Importance of circadian rhythms in dairy nutrition
Kevin J. Harvatine
A Department of Animal Science, Penn State University, University Park, PA, USA.
Abstract
Biological rhythms are repeating patterns that are driven by time-keeping mechanisms within the animal and are adaptive as they coordinate physiology and metabolism with the external environment. The dairy cow has a well recognised natural daily pattern of feed intake and milk synthesis, but regulation of these rhythms has not been well described in the literature or well considered in current dairy management. Recent discoveries have clearly described circadian time-keeping mechanisms in peripheral tissues that are responsive to the timing of food availability. Some management strategies on dairy farms may desynchronise the interactions between central and mammary circadian timekeepers, resulting in reduced milk yield and efficiency. Feeding a total mixed ration is commonly assumed to create constant ruminal conditions, but the large variation in the rate of feed intake across the day causes large fluctuations in rumen fermentation and absorbed nutrients. Milk composition also differs across the day due to both dynamics in nutrient absorption and biological regulation attempting to match milk yield and composition with calf requirements across the day. Recent work has shown that milk synthesis varies over the day and is modified by the timing of feed intake and nutrient absorption. These rhythms have also been shown to be affected by the timing of feed delivery. We expect that maximal milk yield and efficiency are achieved when we have more consistent rumen fermentation and match the timing of nutrient absorption and mammary capacity for milk synthesis. Managing feeding times provides the opportunity to modify feed intake across the day, but behavioural responses are complex. Appreciating the impact of circadian rhythms provides the foundation to develop nutrition and management strategies considering circadian dynamics of intake and milk synthesis and provides opportunities for new gains in cow efficiency, welfare, and health.
Keywords: chrononutrition, daily pattern, diurnal, feeding behaviour, rumen fermentation, rumination, synchrony.
Introduction
Feed represents the largest and most variable cost for dairy producers. The goal of nutrition is not to simply minimise feed cost, but also to optimise conversion of feed into milk. Improvements in production and efficiency of dairy cows are attributable to advances in genetics, nutrition, and management. Dilution of maintenance has been one of the most powerful tools for improving efficiency and, as milk yield increases, energy intake must also increase (Bauman et al. 1985). The ability to feed highly fermentable feedstuffs that support high levels of milk yield while maintaining normal rumen function and fermentation has been central to success, as decreases in fibre digestibility and microbial protein synthesis or induction of diet-induced milk fat depression would counter the benefits of dilution of maintenance. Adoption of total mixed rations (TMR) and partial mixed rations (PMR) that spread highly fermentable feeds across more meals per day have been key to this effort (Schingoethe 2017). However, ruminal fermentation of cows in these systems is not constant because of variation in feed consumption across the day (Salfer et al. 2018), which results in more fermentable substrate in the rumen during the high intake period of the day. We have proposed that appreciation of these daily rhythms may allow optimisation of rumen fermentation and, subsequently, milk synthesis (e.g. Rottman et al. 2015) and this review will outline the support for this concept and key opportunities.
In animal nutrition, feed intake, milk production, and growth are reported and discussed on a ‘per-day’ basis. This is undertaken because we recognise that these vary over the day, making ‘a day’ a biologically meaningful unit. Nutritionists stoichiometrically balance daily nutrient intake and requirements in ration formulation. Researchers observe milk yield and composition at all milkings across a day, but rarely report them individually. In digestion studies, rumen digesta, duodenal digesta, and faeces are also sampled multiple times per day. Blood samples are collected multiple times per day since metabolites and metabolic hormones clearly vary across the day. Obviously, dairy nutritionists and researchers appreciate the dynamic nature of feeding behaviour, digestion, nutrient absorption, and metabolism, but little effort has been directed toward understanding the regulation of the changes within a day.
Rather than simply responding to an environmental stimulus, endogenous timekeepers allow the animal to anticipate daily environmental changes before they occur (Bass and Takahashi 2010). These daily or ‘circadian’ timekeepers create rhythms that drive adaptive changes in behaviour and physiological processes. Two important aspects are that the timing of the rhythms are set or ‘entrained’ by environmental signals, such as light–dark cycles, and that the rhythms will persist if the animal is held under constant conditions. It is very important to appreciate that these rhythms are adaptive and improve efficiency and survival. They allow prediction of the timing of food availability and predator risk and pro-actively drive changes that prepare for these future environmental changes. They also allow coordination of complementary processes across organ systems. Since feeding behaviour and feed intake are influenced by circadian rhythms, it is easy to appreciate that metabolism would also be controlled by timekeepers aiming to proactively adjust metabolic pathways with timing in nutrient absorption.
A full discussion of the impact of circadian rhythms in the dairy cow requires the integration of feeding behaviour, rumen fermentation, post-absorptive metabolism, and endocrine regulation, and is beyond the scope of what can be covered in a single review. There are numerous high-quality reviews of the fundamental biology of circadian rhythms that are conserved across species, with many focusing on implications for metabolism (e.g. Litichevskiy and Thaiss 2022; Sinturel et al. 2022; Taleb and Karpowicz 2022; Chamorro et al. 2023). Casey, Boerman, and Plaut at Purdue University have extensively studied the role of circadian rhythms in mammary gland development in the cow and have published very complete reviews focusing on lactational biology, endocrinology, and reproduction (e.g. Casey and Plaut 2012, 2022; Plaut and Casey 2012; Suárez-Trujillo and Casey 2016). There is also a recent review by Li et al. (2021) focusing on the implication of circadian rhythms for health and disease in the cow. These topics will be briefly integrated below, but the reader is directed to these reviews for a complete discussion.
Recent discoveries have clearly described circadian time-keeping mechanisms in metabolically important peripheral tissues (e.g. adipose and liver) that are responsive to environmental factors such as timing of food availability. Interestingly, in many experimental models, the timing of food intake can alter the synchronisation between the central master timekeeper and peripheral clocks, resulting in development of numerous disorders, including obesity, insulin resistance, and metabolic diseases (Takahashi et al. 2008). We expect that the circadian rhythms of intake and milk synthesis are primarily regulated by the light–dark cycle, the timing of feeding, milking, and the daily rhythm of nutrient absorption and interactions among these factors (Fig. 1). This complicates the discussion, but also allows the opportunity to better understand and integrate behaviour, rumen function, and metabolism.
Light–dark cycles, milking, and feeding are activities that occur daily and may entrain a circadian rhythm of milk synthesis through modification of biological clocks and dynamics in nutrient availability.
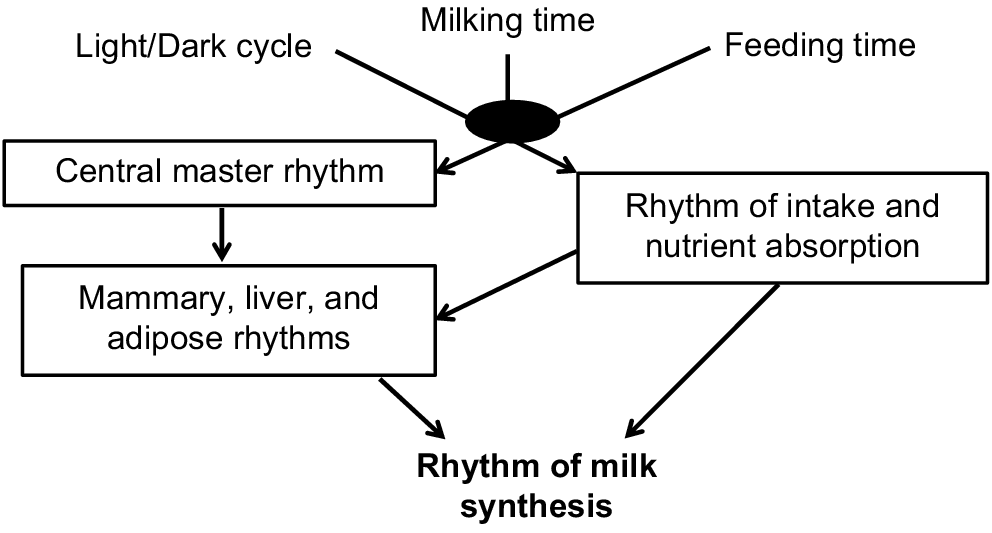
This review will focus on the daily pattern of feed intake and understanding the impact of the timing of feed intake on rumen function and milk synthesis that have implications for dairy nutrition. The central concept is that there is a daily pattern of feed intake that creates dynamics in rumen fermentation and nutrient absorption and that there is also a daily pattern in the capacity for milk synthesis. We propose that decreasing variation in intake will improve rumen fermentation and that temporally matching nutrient absorption and mammary demands will allow maximal milk yield and efficiency (Fig. 2). If the rhythm of nutrient absorption is not synchronised with the rhythm of milk synthesis, we expect milk yield to suffer as the demand for nutrients may not be met for part of the day and efficiency will decrease as excess nutrients during other parts of the day are partitioned to other tissues.
Defining circadian rhythms
Circadian rhythms refer to ~24-h endogenously generated repeating cycles found in most tissues of virtually all living organisms (from bacteria to mammals) and have been the subject of intensive investigation in humans and basic science animal models over the past two decades. A quick summary of reviews indexed in Pubmed with ‘circadian’ in their title shows an increase from less than 50 per year in the 1990s to over 250 per year in recent years (Fig. 3). Although the central circadian timekeeper was discovered in 1972, the increased interest in circadian rhythms occurred after the discovery of the independent timekeepers in peripheral tissues in 1998 and their subsequent roles in physiology, metabolism, and many diseases (Finger and Kramer 2021).
Circadian rhythms fit a cosine function and can vary in their amplitude, period (length of cycle), or phase (time of peak). They are created by endogenous timekeeping mechanisms and their endogenous rhythms are slightly longer than 24 h and are reset or ‘entrained’ each day by ‘zeitbergers’ (translates to timekeepers). It is important to distinguish between a ‘daily’ and ‘circadian’ rhythm. In general, any variable with a consistent repeating pattern can be called a daily pattern, but may or may not be a circadian rhythm. Since circadian rhythms are under the control of endogenous timekeepers, circadian rhythms continue when the animal is held under constant conditions, for example, constant darkness and no repeating daily activities including feed delivery. Without daily entrainment, the rhythm will be ‘free running’, where a cycle slightly greater than 24 h is maintained. Some daily rhythms may simply be secondary responses to the circadian rhythm and not under primary control by an endogenous timekeeper. For example, hunger and, subsequently, feed intake follow a circadian rhythm that causes changes in plasma metabolites across the day because nutrient absorption follows feed intake. In the absence of the feeding pattern, the plasma metabolites may not have a rhythm. However, the daily rhythm in nutrient metabolism by a tissue may be because of changes in plasma concentration and mass action kinetics or may be driven by a circadian rhythm where the cell’s timekeeper controls expression and activity of metabolic enzymes. It becomes even more complicated as a daily pattern of nutrient absorption may actually be a signal that entrains circadian rhythms in peripheral tissues such as the mammary gland. It is very hard to experimentally create constant conditions with no entraining factor, such as feeding or milking, when conducting experiments with lactating cows. The differentiation between circadian and daily rhythms is important when determining the correct intervention to modify the rhythm. For example, we have explored the timing of feed delivery as a primary mechanism to entrain the circadian pattern of feed intake that then drives daily rhythms of rumen fermentation and nutrient absorption. It is also important to appreciate daily rhythm and the implications they have on metabolism. For example, a goal may be to decrease the variation in rumen ammonia across the day to increase nitrogen efficiency.
Importance of circadian rhythms
Anyone who has flown across multiple time zones, lost a night of sleep, or even just changed clocks for daylight savings time, appreciates the influence of circadian rhythms on physiology. Their importance is also strongly supported by scientific evidence. Circadian rhythms have been shown to affect physiological, immunological, and psychological health, and disrupted rhythms have been implicated in numerous diseases. The effect of night shift work has been extensively investigated using epidemiological approaches and related to increases in overall morbidity and mortality and incidence of diseases from obesity and cardiovascular diseases to mood disorders and other mental health conditions to breast cancer (Hansen 2017; Jin et al. 2017; Strohmaier et al. 2018; Walker et al. 2020). Metabolic diseases during night shift work are thought to arise from a dys-synchrony of the circadian clock in the brain that is mainly entrained by lighting and peripheral clocks in the liver and adipose tissue that can also be entrained to other environmental cues, such as timing of food intake. Allowing mice to only eat during the day when their natural feeding pattern is to eat at night increases obesity and insulin resistance (Asher and Schibler 2011; Adamovich et al. 2014). There is also a circadian rhythm in many components of immune responses and immune cells and lymphoid organs have endogenous biological clocks that drive daily rhythms (reviewed by Cermakian et al. (2013), Evans and Davidson (2013), and Golombek et al. (2013)). These daily rhythms drive changes in susceptibility to infection and disease and presentation of clinical symptoms and are likely to be adaptive because the response to bacterial challenge increases during the active period of the day. From a nutritional perspective, the circadian pattern of feed intake and rhythms in nutrient metabolism have the most direct impact on rumen fermentation and milk yield and are the focus of the discussion below.
Synchronisation of central and peripheral circadian rhythms
The circadian pacemaker is in the suprachiasmatic nucleus (SCN) in the brain. Independent, endogenous biological clocks have been identified in peripheral tissues, including liver, mammary, and adipose tissue (see reviews Dibner et al. 2010, Doherty and Kay 2010, and Asher and Schibler 2011). Peripheral rhythms are normally synchronised with the SCN through its rhythmic regulation of neural or hormonal signals (see review Dibner et al. 2010). Melatonin is the most well described, but rhythmic secretion of prolactin, glucocorticoids, insulin-like growth factor-1, thyroid hormones, and growth hormone may also have roles. Thus, these signals from the SCN serve to entrain the peripheral clocks and normally synchronise central and peripheral clocks. However, peripheral clocks are also directly responsive to entrainment of the timing of nutrient absorption and do not have robust feedback to the central clock.
Molecular clockwork
Memorising the components of the molecular clock is not needed to understand the impact of circadian rhythms in dairy nutrition, but a brief introduction will highlight that the system has been described in great detail (reviewed by Dibner et al. (2010), Mohawk et al. (2012)). Mammalian circadian clocks include a network of transcription factors that are referred to as the ‘core clock’ genes. These include Period 1 and 2 (PER1, PER2), brain-muscle-arnt-like1 (BMAL1), cryptochrome 1 and 2 (CRY1, CRY2), and the CLOCK protein. The transcription and translation of these ‘clock genes’ are rhythmic and their collective output regulates the timing of circadian rhythms at the cellular level. Briefly, CLOCK and BMAL are the ‘positive arm’ of the circadian molecular clockwork and drive expression of PER and CRY genes. PER and CRY proteins are the ‘negative arm’ of the molecular clock and inhibit CLOCK/BMAL. RAR-related orphan receptor A (RORα) and REV-ERBalpha and beta (REV-ERBα&β) are nuclear hormone receptor family proteins that are also involved in accessory feedback loops. These clock genes bind to the promoters of many genes, including metabolic enzymes, thus providing their impact on cell physiology. Mechanisms beyond this transcription–translation feedback loop have been described including post-transcriptional and post-translational modifications and the reader is directed to the many great reviews in the area for additional reading. Importantly, expression and functional activity of the core clock genes have been demonstrated in mammary cells (e.g. Casey et al. 2016, 2021) and the bovine mammary gland (Salfer and Harvatine 2018). Additionally, work in model organisms has demonstrated feed intake as an input signal and provides a direct link from the clock to regulation of nutrient metabolism.
Nutritional entrainment of peripheral tissues
The light–dark cycle is the main entrainer of the central clock in the SCN, but it is well established in animal models that the timing of food availability can entrain circadian rhythms without affecting the timing of the biological clock in the SCN (Hara et al. 2001; Stokkan et al. 2001; Escobar et al. 2009). The effect of diet entrainment of peripheral tissues has been a prolific area of research because it provides the basis for dys-synchrony of peripheral tissues from the central rhythm that is thought to contribute to many chronic diseases (see reviews Bass and Takahashi 2010, Dibner et al. 2010, Asher and Schibler 2011, and Sinturel et al. 2022). The bioactive compounds and nutrient sensors that entrain the clock are not clear, but may involve NAD/NADH ratios (Rutter 2001; Isobe et al. 2011), free radicals (Boehning and Snyder 2002), and specific metabolites, including lipids through PPARγ activation (Rutter et al. 2002; Nakahata et al. 2009; Ramsey et al. 2009). With constant feed availability in most dairy management systems, there is a large influence of the circadian pattern of hunger on the timing of feed intake, and thus nutrient absorption is expected to be in synchrony with the central clock. However, limiting the timing of feed availability, specific feeding times, or timing of supplementation provides the opportunity for entrainment by nutrient absorption. There are multiple applications and goals. First, since different physiological processes, such as intake and milk synthesis, may differ in their major entrainers, a first principle goal is to synchronise these rhythms through manipulating entrainment factors. It is important to highlight that some entrainers, such as daylight, are dynamic and outside of our control and may require specific management to maintain synchrony. Second, there may be an advantage in decreasing the amplitude of some daily patterns. For example, the impact of changes in feed intake on rumen fermentation and nutrient absorption are discussed below.
Evidence of daily rhythms in the dairy cow
Many physiological variables in the cow are known to follow a daily pattern. For example, plasma glucose, non-esterified fatty acids (NEFA), blood urea nitrogen (BUN), and insulin all have well documented daily patterns (Lefcourt et al. 1999; Giannetto and Piccione 2009). Body temperature is considered a good indication of the timing of the central clock and Giannetto and Piccione (2009) reported that body temperature was highly rhythmic in the cow (robustness = 0.975). Outside of heat-stress conditions, the circadian pattern of body temperature is not directly related to environmental temperatures (Verwoerd et al. 2006). Disruption of light–dark cycles prepartum modified circadian rhythms of multiple plasma metabolites, melatonin, and body temperature and affected milk yield postpartum through modification of mammary development (Suarez-Trujillo et al. 2020, 2022; McCabe et al. 2021). We have also recently observed that plasma NEFA, BUN, and insulin and core body temperature are modified by the timing of feeding and timing of protein and acetate infusion (Niu et al. 2014; Salfer and Harvatine 2020; Matamoros et al. 2021). It is difficult to say whether these daily patterns are the result of circadian clocks or simply due to the daily pattern of nutrient absorption and subsequent metabolism.
Circadian pattern of intake
Discussion of the circadian rhythm of rumen fermentation and metabolism must be integrated with consideration of the pattern of feed intake and nutrient absorption. Feeding behaviour is centrally regulated through the integration of many factors, including hunger, satiety, physiological state, environment, and endogenous circadian rhythms (Reviewed by Allen et al. (2005), von Keyserlingk and Weary (2010)). The dairy cow has a preferred feeding rhythm, but must also consume a large amount of feed to meet energy demands and adapt to feeding and milking times selected by farm management.
The feeding pattern of grazing cows is commonly described as ‘crepuscular’, with a larger proportion of intake being consumed at dawn and dusk (reviewed by Albright 1993). Feeding behaviour of TMR-fed cows has also been extensively studied using multiple automated observation systems and has a daily pattern with the greatest intake in the afternoon and early evening and lower intake during the overnight period (e.g. Yang and Beauchemin 2006; DeVries et al. 2007; Hosseinkhani et al. 2008). These analyses clearly show different phases of feed intake over the day, but intake is also influenced by other factors, with delivery of fresh feed being the strongest stimulus (DeVries et al. 2005). Controlled experiments have shown a much smaller effect of feed pushup, although a larger effect is expected in commercial settings when feed is more commonly out of reach. Both feeding and push-up stimulation diminish with an increasing frequency. Additionally, there was little effect of fibre and starch concentration and digestibility, or fat level and FA saturation on the daily pattern of feed intake and rumination (Salfer et al. 2018). Variation in the daily pattern of intake between individual cows is often noticed while managing individual animals, but has not been well characterised and is ripe for future work.
The daily pattern of intake in high-producing cows and the effect of feeding time is well illustrated in an experiment where we fed cows once per day at 0830 hours or 2030 hours (Niu et al. 2014; Fig. 4). Over 20% and 34% of daily intake was consumed in the 2 h after feeding in cows fed at 0830 hours and 2030 hours respectively. The intake rate at other times of day did not differ greatly, with both groups having lower intake overnight and higher intake in the afternoon. The feeding pattern was replicated in a second experiment conducted in the summer (Niu and Harvatine 2018a) and by others (Nikkhah et al. 2008). A common perception is that cows consume more feed during the day because that is when it is the freshest. Feed delivery obviously is a strong stimulus of feeding for 1–2 h. However, it is interesting to note that cows fed in the evening had low intake during the overnight (not different from morning-fed cows) and waited until the following afternoon when feed was over 16 h old to increase intake to twice that of the overnight period. This highlights that cows have a strong natural drive to consume feed during the afternoon and early evening. This may be a conserved adaptation as pasture forages would be highest in sugar and amino acids in the afternoon and predator risk is likely to be lowest. It is also interesting to note that the conditioned eating occurring in the 2 h after feed delivery was consistently ~50% greater when cows were fed in the evening than when they were fed in the morning. This may be due to increased hunger and compensatory intake before the overnight resting period. Regardless of the mechanism, this slug eating increases risks for disrupted rumen fermentation and milk fat depression.
Circadian pattern of the rate of feed intake, plasma insulin, and faecal neutral detergent fibre (NDF) concentration in cows fed once per day at 0800 hours or 2000 hours. Treatment differences at each time point are shown for insulin and faecal NDF (*P < 0.05, **P < 0.01). Originally published in Niu et al. (2014).
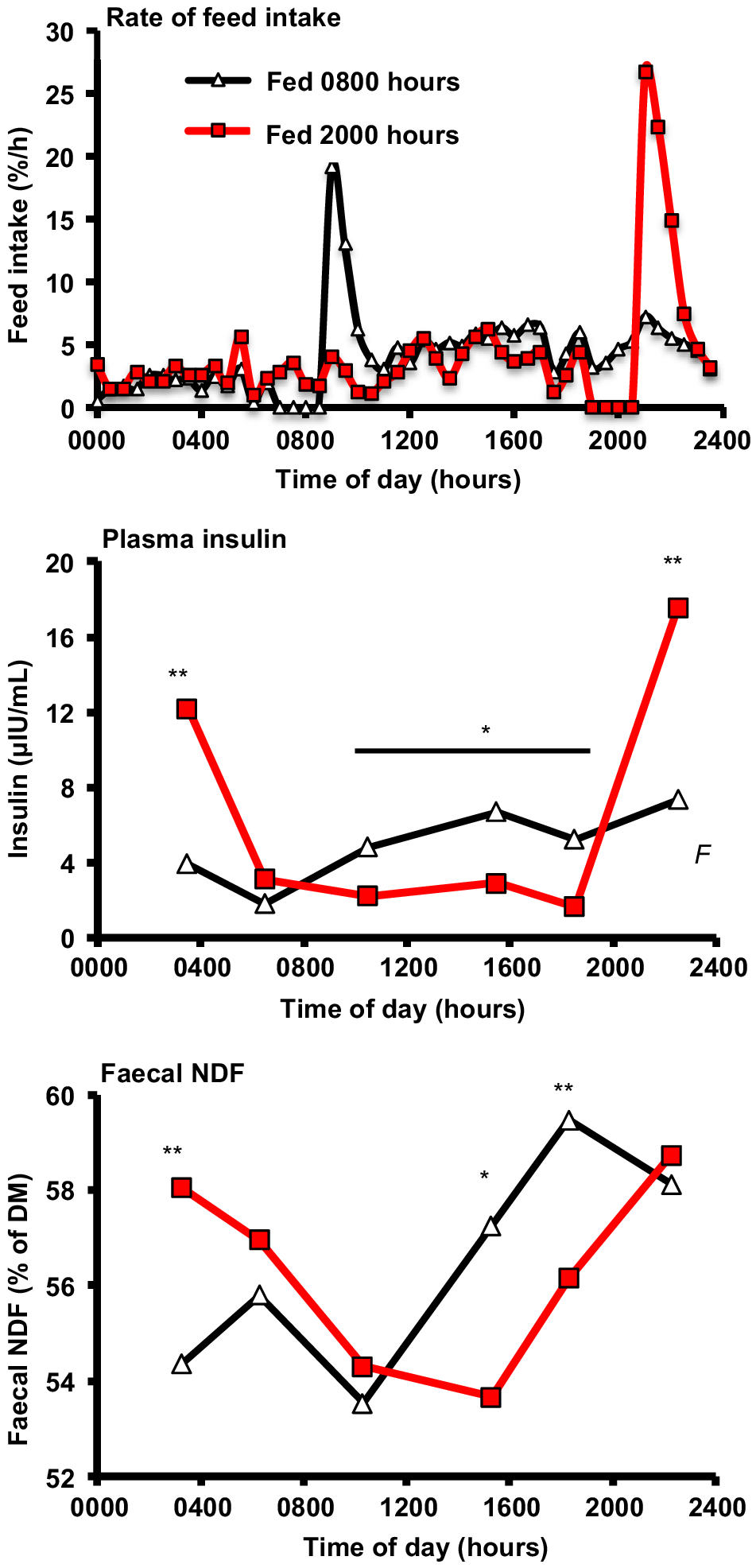
Physiological significance of the circadian pattern of intake
The ruminant has a more consistent absorption of nutrients over the day because of the larger number of meals (6–12 per day), the size of the rumen, and the slow rate of ruminal digestion and passage. However, even when feeding a TMR, the circadian pattern of feeding results in a large difference in the amount of fermentable substrate entering the rumen over the day.
Rumen pH provides the highest-resolution marker of rumen fermentation, and a daily pattern of rumen pH is very repeatable, with a nadir approximately 10 h after initial feed offering (e.g. Yang and Beauchemin 2006; Harvatine 2012; Salfer et al. 2018). A daily pattern to rumen volatile fatty acids (VFA) and digesta composition is also well established (e.g. Maulfair et al. 2011). Additionally, we have observed that ruminal starch and NDF concentration fit a cosine function with a 24 h period (Ying et al. 2015). Changes in rumen-digesta pool size across the day are normally not reported, but Salfer et al. (2018) reported that ruminal digesta weight was ~25% greater after feeding and starch pool size 50% to >100% greater than before feeding. Ying et al. (2015) also reported a three-fold increase in rumen starch pool 6 h after feeding. Rumen microbial populations are dynamic across the day in response to these changes in substrate availability and rumen environment (Salfer et al. 2021). This change in microbial populations also caused a rhythm in amylase, lichenase, endoglucanase, and xylanase enzymatic capacity (Raffrenato et al. 2022). Changes in the rate and composition of duodenal flow are not known, but a daily rhythm has also been reported for faecal particle size, NDF, iNDF, and starch concentration and is likely to indicate dynamic changes in rumen outflow (Maulfair et al. 2011). The daily pattern of faecal NDF and iNDF was also dependent on the time of feeding (Niu et al. 2014; Niu and Harvatine 2018a; Fig. 4). It is clear that TMR feeding does not create constant rumen or post-rumen conditions and has implications for optimisation of rumen fermentation and the timing of nutrient absorption that affects mammary metabolism. This highlights that it is not just TMR composition and mixing that is important, but feeding behaviour must also be considered and has not been commonly observed.
It is interesting to consider the relevance of the idea of ‘nutrient synchrony’ that has been discussed for decades in ruminant nutrition and focuses on matching rates of fermentation of carbohydrate and protein fractions. Although the concept is logical and insightful for integrating concepts of digestion and fermentation, it has been difficult to demonstrate benefits in vivo (Hall and Huntington 2008). Part of the challenge is that meals cause overlapping of fermentation patterns. The daily pattern of intake adds an additional level of complexity, but integrating the traditional concept of rumen nutrient synchrony with the circadian rhythms of intake and milk synthesis may provide a more robust theoretical model and improve success of the application in the field. An important implication is that meals overlap more during the active feeding period of the day than the overnight low-intake period. This may decrease the importance of nutrient synchrony during the day and increase the importance of controlled release of nutrients during lower-intake periods.
Evidence of circadian regulation of milk synthesis
It is commonly recognised that morning and evening milking differ in milk yield and composition, but very few experiments have reported individual milkings. Quist et al. (2008) conducted a survey of the milking-to-milking variation in milk yield and composition on 16 dairy farms. Milk yield and milk fat concentration showed a clear repeated daily pattern over the 5 days of observation in herds that milked twice and three times per day. Milk protein also followed a repeating pattern with a smaller amplitude. Generally, milk yield is higher at the morning milking, but milk fat and protein concentration are higher at the evening milking. Rottman et al. (2014) tested the effect of the timing of feed intake on the daily pattern of milk yield and composition by feeding cows ad libitum or in equal meals every 6 h and milking all cows four times per day to increase resolution across the day. The daily rhythm of milk yield was modified and the amplitude of milk fat concentration was decreased ~50%, demonstrating that the daily pattern of milk synthesis is at least partially dependent on the timing of feed intake. Next, Salfer and Harvatine (2020) fasted cows for 7 h per day either during the day or the night (Fig. 5). Daily milk fat yield and average milk fat and protein concentration were not changed, but the rhythms of milk yield and milk fat and protein concentration were nearly inverted and the amplitudes of the rhythms were also modified. Restricted feeding modifies energy and all nutrients, so follow-up experiments were conducted that ruminally infused acetate and duodenally infused casein either continuously or for 7 h during the day or the night (Matamoros et al. 2022; Salfer et al. 2023). The timing of both protein and acetate infusion modified the daily pattern of milk synthesis. Specifically, acetate infusion during the day maintained higher milk fat during this period of the day and shifted peak milk fat concentration. Importantly, these experiments demonstrated the daily pattern of milk synthesis and its dependence on the timing of nutrient absorption.
Effect of feed available for 16 h during the day (solid line; feed from 0700 hours to 2300 hours) or feed for 16 h during the night (dotted line; feed from 1900 hours to 1100 h hours) on the timing of milk yield in cows milked four times per day. Amplitude (Amp), difference between peak and mean; and Acrophase (Acro), time at peak of the rhythm. P-values of the zero-amplitude test are shown. The black and white bars above the x-axis display the light–dark cycle. Values in a column that do not share a superscript letter are different (at P = 0·05). Originally published in Salfer and Harvatine (2020).
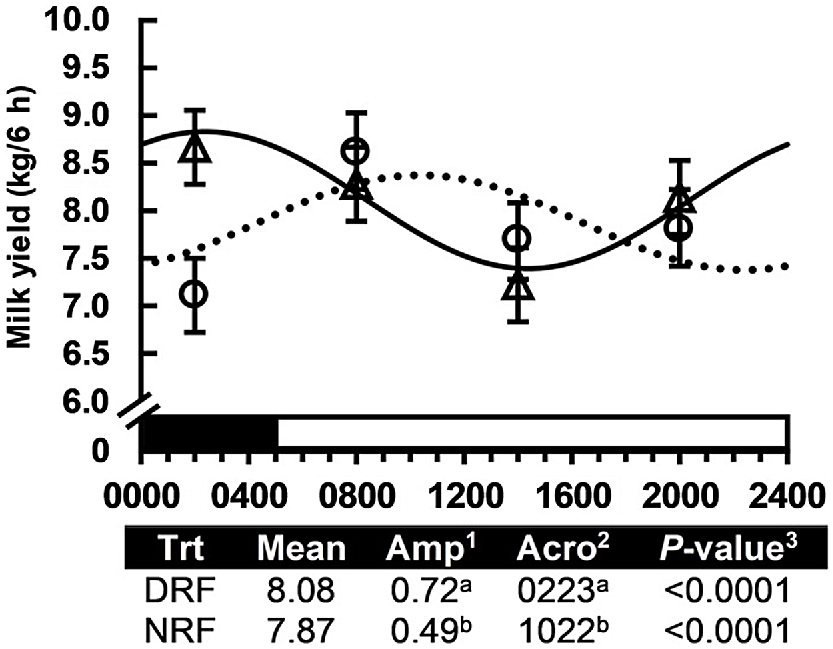
Managing circadian rhythms
Circadian rhythms are a natural biological function and, on the basis of the basic biology, it should be advantageous to manage to maintain consistent daily patterns of lighting, feed delivery, and milking. This will reduce the occurrence of ‘jet lag’ where rhythms are undergoing major adjustment. The next question is the correct timing of daily activities on farms to allow synchronisation of rhythms.
A major challenge is that the daily pattern of feed intake increases fluctuations in the rumen and our current dogma is that more constant conditions would be advantageous. Spreading intake across a larger portion of the day is the first approach to achieving a more consistent rumen environment. The timing of feed delivery is the first opportunity to modify feed intake, and it is logical to try to deliver feed during the lower-intake periods of the day. However, feed delivery at night stimulates slug eating and should be avoided (Niu et al. 2014; Niu and Harvatine 2018a). Since cows naturally eat more during the afternoon and early evening, early morning feed delivery may stimulate intake earlier in the day when the rumen starch pool is low and rumen pH is higher.
The second proposed approach is to feed diets that differ in nutrient composition across the day to complement the rate of feed intake. For example, feeding a lower-starch diet during the afternoon and early evening when intake is high and a higher-starch diet during the overnight when intake is low. We conducted a series of experiments to investigate this approach. In the first experiment, a control TMR was fed once per day at 0900 hours or the same feed components were fed as a lower-starch diet at 70% of daily intake and a higher-starch diet at 30% of daily intake (Rottman et al. 2015). When the lower-starch diet was fed first (0900 hours), followed by the higher-starch diet in the evening (2200 hours), milk yield and milk composition and empty body weight gain did not change, but dry-matter intake decreased by ~6%. The experiment also included a treatment that fed the high-starch diet first at 0900 hours, followed by the low-starch diet at 1300 hours, which was expected to increase starch load during the large conditioned meal after morning feeding and negatively affect rumen fermentation. However, it actually increased milk and milk fat yield compared with feeding the low-starch followed by the high-starch diet. This was because delivering the high-starch diet late in the evening caused a large increase in intake at feed delivery, resulting in slug feeding when the rumen was already higher in starch after the high intake period of the day. This highlighted that the effect on feeding behaviour must be considered. We then proposed that feeding starch early in the day may be advantageous because rumen starch pool is at its nadir and amylolytic capacity is also likely to be lower before feeding (Raffrenato et al. 2022).
In a follow-up experiment, Niu et al. (2017) similarly fed a control TMR and two PMRs that delivered the same nutrients as the control, but the diets differed in both starch concentration and rate of digestibility as the high starch diet contained steam-flaked corn and corn silage as starch sources. The combination of feeding a diet with higher starch and faster digestibility first in the morning at 0700 hours decreased milk fat yield largely through a decrease at the morning milking, contrary to our hypothesis based on the previously described study. Interestingly, there was no increase in trans-10 18:1 in milk, indicating that the decrease was not due to biohydrogenation-induced milk fat depression. We have observed changes in milk fat in response to acetate treatments (Urrutia and Harvatine 2017) and changes in acetate production may be involved but were not investigated. It is likely that the combination of starch level and fermentability pushed digestibility too far, highlighting a delicate balancing act in designing these interventions.
A third experiment tested feeding a TMR that contained 7.8% steam-flaked corn compared with feeding the steam-flaked corn prior to the morning feeding, followed by a PMR that contained the other components of the TMR (Niu and Harvatine 2018b). Although we expected this slug-feeding of steam-flaked corn to be well tolerated, because the rumen starch pool and amylolytic capacity are low, it tended to decrease milk yield and decreased milk fat yield, with no change in trans-10 18:1.
We have not explored changing dietary protein across the day, but Robinson et al. (1997) stabilised ruminal fermentation by feeding a protein supplement (15% DMI) at 0300 hours compared with 0830 hours in cows fed a TMR twice daily at 0800 hours and 1800 hours. They observed increased ruminal organic matter and crude protein digestibility, increased ruminal VFA concentration, a more stable ruminal pH, and increased milk fat with supplementation at 0300 hours. Milk yield was not changed, but milk fat yield was higher with protein supplement fed at 0300 hours. There is considerable potential for modification of dietary protein to both match rumen microbial needs, maximise rumen escape of high-quality protein, match amino acid requirements, and possibly stimulate milk protein synthesis through activation of mTOR signalling.
We remain excited about the potential to design feeding approaches where diet composition and timing of supplementation complement the circadian pattern of intake. However, the optimal combination has been difficult to determine due to many interacting factors and dynamic cow behaviour. We also expect that there is an optimal time of day for many supplements. For example, a direct-fed microbial may be more advantageous during the high-intake period of the day or a rumen-protected product may have a higher rumen escape during a certain period of the day.
Traditionally, daily rhythms have been difficult to measure in both research and field settings due to the need to have multiple observations over the day. However, new technology and data analysis methods are rapidly providing high-resolution cow-level data that will greatly aid work in this area. For example, activity observation systems that predict rumination, intake, and general activity in real time are rapidly being adopted on farms. We expect that analysis of daily patterns and appreciation for their importance will increase the usefulness of these data beyond observing daily averages. Future developments in video-based systems and parlour sensors also provide exciting potential to better understand the interaction of cow behaviour and management.
Conclusions
Circadian clocks are endogenous rhythms in the brain and peripheral tissue that are entrained by light and feeding. This robust system coordinates physiology and metabolism with the external environment. The dairy cow has a clear daily pattern of feed intake that has implications for stable rumen fermentation and nutrient absorption and a daily pattern of milk synthesis that is modified by the timing of nutrient absorption. The timing of feed delivery and feed management are our best opportunities to modify the daily pattern of feed intake and nutrient absorption. We expect that appreciating the circadian rhythms of the dairy cow will lead to improved nutrition and management practices that increase efficiency through both rumen and post-absorptive mechanisms.
Data availability
Data sharing is not applicable as no new data were generated or analysed during this study.
Declaration of funding
Our investigation of circadian rhythms was supported in part by USDA Agriculture and Food Research Initiative Competitive Grant no. 2009-34281-20116 and 2015-67015-23358 from the USDA National Institute of Food and Agriculture [PI KJH] and Penn State University including USDA (Kansas City, MO) National Institute of Food and Agriculture Federal Appropriations under Project number PEN04664 and accession number 1017181 [KJH].
Acknowledgements
Dr Paul Bartell, Penn State University, is an expert in circadian biology and was a key collaborator in our work investigating rhythms in dairy cows. L. Whitney Rottman, Dr Isaac Salfer, and Dr Mutian Niu conducted most of the experiments investigating circadian rhythms at Penn State while graduate students with the assistance of Yun (Jackie) Ying.
References
Adamovich Y, Rousso-Noori L, Zwighaft Z, Neufeld-Cohen A, Golik M, Kraut-Cohen J, Wang M, Han X, Asher G (2014) Circadian clocks and feeding time regulate the oscillations and levels of hepatic triglycerides. Cell Metabolism 19, 319-330.
| Crossref | Google Scholar | PubMed |
Albright JL (1993) Feeding behavior of dairy cattle. Journal of Dairy Science 76, 485-498.
| Crossref | Google Scholar |
Allen MS, Bradford BJ, Harvatine KJ (2005) The cow as a model to study food intake regulation. Annual Review of Nutrition 25, 523-547.
| Crossref | Google Scholar | PubMed |
Asher G, Schibler U (2011) Crosstalk between components of circadian and metabolic cycles in mammals. Cell Metabolism 13, 125-137.
| Crossref | Google Scholar | PubMed |
Bass J, Takahashi JS (2010) Circadian integration of metabolism and energetics. Science 330, 1349-1354.
| Crossref | Google Scholar | PubMed |
Bauman DE, McCutcheon SN, Steinhour WD, Eppard PJ, Sechen SJ (1985) Sources of variation and prospects for improvement of productive efficiency in the dairy cow: a review. Journal of Animal Science 60, 583-592.
| Crossref | Google Scholar | PubMed |
Boehning D, Snyder SH (2002) Carbon monoxide and clocks. Science 298, 2339-2340.
| Crossref | Google Scholar | PubMed |
Casey TM, Plaut K (2012) Lactation Biology Symposium: circadian clocks as mediators of the homeorhetic response to lactation. Journal of Animal Science 90, 744-754.
| Crossref | Google Scholar | PubMed |
Casey TM, Plaut K (2022) Circadian clocks and their integration with metabolic and reproductive systems: our current understanding and its application to the management of dairy cows. Journal of Animal Science 100, skac233.
| Crossref | Google Scholar |
Casey T, Crodian J, Suárez-Trujillo A, Erickson E, Weldon B, Crow K, Cummings S, Chen Y, Shamay A, Mabjeesh SJ, Plaut K (2016) CLOCK regulates mammary epithelial cell growth and differentiation. American Journal of Physiology: Regulatory, Integrative and Comparative Physiology 311, R1125-R1134.
| Crossref | Google Scholar | PubMed |
Casey T, Suarez-Trujillo A, Cummings S, Huff K, Crodian J, Bhide K, Aduwari C, Teeple K, Shamay A, Mabjeesh SJ, San Miguel P, Thimmapuram J, Plaut K (2021) Core circadian clock transcription factor BMAL1 regulates mammary epithelial cell growth, differentiation, and milk component synthesis. PLoS ONE 16, e0248199.
| Crossref | Google Scholar | PubMed |
Cermakian N, Lange T, Golombek D, Sarkar D, Nakao A, Shibata S, Mazzoccoli G (2013) Crosstalk between the circadian clock circuitry and the immune system. Chronobiology International 30, 870-888.
| Crossref | Google Scholar | PubMed |
Chamorro R, Jouffe C, Oster H, Uhlenhaut NH, Meyhöfer SM (2023) When should I eat: a circadian view on food intake and metabolic regulation. Acta Physiologica 237, e13936.
| Crossref | Google Scholar |
DeVries TJ, von Keyserlingk MAG, Beauchemin KA (2005) Frequency of feed delivery affects the behavior of lactating dairy cows. Journal of Dairy Science 88, 3553-3562.
| Crossref | Google Scholar | PubMed |
DeVries TJ, Beauchemin KA, von Keyserlingk MAG (2007) Dietary forage concentration affects the feed sorting behavior of lactating dairy cows. Journal of Dairy Science 90, 5572-5579.
| Crossref | Google Scholar | PubMed |
Dibner C, Schibler U, Albrecht U (2010) The mammalian circadian timing system: organization and coordination of central and peripheral clocks. Annual Review of Physiology 72, 517-549.
| Crossref | Google Scholar | PubMed |
Doherty CJ, Kay SA (2010) Circadian control of global gene expression patterns. Annual Review of Genetics 44, 419-444.
| Crossref | Google Scholar | PubMed |
Escobar C, Cailotto C, Angeles-Castellanos M, Delgado RS, Buijs RM (2009) Peripheral oscillators: the driving force for food-anticipatory activity. European Journal of Neuroscience 30, 1665-1675.
| Crossref | Google Scholar | PubMed |
Evans JA, Davidson AJ (2013) Health consequences of circadian disruption in humans and animal models. Progress in Molecular Biology and Translational Science 119, 283-323.
| Crossref | Google Scholar | PubMed |
Finger A-M, Kramer A (2021) Peripheral clocks tick independently of their master. Genes & Development 35, 304-306.
| Crossref | Google Scholar | PubMed |
Giannetto C, Piccione G (2009) Daily rhythms of 25 physiological variables in Bos taurus maintained under natural conditions. Journal of Applied Biomedicine 7, 55-61.
| Crossref | Google Scholar |
Golombek DA, Casiraghi LP, Agostino PV, Paladino N, Duhart JM, Plano SA, Chiesa JJ (2013) The times they’re a-changing: effects of circadian desynchronization on physiology and disease. Journal of Physiology–Paris 107, 310-322.
| Crossref | Google Scholar | PubMed |
Hall MB, Huntington GB (2008) Nutrient synchrony: sound in theory, elusive in practice. Journal of Animal Science 86, E287-E292.
| Crossref | Google Scholar | PubMed |
Hansen J (2017) Night shift work and risk of breast cancer. Current Environmental Health Reports 4, 325-339.
| Crossref | Google Scholar | PubMed |
Hara R, Wan K, Wakamatsu H, Aida R, Moriya T, Akiyama M, Shibata S (2001) Restricted feeding entrains liver clock without participation of the suprachiasmatic nucleus. Genes to Cells 6, 269-278.
| Crossref | Google Scholar | PubMed |
Hosseinkhani A, Devries TJ, Proudfoot KL, Valizadeh R, Veira DM, von Keyserlingk MAG (2008) The effects of feed bunk competition on the feed sorting behavior of close-up dry cows. Journal of Dairy Science 91, 1115-1121.
| Crossref | Google Scholar | PubMed |
Isobe Y, Hida H, Nishino H (2011) Circadian rhythm of enolase in suprachiasmatic nucleus depends on mitochondrial function. Journal of Neuroscience Research 89, 936-944.
| Crossref | Google Scholar | PubMed |
Jin Y, Hur T-Y, Hong Y (2017) Circadian rhythm disruption and subsequent neurological disorders in night-shift workers. Journal of Lifestyle Medicine 7, 45-50.
| Crossref | Google Scholar | PubMed |
Lefcourt AM, Huntington JB, Akers RM, Wood DL, Bitman J (1999) Circadian and ultradian rhythms of body temperature and peripheral concentrations of insulin and nitrogen in lactating dairy cows. Domestic Animal Endocrinology 16, 41-55.
| Crossref | Google Scholar | PubMed |
Li H, Li K, Zhang K, Li Y, Gu H, Liu H, Yang Z, Cai D (2021) The circadian physiology: implications in livestock health. International Journal of Molecular Sciences 22, 2111.
| Crossref | Google Scholar |
Litichevskiy L, Thaiss CA (2022) The oscillating gut microbiome and its effects on host circadian biology. Annual Review of Nutrition 42, 145-164.
| Crossref | Google Scholar | PubMed |
Matamoros C, Cai J, Patterson AD, Harvatine KJ (2021) Comparison of the effects of short-term feeding of sodium acetate and sodium bicarbonate on milk fat production. Journal of Dairy Science 104, 7572-7582.
| Crossref | Google Scholar | PubMed |
Matamoros C, Salfer IJ, Bartell PA, Harvatine KJ (2022) Effect of the timing of sodium acetate infusion on the daily rhythms of milk synthesis and plasma metabolites and hormones in Holstein cows. Journal of Dairy Science 105, 7432-7445.
| Crossref | Google Scholar | PubMed |
Maulfair DD, Fustini M, Heinrichs AJ (2011) Effect of varying total mixed ration particle size on rumen digesta and fecal particle size and digestibility in lactating dairy cows. Journal of Dairy Science 94, 3527-3536.
| Crossref | Google Scholar | PubMed |
McCabe CJ, Suarez-Trujillo A, Teeple KA, Casey TM, Boerman JP (2021) Chronic prepartum light-dark phase shifts in cattle disrupt circadian clocks, decrease insulin sensitivity and mammary development, and are associated with lower milk yield through 60 days postpartum. Journal of Dairy Science 104, 2422-2437.
| Crossref | Google Scholar | PubMed |
Mohawk JA, Green CB, Takahashi JS (2012) Central and peripheral circadian clocks in mammals. Annual Review of Neuroscience 35, 445-462.
| Crossref | Google Scholar | PubMed |
Nakahata Y, Sahar S, Astarita G, Kaluzova M, Sassone-Corsi P (2009) Circadian control of the NAD+ salvage pathway by CLOCK-SIRT1. Science 324, 654-657.
| Crossref | Google Scholar | PubMed |
Nikkhah A, Furedi CJ, Kennedy AD, Crow GH, Plaizier JC (2008) Effects of feed delivery time on feed intake, milk production, and blood metabolites of dairy cows. Journal of Dairy Science 91, 4249-4260.
| Crossref | Google Scholar | PubMed |
Niu M, Harvatine KJ (2018a) Short communication: the effects of morning compared with evening feed delivery in lactating dairy cows during the summer. Journal of Dairy Science 101, 396-400.
| Crossref | Google Scholar | PubMed |
Niu M, Harvatine KJ (2018b) The effects of feeding a partial mixed ration plus a top-dress before feeding on milk production and the daily rhythm of feed intake and plasma hormones and metabolites in dairy cows. Journal of Dairy Science 101, 164-171.
| Crossref | Google Scholar | PubMed |
Niu M, Ying Y, Bartell PA, Harvatine KJ (2014) The effects of feeding time on milk production, total-tract digestibility, and daily rhythms of feeding behavior and plasma metabolites and hormones in dairy cows. Journal of Dairy Science 97, 7764-7776.
| Crossref | Google Scholar | PubMed |
Niu M, Ying Y, Bartell PA, Harvatine KJ (2017) The effects of feeding rations that differ in fiber and fermentable starch within a day on milk production and the daily rhythm of feed intake and plasma hormones and metabolites in dairy cows. Journal of Dairy Science 100, 187-198.
| Crossref | Google Scholar | PubMed |
Plaut K, Casey T (2012) Does the circadian system regulate lactation? Animal: an International Journal of Animal Bioscience 6, 394-402.
| Crossref | Google Scholar |
Quist MA, LeBlanc SJ, Hand KJ, Lazenby D, Miglior F, Kelton DF (2008) Milking-to-milking variability for milk yield, fat and protein percentage, and somatic cell count. Journal of Dairy Science 91, 3412-3423.
| Crossref | Google Scholar | PubMed |
Raffrenato E, Badenhorst MJ, Harvatine KJ, Shipandeni MNT, du Plessis L, Esposito G, van Zyl WH (2022) The diurnal patterns of ruminal enzymatic activity and in vitro digestibility of starch, neutral detergent fiber, and protein. Journal of Dairy Science 105, 4961-4970.
| Crossref | Google Scholar | PubMed |
Ramsey KM, Yoshino J, Brace CS, Abrassart D, Kobayashi Y, Marcheva B, Hong H-K, Chong JL, Buhr ED, Lee C, Takahashi JS, Imai S-I, Bass J (2009) Circadian clock feedback cycle through NAMPT-mediated NAD+ biosynthesis. Science 324, 651-654.
| Crossref | Google Scholar | PubMed |
Robinson PH, Gill M, Kennelly JJ (1997) Influence of time of feeding a protein meal on ruminal fermentation and forestomach digestion in dairy cows. Journal of Dairy Science 80, 1366-1373.
| Crossref | Google Scholar | PubMed |
Rottman LW, Ying Y, Zhou K, Bartell PA, Harvatine KJ (2014) The daily rhythm of milk synthesis is dependent on the timing of feed intake in dairy cows. Physiological Reports 2, e12049.
| Crossref | Google Scholar | PubMed |
Rottman LW, Ying Y, Zhou K, Bartell PA, Harvatine KJ (2015) The effects of feeding rations that differ in neutral detergent fiber and starch concentration within a day on production, feeding behavior, total-tract digestibility, and plasma metabolites and hormones in dairy cows. Journal of Dairy Science 98, 4673-4684.
| Crossref | Google Scholar | PubMed |
Rutter GA (2001) Nutrient-secretion coupling in the pancreatic islet β-cell: recent advances. Molecular Aspects of Medicine 22, 247-284.
| Crossref | Google Scholar | PubMed |
Rutter J, Reick M, McKnight SL (2002) Metabolism and the control of circadian rhythms. Annual Review of Biochemistry 71, 307-331.
| Crossref | Google Scholar | PubMed |
Salfer IJ, Harvatine KJ (2018) The effect of night restricted feeding on the molecular circadian clock of the mammary gland. Journal of Dairy Science 101(Suppl. 1), 405.
| Google Scholar |
Salfer IJ, Harvatine KJ (2020) Night-restricted feeding of dairy cows modifies daily rhythms of feed intake, milk synthesis and plasma metabolites compared with day-restricted feeding. British Journal of Nutrition 123, 849-858.
| Crossref | Google Scholar |
Salfer IJ, Morelli MC, Ying Y, Allen MS, Harvatine KJ (2018) The effects of source and concentration of dietary fiber, starch, and fatty acids on the daily patterns of feed intake, rumination, and rumen pH in dairy cows. Journal of Dairy Science 101, 10911-10921.
| Crossref | Google Scholar | PubMed |
Salfer IJ, Crawford CE, Rottman LW, Harvatine KJ (2021) The effects of feeding rations that differ in neutral detergent fiber and starch within a day on the daily pattern of key rumen microbial populations. JDS Communications 2, 334-339.
| Crossref | Google Scholar | PubMed |
Salfer IJ, Matamoros CI, Bartell PA, Harvatine KJ (2023) Effects of the timing of protein infusion on the daily rhythms of milk synthesis and plasma hormones and metabolites in dairy cows. Journal of Dairy Science 106, Accepted.
| Crossref | Google Scholar |
Schingoethe DJ (2017) A 100-year review: total mixed ration feeding of dairy cows. Journal of Dairy Science 100, 10143-10150.
| Crossref | Google Scholar | PubMed |
Sinturel F, Spaleniak W, Dibner C (2022) Circadian rhythm of lipid metabolism. Biochemical Society Transactions 50, 1191-1204.
| Crossref | Google Scholar | PubMed |
Stokkan K-A, Yamazaki S, Tei H, Sakaki Y, Menaker M (2001) Entrainment of the circadian clock in the liver by feeding. Science 291, 490-493.
| Crossref | Google Scholar | PubMed |
Strohmaier S, Devore EE, Zhang Y, Schernhammer ES (2018) A review of data of findings on night shift work and the development of DM and CVD events: a synthesis of the proposed molecular mechanisms. Current Diabetes Reports 18, 132.
| Crossref | Google Scholar | PubMed |
Suárez-Trujillo A, Casey TM (2016) Serotoninergic and circadian systems: driving mammary gland development and function. Frontiers in Physiology 7, 301.
| Crossref | Google Scholar | PubMed |
Suarez-Trujillo A, Wernert G, Sun H, Steckler TS, Huff K, Cummings S, Franco J, Klopp RN, Townsend JR, Grott M, Johnson JS, Plaut K, Boerman JP, Casey TM (2020) Exposure to chronic light-dark phase shifts during the prepartum nonlactating period attenuates circadian rhythms, decreases blood glucose, and increases milk yield in the subsequent lactation. Journal of Dairy Science 103, 2784-2799.
| Crossref | Google Scholar | PubMed |
Suarez-Trujillo A, Hoang N, Robinson L, McCabe CJ, Conklin D, Minor RC, Townsend J, Plaut K, George UZ, Boerman J, Casey TM (2022) Effect of circadian system disruption on the concentration and daily oscillations of cortisol, progesterone, melatonin, serotonin, growth hormone, and core body temperature in periparturient dairy cattle. Journal of Dairy Science 105, 2651-2668.
| Crossref | Google Scholar | PubMed |
Takahashi JS, Hong H-K, Ko CH, McDearmon EL (2008) The genetics of mammalian circadian order and disorder: implications for physiology and disease. Nature Reviews Genetics 9, 764-775.
| Crossref | Google Scholar | PubMed |
Taleb Z, Karpowicz P (2022) Circadian regulation of digestive and metabolic tissues. American Journal of Physiology: Cell Physiology 323, C306-C321.
| Crossref | Google Scholar | PubMed |
Urrutia NL, Harvatine KJ (2017) Acetate dose-dependently stimulates milk fat synthesis in lactating dairy cows. The Journal of Nutrition 147, 763-769.
| Crossref | Google Scholar | PubMed |
Verwoerd W, Wellby M, Barrell G (2006) Absence of a causal relationship between environmental and body temperature in dairy cows (Bos taurus) under moderate climatic conditions. Journal of Thermal Biology 31, 533-540.
| Crossref | Google Scholar |
von Keyserlingk MAG, Weary DM (2010) Review: feeding behaviour of dairy cattle: measures and applications. Canadian Journal of Animal Science 90, 303-309.
| Crossref | Google Scholar |
Walker WH, II, Walton JC, DeVries AC, Nelson RJ (2020) Circadian rhythm disruption and mental health. Translational Psychiatry 10, 28.
| Crossref | Google Scholar | PubMed |
Yang WZ, Beauchemin KA (2006) Effects of physically effective fiber on chewing activity and ruminal pH of dairy cows fed diets based on barley silage. Journal of Dairy Science 89, 217-228.
| Crossref | Google Scholar | PubMed |
Ying Y, Rottman LW, Crawford C, Bartell PA, Harvatine KJ (2015) The effects of feeding rations that differ in neutral detergent fiber and starch concentration within a day on rumen digesta nutrient concentration, pH, and fermentation products in dairy cows. Journal of Dairy Science 98, 4685-4697.
| Crossref | Google Scholar | PubMed |