Range decline and conservation status of Westralunio carteri Iredale, 1934 (Bivalvia : Hyriidae) from south-western Australia
Michael W. Klunzinger A B D , Stephen J. Beatty A , David L. Morgan A , Adrian M. Pinder C and Alan J. Lymbery AA Freshwater Fish Group and Fish Health Unit, Centre for Fish and Fisheries Research, School of Veterinary and Life Sciences, Murdoch University, Murdoch, WA 6150, Australia.
B Malacology Section, Department of Aquatic Zoology, Collections and Research Facility, Western Australian Museum, Welshpool, WA 6160, Australia.
C Science and Conservation Division, Department of Parks and Wildlife, Kensington, WA 6151, Australia.
D Corresponding author. Email: m.klunzinger@murdoch.edu.au
Australian Journal of Zoology 63(2) 127-135 https://doi.org/10.1071/ZO15002
Submitted: 5 January 2015 Accepted: 21 April 2015 Published: 12 May 2015
Abstract
Westralunio carteri is the only species of freshwater mussel found in south-western Australia and, owing to a lack of comprehensive information on its ecology, its conservation status has been speculative. To more accurately predict the true conservation status of this species, the historical and contemporary distributional records were modelled with environmental data that identified salinity, perenniality and total nitrogen as variables responsible for limiting the species’ current extent of occurrence, inferring threatening processes. The species was found to have undergone a 49% reduction in extent of occurrence in less than three generations, due primarily to secondary salinisation. Current distribution is bounded by Gingin Brook in the north to the Kent, Goodga and Waychinicup Rivers in the South, within 50–100 km of coastal south-western Australia. Field observations indicated that W. carteri was almost never found at sites where mean salinity was >1.6 g L–1. This was corroborated by laboratory tolerance trials that showed that W. carteri has an acute salinity tolerance (LD50) of 1.6–3.0 g L–1. Application of IUCN Red List criteria indicates that W. carteri qualifies for listing as vulnerable. Conservation management measures should focus on maintaining existing populations.
Additional keywords: freshwater mussel, salinity, species distribution model, threatened species.
Introduction
Freshwater mussels (Unionida) are a widely distributed group of bivalve molluscs, inhabiting streams, rivers, wetlands and lakes on every continent except Antarctica (Bogan and Roe 2008; Graf 2013). They are important in freshwater ecosystem functionality (Spooner and Vaughn 2006; Aldridge et al. 2007; Haag 2013). Owing to their relatively limited migration and dispersal abilities, their sensitivity to changes in the environment has become evident through heavy declines in recent decades; freshwater mussels are among the most threatened group of fauna globally (Seddon et al. 2012; Lopes-Lima et al. 2014).
Australia’s freshwater mussels belong to six genera, all from the Hyriidae, believed to have arisen between the Jurassic and Cretaceous periods, with Westralunio carteri as perhaps the most ancient (Graf et al. 2015) and the sole representative of the genus in Australia (McMichael and Hiscock 1958). The taxon was described as a subspecies (W. ambiguus carteri) by Iredale (1934) and as a distinct species (W. carteri) by McMichael and Hiscock (1958). However, until recently, little was known of its biology. The species is now known to be a sexually dimorphic seasonal brooder with females producing spineless hooked larvae (glochidia) that are parasitic on several host fishes (making W. carteri a host-generalist); they become sexually mature within 3–6 years and live for at least 50 years (Klunzinger et al. 2012a, 2013, 2014).
Declines in populations of W. carteri were first observed in two of the largest rivers throughout its range, i.e. the Avon River (Kendrick 1976) and the Blackwood River (Williams et al. 1991), with their disappearance attributed to increases in salinity due to secondary salinisation of these rivers. As is evident from other regions of the world where freshwater mussels are in serious decline, changes in land-use practices may have altered the physico-chemical state of habitats that support W. carteri. To what degree those changes present a conservation challenge to the survival of the species, however, has not been clear (e.g. IUCN 1996 versus Köhler 2011). Understanding how such impacts affect species is difficult to determine without sound ecological data.
The International Union for the Conservation of Nature (IUCN) Red List of Threatened Species is widely recognised as one of the most authoritative sources of information on the conservation status of plants and animals (Lamoreux et al. 2003; Rodrigues et al. 2006). The use of the IUCN Red List in conservation policy and planning places a need to provide high-quality data in order to apply the listing criteria to species conservation assessments. In practice, however, such data are often lacking, especially for invertebrates (Newton 2010; Cardoso et al. 2012), which appears to have been the case for W. carteri.
The aims of this study were to accurately assess the conservation status of W. carteri through a multimodel inference approach and to determine the key threatening processes for the conservation of the species. The data presented will assist in guiding future research and conservation management of the species and habitats in which it lives.
Methods
Distribution
Two databases of W. carteri occurrence were compiled: (1) a historic (pre-1992) database of 255 presence-only records from museum specimens and unpublished sources; and (2) a current (1992–2012) database of 816 records of presence or absence determined from site visits, each consisting of 10–20 min of visual and tactile searching, unpublished data – A. M. Pinder and unpublished technical reports and returns from citizen science surveys (Murdoch University and SERCUL 2010). The data are provided in the supplementary material (Table S1). Seventy-seven sites from the current database were able to be matched with records of mussel presence from the historical database. All sites were within the South West Coast Drainage Division of Western Australia, which covers 326 000 km2 and includes 19 river basins (AWRC 1976).
Historic and current presence records were mapped as vector data in ArcGIS™ Desktop 10.2 using the GCS_GDA_1994 coordinate system (Geoscience Australia 2003). Extent of occurrence was determined by constructing minimum convex polygons (α-hulls) in ArcGIS™ Desktop 10.2, following IUCN guidelines (IUCN 2013). Two α-hulls were constructed from species distributions: one was drawn for historic extent of occurrence (EOO) and another for the current EOO. The areas of the resulting polygons were determined using the feature properties tool and summed to provide a total area. Thus, the percentage change between the α-hulls estimated the temporal change in EOO.
Environmental variables
There were 39 potential reach-scale environmental variables for sites in the current database, derived from visual assessments during site surveys or chemical parameters from gauging station and discrete sampling data supplied by the Western Australian Department of Water, under licence. Fish species diversity was not included in the list of potential environmental variables, because it was available only for some of the sites and because we considered it unlikely to be a major determining factor in the distribution of W. carteri, which is a host generalist (Klunzinger et al. 2012a). Given the importance of variable selection and the need to avoid over-fitting the data during model construction (Burnham and Anderson 2002), several approaches were used to reduce the variable set. Principal components analysis (PCA) on the complete variable set did not produce interpretable results. Instead, variables were initially categorised as ‘water habitat’, ‘bank and substratum assessment’ and ‘water chemistry’, and PCA was performed within each category to identify correlated variables. Rather than reduce the variable set to components, a subset of environmental variables was chosen, with potential functional relationships to physiological and behavioural attributes of the species (Leathwick et al. 2005; Elith and Leathwick 2009). Where variables were highly correlated (r > 0.6), distal variables (e.g. rainfall) were removed in favour of proximal variables (e.g. flow status), which are more likely to have a direct effect on species distribution (Wintle et al. 2005). This yielded seven largely independent environmental predictors (Table 1: all correlations for continuous variables <0.4).
Threat quantification
Current occurrence data were related to environmental variables using generalised linear models (GLM) with a binomial distribution and logit link function. An information-theoretic, multimodel inference approach was employed as the goal was to determine the relative importance of environmental factors, and not to develop a predictive model (Burnham and Anderson 2002). A global model was fitted to a subset of 299 sites for which full environmental data were available, using the R statistical package (R Development Core Team 2013). Spatial autocorrelation of model residuals with straight-line distance between sites was tested using Moran’s I (Dormann et al. 2007). The global model was used to generate a set of all possible models using the R package MuMIn (Bartoñ 2013). Models were then ranked by the Akaike Information Criterion, corrected for small sample size (AICc) (Burnham and Anderson 2002), and model averaging was performed using MuMIn across all models within four AICc values of the best model (i.e. models were selected for inclusion if Δi < 4, where Δi = AICc (i) – AICc (minimum)). The importance of each variable was determined by summing Aikaike likelihood weights across all models within the top-ranked set in which the variable occurred, providing the selection probability that a given variable will appear in the AIC best model (Burnham and Anderson 2002). To discover which environmental predictors may be responsible for changes in mussel distribution, current and historical mussel occurrence were compared at 41 sites where mussels occurred historically and where current data on all environmental variables were available. Changes in occurrence were coded as 0 (historically present, currently absent) or 1 (historically and currently present), and related to environmental variables as described above. Unfortunately, historical values for environmental variables were not available, so changes in occurrence could be related only to current values.
Estimates of parameters and variable importance from model averaging can be adversely affected by collinearity among predictor variables (Murray and Connor 2009; Freckleton 2011). Collinearity was assessed by calculating variance inflation factors (VIF) for each variable, using the R package HH (Heiberger 2013). The VIF of a variable represents the extent to which the variance of the regression coefficient for that variable is inflated due to its correlation with other variables. As a rule of thumb, VIF >10 indicates excessive collinearity (Myers 1990), although Montgomery and Peck (1992) suggest that any VIF >5 is a cause for concern. For variables that were significantly related to mussel presence in model-averaging analysis, a decision-tree analysis was performed using JMP 10.0 (SAS Institute, Carey, NC). The tree indicated environmental values that maximised the difference between sites where mussels were present and sites where they were absent.
Salinity tolerance trials
Because salinity was identified as a potential key threatening process for W. carteri through distribution analysis (see Results), this hypothesis was tested in the laboratory. Live W. carteri, with shell lengths between 52 and 87 mm, were hand-collected from two populations: a fresh to brackish site (salinity range 0.39–3.16 g L–1 and a mean of 1.10 g L–1) in the Collie River (33.3003°S, 115.8169°E), and a low-salinity site (salinity range 0.04–0.05 g L–1 and a mean of 0.05 g L–1) in Yalyal Brook (31.5025°S, 115.9964°E). Mussels were transported to the laboratory and acclimated in aquaria with fresh water (salinity <0.5 g L–1) and a recirculated filtration system for two days before commencing acute (instantaneous) salinity tolerance experiments. Instantaneous tolerance experiments were used, rather than a gradual increase in salinity, because this matches the typical pattern of salinity increases in rivers in the south-west of Western Australia, where salinity rises sharply with the onset of winter rains due to water flow from the upper, saline parts of the catchment (Mayer et al. 2005; Beatty et al. 2011).
Salinity tolerance experiments were conducted at room temperature in 20 continuously circulated, aerated aquaria (54 L) with four replicates per salinity treatment in each experiment. Mussels from the Collie River were tested in the first experiment, followed by mussels from Yalyal Brook in the second experiment. Treatments for both experiments consisted of five salinity concentrations: control (<0.5 g L–1), 1, 2, 3 and 4 g L–1. Treatment solutions were prepared by dissolving lake salt (WA Salt Supply, Inc., North Coogee, WA 6163) in dechlorinated tap water and salinity concentrations determined using an Oakton™ PCD650 portable water-testing meter. The ionic composition of heavily salinised rivers in Western Australia varies geographically and seasonally, but is typically similar to that of seawater, except for lower concentrations of potassium (Partridge et al. 2008). Salinity concentrations were maintained by ensuring aquaria volumes remained topped up with dechlorinated tap water and salinities measured to ensure they matched the treatment salinity concentration. After the acclimation period, mussels were allocated randomly to each experimental aquarium. Mussels were observed daily and removed when dead, which was indicated by gaping valves that did not close when prodded. In some of the higher-salinity treatments (3–4 g L–1), shells appeared to remain shut for the entire experiment, but mortality was evident when mussels became autolytic and began to float as a result of a build-up of gas within the shell cavities. Experiments were carried out for a total of 30 days each.
Bootstrap logistic regression analysis was used to relate mortality of mussels at each salinity treatment (S). The logistic regression curve was fitted by bootstrapping 1000 random samples, and the LD50 and LD95 values were determined according to the logistic model:
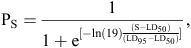
where PS is the proportion (P) of mussels that died at each salinity concentration (S), and LD50 and LD95 are the salinity concentrations at which 50 and 95% of the total sample of mussels died, respectively. This model was developed from Kelly (2001) and White et al. (2002) and has been utilised in several length-at-maturity growth studies and the lethal salinity toxicity study of Beatty et al. (2011). Bootstrapping was also used to determine upper and lower 95% confidence intervals (CI) of the parameters being estimated. As experiments were conducted at different times for the two different populations of mussels, we were not able to statistically test differences among populations in LD50 or LD95 values.
Results
Distribution
The current distribution of W. carteri includes freshwater streams, rivers, reservoirs and lakes within 50–100 km of the coast, from Gingin Brook southward to the Kent River, Goodga River and Waychinicup River of south-western Australia (Fig. 1a). From historic records, W. carteri once extended from the Moore River and possibly as far north as the Gascoyne River (based on one museum record from ~1824) and inland to the Avon and Blackwood Rivers, bounded by the Bow River (Fig. 1b). Seventy-seven sites where W. carteri was historically present were able to be resampled, and the species had disappeared from 34% of these sites. The estimated current EOO of the species is 22 600 km2; historic EOO is 44 900 km2 and, from other surveyed sites, the species is absent from 275 500 km2 in the South West Coast Drainage Division (Fig. 1c).
Environmental predictors of distribution
From the full GLM model, containing all seven environmental variables, only three variables had a significant effect on model fit: salinity, availability of perennial water and total nitrogen (Table 2). The full model had a residual deviance of 262.9 on 291 degrees of freedom, indicating that no correction was necessary for overdispersion (the tendency of the data to show greater variability than predicted from the model). There was also no significant spatial autocorrelation of model residuals. From model-averaging results, the selection probability (i.e. the chance of being included in the best-fitting model) for salinity was 1.0, for availability of permanent water and total nitrogen 0.99 and for all other variables <0.60 (Table 2). VIFs were <2.2 for all variables (Table 2), suggesting that collinearity among environmental variables was unlikely to have biased the results. The decision tree analysis (Fig. 2) indicated that mussels were very rarely found at sites with mean salinity concentrations of >1.62 g L–1, were never found at sites that did not have perennial water availability, and were more common at sites with mean total nitrogen concentrations of <0.69 mg L–1.
![]() |
For the 41 historic sites where changes in mussel distribution could be related to environmental variables, all had perennial water, leaving six variables as potential predictors. The only variable with a significant effect on model fit was salinity (P < 0.0001; residual deviance 3.81 on 35 degrees of freedom). There was no significant spatial autocorrelation of model residuals. From model-averaging results, the selection probability for salinity was 0.94, and for all other variables it was <0.48. The mean salinity of 16 sites at which mussels were historically present but are now absent was 5.65 g L–1, compared with a mean salinity of 0.48 g L–1 (±0.30 g L–1) for 42 sites where mussels were historically present and still occur (significantly different by t-test, t56 = 9.39, P < 0.0001). To examine regional variation in this trend, data for catchments on the south coast (i.e. those flowing into the Southern Ocean) and west coast (i.e. those flowing into the Indian Ocean) were analysed separately. In both regions, salinities were significantly greater in sites where mussels were historically present but are now absent than in sites where mussels were historically present and still occur (south coast sites, t7 = 3.71, P = 0.008; west coast sites, t47 = 8.78, P < 0.0001).
Salinity tolerance experiments
Results of salinity tolerance experiments are illustrated graphically in Fig. 3. Collie River mussels that were exposed to salinities of <0.5, 1 and 2 g L–1 experienced virtually no mortality, but those exposed to 3 g L–1 had a cumulative mortality of 38% and none survived the 4 g L–1 treatment. Mussels from Yalyal Brook that were exposed to salinities of <0.5 and 1 g L–1 experienced very low mortality, but those that were exposed to 2 g L–1 and 3–4 g L–1 had cumulative mortalities of 43.7% and 100%, respectively.
From the logistic regression curves, the LD50 value for mussels from the Collie River was 3.04 g L–1 (95% CI = 2.80–3.30 g L–1) and the LD95 value was 4.25 g L–1 (95% CI = 3.13–5.47) g L–1. The LD50 and LD95 values for mussels from Yalyal Brook were 1.29 g L–1 (95% CI = 0.74–1.80 g L–1) and 3.57 g L–1 (95% CI = 2.87–4.38 g L–1), respectively. As the salinity tolerance of mussels from the Collie River and Yalyal Brook were tested in different experiments, it is not appropriate to directly compare their LD50 and LD95 values. The lower values for mussels from Yalyal Brook may have been a consequence of the initial mortality that was seen in all treatments.
Discussion
Salinity as a threatening process
Of the variables that were measured in the ecological models, salinity was the most important in explaining the current distribution of W. carteri. Determinants of current species distribution, however, do not necessarily provide a guide to either past causes of population decline or future environmental threats (Hortal et al. 2008). An important aspect of our study was the ability to infer environmental factors responsible for past range reductions of W. carteri, by resampling sites at which mussels had previously been present, as determined from museum records. Salinity, as well as being an important predictor of current distribution, was by far the most important variable explaining the reduction in EOO from historic levels.
The identification of salinity as the most important predictor variable of both the current distribution of W. carteri and the difference in distribution between current and historical records provides strong circumstantial evidence that it represents a major threatening process for the species. Inferring causation, however, is still compromised by the possibility of correlations between salinity and other (unmeasured) environmental variables. For W. carteri, a causative role for salinity was supported by salinity tolerance experiments that showed that the species is very sensitive to acute changes in salinity, with a LD50 for adult mussels of between ~1.3 and 3.0 g L–1. This level of salinity tolerance is consistent with results from the field, where regression tree analysis indicated that mussels were almost never found in sites where the mean salinity was greater than 1.6 g L–1. The difference in salinity tolerance between populations of W. carteri from rivers with different levels of salinisation suggests that some adaptation to small increases in salinity may have occurred. The salinity tolerance of mussels from these different populations was not, however, directly compared in this study and further research is necessary to determine the extent to which salinity tolerance may vary among populations of freshwater mussels.
Secondary salinisation of freshwater ecosystems is a major environmental problem in arid and semi-arid areas of the world, including Australia, Central and South America, large tracts of northern and southern Africa, the Middle East and central Asia (Cañedo-Argüelles et al. 2013). In Australia, secondary salinisation is principally caused by rising watertables as a consequence of reduced evapotranspiration following changes in land use, typically land clearing for agriculture. The south-west corner of Australia, where ~80–90% of the land has been cleared, is a hot spot in terms of secondary salinisation (NLWRA 2001; Halse et al. 2003; Pinder et al. 2004). As a result, most of the freshwater ecosystems in south-western Australia are affected by increasing salinity, with 56% having become brackish (1.0–2.0 g L–1) or saline (>2.0 g L–1) (see Mayer et al. 2005). This trend is also evident from substantial range expansions of normally estuarine organisms (Smith 1996; Morgan et al. 2003; Beatty et al. 2011), including the estuarine bivalve Fluviolanatus subtorta (Kendrick 1976; Pen 1999; Klunzinger et al. 2012b).
Most Australian freshwater mussels are assumed to be generally intolerant of exposure to salinities above 3 g L–1 (Walker et al. 2001), although few studies of their salinity tolerance have been published. Both Alathyria jacksoni and Velesunio ambiguus, species that are widespread in eastern Australia, apparently withstand slightly elevated salinity exposures for at least brief periods (Walker 1981). Dryland salinity (i.e. secondary salinisation) has also been suggested as a limiting factor for Hyridella species from south-eastern Australia (Walker et al. 2001, 2014; Playford and Walker 2008; Jones and Byrne 2014). During 2010, at least two cases of freshwater mussel deaths were the result of low freshwater flow into lower reaches of rivers in south-western Australia (Canning River) and south-eastern Australia (Murray River) which resulted in the upstream movement of salt wedges from estuaries, causing mass losses of W. carteri and V. ambiguus, respectively (Brainwood et al. 2014). Velesunio angasi has been known to experience similar mass mortality events from estuarine influence in northern Australia (Dr Clint McCullough, pers. comm.).
Other threatening processes
Apart from salinity, perenniality of stream flow was the other major limiting variable in the distribution of W. carteri, suggesting that habitat drying, inadequate provision of environmental stream flows and dewatering could pose further conservation constraints on the species. To a lesser extent, total nitrogen was a limiting variable on the distribution of W. carteri, suggesting that nutrient pollution may be an important threatening process, although the effect of nutrients on the species is probably indirect, as suggested for other species of freshwater mussels (cf. Haag 2013). At a finer scale, reductions in localised numbers of W. carteri have been attributed to rapid drawdown of impoundments, leading to stranding and mortality from exposure, barriers (e.g. dams and weirs) that may inhibit dispersal by host fishes, smothering by mobilised sediments, trampling and habitat destruction by livestock, and predation by alien species (Walker et al. 2014).
Other factors have also been implicated in the decline and loss of Australian hyriids (e.g. Walker et al. 2014). These include river diversion and the loss of connectivity between subpopulations, altered flow regimes, cold water releases from large impoundments resulting in interrupted breeding cycles, scouring and exposure to fast currents with the loss of in-stream woody debris, pollution from pesticides, hydrocarbons, mining wastes and nutrients, acid sulfate soil mobilisation, loss of riparian vegetation, low dissolved oxygen, and invasive aquatic weed growth.
Conservation status and management implications
This study has provided the first comprehensive analysis of the distribution of W. carteri, the only species of freshwater mussel in south-western Australia. Given that the EOO of the species has declined by 49% in less than 50 years (less than three generations), due principally to secondary salinisation, the effects of which are expected to continue into the future (Williams 2001; Mayer et al. 2005; Beatty et al. 2011), we suggest that this qualifies the species as vulnerable under criterion A2c of the IUCN Red List Guidelines. This status was recently adopted by the IUCN Red List Mollusc Specialist Group (cf. Klunzinger and Walker 2014) and the species was listed as threatened under the Western Australian Wildlife Conservation Act 1950 (State of Western Australia 2014) following nomination with supporting data from this study. Westralunio carteri also qualifies for listing as vulnerable under the Commonwealth of Australia Environment Protection and Biodiversity Conservation Act 1999.
Maintaining existing habitats and population strongholds for W. carteri and mitigating the effects of secondary salinisation is paramount for the survival of the species. Quantification of dehydration tolerance and toxicology studies of nutrients (e.g. nitrite, ammonia etc.) is needed to further refine knowledge of distribution constraints and, thus, the threatening processes for W. carteri. Its vulnerable status should drive further conservation research and management priorities for this, the sole endemic freshwater mussel of south-western Australia. Supplementary data are available online to accompany this publication and assist resource managers in this respect.
Acknowledgements
Permits for field and laboratory studies were obtained from the Western Australian Department of Environment and Conservation (SF007049) and Department of Fisheries (1724-2010-06). Spatial hydrology data were supplied under licence by the Western Australian Department of Water. This work was supported by a Murdoch University Ph.D. Scholarship, a grant from the Holsworth Wildlife Research Endowment and a community partnership grant from Lotterywest and the South East Regional Centre for Urban Landcare (SERCUL). We are grateful to community members for information through the ‘Mussel Watch WA’ website (www.musselwatchwa.com) and to Robyn Walters for designing the web page. We especially thank Pat Hart, Julie Robert and the SERCUL executive board for their support, and Mark Allen, Iain Foster, James Keleher, Rowan and Samuel Lymbery, Jon Murphy and SERCUL staff for assistance in field surveys. Supplementary mapping (Fig. S1) of the distribution of W. carteri was produced in collaboration with Chris Meakin (Environmental Resource Information Network, Department of Sustainability, Environment, Water, Population and Communities, Commonwealth of Australia).
References
Aldridge, D. C., Fayle, T. M., and Jackson, N. (2007). Freshwater mussel abundance predicts biodiversity in UK lowland rivers. Aquatic Conservation: Marine and Freshwater Ecosystems 17, 554–564.| Freshwater mussel abundance predicts biodiversity in UK lowland rivers.Crossref | GoogleScholarGoogle Scholar |
AWRC (1976). ‘Review of Australia’s Water Resources 1975’. (Australian Water Resources Council (AWRC), Department of Natural Resources: Canberra.)
Bartoñ, K. (2013). ‘MuMIn: Multi-model Inference. Version 1.9.5’. Available at: http://cran.r-project.org/web/packages/MuMIn [Accessed on 01 September 2013].
Beatty, S. J., Morgan, D. L., Rashnavadi, M., and Lymbery, A. J. (2011). Salinity tolerances of endemic freshwater fishes of south-western Australia: implications for conservation in a biodiversity hotspot. Marine and Freshwater Research 62, 91–100.
| Salinity tolerances of endemic freshwater fishes of south-western Australia: implications for conservation in a biodiversity hotspot.Crossref | GoogleScholarGoogle Scholar | 1:CAS:528:DC%2BC3MXmtFGlsg%3D%3D&md5=2c683c0dabbcd679cd9df47f8b853d1dCAS |
Bogan, A. E., and Roe, K. J. (2008). Freshwater bivalve (Unioniformes) diversity, systematics, and evolution: status and future directions. Journal of the North American Benthological Society 27, 349–369.
| Freshwater bivalve (Unioniformes) diversity, systematics, and evolution: status and future directions.Crossref | GoogleScholarGoogle Scholar |
Brainwood, M. A., Klunzinger, M. W., and Walker, K. F. (2014). Now you see them, soon you won’t—freshwater mussel conservation in Australia. Australian Society for Fish Biology and Australian Society of Limnology Congress, 30 June – 4 July, 2014, Darwin, Northern Territory, Australia. (Abstract #13410).
Burnham, K. P., and Anderson, D. R. (2002). ‘Model Selection and Multi-Model Inference: A Practical Information-Theoretic Approach’. (Springer: New Jersey.)
Cañedo-Argüelles, M., Kefford, B. J., Piscart, C., Prat, N., Schäfer, R. B., and Schulz, C. J. (2013). Salinisation of rivers: An urgent ecological issue. Environmental Pollution 173, 157–167.
| Salinisation of rivers: An urgent ecological issue.Crossref | GoogleScholarGoogle Scholar | 23202646PubMed |
Cardoso, P., Borges, P. A. V., Triantis, K. A., Ferrández, M. A., and Martín, J. L. (2012). The underrepresentation and misrepresentation of invertebrates in the IUCN Red List. Biological Conservation 149, 147–148.
| The underrepresentation and misrepresentation of invertebrates in the IUCN Red List.Crossref | GoogleScholarGoogle Scholar |
Dormann, C. F., McPherson, J. M., Araújo, M. B., Bivand, R., Bolliger, J., Carl, G., Davies, R. G., Hirzel, A., Jetz, W., Kissling, W. D., Kühn, I., Ohlemüller, R., Peres-Neto, P. R., Reineking, B., Schröder, B., Schurr, F. M., and Wilson, R. (2007). Methods to account for spatial autocorrelation in the analysis of distributional species data: A review. Ecography 30, 609–628.
| Methods to account for spatial autocorrelation in the analysis of distributional species data: A review.Crossref | GoogleScholarGoogle Scholar |
Elith, J., and Leathwick, J. (2009). Species distribution models: Ecological explanation and prediction across space and time. Annual Review of Ecology, Evolution and Systematics 40, 677–697.
| Species distribution models: Ecological explanation and prediction across space and time.Crossref | GoogleScholarGoogle Scholar |
Freckleton, R. P. (2011). Dealing with collinearity in behavioural and ecological data: model averaging and the problems of measurement error. Behavioral Ecology and Sociobiology 65, 91–101.
| Dealing with collinearity in behavioural and ecological data: model averaging and the problems of measurement error.Crossref | GoogleScholarGoogle Scholar |
Geoscience Australia (2003). ‘GEODATA TOPO 250K, Series 2’. (National Mapping Division, Commonwealth of Australia Department of Industry, Tourism and Resources: Canberra.)
Graf, D. L. (2013). Patterns of freshwater bivalve global diversity and the state of phylogenetic studies on the Unionoida, Sphaeriidae, and Cyrenidae. American Malacological Bulletin 31, 135–153.
| Patterns of freshwater bivalve global diversity and the state of phylogenetic studies on the Unionoida, Sphaeriidae, and Cyrenidae.Crossref | GoogleScholarGoogle Scholar |
Graf, D. L., Jones, H., Geneva, A. J., Pfeiffer, J. M., and Klunzinger, M. W. (2015). Molecular phylogenetic analysis supports a Gondwanan origin of the Hyriidae (Mollusca: Bivalvia: Unionida) and the paraphyly of Australasian taxa. Molecular Phylogenetics and Evolution 85, 1–9.
| Molecular phylogenetic analysis supports a Gondwanan origin of the Hyriidae (Mollusca: Bivalvia: Unionida) and the paraphyly of Australasian taxa.Crossref | GoogleScholarGoogle Scholar | 25659337PubMed |
Haag, W. R. (2013). ‘North American Freshwater Mussels: Natural History, Ecology and Conservation.’ (Cambridge University Press: New York.)
Halse, S. A., Ruprecht, J., and Pinder, A. M. (2003). Salinisation and prospects for biodiversity in rivers and wetlands of south-west Western Australia. Australian Journal of Botany 51, 673–688.
| Salinisation and prospects for biodiversity in rivers and wetlands of south-west Western Australia.Crossref | GoogleScholarGoogle Scholar |
Heiberger, R. M. (2013). ‘HH: Statistical Analysis and Data Display. Version 2.3–37.’ Available at: http://cran.r-project.org/web/packages/HH/ [accessed 01 September 2013].
Hortal, J., Jiménez-Valverde, J., Goméz, J. F., Lobo, J. M., and Baselga, A. (2008). Historical bias in biodiversity inventories affects the observed environmental niche of the species. Oikos 117, 847–858.
| Historical bias in biodiversity inventories affects the observed environmental niche of the species.Crossref | GoogleScholarGoogle Scholar |
Iredale, T. (1934). The freshwater mussels of Australia. Type species: Westralunio ambiguus carteri. Australian Zoologist 8, 57–78.
IUCN (1996). Mollusc Specialist Group 1996. Westralunio carteri. In ‘International Union for the Conservation of Nature Red List of Threatened Species. Version 2.3’. Available at: http://www.iucnredlist.org. [accessed 01 June 2009].
IUCN (2013). ‘Guidelines for using the IUCN Red List categories and criteria. Version 10’. Available at: http://www.iucnredlist.org/documents/RedListGuidelines.pdf. [accessed 01 May 2013].
Jones, H. A., and Byrne, M. (2014). Changes in the distributions of freshwater mussels (Unionoida: Hyriidae) in coastal south-eastern Australia and implications for their conservation status. Aquatic Conservation: Marine and Freshwater Ecosystems 24, 203–217.
| Changes in the distributions of freshwater mussels (Unionoida: Hyriidae) in coastal south-eastern Australia and implications for their conservation status.Crossref | GoogleScholarGoogle Scholar |
Kelly, G. E. (2001). The median lethal dose – design and estimation. The Statistician 50, 41–50.
| The median lethal dose – design and estimation.Crossref | GoogleScholarGoogle Scholar |
Kendrick, G. W. (1976). The Avon: faunal and other notes on a dying river in south-western Australia. Western Australian Naturalist (Perth) 13, 97–114.
Klunzinger, M., and Walker, K. F. (2014). Westralunio carteri. In ‘IUCN Red List of Threatened Species. Version 2014.3’. Available at: www.iucnredlist.org. [accessed 21 December 2014].
Klunzinger, M. W., Beatty, S. J., Morgan, D. L., Thomson, G. J., and Lymbery, A. J. (2012a). Glochidia ecology in wild fish populations and laboratory determination of competent host fishes for an endemic freshwater mussel of south-western Australia. Australian Journal of Zoology 60, 26–36.
| Glochidia ecology in wild fish populations and laboratory determination of competent host fishes for an endemic freshwater mussel of south-western Australia.Crossref | GoogleScholarGoogle Scholar |
Klunzinger, M. W., Beatty, S. J., Morgan, D. L., Lymbery, A. J., Pinder, A. M., and Cale, D. J. (2012b). Distribution of Westralunio carteri Iredale 1934 (Bivalvia: Unionoida: Hyriidae) on the south coast of southwestern Australia, including new records of the species. Journal of the Royal Society of Western Australia 95, 77–81.
Klunzinger, M. W., Thomson, G. J., Beatty, S. J., Morgan, D. L., and Lymbery, A. J. (2013). Morphological and morphometrical description of the glochidia of Westralunio carteri Iredale, 1934 (Bivalvia: Unionoida: Hyriidae). Molluscan Research 33, 104–109.
| Morphological and morphometrical description of the glochidia of Westralunio carteri Iredale, 1934 (Bivalvia: Unionoida: Hyriidae).Crossref | GoogleScholarGoogle Scholar |
Klunzinger, M. W., Beatty, S. J., Morgan, D. L., Lymbery, A. J., and Haag, W. R. (2014). Age and growth in the Australian freshwater mussel, Westralunio carteri, with an evaluation of the fluorochrome calcein for validating the assumption of annulus formation. Freshwater Science 33, 1127–1135.
| Age and growth in the Australian freshwater mussel, Westralunio carteri, with an evaluation of the fluorochrome calcein for validating the assumption of annulus formation.Crossref | GoogleScholarGoogle Scholar |
Köhler, F. (2011). Westralunio carteri. In ‘IUCN Red List of Threatened Species. Version 2011.2’. Available at: http://www.iucnredlist.org. [accessed 03 February 2012].
Lamoreux, J., Akçakaya, H. R., Bennun, L., Collar, N. J., Boitani, L., Brackett, D., Brautigam, A., Brooks, T. M., da Fonseca, G. A. B., Mittermeier, R. A., Rylands, A. B., Gärdenfors, U., Hilton-Taylor, C., Mace, G., Stein, B. A., and Stuart, S. (2003). Value of the IUCN Red List. Trends in Ecology & Evolution 18, 214–215.
| Value of the IUCN Red List.Crossref | GoogleScholarGoogle Scholar |
Leathwick, J. R., Rowe, D., Richardson, J., Elith, J., and Hastie, T. (2005). Using multivariate adaptive regression splines to predict the distributions of New Zealand’s freshwater diadromous fish. Freshwater Biology 50, 2034–2052.
| Using multivariate adaptive regression splines to predict the distributions of New Zealand’s freshwater diadromous fish.Crossref | GoogleScholarGoogle Scholar |
Lopes-Lima, M., Teixiera, A., Froufe, E., Lopes, A., Varandas, S., and Sousa, R. (2014). Biology and conservation of freshwater bivalves: past, present and future perspectives. Hydrobiologia 735, 1–13.
| Biology and conservation of freshwater bivalves: past, present and future perspectives.Crossref | GoogleScholarGoogle Scholar |
Mayer, X., Ruprecht, J., and Bari, M. (2005). Stream salinity status and trends in southwest Western Australia. Salinity and Land Use Impacts. SLUI 38. Department of Environment, Perth.
McMichael, D. F., and Hiscock, I. D. (1958). A monograph of the freshwater mussels (Mollusca: Pelecypoda) of the Australian region. Australian Journal of Marine and Freshwater Research 9, 372–507.
| A monograph of the freshwater mussels (Mollusca: Pelecypoda) of the Australian region.Crossref | GoogleScholarGoogle Scholar |
Montgomery, D. C., and Peck, E. A. (1992). ‘Introduction to Linear Regression Analysis. ’ 2nd edn. (John Wiley & Sons: New York.)
Morgan, D. L., Thorburn, D. C., and Gill, H. S. (2003). Salinization of southwestern Western Australian rivers and the implications for the inland fish fauna – the Blackwood River, a case study. Pacific Conservation Biology 9, 161–171.
Murdoch University and SERCUL (2010). ‘Mussel Watch Western Australia.’ Archived by PANDORA, Australia’s Web Archive. National Library of Australia and Partners. Available at: http://nla.gov.au/nla.arc-131363. [archived 13 January 2012].
Murray, K., and Connor, M. M. (2009). Methods to quantify variable importance: implications for the analysis of noisy ecological data. Ecology 90, 348–355.
| Methods to quantify variable importance: implications for the analysis of noisy ecological data.Crossref | GoogleScholarGoogle Scholar | 19323218PubMed |
Myers, R. (1990). ‘Classical and Modern Regression with Applications.’ (Duxbury: Boston.)
Newton, A. C. (2010). Use of a Bayesian network for Red Listing under uncertainty. Environmental Modelling & Software 25, 15–23.
| Use of a Bayesian network for Red Listing under uncertainty.Crossref | GoogleScholarGoogle Scholar |
NLWRA (2001). Australian Dryland Salinity Assessment 2000: extent, impacts, processes, monitoring and management options. National Land and Water Resources Audit (NLWRA), Canberra.
Partridge, G. J., Lymbery, A. J., and George, R. J. (2008). Finfish mariculture in inland Australia: a review of potential water sources, species and production platforms. Journal of the World Aquaculture Society 39, 291–310.
| Finfish mariculture in inland Australia: a review of potential water sources, species and production platforms.Crossref | GoogleScholarGoogle Scholar |
Pen, L. J. (1999). ‘Managing our Rivers. A Guide to the Nature and Management of the Streams of South-West Western Australia.’ (Water and Rivers Commission: East Perth.)
Pinder, A. M., Halse, S. A., McRae, J. M., and Shiel, R. J. (2004). Aquatic invertebrate assemblages of wetlands and rivers in the wheat belt region of Western Australia. Records of the Western Australian Museum, , 7–37.
Playford, T. J., and Walker, K. F. (2008). Status of the endangered Glenelg River mussel Hyridella glenelgensis (Unionoida: Hyriidae) in Australia. Aquatic Conservation: Marine and Freshwater Ecosystems 18, 679–691.
| Status of the endangered Glenelg River mussel Hyridella glenelgensis (Unionoida: Hyriidae) in Australia.Crossref | GoogleScholarGoogle Scholar |
R Development Core Team (2013). ‘R: A Language and Environment for Statistical Computing. Version 3.0.1’. (R Foundation for Statistical Computing: Vienna.)
Rodrigues, A. S. L., Pilgrim, J. D., Lamoreux, J. F., Hoffmann, M., and Brooks, T. M. (2006). The value of the IUCN Red List for conservation. Trends in Ecology & Evolution 21, 71–76.
| The value of the IUCN Red List for conservation.Crossref | GoogleScholarGoogle Scholar |
Seddon, M., Darwall, M., Bohn, M., Allen, D., and Smith, K. (2012). Update on the status of the Global Freshwater Mollusc Assessment. In ‘International Meeting on Biology and Conservation of Freshwater Bivalves: Book of Abstracts’. (Eds A. Teixiera, M. Lopes-Lima, S. Varandas, R. Sousa, E. Froufe and F. Teiga.) p. 30. (School of Agriculture, Polytechnic Institute of Bragança: Bragança, Portugal.)
Smith, T. G. (1996). The water quality and invertebrate fauna of the Avon River: a highly saline but productive aquatic ecosystem. B.Sc.(Honours) Thesis, Murdoch University, Perth.
Spooner, D. E., and Vaughn, C. C. (2006). Context‐dependent effects of freshwater mussels on stream benthic communities. Freshwater Biology 51, 1016–1024.
| Context‐dependent effects of freshwater mussels on stream benthic communities.Crossref | GoogleScholarGoogle Scholar | 1:CAS:528:DC%2BD28XmvVymsrw%3D&md5=4b06e9b42fe5cf9f98f55c7205ebd92cCAS |
State of Western Australia (2014). Division 6 – Molluscs: 169. Westralunio carteri – Carter’s Freshwater Mussel. In ‘Wildlife Conservation (Specially Protected Fauna) Notice 2014 – Schedule 1 – Fauna that is Rare or is Likely to Become Extinct’. p. 4477. Government Gazette, WA: 2 December, 2014: No. 188. State Law Publisher of Western Australia, Perth.
Walker, K. F. 1981. ‘Ecology of Freshwater Mussels in the River Murray.’ (Australian Water Resources Council: Canberra.)
Walker, K. F., Byrne, M., Hickey, C. W., and Roper, D. S. (2001). Freshwater mussels (Hyriidae) of Australasia. In ‘Ecology and Evolution of the Freshwater Mussels Unionoida’. (Eds G. Bauer and K. Wächtler.) pp. 5–31. (Springer: Berlin.)
Walker, K. F., Jones, H. A., and Klunzinger, M. W. (2014). Bivalves in a bottleneck: taxonomy, phylogeography and conservation of freshwater mussels (Bivalvia: Unionoida) in Australasia. Hydrobiologia 735, 61–79.
| Bivalves in a bottleneck: taxonomy, phylogeography and conservation of freshwater mussels (Bivalvia: Unionoida) in Australasia.Crossref | GoogleScholarGoogle Scholar |
White, W. T., Hall, N. G., and Potter, I. C. (2002). Size and age compositions and reproductive biology of the nervous shark Carcharhinus cautus in a large subtropical embayment, including an analysis of growth during pre- and postnatal life. Marine Biology 141, 1153–1164.
| Size and age compositions and reproductive biology of the nervous shark Carcharhinus cautus in a large subtropical embayment, including an analysis of growth during pre- and postnatal life.Crossref | GoogleScholarGoogle Scholar |
Williams, W. D. (2001). Anthropogenic salinisation of inland waters. Hydrobiologia 466, 329–337.
| Anthropogenic salinisation of inland waters.Crossref | GoogleScholarGoogle Scholar |
Williams, W. D., Taaffe, R. G., and Boulton, A. J. (1991). Longitudinal distribution of macroinvertebrates in two rivers subject to salinisation. Hydrobiologia 210, 151–160.
| Longitudinal distribution of macroinvertebrates in two rivers subject to salinisation.Crossref | GoogleScholarGoogle Scholar |
Wintle, B. A., Elith, J., and Potts, J. M. (2005). Fauna habitat modelling and mapping: a review and case study in the Lower Hunter Central Coast region of NSW. Austral Ecology 30, 719–738.
| Fauna habitat modelling and mapping: a review and case study in the Lower Hunter Central Coast region of NSW.Crossref | GoogleScholarGoogle Scholar |