What can wildlife-detection dogs offer for managing small mammalian predators?
Alistair S. Glen
A
B
C
D
E
Abstract
Small mammalian predators can have low population densities, as well as cryptic and highly mobile behaviours, making these species challenging to manage. Monitoring tools such as camera traps, hair traps and footprint tracking devices can help detect cryptic species, but they require an animal to approach and, in most cases, interact with a device. They also have limited capacity to help capture animals. Wildlife-detection dogs can detect a wide range of species with a similar or higher efficiency than do other methods, partly because they are much less dependent on volitional decisions of target animals to interact with devices. By following scent trails, dogs can track down animals that actively avoid capture or detection. Dog-handler teams also have another advantage, namely, the handler can mount a rapid management response to capture or remove animals as soon as they are detected. We review ways in which dog-handler teams can contribute to active management of small mammalian predators by combining the dogs’ ability to detect animals with their handlers’ ability to mount a rapid response.
Keywords: conservation dog, cryptic species, detector dog, feral cat, introduced predators, invasive predators, mustelid, predator management.
Introduction
Small mammalian predators are often intensively managed either for their own conservation (e.g. Biggins et al. 1999; Sainsbury et al. 2019; Willcox 2020), to mitigate undesired impacts (e.g. Bryce et al. 2011; Russell et al. 2015), or both (e.g. Shields and Austin 2018). Low population densities and small body size, as well as cryptic and highly mobile behaviours, present challenges to managing many of these species. Individuals are often difficult to detect, and even more difficult to capture or remove. Monitoring tools such as camera traps, hair traps and footprint tracking devices can help detect cryptic species (e.g. Clayton et al. 2011; Pickerell et al. 2014; Kays et al. 2020), but they require an animal to approach and, in some cases, interact with a device. These tools provide information on where an animal has been at a specific time, but cannot follow the animal to its present location (e.g. so as to capture the animal).
Wildlife-detection dogs can detect a wide range of species (Grimm-Seyfarth et al. 2021) with similar or higher efficiency than do other methods (e.g. Long et al. 2007a; Glen et al. 2014, 2016; Bennett et al. 2020). This is partly because they do not depend on volitional decisions by target animals to interact with devices in particular ways. By following scent trails, dogs can track down animals that actively avoid capture or detection. Dog-handler teams also have other advantages; namely, they can facilitate rapid management responses allowing the handler to capture or remove animals as soon as they are detected, and they can help confirm success of eradication programs with greater certainty by increasing the probability of detecting any remaining pests (e.g. Russell et al. 2008; Shapira et al. 2011; McGregor et al. 2016; Kim et al. 2020).
We explore ways in which dog-handler teams can contribute to active management of small mammalian predators by combining the dogs’ ability to detect animals with their handlers’ ability to mount a rapid response. Unlike hunting dogs, wildlife-detection dogs usually have no direct interaction with target animals. Mustelids (Mustela spp.), rats (Rattus spp.) and brushtail possums (Trichosurus vulpecula) are of particular interest because these invasive predators are targeted for eradication in Aotearoa New Zealand (Russell et al. 2015), where they are a threat to endangered species such as kiwi (Apteryx spp.) (Basse et al. 1999). Mustelids are also the focus of intensive management (either to remove or conserve them) in many other parts of the world (e.g. Reindl-Thompson et al. 2006; Harrington et al. 2010). We also discuss applications for other small mammalian predators such as feral cats (Felis catus), mongooses (Herpestes auropunctatus), kit foxes (Vulpes macrotis) and quolls (Dasyurus spp.), which are intensively managed in their native and/or introduced range. We summarise where and how dog-handler teams have been used, and identify opportunities for wider application.
Methods
For the purposes of this review, we define small mammalian predators as mammals with an average body mass of ≤4 kg (the approximate size of a feral cat) whose diet includes live prey. This definition includes some species (e.g. brushtail possums) that are predominantly herbivorous, but can be significant predators of some vertebrate species (Brown et al. 1996).
We searched Google Scholar and the ISI Web of Science by using the following keywords: (conservation dog OR conservation detect* dog OR wildlife detect* dog OR scat detect* dog) AND (Mustel* OR Rattus OR Felis OR Trichosurus OR mongoose OR small mammal*). We scanned the resulting titles for studies describing the use of dogs to locate and/or capture small mammalian predators. We also searched the reference lists of these publications for additional articles.
Results
Our literature search yielded 97 publications (Supplementary Table S1). The search results were dominated by studies from North America (31%), New Zealand (30%) and Australia (10%), whereas a further 7% were global studies with no regional focus. A greater number of studies related to invasive predators (56%) than to native predators (36%), the remainder having no taxonomic focus. In 62% of studies, dogs were used only to detect predators, whereas 34% described the use of dog-handler teams to assist in detection, with a subsequent capture by darting, shooting, or trapping (Fig. 1).
Global distribution of studies that used dog-handler teams to detect small mammalian predators. Yellow shading represents detection of native species, dark blue shows detection of invasive species, and pale blue represents detection of invasive species plus intervention (e.g. capturing animals). The length of each bar indicates the number of studies. An additional five studies (not shown) did not focus on a particular species or geographic region.
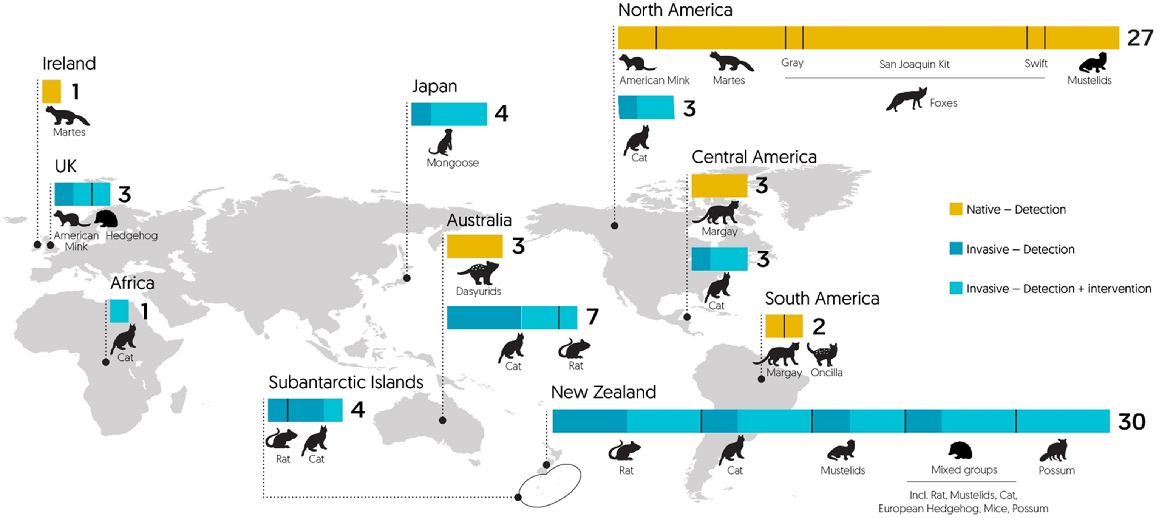
Case study 1: predator-free islands and sanctuaries in New Zealand
Dog-handler teams have played an important role in creating and maintaining many of New Zealand’s predator-free sanctuaries, which sustain native species unable to coexist with (introduced) mammalian predators. For example, possums were eradicated from Kapiti Island in 1986 (Cowan 1992; Sherley 1992; Brown and Sherley 2002). Kapiti Island is 1965 ha in area, lies 5 km off the western coast of New Zealand’s North Island, and supports native species that are rare or extinct on the mainland, including little spotted kiwi (Apteryx oweni).
After several years of trapping to reduce the possum population to low density, the eradication was undertaken by two trappers and three dog-handler teams. Of the 80 possums removed, 32 were found using dog-handler teams (Brown and Sherley 2002). Many of these animals were thought to have interacted with traps without being captured, and may subsequently have become trap-shy and avoided traps (Cowan 1992).
Each team consisted of a handler and one or two dogs, and searched areas of ~40 ha at a time (Sherley 1992). In general, the dog-handler teams worked in areas that had already been intensively trapped. Thus, they mainly targeted possums that had previously evaded capture. After tracking a possum to its location, the dogs either captured and killed the animal on the ground (a method that is no longer recommended for animal-welfare reasons) or chased it up a tree where it could be shot by the handler (Brown and Sherley 2002). Each block was searched independently by all three dog-handler teams; however, no possums were found in blocks that had previously been searched by another team. This suggests that a single search was enough to ensure a high probability of capturing any possums present (Brown and Sherley 2002).
The dog-handler teams on Kapiti Island also helped increase confidence in declaring eradication success. After all search blocks had been covered, they completed an additional sweep of all walking tracks. No possums were detected, which helped confirm absence (Brown and Sherley 2002). Dog-handler teams have also been used effectively in eradicating possums from other islands, including Rangitoto, Motutapu and Codfish Island/Whenua Hou (Brown and Sherley 2002).
Dog-handler teams also play a vital role in biosecurity and surveillance of pest-free areas. They help prevent incursions by intercepting invasive animals in vessels or cargo, and they help with detection and response to incursions (Russell et al. 2008; Gsell et al. 2010; Shapira et al. 2011; Bassett et al. 2016). For example, in 2018 a dog-handler team was used to confirm a rat (Rattus norvegicus) incursion on pest-free Motutapu Island, and to identify the area in which the rat was active. This information guided placement of traps, resulting in the rat’s timely capture and removal (DOC 2018). In May 2020, a stoat (Mustela erminea) incursion occurred on the same island, involving three individuals. Despite a network of over 600 traps, one of these stoats eluded capture until November 2021. It was eventually trapped after a detection dog identified an area where the animal had recently been active. A trap was set in an ‘artificial den’, using a combination of scent and sound lures, which captured the animal in less than a week (DOC 2021; A. J. Veale, pers. comm.). Similarly, a detection dog located a stoat den after a recent incursion into Shakespear Regional Park, a fenced sanctuary in the north of Auckland. The animal was captured by placing a trap at the den entrance (RNZ 2021).
Using a detection dog helped increase statistical power to confirm success of an incursion response on rat-free Great Mercury Island. This was partly because the dog-handler team achieved greater spatial coverage of the island than did static detection devices, and partly because dogs can detect individual rats that do not interact with other detection devices (Kim et al. 2020). Dog-handler teams also routinely inspect vessels, cargo and passengers travelling to pest-free islands in New Zealand to intercept invasive species, and therefore reduce the risk of reinvasion (Brown et al. 2015).
Dog-handler teams can also be useful in the planning phase of an eradication by helping gather information on the abundance, distribution, and movements of the target species. For example, preparations are currently underway to eradicate feral cats from Auckland Island in the New Zealand subantarctic region (Horn et al. 2022). During a research and monitoring trip in 2019, dog-handler teams located more cat scats than did human searchers, and also recorded nine cat detections not associated with scats. By matching scats to known individuals through analysis of faecal DNA, information was also obtained on the movements of individual cats (Glen et al. 2022). This provided useful information in addition to that gained from camera-trapping, as not all cats captured on camera could be individually identified (Glen et al. 2022).
Case study 2: mongoose eradication on Japanese islands
The island of Amami Oshima in the south of Japan contains many endangered species, a number of which are island endemics. The invasive small Indian mongoose (H. auropunctatus) was a major driver of declines in native wildlife populations (Watari et al. 2008), and has been the subject of intensive eradication efforts since 2003 (Barun et al. 2011; Fukasawa et al. 2013). The mongoose has now been eliminated from most of its former range on Amami Oshima (Abe 2013). The majority of animals were captured using a network of 30 000 kill-traps deployed throughout the island. However, as the mongoose population declined, annual trap catch fell from 946 animals in 2008 to a single animal in 2018. During the same period, 102 mongooses were tracked by dog-handler teams to their dens, where they were captured by the dog handlers (Abe 2013; Glen and Hoshino 2020; MoE 2021).
Since 2018, no mongoose has been captured in over four million trap-nights, and there have been no detections by camera-traps in over 260 000 camera-days (MoE 2021). Monitoring will continue by using traps, cameras and dog-handler teams so as to confirm successful eradication (MoE 2021). Dog-handler teams have also been used in an ongoing mongoose eradication effort on the island of Okinawa, where the mongoose is a threat to endemic species such as the Okinawa rail (Rallus okinawae) (Fukuhara et al. 2010).
Case study 3: conserving native predators
Dog-handler teams can be particularly useful for detecting endangered carnivores, which usually have low population densities, and are often cryptic (Ralls et al. 2010; Leigh and Dominick 2015; Jamieson et al. 2021). For example, in Australia, a dog trained to detect scats of the endangered spotted-tailed quoll (Dasyurus maculatus) was able to detect ≥83% of quoll scats placed in a variety of habitats, and in a range of weather conditions (Leigh and Dominick 2015). Similiarly, dog-handler teams found 100% of spotted-tailed quoll and northern quoll (D. hallucatus) scats placed in large experimental arenas (Jamieson et al. 2021). In subsequent field surveys, the same dogs found northern quoll scats in a single visit to a site where camera-trapping had failed to detect the species after 1898 camera-trap days (Jamieson et al. 2021).
Thomas et al. (2020) compared effectiveness of live traps, camera-traps, and a detection dog for detecting the endangered black-tailed antechinus (Antechinus arktos) in eastern Australia. The dog indicated presence of A. arktos at 31 sites. Subsequent camera-trapping confirmed the species’ presence at 100% of these. The dog also located A. arktos at a site where extensive sampling with live traps (>5000 trap nights) and camera-traps (120 camera-trap nights) had previously failed to detect it. Similarly, scat detection dogs in North America had an estimated 95% probability of detecting fishers (Martes pennanti) when present (Long et al. 2007b), and were more cost-effective than were camera-traps or hair snares (Long et al. 2007a).
Dogs can not only detect a high proportion of their targets, but can also work more quickly than do human searchers. Reindl-Thompson et al. (2006) found that dogs detected endangered black-footed ferrets (Mustela nigripes) almost 10 times more quickly than did human searchers, and could search more than 10 times the area per hour. Dogs were also significantly faster than human searchers in locating northern quoll scats (Jamieson et al. 2021).
In the USA, dog-handler teams have been used extensively in monitoring the endangered San Joaquin kit fox (Vulpes macrotis mutica). Smith (2006) reported that dogs were more efficient than were human searchers at finding scats of kit foxes; one trained dog found approximately four times more scats than did an experienced human searcher. Scats not only provided useful indices of relative abundance, but analysis of scat DNA yielded information important for population management, including sex ratio, relatedness, movement patterns, and behaviour (Smith et al. 2005, 2006; Smith 2006; Ralls et al. 2010). More recently, researchers have used similar techniques to determine sex and identity of individual kit foxes, and to investigate abundance, habitat use and genetic diversity (Wilbert et al. 2015). Similar approaches have also been used to investigate relatedness (Purcell et al. 2012), diet, abundance, and habitat use in fishers (Thompson et al. 2012, 2015).
A scat-detection dog was used to find samples in a study of diet, stress hormone concentrations and parasites of free-roaming cats (F. catus) and other felids in Central America (Mesa-Cruz et al. 2016). Scat-detection dogs have also been used to investigate the abundance of pine martens (Martes martes) in Ireland (Sheehy et al. 2014), and to obtain genetic samples from long-tailed weasels (Mustela frenata) in North America (Zielinski et al. 2020).
Case study 4: research and management of feral cats
Feral cats can be difficult to trap, and even more difficult to recapture. This can present problems for researchers attempting to estimate population density, or to fit and remove telemetry collars or other devices (Buckmaster 2012; McGregor et al. 2016). McGregor et al. (2016) used dog-handler teams to track feral cats so that they could be captured by hand net, or sedated using a dart gun. The dogs were trained to corner cats (e.g. up a tree) so that they were unable to flee, a method known as ‘bailing’. After detecting the scent trail of a cat, bailing was successful on 71% of attempts, and took between 30 s and 60 min. In terms of captures per person-hour, the use of dog-handler teams was six times more efficient, and caused fewer injuries, than did leg-hold trapping, which is the most commonly used live-capture method for feral cats (McGregor et al. 2016). Dog-handler teams have also been used to help capture feral cats for a GPS telemetry study in New Zealand (Recio et al. 2010), and to aid hunters in eradicating feral cats from islands (e.g. Fitzgerald and Veitch 1985).
The extent to which dogs can be used to hunt feral cats may differ among legal jurisdictions; for example, in different parts of Australia, dogs may be used to locate, point, flush, bail and/or retrieve cats (after shooting) within the limitations of local animal welfare legislation (Johnston and Algar 2020). Dogs are also useful for detecting the presence of feral cats in an area, and for finding scats (Johnston and Algar 2020). For example, the feral cat eradication on San Nicolas Island (USA) used dogs to guide placement of traps for feral cats, and to help hunters locate target animals (Hanson et al. 2010, 2015). Dog-handler teams were also used in combination with other detection techniques to confirm the eradication of feral cats on Dirk Hartog Island in Western Australia (Algar et al. 2020).
Case study 5: scat-detection dogs
Through genetic, chemical, or morphological analyses, scats can yield information on abundance, occupancy, sex ratio, diet, microbiome, disease, home range, habitat use, and physiological stress (Morgan et al. 2007; MacKay et al. 2008; Woollett et al. 2013; San Juan et al. 2021). Dogs trained to find scats can be particularly useful. For example, during the final stages of an eradication, or after an incursion into a pest-free area, scat DNA can be used to determine whether multiple scats come from the same individual. This could also be useful in conservation of rare species. When a species is recorded in an area for the first time, scat searches combined with genetic analysis could determine whether there is a population present, or just a transient individual. By searching for scats and other signs, dog-handler teams can also delineate an area in which traps or other devices should be deployed. However, scat-detection dogs also have limitations. For example, they cannot provide information on the age of a scat, although the handler can often judge this. When scats are found following an incursion into a pest-free area, standard practice is to remove them. If scats are found at the same location at a later date, they can be assumed to be fresh. Another limitation of scat-detection dogs is that they cannot indicate the current location of the target animal.
Discussion
Our literature search suggested that the use of dog-handler teams to detect small mammalian predators is most common in North America, Australia, and New Zealand (although there may be some publication bias in the English-language literature). There is scope for dog-handler teams to be used much more widely. One potential obstacle to wider uptake may be difficulty in finding dogs and handlers with appropriate skills and accreditation. To our knowledge, New Zealand is the only country to have a national certification standard for wildlife-detection dogs and their handlers. The Conservation Dogs Programme (https://www.doc.govt.nz/our-work/conservation-dog-programme/) is administered by the Department of Conservation (DOC), but is responsible for certifying dog-handler teams throughout New Zealand, regardless of whether the handler is employed by DOC. The certification process includes demonstrating the dog’s competency in locating target species or signs, as well as safety of non-target species, and the ability of the handler to read the dog’s behaviour. Although there is no national certification standard in Australia, the Australasian Conservation Dog Network (https://conservationdognetwork.com.au/) provides resources and support for dog handlers and wildlife managers (Bennett et al. 2022), and has published guidelines for evaluating the performance of dog-handler teams in terms of welfare, safety and effectiveness (ACDN 2022). Similar organisations also exist in North America (https://wd4c.org/), Europe (https://www.wildlifedetectiondogs.org) and the UK (https://www.ecologydetectiondogwg.org). We suggest that similar structures could help promote the use of dog-handler teams in other parts of the world. When contracting dog-handler teams, we suggest that membership of, or accreditation from, such organisations may be a useful indicator of high professional standards.
One of the advantages of using dog-handler teams for wildlife detection is that sampling can be non-invasive (e.g. see Long et al. (2008), and chapters therein). However, the potential for dog-handler teams to contribute to management intervention, rather than detection alone, is under-utilised. Depending on the way they are used, wildlife-detection dogs can be non-invasive (e.g. when dogs locate scat or other sign), minimally invasive (e.g. when dogs indicate areas of animal activity to guide trap placement), or invasive (e.g. pursuing animals to aid capture by humans). The live-capture methods used by McGregor et al. (2016) for feral cats could be used more widely, and potentially adapted for other species. For example, the possum eradication on Kapiti Island used a similar technique of bailing and shooting, but with firearms rather than a tranquiliser gun (Brown and Sherley 2002). For research applications, tranquiliser darts could readily be used to live-capture possums after bailing by dogs. This may be particularly useful where researchers are interested in the behaviours of atypical animals that avoid capture by other methods (Garvey et al. 2020). For example, it has been suggested that some individual possums may be entirely arboreal, and therefore unlikely to encounter traps or baits at ground level. However, scats from such animals can be located on the ground (Morgan et al. 2007).
There is also scope for the use of dog-handler teams to be combined with other modern management techniques. For example, the use of dog-handler teams in combination with scat analysis is a powerful research tool that could be applied much more widely (Woollett et al. 2013).
Future research
Priorities for future research can be categorised into optimising detection of target species, and facilitating a management response (e.g. setting traps) when a target is detected. The time and/or effort required to locate target animals can be minimised using established principles of search theory (reviewed by Glen and Veltman 2018). To optimise the search path followed by a dog-handler team, estimates of effective sweep width are required. This parameter describes the distance on either side of a search path that is effectively covered in a single pass (Koopman 1980). Searchers vary in their abilities, and some search targets are more conspicuous than others; therefore, effective sweep width will vary. Experiments should be conducted to estimate effective sweep width for a range of targets, including live or dead animals, scats, and scent trails left by animals. Detectability is also likely to vary over time; for example, effective sweep width may be influenced by weather conditions, and may be lower for old scats and scent trails than for fresh ones (Glen et al. 2018; Baker et al. 2021).
Another potentially fruitful area of research is the trade-off between target specificity and generalisation. For example, is it more efficient to train dogs to indicate only scats of a particular species, or to find scats from a range of target animals, and use faecal DNA to determine the species? The answer to this question will depend on the range of species present, the degree of similarity among them, and the research or management aims being addressed. An additional consideration is that faecal DNA can usually be extracted only from scats that are fresh.
Parallel research on movement and scent-laying behaviour of target small mammals may find ways in which dog-handler teams can be used even more efficiently. The two commonest rat species in New Zealand, Norway rats (R. norvegicus) and ship rats (R. rattus), are both primarily nocturnal and lay scent prodigiously, i.e. up to 250 urine marks per hour (Mallick 1992). Reinvader rats in rat-free or rat-sparse settings move vastly longer distances than usual home-range movements (Russell et al. 2005; Innes et al. 2011; Nathan et al. 2020; J. Carpenter, unpubl. data). Verifying the size and pattern of these movements with radio transmitters may show ways in which dog-handler teams can be used with greater efficiency, such as searching in long straight lines with a ‘scent-trail intercept’ strategy.
Once a target individual has been located, effective and humane methods to capture or kill the animal would be useful for many researchers and managers. Theobald and Coad (2002) conducted trials using dog-handler teams to detect maternal dens of stoats, which were then fumigated using magnesium phosphide. Although dogs were effective at locating dens, fumigation was unsuccessful on most occasions, mainly because not all entrances were found and blocked (Theobald and Coad 2002). Owing to efficacy and welfare concerns, magnesium phosphide is no longer used for stoat control in New Zealand. We suggest future trials using carbon monoxide to fumigate stoat dens located by dog-handler teams. Carbon monoxide fumigation is effective for fox dens (Saunders et al. 2010) and rabbit warrens (Gigliotti et al. 2009), and may provide a humane and effective way to remove adult female stoats and their offspring.
By indicating areas of high activity for invasive species, dog-handler teams can also assist with more targeted use of other toxins (Woollett et al. 2013). For example, rat carcasses containing the toxin 1080 (sodium fluoroacetate) have recently been used to target stoats detected by camera-traps (Nichols et al. 2022). The use of dog-handler teams instead of camera-traps could potentially make this method more time- and cost-efficient, and could also help with targeted placement of toxic carcasses (e.g. near den entrances).
Although detection dogs have been used to detect disease in some wildlife species (Alasaad et al. 2012; Cristescu et al. 2019), we are not aware of any examples of such methods being used for small mammalian predators. Population declines of endangered black-footed ferrets and San Joaquin kit foxes may be driven in part by zoonotic diseases (Williams et al. 1988, 1994; Cypher et al. 2017; Schuler et al. 2020). Invasive species such as cats, possums and ferrets also carry zoonoses, which can threaten native species, livestock, and humans (Cowan and Glen 2021; Garvey and Byrom 2021; Roberts et al. 2021). Recent research reviewed by Jendrny et al. (2021) shows that dogs can detect a range of infectious and non-infectious diseases quickly, cheaply and accurately. Further research should explore the use of detection dogs in managing wildlife disease.
Increased use of dog-handler teams has potential to improve the efficiency of a range of conservation activities, including control of invasive species, monitoring native species, and rapid detection of disease. Further research, as outlined here, could unlock even more potential.
Declaration of funding
This work was jointly funded by the MBIE Kiwi Rescue and Eradication Science Endeavour programmes.
Acknowledgements
We thank A. J. Veale for providing valuable information, N. Faville for preparing the graphic art, and D. W. and G. D. Glen for sharing their expertise in canine behaviour. C. J. Veltman, C. N. Niebuhr and two anonymous referees provided valuable insights and comments on an earlier draft.
References
ACDN (2022) Conservation detection dog team evaluation guidelines. Australasian Conservation Dog Network. Available at https://conservationdognetwork.com.au/wp-content/uploads/2022/12/ACDN-CDD-Team-Evaluation-Guidelines-2022.pdf
Alasaad S, Permunian R, Gakuya F, Mutinda M, Soriguer RC, Rossi L (2012) Sarcoptic-mange detector dogs used to identify infected animals during outbreaks in wildlife. BMC Veterinary Research 8, 110.
| Crossref | Google Scholar | PubMed |
Algar D, Johnston M, Tiller C, Onus M, Fletcher J, Desmond G, Hamilton N, Speldewinde P (2020) Feral cat eradication on Dirk Hartog Island, Western Australia. Biological Invasions 22, 1037-1054.
| Crossref | Google Scholar |
Baker GB, Candy S, Robinson S, Friend JA, Holdsworth M, Jensz K, Page M, Algar D (2021) Effectiveness of dogs for detecting feral cat scats in wheatbelt reserves of Western Australia. Wildlife Research 48, 690-700.
| Crossref | Google Scholar |
Basse B, McLennan JA, Wake GC (1999) Analysis of the impact of stoats, Mustela erminea, on northern brown kiwi, Apteryx mantelli, in New Zealand. Wildlife Research 26, 227-237.
| Crossref | Google Scholar |
Bassett IE, Cook J, Buchanan F, Russell JC (2016) Treasure Islands: biosecurity in the Hauraki Gulf marine park. New Zealand Journal of Ecology 40, 250-266.
| Crossref | Google Scholar |
Bennett EM, Hauser CE, Moore JL (2020) Evaluating conservation dogs in the search for rare species. Conservation Biology 34, 314-325.
| Crossref | Google Scholar | PubMed |
Bennett E, Jamieson LT, Florent SN, Gill N, Hauser C, Cristescu R (2022) Detection dogs provide a powerful method for conservation surveys. Austral Ecology 47, 894-901.
| Crossref | Google Scholar |
Biggins DE, Vargas A, Godbey JL, Anderson SH (1999) Influence of prerelease experience on reintroduced black-footed ferrets (Mustela nigripes). Biological Conservation 89, 121-129.
| Crossref | Google Scholar |
Brown KP, Moller H, Innes J (1996) Sign left by brushtail possums after feeding on bird eggs and chicks. New Zealand Journal of Ecology 20, 277-284.
| Google Scholar |
Bryce R, Oliver MK, Davies L, Gray H, Urquhart J, Lambin X (2011) Turning back the tide of American mink invasion at an unprecedented scale through community participation and adaptive management. Biological Conservation 144, 575-583.
| Crossref | Google Scholar |
Clayton RI, Anderson D, Byrom A, Edge K-A, McMurtrie PM, Veale A, Torr N (2011) Using genetic analysis and trapping data to model the probability of persistence of feral stoats (Mustela erminea) on Resolution Island, New Zealand. In ‘Island invasives: eradication and management’. (Eds CR Veitch, MN Clout, DR Towns) pp. 413–417. (IUCN: Gland, Switzerland and Auckland, New Zealand)
Cowan PE (1992) The eradication of introduced Australian brushtail possums, Trichosurus vulpecula, from Kapiti Island, a New Zealand nature reserve. Biological Conservation 61, 217-226.
| Crossref | Google Scholar |
Cristescu RH, Miller RL, Schultz AJ, Hulse L, Jaccoud D, Johnston S, Hanger J, Booth R, Frère CH (2019) Developing noninvasive methodologies to assess koala population health through detecting Chlamydia from scats. Molecular Ecology Resources 19, 957-969.
| Crossref | Google Scholar | PubMed |
Cypher BL, Rudd JL, Westall TL, Woods LW, Stephenson N, Foley JE, Richardson D, Clifford DL (2017) Sarcoptic mange in endangered kit foxes (Vulpes macrotis mutica): case histories, diagnoses, and implications for conservation. Journal of Wildlife Diseases 53, 46-53.
| Crossref | Google Scholar | PubMed |
DOC (2018) Rat caught on pest-free Motutapu Island. Available at https://www.doc.govt.nz/news/media-releases/2018/rat-caught-on-pest-free-motutapu-island/ [Accessed 12 March 2021]
DOC (2021) Breakthrough in Motutapu stoat hunt. Available at https://www.doc.govt.nz/news/media-releases/2021-media-releases/breakthrough-in-motutapu-stoat-hunt/ [Accessed 9 November 2021]
Fitzgerald BM, Veitch CR (1985) The cats of Herekopare Island, New Zealand; their history, ecology and affects on birdlife. New Zealand Journal of Zoology 12, 319-330.
| Crossref | Google Scholar |
Fukasawa K, Hashimoto T, Tatara M, Abe S (2013) Reconstruction and prediction of invasive mongoose population dynamics from history of introduction and management: a Bayesian state-space modelling approach. Journal of Applied Ecology 50, 469-478.
| Crossref | Google Scholar |
Fukuhara R, Yamaguchi T, Ukuta H, Roy S, Tanaka J, Ogura G (2010) Development and introduction of detection dogs in surveying for scats of small Indian mongoose as invasive alien species. Journal of Veterinary Behavior 5, 101-111.
| Crossref | Google Scholar |
Garvey PM, Banks PB, Suraci JP, Bodey TW, Glen AS, Jones CJ, McArthur C, Norbury GL, Price CJ, Russell JC, Sih A (2020) Leveraging motivations, personality, and sensory cues for vertebrate pest management. Trends in Ecology & Evolution 35, 990-1000.
| Crossref | Google Scholar | PubMed |
Gigliotti F, Marks CA, Busana F (2009) Performance and humaneness of chloropicrin, phosphine and carbon monoxide as rabbit-warren fumigants. Wildlife Research 36, 333-341.
| Crossref | Google Scholar |
Glen AS, Hoshino K (2020) Social and logistical challenges in managing invasive predators: insights from islands in Japan and New Zealand. Pacific Conservation Biology 26, 344-352.
| Crossref | Google Scholar |
Glen AS, Veltman CJ (2018) Search strategies for conservation detection dogs. Wildlife Biology 2018, 1-9.
| Crossref | Google Scholar |
Glen AS, Warburton B, Cruz J, Coleman M (2014) Comparison of camera traps and kill traps for detecting mammalian predators: a field trial. New Zealand Journal of Zoology 41, 155-160.
| Crossref | Google Scholar |
Glen AS, Anderson D, Veltman CJ, Garvey PM, Nichols M (2016) Wildlife detector dogs and camera traps: a comparison of techniques for detecting feral cats. New Zealand Journal of Zoology 43, 127-137.
| Crossref | Google Scholar |
Glen AS, Russell JC, Veltman CJ, Fewster RM (2018) I smell a rat! Estimating effective sweep width for searches using wildlife-detector dogs. Wildlife Research 45, 500-504.
| Crossref | Google Scholar |
Glen AS, Sagar RL, Brav-Cubitt T, Jacques PM (2022) Monitoring and detection of feral cats on Auckland Island. New Zealand Journal of Ecology 46, 3494.
| Google Scholar |
Grimm-Seyfarth A, Harms W, Berger A (2021) Detection dogs in nature conservation: a database on their world-wide deployment with a review on breeds used and their performance compared to other methods. Methods in Ecology and Evolution 12, 568-579.
| Crossref | Google Scholar |
Gsell A, Innes J, de Monchy P, Brunton D (2010) The success of using trained dogs to locate sparse rodents in pest-free sanctuaries. Wildlife Research 37, 39-46.
| Crossref | Google Scholar |
Hanson CC, Bonham JE, Campbell KJ, Keitt BS, Little AE, Smith G (2010) The removal of feral cats from San Nicolas Island: methodology. In ‘Proceedings of the 24th vertebrate pest conference. Vol. 24’. (Eds RM Timm, KA Fagerstone) pp. 72–78. (University of California: Davis, CA, USA) doi:10.5070/V424110435
Hanson CC, Jolley WJ, Smith G, Garcelon DK, Keitt BS, Little AE, Campbell KJ (2015) Feral cat eradication in the presence of endemic San Nicolas Island foxes. Biological Invasions 17, 977-986.
| Crossref | Google Scholar |
Harrington LA, Harrington AL, Hughes J, Stirling D, Macdonald DW (2010) The accuracy of scat identification in distribution surveys: American mink, Neovison vison, in the northern highlands of Scotland. European Journal of Wildlife Research 56, 377-384.
| Crossref | Google Scholar |
Horn SR, Sagar RL, Frank VK, Cox FX, Jacques PM, Ware J, Hanley-Nickolls R, Leask EP, MacDonald NL, Kirby Crowe MS, Le Lievre ME, Broome KG (2022) The next frontier: assessing the feasibility of eradicating mammalian pests from Auckland Island. New Zealand Journal of Ecology 46, 3500.
| Crossref | Google Scholar |
Innes J, Watts C, Fitzgerald N, Thornburrow D, Burns B, MacKay J, Speedy C (2011) Behaviour of invader ship rats experimentally released behind a pest-proof fence, Maungatautari, New Zealand. In ‘Island invasives: eradication and management’. (Eds CR Veitch, CM Clout, DR Towns) pp. 437−440. (IUCN: Gland, Switzerland and Auckland, New Zealand)
Jamieson LTJ, Hancock AL, Baxter GS, Murray PJ (2021) How quoll-ified are northern and spotted-tailed quoll detection dogs? Wildlife Research 48, 376-384.
| Crossref | Google Scholar |
Jendrny P, Twele F, Meller S, Osterhaus ADME, Schalke E, Volk HA (2021) Canine olfactory detection and its relevance to medical detection. BMC Infectious Diseases 21, 838.
| Crossref | Google Scholar | PubMed |
Kays R, Arbogast BS, Baker-Whatton M, Beirne C, Boone HM, Bowler M, Burneo SF, Cove MV, Ding P, Espinosa S, Gonçalves ALS, Hansen CP, Jansen PA, Kolowski JM, Knowles TW, Lima MGM, Millspaugh J, McShea WJ, Pacifici K, Parsons AW, Pease BS, Rovero F, Santos F, Schuttler SG, Sheil D, Si X, Snider M, Spironello WR (2020) An empirical evaluation of camera trap study design: how many, how long and when? Methods in Ecology and Evolution 11, 700-713.
| Crossref | Google Scholar |
Kim JHK, Corson P, Mulgan N, Russell JC (2020) Rapid eradication assessment (REA): a tool for pest absence confirmation. Wildlife Research 47, 128-136.
| Crossref | Google Scholar |
Leigh KA, Dominick M (2015) An assessment of the effects of habitat structure on the scat finding performance of a wildlife detection dog. Methods in Ecology and Evolution 6, 745-752.
| Crossref | Google Scholar |
Long RA, Donovan TM, MacKay P, Zielinski WJ, Buzas JS (2007a) Comparing scat detection dogs, cameras, and hair snares for surveying carnivores. Journal of Wildlife Management 71, 2018-2025.
| Crossref | Google Scholar |
Long RA, Donovan TM, MacKay P, Zielinski WJ, Buzas JS (2007b) Effectiveness of scat detection dogs for detecting forest carnivores. Journal of Wildlife Management 71, 2007-2017.
| Crossref | Google Scholar |
Mallick SA (1992) Urine-marking in three species of Rattus. Wildlife Research 19, 89-93.
| Crossref | Google Scholar |
McGregor HW, Hampton JO, Lisle D, Legge S (2016) Live-capture of feral cats using tracking dogs and darting, with comparisons to leg-hold trapping. Wildlife Research 43, 313-322.
| Crossref | Google Scholar |
Mesa-Cruz JB, Brown JL, Waits LP, Kelly MJ (2016) Non-invasive genetic sampling reveals diet shifts, but little difference in endoparasite richness and faecal glucocorticoids, in Belizean felids inside and outside protected areas. Journal of Tropical Ecology 32, 226-239.
| Crossref | Google Scholar |
Nathan H, Agnew T, Mulgan N (2020) Movement behaviour of a translocated female ship rat and her offspring in a low rat density New Zealand forest. New Zealand Journal of Ecology 44, 3402.
| Crossref | Google Scholar |
Nichols M, Dent J, Edwards A (2022) Toxin-laced rat carcass baits for stoat elimination. New Zealand Journal of Ecology 46, 3453.
| Crossref | Google Scholar |
Pickerell GA, O’Donnell CFJ, Wilson DJ, Seddon PJ (2014) How can we detect introduced mammalian predators in non-forest habitats? A comparison of techniques. New Zealand Journal of Ecology 38, 86-102.
| Google Scholar |
Purcell KL, Thompson CM, Garner JD, Green RE (2012) The Kings River fisher project: links between fisher population viability and habitat at multiple scales. In ‘Biology and conservation of martens, sables, and fishers: a new synthesis’. (Eds KB Aubry, WJ Zielinski, MG Raphael, G Proulx, SW Buskirk) p. 13. (Cornell University Press: Ithaca, NY, USA)
Ralls K, Sharma S, Smith DA, Bremner-Harrison S, Cypher BL, Maldonado JE (2010) Changes in kit fox defecation patterns during the reproductive season: implications for noninvasive surveys. The Journal of Wildlife Management 74, 1457-1462.
| Crossref | Google Scholar |
Recio MR, Mathieu R, Maloney R, Seddon PJ (2010) First results of feral cats (Felis catus) monitored with GPS collars in New Zealand. New Zealand Journal of Ecology 34, 288-296.
| Google Scholar |
Reindl-Thompson SA, Shivik JA, Whitelaw A, Hurt A, Higgins KF (2006) Efficacy of scent dogs in detecting black-footed ferrets at a reintroduction site in South Dakota. Wildlife Society Bulletin 34, 1435-1439.
| Crossref | Google Scholar |
RNZ (2021) Rangers get creative to catch elusive stoats. Available at https://www.rnz.co.nz/news/national/445437/rangers-get-creative-to-catch-elusive-stoats [Accessed 2 November 2021]
Roberts JO, Jones HFE, Roe WD (2021) The effects of Toxoplasma gondii on New Zealand wildlife: implications for conservation and management. Pacific Conservation Biology 27, 208-220.
| Crossref | Google Scholar |
Russell JC, Towns DR, Anderson SH, Clout MN (2005) Intercepting the first rat ashore. Nature 437, 1107.
| Crossref | Google Scholar | PubMed |
Russell JC, Beaven BM, MacKay JWB, Towns DR, Clout MN (2008) Testing island biosecurity systems for invasive rats. Wildlife Research 35, 215-221.
| Crossref | Google Scholar |
Russell JC, Innes JG, Brown PH, Byrom AE (2015) Predator-free New Zealand: conservation country. BioScience 65, 520-525.
| Crossref | Google Scholar | PubMed |
Sainsbury KA, Shore RF, Schofield H, Croose E, Campbell RD, Mcdonald RA (2019) Recent history, current status, conservation and management of native mammalian carnivore species in Great Britain. Mammal Review 49, 171-188.
| Crossref | Google Scholar |
San Juan PA, Castro I, Dhami MK (2021) Captivity reduces diversity and shifts composition of the Brown Kiwi microbiome. Animal Microbiome 3, 48.
| Crossref | Google Scholar | PubMed |
Saunders GR, Gentle MN, Dickman CR (2010) The impacts and management of foxes Vulpes vulpes in Australia. Mammal Review 40, 181-211.
| Crossref | Google Scholar |
Schuler K, Claymore M, Schnitzler H, Dubovi E, Rocke T, Perry MJ, Bowman D, Abbott RC (2020) Sentinel coyote pathogen survey to assess declining black-footed ferret (Mustela nigripes) population in south dakota, USA. Journal of Wildlife Diseases 57, 264-272.
| Crossref | Google Scholar |
Shapira I, Buchanan F, Brunton DH (2011) Detection of caged and free-ranging Norway rats Rattus norvegicus by a rodent sniffing dog on Browns Island, Auckland, New Zealand. Conservation Evidence 8, 38-42.
| Google Scholar |
Sheehy E, O’Meara DB, O’Reilly C, Smart A, Lawton C (2014) A non-invasive approach to determining pine marten abundance and predation. European Journal of Wildlife Research 60, 223-236.
| Crossref | Google Scholar |
Smith DA, Ralls K, Cypher BL, Maldonado JE (2005) Assessment of scat-detection dog surveys to determine kit fox distribution. Wildlife Society Bulletin 33, 897-904.
| Crossref | Google Scholar |
Smith DA, Ralls K, Cypher BL, Clark HO, Jr, Kelly PA, Williams DF, Maldonado JE (2006) Relative abundance of endangered San Joaquin kit foxes (Vulpes macrotis mutica) based on scat–detection dog surveys. The Southwestern Naturalist 51, 210-219.
| Crossref | Google Scholar |
Thomas ML, Baker L, Beattie JR, Baker AM (2020) Determining the efficacy of camera traps, live capture traps, and detection dogs for locating cryptic small mammal species. Ecology and Evolution 10, 1054-1068.
| Crossref | Google Scholar | PubMed |
Thompson CM, Purcell KL, Green RE, Garner JD (2012) Use of scat-detector dogs to survey fishers in the Sierra National Forest, California. In ‘Biology and conservation of martens, sables, and fishers: a new synthesis’. (Eds KB Aubry, WJ Zielinski, MG Raphael, G Proulx, SW Buskirk) p. 16. (Cornell University Press: Ithaca, NY, USA)
Thompson CM, Purcell K, Green R, Sweitzer R (2015) Role of oaks in fisher habitat quality in the Sierra Nevada mountains at multiple spatial scales. In ‘Proceedings of the seventh California oak symposium: managing oak woodlands in a dynamic world’. (Eds RB Standiford, KL Purcell) pp. 83–95. (Department of Agriculture, Forest Service: Berkeley, CA, USA)
Watari Y, Takatsuki S, Miyashita T (2008) Effects of exotic mongoose (Herpestes javanicus) on the native fauna of Amami-Oshima Island, southern Japan, estimated by distribution patterns along the historical gradient of mongoose invasion. Biological Invasions 10, 7-17.
| Crossref | Google Scholar |
Wilbert TR, Woollett DA, Whitelaw A, Dart J, Hoyt JR, Galen S, Ralls K, Meade DE, Maldonado JE (2015) Non-invasive baseline genetic monitoring of the endangered San Joaquin kit fox on a photovoltaic solar facility. Endangered Species Research 27, 31-41.
| Crossref | Google Scholar |
Willcox D (2020) Conservation status, ex situ priorities and emerging threats to small carnivores. International Zoo Yearbook 54, 19-34.
| Crossref | Google Scholar |
Williams ES, Thome ET, Appel MJG, Belitsky DW (1988) Canine distemper in black-footed ferrets (Mustela nigripes) from Wyoming. Journal of Wildlife Diseases 24, 385-398.
| Crossref | Google Scholar | PubMed |
Williams ES, Mills K, Kwiatkowski DR, Thome ET, Boerger-Fields A (1994) Plague in a black-footed ferret (Mustela nigripes). Journal of Wildlife Diseases 30, 581-585.
| Crossref | Google Scholar | PubMed |
Zielinski WJ, Linnell MA, Schwartz MK, Pilgrim K (2020) Exploiting the winter trophic relationship between weasels (Mustela spp.) and their microtine prey as a survey method for weasels in meadow ecosystems. Northwest Science 93, 185-192.
| Crossref | Google Scholar |