN pair and ssODN repair template as before. Plasmid-injected embryos were associated with a better development rate to grade 1 and 2 blastocysts compared with mRNA-injected embryos (20% vs 14%, respectively), although this difference was not statistically significant (P = 0.13). Based on the embryo biopsies, assessment of the editing outcomes showed that HDR editing with plasmid-encoded TALENs again outperformed injection of the TALENs as mRNA. The efficiency of full conversion into the precision HDR genotype was significantly higher (P = 0.0082) with zygotes injected with TALEN plasmids (53%) than with TALEN mRNA injections (17%).
Transfer of edited embryos for development to term
We initially transferred 15 injected OPU-derived IVF embryos where the biopsy had shown complete, non-mosaic HDR editing and 10 non-edited OPU control embryos into recipients. This only generated a single pregnancy with the edited (1/15; 7%) and four with the control embryos (4/10; 40%) at gestational day 35 (Table 2). Because of the low pregnancy rate with the PMEL-edited embryos, we transferred additional edited and non-edited control embryos, also including some embryos that were produced with abattoir-derived oocytes on two occasions, 2 days apart (embryo transfers 2 and 3). This resulted in four additional PMEL OPU pregnancies (4/11; 36%), no further OPU control pregnancies (0/4; 0%), seven pregnancies with abattoir-derived embryos (7/17; 41%) and one pregnancy for the abattoir-derived control embryos (1/8; 13%). Except for one PMEL abattoir pregnancy, which was no longer detectable at the second scan at day 60 of gestation, all held to term (Table 2). However, two calves were lost thereafter: one abattoir-derived calf was stillborn (ID 29) and one OPU-derived, low birth weight calf (ID 28) was euthanised on welfare grounds. Rather than the biallelic precision PMEL-edits of these calves, both losses were likely attributable to in vitro production of embryos, which is associated with altered birth weight and increased calf mortality (Bonilla et al. 2014). Furthermore, after a few days it became apparent that calf 15 had impaired vision. Following veterinary assessment, it was concluded that the calf was blind, and it was subsequently euthanised on animal welfare grounds.
Embryo transfer | Genotype | Oocyte source | Embryos transferred | Pregnancy at D37 of gestation (%) A | Development to term (%) B | Development to weaning (%) B | |
---|---|---|---|---|---|---|---|
1 | 3 bp del. | OPU | 15 | 1 (7) | 1 (7) | 0 (0) | |
WT | OPU | 10 | 4 (40) | 4 (40) | 4 (40) | ||
2 | 3 bp del. | OPU | 5 | 1 (14) | 1 (14) | 1 (14) | |
WT | OPU | 2 | 0 (0) | 0 (0) | 0 (0) | ||
3 bp del. | Abattoir | 9 | 3 (33) | 2 (22) | 1 (11) | ||
WT | Abattoir | 4 | 1 (25) | 1 (25) | 1 (25) | ||
3 | 3 bp del. | OPU | 5 | 3 (60) | 3 (60) | 2 (40) | |
WT | OPU | 2 | 0 (0) | 0 (0) | 0 (0) | ||
3 bp del. | Abattoir | 9 | 4 (44) | 4 (44) | 4 (44) | ||
WT | Abattoir | 4 | 0 (0) | 0 (0) | 0 (0) |
Genotype and genetic relationship
Editing outcomes at the PMEL target site were determined with DNA prepared from blood and fibroblasts derived from an ear biopsy. Results from both DNA sources were consistent and revealed that three of the five edited OPU calves and five of six abattoir-derived calves produced were 100% edited for the intended 3 bp deletion variant (Table 3). The calves that were not fully converted showed a range of different on-target genotypes. The different edited PMEL alleles and impacts of the edits on the PMEL reading frame are shown in Fig. 1. Besides the intended 3 bp deletion, the blind OPU calf (ID 15) had a second allele with a 6 bp deletion. To determine whether the calf was mosaic or heterozygous for the two alleles, we isolated cell clones from ear biopsy-derived fibroblasts. Both deletion alleles were determined in 20 cell clones with only one cell clone showing a lower than the expected 50% presence of the 6 bp deletion allele (1/20; 5%). Overall, it provided evidence that the calf was heterozygous for the intended allele and most likely for the 6 bp deletion allele.
Calf ID | Oocyte source | Sex | Edited | On-target mutation | HDR allele contribution (%) A | |
---|---|---|---|---|---|---|
15 | OPU | F | Yes | 3 bp deletion | 50 | |
6 bp deletion | 50 | |||||
22 | OPU | M | Yes | 3 bp deletion | 100 | |
24 | OPU | M | Yes | 3 bp deletion | 100 | |
25 | OPU | F | Yes | 3 bp deletion | 63 | |
4 bp deletion | 24 | |||||
12 bp deletion | 11 | |||||
28 | OPU | M | Yes | 3 bp deletion | 100 | |
14 | OPU | M | No | WT | NA | |
16 | OPU | M | No | WT | NA | |
17 | OPU | M | No | WT | NA | |
18 | OPU | F | No | WT | NA | |
20 | Abattoir | F | Yes | 5 bp deletion | 50 | |
163 bp deletion | 50 | |||||
21 | Abattoir | F | Yes | 3 bp deletion | 100 | |
23 | Abattoir | F | Yes | 3 bp deletion | 100 | |
26 | Abattoir | F | Yes | 3 bp deletion | 100 | |
27 | Abattoir | M | Yes | 3 bp deletion | 100 | |
29 | Abattoir | M | Yes | 3 bp deletion | 100 | |
19 | Abattoir | F | No | WT | NA |
PMEL alleles determined in edited and control calves. Depicted is the DNA sequence of the non-edited, wild type (WT) allele corresponding to the PMEL signal peptide with amino acids shown in single letter code beneath the DNA sequence. The PMEL deletion alleles generated by genome editing are illustrated via their amino acid sequences with dashes indicating deletions of individual base pairs. Because the 163 bp deletion allele also deletes the PMEL start codon and has no valid PMEL reading frame, the DNA sequence was provided for this allele. Shown are all alleles for the control calves (WT; calves 14, 16–19), the fully HDR-edited calves (3 bp del: 3 bp deletion p.Leu18 del HDR mutation; calves 21–24, 26–29), calf 15 with two deletion alleles (3 bp del and 6 bp del), calf 25 with three deletion alleles (3 bp del, 4 bp del and 12 bp del) and calf 20 (5 bp del and 163 bp del).
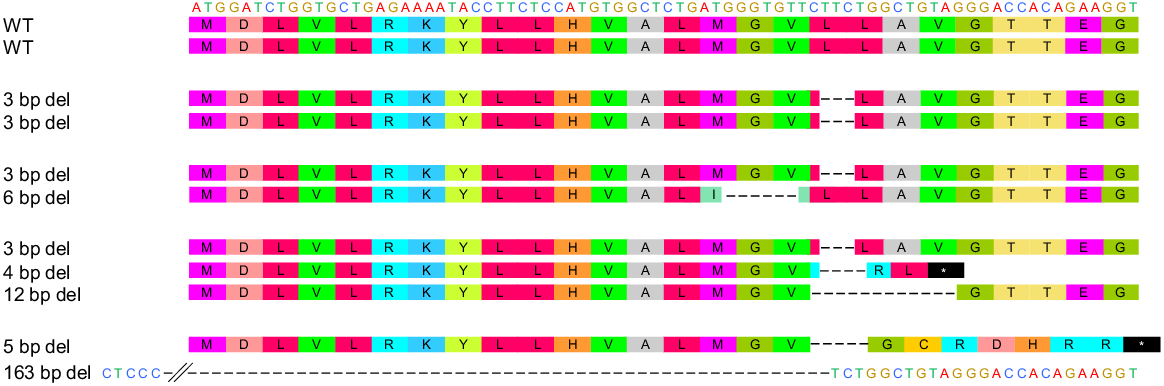
For the other OPU calf (ID 25), two minor deletion alleles (4 bp/24% and 12 bp/11%) were detected by TIDE analysis in addition to the intended 3 bp HDR allele (63%), whereas the abattoir-derived calf (ID 20) possessed 5 bp and 163 bp deletion alleles and lacked the 3 bp HDR allele. Again, cell clones were isolated and analysed to confirm the allelic variants and sequence at the target site. For calf 25, 30% of the 20 isolated cell clones (6/20) were homozygous for the intended 3 bp HDR allele, 45% (9/20) were heterozygous for the 3 bp HDR and 4 bp deletion allele and 25% (5/20) were heterozygous for the 3 bp HDR and 12 bp deletion allele. This confirmed that calf 25 was mosaic.
Analysis of the genotypes of 17 cell clones derived from calf 20 revealed consistent amplification of two PCR fragments that comprised the 5 bp and 163 bp deletion alleles, both disrupting the reading frame.
Since the OPU calves were produced with oocytes from a pool of high breeding worth donor cows, we wanted to establish the relationship between the edited and control OPU calves. The SNP chip analysis confirmed that OPU calves 14, 15, 16, 22 and 24 were siblings and only half-siblings to OPU calves 17, 18, 25 and 28. The abattoir-derived calves were produced with semen from a different bull than the OPU calves and had an unknown maternal genetic background. Therefore, the abattoir-derived calves were not further tested for sister/brother relationship and were classified as half-siblings to each other.
Since plasmids were used to deliver TALENs, all edited calves were assessed for potential integration of the TALEN vectors. Calf 20 was the only calf that was edited following delivery of the TALENs by mRNA and served as an internal negative control. A 336 bp fragment of the N-terminal TALE domain and part of both TALEN plasmids was detectable by PCR in the control samples but failed to amplify from genomic DNA of the edited calves. By contrast, parallel amplification of a fragment from an endogenous bovine gene was successful for all calves indicating the presence of amplifiable DNA and the absence of integrated plasmids in all PMEL-edited calves (Fig. S2).
Coat colour phenotype
The OPU-derived, non-edited control calves showed a predominantly black coat with only small areas of white hair (Fig. 2). By contrast, the fully HDR-edited (p.Leu18del-edited) OPU calves 22, 24 and 28, as well as the heterozygous calf 15 with the 3 bp and 6 bp deletion alleles, displayed a strong colour dilution phenotype with grey and white coats. The direct comparison of edited and non-edited OPU calves revealed that besides lightening black, p.Leu18del editing markedly reduced darker areas compared to the controls. The mosaic OPU calf 25 had only a few small grey and white patches and mainly a darker colouring that was nevertheless noticeably lighter in colour than the controls, particularly around the head.
Coat colour phenotype of PMEL-edited and non-edited control calves. The pictures depict the calves within their first week, except for 14 and 16 which are shown at 2 months of age. Source of oocytes used for their production is indicated on the left and calf IDs are indicated below the images.
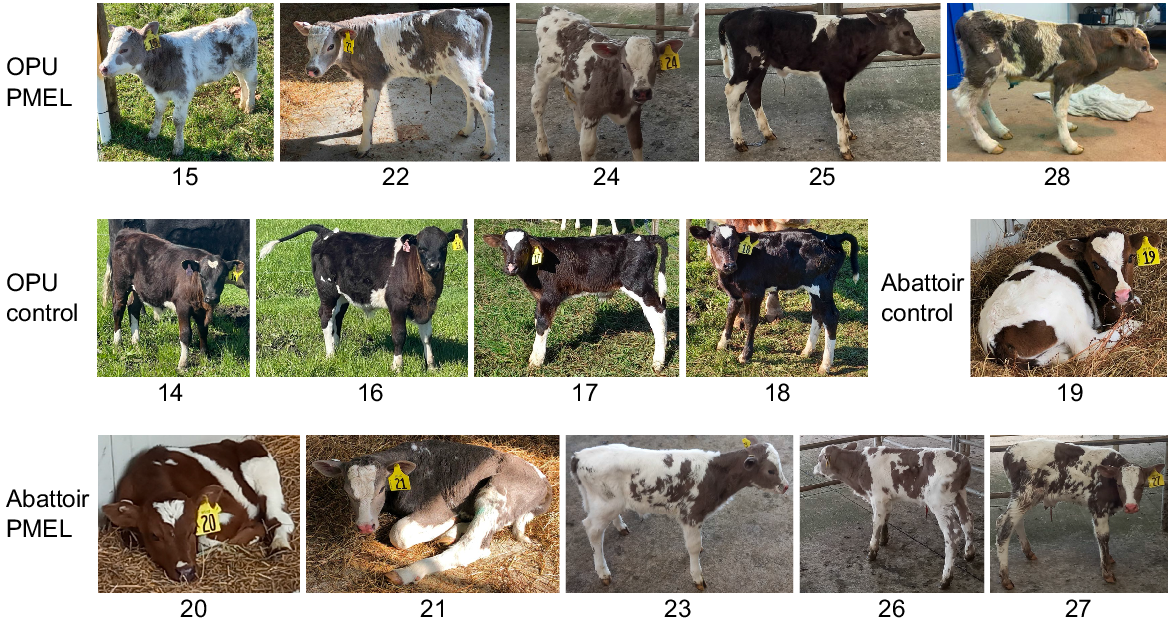
The completely p.Leu18del-edited abattoir-derived calves 21, 23, 26 and 27 displayed the characteristic grey and white colour dilution phenotype. The appearance of calf 21 was unique in that its coat was mainly the colour-diluted grey with very little white spotting.
The only calf that showed no obvious sign of colour dilution was the abattoir-derived calf 20. This calf did not possess the intended 3 bp deletion allele but was heterozygous for two edited alleles, both with frameshift mutations in PMEL most likely resulting in complete disruption of PMEL function. Further, it showed a brown and white coat, rather than the characteristic black and white pattern of wild type Holstein Friesian cattle or the diluted grey and white phenotype of the PMEL mutation. The abattoir control calf 19 was of a similar brown and white coat colour phenotype, which highlighted the genetic diversity of genetic backgrounds for the abattoir-derived calves. Over time, the brown colour of calf 19 changed into a blackish phenotype, while the edited calf 20 retained its brown appearance (Fig. S1).
Impact of coat colour on absorption of thermal energy
To assess what impact the diluted coat colour has on radiative heat gain, we determined the absorbance across the visible and NIR spectra in four genetically closely related calves. This included the OPU-derived PMEL calves 22 and 24, which had fully converted HDR-edited genotypes, and their two non-edited OPU siblings 14 and 16.
Deduced from selected pixels in light and dark spots, the p.Leu18del-edited calves absorbed incident light more strongly in the green–orange wavelengths and, therefore, reflected more strongly yellow–red and blue wavelengths which results in a grey/brownish appearance (Fig. 3a, b). Across the NIR spectrum they absorbed incident radiant heat energy evenly. The control calves’ black and white hair absorbed or reflected, respectively, evenly across both the visible and NIR spectra. The black hair of the controls showed the highest absorbance in the NIR range and was about 30% lower for the dark hues in p.Leu18del-edited calf 22 and approximately 50% lower for calf 24 (Fig. 3a).
Average absorbance across the visible and NIR spectra for light and dark spots of each calf’s coat. (a) Shown is the average absorbance for three pixels selected in a coat region with dark hues on the left side trunk of the indicated calves. (b) Average absorbance for three pixels selected in a coat region with light hues. As a reference the absorbance of the NIR reference tile was included.
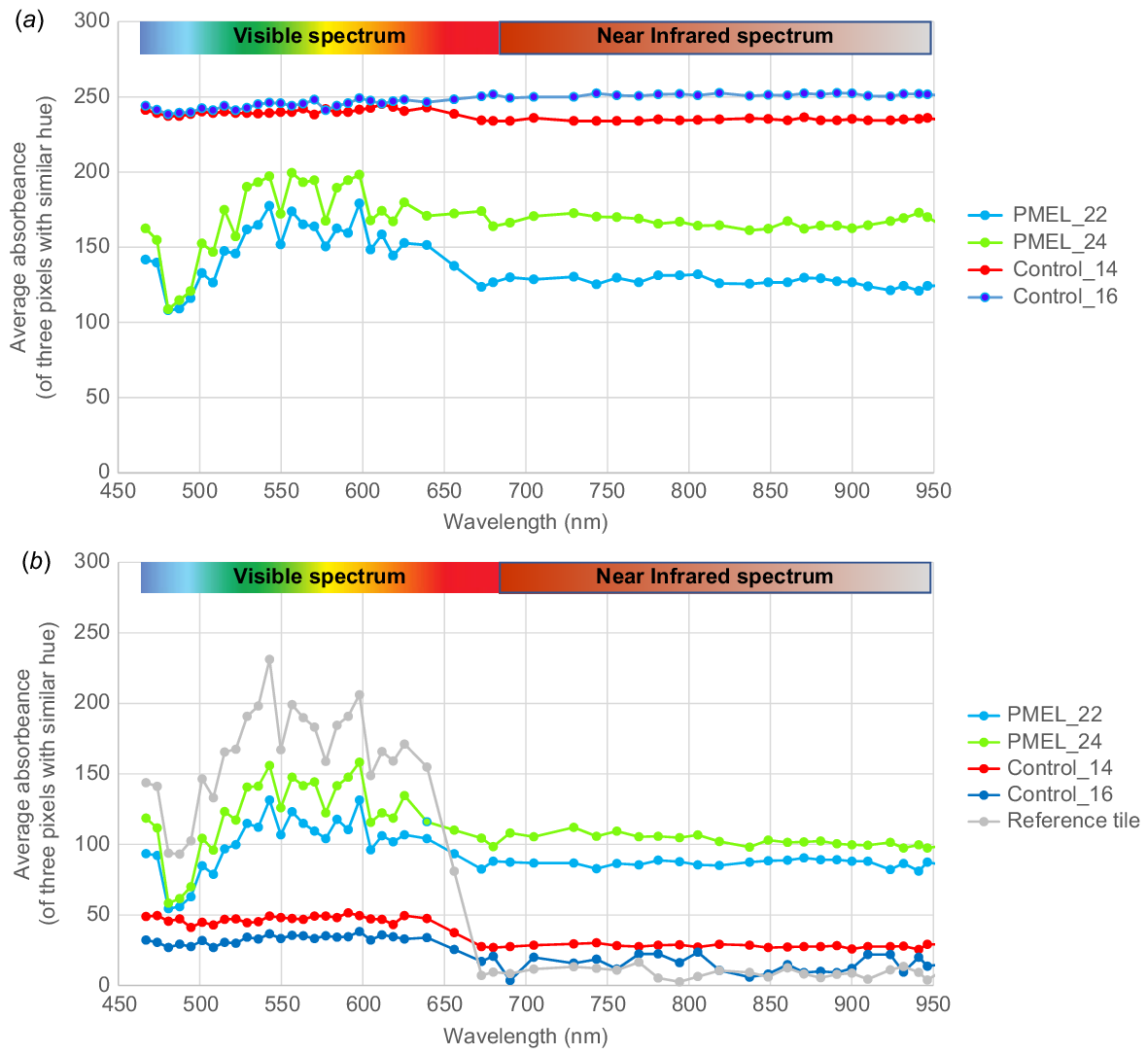
To better assess the impact of the p.Leu18del mutation which resulted in coat colour dilution and reduction of darker coat areas, we averaged values across all pixels of the entire scanned region (Fig. S3). The p.Leu18del-edited calves had lower overall absorption scores (Visible = 46–50; NIR = 58–60) than control calves 14 and 16 (Visible = 96 and 97; NIR = 98 and 98), in both spectra (Fig. 4). This represented a 39–41% reduction of thermal energy absorption in the NIR spectrum for the PMEL calves.
Overall absorbance scores of p.Leu18del-edited cattle and non-edited siblings. Shown are the values for visible and infrared spectra for the scanned left side trunk of the OPU-derived control calves 14 and 16 (Control_14, Control_16); scanned white patches of control calves 14 and 16 (WhitePatch_14, WhitePatch_16) and scanned left side trunk of the OPU-derived p.Leu18del-edited calves 22 and 24 (PMEL 22, PMEL 24).
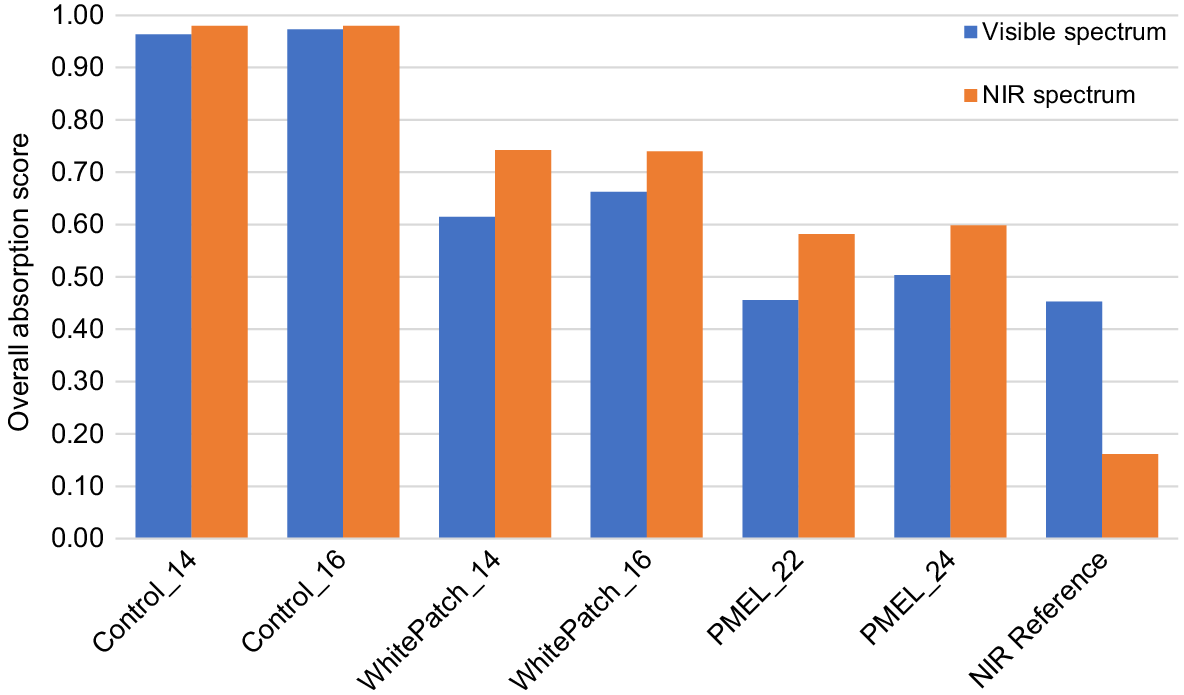
One of the few markings with visually strong overall white appearance on the control animals (white patch) was independently analysed for their overall absorbance scores (Figs S3 and 4). In both animals, even the white patches had higher absorption (Visible = 61 and 66; NIR = 74 and 74) compared to the two p.Leu18del-edited calves which were 19–23% lower.
Discussion
Transfer of the vitrified, biopsied embryos resulted in highly variable pregnancy rates. However, the observed variability for pregnancy establishment showed no correlation with the type of embryo transferred. Non-edited, wild-type (WT) embryos, as well as HDR-edited OPU- and abattoir-derived embryos, resulted in highly variable embryo transfer efficiencies across three independent experimental runs. Limited access to a small group of recipient cows in containment and their sporadic use over long periods of time might have both contributed to the variable pregnancy outcomes.
The embryo-mediated approach applied here avoids somatic cloning-related inefficiencies in producing healthy live calves that we and others have experienced (Liu et al. 2013; Luo et al. 2014; Wu et al. 2015; Carlson et al. 2016; Carvalho et al. 2019; Laible et al. 2021). However, microinjecting editors into zygotes offers less control over the edited genotypes that are achieved and fixed in the resulting calves (Hennig et al. 2020). This is particularly problematic for the introduction of precise sequence changes based on HDR, which competes with the more efficient NHEJ repair and frequently results in mosaic animals (Park et al. 2020). To avoid production of calves with unintended edits, we analysed trophoblast biopsies as a proxy for the editing status of the embryo. This strategy appeared to be suitable to identify successfully HDR-edited embryos in a previous study, which was based on only three edited calves that were produced from biopsied embryos (Wei et al. 2018). With increased numbers (11 HDR-edited calves), the present study revealed some shortcomings in the accuracy of the biopsy screening. Only eight of the 11 calves were fully edited with the intended 3 bp deletion in the PMEL gene and the other three possessed alleles with unintended edits.
Trophoblast biopsies have been successfully used for predicting genomic estimating breeding values (Mullaart and Wells 2018; Fujii et al. 2019). However, genome editing introduces further complexities with the potential to generate mosaic genotypes. Concerns about the ability to accurately predict editing outcomes from trophoblast biopsies were raised previously (Vilarino et al. 2018). The paired gRNA/Cas9 approach to disrupt the PDX1 gene in sheep embryos generated a high level of mosaic editing outcomes, producing discordant results between biopsies and corresponding embryos and fetuses. Further, the results depended on the screening assay, with PCR relying on differential fragment sizes and ddPCR results showing approximately 70% and 50%, respectively, of concordance between biopsies and embryos.
This highlights the importance of the screening strategy, which needs to be sufficiently sensitive to detect all intended and unintended on-target edits, even if present at low levels. Amplification by PCR from low amounts of starting material provides sensitivity but can be prone to contamination and introduction of amplification bias resulting in allelic dropouts (Piyamongkol et al. 2003; Dreesen et al. 2008; Liang et al. 2023). This might explain the observed discordance between biopsies and calves 15 and 25 with additional alleles that had not been detected in the corresponding biopsy samples. However, it is less likely to explain the complete change from the 3 bp deletion HDR allele as the sole allele detected in the biopsy to two different deletion alleles in calf 20. This could be due to the limitation of the biopsy sample not necessarily representing the inner cell mass which gives rise to the embryo proper. However, preimplantation genetic testing suggests that the concordance of mosaic aneuploidies in trophoblast vs inner cell mass cells is very high, both in human and bovine blastocysts (Lawrenz et al. 2019; Tutt et al. 2021). Due to small size (about 10% of the blastocyst cells) and trophoblast-restricted sampling, biopsies may not fully represent their corresponding embryos, which limits the accurate prediction of editing outcomes. Consequently, mosaicism should be minimised by maximising the efficiency for the intended HDR edits. Likewise, the use of multiple screening assays and amplification strategies is important to reduce the potential for allelic dropouts. While the biopsy approach might not be able to deliver absolute certainty, it can be expected to provide a strong enrichment in animals with the intended genotypes.
Of the calves with unintended genotypes, calf 20 had two alleles disrupting PMEL expression but no obvious coat colour dilution phenotype. This is consistent with a mouse PMEL knockout model which showed only very subtle effects on the coat colour phenotype in four different genetic backgrounds, while the mice were otherwise healthy (Hellström et al. 2011). The mosaic calf 25 had only some cells that are homozygous for the p.Leu18del mutation, most likely associated with patches of coat showing the colour-diluted grey. All other cells are heterozygous for the HDR allele and have either an additional 4 bp deletion nonsense allele or a 12 bp in frame deletion. The slight lightening of the coat compared to the black coats of its non-edited sibling could be the impact of the heterozygous HDR allele (Schmutz and Dreger 2013; Kimura et al. 2022). The presence of the two non-intended alleles generated no obvious additional phenotypes.
This was different with calf 15, which was fully colour-diluted but heterozygous for the HDR allele with an unintended 6 bp deletion as its second allele and was confirmed to be blind.
In horses, a PMEL missense mutation (p.Arg618Cys) in the transmembrane domain causative for coat colour dilution has been reported to be associated with vision impairments, most severely affecting homozygous carriers (Komáromy et al. 2011; Andersson et al. 2013). Multiple missense mutations in different functional domains of the PMEL protein were identified in independent human pedigrees as causative for pigmentary glaucoma (Lahola-Chomiak et al. 2019). Moreover, a coat dilution phenotype, Merle, found in dogs has been shown to result from an insertion of a repetitive short interspersed nuclear element just upstream of PMEL exon 11 that affects splicing and can generate abnormal PMEL proteins (Varga et al. 2020). Carriers of the Merle genotype predispose these dogs to hearing and/or vision impairments (Clark et al. 2006). The described mutations have in common that they affect functional domains of the protein itself, resulting in defective processing of these PMEL variants and impaired ability to form the amyloid fibrillar sheets required for melanin deposition (Andersson et al. 2013; Lahola-Chomiak et al. 2019). The 6 bp deletion mutation reported here is located within the signal peptide of the protein but appears to also interfere with correct processing of the protein, ultimately affecting vision either alone or in combination with the 3 bp deletion variant. This is not the case for the other edited mutations, deleting 3 bp, 4 bp, 12 bp of signal peptide or the entire signal peptide (163 bp deletion) and further research will be required to provide greater mechanistic insights.
The fate of calf 15 highlighted that our biopsy screening was not accurate enough to completely avoid the transfer of embryos with unintended on-target edits that can result in calves with compromised health. As discussed earlier, the alternative cell-mediated approach using SCNT offers better control over on-target edits but also carries an animal welfare burden as it can compromise the development and health of cloned calves (Wells 2005). Scope and continued efforts for improving the design of editors, detection of HDR editing outcomes in embryos and cloning efficiencies will help to minimise or avoid undesirable editing outcomes that adversely affect the welfare of animals in the future.
Beside inaccurate on-target HDR edits, genome editors have a known potential for the unintended introduction of mutations at off-target sites due to residual binding and cleavage at sites that share a degree of homology with the actual target site (Yee 2016). Contrary to in vitro experiments, there is increasing evidence that with well-designed editors, edited animals show very little if any off-target mutagenesis (Akcakaya et al. 2018; Li et al. 2018; Wang et al. 2018; Wei et al. 2018; Jivanji et al. 2021). Compared to Cas9 editors with a single short gRNA providing the target specificity for the Cas9 nuclease, TALEN-mediated cleavage is dependent on the concerted binding of two TALENs to enable the dimerisation of their FokI nuclease domains required for cleavage activity. This increased stringency for cleavage makes TALENs even less prone to introduce off-target mutations, which was shown in a recent comparative study between gRNA/Cas9- and TALEN-mediated editing of sheep (Zhang et al. 2019). Still, the potential for off-target events is a major concern associated with this relatively new technology. Therefore, we are in the process of analysing whole genome sequencing data to determine if any of the PMEL-edited cattle might harbour off-target mutations. Once available, it will provide further information on the editing fidelity and safety of edited livestock.
Consistent with our previous study, we achieved better efficiencies for embryos assessed as 100% HDR-edited when the PMEL-specific TALENs were delivered as plasmids compared to mRNA delivery (Wei et al. 2022). However, this approach carries a low risk for the unintended integration of a plasmid into the genome. Such integration events are rare but can be readily identified by PCR-screening or whole genome sequencing and managed accordingly. As we had reported previously for other HDR-edited cattle, we found no evidence in the PMEL-edited cattle for the integration of plasmids used for the delivery of editors into embryos (Wei et al. 2018; Jivanji et al. 2021; Laible et al. 2021).
This is contrary to a recent report where an entire plasmid became integrated at the target site (Norris et al. 2020). This plasmid had been used as donor template to provide the information for editing the polled mutation in dairy cattle. While the expression plasmids for editors play no role in the screening for editing outcomes, screening for the presence of the edited polled mutation, also present on the donor plasmid, would have increased the chance to pick up a rare plasmid integration event. The integration was initially missed because of inadequate PCR assays and analysis of whole genome sequencing results to fully characterise the on-target editing outcome (Norris et al. 2020). Informative assays would have identified the unintended genotype prior to the production of a live animal, although the unwanted plasmid can be later deleted by breeding and segregation in the next generation (Young et al. 2020).
Coats with lighter coloured hair can reflect more of the radiant heat from the exposure of grazing cattle to sunlight and reduce their heat load (Cena and Monteith 1975; Maia et al. 2005a). This improves their ability to mitigate effects of heat stress and was shown to be linked to lower surface and rectal temperatures and higher milk production in lighter compared to darker coloured cattle (Hansen 1990; Becerril et al. 1993; Maia et al. 2005b). In our study, we were able to investigate the impact of colour dilution in precisely edited cattle and their genetically closely linked non-edited siblings. Both p.Leu18del-edited calves very weakly absorbed blue light energy (the most intense wavelengths in sunlight) and red/NIR energy (associated with radiant heat from the sun). Although the selected spots in light and dark areas of the coat varied in their average absorbance for individual p.Leu18del-edited calves, the absorbance for the dark areas was markedly lower and for the lighter areas higher for the edited calves compared to the black and white spots of the controls. Importantly, the ratio of light to dark coat areas across the entire body was much greater in the edited calves compared to the almost exclusively black coats of the control animals. Averaging over a larger body section highlighted the strong reduction of thermal energy absorption by the colour-diluted coats of the pLeu18del-edited calves in comparison to the black coats of the control calves. The control calves had few patches of white coat, albeit small, that strongly stood out visually against the surrounding black coats. Despite the white appearance, the scans of these patches revealed that their absorbance ratio in the visible and NIR was indeed higher than that of the p.Leu18del-edited calves’ left sides, including light and dark areas of the colour-diluted animals. The white patches also comprised some black hairs, and the white hair of the patch might have overlapped with skin areas of strong pigmentation, which could explain the observed absorbance characteristics. Similar colour dilution effects were observed in cloned calves on an unrelated genetic background (Laible et al. 2021). This suggests that the strong lightening of the coat colour and associated reduced absorbance of thermal energy are unlikely to be dependent on the specific genetic background of the OPU-derived calves and achievable on a wider range of Holstein Friesian genetics.
In summary, we used embryo-mediated editing to introduce a known natural mutation in the PMEL gene, producing non-mosaic, coat colour-diluted calves from high genetic merit parents. Screening of embryo biopsies for complete editing strongly enriched the desired embryo genotype prior to transfer, resulting in eight calves with fully converted genotypes. Optimisation of editing efficiencies and PCR screening protocols should help to further minimise unintended outcomes. The colour dilution coats caused by the introduced p.Leu18del PMEL mutation reduced the absorption of thermal energy in the NIR spectrum which should lower the radiative heat gain of the edited calves from exposure to the sun. More in-depth characterisation of the pLeu18del-edited calves in the coming years will provide the opportunity to assess the impact of the colour dilution under hot and cold environmental conditions on behaviour, health and production traits.
Data availability
Data supporting this study are available in the article and accompanying online supplementary material.
Conflicts of interest
J.W., B.B., S-A.C., B.O., S.L., D.N.W. and G.L. are employees of AgResearch and received co-funding from CRV Ltd and Livestock Improvement Corporation in support of the Ministry of Business, Innovation and Employment (MBIE) research grant. J.B. is a part owner of patents concerning the use of TALEs and TALENs.
Declaration of funding
This study was funded by the Ministry of Business, Innovation and Employment (MBIE) (https://www.mbie.govt.nz/) Endeavour Fund CONT-62639-ENDRP-AGR, with support from CRV Ltd and Livestock Improvement Corporation.
Acknowledgements
The authors would like to thank members of the Animal Biotech team and farm staff for their support. We are grateful for funding of this study by AgResearch, CRV Ltd, Livestock Improvement Corporation and the Ministry of Business, Innovation and Employment.
References
Akcakaya P, Bobbin ML, Guo JA, Malagon-Lopez J, Clement K, Garcia SP, Fellows MD, Porritt MJ, Firth MA, Carreras A, Baccega T, Seeliger F, Bjursell M, Tsai SQ, Nguyen NT, Nitsch R, Mayr LM, Pinello L, Bohlooly-Y M, Aryee MJ, Maresca M, Joung JK (2018) In vivo CRISPR editing with no detectable genome-wide off-target mutations. Nature 561(7723), 416-419.
| Crossref | Google Scholar | PubMed |
Andersson LS, Wilbe M, Viluma A, Cothran G, Ekesten B, Ewart S, Lindgren G (2013) Equine multiple congenital ocular anomalies and silver coat colour result from the pleiotropic effects of mutant PMEL. PLoS ONE 8(9), e75639.
| Crossref | Google Scholar | PubMed |
Becerril CM, Wilcox CJ, Lawlor TJ, Wiggans OR, Webb DW (1993) Effects of percentage of white coat color on holstein production and reproduction in a subtropical environment. Journal of Dairy Science 76(8), 2286-2291.
| Crossref | Google Scholar |
Bishop TF, Van Eenennaam AL (2020) Genome editing approaches to augment livestock breeding programs. Journal of Experimental Biology 223(Suppl_1), jeb207159.
| Crossref | Google Scholar |
Bols PEJ, Vandenheede JMM, Van Soom A, de Kruif A (1995) Transvaginal ovum pick-up (OPU) in the cow: a new disposable needle guidance system. Theriogenology 43(3), 677-687.
| Crossref | Google Scholar | PubMed |
Bonilla L, Block J, Denicol AC, Hansen PJ (2014) Consequences of transfer of an in vitro-produced embryo for the dam and resultant calf. Journal of Dairy Science 97(1), 229-239.
| Crossref | Google Scholar | PubMed |
Brinkman EK, Chen T, Amendola M, van Steensel B (2014) Easy quantitative assessment of genome editing by sequence trace decomposition. Nucleic Acids Research 42(22), e168.
| Crossref | Google Scholar | PubMed |
Brophy B, Smolenski G, Wheeler T, Wells D, L’Huillier P, Laible G (2003) Cloned transgenic cattle produce milk with higher levels of β-casein and κ-casein. Nature Biotechnology 21(2), 157-162.
| Crossref | Google Scholar | PubMed |
Carlson DF, Lancto CA, Zang B, Kim E-S, Walton M, Oldeschulte D, Seabury C, Sonstegard TS, Fahrenkrug SC (2016) Production of hornless dairy cattle from genome-edited cell lines. Nature Biotechnology 34(5), 479-481.
| Crossref | Google Scholar | PubMed |
Carvalho BP, Cunha ATM, Silva BDM, Sousa RV, Leme LO, Dode MAN, Melo EO (2019) Production of transgenic cattle by somatic cell nuclear transfer (SCNT) with the human granulocyte colony-stimulation factor (hG-CSF). Journal of Animal Science and Technology 61(2), 61-68.
| Crossref | Google Scholar | PubMed |
Cena K, Monteith JL (1975) Transfer processes in animal coats. I. Radiative transfer. Proceedings of the Royal Society B: Biological Sciences 188(1093), 377-393.
| Crossref | Google Scholar | PubMed |
Clark LA, Wahl JM, Rees CA, Murphy KE (2006) Retrotransposon insertion in SILV is responsible for merle patterning of the domestic dog. Proceedings of the National Academy of Sciences of the United States of America 103(5), 1376-1381.
| Crossref | Google Scholar | PubMed |
Davis SR, Spelman RJ, Littlejohn MD (2017) Breeding and Genetics Symposium:Breeding heat tolerant dairy cattle: the case for introgression of the “slick” prolactin receptor variant into Bos taurus dairy breeds. Journal of Animal Science 95(4), 1788-1800.
| Crossref | Google Scholar | PubMed |
Dreesen J, Drusedau M, Smeets H, de Die-Smulders C, Coonen E, Dumoulin J, Gielen M, Evers J, Herbergs J, Geraedts J (2008) Validation of preimplantation genetic diagnosis by PCR analysis: genotype comparison of the blastomere and corresponding embryo, implications for clinical practice. Molecular Human Reproduction 14(10), 573-579.
| Crossref | Google Scholar | PubMed |
Fujii T, Naito A, Hirayama H, Kashima M, Yoshino H, Hanamure T, Domon Y, Hayakawa H, Watanabe T, Moriyasu S, Kageyama S (2019) Potential of preimplantation genomic selection for carcass traits in Japanese Black cattle. Journal of Reproduction and Development 65(3), 251-258.
| Crossref | Google Scholar | PubMed |
Gebremedhin KG, Lee CN, Hillman PE, Brown-Brandl TM (2011) Body temperature and behavioral activities of four breeds of heifers in shade and full sun. Applied Engineering in Agriculture 27(6), 999-1006.
| Crossref | Google Scholar |
Georges M, Charlier C, Hayes B (2019) Harnessing genomic information for livestock improvement. Nature Reviews Genetics 20(3), 135-156.
| Crossref | Google Scholar | PubMed |
Hansen PJ (1990) Effects of coat colour on physiological responses to solar radiation in holsteins. Veterinary Record 127(13), 333-334.
| Google Scholar | PubMed |
Hellström AR, Watt B, Fard SS, Tenza D, Mannström P, Narfström K, Ekesten B, Ito S, Wakamatsu K, Larsson J, Ulfendahl M, Kullander K, Raposo G, Kerje S, Hallböök F, Marks MS, Andersson L (2011) Inactivation of Pmel alters melanosome shape but has only a subtle effect on visible pigmentation. PLoS Genetics 7(9), e1002285.
| Google Scholar | PubMed |
Hennig SL, Owen JR, Lin JC, Young AE, Ross PJ, Van Eenennaam AL, Murray JD (2020) Evaluation of mutation rates, mosaicism and off target mutations when injecting Cas9 mRNA or protein for genome editing of bovine embryos. Scientific Reports 10(1), 22309.
| Crossref | Google Scholar | PubMed |
Jivanji S, Harland C, Cole S, Brophy B, Garrick D, Snell R, Littlejohn M, Laible G (2021) The genomes of precision edited cloned calves show no evidence for off-target events or increased de novo mutagenesis. BMC Genomics 22(1), 457.
| Crossref | Google Scholar | PubMed |
Kimura S, Hatakeyama T, Koutaka T, Kubo K, Morita S, Eguchi K, Saitoh K, Yamauchi K, Imai S, Kashimura A, Inenaga T, Matsumoto H (2022) PMEL p.Leu18del dilutes coat color of Kumamoto sub-breed of Japanese brown cattle. BMC Genomics 23(1), 694.
| Crossref | Google Scholar | PubMed |
Komáromy AM, Rowlan JS, La Croix NC, Mangan BG (2011) Equine multiple congenital ocular anomalies (MCOA) syndrome in PMEL17 (silver) mutant ponies: five cases. Veterinary Ophthalmology 14(5), 313-320.
| Crossref | Google Scholar | PubMed |
Lahola-Chomiak AA, Footz T, Nguyen-Phuoc K, Neil GJ, Fan B, Allen KF, Greenfield DS, Parrish RK, Linkroum K, Pasquale LR, Leonhardt RM, Ritch R, Javadiyan S, Craig JE, Allison WT, Lehmann OJ, Walter MA, Wiggs JL (2019) Non-synonymous variants in premelanosome protein (PMEL) cause ocular pigment dispersion and pigmentary glaucoma. Human Molecular Genetics 28(8), 1298-1311.
| Crossref | Google Scholar | PubMed |
Laible G, Cole S-A, Brophy B, Wei J, Leath S, Jivanji S, Littlejohn MD, Wells DN (2021) Holstein Friesian dairy cattle edited for diluted coat color as a potential adaptation to climate change. BMC Genomics 22(1), 856.
| Crossref | Google Scholar | PubMed |
Lawrenz B, El Khatib I, Liñán A, Bayram A, Arnanz A, Chopra R, De Munck N, Fatemi HM (2019) The clinicians’ dilemma with mosaicism-an insight from inner cell mass biopsies. Human Reproduction 34(6), 998-1010.
| Crossref | Google Scholar | PubMed |
Li C, Zhou S, Li Y, Li G, Ding Y, Li L, Liu J, Qu L, Sonstegard T, Huang X, Jiang Y, Chen Y, Petersen B, Wang X (2018) Trio-based deep sequencing reveals a low incidence of off-target mutations in the offspring of genetically edited goats. Frontiers in Genetics 9, 449.
| Crossref | Google Scholar | PubMed |
Liang D, Mikhalchenko A, Ma H, Marti Gutierrez N, Chen T, Lee Y, Park S-W, Tippner-Hedges R, Koski A, Darby H, Li Y, Van Dyken C, Zhao H, Wu K, Zhang J, Hou Z, So S, Han J, Park J, Kim C-J, Zong K, Gong J, Yuan Y, Gu Y, Shen Y, Olson SB, Yang H, Battaglia D, O’Leary T, Krieg SA, Lee DM, Wu DH, Duell PB, Kaul S, Kim J-S, Heitner SB, Kang E, Chen Z-J, Amato P, Mitalipov S (2023) Limitations of gene editing assessments in human preimplantation embryos. Nature Communications 14(1), 1219.
| Crossref | Google Scholar | PubMed |
Liu X, Wang Y, Guo W, Chang B, Liu J, Guo Z, Quan F, Zhang Y (2013) Zinc-finger nickase-mediated insertion of the lysostaphin gene into the beta-casein locus in cloned cows. Nature Communications 4, 2565.
| Crossref | Google Scholar | PubMed |
Luo J, Song Z, Yu S, Cui D, Wang B, Ding F, Li S, Dai Y, Li N (2014) Efficient generation of myostatin (MSTN) biallelic mutations in cattle using zinc finger nucleases. PLoS ONE 9(4), e95225.
| Crossref | Google Scholar | PubMed |
Maia ASC, daSilva RG, Battiston Loureiro CM (2005a) Sensible and latent heat loss from the body surface of holstein cows in a tropical environment. International Journal of Biometeorology 50(1), 17-22.
| Crossref | Google Scholar | PubMed |
Maia ASC, Silva RGd, Bertipaglia ECA, Muñoz MC (2005b) Genetic variation of the hair coat properties and the milk yield of holstein cows managed under shade in a tropical environment. Brazilian Journal of Veterinary Research and Animal Science 42, 181-187.
| Crossref | Google Scholar |
McLean Z, Oback B, Laible G (2020) Embryo-mediated genome editing for accelerated genetic improvement of livestock. Frontiers of Agricultural Science and Engineering 7, 148-160.
| Crossref | Google Scholar |
Norris AL, Lee SS, Greenlees KJ, Tadesse DA, Miller MF, Lombardi HA (2020) Template plasmid integration in germline genome-edited cattle. Nature Biotechnology 38(2), 163-164.
| Crossref | Google Scholar | PubMed |
Park K-E, Frey JF, Waters J, Simpson SG, Coutu C, Plummer S, Campbell M, Donovan DM, Telugu BP (2020) One-step homology mediated CRISPR-Cas editing in zygotes for generating genome edited cattle. The CRISPR Journal 3(6), 523-534.
| Crossref | Google Scholar | PubMed |
Piyamongkol W, Bermudez MG, Harper JC, Wells D (2003) Detailed investigation of factors influencing amplification efficiency and allele drop-out in single cell PCR: implications for preimplantation genetic diagnosis. Molecular Human Reproduction 9(7), 411-420.
| Crossref | Google Scholar | PubMed |
Ponsart C, Le Bourhis D, Knijn H, Fritz S, Guyader-Joly C, Otter T, Lacaze S, Charreaux F, Schibler L, Dupassieux D, Mullaart E (2013) Reproductive technologies and genomic selection in dairy cattle. Reproduction, Fertility and Development 26(1), 12-21.
| Crossref | Google Scholar | PubMed |
Ruan J, Xu J, Chen-Tsai RY, Li K (2017) Genome editing in livestock: are we ready for a revolution in animal breeding industry? Transgenic Research 26(6), 715-726.
| Crossref | Google Scholar | PubMed |
Schmutz SM, Dreger DL (2013) Interaction of MC1R and PMEL alleles on solid coat colors in highland cattle. Animal Genetics 44(1), 9-13.
| Crossref | Google Scholar | PubMed |
Tutt DAR, Silvestri G, Serrano-Albal M, Simmons RJ, Kwong WY, Guven-Ates G, Canedo-Ribeiro C, Labrecque R, Blondin P, Handyside AH, Griffin DK, Sinclair KD (2021) Analysis of bovine blastocysts indicates ovarian stimulation does not induce chromosome errors, nor discordance between inner-cell mass and trophectoderm lineages. Theriogenology 161, 108-119.
| Crossref | Google Scholar | PubMed |
Varga L, Lénárt X, Zenke P, Orbán L, Hudák P, Ninausz N, Pelles Z, Szőke A (2020) Being merle: the molecular genetic background of the canine merle mutation. Genes 11(6), 660.
| Crossref | Google Scholar |
Vilarino M, Suchy FP, Rashid ST, Lindsay H, Reyes J, McNabb BR, van der Meulen T, Huising MO, Nakauchi H, Ross PJ (2018) Mosaicism diminishes the value of pre-implantation embryo biopsies for detecting CRISPR/Cas9 induced mutations in sheep. Transgenic Research 27(6), 525-537.
| Crossref | Google Scholar | PubMed |
Wagner S, Cullum A, Wells DN, Laible G (2017) Taillessness in a cloned cow is not genetically transmitted. Cellular Reprogramming 19(6), 331-336.
| Crossref | Google Scholar | PubMed |
Wang X, Liu J, Niu Y, Li Y, Zhou S, Li C, Ma B, Kou Q, Petersen B, Sonstegard T, Huang X, Jiang Y, Chen Y (2018) Low incidence of SNVs and indels in trio genomes of Cas9-mediated multiplex edited sheep. BMC Genomics 19(1), 397.
| Crossref | Google Scholar | PubMed |
Wei J, Wagner S, Lu D, Maclean P, Carlson DF, Fahrenkrug SC, Laible G (2015) Efficient introgression of allelic variants by embryo-mediated editing of the bovine genome. Scientific Reports 5, 11735.
| Crossref | Google Scholar | PubMed |
Wei J, Wagner S, Maclean P, Brophy B, Cole S, Smolenski G, Carlson DF, Fahrenkrug SC, Wells DN, Laible G (2018) Cattle with a precise, zygote-mediated deletion safely eliminate the major milk allergen beta-lactoglobulin. Scientific Reports 8(1), 7661.
| Crossref | Google Scholar | PubMed |
Wei J, Brophy B, Cole S-A, Moormann J, Boch J, Laible G (2022) Cytoplasmic injection of zygotes to genome edit naturally occurring sequence variants into bovine embryos. Frontiers in Genetics 13, 925913.
| Crossref | Google Scholar | PubMed |
Wells DN (2005) Animal cloning: problems and prospects. Revue Scientifique et Technique 24(1), 251-264.
| Crossref | Google Scholar | PubMed |
Wu H, Wang Y, Zhang Y, Yang M, Lv J, Liu J, Zhang Y (2015) TALE nickase-mediated SP110 knockin endows cattle with increased resistance to tuberculosis. Proceedings of the National Academy of Sciences of the United States of America 112(13), E1530-E1539.
| Crossref | Google Scholar | PubMed |
Yee J-K (2016) Off-target effects of engineered nucleases. The FEBS Journal 283(17), 3239-3248.
| Crossref | Google Scholar | PubMed |
Young AE, Mansour TA, McNabb BR, Owen JR, Trott JF, Brown CT, Van Eenennaam AL (2020) Genomic and phenotypic analyses of six offspring of a genome-edited hornless bull. Nature Biotechnology 38(2), 225-232.
| Crossref | Google Scholar | PubMed |
Zhang J, Liu J, Yang W, Cui M, Dai B, Dong Y, Yang J, Zhang X, Liu D, Liang H, Cang M (2019) Comparison of gene editing efficiencies of CRISPR/Cas9 and TALEN for generation of MSTN knock-out cashmere goats. Theriogenology 132, 1-11.
| Crossref | Google Scholar | PubMed |