Effect of arachidonic acid on pre- and post-hatching in vitro bovine embryo development
L. González-Brusi A , A. Pérez-Gómez A , A. C. Quiroga A , C. Tamargo B , P. Ramos-Ibeas A and P. Bermejo-Álvarez
A Animal Reproduction Department, INIA, CSIC, Madrid 28040, Spain.
B Department of Animal Selection and Reproduction, SERIDA, Gijón 33394, Spain.
Reproduction, Fertility and Development 35(12) 614-621 https://doi.org/10.1071/RD23053
Published online: 11 July 2023
© 2023 The Author(s) (or their employer(s)). Published by CSIRO Publishing. This is an open access article distributed under the Creative Commons Attribution-NonCommercial-NoDerivatives 4.0 International License (CC BY-NC-ND)
Abstract
Context: Arachidonic acid (AA) is the precursor of prostaglandins, which may play autocrine roles during early embryo development.
Aims: To test the developmental effects of addition of AA to pre- and post-hatching culture media on in vitro–produced bovine embryos.
Methods: Pre-hatching effects of AA were tested by culturing bovine zygotes in synthetic oviductal fluid (SOF) supplemented with 100 or 333 μM AA. Post-hatching effects of AA were tested by culturing Day 7 blastocysts in N2B27 supplemented with 5, 10, 20 or 100 μM AA up to Day 12.
Key results: Pre-hatching development to blastocyst was completely abrogated at 333 μM AA, whereas blastocyst rates and cell numbers were not altered at 100 μM AA. Impaired post-hatching development was observed at 100 μM AA, whereas no effect on survival rates was noted at 5, 10 and 20 μM AA. However, a significant reduction in Day 12 embryo size was observed at 10 and 20 μM AA. Hypoblast migration, epiblast survival and formation of embryonic-disc-like structures were unaffected at 5–10 μM AA. AA exposure downregulated the genes PTGIS, PPARG, LDHA and SCD in Day 12 embryos.
Conclusions: Pre-hatching embryos are mostly irresponsive to AA, whereas AA was observed to have negative effects during early post-hatching development.
Implications: AA does not improve in vitro bovine embryo development and is not required up to early post-hatching stages.
Keywords: arachidonic, bovine, conceptus, elongation, embryo development, lipids, PPARG, prostaglandin, SCD, trophectoderm.
Introduction
Preimplantation embryo development relies on the nutrients, hormones and growth factors present in oviductal and uterine fluids. The media developed for in vitro embryo culture have been designed to roughly mimic the conditions an embryo encounters through its journey from the oviduct to the uterus (Holm et al. 1999). Diverse embryo culture media are able to sustain embryo development from the zygote stage to blastocyst formation, although embryo quality, defined as the ability to establish a viable pregnancy, is reduced compared to in vivo developed blastocysts (van de Leemput et al. 1999; Dieleman et al. 2002; Rizos et al. 2002). Around blastocyst hatching, embryonic requirements change and conventional embryo culture media are unable to support embryo development (reviewed by Perez-Gomez et al. 2021). Recently, N2B27 medium has been found to be able to sustain early post-hatching development, attaining critical developmental landmarks such as complete hypoblast migration and the formation of embryonic-disc-like (ED-like) structures (Ramos-Ibeas et al. 2020). Compared to conventional embryo culture media, such as synthetic oviductal fluid (SOF), N2B27 – originally designed for stem cell culture (Ying and Smith 2003) – exhibits a more complex composition, including hormones and diverse compounds such as lipids.
Lipids constitute a major component of the uterine fluid where embryos develop (Ribeiro et al. 2016a; Simintiras et al. 2019), playing essential structural roles in biological membranes, being involved in metabolic pathways and acting as precursors of lipid signalling molecules. Prostaglandins (PGs) constitute a group of lipid signalling molecules known to play critical roles during gestation by promoting luteolysis (PGF2α) or corpus luteum maintenance (PGE2) (Reynolds et al. 1983; Lee et al. 2012). Besides these well-known roles, PGs have been suggested to play relevant functions before maternal recognition of pregnancy, during early conceptus elongation (Dorniak et al. 2011), a period of embryo development exclusive to ungulates that spans blastocyst hatching to implantation. Several observations suggest that conceptus-derived prostaglandins may play paracrine (i.e. signalling to the endometrium) and autocrine (i.e. signalling in the conceptus) roles during conceptus elongation. As conceptus elongation progresses, the concentrations of PGs (PGF2α and PGE) and other prostanoids such as prostacyclin (PGI2), mainly produced by the conceptus, increase in the uterine fluid (Ulbrich et al. 2009). In turn, PGE2 receptors (PTGER2 and PTGER4) and the PGF2α receptor PTGFR are abundant in the conceptus trophectoderm and endometrial epithelia (Dorniak et al. 2011), and the PGI2 receptor PTGIR and the nuclear PG receptors PPARs are also present in ovine and bovine endometrial epithelia and conceptuses (Cammas et al. 2006; Ribeiro et al. 2016b).
PGs and all other prostanoids derive from arachidonic acid (AA) by the cyclooxygenase pathway. AA is a polyunsaturated omega-6 fatty acid 20:4(ω−6) that can be acquired from the diet or synthesised from the essential α-linolenic acid through an initial step of desaturation by the Δ6-desaturase FADS2, followed by 2-carbon elongation by the elongase ELOV5, then desaturation by the Δ5-desaturase FADS1 (Sergeant et al. 2016). However, it is unclear if the blastomeres of preimplantation embryos are able to convert α-linolenic into AA, and AA is not present in serum-free media such as plain SOF – which does not contain any lipid compounds – or N2B27. AA concentrations in the uterine fluid where embryos develop are responsive to progesterone (Simintiras et al. 2019). AA can be metabolised to PGs by elongated bovine conceptuses (Lewis et al. 1982; Hwang et al. 1988), and AA content in the embryo increases significantly from pre-hatching (Day 7) to elongating (Day 14) stages (Menezo et al. 1982), suggesting that AA may be required for embryo development.
The developmental effects of the addition of AA to conventional (pre-hatching) embryo culture have been scarcely explored. Two early studies observed that the addition of AA at 50 ng/mL (~164 nM) to BECM medium increased morula formation rate without achieving development to blastocyst in a single embryo culture system (Lim and Hansel 1996) and increased morula formation rate without significantly improving blastocyst rate when combined with glutathione in a group culture system (Lim and Hansel 2000). Given the novelty of the post-hatching culture system (Ramos-Ibeas et al. 2020), the addition of AA to post-hatching culture has not been tested. Testing the addition of AA following hatching is particularly relevant, as this developmental period is particularly susceptible to embryonic loss in cattle (Dunne et al. 2000; Diskin and Morris 2008; Berg et al. 2010), prostaglandin secretion by the conceptus increases significantly along conceptus elongation (Lewis et al. 1982; Ulbrich et al. 2009), and the expression of different prostaglandin receptors which may mediate autocrine signalling also increases in the elongating conceptus (Cammas et al. 2006; Dorniak et al. 2011; Ribeiro et al. 2016b). In this study, we have analysed the developmental effects of the addition of AA to pre- and post-hatching culture media on in vitro–produced bovine embryos in two independent experiments.
Materials and methods
In vitro production of bovine blastocysts
Bovine embryos were produced in vitro as previously described (Bermejo-Alvarez et al. 2011) with specific modifications in the culture system tailored to reliably test the addition of a lipophilic compound naturally present in serum. Briefly, immature cumulus-oocyte complexes (COCs) were obtained by aspirating 2–8 mm follicles from bovine ovaries collected at local slaughterhouses. COCs with homogeneous cytoplasm and intact cumulus cells were selected and matured for 24 h in groups of 50 in 500 μL of TCM-199 medium supplemented with 10% (v/v) fetal calf serum (FCS) and 10 ng/mL epidermal growth factor (EGF) at 38.5°C under an atmosphere of 5% CO2 in air with maximum humidity. For in vitro fertilisation (IVF), matured COCs were inseminated with frozen-thawed bull sperm separated through a gradient of 40–80% BoviPure (Nidacon), at a final concentration of 106 spermatozoa/mL. Gametes were co-incubated in 500 μL of Tyrode’s albumin lactate pyruvate (TALP) medium supplemented with 10 mg/mL heparin at 38.5°C in a water saturated atmosphere of 5% CO2. Semen from the same bull was used for all experiments to avoid a possible confounding bull effect on developmental rates and to reduce inter-replicate variation. At approximately 20 h post-insemination (hpi), presumptive zygotes were denuded by vortexing for 3 min and cultured in vitro. To contrast the hypothesis of a potential beneficial effect of AA addition to the media used for in vitro culture of bovine embryos, two experiments were conducted.
For Experiment 1 (effect of AA during pre-hatching culture), presumptive zygotes were cultured in groups of ~50 in 500 μL of SOF (Holm et al. 1999) supplemented with 3 g/L of bovine serum albumin (BSA) essentially fatty acid free (Sigma A6003; Sigma-Aldrich) and different concentrations of AA as described below. The use of mineral oil during culture was avoided to impede the migration of AA from the aqueous culture medium to the mineral oil, and serum was not used to avoid the addition of the AA naturally present in blood. To prevent media evaporation, 4-well dishes were used and the inner cavity surrounding the wells was filled with distilled water. AA (Sigma A3611; Sigma-Aldrich) addition was tested at two concentrations (100 or 333 μM) resulting in three experimental groups: no AA (Control), AA 100 μM and AA 333 μM. As AA was dissolved in ethanol, an equivalent amount of ethanol (0.3%) was added to the control group. Half of the culture medium was replaced by fresh medium on Days 3 and 6 post-fertilisation, and culture took place at 38.5°C in an atmosphere of 5% CO2, 5% O2 and 90% N2 with maximum humidity. Developmental rates were recorded at 48 hpi (cleavage rate) and at Day 7 and Day 8. At Day 8, blastocysts were fixed in a solution of 4% paraformaldehyde (PFA) in PBS for 15 min at room temperature (RT) and stored in PBS-1% BSA at 4°C until analysis.
For Experiment 2 (effect of AA during post-hatching culture), presumptive zygotes were cultured in 25 μL drops of SOF medium supplemented with 5% fetal calf serum under mineral oil up to Day 7. Beyond Day 7, embryo culture was performed as described in Ramos-Ibeas et al. (2020) but starting exposure to N2B27 medium at an earlier stage (Day 7), a modification that improves post-hatching developmental rates (Ramos-Ibeas et al. 2023). N2B27 medium is composed of 1:1 Neurobasal and DMEM/F12 media supplemented with penicillin/streptomycin, 2 mM glutamine, N2 and B27 supplements (Gibco). A detailed comparison between SOF and N2B27 media composition can be found in Ramos-Ibeas et al. (2020). Day 7 blastocysts (6–15/well) were transferred to 500 μL N2B27, and AA addition was tested at four concentrations (5, 10, 20 and 100 μM), resulting in five experimental groups: no AA (Control), AA 5 μM, AA 10 μM, AA 20 μM and AA 100 μM. As AA was dissolved in ethanol, an equivalent amount of ethanol (0.3%) was added to the control group. Half of the media was replaced at Day 9 and Day 11, and culture took place at 38.5°C in an atmosphere of 5% CO2, 5% O2 and 90% N2 with maximum humidity. At Day 12, survival rates were recorded (alive embryos were able to maintain the blastocoel, whereas dead embryos collapsed, see Supplementary Fig. S1), pictures were taken on a stereomicroscope (Zeiss Stemi 305) to measure embryo diameter, and surviving embryos were snap frozen in liquid nitrogen and stored at −80°C until transcriptional analysis or fixed in a solution of 4% PFA in PBS for 15 min at RT and stored in PBS-1% BSA at 4°C until immunofluorescence analysis.
Immunofluorescence analysis
Fixed embryos were washed in PBS-1% BSA, permeabilised in 1% Triton X-100 in PBS for 15 min at RT and blocked in 10% Donkey Serum-0.02% Tween 10 in PBS for 1 h at RT. Then embryos were incubated overnight at 4°C with primary antibodies to detect trophectoderm (CDX2, Biogenex MU392A-UC, 1:100 dilution), hypoblast (SOX17, R&D AF1924, 1:100 dilution) or inner cell mass/epiblast (SOX2, Invitrogen 14-9811-80, 1:100 dilution) cells. Day 8 blastocysts were immunostained to detect trophectoderm and inner cell mass, and trophectoderm, hypoblast and epiblast lineages were detected on Day 12 embryos. After four washes in PBS-1% BSA, embryos were incubated in the appropriate secondary Alexa-conjugated antibodies (Donkey anti-rat IgG Alexa Fluor 488, Donkey anti-goat IgG Alexa Fluor 555 and Donkey anti-mouse IgG Alexa Fluor 647, Life Technologies) and DAPI for 1 h at RT, followed by four washes in PBS-1% BSA. Finally, embryos were mounted on incubation chambers as described in Bermejo-Alvarez et al. (2012) to preserve their 3D structure and imaged using structured illumination equipment composed of a Zeiss Axio Observer microscope coupled to ApoTome.2 (Zeiss). Hypoblast migration was considered complete when all the inner surface of the trophectoderm was covered by SOX17+ cells. Epiblast survival was identified by the presence of SOX2+ cells in the embryo, whereas ED-like formation was identified by the presence of a compact structure containing SOX2+ cells.
Transcriptional analysis by quantitative PCR
Poly(A) mRNA was extracted from five pools of three Day 12 embryos of each group (Control, AA 5 μM and AA 10 μM) using the Dynabeads mRNA Purification kit (Life Technologies) following the manufacturer’s instructions with minor modifications (Bermejo-Alvarez et al. 2011). Briefly, 100 μL of lysis buffer was added to the sample and incubated at RT for 10 min with gently shaking. Then, 20 μL of beads was added and samples were incubated at RT for 5 min with gently shaking, allowing the formation of bead/mRNA complexes. Finally, bead/mRNA complexes were washed twice in washing buffer A and twice in washing buffer B and resuspended in 10 mM Tris-HCl pH 7.5. Immediately after extraction, samples were treated with DNAse (Promega) at 37°C for 5 min followed by enzyme denaturation at 90°C for 5 min. Retrotranscription reaction was carried out with qScript cDNA Supermix (Quantabiosciences) in a total volume of 20 μL. Tubes were incubated at 25°C for 5 min followed by 42°C for 60 min and 85°C for 5 min.
Quantitative PCR (qPCR) analysis was conducted in duplicate for all genes of interest. Prior to the analysis, all primers used (Table 1) were tested to display qPCR efficiencies above 0.9. qPCR was performed by adding 2 μL of diluted cDNA of each sample to the PCR mix (GoTaq qPCR Master Mix, Promega) containing the specific primers. The comparative cycle threshold (CT) method was used to quantify expression levels. Fluorescence was acquired in each cycle to determine the threshold cycle. According to the comparative CT method, the ΔCT value was determined by subtracting the endogenous control H2AFZ CT value – tested for stability in previous publications (Bermejo-Alvarez et al. 2010a; Bermejo-Alvarez et al. 2011) – of each sample from the CT value of each gene in the sample. ΔΔCT was calculated using the highest CT group value (i.e. the group with the lowest target expression) as an arbitrary constant to be subtracted from all other sample values. Fold changes in the relative gene expression of the target were determined using the formula 2−ΔΔCT.
Gene | Primer sequence (5′−3′) | Fragment size (bp) | GenBank accession no. |
---|---|---|---|
H2AFZ | AGGACGACTAGCCATGGACGTGTG CCACCACCAGCAATTGTAGCCTTG | 209 | NM_174809.2 |
IFNT2 | GCTATCTCTGTGCTCCATGAGATG AGTGAGTTCAGATCTCCACCCATC | 359 | NM_001015511.3 |
PTGS2 | CTGGCGGTAGGAATCTTCCA CCGAAAGTGCTAGGCTTCCA | 349 | NM_174445.2 |
PTGES | CGCGCTGCTGGTCATCAAA CTGAGGCAGCGTTCCACAT | 190 | NM_174443.2 |
PTGIS | GAGCAGAGCAGCCCATATCA GGGACTCAGGAAGGGAAAGAG | 185 | NM_174444.1 |
PTGFR | GGTCATCCAGCTTCTGGGTAT AACAGCGTCTGGTACACACATA | 227 | NM_181025.3 |
PPARG | GCCCAAGTTCGAGTTTGCTG AGGCTTGCAGCAGATTGTCT | 155 | NM_037343.1 |
SCD | CCACACTCGTGCCATGGTAT TGGCAGCCATGCAATCAATG | 300 | NM_174809.2 |
CS | ATCCTCCTAGAGCAGGGCAA TGTGCTCATGGACTTGGGTC | 204 | NM_001044721.1 |
LDHA | TTCTTAAGGAAGAACATGTC TTCACGTTACGCTGGACCAA | 310 | NM_174099.2 |
Statistical analysis
Data were analysed using the statistical software SigmaStat (Jandel Scientific). Cleavage, blastocysts and Day 8 to Day 12 survival rates and cell numbers were analysed by one-way ANOVA, whereas differences in complete hypoblast migration, epiblast survival and ED-like formation were analysed by Chi-square test.
Results
AA addition during conventional in vitro culture (i.e. from Day 1 zygotes to Day 8 blastocysts) exerted a dose-dependent effect on embryo development (Table 2). Cleavage rates at 48 h after insemination – embryos cleaved out of presumptive zygotes in culture, n = 263 – were not affected by AA addition up to 333 μM, but blastocyst formation was completely abolished when embryos were exposed to AA at 333 μM. In contrast, the addition of AA at 100 μM did not impact negatively embryo development up to the blastocyst stage, as developmental rates – embryos developing to blastocyst out of presumptive zygotes in culture – were comparable to the control (Table 2). Lineage allocation and cell proliferation were also unaffected by the addition of AA at 100 μM, as no differences were noted in total, inner cell mass or trophectoderm cell numbers in Day 8 blastocysts cultured in SOF supplemented with 100 μM AA or without AA (Table 3, Fig. 1a).
Group | Replicates | Zygotes | Cleaved | Day 7 blastocysts | Day 8 blastocysts |
---|---|---|---|---|---|
Control | 4 | 156 | 85.6 ± 3.7% (125)a | 25.3 ± 1.4% (37)a | 32.2 ± 2.5% (47)a |
AA 100 μM | 4 | 154 | 83.1 ± 3.9% (128)a | 22.7 ± 3.3% (35)a | 28.6 ± 1.7% (44)a |
AA 333 μM | 3 | 73 | 84.9 ± 3.6% (62)a | 0 ± 0 (0)b | 0 ± 0 (0)b |
Developmental rates (out of zygotes cultured) are represented as mean ± s.e.m. (number). Different superscript letters within each column indicate statistically significant differences based on ANOVA (P < 0.05).
Group | n | Total | ICM | TE |
---|---|---|---|---|
Control | 13 | 80.2 ± 11.4 | 17.5 ± 2.7 | 62.7 ± 10.0 |
AA 100 μM | 14 | 87.5 ± 9.8 | 19.9 ± 2.2 | 67.6 ± 8.0 |
Representative images of immunohistochemistry to detect embryonic lineages at different developmental days. (a) Day 8 blastocysts were stained for SOX2 (inner cell mass) and CDX2 (trophectoderm), nuclei were counterstained with DAPI. (b) Day 12 embryos were stained for SOX2 (epiblast), SOX17 (hypoblast, partial migration in upper image, complete migration in lower image) and CDX2 (trophectoderm), nuclei were counterstained with DAPI. Pictures are maximum projections of z-stacks.
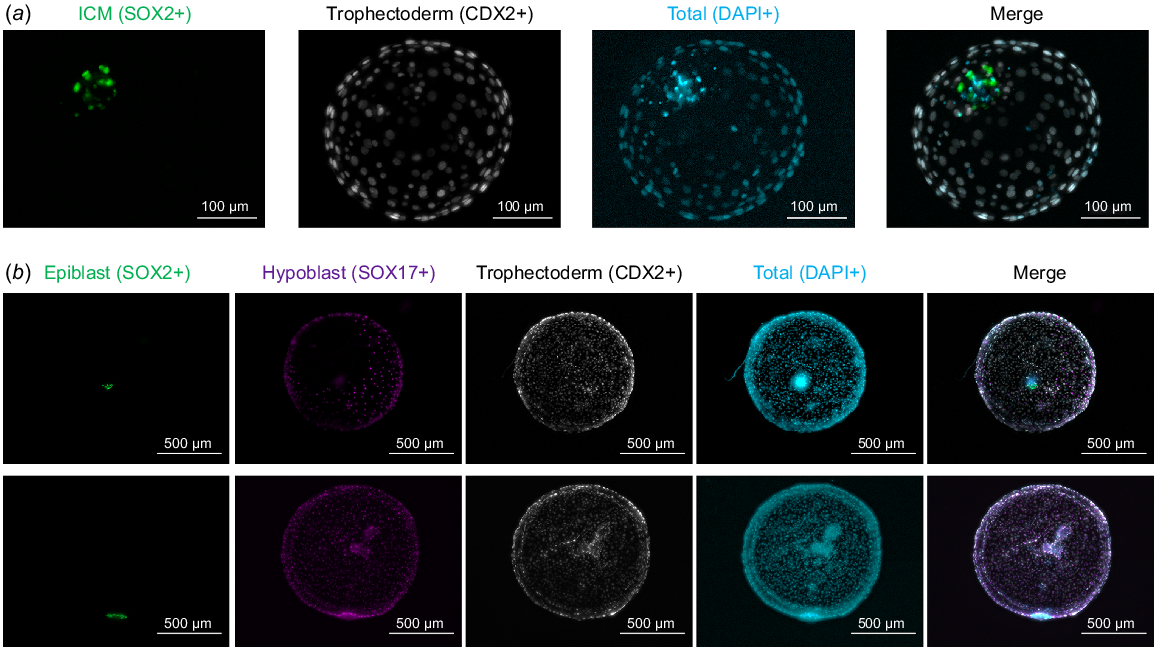
A dose-dependent effect of AA exposure during post-hatching in vitro culture (i.e. from Day 7 to Day 12) was evidenced in Day 12 survival rates (Table 4). Exposure to 100 μM AA was not compatible with post-hatching embryo development, contrasting with the lack of a negative effect observed upon AA supplementation at that concentration from Day 1 to Day 8. AA concentrations below 20 μM (5, 10 or 20 μM) did not reduce Day 7 survival rate to Day 12. However, Day 12 embryo diameter was gradually reduced in the presence of AA, being significantly diminished in 10 or 20 μM groups compared to the control (Table 5). Despite the reduction in Day 12 embryo size at 10 μM, lineage development in Day 12 embryos was comparable between 0, 5 and 10 μM groups (Table 6, Fig. 1b). Complete hypoblast migration was observed in about half of the embryos in all groups, epiblast survival ranged from ~65–80% and the development of an ED-like structure was observed in roughly half of the embryos analysed irrespective of the concentration of AA (0–10 μM).
Group | Replicates | Day 7 blastocysts | Day 12 blastocysts |
---|---|---|---|
Control | 11 | 106 | 85.8 ± 4.4% (91)a |
AA 5 μM | 6 | 109 | 84.4 ± 4.8% (92)a |
AA 10 μM | 6 | 74 | 89.2 ± 3.1% (66)a |
AA 20 μM | 3 | 43 | 72.1 ± 4.2% (31)a |
AA 100 μM | 2 | 19 | 0% (0)b |
Developmental rates are represented as mean ± s.e.m. (number). Different superscript letters indicate statistically significant differences based on ANOVA (P < 0.05).
Group | N | Ø (mm) |
---|---|---|
Control | 81 | 0.71 ± 0.05a |
AA 5 μM | 64 | 0.64 ± 0.04ab |
AA 10 μM | 66 | 0.51 ± 0.04bc |
AA 20 μM | 20 | 0.32 ± 0.02c |
Embryo diameter is represented as mean ± s.e.m. Different superscript letters indicate statistically significant differences based on ANOVA (P < 0.05).
Group | Hypoblast migration (%) | Epiblast survival (%) | ED-like structure (%) |
---|---|---|---|
Control | 18/40 (45.00)a | 30/40 (75.00)a | 22/40 (55.00)a |
AA 5 μM | 15/29 (51.72)a | 23/29 (79.31)a | 15/29 (51.72)a |
AA 10 μM | 22/35 (62.86)a | 23/35 (65.71)a | 17/35 (48.57)a |
Different superscript letters indicate statistically significant differences within each column based z-test (P < 0.05).
To determine the possible molecular changes triggered by AA exposure, the expression level of genes related to pregnancy recognition (IFNT2), prostaglandin synthesis (PTGS2, PTGES and PTGIS), prostaglandin signalling (PTGFR), lipid signalling and metabolism (PPARG and SCD), and Krebs cycle and anaerobic glycolysis (CS and LDHA) were determined by qPCR on Day 12 embryos from control, AA 5 μM and AA 10 μM groups (Fig. 2). PTGIS and PPARG were significantly upregulated in Day 12 embryos cultured without AA compared with those cultured at 5 μM AA. SCD and LDHA were significantly upregulated in Day 12 blastocysts cultured without AA compared with those cultured at 10 μM. No significant differences were noted for the other genes analysed.
Discussion
The addition of lipid compounds to improve in vitro culture conditions has been scarcely explored. Conventional pre-hatching culture systems – based on microdrop culture – are not suited for testing such compounds, as the overlying mineral oil captures the lipid compounds from the aqueous media. Being the precursor of PGs and all other prostanoids, AA could be required by the embryo to produce such signalling molecules at specific stages of development. At pre-hatching stages, bovine embryos developed normally in a relatively high concentration of AA (100 μM). The lack of a developmental effect at a concentration significantly higher than that previously used in cultures of bovine embryos (~164 nM) (Lim and Hansel 1996; Lim and Hansel 2000), ovine placental cells (~1.6 μM) (Weems et al. 2003) or mouse endometrial stromal cells (15 μM) (Zhao et al. 2012) strongly suggests that pre-hatching bovine embryos are largely irresponsive to AA. Nevertheless, we cannot rule out a biphasic effect of AA, which may mask a potential beneficial effect of AA at lower concentrations than 100 μM, which were not tested in this experiment as the main focus was on post-hatching development. Besides, the number of replicates and embryos analysed in the pre-hatching development experiment (Tables 2 and 3) was relatively low and maybe insufficient to identify a subtle developmental effect of AA supplementation at 100 μM on embryo development. The overtly unaltered pre-hatching development following AA supplementation at 100 μM may indicate a relatively lower ability of pre-hatching embryos to produce PGs, as no noticeable conceptus-induced changes in endometrium gene expression are observed before maternal recognition of pregnancy on Day 16 (Forde et al. 2011), and PTGS2 – the rate limiting enzyme for the production of prostanoids (Simmons et al. 2004) – is sharply upregulated on Day 16 bovine conceptus (Mamo et al. 2011).
In contrast to pre-hatching embryos, post-hatching embryos were highly sensitive to AA: the same concentration (100 μM) at which no developmental effects were observed during pre-hatching embryo culture completely abrogated embryo development in the post-hatching culture, and a gradual reduction of embryonic size at Day 12 of in vitro culture was observed at 5–20 μM AA. This was an unexpected outcome, as a beneficial effect, particularly on the proliferation of extra-embryonic membranes (i.e. embryo size) was intended, given the expression of PGs receptors in the trophectoderm (Dorniak et al. 2011). A plausible explanation for the negative effect of AA addition at these stages is that the ability to metabolise AA could arise around blastocyst hatching but it may be too incipient to properly catalyse the conversion of AA into signalling molecules. In vitro Day 12 embryos are developmentally equivalent to in vivo E10-11 embryos (Ramos-Ibeas et al. 2020), an earlier developmental stage than in vivo Day 12, when PGs production is well documented (Ulbrich et al. 2009). Further supporting this hypothesis, AA content in the embryo increases significantly from Day 7 to Day 14 (Menezo et al. 1982) and the ability of bovine embryos to metabolise AA changes substantially as conceptus elongation progresses (Lewis et al. 1982; Hwang et al. 1988). In particular, elongated conceptuses were found to metabolise AA into 13, 14-dihydro-15-keto-PGF2α, PGF2α and PGE2 at Days 16 and 19 of in vivo development, and PG production increased from Day 16 to Day 19 (Lewis et al. 1982). At earlier stages, in vivo embryos collected at Day 12 of the oestrous cycle metabolised AA primary to PGE2, whereas those collected one day later metabolised AA to both PGE2 and PGF2α, and embryos collected after Day 15 of the cycle metabolised AA to PGI in addition to PGE2 and PFG2α (Hwang et al. 1988). Further improvements in the post-hatching system, such as those obtained in sheep embryos upon the addition of cytokines (Ramos-Ibeas et al. 2022), would serve to test if AA is beneficial at later stages of development, currently inaccessible by in vitro systems.
AA supplementation exerted a transcriptional regulation in Day 12 in vitro–derived embryos. Lactate dehydrogenase A (LDHA) and Stearoyl-CoA desaturase (SCD) transcription decreased gradually upon AA exposure. LDHA is a rate limiting enzyme of anaerobic glycolysis whose transcript abundance correlates with the activity of that metabolic pathway (Bermejo-Alvarez et al. 2010b). LDHA expression increases as embryo development progresses from Day 9 to Day 15 bovine in vitro development (Ramos-Ibeas et al. 2020), and from pre- to post-hatching porcine in vivo embryos (Liu et al. 2021), which suggests that its downregulation in response to AA may reflect a developmental delay caused by exposure to AA. SCD catalyses a rate limiting step in the synthesis of unsaturated fatty acids and, as occurred for LDHA, its expression increases progressively from the ovoid to the filamentous conceptus stage in bovines (Ribeiro et al. 2016b) and, therefore, its downregulation in AA exposed embryos may be also consequence of a developmental delay.
PPARG and PTGIS were downregulated in the presence of AA, showing a statistically significant downregulation in the group exposed to 5 μM AA compared to the control. The downregulation of both PTGIS and PPARG are seemingly related, as PTGIS is the synthase of prostacyclin PGI2, and PGI2 is a known activator of PPARs (Desvergne and Wahli 1999). The downregulation of PTGIS and PPARG is consistent with a detrimental effect of AA addition to the post-hatching culture, as their mRNA levels increase along conceptus elongation (Cammas et al. 2006) and PGI2 may be required for post-hatching development, given that PGI2 supplementation during pre-hatching development has been reported to reduce apoptosis and enhance blastocyst expansion (Song et al. 2009). PPARG downregulation may be also associated with a developmental delay, as its transcription increases progressively from the ovoid to the filamentous conceptus stage (Ribeiro et al. 2016b).
In conclusion, pre-hatching embryos are mostly irresponsive to AA, whereas a negative effect of AA was observed at early post-hatching development, suggesting that AA addition is not required to mimic in vivo conditions during early post-hatching development. This developmental delay was associated with downregulation of metabolism-related enzymes, a PG synthase and a lipid signalling receptor.
Data availability
The data that support this study will be shared upon reasonable request to the corresponding author.
Declaration of funding
This work was supported by the projects StG-757886-ELONGAN from the European Research Council and AGL2017-84908-R, PID2020-117501RB-I00 and ECQ2018-005184-P from the Spanish Ministry of Science and Innovation to PBA, and PID2021-122153NA-I00 from the Spanish Ministry of Science and Innovation to PRI.
Acknowledgements
The authors want to acknowledge the slaughterhouse ‘Transformación Ganadera de Leganés SA’ for gently providing bovine ovaries to conduct the experiments.
References
Berg DK, van Leeuwen J, Beaumont S, Berg M, Pfeffer PL (2010) Embryo loss in cattle between Days 7 and 16 of pregnancy. Theriogenology 73(2), 250-260.
| Crossref | Google Scholar |
Bermejo-Alvarez P, Rizos D, Rath D, Lonergan P, Gutierrez-Adan A (2010a) Sex determines the expression level of one third of the actively expressed genes in bovine blastocysts. Proceedings of the National Academy of Sciences 107(8), 3394-3399.
| Crossref | Google Scholar |
Bermejo-Alvarez P, Lonergan P, Rizos D, Gutierrez-Adan A (2010b) Low oxygen tension during IVM improves bovine oocyte competence and enhances anaerobic glycolysis. Reproductive BioMedicine Online 20(3), 341-349.
| Crossref | Google Scholar |
Bermejo-Alvarez P, Rizos D, Lonergan P, Gutierrez-Adan A (2011) Transcriptional sexual dimorphism in elongating bovine embryos: implications for XCI and sex determination genes. Reproduction 141(6), 801-808.
| Crossref | Google Scholar |
Bermejo-Alvarez P, Roberts RM, Rosenfeld CS (2012) Effect of glucose concentration during in vitro culture of mouse embryos on development to blastocyst, success of embryo transfer, and litter sex ratio. Molecular Reproduction and Development 79, 329-336.
| Crossref | Google Scholar |
Cammas L, Reinaud P, Bordas N, Dubois O, Germain G, Charpigny G (2006) Developmental regulation of prostacyclin synthase and prostacyclin receptors in the ovine uterus and conceptus during the peri-implantation period. Reproduction 131(5), 917-927.
| Crossref | Google Scholar |
Desvergne B, Wahli W (1999) Peroxisome proliferator-activated receptors: nuclear control of metabolism. Endocrine Reviews 20(5), 649-688.
| Crossref | Google Scholar |
Dieleman SJ, Hendriksen PJM, Viuff D, Thomsen PD, Hyttel P, Knijn HM, Wrenzycki C, Kruip TAM, Niemann H, Gadella BM, Bevers MM, Vos PLAM (2002) Effects of in vivo prematuration and in vivo final maturation on developmental capacity and quality of pre-implantation embryos. Theriogenology 57(1), 5-20.
| Crossref | Google Scholar |
Diskin MG, Morris DG (2008) Embryonic and early foetal losses in cattle and other ruminants. Reproduction in Domestic Animals 43(S2), 260-267.
| Crossref | Google Scholar |
Dorniak P, Bazer FW, Spencer TE (2011) Prostaglandins regulate conceptus elongation and mediate effects of interferon tau on the ovine uterine endometrium. Biology of Reproduction 84(6), 1119-1127.
| Crossref | Google Scholar |
Dunne LD, Diskin MG, Sreenan JM (2000) Embryo and foetal loss in beef heifers between day 14 of gestation and full term. Animal Reproduction Science 58, 39-44.
| Crossref | Google Scholar |
Forde N, Carter F, Spencer TE, Bazer FW, Sandra O, Mansouri-Attia N, Okumu LA, McGettigan PA, Mehta JP, McBride R, O’Gaora P, Roche JF, Lonergan P (2011) Conceptus-induced changes in the endometrial transcriptome: how soon does the cow know she is pregnant? Biology of Reproduction 85(1), 144-156.
| Crossref | Google Scholar |
Holm P, Booth PJ, Schmidt MH, Greve T, Callesen H (1999) High bovine blastocyst development in a static in vitro production system using sofaa medium supplemented with sodium citrate and myo-inositol with or without serum-proteins. Theriogenology 52(4), 683-700.
| Crossref | Google Scholar |
Hwang DH, Pool SH, Rorie RW, Boudreau M, Godke RA (1988) Transitional changes in arachidonic acid metabolism by bovine embryos at different developmental stages. Prostaglandins 35(3), 387-402.
| Crossref | Google Scholar |
Lee J, McCracken JA, Stanley JA, Nithy TK, Banu SK, Arosh JA (2012) Intraluteal prostaglandin biosynthesis and signaling are selectively directed towards PGF2alpha during luteolysis but towards PGE2 during the establishment of pregnancy in sheep. Biology of Reproduction 87(4), 97.
| Crossref | Google Scholar |
Lewis GS, Thatcher WW, Bazer FW, Curl JS (1982) Metabolism of arachidonic acid in vitro by bovine blastocysts and endometrium. Biology of Reproduction 27(2), 431-439.
| Crossref | Google Scholar |
Lim JM, Hansel W (1996) Roles of growth factors in the development of bovine embryos fertilized in vitro and cultured singly in a defined medium. Reproduction, Fertility and Development 8(8), 1199-1205.
| Crossref | Google Scholar |
Lim JM, Hansel W (2000) Exogeneous substances affecting development of in vitro-derived bovine embryos before and after embryonic genome activation. Theriogenology 53(5), 1081-1091.
| Crossref | Google Scholar |
Liu T, Li J, Yu L, Sun H-X, Li J, Dong G, Hu Y, Li Y, Shen Y, Wu J, Gu Y (2021) Cross-species single-cell transcriptomic analysis reveals pre-gastrulation developmental differences among pigs, monkeys, and humans. Cell Discovery 7(1), 8.
| Crossref | Google Scholar |
Mamo S, Mehta JP, McGettigan P, Fair T, Spencer TE, Bazer FW, Lonergan P (2011) RNA sequencing reveals novel gene clusters in bovine conceptuses associated with maternal recognition of pregnancy and implantation. Biology of Reproduction 85(6), 1143-1151.
| Crossref | Google Scholar |
Menezo Y, Renard J-P, Delobel B, Pageaux J-F (1982) Kinetic study of fatty acid composition of day 7 to day 14 cow embryos. Biology of Reproduction 26(5), 787-790.
| Crossref | Google Scholar |
Perez-Gomez A, Gonzalez-Brusi L, Bermejo-Alvarez P, Ramos-Ibeas P (2021) Lineage differentiation markers as a proxy for embryo viability in farm ungulates. Frontiers in Veterinary Science 8, 680539.
| Crossref | Google Scholar |
Ramos-Ibeas P, Lamas-Toranzo I, Martinez-Moro A, de Frutos C, Quiroga AC, Zurita E, Bermejo-Alvarez P (2020) Embryonic disc formation following post-hatching bovine embryo development in vitro. Reproduction 160(4), 579-589.
| Crossref | Google Scholar |
Ramos-Ibeas P, Gonzalez-Brusi L, Used MT, Cocero MJ, Marigorta P, Alberio R, Bermejo-Alvarez P (2022) In vitro culture of ovine embryos up to early gastrulating stages. Development 149(6), dev199743.
| Crossref | Google Scholar |
Ramos-Ibeas P, Perez-Gomez A, Gonzalez-Brusi L, Quiroga AC, Bermejo-Alvarez P (2023) Pre-hatching exposure to N2B27 medium improves post-hatching development of bovine embryos in vitro. Theriogenology 205, 73-78.
| Crossref | Google Scholar |
Reynolds LP, Robertson DA, Ford SP (1983) Effects of intrauterine infusion of oestradiol-17β and prostaglandin E-2 on luteal function in non-pregnant heifers. Journal of Reproduction and Fertility 69(2), 703-709.
| Crossref | Google Scholar |
Ribeiro ES, Santos JEP, Thatcher WW (2016a) Role of lipids on elongation of the preimplantation conceptus in ruminants. Reproduction 152(4), R115-R126.
| Crossref | Google Scholar |
Ribeiro ES, Greco LF, Bisinotto RS, Lima FS, Thatcher WW, Santos JE (2016b) Biology of preimplantation conceptus at the onset of elongation in dairy cows. Biology of Reproduction 94(4), 97.
| Crossref | Google Scholar |
Rizos D, Ward F, Duffy P, Boland MP, Lonergan P (2002) Consequences of bovine oocyte maturation, fertilization or early embryo development in vitro versus in vivo: implications for blastocyst yield and blastocyst quality. Molecular Reproduction and Development 61(2), 234-248.
| Crossref | Google Scholar |
Sergeant S, Rahbar E, Chilton FH (2016) Gamma-linolenic acid, dihommo-gamma linolenic, eicosanoids and inflammatory processes. European Journal of Pharmacology 785, 77-86.
| Crossref | Google Scholar |
Simintiras CA, Sanchez JM, McDonald M, Lonergan P (2019) Progesterone alters the bovine uterine fluid lipidome during the period of elongation. Reproduction 157(4), 399-411.
| Crossref | Google Scholar |
Simmons DL, Botting RM, Hla T (2004) Cyclooxygenase isozymes: the biology of prostaglandin synthesis and inhibition. Pharmacological Reviews 56(3), 387-437.
| Crossref | Google Scholar |
Song B-S, Kim J-S, Kim C-H, Han Y-M, Lee D-S, Lee K-K, Koo D-B (2009) Prostacyclin stimulates embryonic development via regulation of the cAMP response element-binding protein–cyclo-oxygenase-2 signalling pathway in cattle. Reproduction, Fertility and Development 21(3), 400-407.
| Crossref | Google Scholar |
Ulbrich SE, Schulke K, Groebner AE, Reichenbach HD, Angioni C, Geisslinger G, Meyer HHD (2009) Quantitative characterization of prostaglandins in the uterus of early pregnant cattle. Reproduction 138(2), 371-382.
| Crossref | Google Scholar |
van de Leemput EE, Vos PLAM, Zeinstra EC, Severs MM, van der Weijden GC, Dieleman SJ (1999) Improved in vitro embryo development using in vivo matured oocytes from heifers superovulated with a controlled preovulatory lh surge. Theriogenology 52(2), 335-349.
| Crossref | Google Scholar |
Weems YS, Kim L, Humphreys V, Tsuda V, Weems CW (2003) Effect of luteinizing hormone (LH), pregnancy specific protein B (PSPB), or arachidonic acid (AA) on ovine endometrium of the estrous cycle or placental secretion of prostaglandins E2 (PGE2) and F2α (PGF2α) and progesterone in vitro. Prostaglandins & Other Lipid Mediators 71(1–2), 55-73.
| Crossref | Google Scholar |
Ying Q-L, Smith AG (2003) Defined conditions for neural commitment and differentiation. Methods in Enzymology 365, 327-341.
| Crossref | Google Scholar |
Zhao Z-A, Zhang Z-R, Xu X, Deng W-B, Li M, Leng J-Y, Liang X-H, Yang Z-M (2012) Arachidonic acid regulation of the cytosolic phospholipase A2α/cyclooxygenase-2 pathway in mouse endometrial stromal cells. Fertility and Sterility 97(5), 1199-1205.e9.
| Crossref | Google Scholar |