Effect of soil treatment on the growth and foliage chemistry of three Eucalyptus species grown in a plantation as a food source for koalas
Teresa Cochrane



A School of Agricultural, Environmental and Veterinary Sciences, Charles Sturt University, Wagga Wagga, NSW 2678, Australia.
B Koala Conservation Australia, Koala Hospital, 154 Lord Street, Port Macquarie, NSW 2444, Australia.
Abstract
Koalas in captivity are often provided with food from purpose-grown eucalypt plantations. In this study the growth/establishment of a long-term koala food plantation and the foliage chemistry of three Eucalyptus species (Eucalyptus microcorys, E. propinqua and E. tereticornis) that had applications of soil treatments (Terracottem™, mycorrhiza or a combination of both, and a control) at the time of planting were investigated. As well as tree growth, the foliage chemistry measured included total nitrogen (N), available N, ash, and moisture contents, and dry matter digestibility. Shortly after planting, the soil treatments had significant effects on tree growth but after approximately 2.5 years, they did not impact either tree growth or foliage chemistry. However, foliage age affected foliage chemistry, with new foliage having higher moisture and total N concentrations and lower ash contents than mature foliage. There were significant differences in tree growth and foliage chemistry between replicate blocks, which may have been related to environmental or geographical variables. This research provided critical information concerning the establishment of a suitable feed source for captive koalas and, more generally, furthered knowledge of Eucalyptus foliage chemistry. Future studies should investigate impacts of soil treatments on plant secondary metabolites including formylated phloroglucinol compounds. Understanding dietary selection is an important consideration when selecting species and managing food plantations for koalas. Providing captive koalas with a variety of different Eucalyptus species and foliage maturity ensures that they can select species and nutrients that they require.
Keywords: diet, Eucalyptus chemistry, foliage age, koalas, nutrients, soil treatment.
Introduction
Australia has over 800 named species of Eucalyptus across a variety of ecosystems (Thornhill et al. 2019). The koala population consumes only 5–10% or ~25–70 different Eucalyptus species across their distribution range (Bercovitch 2007; Marsh et al. 2021) and, recently, OEH (2018) found evidence for 137 species, with State of New South Wales and Department of Planning, Industry, and Environment (DPIE), (2019) refining this to 123 species. Eucalypt species from the Symphyomyrtus subgenus are the primary dietary choice for koalas (DeGabriel et al. 2010) with the other subgenera within Eucalyptus being secondary diet selection species for koalas (Noble 1989; Melzer et al. 2014; Marsh et al. 2019, 2021).
Koalas are regarded as ‘picky’ or ‘fussy’ eaters and are commonly observed to either sniff or chew Eucalyptus foliage before either consuming it or moving on to another branch or tree (Marsh et al. 2013; Pennisi 2018). Koalas have developed the ability to sense the foliage chemistry, which consequently influences their diet selection (Moore and Foley 2000; Johnson et al. 2018). Eucalyptus foliage generally contains adequate concentrations of all nutrients (Moore et al. 2005) except N (Wu et al. 2012) to meet the koala’s requirements. Moore and Foley (2005) and Stalenberg et al. (2014) found that koalas were less likely to visit trees/foliage that had low N (or available N [AvailN] concentrations, and in turn would visit and consume foliage with high N concentrations. Selecting foliage with high N concentrations also allows herbivores to deal with formylated phloroglucinol compounds (FPC) (Au et al. 2013), which are feeding deterrent and influence diet selection, with koalas selecting against high FPC-containing foliage (Moore and Foley 2005).
In discussing the impact of foliage N concentration on koala diet selection it is important to differentiate between total N and AvailN. Total N is the total N concentration of the foliage and generally Eucalyptus foliage has a total N concentration of 0.8–2.0% on a dry matter (DM) basis (DeGabriel et al. 2010; Stalenberg et al. 2014). There are a number of factors that influence the total N content of Eucalyptus foliage including species, tree genetics, soil N status, and the presence or absence of mycorrhizal symbionts (Moore et al. 2004; Andrew et al. 2005). Some of this N (in the form of protein) will be bound to plant secondary metabolites (PSM), particularly tannins, rendering it unavailable for digestion by the animal (Asquith and Butler 1986). Cork et al. (1983) determined that for koalas, the average apparent digestibility of total N (i.e. AvailN) in Eucalyptus foliage was only 45%, although this varied depending on species (Cork 1986). The AvailN content influences both the selection of trees and foliage from these trees by koalas (Marsh et al. 2014; Stalenberg et al. 2014).
Available N concentrations are often lower in many species from subgenus Eucalyptus than in species favoured by koalas from subgenus Symphyomyrtus (Stalenberg et al. 2014; Marsh et al. 2021). Leaf maturity also affects total N concentration, which likely affects the nutritional value for koalas (Hume 1999; Marsh et al. 2018).
The moisture content of Eucalyptus foliage has an influence on koala diet selection and in certain conditions koalas will choose water availability (Wu et al. 2012) over foliage nutrients. Koalas predominantly select foliage containing more than 55% moisture (Hume and Esson 1993). Young foliage has a higher moisture content and higher N concentration than mature foliage (Martin 1985; Krockenberger et al. 1998).
Many studies have investigated the impacts of foliage age on selection by koalas and the findings have been variable. For example, Martin (1985), Eberhard et al. (1975), and Krockenberger et al. (1998) found that when koalas were offered young and mature foliage, they would select the younger leaves, potentially due to its higher moisture and N contents.
Other studies have found koalas may prefer either young or mature leaves when offered a choice, depending on the eucalypt species and interactions between the concentrations of nutrients, PSM and moisture in foliage (Wu et al. 2012). Understanding dietary selection is an important consideration when selecting species and managing food plantations for koalas.
The 2019–20 megafires had a major impact on native Eucalyptus forest vegetation in eastern Australia, affecting 25.9% of all moderate to very high suitability koala habitat. As a result, the future viability of koala populations has become the focus of extensive conservation efforts. The Port Macquarie Koala Hospital (PMKH) is a not-for-profit organisation involved in the care and conservation of wild koalas who have suffered injuries from fire or other causes. In 2019, the PMKH established a second Eucalyptus tree plantation to provide a readily accessible food source for the large number of koalas in their care.
Mycorrhizal treatments provide nutrients to plants, especially ones with low mobility in soil and assists with biological N fixation and regulation of phosphorus toxicity, which can reduce growth (Santana et al. 2020; Tibbett et al. 2022). Adams et al. (2006) showed that two types of mycorrhiza naturally occur with Eucalyptus and can promote seedling growth.
TerraCottem has been shown to promote seedling establishment but also to enhance the tree coverage and growth (Bulíř 2006; Navroski et al. 2016; Crous 2017).
PMKH selected the soil treatments used at the time the plantation was established and the study design. The aim of this research was to investigate if one soil treatment was more effective, to enable the most cost-effective plantation establishment at future locations that also provided nutrients favoured by koalas. Due to the time between planting and this research starting seedling survival was not addressed and the study focused on the generation of most nutritious leaves as koala feed.
This research investigated (1) the relative success of the different planting techniques used at the plantation, and (2) the nutritional composition of new and mature leaves from the plantation seedlings. This will provide critical information in relation to the establishment of a suitable feed source for captive koalas, primarily through selection of effective planting treatments and techniques and secondly through selection of preferred species in establishing future feed plantations. The outcomes of this research will also further our knowledge of Eucalyptus leaf chemistry and its role in koala diet selection.
Materials and methods
Study site
The study site was located within Koala Conservation Australia’s Moripo Park Research Plantation (−31.456408, 152.578100), a long-term koala food tree and research plantation managed by PMKH. The plantation was located within the Port Macquarie–Hasting local government area on Birpai/Biripi Country, ~18 km west of Wauchope, NSW. The study site was located on a hillslope with 6.50° elevation and this hillslope structure was a crest at the top followed by a simple slope and a flat at the bottom (Fig. 1). The soil was predominantly silt and sandstone on top of a rock bed made of sedimentary and conglomerate rocks (Australian Government 2022).
The study site has a temperate climate, with high rainfall in summer with a mean maximum temperature of 32°C, and during winter has low rainfall with mean minimum temperature of 7°C (Bureau of Meteorology 2022a, 2022b).
Experimental design
A factorial block design was used. It consisted of four soil treatments, and three tree species replicated three times (i.e. three blocks). The replicate blocks were located down a hill slope (Fig. 1).
The four soil treatments were: (1) TerraCottem™; (2) mycorrhiza; (3) TerraCottem™ and mycorrhiza; and (4) a control with nil treatment. Application rates at the time of planting for each of the soil treatments was 8 g per treatment per seedling. TerraCottem is a hydrogel polymer compound developed for agricultural applications in arid conditions and poor soil formations. Its application replenishes soil components consumed during the growth season by plants. It includes growth precursors to promote root strength and growth, acrylamide to store water normally lost due to evaporation, and fertiliser to provide balanced plant nutrition (Chiorescu 2019). Within each soil treatment within each block, four seedlings of tallowwood (E. microcorys), four seedlings of grey gum (E. propinqua), and four seedlings of forest red gum (E. tereticornis) were planted, which resulted in a total of 144 seedlings [(three replicated blocks) × (four soil treatments) × (12 trees per soil treatment per block)] (Fig. 2). The seedlings were sourced as tube stock from the Forestry Corporation of NSW nursery at Grafton, and were selected because they are known food trees for koalas in the region.
Prior to planting (May 2019), the soil was keyline ploughed, a technique used to help with water irrigation by increasing water retention and collection (Savory and Duncan 2016). A coir fibre weed mat was then placed around each planted seedling to supress weed development. A black shallow cone was placed over the mat to help with capturing water and delivering it to the root system, and a tree guard was added to prevent native wildlife from interfering with plant establishment. The site was continually mown between the seedlings to minimise grass and weed competition.
Tree measurements
On 27 May 2019, an initial pilot survey was carried out to record the heights of the tallowwood, grey gum and forest red gum seedlings. This survey was carried out to assess the effectiveness of the soil treatments in the short term. For our main survey, measurements of seedling height, stem diameter and seedling canopy coverage were recorded over the period 8–12 November 2021.
Seedling heights were measured using either a 1 m or 5 m ruler. Seedling stem diameter was measured using Vernier callipers. Measurements of stem diameter were recorded at a height 300 mm from the ground. For seedlings that had multiple stems, the diameter of the largest stem was recorded. Seedling canopy cover was recorded using the line intercept method, where a tape measure was placed on the ground under the seedling canopy, and the longest diameter recorded (Fiala et al. 2006) using a metal tape measure.
Foliage sampling
Leaves from the planted seedlings were collected over the period 3–8 January 2022 between 0700 and 0800 hours. Sunrise during the sampling period was approximately 0550 hours. Within each soil treatment area within each block, species were combined. Composite samples representing either young and mature leaves were collected separately for each tree species for leaf chemistry analysis (Wu et al. 2012). To create a representative sample, both young and mature leaves were randomly collected from the north, south, east, and west sections of the seedling canopy of each tree using a random number generator. Young and mature foliage were determined using the methodology described by Johnson (1926).
In the field, approximately 50 g (known weight, to nearest 0.01 g) of both mature and young leaves were weighed in a prelabelled, sealable plastic bag using an electronic scale (AMIR 1-200 Superior Mini Digital Platform Scale). The samples were then transferred into prelabelled paper bags, transported to the laboratory, and dried at 60°C in a forced-air oven (Thermo Scientific Heratherm OGS400) to a constant weight.
Foliage chemical analysis
Dried leaves were ground to first pass through a 5 mm coarse sieve, and then a 1 mm fine sieve (FOSS Cyclotec 1093 Plant Sample mill grinder). The samples were stored in plastic 70 mL screw-top specimen containers at room temperature until subsequent leaf chemistry analyses were performed.
The analytical DM content of the ground leaf samples was determined by drying a known weight (~1 g) of sample into a (dried) porcelain crucible before being dried in an air-forced oven at 80°C for 24 h. After cooling in a desiccator and reweighing, the dried samples were then ignited in the muffle furnace at 200°C for 2 h, then 550°C for 6 h until all carbon was removed. After cooling in a desiccator, the weight of ash was measured, and recorded.
The total N content of the leaf samples was determined on duplicate samples (250 ± 10 mg) using the Dumas combustion method with a Leco CNS 2000® analyser (Leco, St Joseph, MI, USA).
Modification of the methods of DeGabriel et al. (2008) and Wallis et al. (2010) were used to determine in vitro AvailN. This method was chosen as it mimics digestion in hindgut-fermenter mammals such as koalas. The assay involved digesting duplicate samples (500 ± 20 mg) in porous bags (Ankom F57, Ankom Technology, Macedon, NY), first with pepsin (24 h) and then with cellulase (48 h). The N content of the residual material was determined as described above. The AvailN was calculated as follows:
where
Knowing the mass of DM in the bag at the end of the assay also enabled calculation of DM digestibility (DMD), using the following equation:
Statistical analysis
Statistical analyses were carried out on mean seedling height, stem diameter and canopy cover of the three Eucalyptus species, and for foliage chemical analysis results, using ANOVA and Kruskal–Wallis ANOVA tests, and Tukey’s Post hoc analysis to determine the effects of soil treatment and blocks on seedling parameters and leaf chemistry and the effects of foliage age on leaf chemistry. Statistical analyses were completed using Microsoft Excel 2019 (Excel), Statistical Kingdom (Statistics Kingdom, 2021), and R statistical software (Rstudio) (R Core Team 2022).
Results
Seedling height
The 2019 pilot survey of seedling growth showed a difference (one-way ANOVA, F(2,42) = 19.93, P < 0.001) in the mean height (mm) of tallowwood (485 ± 76.3), grey gum (319 ± 100.7) and forest red gum (255 ± 126.7) seedlings. However, when seedlings were surveyed again in 2021, there were no species differences (one-way ANOVA, F(2,138) = 0.60, P > 0.05) in the average height of the seedlings for all three species (Table 1, Fig. 3).
Parameter | Forest red gum | Grey gum | Tallowwood | P value | ||||
---|---|---|---|---|---|---|---|---|
S | T | B | S × B | |||||
Height (mm) | 2781 ± 779.0 | 2703 ± 901.4 | 2921 ± 1183.5 | 0.548 | 0.17 | <0.0001 | <0.0001 | |
Stem diameter (mm) | 45 ± 19.4 | 47 ± 21.8 | 44 ± 20.0 | 0.685 | 0.334 | <0.0001 | <0.0001 | |
Canopy cover (mm) | 1970 ± 1049.3 | 2003 ± 778.1 | 2001 ± 765.2 | 0.563 | 0.88 | <0.0001 | <0.0001 |
s.d., standard deviation; S, species; T, soil treatment; B, block (elevation).
The average height of tallowwood (T), grey gum (G) and forest red gum measured in 2019 and 2021.
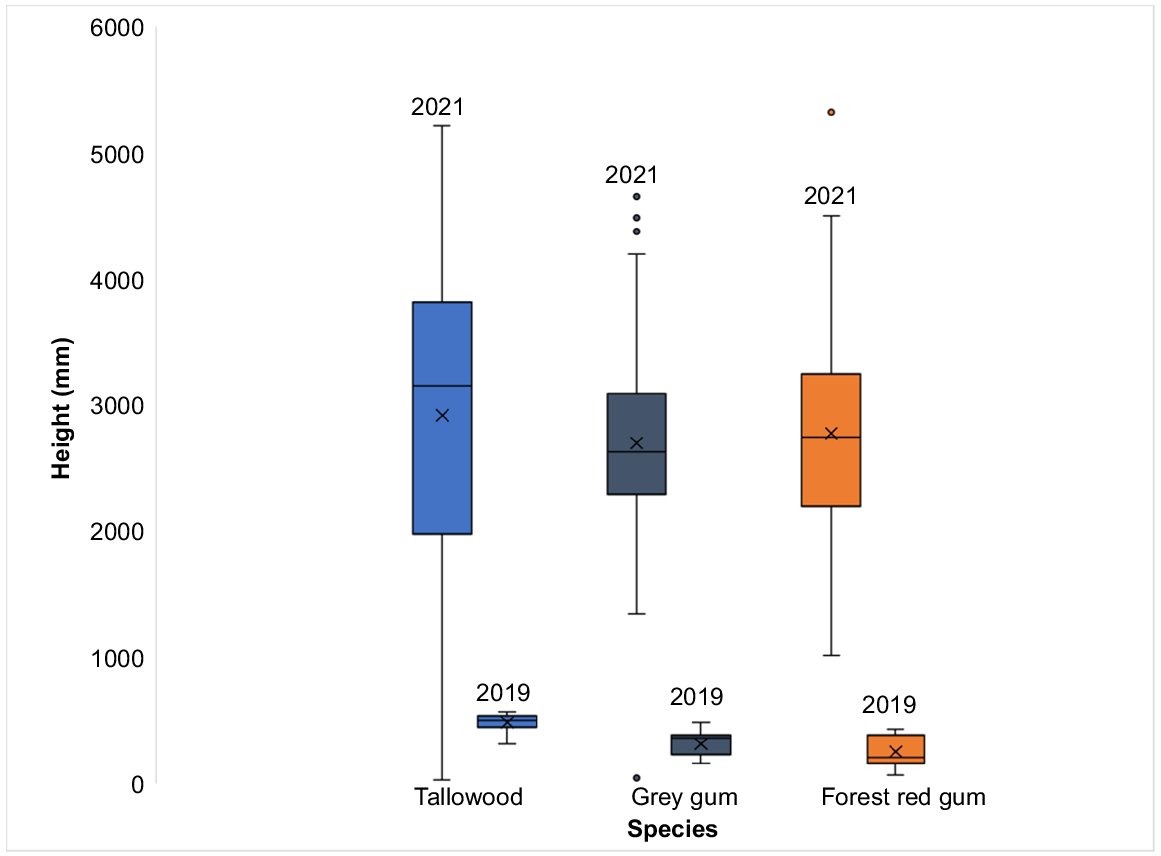
There was a difference (one-way ANOVA, F(2,138) = 20.14, P < 0.001) in the average height of the trees between Blocks 2 and 3 (Tukey’s HSD Test, P < 0.001, 95% C.I. = [693.4, 1519.6]), but not between Blocks 1 and 2 and Blocks 1 and 3 (Tukey’s HSD Test, P > 0.05). There was a significant interaction (one-way ANOVA, F(3,137) = 8.78, P < 0.001) between species and blocks. Soil treatment had no effect (one-way ANOVA, F(3,137) = 1.69, P > 0.05) on the height of the trees (Table 1).
Seedling stem diameter
There was no difference in stem diameter (one-way ANOVA, F(2,138) = 0.38, P > 0.05) between the three Eucalyptus species (Table 1). However, there was a difference in the average stem diameter of the trees between all blocks (one-way ANOVA, F(2,138) = 33.04, P < 0.001) (Fig. 4). There was a significant interaction (one-way ANOVA, F(3,137) = 18.72, P < 0.001) between species and blocks. Soil treatment had no effect (one-way ANOVA, F(3,137) = 1.14, P > 0.05) on the stem diameter.
Canopy coverage
There was no difference in canopy coverage (one-way ANOVA, F(2,138) = 0.58, P > 0.05) between the three Eucalyptus species (Table 1) However, there were differences (one-way ANOVA, F(2,138) = 23.13, P < 0.0001) in average canopy cover of the trees between Blocks 1 and 3 and Blocks 2 and 3 but not between Blocks 1 and 2 (Fig. 4). There was a significant interaction (one-way ANOVA, F(3,137) = 8.73, P < 0.001) between species and blocks but no interactions between species and soil treatments or soil treatments and blocks. Soil treatment had no effect (one-way ANOVA, F(3,137) = 0.22, P > 0.05) on canopy cover.
Foliage moisture content
There were no differences (one-way ANOVA, (F(2,69) = 1.07, P > 0.05) in the average foliage moisture content (g/100 g fresh weight) between tallowwood (63.19 ± 7.604), grey gum (66.29 ± 7.730) and forest red gum (65.86 ± 8.507) for mature and young leaves combined. However, the average moisture content of new foliage (72.24 ± 3.294 g/100 g fresh weight) was higher (one-way ANOVA, F(1,70) = 303.2, P < 0.0001) than that of mature foliage (57.98 ± 3.644 g/100 g fresh weight) and this was consistent across all species, soil treatments and blocks. For average foliage moisture content, there were no interactions between species and soil treatment (ANOVA, F(1,70) = 0.05, P > 0.05) or between species and block (ANOVA, F(1,70) = 3.87, P > 0.05).
As shown in Table 2, the moisture contents of both the new and mature foliage of tallowwood were different (new leaves (ANOVA F(2,33) = 4.9, P < 0.015; Tukey’s HSD Test, P < 0.01, 95% C.I. = [0.64, 6.60]) and mature leaves (ANOVA, F(2,33) = 4.96, P < 0.015; Tukey’s HSD Test, P < 0.01, 95% C.I. = [0.17, 6.79])) from those of grey gum foliage, but there were no other species differences (Tukey’s HSD Test, P > 0.05) in the moisture contents of the new and mature foliage. There were differences (one-way ANOVA, F(2,33) = 20.68, P < 0.0001) in the average new foliage moisture content (g/100 g fresh weight) between Blocks 1 (68.8 ± 2.90) and 3 (73.6 ± 1.57) (Tukey’s HSD Test, P < 0.0001, 95% C.I. = [2.48, 7.01]), and Blocks 1 and 2 (74.3 ± 2.11) (Tukey’s HSD Test, P < 0.0001, 95% C.I. = [3.19, 7.72]), but not between Blocks 2 and 3 (Tukey’s HSD Test, P > 0.05). There were significant differences (one-way ANOVA, F(2,33) = 7.47, P < 0.0001) in the average mature foliage moisture contents between Blocks 1 (55.21 ± 3.82) and 3 (58.9 ± 2.01) (Tukey’s HSD Test, P < 0.0001, 95% C.I. = [0.55, 6.79]), and Blocks 1 and 2 (59.9 ± 2.01) (Tukey’s HSD Test, P < 0.0001, 95% C.I. = [1.55, 7.78]), but not between Blocks 2 and 3 (Tukey’s HSD Test, P > 0.05).
Parameter | Forest red gum | Grey gum | Tallowwood | P value | |||||||
---|---|---|---|---|---|---|---|---|---|---|---|
New leaf | Mature leaf | New leaf | Mature leaf | Young leaf | Mature leaf | Species | Leaf maturity | Soil treatment | Block | ||
Moisture (g/100 g fresh) | 73.69 ± 2.85 | 58.02 ± 3.05 | 72.96 ± 2.75 | 59.62 ± 4.50 | 70.07 ± 3.30 | 56.31 ± 2.59 | 0.35 | <0.0001 | 0.876 | 0.060 | |
Total N (g/100 g DM | 1.73 ± 0.293 | 1.17 ± 0.288 | 1.62 ± 0.278 | 1.20 ± 0.278 | 1.41 ± 0.408 | 1.08 ± 0.276 | 0.0291 | <0.0001 | 0.991 | 0.022 | |
Available N (g/100 g DM) | 0.83 ± 0.200 | 0.80 ± 0.203 | 0.65 ± 0.169 | 0.67 ± 0.172 | 0.75 ± 0.168 | 0.70 ± 0.168 | 0.0248 | 0.71 | 0.184 | 0.005 | |
Ash (g/100 g DM) | 4.83 ± 0.340 | 6.91 ± 1.211 | 4.49 ± 0.253 | 7.61 ± 0.919 | 3.32 ± 0.324 | 5.00 ± 0.646 | <0.0001 | <0.0001 | 0.855 | 0.396 | |
Dry matter digestibility (%) | 66.24 ± 4.175 | 54.96 ± 1.785 | 64.48 ± 2.804 | 56.95 ± 4.061 | 72.74 ± 4.981 | 64.01 ± 7.409 | <0.0001 | <0.0001 | 0.607 | 0.844 |
s.d., standard deviation; DM, dry matter; N, nitrogen; S, species; M, leaf maturity; T, soil treatment; B, block (elevation).
Foliage total and available N contents
The average total N content of the forest red gum foliage (1.45 ± 0.335 g/100 g DM) was higher (one-way ANOVA, F(2,69) = 3.72, P < 0.05; Tukey’s HSD Test, 95% C.I. = [0.013, 0.395]) than that of tallowwood foliage (1.24 ± 0.228 g/100 g DM) for both young and mature foliage combined; however, there was no difference (Tukey’s HSD Test, P > 0.05) in the average total N content of the foliage of tallowwood and grey gum (1.41 ± 0.255 g/100 g DM) or between grey gum and forest red gum foliage. The average total N content of new foliage (1.58 ± 0.204 g/100 g DM) was higher (one-way ANOVA, F(1,70) = 98.92, P < 0.001) than that of mature foliage (1.15 ± 0.166 g/100 g DM) for all species combined. The total N content of the new and mature foliage of the three Eucalyptus species is presented in Table 2. The average total N content of the foliage of the three Eucalyptus species (one-way ANOVA, F(2,69) = 4.02, P < 0.05) was higher for Block 1 (1.48 ± 0.275 g/100 g DM) compared with Block 3 (1.26 ± 0.288 g/100 g DM) (Tukey’s HSD Test, P < 0.05, 95% C.I. = [0.04, 0.42]); however, there was no difference (Tukey’s HSD Test, P > 0.05) between Block 1 and Block 2 (1.36 ± 0.263 g/100 g DM) or between Block 2 and Block 3. Soil treatment had no effect (one-way ANOVA, F(3,68) = 0.034, P > 0.05) on the total N content of the foliage of the three Eucalyptus species.
The average AvailN showed a difference between species (one-way ANOVA, F(2,69) = 3.90, P < 0.05) with the content of the forest red gum foliage (0.81 ± 0.220 g/100 g DM) higher (Tukey’s HSD Test, P < 0.05, 95% C.I. = [0.021, 0.286]) than that of grey gum foliage (0.66 ± 0.175 g/100 g DM); however, there was no difference (Tukey’s HSD Test, P > 0.05) between average AvailN content of the foliage of tallowwood (0.72 ± 0.177 g/100 g DM) and grey gum or between tallowwood and forest red gum. The average AvailN content of the new Eucalyptus foliage (0.74 ± 0.237 g/100 g DM) did not differ (one-way ANOVA, F(1,70) = 0.14, P > 0.05) from that of mature foliage (0.73 ± 0.155 g/100 g DM). The AvailN of the new and mature foliage of the three Eucalyptus species is presented in Table 2. The average AvailN differed between blocks (one-way ANOVA, F(2,69) = 5.62, P < 0.05) with foliage AvailN higher for Block 1 (0.83 ± 0.214 g/100 g DM) compared with Block 3 (0.65 ± 0.171 g/100 g DM) (Tukey’s HSD Test, P < 0.05, 95% C.I. = [0.049, 0.309]); however, there was no difference (Tukey’s HSD Test, P > 0.05) between Block 1 and Block 2 (0.72 ± 0.175 g/100 g DM) or between Block 2 and Block 3. Soil treatment had no effect (one-way ANOVA, F(3,68) = 1.66, P > 0.05) on the AvailN content of the foliage of the three Eucalyptus species.
Foliage ash content
There was a difference in average ash content (one-way ANOVA, F(2,69) = 9.20, P < 0.0001), with that of tallowwood foliage (4.16 ± 0.994 g/100 g DM) lower than that of both grey gum foliage (6.05 ± 1.727 g/100 g DM) (Tukey’s HSD Test, P < 0.0001, 95% C.I. = [0.93, 2.86]) and forest red gum foliage (5.87 ± 1.373 g/100 g DM) (Tukey’s HSD Test, P < 0.0001, 95% C.I. = [0.75, 2.28]); however, there was no difference (Tukey’s HSD Test, P > 0.05) in the foliage ash content of the grey gum and forest red gum.
The average ash content of new foliage (4.21 ± 0.722 g/100 DM) was lower (one-way ANOVA, F(1,70) = 72.02, P < 0.0001) than that of mature foliage (6.51 ± 1.454 g/100 DM). The ash content of the young and mature foliage for each of the three tree species is presented in Table 2. For the new foliage, the ash content of tallowwood was lower (one-way ANOVA, F(2,33) = 79.63; Tukey’s HSD Test, P < 0.0001, 95% C.I. = [0.86, 1.48]) than that of grey gum; however, there was no difference (Tukey’s HSD Test, P > 0.05) in the new foliage ash content between tallowwood and forest red gum or between grey gum and forest red gum. For the mature foliage, there was a difference (one-way ANOVA, F(2,33) = 24.16, P < 0.0001) in ash content between all species, with tallowwood having the lowest and grey gum the highest. There were no interactions (one-way ANOVA, F(3,68) = 2.74, P > 0.05) between species and soil treatment, or species and block (one-way ANOVA, F(3,68) = 2.74, P > 0.05).
Foliage dry matter digestibility
There was a difference between species in average DMD (one-way ANOVA, F(2,69) = 11.21, P < 0.0001). The average DMD of tallowwood foliage (68.38 ± 7.615%) was higher (Tukey’s HSD Test, P < 0.0001, 95% C.I. = [3.27, 12.28]) than that of both forest red gum (60.60 ± 6.56%) and grey gum (60.71 ± 5.145%) (Tukey’s HSD Test, P < 0.001, 95% C.I. = [3.15, 12.17]); however, there was no difference (Tukey’s HSD Test, P > 0.05) between the foliage DMD of forest red gum and grey gum.
The average DMD of new foliage (67.82 ± 5.359%) was higher (one-way ANOVA, F(1,70) = 44.85, P < 0.0001) than that of mature foliage (58.64 ± 6.241%), and this was consistent across all three tree species (Table 2). For both new and mature foliage, DMD was higher (new foliage: one-way ANOVA, F(2,33) = 13.6, P < 0.001; mature foliage: one-way ANOVA, F(2,32) = 10.14, P < 0.001) for tallowwood compared with both forest red gum and grey gum; however, there was no difference (Tukey’s HSD test, P > 0.05) in foliage DMD between forest red gum and grey gum (Table 2). There were no interactions between species and soil treatment (one-way ANOVA, F(3,31) = 2.91, P > 0.05), or species and block (one-way ANOVA, F(3,31) = 2.91).
Discussion
Seedling growth versus soil preparation methods
Soil treatments have been shown to impact the establishment and growth of vegetation across a variety of studies (Hammer et al. 2015; Navroski et al. 2016; Crous 2017). In this study, the addition of either Terracottem™, mycorrhiza or both may have influenced the initial establishment and growth of the trees (for the 2019 pilot study only species data and not treatment data were collected) but by ~2.5 years after planting, there was no difference in seedling height, stem diameter or canopy cover according to the soil treatments.
Terracottem™, a commonly used commercial soil treatment applied in the establishment process of industrial wood plantations (Crous 2017) has been shown to increase initial tree height and canopy coverage (Navroski et al. 2016). In their study involving Austrian pine (Pinus nigra), Bulíř (2006) found that application of Terracottem™ at the time of planting resulted in better growth of trees (compared to the control) in the second year of growth, but by the third and fourth years there were no significant differences. Likewise, the seedlings in this study were entering their third year of growth. This suggests that the reported positive effects of Terracottem™ on seedling establishment are short term in nature.
Inoculation of seedlings with mycorrhiza has been shown to increase plant biomass (Duponnois et al. 2005), increase canopy coverage (Lu and Koide 1994), and increase plant growth (Hammer et al. 2015). These responses have been attributed to increased root biomass (Gujre et al. 2021), protection from disease (Huggins 2020) and increased drought tolerance (Mason et al. 2000). Inoculation of Eucalyptus species typically occurs at, and is assessed at, the nursery seedling stage (Adjoud et al. 1996; Dunstan et al. 1998; Lu et al. 1998; Chen et al. 2000). The intensity of root colonisation and associated increased root biomass varies depending on the ectomycorrhiza used and the host species, and is reflected in species differences in seedling growth (Adjoud et al. 1996). Chen et al. (2000) reported that differences in plant height (for E. globulus and E. urophylla) in response to mycorrhiza treatments were apparent after 10 weeks. As for Terracottem™ treatments, our results suggest that the benefits of inoculating seedlings with mycorrhiza appear to be short term in nature.
Although we recorded no differences in seedling measurements in response to soil treatments, a significant block effect was shown. The three blocks for this design were located down a hillslope, and therefore, block effect may have been linked to slope. The gradient and elevation difference was relatively small; however, there were noticeable localised changes in slope, which may promote other environmental factors such as soil properties and water availability, which were not investigated. Other studies have described how such gradients can influence revegetation success (Jin et al. 2008; Liang et al. 2016). Differences in elevation may also influence water absorption rates (Chen et al. 2000) and also contributed to the observed block effect. However, such assertions are speculative, as soil properties within each block were not evaluated in this study.
Foliage chemical analyses – moisture
Typically, Eucalyptus foliage moisture thresholds fall between the range of 55% to 65% (Pahl and Hume 1990; Hume and Esson 1993; Moore and Foley 2000). In the current study, the moisture content of the mature foliage for all three species (53–58%) was within this range; however, that of new foliage was >70% for all three species. Young plant material typically has a higher moisture content than mature material, and this has been previously reported for Eucalyptus foliage (Larsson and Ohmart 1988; Steinbauer 2001).
These moisture level results we recorded for young and mature leaves are critical for managers in terms of understanding koala feeding behaviours.
Hume and Esson (1993) found koalas predominantly consume foliage with moisture levels of more than 55%. Thus, on this basis both the new and mature foliage results we recorded for all three Eucalyptus species would provide suitable food for koalas in terms of moisture. Importantly, we found that moisture levels varied according to leaf age. However, Moore and Foley (2000) highlighted that moisture thresholds should be used with some caution when determining if foliage is a ‘good’ food source, because environmental factors, koala biology, as well as nutrient and deterrent concentrations in foliage can also influence diet selection.
Foliage total and available N contents
Koalas may select Eucalyptus foliage based on its N content, and have been observed visiting and consuming foliage from trees with known higher N concentrations (Moore and Foley 2005; Stalenberg et al. 2014). The total amount of N present in Eucalyptus foliage generally falls within the range of 0.8–2.0% of DM (Fox and Macauley 1977; DeGabriel et al. 2010; Stalenberg et al. 2014), as was found in the current study (~1.0–1.8% N). While no differences in foliage total N concentration were found between species, it was found that young foliage had a higher total N concentration than mature foliage. This has been reported in several other studies (Fox and Macauley 1977; Goodger et al. 2006). In this study, AvailN ranged between 0.65 and 0.83 g/100 g DM, with no difference between new and mature foliage. AvailN is a measure of foliage protein that considers the influence of fibre and tannins on the digestibility of N (Au et al. 2019) and young foliage typically has higher tannin activity as a deterrent to herbivory (Gras et al. 2005), and, in this case, counteracting the higher total N (of the new foliage compared to mature foliage). Wallis et al. (2010) suggested that AvailN better explained differences in diet selection than total N concentration. Stalenberg et al. (2014) found koalas selected individual trees with higher foliar concentrations of AvailN, when compared with a neighbouring tree of the same species. Rus et al. (2021) also found that AvailN was an important factor for koala tree selection. The average foliage AvailN concentrations for all three species were higher than reported by Wallis et al. (2010) and Youngentob et al. (2011) but lower than that reported by Au et al. (2019) for other Eucalyptus species. This means that in the context of this study, AvailN varied between species, but was not affected by foliage age and thus AvailN would not be an influencing factor in the selection of new versus mature foliage by koalas.
Some species differences in AvailN were found, with the average AvailN content of the forest red gum foliage higher than that of grey gum foliage; however, there were no differences between tallowwood and grey gum or between tallowwood and forest red gum. Wallis et al. (2010) and Jensen et al. (2014) also found significant differences in AvailN between species. If koalas were selecting foliage on the basis of AvailN, koalas would not preferentially select new foliage over mature foliage but would select the foliage from forest red gum over that of grey gum.
Soil treatments applied at the time of planting had no impact on the AvailN concentration of new or mature Eucalyptus foliage harvested ~2.5 years after establishment. This has an impact on only selecting soil treatments that enhance establishment of seedlings rather than selecting for improving nutritional quality of leaves for captive koalas in future plantations.
Foliage ash content
Foliage ash content, which represents the mineral content of plants, varied according to tree species and foliage age. The average ash content of tallowwood foliage (~4.2 g/100 g DM) was significantly lower than that of both grey gum (~6.1 g/100 g DM) and forest red gum foliage (~5.9 g/100 g DM). These ash contents were higher than those reported for other species, e.g. E. citriodora and E. camaldulensis foliage (Bello et al. 2013). Dickinson and Kirkpatrick (1985) also reported differences in ash content between Eucalyptus species. The average ash content of new foliage (~4.2 g/100 g DM) was lower than that of mature foliage (~6.51 g/100 g DM), which contrasted with that reported by Dickinson and Kirkpatrick (1985). The effect of foliage ash content on food value and diet selection by koalas appears unknown and warrants further research.
Foliage dry matter digestibility (DMD)
DMD varied between tree species and with foliage age. For the three Eucalyptus species in vitro DMD ranged from ~55% to 73% which was similar to the range of 54–70% reported by Ullrey et al. (1981), 46–66% reported by Harrop and Degabriele (1976) and 60% reported by Eberhard et al. (1975) from in vivo measures.
In this study, the average DMD of tallowwood foliage (68.4%) was higher than that of both forest red gum (60.6%) and grey gum (60.7%). Brice et al. (2019) also reported species differences in Eucalyptus DMD. Stalenberg et al. (2014) found koalas selected individual trees with higher foliar DMD when compared with a neighbouring tree of the same species.
Study results for DMD in new foliage (67.8%) as compared to mature foliage (58.6%), suggest that new tallowwood foliage may be selected in preference by koalas and this should be investigated further along with other factors such as secondary metabolites.
Conclusions
In the context of this study, in a 2.5-year-old plantation on the NSW Mid North Coast, tallowwood, grey gum and forest red gum foliage had differences in AvailN, ash and DMD, but not moisture levels. In addition, young foliage had higher DMD and moisture content but no difference in AvailN compared with mature foliage. Foliage chemistry is an important factor for koala diet selection, both in the wild and captivity. These results build on the importance of providing captive koalas with a variety of different Eucalyptus species and foliage maturity which ensures that they can select species and nutrients that they require.
Soil treatments were also tested and found that 2.5 years on from initial planting had no observable impact on the foliage chemistry or the difference in growth among species. Other studies have found that when soil treatments were applied at planting, they did have an impact on the growth and establishment. Further studies could be conducted to see if these observations apply to Eucalyptus species of tallowwood, grey gum, and forest red gum.
Eucalyptus species also contain plant secondary metabolites that may influence the diet selection of koalas. Future studies should be undertaken to further investigate foliage FPC concentrations for the selected plantation eucalypt species, to further inform future plantation development and captive management feeding practice.
Acknowledgements
We thank Lachlan Binskin, Kara Westaway and Joey Hammerton, who assisted us in the field. We also thank Richard Meyer for his assistance with the forage chemistry analyses. The assistance of Brooke Sanders and Stephanie McManus with the GPS surveying was also greatly appreciated.
References
Adams F, Reddell P, Webb MJ, Shipton WA (2006) Arbuscular mycorrhizas and ectomycorrhizas on Eucalyptus grandis (Myrtaceae) trees and seedlings in native forests of tropical north-eastern Australia. Australian Journal of Botany 54(3), 271-281.
| Crossref | Google Scholar |
Adjoud D, Plenchette C, Halli-Hargas R, Lapeyrie F (1996) Response of 11 Eucalyptus species to inoculation with three arbuscular mycorrhizal fungi. Mycorrhiza 6(2), 129-135.
| Crossref | Google Scholar |
Andrew RL, Peakall R, Wallis IR, Wood JT, Knight EJ, Foley WJ (2005) Marker-based quantitative genetics in the wild?: The heritability and genetic correlation of chemical defenses in Eucalyptus. Genetics 171(4), 1989-1998 PMID:.
| Crossref | Google Scholar | PubMed |
Asquith TN, Butler LG (1986) Interactions of condensed tannins with selected proteins. Phytochemistry 25(7), 1591-1593.
| Crossref | Google Scholar |
Au J, Marsh KJ, Wallis IR, Foley WJ (2013) Whole-body protein turnover reveals the cost of detoxification of secondary metabolites in a vertebrate browser. Journal of Comparative Physiology. B, Biochemical, Systemic, and Environmental Physiology 183(7), 993-1003 PMID:.
| Crossref | Google Scholar | PubMed |
Au J, Clark RG, Allen C, Marsh KJ, Foley WJ, Youngentob KN (2019) A nutritional mechanism underpinning folivore occurrence in disturbed forests. Forest Ecology and Management 453, 117585.
| Crossref | Google Scholar |
Australian Government (2022) AUSGIN Geoscience Portal. Available at https://portal.geoscience.gov.au/ [Accessed 27 February 2022]
Bello MO, Olabanji IO, Ibrahim AO, Yekeen TA, Oboh LM (2013) Nutraceuticals in leaves of Eucalyptus citriodora and Eucalyptus camaldulensis. Food Science 62, 17873-17876.
| Google Scholar |
Bercovitch FB (2007) Biodiversity Animal Treasury: Connecting conservation biology with evolutionary ecology: the case of the koala. Biodiversity 8(3), 33-37.
| Crossref | Google Scholar |
Brice KL, Trivedi P, Jefferies TC, Blyton MDJ, Mitchell C, Singh BK, Moore BD (2019) The koala (Phascolarctos cinereus) faecal microbiome differs with diet in a wild population. PeerJ 7, 6534 PMID:.
| Crossref | Google Scholar | PubMed |
Bulíř P (2006) Growth of Austrian pine (Pinus nigra Arnold) treated with soil conditioners on Loket spoil bank. Journal of Forest Science 52(12), 556-564.
| Crossref | Google Scholar |
Bureau of Meteorology (2022a) Climate Classification Map. Available at http://www.bom.gov.au/jsp/ncc/climate_averages/climate-classifications/index.jsp?maptype=seasgrpb#map [Accessed 28 February 2022].
Bureau of Meteorology (2022b) Climate Statistics for Australian Locations – Summary Statistics Yarras (Mount Seaview). Available at http://www.bom.gov.au/climate/averages/tables/cw_060085.shtml [Accessed 24 February 2022].
Chen YL, Brundrett MC, Dell B (2000) Effects of ectomycorrhizas and vesicular–arbuscular mycorrhizas, alone or in competition, on root colonization and growth of Eucalyptus globulus and E. urophylla. The New Phytologist 146(3), 545-555.
| Crossref | Google Scholar |
Chiorescu E (2019) Research on the influence of hydrogels stockosorb and terracottem on the development of some agricultural plants species. Lucrări Ştiinţifice 62(1), 29-32.
| Google Scholar |
Cork SJ (1986) Foliage of Eucalyptus punctata and the maintenance nitrogen requirements of koalas, Phascolarctos cinereus. Australian Journal of Zoology 34(1), 17-23.
| Crossref | Google Scholar |
Cork SJ, Hume ID, Dawson T (1983) Digestion and metabolism of a natural foliar diet (Eucalyptus punctata) by an arboreal marsupial, the koala (Phascolarctos cinereus). Journal of Comparative Physiology 153(2), 181-190.
| Crossref | Google Scholar |
Crous JW (2017) Use of hydrogels in the planting of industrial wood plantations. Southern Forests: A Journal of Forest Science 79(3), 197-213.
| Crossref | Google Scholar |
DeGabriel JL, Wallis IR, Moore BD, Foley WJ (2008) A simple, integrative assay to quantify nutritional quality of browses for herbivores. Oecologia 156(1), 107-116 PMID:.
| Crossref | Google Scholar | PubMed |
DeGabriel JL, Moore BD, Marsh KJ, Foley WJ (2010) The effect of plant secondary metabolites on the interplay between the internal and external environments of marsupial folivores. Chemoecology 20(2), 97-108.
| Crossref | Google Scholar |
Dickinson KJM, Kirkpatrick JB (1985) The flammability and energy content of some important plant species and fuel components in the forests of southeastern Tasmania. Journal of Biogeography 12, 121-134.
| Crossref | Google Scholar |
Dunstan WA, Malajczuk N, Dell B (1998) Effects of bacteria on mycorrhizal development and growth of container grown Eucalyptus diversicolor F. Muell. Seedlings. Plant and Soil 201(2), 241-249.
| Crossref | Google Scholar |
Duponnois R, Colombet A, Hien V, Thioulouse J (2005) The mycorrhizal fungus Glomus intraradices and rock phosphate amendment influence plant growth and microbial activity in the rhizosphere of Acacia holosericea. Soil Biology and Biochemistry 37(8), 1460-1468.
| Crossref | Google Scholar |
Eberhard IH, McNamara J, Pearse RJ, Southwell IA (1975) Ingestion and excretion of Eucalyptus punctata Dc and its essential oil by the koala, Phascolarctos cinereus (Goldfuss). Australian Journal of Zoology 23(2), 169-179.
| Crossref | Google Scholar |
Fiala ACS, Garman SL, Gray AN (2006) Comparison of five canopy cover estimation techniques in the western Oregon Cascades. Forest Ecology and Management 232(1–3), 188-197.
| Crossref | Google Scholar |
Fox LR, Macauley BJ (1977) Insect grazing on Eucalyptus in response to variation in leaf tannins and nitrogen. Oecologia 29(2), 145-162 PMID:.
| Crossref | Google Scholar | PubMed |
Goodger JQD, Gleadow RM, Woodrow IE (2006) Growth cost and ontogenetic expression patterns of defence in cyanogenic Eucalyptus spp. Tree 20(6), 757-765.
| Crossref | Google Scholar |
Gras EK, Read J, Mach CT, Sanson GD, Clissold FJ (2005) Herbivore damage, resource richness and putative defences in juvenile versus adult Eucalyptus leaves. Australian Journal of Botany 53(1), 33-44.
| Crossref | Google Scholar |
Gujre N, Soni A, Rangan L, Tsang DCW, Mitra S (2021) Sustainable improvement of soil health utilizing biochar and arbuscular mycorrhizal fungi: a review. Environmental Pollution 268, 115549 PMID:.
| Crossref | Google Scholar | PubMed |
Hammer EC, Forstreuter M, Rillig MC, Kohler J (2015) Biochar increases arbuscular mycorrhizal plant growth enhancement and ameliorates salinity stress. Applied Soil Ecology 96, 114-121.
| Crossref | Google Scholar |
Harrop CJF, Degabriele R (1976) Digestion and nitrogen metabolism in the koala, Phascolarctos cinereus. Australian Journal of Zoology 24(2), 201-215.
| Crossref | Google Scholar |
Huggins N (2020) Mycorrhizal Fungi: Nature’s Gardeners. Available at https://www.plantingsystemsaustralia.com.au/blogs/news/mycorrhizal-fungi-nature-s-gardeners [Accessed 7 June 2022].
Hume ID, Esson C (1993) Nutrients, antinutrients and leaf selection by captive koalas (Phascolarctos cinereus). Australian Journal of Zoology 41, 379-392.
| Crossref | Google Scholar |
Jensen LM, Wallis IR, Marsh KJ, Moore BD, Wiggins NL, Foley WJ (2014) Four species of arboreal folivore show differential tolerance to a secondary metabolite. Oecologia 176(1), 251-258 PMID:.
| Crossref | Google Scholar | PubMed |
Jin XM, Zhang YK, Schaepman ME, Clevers JGPW, Su Z (2008) Impact of elevation and aspect on the spatial distribution of vegetation in the Qilian mountain area with remote sensing data. In ‘XXIth ISPRS Congress’, 3 July 2008–11 July 2008, Beijing. pp. 1385–1390. (International Society for Photogrammetry and Remote Sensing)
Johnson ED (1926) A comparison of the juvenile and adult leaves of Eucalyptus globulus. The New Phytologist 25(3), 202-212.
| Crossref | Google Scholar |
Johnson RN, O’Meally D, Chen Z, Etherington GJ, Ho SYW, Nash WJ, Grueber CE, Cheng Y, Whittington CM, Dennison S, Peel E, et al. (2018) Adaptation and conservation insights from the koala genome. Nature Genetics 50(8), 1102-1111 PMID:.
| Crossref | Google Scholar | PubMed |
Krockenberger AK, Hume ID, Cork SJ (1998) Production of milk and nutrition of the dependent young of free-ranging koalas (Phascolarctos cinereus). Physiological Zoology 71(1), 45-56 PMID:.
| Crossref | Google Scholar | PubMed |
Larsson S, Ohmart CP (1988) Leaf age and larval performance of the leaf beetle Paropsis atomaria. Ecological Entomology 13(1), 19-24.
| Crossref | Google Scholar |
Liang J, Reynolds T, Wassie A, Collins C, Wubalem A (2016) Effects of exotic Eucalyptus spp. plantations on soil properties in and around sacred natural sites in the northern Ethiopian Highlands. AIMS Agriculture and Food 1(2), 175-193.
| Crossref | Google Scholar |
Lu X, Koide RT (1994) The effects of mycorrhizal infection on components of plant growth and reproduction. New Phytologist 128(2), 211-218 PMID:.
| Crossref | Google Scholar | PubMed |
Lu X, Malajczuk N, Dell B (1998) Mycorrhiza formation and growth of Eucalyptus globulus seedlings inoculated with spores of various ectomycorrhizal fungi. Mycorrhiza 8(2), 81-86.
| Crossref | Google Scholar |
Marsh KJ, Moore BD, Wallis IR, Foley WJ (2013) Continuous monitoring of feeding by koalas highlights diurnal differences in tree preferences. Wildlife Research 40(8), 639-646.
| Crossref | Google Scholar |
Marsh KJ, Moore BD, Wallis IR, Foley WJ (2014) Feeding rates of a mammalian browser confirm the predictions of a ‘foodscape’ model of its habitat. Oecologia 174(3), 873-882 PMID:.
| Crossref | Google Scholar | PubMed |
Marsh KJ, Ward J, Wallis IR, Foley WJ (2018) Intraspecific variation in nutritional composition affects the leaf age preferences of a mammalian herbivore. Journal of Chemical Ecology 44, 62-71 PMID:.
| Crossref | Google Scholar | PubMed |
Marsh KJ, Saraf I, Hocart CH, Youngentob K, Singh IP, Foley WJ (2019) Occurrence and distribution of unsubstituted B-ring flavanones in Eucalyptus foliage. Phytochemistry 160, 31-39 PMID:.
| Crossref | Google Scholar | PubMed |
Marsh KJ, Blyton MDJ, Foley WJ, Moore BD (2021) Fundamental dietary specialisation explains differential use of resources within a koala population. Oecologia 196(3), 795-803 PMID:.
| Crossref | Google Scholar | PubMed |
Martin RW (1985) Overbrowsing, and decline of a population of the koala, Phascolarctos cinereus, in Victoria. I. Food preference and food tree defoliation. Wildlife Research 12(3), 355-365.
| Crossref | Google Scholar |
Mason PA, Ibrahim K, Ingleby K, Munro RC, Wilson J (2000) Mycorrhizal development and growth of inoculated Eucalyptus globulus (Labill.) seedlings in wet and dry conditions in the glasshouse. Forest Ecology and Management 128(3), 269-277.
| Crossref | Google Scholar |
Melzer A, Cristescu R, Ellis W, FitzGibbon S, Manno G (2014) The habitat and diet of koalas (Phascolarctos cinereus) in Queensland. Australian Mammalogy 36, 189-199.
| Crossref | Google Scholar |
Moore BD, Foley WJ (2000) A review of feeding and diet selection in koalas (Phascolarctos cinereus). Australian Journal of Zoology 48, 317-333.
| Crossref | Google Scholar |
Moore BD, Foley WJ (2005) Tree use by koalas in a chemically complex landscape. Nature 435(7041), 488-490 PMID:.
| Crossref | Google Scholar | PubMed |
Moore BD, Wallis IR, Wood JT, Foley WJ (2004) Foliar nutrition, site quality, and temperature influence foliar chemistry of tallowwood (Eucalyptus microcorys). Ecological Monographs 74(4), 553-568.
| Crossref | Google Scholar |
Moore BD, Foley WJ, Wallis IR, Cowling A, Handasyde KA (2005) Eucalyptus foliar chemistry explains selective feeding by koalas. Biology Letters 1(1), 64-67 PMID:.
| Crossref | Google Scholar | PubMed |
Navroski MC, Araújo MM, Cunha FS, Berghetti ÁLP, Pereira MO (2016) Reduction of manure and improvement of the characteristics of substrate using hydrogel in the seedling production of Eucalyptus dunnii Maiden. Ciência Florestal 26(4), 1155-1165.
| Crossref | Google Scholar |
Noble IR (1989) Ecological traits of the Eucalyptus L’Herit subgenera Monocalyptus and Symphyomyrtus. Australian Journal of Botany 37(3), 207-224.
| Crossref | Google Scholar |
Pennisi E (2018) A koala’s diet would kill most mammals. Their genome reveals how they survive. Available at https://www.science.org/content/article/koala-s-diet-would-kill-most-mammals-their-genome-reveals-how-they-survive
R Core Team (2022) R: A language and environment for statistical computing. R Foundation for Statistical Computing, Vienna, Austria. Available at https://www.R-project.org/
Rus AI, McArthur C, Mella VSA, Crowther MS (2021) Habitat fragmentation affects movement and space use of a specialist folivore, the koala. Animal Conservation 24(1), 26-37.
| Crossref | Google Scholar |
Santana MC, Pereira AP, de Bacco Lopes BA, Robin A, Silva AM, Bran Nogueira Cardoso EJ (2020) Mycorrhiza in mixed plantations. In ‘Mixed plantations of Eucalyptus and leguminous trees: soil, microbiology and ecosystem services.’ (Eds EJ Bran Nogueira Cardoso, JLdM Gonçalves, FdC Balieiro, AA Franco) (pp. 137–154) (Springer International Publishing)
Stalenberg E, Wallis IR, Cunningham RB, Allen C, Foley WJ (2014) Nutritional correlates of koala persistence in a low-density population. PLoS ONE 9(12), e113930 PMID:.
| Crossref | Google Scholar | PubMed |
State of New South Wales and Department of Planning, Industry, and Environment (DPIE) (2019) Koala habitat information base technical guide. Available at https://www.environment.nsw.gov.au/-/media/OEH/Corporate-Site/Documents/Animals-and-plants/Threatened-species/koala-habitat-information-base-technical-guide-190534.pdff
State of New South Wales and Office of Environment and Heritage (OEH) (2018) A review of koala tree use across New South Wales. Available at https://www.environment.nsw.gov.au/research-and-publications/publications-search/a-review-of-koala-tree-use-across-new-south-wales
Steinbauer MJ (2001) Specific leaf weight as an indicator of juvenile leaf toughness in Tasmanian bluegum (Eucalyptus globulus ssp. globulus): implications for insect defoliation. Australian Forestry 64(1), 32-37.
| Crossref | Google Scholar |
Thornhill AH, Crisp MD, Külheim C, Lam KE, Nelson LA, Yeates DK, Miller JT (2019) A dated molecular perspective of eucalypt taxonomy, evolution and diversification. Australian Systematic Botany 32, 29-48.
| Crossref | Google Scholar |
Tibbett M, Daws MI, Ryan MH (2022) Phosphorus uptake and toxicity are delimited by mycorrhizal symbiosis in P-sensitive Eucalyptus marginata but not in P-tolerant Acacia celastrifolia. AoB Plants 14(5), plac037.
| Crossref | Google Scholar |
Ullrey DE, Robinson PT, Whetter PA (1981) Eucalyptus digestibility and digestible energy requirements of adult male koalas, Phascolarctos cinereus (Marsupialia). Australian Journal of Zoology 29(6), 847-852.
| Crossref | Google Scholar |
Wallis IR, Nicolle D, Foley WJ (2010) Available and not total nitrogen in leaves explains key chemical differences between the eucalypt subgenera. Forest Ecology and Management 260(5), 814-821.
| Crossref | Google Scholar |
Wu H, McAlpine C, Seabrook L (2012) The dietary preferences of koalas, Phascolarctos cinereus, in southwest Queensland. Australian Zoologist 36(1), 93-102.
| Crossref | Google Scholar |
Youngentob KN, Wallis IR, Lindenmayer DB, Wood JT, Pope ML, Foley WJ (2011) Foliage chemistry influences tree choice and landscape use of a gliding marsupial folivore. Journal of Chemical Ecology 37(1), 71-84 PMID:.
| Crossref | Google Scholar | PubMed |