The nutritional quality of post-fire eucalypt regrowth and its consumption by koalas in the New South Wales Southern Tablelands
Murraya R. Lane
A
B
C
Abstract
Most forests and woodlands of south-eastern Australia are fire prone. These landscapes are typically dominated by tree species of the genus Eucalyptus, and many of these are proficient epicormic resprouters after fire. Several marsupial folivores rely on Eucalyptus foliage, including the koala. Little is known about the nutritional composition of epicormic leaves and whether folivores can utilise them. Following the 2019–20 megafires, we offered koalas epicormic leaves from eight eucalypt species that occur in the New South Wales Southern Tablelands and measured concentrations of foliar chemical constituents known to influence koala feeding (total and available nitrogen, formylated phloroglucinol compounds (FPCs), and unsubstituted B-ring flavanones (UBFs)). Captive koalas ate epicormic leaves from the four eucalypt species belonging to the subgenus Symphyomyrtus but ate relatively little from the species of the subgenus Eucalyptus. Available nitrogen was significantly higher in symphyomyrtle epicormic regrowth than in monocalypts and differed between species. Concentrations of FPCs and UBFs also differed between eucalypt species. Our results suggest that post-fire epicormic regrowth from symphyomyrtle species may be more readily browsed than that of monocalypts by koalas in the NSW Southern Tablelands. Therefore, some burnt areas are likely to be better quality habitat for koalas than others.
Keywords: browse quality, bushfire, dry matter intake, epicormic leaves, herbivory, marsupial folivores, nitrogen, plant secondary metabolites.
Introduction
Fires can cause major disturbance to ecosystems (Vernes et al. 2001) and are a common occurrence in the Australian landscape. The dry sclerophyll forests in south-eastern Australia are considered to be one of the most fire-prone areas in the world (Melzer et al. 2000; Penman et al. 2007; Russell-Smith et al. 2007; Attiwill and Adams 2013). Several arboreal marsupial species utilise the eucalypt forests of south-eastern Australia and feed on the foliage of a variety of eucalypt species. These include: the common brushtail possum (Trichosurus vulpecula), the common ringtail possum (Pseudocheirus peregrinus), the southern greater glider (Petauroides volans) and the koala (Phascolarctos cinereus) (Moore et al. 2004a). Their distribution throughout eucalypt forests and woodlands is patchy, and this is thought to be, in part, due to nutritional factors (Moore et al. 2004b).
Eucalypt foliage, like other plant material, can vary considerably in the types and/or concentrations of plant nutrients and herbivore-deterrent plant secondary metabolites (PSMs) both between and within species (Lawler et al. 1998, 2000; Moore et al. 2004a; Wallis et al. 2010). This creates a nutritionally complex landscape that can influence diet selection and folivore distribution (Jensen et al. 2014; Au et al. 2019). There has been much research on how chemical constituents in the mature foliage of eucalypts influence feeding by browsing marsupials (Marsh et al. 2003a, 2007; Moore et al. 2005; Beale et al. 2022), but little is known about the chemical composition of eucalypt post-fire regrowth and whether it is utilised by arboreal folivores after fire.
Resprouting after fire is a key functional trait and strategy for many plant species (Clarke et al. 2013; He 2014; Pausas et al. 2016), and, as a group, eucalypts are considered to be the most proficient and successful epicormic resprouters (Burrows 2002, 2008b; Waters et al. 2010; Bradstock et al. 2012; Clarke et al. 2013). In many eucalypt species, after fire, numerous buds can develop from meristems across the entire bark at different depths (Burrows 2008a, 2013; Waters et al. 2010; Bradstock et al. 2012), enabling them to produce large numbers of shoots on both the bole and branches of the tree (Clarke et al. 2013). Epicormic resprouters are also able to help maintain tree growth and structure following fire; therefore, the production of epicormic regrowth enables a relatively rapid recovery of forests and woodlands from fire (Pausas and Keeley 2017).
The 2019–20 Australian megafires were unprecedented in duration, severity and scale, with over 12 million ha burnt between August 2019 and March 2020 (Wintle et al. 2020). Fires can have benefits for biodiversity (Gill 1994; Bowman and Murphy 2010), but the intensity and severity of the 2019–20 bushfires caused large areas of habitat to be significantly altered, including more than a quarter of the remaining, highly suitable koala habitat (or 3.5 million ha) in New South Wales (NSW) (State of NSW and Department of Planning Industry and Environment 2020). In many areas, there were few unburnt refuges for wildlife (State of NSW and Department of Planning Industry and Environment 2020), increasing the need to understand how post-fire conditions affect wildlife and their habitat.
After severe fire, epicormic leaves are normally the first type of growth available (Pausas and Keeley 2017), and yet there have been no studies on the nutritional composition of epicormic leaves for koalas and how this may differ within and between species and from mature foliage. Some studies have observed koalas occupying burnt areas and feeding in trees with epicormic growth (Lunney et al. 2004; Matthews et al. 2007). However, little is known about the extent to which they feed on them or whether the epicormic leaves from all eucalypt species are equally palatable. This information is critical to understanding how koalas may utilise a burnt landscape, whether koalas recovering in care can be supported by epicormic leaves and when a landscape may be suitable for koalas to be released.
The aim of this study was to quantify whether captive koalas would consume epicormic leaves from eight common eucalypt species in the NSW Southern Tablelands (E. rossii, E. mannifera, E. dives, E. macrorhyncha, E. radiata, E. viminalis, E. rubida and E. bridgesiana). We also compared the nutritional composition of epicormic foliage with mature foliage from the same species collected as part of an earlier study on the nutritional quality of foliage for koalas (Au 2018). A number of foliar constituents are known to influence the feeding choices of eucalypt folivores and the digestibility of essential nutrients (Wallis et al. 2010). These include specific PSMs: formylated phloroglucinol compounds (FPCs) in eucalypt species from the subgenus Symphyomyrtus (common name symphyomyrtle), unsubstituted B-ring flavanones (UBFs) in species from the subgenus Eucalyptus (common name monocalypt), and tannins (Marsh et al. 2003b, 2019; Moore et al. 2005). Foliar nitrogen (N) (as a proxy for protein) is considered a key limiting nutrient for many eucalypt folivores, and marsupial browsers often select food that is higher in digestible protein and lower in PSMs (Moore and Foley 2005; Stalenberg et al. 2014; Au 2018). Understanding the nutritional quality of epicormic regrowth and whether koalas can consume the regrowth has important implications for determining whether fire-affected landscapes can aid in supporting koala populations. This information can also be used to guide decision-making about when it is safe, from a nutritional perspective, to release koalas that were rescued from wildfires.
Methods
Study animals and housing
Four adult male koalas were kept in individual outdoor roofed enclosures (approximately 2.5 m × 2.5 m × 3 m, (w × d × h)) containing multiple vertical and angled trunks and branches with forks. The koalas had been rescued from the vicinity of Peak View, NSW, Australia (36.07°S, 149.38°E) after a severe fire burnt through the area in January 2020. Koalas are aged based on the wear of the fourth upper premolars, according to Martin (1981), on a scale of 1 (minimal wear; youngest animals) to 7 (root wear; oldest animals). The tooth wear of individual animals was between 2 and 4, and weights ranged from 4.9 to 7.8 kg. While in care, the koalas were offered a variety of eucalypt species found in their natural habitat. Fresh leaves were supplied daily by placing large stems into polythene tubes that were filled with water and secured to branches. Drinking water was available ad libitum.
Study design
The study was designed so that eight eucalypt species (Table 1) were offered separately in a series of experiments that were undertaken between 4 April and 12 June 2020. Four of the species belong to the subgenus Symphyomyrtus and the other four to the subgenus Eucalyptus (Table 1). The selected species are used to varying degrees by koalas in the Central and Southern Tablelands koala management area (KMA) for feeding, shelter and/or social purposes (State of NSW and Office of Environment and Heritage 2018) (Table 1).
Tree species | Subgenus | Tree use score | |
---|---|---|---|
E. bridgesiana | Symphyomyrtus | Irregular | |
E. mannifera | Symphyomyrtus | High | |
E. rubida | Symphyomyrtus | Irregular | |
E. viminalis | Symphyomyrtus | High | |
E. dives | Eucalyptus | High | |
E. macrorhyncha | Eucalyptus | High | |
E. radiata | Eucalyptus | Irregular | |
E. rossii | Eucalyptus | High |
Usage was classified based on relative koala tree ‘use levels’ for feeding, shelter and/or social purposes (State of NSW and Office of Environment and Heritage 2018).
The experiments were conducted as a series of 4 × 4 Latin squares, in which four sets of trees were offered to four koalas over four consecutive nights (Table 2). No koala received the same set of trees on the same night as any other koala, and all koalas were offered all four sets of trees for one night each. For all species except E. mannifera and E. rubida, there were three individual trees in each set. Each set always contained the same three trees, so that 12 individual trees were used for each experiment. The purpose of this was twofold: first, to encompass and control for potential variation between individual trees within species, and, second, to ensure that sufficient leaf could be harvested to provide in excess of voluntary intake for all four koalas.
Koala | Day | ||||
---|---|---|---|---|---|
1 | 2 | 3 | 4 | ||
1 | A | B | D | C | |
2 | B | C | A | D | |
3 | C | D | B | A | |
4 | D | A | C | B |
For Eucalyptus bridgesiana, E. macrorhyncha, E. radiata, E. rossii, E. viminalis and E. dives, each treatment consisted of a group of three trees that were always offered together: A = trees 1, 2 and 3; B = trees 4, 5 and 6; C = trees 7, 8 and 9; D = trees 10, 11 and 12. For E. mannifera and E. rubida, the first tree from each treatment was the same across all four nights. For the first two days, the second tree of the treatment was offered in conjunction with the first tree (e.g. A = trees 1 and 2) and on the last two days the last tree of the treatment was offered (e.g. A = trees 1 and 3).
For E. mannifera and E. rubida, we were unable to find 12 trees with enough epicormic growth for four consecutive nights. Instead, we used a modified version of the 4 × 4 Latin square (Table 2) with four trees that had enough growth for the four-night period and eight other trees that we broke into groups of two, whereby koalas received trees from the first group for the first two nights and trees from the other group for the last two nights. For those two species, we have four observations per tree for four trees and two observations per tree for the other eight trees. Therefore, for these two species, each koala received two individual trees per night rather than three.
Leaf collection
For each group of three trees, we selected a central tree, and two other trees that were within 25 m of the central tree. There was a minimum distance of 60 m between each group of trees to reduce the chance of genetic and chemical similarity between groups (Andrew et al. 2007). Individual trees were selected based on whether they had sufficient epicormic growth to feed four koalas for four consecutive nights in addition to providing enough leaf for control bunches and samples for nutritional analysis.
Leaf collection was undertaken between 0930 and 1230 hours on each day of the experiment. Epicormic growth was collected by either pulling it off the trunk by hand, or by using an extendable cutting pole if it was higher in the tree. Stems were placed into buckets of water within 30 min of collection and stored indoors until feeding in the late afternoon.
Leaf preparation and koala feeding
The epicormic growth from each tree was divided into two bunches: one to feed to koalas and the other served as a control to identify any weight changes in the absence of herbivory. At 1600 hours, bunches were weighed and the stems were placed into labelled plastic bags (35 cm × 25 cm) and filled with water so all stems were covered.
Each koala was provided with three separate bunches of epicormic leaves comprising their allocated set of trees for that day. Koalas were also offered their regular diet, which consisted of branches of mature and young leaves from a minimum of three of the following species: E. bridgesiana, E. rossii, E. mannifera, E. rubida and occasionally E. viminalis, E. pauciflora and E. dives. The control bunches were placed outside under cover to mimic the conditions in the koala enclosures.
The epicormic leaf bunches were removed from the koala enclosures the following morning between 0800 hours and 0830 hours and reweighed to determine each koala’s wet matter intake. Occasionally, a feeding bunch was tipped during the night so that stems were no longer sitting in water. These leaves were likely to have lost water mass during the night, and so when this happened, we removed all of the leaves from the stems before weighing only the stems. The leaves that had been removed and any other leaves that koalas had dropped onto the ground while feeding were collected into paper bags and dried at 60°C until constant mass to determine their dry weight. Control bunches were also reweighed, and a sample resembling that eaten by koalas (e.g. tips, leaves, stems or a combination) was removed from each control bunch and dried to constant mass to determine the percentage dry matter (% DM). A separate sample was collected and frozen at −20°C for later chemical analysis. Dry matter intake (DMI) per tree was calculated by multiplying the % DM by apparent wet matter intake and then subtracting the dry weight of dropped leaves for each tree. We also calculated nightly DMI of epicormic leaves for each koala by adding together the DMI of the two or three trees that were offered together.
Chemical analysis
Leaf samples were freeze-dried and then ground in a Foss Tecator 1093 Cyclotec sample mill (Foss Tecator, Höganäs, Sweden) with a 1 mm particle sieve. For all dried, ground foliage samples, we collected reflectance spectrum from 400 to 2498 nm using a lab-based Foss XDS NIR spectrometer (Foss-NIRsystems Inc., Silver Spring, MD, USA).
Total and available N were determined in a subset of samples (n = 73) using a modified version of the method of DeGabriel et al. (2008) as outlined in Marsh et al. (2014a). For both digested and undigested leaves, the N concentration was determined in 0.100 ± 0.020 g of sample using a Leco Truspec C/N analyser (Leco Corporation, Sydney, NSW, Australia). These values were used to calculate the in vitro N digestibility and available N.
We used Win ISI II, Version 10.2 (Intrasoft International, Port Matilda, Pennsylvania, USA) to develop calibrations from the NIR spectra to predict total and available N in all epicormic leaf samples using the lab values in the subset of chemically analysed samples for model training and testing. We applied a standard normal variate and detrend scatter correction to raw spectra to minimise noise and then used modified partial least square regression with cross-validation and further independent validation. The resulting calibration accurately predicted concentrations of both total N (r2 = 0.995, SECV = 0.063) and available N (r2 = 0.974, SECV = 0.174) in the training data set and in the independent validation set for N (r2 = 0.978, slope = 0.971, standard error of prediction (SEP) = 0.094) and available N (r2 = 0.869, slope = 0.998, and SEP = 0.331) Concentrations of UBFs and FPCs were predicted using the pre-existing calibrations made with mature eucalypt foliage described in Au (2018) and Marsh et al. (2019).
Comparison with mature leaves
Au (2018) measured concentrations of total N, available N, UBFs and FPCs in mature leaves of 2368 individual eucalypt trees, from 63 species across the range of the koala. Five of the sites included in that study covered the region from which the koalas in our study were rescued. We collated the data from those five sites and plotted the mean and range of concentrations of each nutritional constituent as an indication of the potential differences in nutritional composition between mature and epicormic leaves of each species. We did not statistically compare the chemical composition of epicormic leaves with mature leaves from the same species because the measurements could be partially influenced by the different individual trees sampled and slight differences in the analytical methods.
Data analysis
All analyses were completed using R statistical Software (v4.2.2) (R Core Team 2022). We tested for differences in daily DMI between eucalypt species and subgenera using a linear mixed-effects model with the lme4 package (v1.1.31) (Bates et al. 2015) and LmerTest (v3.1.3) (Kuznetsova et al. 2017). Daily DMI was the dependent variable, and the independent variables were eucalypt species and subgenera. We included koala and tree group as random effects to account for the experimental design. We used ANOVAs for comparisons between species and subgenera using the car package (v3.1.2) (Fox and Weisberg 2018). For post hoc analyses we used the emmeans package (v1.8.5) (Lenth 2023) to generate pairwise comparisons between the different eucalypt species and also to see the differences between the two subgenera. We used the square root of DMI so the residuals were normally distributed.
To look at chemistry differences in epicormic leaves between species, we ran linear regression models using the stats package (v4.2.2) (R Core Team 2022). We ran separate models with available N, FPCs and UBFs as the dependent variables Eucalypt species and subgenus were the independent variables in the available N model, whereas species was the only independent variable in the FPC and UBF models.
Results
Koalas ate between 0 and 95 g DM of epicormic leaves per night. Koalas ate more from some eucalypt species than others (F6,24 = 11.90, P < 0.001). On average, they ate most per day from epicormic E. viminalis and E. bridgesiana and the least from epicormic E. radiata and E. macrorhyncha (Fig. 1). There was also an effect of subgenus, with koalas eating over four times more from symphyomyrtle species than monocalypt species (F1,24 = 58.53, P < 0.001). All four koalas chose not to eat epicormic leaves from two individual E. radiata trees, eight E. macrorhyncha and one E. rubida.
Mean ± s.e. daily dry matter intake (DMI) of epicormic leaves from eight eucalypt species (n = 4 sets of trees offered to 4 koalas per species). Bars with different letters are significantly different from one another in post hoc testing (P < 0.05).
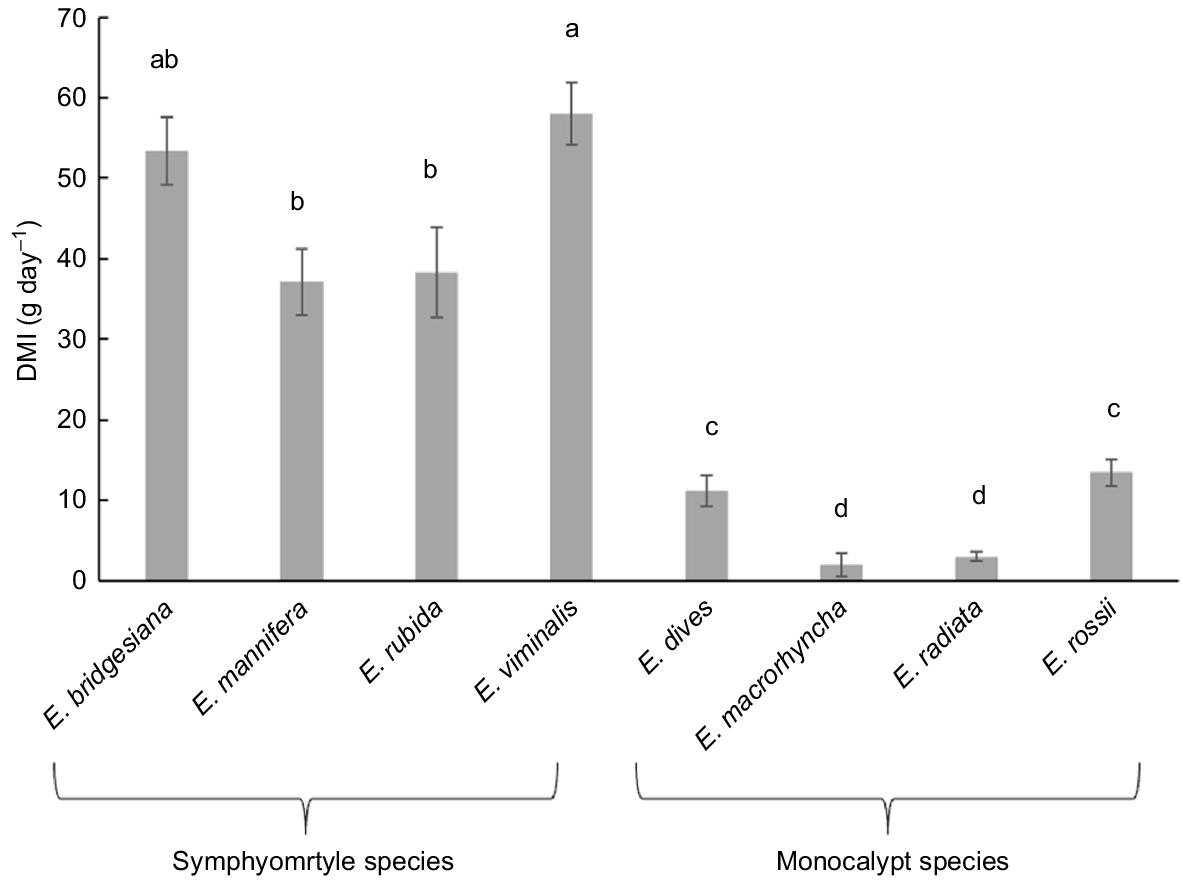
Chemistry of epicormic leaves
Total N concentrations in epicormic leaves ranged from 1.46% DM in an E. rossii individual to 3.97% DM in an individual E. rubida, and available N concentrations ranged from 0.07% DM in an E. macrorhyncha to 3.44% DM in an E. viminalis. Some eucalypt species contained higher concentrations of total N (F6,87 = 12.54, P < 0.001) (Fig. 2a; Table S1) and/or available N than others (F6,87 = 16.84, P < 0.001) (Fig. 2b; Table S1). In particular, on average, the epicormic leaves from symphyomyrtle species had higher concentrations of both total and available N than epicormic leaves from monocalypts (total N: F2,87 = 2745.08, P < 0.001; available N: F2,87 = 1245.20, P < 0.001) (Fig. 2a and b).
Mean concentrations of total N (a), available N (b) and FPCs and UBFs (c) in epicormic leaves (this study) and mature foliage (from Au 2018) of symphoymyrtle and monocalypt species. Different lettering denotes significance between epicormic leaves of eucalypt species (P < 0.05).
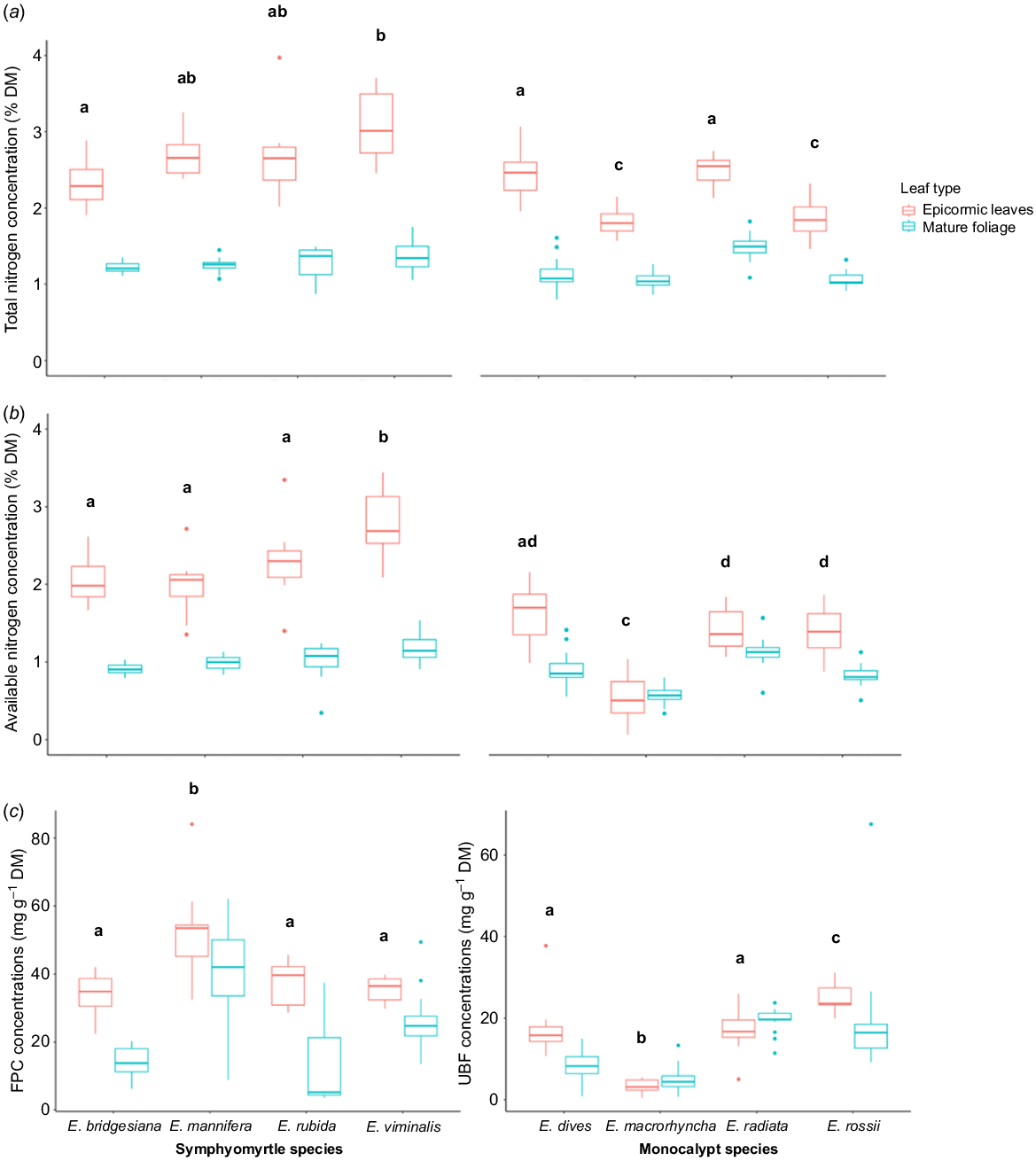
In the symphyomyrtle species, FPC concentrations ranged from 22 to 84 mg g−1 DM across all individuals of all species. In the monocalypt species, UBF concentrations ranged from 0 to 38 mg g−1 DM across all species (Fig. 2c; Table S1). Both FPC concentrations in symphyomyrtles and UBF concentrations in monocalypts differed significantly between tree species (F4,44 = 323.35, P < 0.001 and F4,43 = 155.84, P < 0.001 respectively) (Fig. 2c).
When comparing epicormic leaves to mature leaves of the same species from an earlier study, the general pattern suggests that epicormic leaves may contain more total and available N than mature leaves (Fig. 2a and b), but that the effect for available N may be stronger in symphyomyrtle species than in monocalypts. Most symphyomyrtle species appeared to have slightly higher FPC concentrations than mature leaves, but the pattern was less clear for UBF concentrations in monocalypts.
Discussion
This is the first study to measure both digestible N and PSM concentrations in epicormic eucalypt leaves and to investigate whether koalas will eat them. We have definitively shown that koalas will eat some epicormic leaves, although the amount depended on the species and the individual trees offered. Notably, koalas ate at least four times more epicormic leaves from species from the subgenus Symphyomyrtus than from the subgenus Eucalyptus. As discussed below, these findings substantially increase our understanding of the post-fire ecology of koalas and their habitats, which has been poorly studied to date.
Our findings demonstrate that captive koalas in the NSW Southern Tablelands can consume the epicormic growth of several eucalypt species, suggesting that they could potentially utilise these leaves in the wild as well. This supports previous observations of radio-tracked koalas in burnt eucalypts, with koalas documented using burnt trees for both food and shelter (Matthews et al. 2007). Our study also suggests that koalas may browse more readily on epicormic leaves from symphyomyrtle species than from monocalypts. It is likely that the chemistry of epicormic leaves played a strong role in driving differences in intake between groups of trees, eucalypt species, and subgenera.
Koala feeding decisions are influenced by variation in nutritional composition and subgenera-specific plant secondary metabolites within and between eucalypt species (Lawler et al. 1998, 2000; Marsh et al. 2003b, 2018). For example, koalas have previously been shown to have a relatively high threshold and tolerance for FPCs, and as a result tend to favour and consume more foliage from Symphyomyrtus species (Lawler et al. 1998; Moore and Foley 2005). In contrast, they seem to be deterred by much lower concentrations of UBFs compared to FPCs, which may contribute to their avoidance of some monocalypt trees and species (Marsh et al. 2021). As these subgenera have different PSM profiles, it is not possible to directly compare concentrations of FPCs in symphyomyrtle species to UBF concentrations in monocalypt species. Our findings support the above pattern, with captive koalas consuming more epicormic foliage from the symphyomyrtle species compared to the monocalypts.
Another difference that has previously been noted between the subgenera is that monocalypt species typically contain lower available N concentrations compared to symphyomyrtle species, although total N concentrations are generally similar (Wallis et al. 2010). We found that epicormic leaves also displayed this pattern. Animals require sufficient dietary protein to meet their daily maintenance requirements (283 mg kg−0.75 day−1 for total N and 271 mg kg−0.75 day−1 for digestible N, in the case of the koala: Cork 1986), and diets higher in digestible protein can improve health (French et al. 2017), reproductive capacity (DeGabriel et al. 2009), and the capacity to metabolise PSMs (Marsh et al. 2005; Au et al. 2013). Thus, the higher consumption of symphyomyrtle species could be partly due to selection for a diet higher in digestible protein. This could also mean that the epicormic leaves from symphyomyrtle species may be of greater nutritional value to koalas after fire than monocalypt species, due to the higher concentrations of available N from the trees within this subgenus. It is also worth noting that epicormic foliage of some monocalypt species was higher in available nitrogen than the mature foliage of some symphyomyrtle species. This might explain why koalas still choose to eat some epicormic foliage of monocalypt species when there is a choice.
While the epicormic regrowth from a few symphyomyrtle species had higher concentrations of a well-known herbivore deterrent PSM (FPCs) than mature leaves from the same species, the epicormic regrowth from all measured symphyomyrtles was substantially higher in both available N and total N. Similarly, limited studies on epicormic leaves from mature E. blakelyi trees, a symphyomyrtle species, found they contained higher concentrations of total N than mature foliage (Landsberg and Wylie 1983; Landsberg 1990). However, in the monocalypt species, concentrations of total N, available N and UBFs in the epicormic leaves were more similar to that of the mature foliage, with a few exceptions. These preliminary data suggest that koalas may face trade-offs between acquiring extra nutrients and avoiding higher intakes of potentially toxic PSMs when choosing whether to feed on mature or epicormic leaves, especially for symphyomyrtle species. It is important to note, however, that epicormic and mature leaves were collected from different trees and at different points in time, which can influence leaf chemistry (Moore et al. 2005; Wallis et al. 2011). Future research should prioritise comparing leaf chemistry of epicormic growth and mature foliage from the same trees and/or locations at consistent points in time and investigate how PSM and protein concentrations affect intake of epicormic growth. It would also be interesting to compare the nutritional composition of epicormic growth and young adult-phase leaves from the same species to better understand whether there are nutritional similarities between the effects of fire and flushes of new adult-phase growth on unburnt trees.
Although our results suggest that koalas can find some food in burnt areas, koalas in this study consumed epicormic leaves in conjunction with mature foliage, which raises questions about the extent to which koalas and other marsupial folivores can utilise epicormic leaves in their diet. Captive koalas readily eat 150–250 g DM on average per night (Marsh et al. 2014b, 2007, 2021). At most, the koalas in our study ate an average of 60 g DM epicormic leaves from the ‘best’ species and as little as 2 g DM from the ‘worst’ species. This fits with our observations that they always ate mature leaves in addition to epicormic leaves. It also raises questions about whether wild koalas could depend entirely on epicormic leaves in burnt habitat with no intact canopy, or whether epicormic leaves are only a supplementary food source. Ideally, we would have liked to measure absolute intake of epicormic leaves in the absence of mature foliage to clarify the extent to which koalas could be sustained with epicormic leaves. However, it was not feasible to restrict rescued koalas to diets that may potentially limit their intake. This should be a priority for investigation in future studies to better understand whether koalas can eat enough to meet their nutritional requirements in burnt areas that contain only epicormic leaves. It may also be useful to observe whether captive koalas choose to consume epicormic or mature foliage first and how often they switch between them, or to record how often wild koalas are feeding in stands dominated by epicormic growth during the night.
Interestingly, the amounts of epicormic leaves that koalas ate from each species did not necessarily align with perceptions of their ‘use’ by wild koalas. For example, E. rossii is considered ‘high use’ in the Southern Tablelands of NSW (State of NSW and Office of Environment and Heritage 2018) and the mature leaves are often highly preferred by captive koalas (K. Marsh and M. Lane, pers. obs.). However, koalas ate little epicormic regrowth of E. rossii. It would be interesting to determine whether the apparently higher UBF concentration in epicormic leaves in E. rossii contributed to this, given that koalas can be deterred from feeding as UBF concentrations increase (Marsh et al. 2019). Although PSM concentrations are known to affect how much koalas eat (Moore et al. 2004c, 2005; Moore and Foley 2005; Marsh et al. 2007), it was not possible to relate the chemistry of individual trees in this study to food intake because koalas had a choice between multiple options of epicormic and mature leaves each night. Future research should prioritise looking at the effects of leaf chemistry on intake.
Unlike E. rossii, we observed that captive koalas rarely ate either epicormic or mature leaf from E. macrorhyncha and E. dives, even though both of these species are also considered ‘high use’ in the study area (KMA5) based on sightings of koalas in trees and the presence of scats under trees (State of NSW and Office of Environment and Heritage 2018). Both E. macrorhyncha and E. dives are abundant in the Southern Tablelands region and may therefore be mostly used during the day rather than for feeding at night. It is well recognised that koalas do not necessarily feed from the trees in which they sit during the day (Phillips and Callaghan 2000; Moore and Foley 2005; Matthews et al. 2007; Marsh et al. 2014b). Thus, observations of tree use may be poorly correlated with diet (Marsh et al. 2014b). We suggest that E. macrorhyncha and E. dives are less important as browse species than their ‘use’ by koalas suggests, either before or after fire.
In the same vein, E. rubida and E. bridgesiana are considered to be used irregularly by wild koalas in KMA5 (State of NSW and Office of Environment and Heritage 2018), but captive koalas regularly ate substantial amounts of mature E. rubida and E. bridgesiana leaves in addition to epicormic leaves when they were offered. This suggests that wild koalas in the NSW Southern Tablelands may incorporate E. rubida and E. bridgesiana into their diet when they encounter them, even though they have not been commonly reported to sit in these species (State of NSW and Office of Environment and Heritage 2018).
Conclusion
Wildfires are predicted to increase in frequency and severity in the future due to climate change (Lucas et al. 2007). This study provides important baseline data on the chemistry of epicormic leaves 2–6 months after fire and describes some trends in the feeding behaviour of captive koalas offered a choice between mature and epicormic leaves after fire. This information is critical for understanding some of the indirect effects of fire on koalas and their habitat, and for informing post-fire conservation and management decisions. For example, bushfire events are often patchy, creating a mosaic of different burn severities in many areas (Burrows et al. 2021), which means that koalas may have choices between both mature foliage and epicormic growth. In this instance, the higher available N content of epicormic growth, particularly in symphyomyrtle species, may provide additional nutritional support to their diet during post-fire recovery, as was previously hypothesised by Moore et al. (2004a). It is also important to consider that the preferences for epicormic leaves might differ from preferences for mature leaves of the same species. This means that the species typically preferred by koalas when mature foliage is present may be different when trees are producing epicormic regrowth. These differences should be considered when looking at preferred trees from a management perspective (e.g. for tree retention during development or during revegetation activities) and by ensuring that preferred tree data are based on feeding observations and not just on sightings of koalas and the presence of scats under trees.
Although this example is for koalas in the NSW Southern Tablelands, there may be similar patterns in other areas. It is important to learn more about the nutritional quality of epicormic leaves in other areas known to be used by koalas, as the quality of burnt habitat is likely to depend on which species of eucalypts are present and the proportions of those species. Our results suggest that after fire, epicormic regrowth from symphyomyrtle species may be more readily browsed than regrowth from monocalypts by koalas in the NSW Southern Tablelands. This matches the known preferences of koalas for symphyomyrtle species more broadly (Moore et al. 2004a), but monocalypt species can form an important component of the diet of koalas in some locations (Brice et al. 2019; Marsh et al. 2021), including the Southern Tablelands (K. Marsh and M. Lane, pers. obs.). Therefore, it is particularly important to understand nutritional preferences following fire. Taking this into consideration, some burnt areas are likely to be better quality habitat than others, revealing additional complexities in the spatial and temporal distribution of browse quality, which may influence koala feeding, movement behaviour and habitat requirements after fire.
Ethics
This research was approved by the NSW Government Department of Planning, Industry and Environment Animal Ethics Committee (permit number 200421/04) and was conducted in accordance with the Australian Code for the care and use of animals for scientific purposes.
Data availability
The data will be available online at the Australian National University Data Commons Library.
Declaration of funding
The project was started with a $100 000 donation from Two Thumbs Wildlife Trust in memory of Captain Ian McBeth, First Officer Paul Hudson and Flight Engineer Rick DeMorgan Jr, who lost their lives during firebombing operations on Thursday 23 January 2020, when their C-130 Large Air Tanker crashed after dropping fire retardant on Two Thumbs Wildlife Trust’s koala sanctuary at Peak View, NSW, Australia. Additional funding was provided by the Minderoo Foundation (TI_ANU001) and the NSW Government under the NSW Koala Strategy and the Australian Government Wildlife and Habitat Bushfire Recovery Program (DOC20/500625).
Acknowledgements
We thank Kimberley Schiphof and James Skewes for their assistance in leaf chemistry analysis. We also thank Rod Pietsch for his help in enabling this project.
References
Andrew RL, Peakall R, Wallis IR, Foley WJ (2007) Spatial distribution of defense chemicals and markers and the maintenance of chemical variation. Ecology 88(3), 716-728.
| Crossref | Google Scholar | PubMed |
Attiwill PM, Adams MA (2013) Mega-fires, inquiries and politics in the eucalypt forests of Victoria, south-eastern Australia. Forest Ecology and Management 294, 45-53.
| Crossref | Google Scholar |
Au J, Marsh KJ, Wallis IR, Foley WJ (2013) Whole-body protein turnover reveals the cost of detoxification of secondary metabolites in a vertebrate browser. Journal of Comparative Physiology B 183(7), 993-1003.
| Crossref | Google Scholar |
Au J, Clark RG, Allen C, Marsh KJ, Foley WJ, Youngentob KN (2019) A nutritional mechanism underpinning folivore occurrence in disturbed forests. Forest Ecology and Management 453(8), 117585.
| Crossref | Google Scholar |
Bates D, Mächler M, Bolker B, Walker S (2015) Fitting linear mixed-effects models using lme4. Journal of Statistical Software 67(1), 1-48.
| Crossref | Google Scholar |
Beale PK, Foley WJ, Saraf I, Singh IP, Marsh KJ (2022) Common ringtail possums (Pseudocheirus peregrinus) tolerate high concentrations of unsubstituted B-ring flavanones in their diet. Australian Mammalogy 44(3), 347-351.
| Crossref | Google Scholar |
Brice KL, Trivedi P, Jeffries TC, Blyton MDJ, Mitchell C, Singh BK, Moore BD (2019) The koala (Phascolarctos cinereus) faecal microbiome differs with diet in a wild population. PeerJ 7, e6534.
| Crossref | Google Scholar | PubMed |
Burrows G (2002) Epicormic strand structure in Angophora, Eucalyptus and Lophostemon (Myrtaceae): implications for fire resistance and recovery. New Phytologist 153(1), 111-131.
| Crossref | Google Scholar |
Burrows GE (2008a) Syncarpia and Tristaniopsis (Myrtaceae) possess specialised fire-resistant epicormic structures. Australian Journal of Botany 56(3), 254-264.
| Crossref | Google Scholar |
Burrows N (2008b) Linking fire ecology and fire management in south-west Australian forest landscapes. Forest Ecology and Management 255(7), 2394-2406.
| Crossref | Google Scholar |
Burrows G (2013) Buds, bushfires and resprouting in the eucalypts. Australian Journal of Botany 61(5), 331-349.
| Crossref | Google Scholar |
Burrows N, Stephens C, Wills A, Densmore V (2021) Fire mosaics in south-west Australian forest landscapes. International Journal of Wildland Fire 30(12), 933-945.
| Crossref | Google Scholar |
Clarke PJ, Lawes MJ, Midgley JJ, Lamont BB, Ojeda F, Burrows GE, Enright NJ, Knox KJE (2013) Resprouting as a key functional trait: how buds, protection and resources drive persistence after fire. New Phytologist 197(1), 19-35.
| Crossref | Google Scholar | PubMed |
Cork SJ (1986) Foliage of eucalyptus-punctata and the maintenance nitrogen requirements of koalas, Phascolarctos cinereus. Australian Journal of Zoology 34(1), 17-23.
| Crossref | Google Scholar |
DeGabriel JL, Wallis IR, Moore BD, Foley WJ (2008) A simple, integrative assay to quantify nutritional quality of browses for herbivores. Oecologia 156(1), 107-116.
| Crossref | Google Scholar | PubMed |
DeGabriel JL, Moore BD, Foley WJ, Johnson CN (2009) The effects of plant defensive chemistry on nutrient availability predict reproductive success in a mammal. Ecology 90(3), 711-719.
| Crossref | Google Scholar | PubMed |
French WW, Dridi S, Shouse SA, Wu H, Hawley A, Lee S-O, Gu X, Baum JI (2017) A high-protein diet reduces weight gain, decreases food intake, decreases liver fat deposition, and improves markers of muscle metabolism in obese Zucker rats. Nutrients 9(6), 587.
| Crossref | Google Scholar | PubMed |
He T (2014) Ecological divergence and evolutionary transition of resprouting types in Banksia attenuata. Ecology and Evolution 4(16), 3162-3174.
| Crossref | Google Scholar | PubMed |
Jensen LM, Wallis IR, Marsh KJ, Moore BD, Wiggins NL, Foley WJ (2014) Four species of arboreal folivore show differential tolerance to a secondary metabolite. Oecologia 176(1), 251-258.
| Crossref | Google Scholar | PubMed |
Kuznetsova A, Brockhoff PB, Christensen RH (2017) lmerTest package: tests in linear mixed effects models. Journal of Statistical Software 82(13), 1-26.
| Crossref | Google Scholar |
Landsberg J (1990) Dieback of rural eucalypts: does insect herbivory relate to dietary quality of tree foliage? Australian Journal of Ecology 15(1), 73-87.
| Crossref | Google Scholar |
Landsberg J, Wylie FR (1983) Water stress, leaf nutrients and defoliation: a model of dieback of rural eucalypts. Australian Journal of Ecology 8(1), 27-41.
| Crossref | Google Scholar |
Lawler IR, Foley WJ, Eschler BM, Pass DM, Handasyde K (1998) Intraspecific variation in Eucalyptus secondary metabolites determines food intake by folivorous marsupials. Oecologia 116(1–2), 160-169.
| Crossref | Google Scholar | PubMed |
Lawler IR, Foley WJ, Eschler BM (2000) Foliar concentration of a single toxin creates habitat patchiness for a marsupial folivore. Ecology 81(5), 1327-1338.
| Crossref | Google Scholar |
Lenth R (2023) emmeans: Estimated Marginal Means, aka Least-Square Means (Version 1.8.5). Available at https://CRAN.R-project.org/package=emmeans [accessed 10 March 2023]
Lunney D, Gresser SM, Mahon PS, Matthews A (2004) Post-fire survival and reproduction of rehabilitated and unburnt koalas. Biological Conservation 120(4), 567-575.
| Crossref | Google Scholar |
Marsh KJ, Foley WJ, Cowling A, Wallis IR (2003a) Differential susceptibility to Eucalyptus secondary compounds explains feeding by the common ringtail (Pseudocheirus peregrinus) and common brushtail possum (Trichosurus vulpecula). Journal of Comparative Physiology B 173(1), 69-78.
| Crossref | Google Scholar | PubMed |
Marsh KJ, Wallis IR, Foley WJ (2003b) The effect of inactivating tannins on the intake of Eucalyptus foliage by a specialist Eucalyptus folivore (Pseudocheirus peregrinus) and a generalist herbivore (Trichosurus vulpecula). Australian Journal of Zoology 51(1), 31-42.
| Crossref | Google Scholar |
Marsh KJ, Wallis IR, Foley WJ (2005) Detoxification rates constrain feeding in common brushtail possums (Trichosurus vulpecula). Ecology 86(11), 2946-2954.
| Crossref | Google Scholar |
Marsh KJ, Wallis IR, Foley WJ (2007) Behavioural contributions to the regulated intake of plant secondary metabolites in koalas. Oecologia 154(2), 283-290.
| Crossref | Google Scholar | PubMed |
Marsh KJ, Moore BD, Wallis IR, Foley WJ (2014a) Feeding rates of a mammalian browser confirm the predictions of a ‘foodscape’ model of its habitat. Oecologia 174(3), 873-882.
| Crossref | Google Scholar | PubMed |
Marsh KJ, Moore BD, Wallis IR, Foley WJ (2014b) Continuous monitoring of feeding by koalas highlights diurnal differences in tree preferences. Wildlife Research 40(8), 639-646.
| Crossref | Google Scholar |
Marsh KJ, Ward J, Wallis IR, Foley WJ (2018) Intraspecific variation in nutritional composition affects the leaf age preferences of a mammalian herbivore. Journal of Chemical Ecology 44(1), 62-71.
| Crossref | Google Scholar | PubMed |
Marsh KJ, Saraf I, Hocart CH, Youngentob K, Singh I-P, Foley WJ (2019) Occurrence and distribution of unsubstituted B-ring flavanones in Eucalyptus foliage. Phytochemistry 160, 31-39.
| Crossref | Google Scholar | PubMed |
Marsh KJ, Blyton MDJ, Foley WJ, Moore BD (2021) Fundamental dietary specialisation explains differential use of resources within a koala population. Oecologia 196(3), 795-803.
| Crossref | Google Scholar | PubMed |
Martin RW (1981) Age-specific fertility in three populations of the koala, Phascolarctos cinereus Goldfuss, in Victoria. Wildlife Research 8(2), 275-283.
| Crossref | Google Scholar |
Matthews A, Lunney D, Gresser S, Maitz W (2007) Tree use by koalas (Phascolarctos cinereus) after fire in remnant coastal forest. Wildlife Research 34(2), 84-93.
| Crossref | Google Scholar |
Melzer A, Carrick F, Menkhorst P, Lunney D, John BS (2000) Overview, critical assessment, and conservation implications of koala distribution and abundance. Conservation Biology 14(3), 619-628.
| Crossref | Google Scholar |
Moore BD, Foley WJ (2005) Tree use by koalas in a chemically complex landscape. Nature 435(7041), 488-490.
| Crossref | Google Scholar | PubMed |
Moore BD, Wallis IR, Wood JT, Foley WJ (2004b) Foliar nutrition, site quality, and temperature influence foliar chemistry of tallowwood (Eucalyptus microcorys). Ecological Monographs 74(4), 553-568.
| Crossref | Google Scholar |
Moore BD, Wallis IR, Palá-Paúl J, Brophy JJ, Willis RH, Foley WJ (2004c) Antiherbivore chemistry of Eucalyptus-cues and deterrents for marsupial folivores. Journal of Chemical Ecology 30(9), 1743-1769.
| Crossref | Google Scholar | PubMed |
Moore BD, Foley WJ, Wallis IR, Cowling A, Handasyde KA (2005) Eucalyptus foliar chemistry explains selective feeding by koalas. Biology Letters 1(1), 64-67.
| Crossref | Google Scholar | PubMed |
Pausas JG, Keeley JE (2017) Epicormic resprouting in fire-prone ecosystems. Trends in Plant Science 22(12), 1008-1015.
| Crossref | Google Scholar | PubMed |
Pausas JG, Pratt RB, Keeley JE, Jacobsen AL, Ramirez AR, Vilagrosa A, Paula S, Kaneakua-Pia IN, Davis SD (2016) Towards understanding resprouting at the global scale. New Phytologist 209(3), 945-954.
| Crossref | Google Scholar | PubMed |
Penman TD, Kavanagh RP, Binns DL, Melick DR (2007) Patchiness of prescribed burns in dry sclerophyll eucalypt forests in south-eastern Australia. Forest Ecology and Management 252(1–3), 24-32.
| Crossref | Google Scholar |
Phillips S, Callaghan J (2000) Tree species preferences of koalas (Phascolarctos cinereus) in the Campbelltown area south-west of Sydney, New South Wales. Wildlife Research 27(5), 509-516.
| Crossref | Google Scholar |
R Core Team (2022) R: A language and environment for statistical computing. R Foundation for Statistical Computing, Vienna, Austria. Available at https://www.R-project.org/
Russell-Smith J, Yates CP, Whitehead PJ, Smith R, Craig R, Allan GE, Thackway R, Frakes I, Cridland S, Meyer MCP, Malcolm Gill A (2007) Bushfires ‘down under’: patterns and implications of contemporary Australian landscape burning. International Journal of Wildland Fire 16(4), 361-377.
| Crossref | Google Scholar |
Stalenberg E, Wallis IR, Cunningham RB, Allen C, Foley WJ (2014) Nutritional correlates of koala persistence in a low-density population. PLoS ONE 9(12), e113930.
| Crossref | Google Scholar | PubMed |
Vernes K, Castellano M, Johnson CN (2001) Effects of season and fire on the diversity of hypogeous fungi consumed by a tropical mycophagous marsupial. Journal of Animal Ecology 70(6), 945-954.
| Crossref | Google Scholar |
Wallis IR, Nicolle D, Foley WJ (2010) Available and not total nitrogen in leaves explains key chemical differences between the eucalypt subgenera. Forest Ecology and Management 260(5), 814-821.
| Crossref | Google Scholar |
Wallis IR, Keszei A, Henery ML, Moran GF, Forrester R, Maintz J, Marsh KJ, Andrew RL, Foley WJ (2011) A chemical perspective on the evolution of variation in Eucalyptus globulus. Perspectives in Plant Ecology, Evolution and Systematics 13(4), 305-318.
| Crossref | Google Scholar |
Waters DA, Burrows GE, Harper JDI (2010) Eucalyptus regnans (Myrtaceae): a fire-sensitive eucalypt with a resprouter epicormic structure. American Journal of Botany 97(4), 545-556.
| Crossref | Google Scholar | PubMed |
Wintle BA, Legge S, Woinarski JC (2020) After the megafires: What next for Australian wildlife? Trends in Ecology & Evolution 35(9), 753-757.
| Crossref | Google Scholar | PubMed |