Survival analysis of germination data in response to temperature for Ornithopus species and other temperate pasture legumes
D. R. Kidd





A School of Agriculture and Environment and Institute of Agriculture, The University of Western Australia, 35 Stirling Highway, Perth, WA 6009, Australia.
B Department of Plant Physiology, University of Kaiserslautern, Erwin-Schrödinger-Street, Kaiserslautern 67653, Germany.
C College of Grassland Science, Gansu Agricultural University, Lanzhou, Gansu 730070, China.
D CSIRO Agriculture and Food, GPO Box 1700, Canberra, ACT 2601, Australia.
Functional Plant Biology 50(10) 792-807 https://doi.org/10.1071/FP23095
Submitted: 24 April 2023 Accepted: 1 August 2023 Published: 22 August 2023
Abstract
Cool temperatures can limit productivity of temperate grazing systems as poor pasture growth rates in winter create feed shortages for livestock. Ornithopus spp. (serradella) are broadly adapted annual pasture legumes that produce high-quality forage in soil types considered marginal for other temperate legume species. However, serradella establishment is perceived to be difficult in cool-season environments. We used survival analysis to compare germination rate and seedling emergence for two serradella species (yellow serradella and French serradella) against three reference species (Medicago sativa, M. polymorpha and Trifolium subterraneum) in four temperature treatments (10/5, 15/10, 20/15 and 25/20°C; max/min). We also compared shoot relative growth rate and photosynthetic rate at 15/10°C (cool) and 23/18°C (warm). Cool temperatures (10/5, 15/10°C) did not slow germination rates for serradella relative to the reference species, but warm temperatures (20/15, 25/20°C) delayed emergence and reduced post-emergent shoot growth rates. Once established, Ornithopus spp. had similar mean photosynthetic rates and stomatal conductance at cool temperatures to the reference species. We conclude that, contrary to common perception, cool temperatures did not adversely influence germination, emergence, or early growth of Ornithopus spp. relative to the reference species.
Keywords: abiotic stress, alfalfa, cold temperatures, emergence, forage, legumes, pasture, photosynthesis, serradella.
Introduction
Cool temperatures can limit the productive potential of temperate pasture legumes (Sánchez-Díaz et al. 2000; Baker et al. 2014), particularly during pasture establishment. Slow seedling growth in winter can prolong the summer–autumn feed shortage experienced by livestock producers in Mediterranean environments of southern Australia (Baker et al. 2014) and south-eastern United States (Hoveland and Elkins 1965).
In years when the onset of seasonal rainfall (the ‘break of season’) is early, these self-regenerating pastures, which largely consist of annual clover (Trifolium) and medic (Medicago) species, experience a relatively warm germination and establishment period, favouring high rates of germination and seedling growth; these have long been referred to as ‘clover years’ (Young et al. 1970). However, if the break of season is late, the germination and emergence of temperate legumes can be slow as a result of sub-optimal temperatures (i.e. <10°C) (Butler et al. 2014). Average daily air temperatures below 8°C generally result in minimal pasture growth (Clements et al. 2000) because a decline in photosynthesis and nutrient assimilation reduces the relative growth rate (RGR) (Atwell 1999).
RGR for temperate pasture legumes and grasses across southern Australia are lowest during winter (Saul and Sargeant 2013). This, combined with a late break to the season, can result in a lengthy period before adequate quantities of forage are available for livestock, with plants becoming increasingly susceptible to root pathogens and weed competition (Dear and Ewing 2008; Simpson et al. 2011). However, variation exists within and between species for the degree to which germination is inhibited by low temperatures (Hoveland and Elkins 1965; Young et al. 1970; Moot et al. 2000; Lonati et al. 2009; Nori et al. 2014), suggesting there is potential to select for cool temperature tolerance.
Over several decades, numerous novel, annual pasture legumes have been trialled with the aim to diversify the legume base of Australian pastures (Nichols et al. 2007). Some, such as balansa clover (Trifolium michelianum), persian clover (Trifolium resupinatum) and arrowleaf clover (Trifolium vesiculosum), have shown promise in niche environments where subterranean clover (Trifolium subterraneum) (the most widely grown annual legume) is less well adapted (Nichols 2007). A few, such as biserrula (Biserulla pelecinus), yellow serradella (Ornithopus compressus) and French serradella (Ornithopus sativus) have found broader adaptation in southern Australia.
In regions of southern Australia that experience cool winter temperatures (<10°C daily maximum), the use of serradella has been accompanied by anecdotal reports of poor establishment and low winter growth rates (Rossiter et al. 1985; Revell et al. 1994). However, Rossiter et al. (1985) found comparable herbage production under defoliation (simulated grazing) to that of subterranean clover. Moreover, serradella persist in cold environments such as Germany (Klinkowski and Schwarz 1938), England, Denmark and the former Czechoslovakia (Fu et al. 1994). The modelled adaptation zone of yellow serradella in southern Australia is similar to that of the broadly adapted subterranean clover (Hill 1996) and temperature was not considered an adaptive constraint. The apparent discrepancies between the anecdotal reports, geographic observations and scientific literature require clarification.
This study utilised survival analysis to investigate the impact of cool temperatures on germination and emergence of serradella relative to three reference pasture legumes under four temperature treatments. Commonly used distribution analyses do not account for the failure of seeds to germinate or emerge and thermal time models are not always accurate at suboptimal temperatures (Chen et al. 2021). Survival (or time-to-event) analysis has recently been shown to be a reliable tool for analysing germination data, particularly for temperature treatment effects on germination over time (Eze et al. 2020; Romano and Stevanato 2020). An additional experiment investigated the growth of serradella from 21 to 49 days of growth under two temperature treatments and included measurements of leaf gas exchange. Overall, this study addresses the lack of peer-reviewed evidence on the effect of temperature on the establishment and winter productivity of serradella.
Materials and methods
Experiment 1: germination, emergence and early seedling development
The goal of these experiments (reported collectively as Experiment 1) was to examine variation in germination and emergence rate and early seedling growth in response to temperature between yellow serradella (Ornithopus compressus L.) and French serradella (Ornithopus sativus Brot.). Their response was benchmarked against a set of reference species commonly used in Australian temperate pasture systems: burr medic (Medicago polymorpha L.), lucerne (Medicago sativa L.) and subterranean clover (Trifolium subterraneum L.). The experiment was split into two components based on the test method:
Petri dish: seeds were sown on filter paper in Petri dishes in order to quantify seed germination rate. This method was quick and all seeds could be viewed as they developed. That is, as the seeds germinated, they could be counted frequently, with any remaining hard, dormant or dead seeds identified.
Soil: seeds were sown in a soil/potting mix medium in order to quantify the seedling emergence rate. However, hard, dead and dormant seeds could not be differentiated.
The experiments were conducted in climate-controlled growth rooms at The University of Western Australia, Perth, Australia. Four temperature treatments were used (max/min, °C): (1) 10/5; (2) 15/10; (3) 20/15; and (4) 25/20 with a 12 h day/night interval. It took approximately 15 min for each cabinet temperature change to occur but temperature then remained stable for the duration of the day or night period. Light intensity was 500 μmol photons m−2 s−1 (visible light range), adequate to saturate the photosynthetic system of C3 plants (Sager and McFarlane 1997). The light intensity took up to 30 min to stabilise each morning. Each of the four temperature treatments were imposed within one only of four separate plant growth cabinets, with three replicates arranged as a randomised block within each cabinet.
Table 1 lists the 10 species groups examined, including two species of serradella (Ornithopus compressus and Ornothopus sativus) and three reference pasture cultivars (Medicago polymorpha, Medicago sativa and Trifolium subterraneum). The serradella species groups consisted of the following:
Group A – cultivars that are no longer commercially available.
Group B – cultivars that are or have been commercially available in recent time.
Cool temperature reference – yellow serradella accession (unimproved germplasm) from a cool winter climate in Ouranoupoli, Macedonia (mean winter temperature 7.1°C) (Fick and Hijmans 2017).
Warm temperature reference – yellow serradella accession (unimproved germplasm) from a warm winter climate in Plettenburg Bay, South Africa (mean winter temperature 13.6°C) (Fick and Hijmans 2017).
Portuguese – French serradella cultivars developed in Portugal with potential species diversity due to divergent plant breeding aims.
Genus/species and common name | Seed weight (mg) | Seed nitrogen content | Seed phosphorus content | ||
---|---|---|---|---|---|
Species group | Cultivar or accession | (mg g−1) | |||
Ornithopus compressus (yellow serradella) | |||||
Group A | Elgara | n.a | n.a | n.a | |
Eneabba | 2.66 | 54.4 | 7.7 | ||
Madeira | 2.00 | 59.9 | 8.4 | ||
Paros | 2.98 | 61.5 | 4.7 | ||
Pitman | 2.62 | 56.2 | 6.6 | ||
Tauro | 2.60 | 54.2 | 6.3 | ||
Group B | Avila | 2.49 | 68.5 | 5.7 | |
Charano | 2.62 | 53.7 | 6.7 | ||
King | 2.51 | 59.3 | 7.7 | ||
Santorini | 3.18 | 52.9 | 8.3 | ||
SerraMax | 2.69 | 64.4 | 6.6 | ||
Yelbini | 3.31 | 46.5 | 6.3 | ||
Yellotas | 2.22 | 54.8 | 6.9 | ||
Cool temperature reference | GEH113COM-B1 | 2.29 | 58.2 | 8.2 | |
Warm temperature reference | ZAF61COM.1 | 2.17 | 60.3 | 6.3 | |
Ornithopus sativus (French serradella) | |||||
Group A | Emena | 2.79 | 53.8 | 9.4 | |
Grasslands Koha | 2.34 | n.a | n.a | ||
Group B | Cadiz | 2.62 | 55.3 | 3.3 | |
Erica | 2.27 | 52.6 | 5.8 | ||
Margurita | 2.26 | 51.5 | 8.2 | ||
Serratas | 2.74 | 55.0 | 8.4 | ||
Portuguese cultivars | Aza | 2.04 | 55.3 | 9.4 | |
Biata | 2.20 | 59.2 | 7.4 | ||
Setra | 2.36 | 51.9 | 9.5 | ||
Vinar | 2.20 | 60.9 | 6.7 | ||
Warta | 2.52 | 58.0 | 10.3 | ||
Reference species | |||||
Medicago polymorpha (burr medic) | Scimitar | 3.45 | 59.8 | 5.7 | |
Medicago sativa (lucerne) | SARDI 10 | 2.14 | 60.2 | 4.3 | |
Trifolium subterraneum (subterranean clover) | Dalkeith | 8.89 | 64.5 | 9.5 | |
Trikkala | 8.64 | 66.4 | 6.1 |
n.a, data not available. Insufficient seed for analysis.
Group A and Group B were delineated to assess variation in germination, emergence and early growth between cultivars that are currently (or were until recently) commercially available and those that are no longer available. Even though some cultivars in Group A were developed some time ago, the fact that they are still in commercial production may suggest that there are some favourable traits (such as even and successful establishment) that make them preferred by growers.
Seeds were analysed to demonstrate variation in seed size and nutrition as these can have an influence of seedling emergence and early vigour (Black 1957). Seed weight was determined by averaging the weight of three subsamples of 100 seeds. Seed phosphorus (P) was determined by completing a 5:1 mL nitric:perchloric acid digestion and colorimetric measurement using the yellow method (Motomizu et al. 1983). Seed nitrogen (N) was determined by analysing seeds with an Elementar (Model Vario Macro; Hanau, Germany) using the Dumas combustion method (Dumas 1831).
All seeds were removed from their pods and sterilised for 1 min in a 10% solution of bleach (NaClO). Hard-seeded species were scarified to improve germination and seeding rate was adjusted to achieve equivalent densities of germinable seed. To quantify seed germination rate, 25 germinable seeds were placed into 90 mm Petri dishes with Whatman grade 1 filter paper and wet with 3 mL of 0.5 mM CaSO4 solution; there were three replicate Petri dishes for each cultivar. To quantify seedling emergence rate, 25 germinable seeds were also sown into the soil medium with three replicate for each cultivar. A steam-pasteurised (maintained at 70°C for 30 min) potting mix was used to ensure nutrients were not limiting for seedlings once they had exhausted their seed reserves. Eighty-eight seedling trays (345 L × 295 W × 50 D mm) were steam-pasteurised and 3.5 kg of potting mix weighed into each tray. A row each of five cultivars was sown to a depth of 1 cm in each tray in a randomised and replicated design. After sowing, peat-based rhizobia (Becker Underwood) was applied at a rate of 0.5 g per 9 L watering can. A combination of Group S (WSM471), Group C (WSM1325), Group AL (RRI128) and Group AM (WSM1115) was applied to accommodate the rhizobia requirements of the Ornithopus, Trifolium and Medicago species.
The Petri dish and soil components of the study ran concurrently for 3 weeks. In the Petri dish experiment, germination was recorded daily, with seeds classified as germinated once the radicle was >5 mm in length. Germinated seeds were recorded and removed daily; after 21 days, the total number of germinated, imbibed (dormant), dead and hard seeds (i.e. impermeable to water) were recorded. For analysis purposes, data pertaining only to germinated and imbibed seeds were considered, as dead and hard seeds were not considered germinable. In the soil experiment, seedling emergence (classified as cotyledons in a fully and open horizontal position) was recorded daily. As growth proceeded, leaf development was measured as days to the appearance of the first, second, third, or fourth leaves. The first leaf on burr medic, lucerne and subterranean clover is unifoliate, followed by trifoliate leaves. All leaves on serradella are pinnate leaves. Leaf appearance was deemed to have occurred when ≥50% of the plants in a row had fully developed and open leaves (i.e. not folded). At harvest, 21 days after sowing, plants were cut at the stem base and the roots were washed free of the growth medium. Plant dry mass was recorded and reported as a daily growth rate to account for differences in emergence time. Shoot and root dry mass (DM) productivity is expressed as a rate (mg plant−1 day−1) from emergence to 21 days after sowing to standardise the effect of delayed emergence and variation in the number of plants per row. Root observations confirmed nodulated plants, which were scored on the number and size of nodules present within a row using a classification system provided in Howieson and Dilworth (2016). However, nodule scores were closely associated with root development rather than temperature; thus, no further analysis was completed.
Experiment 2: relative growth rates (RGR) and leaf gas exchange
Experiment 2 assessed RGRs and leaf gas exchange among serradella cultivars at cool temperatures compared to ‘ideal’ growing temperatures. The growth assessments occurred on two cultivars each of yellow serradella (Santorini and Avila) and French serradella (Margurita and Serratas), benchmarked against a winter-active lucerne cultivar (SARDI 10) and a subterranean clover cultivar (Woogenellup), which is also known for good winter vigour (Collins et al. 1996). Two temperature treatments were selected based on preliminary data from the Petri dish experiment and published literature. Although temperatures below 10°C maximum and 5°C minimum are common throughout southern Australia, they were deemed too cold for experimental purposes as little or no growth would occur and identifying differences between species would be difficult. Maximum temperatures of 20–25°C are considered optimal for legumes (Fukai and Silsbury 1976; McWilliam 1978). Hill and Luck (1991) showed that temperate pasture legumes produced the highest DM at 24/20°C (max/min) compared with lower temperatures. However, given that we had strong indications of better early growth at 20/15°C than 25/20°C for serradella in Experiment 1, we opted for an intermediate temperature range of 23/18°C to compare with growth at 15/10°C. There were five harvest times (weekly from 3 to 7 weeks after sowing) and three replicates of each cultivar × temperature × harvest time combination. The pots for each temperature treatment were placed randomly in a single growth cabinet and pot location re-randomised weekly.
Each pot (polyvinyl chloride; 200 mm (H) ×90 mm (D)) was filled with a 1.2 kg mixture of sandy loam and potting mix. The drained upper limit (field capacity) of the potting soil was estimated by determining the amount of water held per gram of dry soil after pots had been watered to saturation and then allowed to drain for 72 h (i.e. until free drainage had ceased). Pots were then maintained at field capacity for the duration of the experiment. Nutrient analyses indicated that the soil contained sufficient nutrients for plant growth over the duration of the experiments (data not shown). All seeds were removed from their pods and sterilised for 1 min in a 10% solution of sodium hypochlorite (NaClO); hard-seeded serradella cultivars required scarification. Ten seeds were sown in pots to a depth of 1 cm. To mimic sward conditions, pots were fitted with reflective sleeves made of insulation sarking that were raised regularly to match canopy height (Jeffery et al. 2017).
The pots were kept in darkened plant growth cabinets at 15°C until the cotyledons started emerging. At this stage, lighting was imposed and temperatures were changed to 23/18°C in both cabinets to encourage good early growth. Plants were grown for 3 weeks before an initial harvest (21 days) where shoots were cut at the soil surface and roots were washed from the soil medium over a 2 mm sieve before being oven-dried at 70°C for 3 days and weighed. Post-harvest, one growth cabinet was reduced to the low temperature (15/10°C) for the remainder of the experiment. Three replicates of each cultivar were harvested from each of the two temperature treatment cabinets every 7 days. Thus, plant measurements and destructive harvests were completed at 21, 28, 35, 42 and 49 days after sowing.
At each harvest, plants were harvested by cutting the shoot at the soil surface. Shoots and roots were processed, dried and weighed as described above.
Immediately before harvest of each treatment, gas exchange (carbon dioxide and water) was measured on five young, fully expanded leaves with an LI-6400 portable gas-exchange system (Li-COR, Lincoln, NE, USA) to calculate photosynthetic rate (CO2 assimilation), stomatal conductance, intracellular CO2 concentration, and transpiration rate. Measurements were made at ambient CO2 (400 μmol mol−1) with fixed airflow (500 μmol s−1) and block temperatures were set at 23°C and 15°C to reflect the maximum daytime temperature of each temperature cabinet. Photosynthetically active radiation was set at 1500 mol quanta m−2 s−1. As the leaves did not fill the chamber, they were removed post-measurement and leaf area was measured on a Li-Cor LI-3000A Portable Area Meter. A stomatal ratio of 0.5 was used for calculations.
Statistical analysis
Statistical analyses were performed using XLSTAT (Addinsoft 2015). Data were collected for 30 individual cultivars/accessions and the raw data grouped for analysis (Table 1). Descriptive statistics relate to germinated or emerged data and do not include data for seeds that did not germinate or seedlings that did not emerge. As the data were not normally distributed and of uneven sample size, they were analysed using a Kruskall–Wallis non-parametric test. A Steel-Dwass-Critchlow-Fligner procedure test was used to assess post hoc significant (P < 0.05) effects of each species group and temperature on germination and emergence. A heat map was prepared to demonstrate the strength and direction of these differences according to the Wij statistics generated. Harmonic means were used to represent mean germination and emergence, as appropriate, for non-parametric data (Sheskin 2003).
Survival analysis was undertaken using a Cox proportional hazards model with regression coefficients to indicate the likelihood of germination and emergence relative to a reference value. Additional information provided by the hazard ratio (HR) indicates the likelihood of an event compared to the next short interval of time (McNair et al. 2012). The reference values are from lucerne for species group and 20/15°C for temperature. The Wald Chi2 indicates the most influential variable on the model with Pr > Chi2 indicating significance.
A two-way analysis of variance (ANOVA) was used to assess the effects of temperature and species group on the rate of total DM (shoot and root) produced. However, despite a significant temperature × species interaction, temperature had the greatest effect on the model, so we conducted a one-way ANOVA to assess the effect of species group within the four temperature treatments. Note that the number of emerged plants varied between cultivars within each species group, so the DM values were standardised by the number of emerged plants. As the number of days to emergence also varied among cultivars within species groups, the rate of DM production was also standardised by the number of days from emergence to the experimental conclusion at 21 days. Due to unequal sample sizes, a Games-Howell post hoc test was used to demonstrate significant differences among species groups.
The effects of temperature treatment and seed phosphorus or seed nitrogen concentration on the rate of shoot DM produced were analysed with a two-way ANCOVA, with the results presented as linear regressions with shoot DM as the independent variable and seed nitrogen or phosphorus as the dependent variable. The correlation coefficient is presented for the linear regressions with significant relationships (P < 0.05).
The effect of temperature treatment on shoot DM was also modelled with linear regression and analysed with a one-way ANCOVA.
Shoot relative growth rates were calculated using the following equation:
where RGR is relative growth rate; ln is natural log; W2 is shoot DM at time 2; W1 is shoot DM at time 1; t2 is number of days to sampling time 2; and t1 is number of days to sampling time 1. A two-way ANOVA was conducted to compare the effects of temperature and cultivar on RGR. The results for each cultivar were then modelled with linear regression and analysed with one-way ANCOVA using Tukey’s post hoc test to compare the cultivar response within each temperature treatment.
Each gas exchange measurement was analysed using a two-way ANOVA with temperature and species as factors; the Games-Howell post hoc test was used to determine significant differences (P < 0.05).
Results
Experiment 1: germination and emergence in response to temperature
Germination was significantly slower at 10/5°C than at the other temperatures (Fig. 1a, b). Yellow serradella (Group A and Group B) and Group B French serradella cultivars generally germinated faster than burr medic and subterranean clover, but not lucerne (Fig. 1a, b). The Group B yellow serradella cultivars germinated more rapidly than the Group A cultivars at the lowest (10/5°C) and highest (25/20°C) temperatures. The cool temperature reference did not differ from the warm temperature reference, except at the highest temperature, where the cool temperature reference germinated more rapidly. Among French serradella, Group B cultivars germinated faster than Group A cultivars at the lowest temperature but were similar at the other temperatures. The French serradella groups germinated more rapidly than burr medic at the warmest temperature but did not differ from lucerne (except at 25/20°C where they were slower to germinate) or subterranean clover (except at 20/15°C where they were faster to germinate). Yellow serradella germinated more rapidly than French serradella at 15/10°C and 25/20°C (Fig. 1, Supplementary Table S3).
(a) Heatmap displaying the extent and direction of Wij statistics of Steel-Dwass-Critchlow-Fligner test for germination. The map compares the variation in germination time (days) in response to temperature (10/5, 15/10, 20/15 and 25/20°C, max/min) among species groups from yellow serradella, French serradella and reference cultivars (burr medic, subterranean clover and lucerne). Colour coding indicates the extent of the variation among groups (compare x-axis then y-axis), with red indicating slower germination time and blue indicating faster germination time. White indicates no significant difference among groups. The harmonic mean and s.d. are also presented. Only data from germinated seeds are included (see Table 2 for survival analysis, including data from non-germinated seeds). (b) Nested plots demonstrate the influence of temperature treatments on the harmonic mean of germination time for each species group. See Table 1 for the description of species groups.
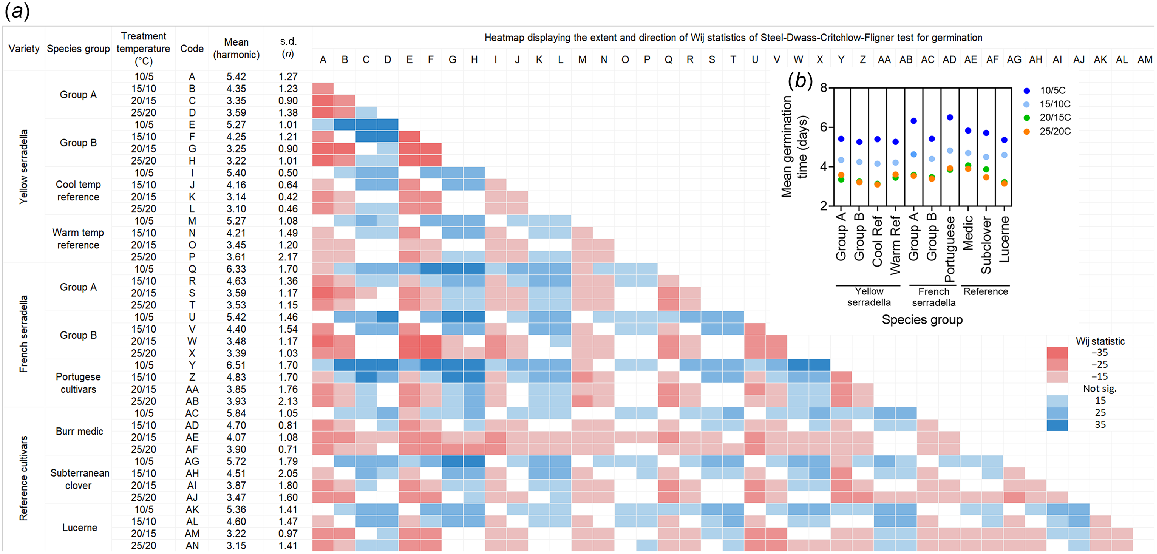
Emergence was also significantly slower at 10/5°C than at the other temperatures (Fig. 2a, b). Yellow and French serradella species groups generally emerged faster than subterranean clover at cooler temperatures (10/5°C, 15/10°C). However, they emerged more slowly than lucerne at warmer temperatures (20/15°C, 25/20°C). For serradella species groups, the fastest rate of emergence was at 20/15°C and emergence at 25/20°C was similar to that achieved at 15/10°C. Group B yellow serradella cultivars emerged faster than Group A cultivars at all temperatures and subterranean clover at the cool temperatures. Despite a lower mean emergence time for the cool temperature reference compared to the warm temperature reference at all temperature treatments, they did not statistically differ (Fig. 2, Table S3). There was no difference in emergence time among French serradella groups. Group B yellow serradella cultivars emerged slower than Group B French serradella cultivars at 15/10°C and 20/15°C.
(a) Heatmap displaying the extent and direction of Wij statistics of Steel-Dwass-Critchlow-Fligner test for emergence. The map compares the variation in emergence time (days) in response to temperature (10/5, 15/10, 20/15 and 25/20°C, max/min) among species groups from yellow serradella, French serradella, and reference cultivars (burr medic, subterranean clover, and lucerne). Colour coding indicates the extent of the variation among groups (compare x-axis then y-axis), with red indicating slower emergence time and blue indicating faster emergence time. White indicates no significant difference among groups. The harmonic mean and s.d. are also presented. Only data from emerged seeds are included (see Table 2 for survival analysis, including data from non-emerged seeds). (b) Nested plots demonstrate the influence of temperature treatments on the harmonic mean of emergence time for each species group. See Table 1 for the description of species groups.
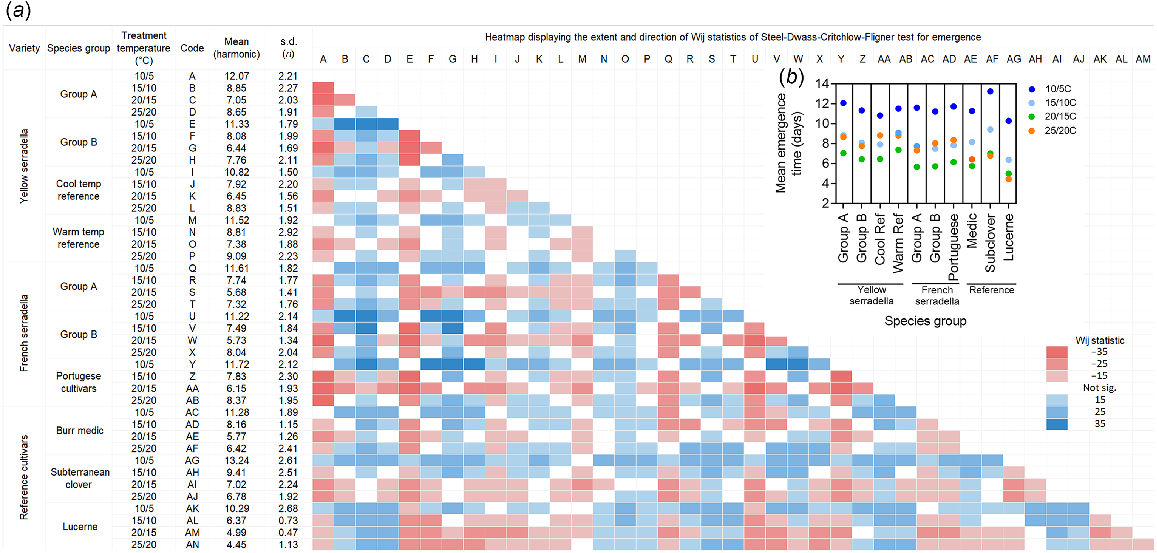
Survival analysis accounted for seeds that did not germinate or emerge. The analysis used lucerne as a reference value for species group and 20/15°C as a reference value for temperature treatment (Table 2). The Wald Chi2 (WChi2) value (depicting the variable with the greatest effect on the model) indicated that the lowest temperature treatment (10/5°C) had the greatest overall effect on germination and emergence. Furthermore, the emergence model generally had greater WChi2 values than the germination model, indicating that the variables (species group and temperature) had a greater effect on seed emergence than germination. Further evidence of this effect was the percentage of seeds that did not emerge being greater than the percentage of seeds that did not germinate (Table 2).
Germination | Emergence | |||||||||
---|---|---|---|---|---|---|---|---|---|---|
Factor | Variable | Wald Chi-square | Pr > Chi2 | Hazard ratio | % Not germinated | Wald Chi-square | Pr > Chi2 | Hazard ratio | % Not emerged | |
Species group | Lucerne | Reference value | Reference value | |||||||
YS (Group A) | 1 | n.s. | 0.93 | 274 | *** | 0.34 | ||||
YS (Group B) | 2 | n.s. | 1.08 | 177 | *** | 0.43 | ||||
Cool temp ref | 2 | n.s. | 1.14 | 104 | *** | 0.42 | ||||
Warm temp ref | 1 | n.s. | 0.92 | 153 | *** | 0.34 | ||||
FS (Group A) | 17 | *** | 0.75 | 61 | *** | 0.57 | ||||
FS (Group B) | 1 | n.s. | 0.93 | 188 | *** | 0.40 | ||||
Portuguese | 67 | *** | 0.59 | 178 | *** | 0.42 | ||||
Burr medic | 8 | ** | 0.79 | 22 | *** | 0.68 | ||||
Subclover | 43 | *** | 0.62 | 208 | *** | 0.34 | ||||
Temp (°C) | 10/5 | 898 | *** | 0.38 | 956 | *** | 0.36 | |||
15/10 | 324 | *** | 0.56 | 178 | *** | 0.65 | ||||
20/15 | Reference value | Reference value | ||||||||
25/20 | 9 | ** | 0.91 | 326 | *** | 0.55 | ||||
Interaction species group × temperature | Lucerne × 10/5 | 55 | *** | 0.29 | 0 | 161 | *** | 0.12 | 1 | |
Lucerne × 15/10 | 36 | *** | 0.37 | 0 | 42 | *** | 0.34 | 7 | ||
Lucerne × 20_15 | Reference value | 0 | Reference value | 3 | ||||||
Lucerne × 25/20 | 0 | n.s. | 1.04 | 0 | 0 | n.s. | 1.08 | 3 | ||
Group A × 10/5 | 99 | *** | 0.28 | 0 | 425 | *** | 0.07 | 28 | ||
Group A YS × 15/10 | 44 | *** | 0.43 | 1 | 281 | *** | 0.11 | 21 | ||
Group AYS × 20/15 | 1 | n.s. | 0.90 | 0 | 189 | *** | 0.17 | 15 | ||
Group A YS × 25/20 | 16 | *** | 0.60 | 3 | 334 | *** | 0.09 | 34 | ||
Group B YS × 10/5 | 85 | *** | 0.31 | 0 | 395 | *** | 0.08 | 21 | ||
Group B YS × 15/10 | 35 | *** | 0.48 | 0 | 219 | *** | 0.15 | 14 | ||
Group B YS × 20/15 | 0 | n.s. | 0.99 | 0 | 138 | *** | 0.22 | 13 | ||
Group B YS × 25/20 | 0 | n.s. | 0.93 | 2 | 262 | *** | 0.13 | 22 | ||
Cool temp ref × 10/5 | 51 | *** | 0.30 | 0 | 202 | *** | 0.09 | 19 | ||
Cool temp ref × 15/10 | 17 | *** | 0.50 | 0 | 104 | *** | 0.18 | 9 | ||
Cool temp ref × 20/15 | 3 | n.s. | 1.33 | 0 | 98 | *** | 0.18 | 21 | ||
Cool temp ref × 25/20 | 4 | n.s. | 1.38 | 0 | 170 | *** | 0.10 | 27 | ||
Warm temp ref × 10/5 | 44 | *** | 0.30 | 0 | 219 | *** | 0.08 | 23 | ||
Warm temp ref × 15/10 | 20 | *** | 0.47 | 0 | 143 | *** | 0.13 | 14 | ||
Warm temp ref × 20/15 | 2 | n.s. | 0.75 | 0 | 100 | *** | 0.18 | 10 | ||
Warm temp ref × 25/20 | 14 | *** | 0.53 | 1 | 213 | *** | 0.06 | 63 | ||
Group A FS × 10/5 | 116 | *** | 0.21 | 1 | 273 | *** | 0.09 | 6 | ||
Group A FS × 15/10 | 47 | *** | 0.37 | 0 | 107 | *** | 0.22 | 3 | ||
Group A FS × 20/15 | 8 | ** | 0.66 | 1 | 33 | *** | 0.44 | 6 | ||
Group A FS × 25/20 | 13 | *** | 0.59 | 4 | 146 | *** | 0.17 | 14 | ||
Group B FS × 10/5 | 91 | *** | 0.28 | 0 | 363 | *** | 0.08 | 25 | ||
Group B FS × 15/10 | 45 | *** | 0.41 | 0 | 219 | *** | 0.14 | 26 | ||
Group B FS × 20/15 | 6 | * | 0.72 | 0 | 135 | *** | 0.21 | 22 | ||
Group B FS × 25/20 | 3 | n.s. | 0.81 | 0 | 258 | *** | 0.11 | 26 | ||
Portugal × 10/5 | 173 | *** | 0.18 | 9 | 375 | *** | 0.08 | 17 | ||
Portugal × 15/10 | 89 | *** | 0.30 | 4 | 207 | *** | 0.15 | 15 | ||
Portugal × 20/15 | 44 | *** | 0.42 | 5 | 124 | *** | 0.23 | 13 | ||
Portugal × 25/20 | 51 | *** | 0.39 | 3 | 277 | *** | 0.11 | 23 | ||
Burr medic × 10/5 | 71 | *** | 0.25 | 0 | 192 | *** | 0.10 | 3 | ||
Burr medic × 15/10 | 37 | *** | 0.38 | 1 | 82 | *** | 0.22 | 0 | ||
Burr medic × 20/15 | 17 | *** | 0.50 | 0 | 8 | ** | 0.63 | 0 | ||
Burr medic × 25/20 | 11 | *** | 0.60 | 0 | 60 | *** | 0.28 | 4 | ||
Subclover × 10/5 | 117 | *** | 0.21 | 9 | 322 | *** | 0.07 | 17 | ||
Subclover × 15/10 | 82 | *** | 0.26 | 15 | 268 | *** | 0.08 | 39 | ||
Subclover × 20/15 | 25 | *** | 0.49 | 1 | 166 | *** | 0.15 | 23 | ||
Subclover × 25/20 | 37 | *** | 0.41 | 16 | 161 | *** | 0.15 | 24 |
The Wald Chi2 indicates the most influential variable on the model with Pr > Chi2 indicating significance at *P ≤ 0.05; **P ≤ 0.01; ***P ≤ 0.001; and n.s., not significant at P ≤ 0.05. Hazard ratio coefficients <1 indicate that germination or emergence is less likely to happen than the reference value. Data are referenced against species group variable lucerne and the 20/15°C treatment variable.
The effect of cool temperatures (i.e. 10/5°C and 15/10°C) on germination was comparable between lucerne (Hazard Ratio (HR) 0.29 at 10/5°C and 0.37 at 15/10°C) and the other species groups, but French serradella (Portuguese and Group A cultivars) and subterranean clover had the lowest HR (range 0.18–0.21 at 10/5°C and 0.26–0.37 at 15/10°C) or largest impact on the germination model (greatest WChi2), demonstrating that cold temperatures impacted them the most. The germination of Group B yellow serradella cultivars did not differ to the reference value at warm temperatures (20/15°C and 25/20°C) and Group B French serradella cultivars were not significantly different to the reference value at 25/20°C. However, warm temperatures adversely affected germination for Group A yellow serradella cultivars and the cool temperature reference (i.e. lower HR at 25/20°C than 20/15°C). Notably, the HR of yellow serradella (cool temperature reference) was >1.3 in the two highest temperature treatments. While not statistically significant, these values were the only HR to exceed lucerne in the experiment.
In contrast to the germination data, where French serradella species groups were most affected, yellow serradella species groups (particularly Group A cultivars) were most affected in the emergence model at cool temperatures; however, subterranean clover remained among the most impacted of the reference species. In addition, all serradella species groups had lower HR and greater model impact (WChi2) at 25/20°C than 20/15°C or 15/10°C, but this was not the case for reference species. In some instances, the highest temperature (25/20°C) affected serradella emergence almost as much as the lowest temperature (10/5°C).
The impact of temperature treatment on the shoot and root DM production rate varied among species groups (species group × temperature treatment interaction (P < 0.001)) (Fig. 3), with this interaction largely driven by the greater DM response of subterranean clover in the cooler temperature treatments than the warmer temperature treatments. No significant variation in the shoot or root DM production rate occurred among serradella species groups at any temperature. At cool temperatures (i.e. 10/5°C and 15/10°C), subterranean clover had the greatest shoot and root DM, but the remaining species groups did not differ. At 20/15°C, subterranean clover had greater shoot and root DM than serradella species groups but similar shoot DM to lucerne. At 25/20°C, lucerne had greater shoot DM than most serradella species (except Portuguese cultivars) but did not differ significantly from the other reference species.
The effect of four temperature treatments (10/5, 15/10, 20/15 and 25/20°C, max/min) and 10 species groups on the rate of shoot (top green bars) and root (bottom beige bars) dry mass production from the time of emergence to 21 days after sowing. Values are species group means (+s.d.). There was a significant (P < 0.001) temperature treatment × species group interaction for total plant DM but only species group differences within each temperature treatment are presented. Temperature treatment means annotated with the same capital letter at the top of the graph do not differ at P < 0.05. Significant (one-way ANOVA, P < 0.05) variation among species groups for shoot or root dry mass within each temperature treatment is denoted by different lowercase letters (Games-Howell post hoc test).
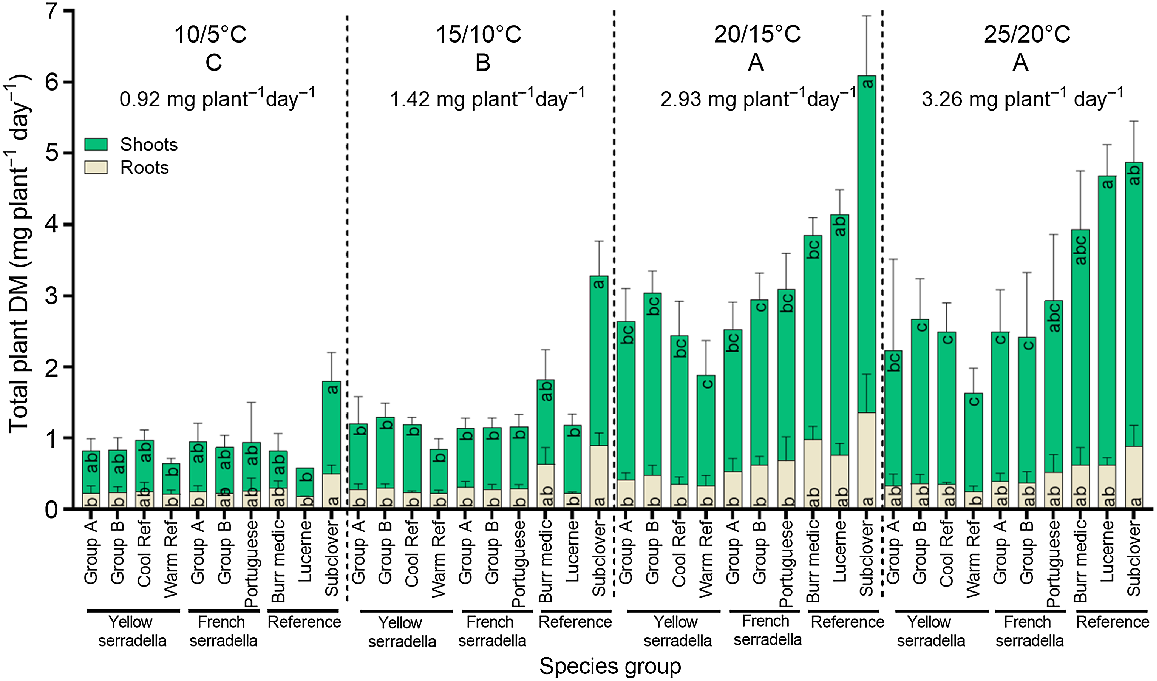
A significant interaction of temperature and seed nitrogen (N) (P = 0.003) and temperature and seed phosphorus (P) (P < 0.001) occurred for shoot DM production rate, primarily driven by temperature (F ≥ 304), not seed N (F = 10) or seed P (F = 13). Moreover, when we applied linear regression to each temperature treatment, no significant relationships occurred between shoot DM production rate and seed P (Fig. 4), except for a weak positive relationship at 10/5°C (R2 = 0.10). In contrast, shoot DM production rate and seed N had a weak but positive correlation for all temperature treatments, which was strongest at 20/15°C (Fig. 4).
The relationship between shoot dry mass and (a) seed nitrogen concentration and (b) seed phosphorus concentration with four temperature treatments (10/5, dark blue; 15/10, light blue; 20/15, green; and 25/20°C, orange; max/min) for yellow serradella, French serradella and reference species groups. The correlation coefficient for each temperature response is shown in the graph if the relationship was significant at P < 0.05. Each data point represents a replicate (n = 3). Significant (P < 0.05) effects of temperature treatment are denoted by different capital letters (2-way ANCOVA).
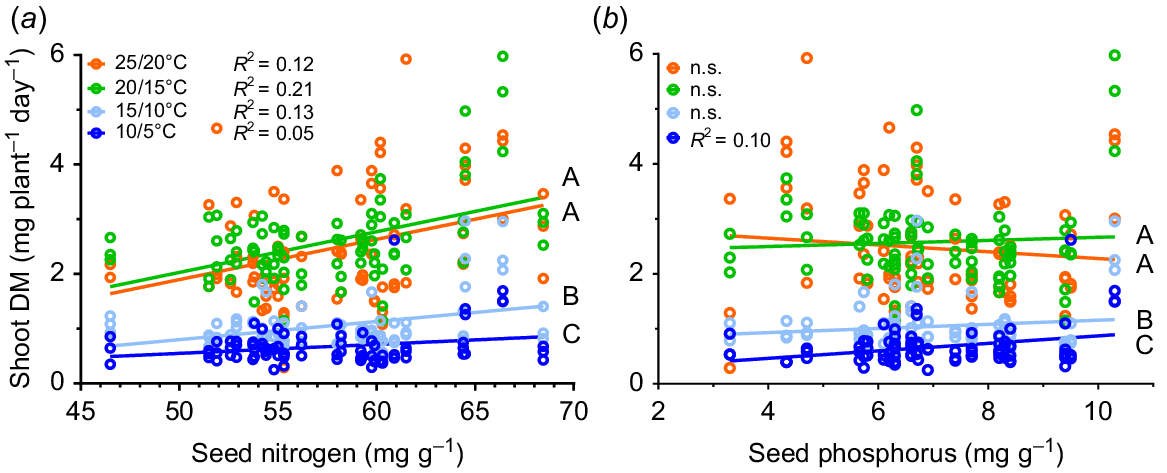
Each temperature treatment had the number of days to leaf appearance recorded over the 21-day experimental period. Leaf appearance was deemed to occur when >50% of the plants within a row had reached a specific leaf stage. At 10/5°C, no species group had >50% of seedlings with an open first leaf during the experimental period (Fig. 5). At 15/10°C, only the reference species produced a first (unifoliate) leaf. No serradella species group reached the third leaf stage in any temperature treatment, whereas the reference species reached the third (all species) and fourth (subterranean clover only) leaf stages.
Time of leaf appearance over the 21-day growth period post-sowing relative to shoot DM for species groups from yellow serradella (a, b, c, d), French serradella (e, f, g) and reference groups (h, i, j) with four temperature treatments (10/5, dark blue; 15/10, light blue; 20/15, green; and 25/20°C, orange; max/min). Different letters denote significant temperature treatment differences (ANCOVA with Games-Howell post hoc test). Numbering within line graphs represents the mean date that ≥50% of plants reached a leaf stage (1, first leaf; 2, second leaf; 3, third leaf; and 4, fourth leaf).
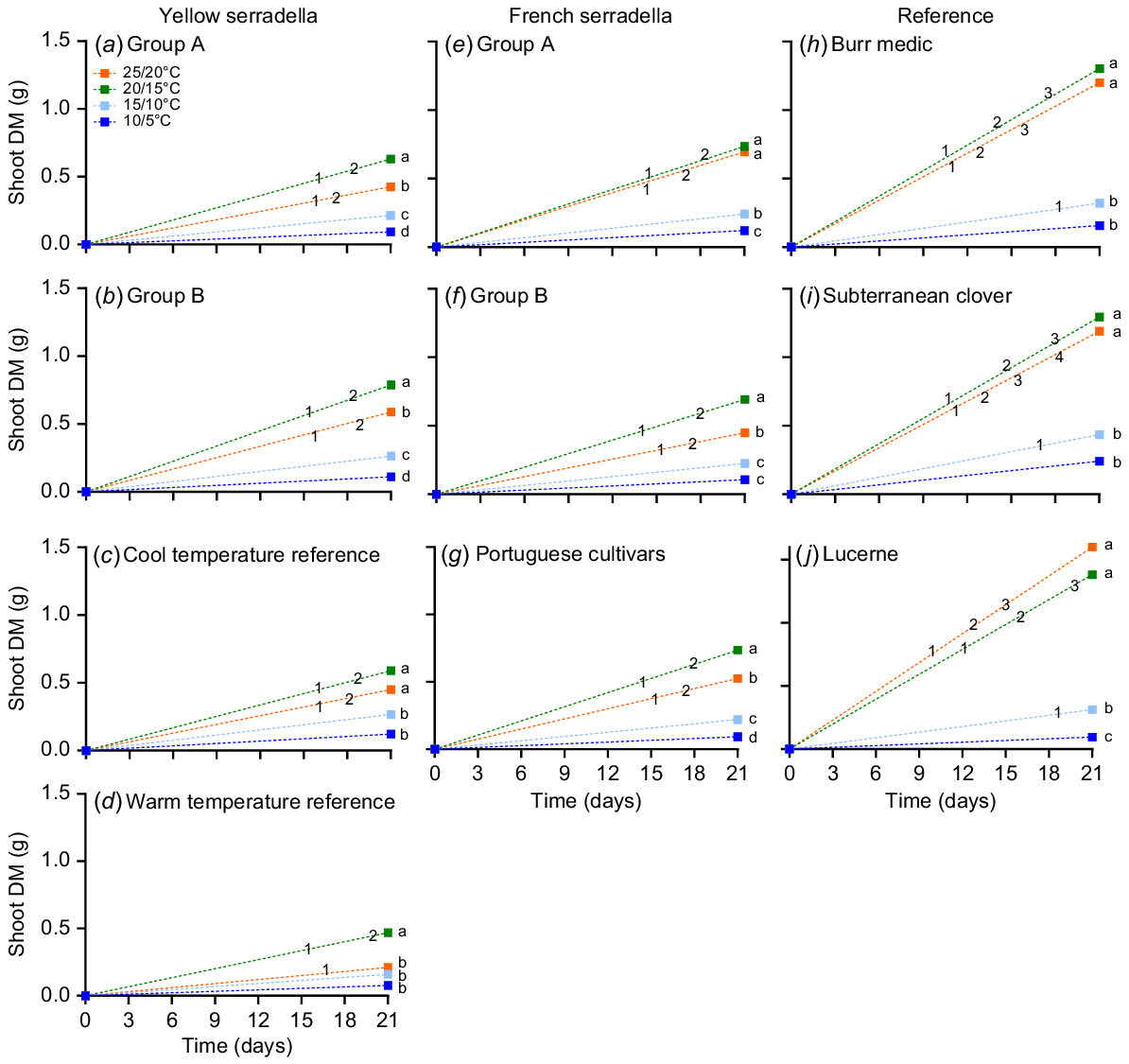
For shoot DM (which includes the effect of delayed germination and/or emergence), significant (ANCOVA P < 0.001) temperature treatment differences occurred between species groups (Fig. 5). Serradella species groups generally had greater shoot DM at 20/15°C than 25/20°C, whereas the reference species did not significantly differ at these temperatures. At 10/5°C, subterranean clover had approximately double the shoot DM of serradella species groups, but no significant differences occurred among species groups at 15/10°C (Table S1). At 20/15°C and 25/20°C, all reference species had more shoot DM than serradella species groups (except Group A French serradella cultivars at 25/20°C).
Experiment 2: temperature effects beyond the seedling stage
Experiment 2 compared shoot RGR of two yellow serradella and two French serradella cultivars with subterranean clover and lucerne from 21 to 49 days after sowing. A positive RGR response occurred for the higher temperature treatment (23/18°C; P = 0.006, two-way ANOVA) but did not differ between cultivars. One-way ANCOVA and pairwise comparisons at 15/10°C demonstrated that lucerne and subterranean clover had greater RGR than all serradella cultivars except ‘Avila’ (Fig. 6). On closer analysis, this result primarily occurred because subterranean clover had more initial shoot DM at the start of the measurement period (i.e. after 21 days) and lucerne had more shoot DM at the last harvest than serradella cultivars (Table S2). At 23/18°C, lucerne had greater RGR than all other cultivars. The RGR of subterranean clover and serradella cultivars did not differ (except for cv. Serratas, which was lower). When we examined the raw DM values, it was evident that temperature affected lucerne the most, with DM at 15/10°C only 60% of that achieved at 25/20°C, compared to serradella (77–85%) and subterranean clover (96%) (Table S2).
Effect of temperature (23/18°C (red line and red circles) and 15/10°C (blue dash line and blue squares)) and harvest time on relative growth rate (RGR) of shoots (log shoot DM (g)) for six cultivars. Yellow serradella cvs (a) Avila and (b) Santorini; French serradella cvs (c) Margurita and (d) Serratas; lucerne cv. (e) SARDI 10; and subterranean clover cv. (f) Woogenellup. Each symbol represents a replicate (n = 3). Different letters indicate significant differences between cultivars at 15/10°C (lower case letters) and 23/18°C (capital letters) based on a comparison of the least square means and Tukey’s post hoc test.
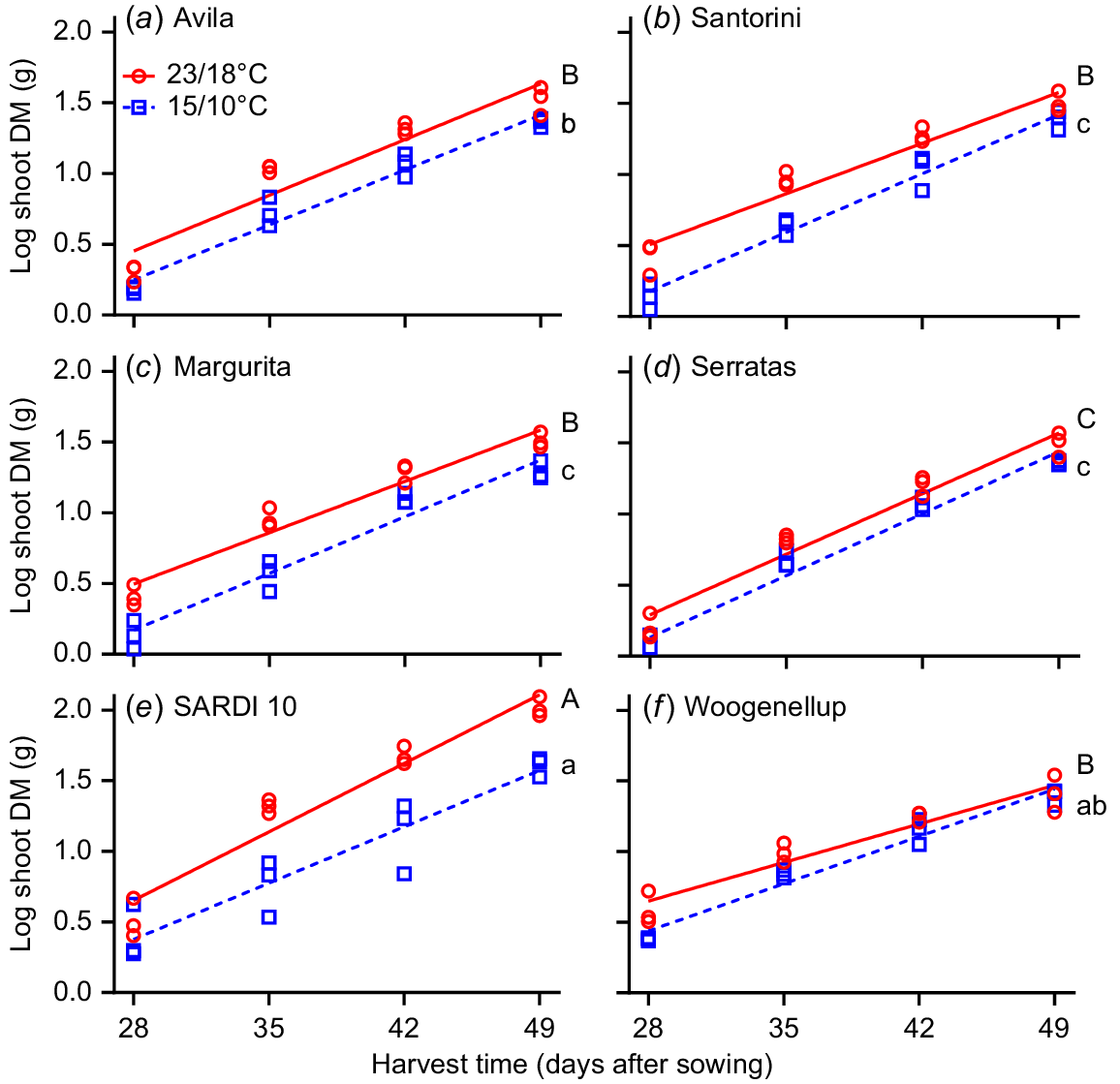
A significant cultivar × temperature treatment interaction occurred for all physiological measurements (photosynthetic rate, stomatal conductance, transpiration rate, and intercellular CO2). While no significant differences occurred between cultivars at 15/10°C, significant differences occurred between cultivars at 23/18°C (Fig. 7a–d). Subterranean clover and lucerne had higher photosynthetic rates (CO2 assimilation) at 23/18°C than French serradella (25 vs 17–20 μmol CO2 m−2 s−1) but did not differ from yellow serradella (21–23 μmol CO2 m−2 s−1). Yellow serradella had a higher photosynthetic rate at 23/18°C than 15/10°C, but French serradella was not responsive to temperature (Fig. 7a).
Effect of two temperature treatments (15/10 (blue bars to left) and 23/18°C (red bars to right for each species) (max/min)) and four species: yellow serradella (YS); French serradella (FS); lucerne (L); and subterranean clover (SC) on leaf gas exchange measurements. (a) CO2 assimilation, (b) stomatal conductance, (c) transpiration rate and (d) intercellular CO2. Different letters within plots indicate significant (P < 0.05) temperature × species differences (Games-Howell post hoc test).
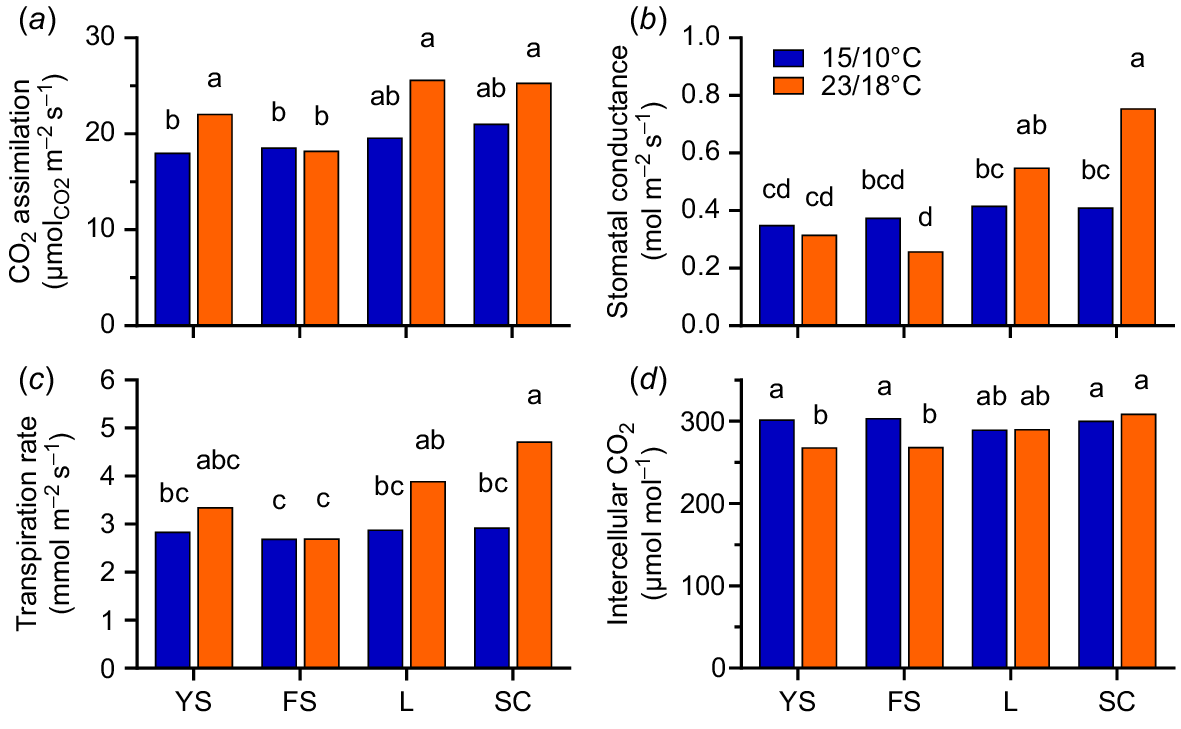
For stomatal conductance and transpiration rate, the significant cultivar × temperature treatment interaction was primarily driven by greater values for subterranean clover at 23/18°C than 15/10°C, as temperature did not affect stomatal conductance or transpiration rate for serradella cultivars or lucerne (Fig. 7b, d). Subterranean clover and lucerne had up to double the stomatal conductance at 23/18°C (0.76 and 0.55 mol m−2 s−1, respectively) than Ornithopus spp. (0.23–0.36 mol m−2 s−1), with subterranean clover higher than all other cultivars and nearly double the stomatal conductance achieved at 15/10°C (Fig. 7b).
For intercellular CO2, no significant differences occurred among species at 15/10°C (range 290–309 μmol mol−1). However, at 23/18°C, intercellular CO2 decreased for yellow and French serradella cultivars, but not lucerne and subterranean clover (Fig. 7c).
Discussion
Seedling germination and emergence
In regions of southern Australia that experience cool winter temperatures, the use of serradella species is adversely affected by anecdotal reports of poor establishment and low winter growth rates relative to other temperate pasture legumes (Rossiter et al. 1985). However, we found no evidence that cool temperatures, in isolation, limit the early productivity of serradella species relative to other commonly used temperate pasture legumes.
Cool temperature treatments delayed germination rates by 1–4 days among the species groups studied, compared to the fastest germination rate in the warm temperature treatments. Rapid and uniform germination is generally a favourable trait for seedling establishment (Covell et al. 1986; Blum 2018) and species competitiveness. Serradella species groups demonstrated (with a higher hazard ratio) that they were more likely to germinate at any temperature (and more rapidly in the case of yellow serradella) than burr medic or subterranean clover. However, warmer temperatures (i.e. 25/20°C, max/min), delayed serradella emergence, which may place some cultivars at a competitive disadvantage in relation to faster emerging weeds and/or grasses.
The most rapid emergence for serradella species groups occurred at 20/15°C (French serradella groups > yellow serradella groups). For some serradella species groups, the emergence rate at 15/10°C did not significantly differ from that at 25/20°C. At these temperatures, fast-growing species such as lucerne emerged from the soil within 2 days after sowing, whereas serradella did not emerge until 8–12 days. Such a delay is not insignificant, with differences of only one day contributing to improved leaf area in cereals (Richards et al. 2002). Genetic studies have subsequently been undertaken to improve the germination and emergence of agronomically important seed crops (Finch-Savage et al. 2010; Zhang et al. 2015). While similar work has not been specifically undertaken for pasture species such as serradella, their characteristic seed germination and emergence patterns are important as they may influence their germination and establishment success on farms.
As seeds were scarified before sowing, the delayed emergence pattern observed in some serradella cultivars cannot be due to variation in seed coat permeability but may reflect some physiological dormancy. Delayed seedling emergence can be an adaptive response to false breaks (i.e. unseasonal rainfall events in summer and/or early autumn that causes pasture seeds to germinate and seedlings to emerge but insufficient further rainfall occurs to ensure seedling survival) (Revell et al. 1998; Dear et al. 2002). In this case, by remaining dormant in the soil, the species may have a greater chance of survival than for species where seeds uniformly emerge. In summary, cool temperatures do not limit serradella germination or emergence relative to other pasture legumes, but warm temperatures (i.e. ≥25°C) delay emergence.
The pairwise comparisons in the heat maps (Figs 1 and 2) reveal the differences between species groups (Table S3). For example, Group B yellow serradella often had more rapid germination and emergence than Group A, presumably a collateral consequence of selection/breeding for improved agronomic fitness. In comparison, minor variation occurred between Group A and Group B cultivars of French serradella for germination or emergence. Late-maturing Portuguese cultivars of French serradella were slower to germinate than Group B French serradella cultivars, but this did not result in a slower emergence time.
We specifically included two yellow serradella accessions collected at locations differing by >5°C in winter temperatures to examine whether provenance was likely to have an impact on germination or emergence at low temperatures. However, no significant differences were evident between these accessions for germination or emergence at any temperature (except for the cool temperature reference germinating faster at warm temperatures than cool temperatures). These results are indicative only, and analysis of a greater suite of germplasm is required to assess whether this is a useful selection method for this trait.
Early dry matter production and leaf development
At temperatures considered sub-optimal for plant growth (i.e. <10°C daily maximum), all pasture species had slow establishment and early growth, with the early DM productivity of serradella comparable to the reference species at low temperatures. Maximum shoot DM for serradella species groups occurred at 20/15°C. Inevitably, late sowing times or late breaks in the season will result in low pasture productivity, regardless of the legume species used. However, at warmer temperatures (i.e. ≥25°C daily maximum), serradella produced up to three times less shoot DM than subterranean clover and lucerne due to delayed emergence and a slower growth rate. In contrast, DM production of subterranean clover plateaued at the warmest temperature, and that of lucerne increased progressively with increasing temperature (McWilliam et al. 1970).
A likely factor for the greater growth rates of burr medic, lucerne, and subterranean clover is their ability to initiate leaf development faster than serradella (Marañón and Grubb 1993), providing a competitive advantage because energy production through photosynthesis (beyond what is produced by cotyledons) can commence earlier. No leaves appeared (i.e. >50% of plants with fully open first leaf) within 21 days in the serradella species groups at the two coolest temperatures (10/5°C and 15/10°C), whereas all reference species had at least the first (unifoliate) leaf by 16–19 days at 15/10°C. Within the optimum temperature treatment for serradella (20/15°C), first leaf development occurred approximately 14 days after sowing, with subterranean clover producing its first trifoliate leaf (in addition to the unifoliate leaf) within this time. The superior growth rates of reference species at warm temperatures resulted in up to three trifoliate leaves (in addition to the unifoliate leaf) within 21 days, likely providing a competitive advantage over serradella in warm environments not limited by water availability.
Seed reserves
Large seeds (with greater nutrient reserves) can improve early pasture productivity (Kidd and West 1919; Black 1956). In our study, the differences in early shoot DM production (up to 21 days) positively correlated with seed N, with a stronger relationship at warmer temperatures (Fig. 4). Serradella cultivars had minimal variation in seed size and nutrient reserves (Table 1). For this reason, coupled with low variation in germination rates at cool temperatures (Young et al. 1970), it is unlikely that serradella species can be improved in this regard.
Relative growth rates
In Experiment 2, serradella cultivars generally had lower RGR than lucerne and subterranean clover beyond the first 21 days of development when grown in cool conditions (15/10°C). However, the raw shoot DM values (Table S2) indicate no significant differences between cultivars 42 days after sowing; it was only at the final sampling time (day 49) that lucerne had significantly greater DM than serradella and subterranean clover. Under warmer conditions (23/18°C), lucerne had far greater RGR and overall DM production than any other species, with no difference between serradella and subterranean clover.
Shoot RGR for all species was greatest at 23/18°C, but the rate of DM increase (indicated by variation in slopes in Fig. 6) for lucerne and subterranean clover differed among the temperature treatments. For lucerne, differences in RGR at the two temperatures increased over time, while the opposite was true for subterranean clover, which had a similar RGR for both temperature treatments by 49 days of growth. There are two possible explanations for this observation. The first might be associated with nutrient limitations or pot space restrictions, with the faster plant growth rates at 23/18°C exhausting nutrient reserves faster than at 15/10°C. The second relates to full canopy closure and a potential reduction in light interception occurring earlier at 23/18°C than 15/10°C. Light interception by the plants mimicked growth in a pasture sward due to the use of reflective sleeves raised regularly to just below canopy height. Once each micro-sward reaches canopy closure, the RGR should decrease due to the shading of lower leaves. However, due to differences in leaf architecture among the three species, full canopy closure only occurred for subterranean clover, which may explain the overlap of shoot RGR in the two temperature treatments.
The greatest negative impact on DM production (i.e. greatest disparity between RGR) at cool temperatures was for lucerne, primarily because its potential DM productivity at warm temperatures is much greater than the other species. Our results also revealed that cool temperatures limited the productivity of the tested reference species, not just serradella, a notion supported by the lack of variability in winter growth rates recorded among pasture species (Saul and Sargeant 2013). The anecdotal observations of poor growth rates by serradella under cool conditions may be due to other co-occurring factors, such as a lack of waterlogging tolerance (Kidd et al. 2020) or soil-borne disease pressure under the cool, wet conditions of autumn–winter.
Leaf physiology
Despite the lack of water stress in Experiment 2, there was no significant change in stomatal conductance or photosynthetic rate for Ornithopus spp. While such an adaptive response may limit the early season productive potential of Ornithopus spp., it may also slow soil water usage, allowing plants to survive longer into the growing season (when temperatures rise and soil moisture rapidly declines) than species that do not have such stomatal adjustments. In the semi-arid regions of Western Australia, where Ornithopus spp. are used widely, this adaptive response allows them to survive and set seed in autumn–spring growing seasons, which are becoming increasingly drier and of shorter duration (Asseng and Pannell 2013).
Conclusion
Serradella species generally had similar, or better germination rates than the reference temperate pasture legumes at all temperature treatments examined in this study, but their ability to emerge from the soil was delayed in warmer conditions (i.e. >25°C). At warmer temperatures, serradella had slower post-emergent growth rates and leaf development than subterranean clover and lucerne; this inability to fully capitalise on warmer growing conditions could adversely affect their competitiveness during the establishment phase of early season pasture growth. The inability of serradella to increase their photosynthetic rates at warm temperatures could be commensurate with adaptation to high irradiance and drought but may conceivably limit their RGR in warm environments when not limited by water availability. At cool temperatures (i.e. <20°C), serradella emergence and DM productivity did not significantly differ from the highly successful pasture legumes used as reference species; lucerne, burr medic and subterranean clover. We conclude that cool temperatures should not inhibit the establishment of serradella and other factors should be investigated when poor early growth occurs in cool environments.
Data availability
The data supporting the findings of this study are available from the corresponding author (Daniel Kidd) upon reasonable request.
Declaration of funding
This work was supported by funding from the Australian Government Department of Agriculture, Fisheries and Forestry as part of its Rural R&D for Profit program and from Meat and Livestock Australia (MLA), Dairy Australia and Australian Wool Innovation (AWI) (RnD4P-15-02-016 Phosphorus Efficient Pastures). This work was also supported by an Australian Endeavour fellowship grant to Marzieh Valifard for The University of Western Australia to collaborate with Shiraz University, Iran.
Author contributions
Daniel Kidd: conceptualisation, investigation, methodology, formal analysis, visualisation, writing – original draft preparation. Joanne Wisdom: formal analysis, visualisation, writing – review and editing. Juan Qi: investigation, writing – review and editing. Richard Simpson: conceptualisation, funding acquisition, methodology, writing – review and editing. Megan Ryan: methodology, project administration, supervision, writing – review and editing. Marzieh Valifard – conceptualisation, investigation, methodology, writing – review and editing.
Acknowledgements
We thank University staff and students for their help with collecting the data, software training and data interpretation. In particular, we thank Greg Cawthray, Jiayin Pang, Evonne Walker, Joshua Magee and Christian Goh for their important contributions.
References
Asseng S, Pannell DJ (2013) Adapting dryland agriculture to climate change: farming implications and research and development needs in Western Australia. Climatic Change 118(2), 167-181.
| Crossref | Google Scholar |
Baker F, Turner G, Court J, Hunter B (2014) Fill the winter feed gap. Available at https://www.evergraze.com.au/library-content/fill-the-winter-feed-gap/ [Accessed 20 November 2017]
Black JN (1956) The influence of seed size and depth of sowing on pre-emergence and early vegetative growth of subterranean clover (Trifolium subterraneum L.). Australian Journal of Agricultural Research 7(2), 98-109.
| Crossref | Google Scholar |
Black JN (1957) Seed size as a factor in the growth of subterranean clover (Trifolium subterraneum L.) under spaced and sward conditions. Australian Journal of Agricultural Research 8(4), 335-351.
| Crossref | Google Scholar |
Butler TJ, Celen AE, Webb SL, Krstic D, Interrante SM (2014) Temperature affects the germination of forage legume seeds. Crop Science 54(6), 2846-2853.
| Crossref | Google Scholar |
Chen D, Chen X, Wang J, Zhang Z, Wang Y, Jia C, Hu X (2021) Estimation of thermal time model parameters for seed germination in 15 species: the importance of distribution function. Seed Science Research 31, 83-90.
| Crossref | Google Scholar |
Covell S, Ellis RH, Roberts EH, Summerfield RJ (1986) The influence of temperature on seed germination rate in grain legumes. A comparison of chickpea, lentil, soyabean and cowpea at constant temperatures. Journal of Experimental Botany 37(5), 705-715.
| Crossref | Google Scholar |
Dear BS, Ewing MA (2008) The search for new pasture plants to achieve more sustainable production systems in southern Australia. Australian Journal of Experimental Agriculture 48(4), 387-396.
| Crossref | Google Scholar |
Dear BS, Wilson BCD, Rodham CA, McCaskie P, Sandral GA (2002) Productivity and persistence of Trifolium hirtum, T. michelianum, T. glanduliferum and Ornithopus sativus sown as monocultures or in mixtures with T. subterraneum in the south-eastern Australian wheat belt. Australian Journal of Experimental Agriculture 42(5), 549-556.
| Crossref | Google Scholar |
Dumas JBA (1831) Methods of organic analysis. Annals of Chemistry and Physics 47, 198-205.
| Google Scholar |
Eze MO, Hose GC, George SC (2020) Assessing the effect of diesel fuel on the seed viability and germination of Medicago sativa using the event-time model. Plants 9(9), 1062 PMID:.
| Crossref | Google Scholar | PubMed |
Fick SE, Hijmans RJ (2017) WorldClim 2: new 1-km spatial resolution climate surfaces for global land areas. International Journal of Climatology 37(12), 4302-4315.
| Crossref | Google Scholar |
Finch-Savage WE, Clay HA, Lynn JR, Morris K (2010) Towards a genetic understanding of seed vigour in small-seeded crops using natural variation in Brassica oleracea. Plant Science 179(6), 582-589.
| Crossref | Google Scholar |
Fu SM, Hampton JG, Williams WM (1994) Description and evaluation of serradella (Ornithopus L.) accessions. New Zealand Journal of Agricultural Research 37(4), 471-479.
| Crossref | Google Scholar |
Fukai S, Silsbury JH (1976) Responses of subterranean clover communities to temperature. I. Dry matter production and plant morphogenesis. Functional Plant Biology 3(4), 527-543.
| Crossref | Google Scholar |
Hill MJ (1996) Potential adaptation zones for temperate pasture species as constrained by climate: a knowledge-based logical modelling approach. Australian Journal of Agricultural Research 47(7), 1095-1117.
| Crossref | Google Scholar |
Hill MJ, Luck R (1991) The effect of temperature on germination and seedling growth of temperate perennial pasture legumes. Australian Journal of Agricultural Research 42(1), 175-189.
| Crossref | Google Scholar |
Hoveland CS, Elkins DM (1965) Germination response of arrowleaf, Bail, and Crimson clover varieties to temperature. Crop Science 5(3), 244-246.
| Crossref | Google Scholar |
Jeffery RP, Simpson RJ, Lambers H, Kidd DR, Ryan MH (2017) Plants in constrained canopy micro-swards compensate for decreased root biomass and soil exploration with increased amounts of rhizosphere carboxylates. Functional Plant Biology 44(5), 552-562 PMID:.
| Crossref | Google Scholar | PubMed |
Kidd F, West C (1919) Physiological pre-determination: the influence of the physiological condition of the seed upon the course of subsequent growth and upon the yield. Annals of Applied Biology 6(1), 1-26.
| Crossref | Google Scholar |
Kidd DR, Di Bella CE, Kotula L, Colmer TD, Ryan MH, Striker GG (2020) Defining the waterlogging tolerance of Ornithopus spp. for the temperate pasture zone of southern Australia. Crop & Pasture Science 71, 506-516.
| Crossref | Google Scholar |
Klinkowski M, Schwarz O (1938) Area formation and systematic position of the cultivated and wild serradella. The Breeder 10(2), 43-51.
| Google Scholar |
Lonati M, Moot DJ, Aceto P, Cavallero A, Lucas RJ (2009) Thermal time requirements for germination, emergence and seedling development of adventive legume and grass species. New Zealand Journal of Agricultural Research 52(1), 17-29.
| Crossref | Google Scholar |
Marañón T, Grubb PJ (1993) Physiological basis and ecological significance of the seed size and relative growth rate relationship in Mediterranean annuals. Functional Ecology 7(5), 591-599.
| Crossref | Google Scholar |
McNair JN, Sunkara A, Frobish D (2012) How to analyse seed germination data using statistical time-to-event analysis: non-parametric and semi-parametric methods. Seed Science Research 22(2), 77-95.
| Crossref | Google Scholar |
McWilliam JR, Clements RJ, Dowling PM (1970) Some factors influencing the germination and early seedling development of pasture plants. Australian Journal of Agricultural Research 21(1), 19-32.
| Crossref | Google Scholar |
Moot DJ, Scott WR, Roy AM, Nicholls AC (2000) Base temperature and thermal time requirements for germination and emergence of temperate pasture species. New Zealand Journal of Agricultural Research 43(1), 15-25.
| Crossref | Google Scholar |
Motomizu S, Wakimoto T, Tôei K (1983) Spectrophotometric determination of phosphate in river waters with molybdate and malachite green. Analyst 108(1284), 361-367.
| Crossref | Google Scholar |
Nichols PGH, Loi A, Nutt BJ, Evans PM, Craig AD, Pengelly BC, Dear BS, Lloyd DL, Revell CK, Nair RM, Ewing MA, Howieson JG, Auricht GA, Howie JH, Sandral GA, Carr SJ, de Koning CT, Hackney BF, Crocker GJ, Snowball R, Hughes SJ, Hall EJ, Foster KJ, Skinner PW, Barbetti MJ, You MP (2007) New annual and short-lived perennial pasture legumes for Australian agriculture—15 years of revolution. Field Crops Research 104(1–3), 10-23.
| Crossref | Google Scholar |
Nori H, Moot DJ, Black AD (2014) Thermal time requirements for germination of four annual clover species. New Zealand Journal of Agricultural Research 57(1), 30-37.
| Crossref | Google Scholar |
Revell CK, Taylor GB, Cocks PS (1998) Long-term softening of surface and buried hard seeds of yellow serradella grown in a range of environments. Australian Journal of Agricultural Research 49(4), 673-686.
| Crossref | Google Scholar |
Richards RA, Rebetzke GJ, Condon AG, van Herwaarden AF (2002) Breeding opportunities for increasing the efficiency of water use and crop yield in temperate cereals. Crop Science 42(1), 111-121 PMID:.
| Crossref | Google Scholar | PubMed |
Romano A, Stevanato P (2020) Germination data analysis by time-to-event approaches. Plants 9(5), 617 PMID:.
| Crossref | Google Scholar | PubMed |
Rossiter RC, Collins WJ, Klein L (1985) Winter growth and nutritive quality of serradella (Ornithopus spp.). Australian Journal of Experimental Agriculture 25(2), 362-366.
| Crossref | Google Scholar |
Saul G, Sargeant K (2013) Regional pasture growth rates. EverGraze. Available at https://www.evergraze.com.au/library-content/regional-pasture-growth-rates/index.html [Accessed 4 July 2022]
Simpson RJ, Richardson AE, Riley IT, McKay AC, McKay SF, Ballard RA, Ophel-Keller K, Hartley D, O’Rourke TA, Li H, Sivasithamparam K, Ryan MH, Barbetti MJ (2011) Damage to roots of Trifolium subterraneum L. (subterranean clover), failure of seedlings to establish and the presence of root pathogens during autumn–winter. Grass and Forage Science 66(4), 585-605.
| Crossref | Google Scholar |
Young JA, Evans RA, Kay BL (1970) Germination characteristics of range legumes. Journal of Range Management Archives 23(2), 98-103.
| Crossref | Google Scholar |
Zhang J, Hu L, Redden B, Yan G (2015) Identification of fast and slow germination accessions of Brassica napus L. for genetic studies and breeding for early vigour. Crop & Pasture Science 66(5), 481-491.
| Crossref | Google Scholar |