Den selection and movement patterns in a tropical savanna population of the northern quoll (Dasyurus hallucatus)
Gavin J. Trewella

A
Abstract
Small mammals are undergoing significant declines across the tropical savannas of northern Australia despite a lack of widespread land clearing. The causes of these declines remain unresolved, but a growing body of evidence suggests that the structural simplification of savannas, namely the degradation of under- and overstorey vegetation by frequent fires and high densities of exotic megaherbivores, is likely to be a significant contributing factor.
We sought to investigate how declining mammals use critical denning resources in high-biomass mesic savannas to help explain drivers of mammal decline in more open and less mesic areas and inform management priorities.
We fitted VHF/GPS collars to nine northern quolls (Dasyurus hallucatus), an Endangered scansorial predatory mammal, from a remnant population located on bauxite plateaus on Cape York Peninsula, Australia, to monitor their nocturnal movement patterns and den use over 1 month.
During this period, northern quolls exclusively denned in trees (either standing hollow trees or fallen logs). The most frequently used den trees tended to be larger, with pronounced trunk leans and multiple hollow entrances from 5 to 20 cm in diameter, whereas the most frequently used den logs tended to be longer and had more hollow entrances from 10 to 20 cm in diameter. All home ranges were confined to the high-biomass savanna habitat found on the bauxite plateaus, with males having a mean home range almost double the size of the mean female home range.
The reliance of this population of northern quolls on large hollow-bearing trees for shelter may explain the regional contraction of the northern quoll to high-biomass mesic savannas with an abundance of large trees.
These high-biomass savanna habitats, such as the bauxite plateaus found on the Cape York Peninsula, are likely to be critical for the persistence of the northern quoll, and should be appropriately protected, through management of fire and avoidance of clearing and logging.
Keywords: bauxite, Dasyuridae, fire management, mammal decline, nocturnal, telemetry, threatened species, tree hollows.
Introduction
The availability of critical habitat resources strongly influences how species use space (Cameron and Spencer 1985; Barton et al. 1992; Berry et al. 2019). The local distribution of resources, such as shelter, food, water and access to potential mates, is often what constitutes an animal’s home range (South 1999; Horne et al. 2008; Powell and Mitchell 2012). As such, the conservation of threatened fauna often relies on understanding the resources fauna require and prioritising management strategies that maximise the availability of those resources (Simberloff and Cox 1987; Macdonald and Rushton 2003; Thornton et al. 2011; Pavey et al. 2017).
Hollow-bearing trees are a critical resource for vertebrate animals in many Australian ecosystems (McElhinny et al. 2006; Goldingay 2011; Remm and Lõhmus 2011). For example, tree hollows provide shelter to ~31% of Australia’s native non-volant mammal species (Gibbons and Lindenmayer 2002), with hollow-dependent mammals preferring large, old trees with numerous large hollows (Goldingay 2011; Lindenmayer et al. 2017; Stobo-Wilson et al. 2021). The European invasion of Australia in the late 18th Century resulted in widespread logging and land-clearing, with particular intensity in the temperate regions of southern Australia, causing significant losses of large hollow-bearing trees (Bradshaw 2012; Kyle and Duncan 2012). As such, many hollow-dependent mammals in temperate Australia are now strongly associated with ‘old-growth’ forests that are characterised by a long absence of severe disturbances (e.g. logging and/or high-intensity wildfire) (Scotts 1991; Incoll et al. 2001) and which have maintained a diverse stand structure of hollow-bearing trees and forest-floor logs (Lindenmayer et al. 1999, 2000; Franklin and Van Pelt 2004).
In contrast, the tropical savannas of northern Australia have remained intact and free from intensive anthropogenic activity (Kutt et al. 2012; Woinarski et al. 2020). Despite this, a wide range of small mammal species have experienced severe ongoing declines across northern Australia in recent decades, with the precise causes remaining uncertain (Woinarski et al. 2010, 2011; Stobo-Wilson et al. 2019; von Takach et al. 2020). The eucalypt-dominated savannas of northern Australia are characterised by regimes of frequent disturbance (e.g. seasonally driven, annual fires and wind-throw events caused by storms and tropical cyclones). However, contemporary land management is believed to have shifted fire regimes from low-intensity, early dry-season (May–July) fires towards hotter, more intensive late dry-season (August–November) fires while also proliferating introduced megaherbivores (Freeland 1990; Russell-Smith 2001; Connor et al. 2018). These changes to savanna disturbance regimes are widely believed to have altered vegetation structure by killing large hollow-bearing trees, which are more susceptible to mortality following wildfires (Williams et al. 1999; Liedloff and Cook 2007), and destroying ground-level shelter resources such as fallen logs during fire, and reducing the density of understorey vegetation (Sharp and Whittaker 2003; Kutt and Woinarski 2007), resulting in a simplified vegetation structure (Williams et al. 1999; Russell-Smith et al. 2012).
Large hollow-bearing trees remain the preferred den resource for hollow-using mammals in tropical savannas (Geyle et al. 2020; Penton et al. 2020b; Stobo-Wilson et al. 2021). Shrub density has also been identified as an important predictor of the abundance of scansorial (tree-climbing) mammal species, indicating a need for understorey vegetation complexity even within largely intact tropical savannas (Davies et al. 2018; Stobo-Wilson et al. 2019; Penton et al. 2021a). As such, the simplification of tropical savanna vegetation has occurred alongside a trend of small mammals contracting to regions of higher rainfall, which tend to support larger trees and greater vegetation structural complexity (McKenzie et al. 2007; Stobo-Wilson et al. 2019; von Takach et al. 2020). This pattern has led to suggestions that the loss of tree hollows, as well as other aspects of habitat complexity, especially in lower-rainfall areas, may be contributing to the decline of tropical savanna-inhabiting small mammals (Woinarski et al. 2014; Woolley et al. 2018; von Takach et al. 2020). These findings have provided support that resource availability and vegetation structural complexity play a significant role in promoting mammal persistence in tropical savannas.
Habitat structure is also intrinsically linked to predator–prey dynamics (Sih 1984; Warfe and Barmuta 2004; Gorini et al. 2012). Predation pressure plays a clear role in shaping how prey species use their environment by affecting their movement behaviour (Ritchie and Johnson 2009), with habitat structure often mediating the relationship between predators and prey (Gorini et al. 2012; McGregor et al. 2015; Miritis et al. 2020). For example, the cat (Felis catus), an introduced mesopredator, is known to increase its activity in areas where habitat structure is simplified, such as following high-intensity fires and/or in overgrazed areas (McGregor et al. 2014; Stobo-Wilson et al. 2020b; Trewella et al. 2023). This increase in cat activity is of great concern when considering that the cat has contributed to the extinction of at least 26 Australian mammals and is a significant threat to many threatened northern Australian mammals (Frank et al. 2014; Doherty et al. 2017; Woinarski et al. 2019). As such, improving our understanding of what resources are critical for small-mammal persistence in savannas will improve our understanding of how habitat structural complexity contributes to small-mammal persistence. Also, identifying how species movement patterns may be influenced by the availability of these critical resources highlights what habitat resources should be prioritised for protection by management programs. The northern quoll (Dasyurus hallucatus) is a native mammal that has experienced one of the most severe ongoing declines across northern Australia (Braithwaite and Griffiths 1994; Woinarski et al. 2010). Once widespread across a variety of northern Australian ecosystems, including savanna, rainforests, rocky escarpments, xeric shrublands and deserts, the northern quoll has severely contracted away from topographically simple, low-rainfall landscapes. It is now predominantly found in rocky uplands or mosaics of rocky uplands and flat savannas, in high-rainfall (≥1000 mm mean annual) regions (Braithwaite and Griffiths 1994; von Takach et al. 2020; Moore et al. 2021a). As such, much of the previous research investigating resource use by the northern quoll has been undertaken primarily in and around rocky settings (Oakwood 1997; Cremona 2015; Hernandez-Santin et al. 2022), and has suggested that the northern quoll preferentially uses rocky habitat because it provides ample den sites in rock crevices (Oakwood 1997; Moore et al. 2021b). As a result, we have a limited understanding of the resource requirements of the northern quoll in flat savanna without rocky habitat.
To address this knowledge gap, we monitored the movement patterns and den selection by the northern quoll in a remnant population restricted to high-rainfall, high-biomass Eucalyptus tetrodonta-dominated savanna on a bauxite plateau on Cape York Peninsula in northern-eastern Australia. By tracking individual northern quolls within this spatially limited environment, where rocky habitat features are entirely absent, we aimed to (i) identify the attributes of the habitat features used as den sites, and (ii) describe the home-range size and excursion behaviour of both male and female northern quolls during the breeding season. By identifying the critical denning resources utilised and the spatial distribution of this population of northern quolls, we will be able to understand better what resources contribute to their current persistence in this non-rocky savanna and, hence, whether decreasing resource availability may be contributing to the decline of small mammals across northern Australia.
Methods
Study species
Endemic to northern Australia, the northern quoll (body mass ~300–1200 g) is a predatory marsupial from the family Dasyuridae (Van Dyck and Strahan 2008). It is scansorial, while still being able to actively move and hunt on the ground (Oakwood 1997; Henderson et al. 2017). As such, the northern quoll can use a variety of den resources such as hollow trees, fallen logs, rock crevices and hollowed termite mounds (Oakwood 1997). Adults are primarily solitary, rarely sharing dens and only seeking out conspecifics during the breeding season between May and August (Oakwood 2002). Males display facultative semelparity, living for only 1 year and dying following the breeding season (Oakwood et al. 2001; Fisher et al. 2013). Breeding males expend a high mating effort, involving more time walking and less time sleeping than do their female counterparts (Gaschk et al. 2023), while travelling large distances in search of receptive females outside their own established home range (Oakwood 2002; Heiniger et al. 2020a). This exhaustive mating behaviour can increase male exposure to threats such as predators (Hernandez-Santin et al. 2016) and is a likely cause of post-mating die-off (Gaschk et al. 2023). Because of this annual die-off of males, the persistence of northern quoll populations is very sensitive to juvenile mortality (Cremona et al. 2017; Moro et al. 2019).
The observed decline of the northern quoll has been attributed to a range of landscape-specific factors, including increased fire frequency, feral cat and dingo (Canis familiaris) predation, habitat modification (e.g. land-clearing and mining) and grazing by megaherbivores (Moore et al. 2021a). However, it is also clear that the introduction and spread of the cane toad (Rhinella marina) across northern Australia has greatly accelerated the decline of the northern quoll, which is poisoned when it preys on the toxic cane toad (Woinarski et al. 2010; Doody et al. 2021). As such, the northern quoll is currently listed as Endangered by the IUCN (Oakwood et al. 2016).
Study area
This study was conducted in April and May 2019, east of the town of Weipa on the north-western coast of Cape York Peninsula in north-eastern Australia (Fig. 1). The study area has a tropical monsoon climate, with mean annual rainfall of 1700 mm, 90% of which occurs between November and April (Australian Bureau of Meteorology 2024). Savanna vegetation dominates the region, with low bauxite plateaus (<110 m above sea level) being distributed across the landscape. Surveys were undertaken across a ~4500-ha bauxite plateau known to be inhabited by the northern quoll. The vegetation of the plateau is tall open savanna dominated by Eucalyptus tetrodonta. Rugged, ecotonal zones occur along the edges of the plateau, grading into low-statured, open savanna surrounding the plateau. The plateau also experiences a fire frequency lower than in the surrounding savanna (~0.6 fires per year for plateaus vs 0.9 fires per year for the surrounding low-lying savanna over a 20-year period (North Australian Fire Information 2023).
Location of the study site near Weipa on Cape York Peninsula, Australia. Dark shading denotes the current distribution of the northern quoll, with the crossed light shading denoting its historical distribution (Moore et al. 2021a).
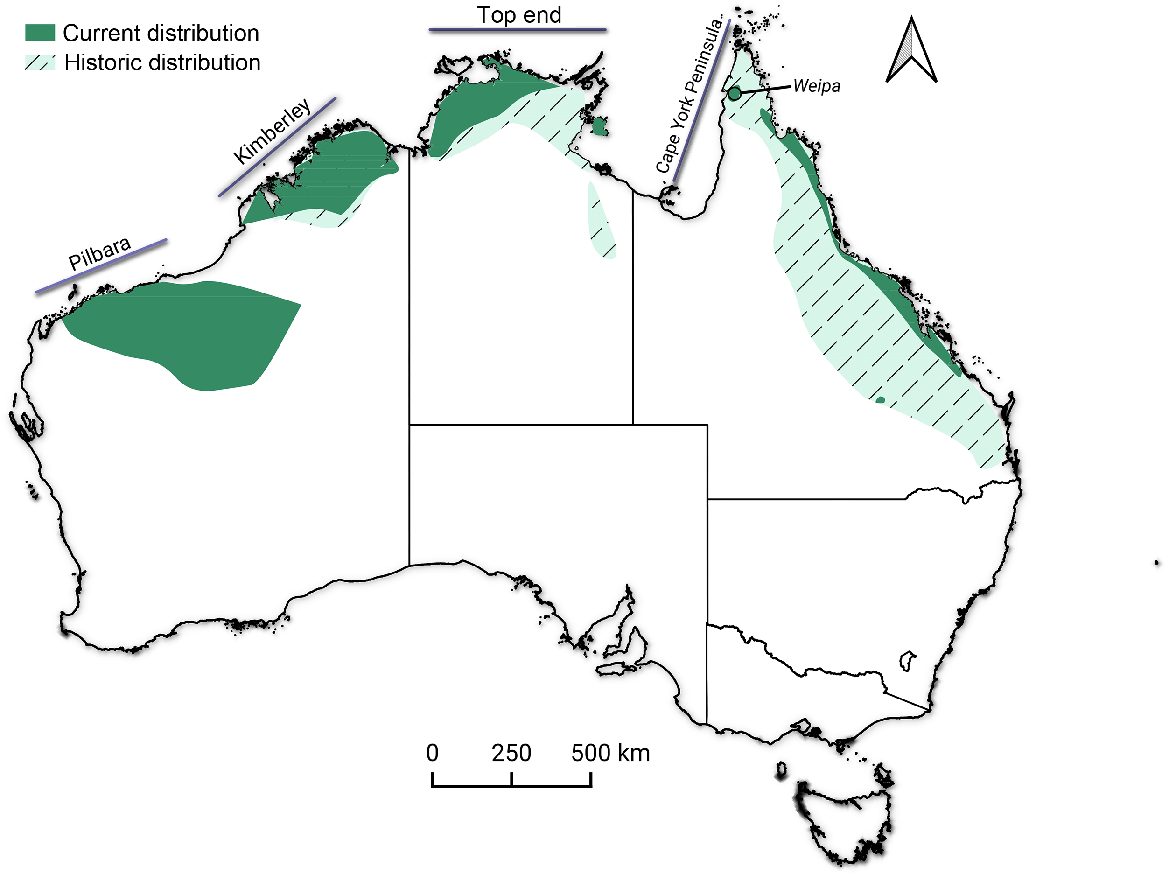
Trapping and GPS-tracking
Between April and May 2019, we established 19 trap lines over a 14-night period. Trap lines were positioned perpendicular to the road, with a minimum distance of 100 m between the lines. Each trap line had eight wire cage traps (66 × 24 × 26 cm), 25 m apart, and was baited with a mixture of peanut butter, oats and tuna for four consecutive nights, for a total of 608 trap-nights. In total, 13 northern quolls were captured during this trapping survey, including six females and seven males. Following ethical guidelines, only northern quolls weighing >400 g were used for this study, so as to ensure that the total weight of the tracking collar was <5% of the animal’s total bodyweight (Sikes and Animal Care and Use Committee of the American Society of Mammalogists 2016). Three trapped females were under the 400 g threshold and were therefore not collared. One male escaped prior to collaring, despite being of adequate weight, and, thus, was also not collared. All northern quolls were processed and released at their site of capture within an hour of sunrise.
Nine northern quolls (six males and three females) were collared and tracked for 11–19 days over a 30-day period. Each northern quoll was fitted with a GPS/VHF collar (LiteTrack 20; Lotek Wireless Inc. Havelock North, New Zealand). Each collar was programmed to record a GPS fix in 20-min intervals from 7 pm to 7 am, to align with the northern quoll’s nocturnal activity, and fixes recorded on each collar were transferred to a computer following recapture. Each collar was also programmed to emit a VHF signal for 2 h each morning for their diurnal den sites to be tracked and identified with a hand-held Yagi-Uda antenna and radio receiver (Sir Track Ultra; Lotek Wireless Inc., Havelock North, New Zealand) at least once every 2 days until their recapture. Collars were successfully retrieved by trapping one night at each northern quoll’s last identified den site following each collar emitting a distinct VHF signal indicating low battery. During the tracking survey, one collar became displaced inside a tree cavity and was unrecoverable. Another collar malfunctioned, giving low-quality GPS data, so we excluded the results from our home-range analysis but was still included in the den tracking surveys as the VHF functionality was still operational. Thus, we successfully tracked a total of eight northern quolls.
Den surveys
Throughout the survey period, our collared northern quolls denned exclusively in hollow trees and fallen logs. Therefore, we collected several variables from each den tree and log following Oakwood (1997). Each den tree was identified to species level, and measured for the diameter at breast height (130 cm; DBH), angle of trunk lean (i.e. deviation from vertical), the total number of observable hollow entrances and the number of observable hollow entrances across three size classes (in total, and in each of three entrance diameter classes: 5–10 cm, 10–20 cm and >20 cm). Trunk lean was measured using an inclinometer (Kincrome Australia, Melbourne, Vic, Australia) at breast height. The total number of observable tree hollows and hollow-size estimates were calculated from ground-level scanning conducted by one individual (G. J. Trewella). Each hollow measured was then grouped into our three hollow size classes following standard hollow size classes of northern Australian forests (Woolley et al. 2018; Penton et al. 2020b) to account for the imprecision of the measuring technique. Ground-level scanning for tree hollows has been shown to overestimate the availability of hollows (i.e. for use for denning by fauna) in northern Australian savanna trees, owing to some hollows being blocked by internal termitaria (termite nesting material; Penton et al. 2020a). As such, our tree-hollow counts provide a relative index of tree-hollow availability among sites, rather than an absolute measure. In instances where a northern quoll denned in a log, we recorded the log’s total length, maximum and minimum external diameter and the diameter of each observable hollow entrance by using a standard 30 cm ruler. The same hollow-entrance diameter classes as for the tree hollows were used for log hollows.
Nearest-neighbour survey
We conducted nearest-neighbour surveys to compare known dens to those on their neighbouring trees and/or logs. The area surrounding each den was split into four quadrants, with the nearest tree and log in each quadrant surveyed for the same set of variables as for the den trees/logs. Oakwood (1997) identified northern quolls using trees with a DBH of ≥15 cm, so we used this number as our minimum DBH required for a neighbouring tree to be included in the survey. If separate den sites were close enough to share neighbouring trees/logs, then only one survey was undertaken.
Statistical analyses
Statistical analyses were undertaken in R (ver. 4.2.2; R Core Team 2023). We assessed whether the frequency of use of trees or logs was correlated with the attributes of the tree or log. Separate analyses were conducted for trees and logs. Each observation of our response variable (frequency of use) was a single tree/log. This included the known den trees/logs (n = 35/19 respectively) (i.e. count of nights each tree/log was used by a northern quoll), plus their neighbouring trees/logs (n = 200/196 respectively), with the neighbouring trees/log allocated a value of 0 (i.e. it was not used by the collared northern quolls during the radio-tracking period). We used generalised linear models (GLMs) with poisson error family to evaluate how frequency of use varied in relation to several explanatory variables. In the case of trees, the explanatory variables were DBH, trunk lean, and the number of hollows in each hollow size class; and in the case of logs, the explanatory variables were total log length, minimum external diameter, maximum external diameter, and the number of hollows in each hollow size class. Tree species was initially included as an explanatory variable for the den tree GLM analysis but was found to be an insignificant variable and was removed to improve model fitness. All explanatory variables were centred and standardised (Gelman 2008), and we checked that there was not excessive collinearity (variance inflation factor was ≥0.7) prior to analysis (Zuur et al. 2010). All possible models derived from a global model were evaluated using the R package ‘MuMIn’ (ver. 1.47.5; https://CRAN.R-project.org/package=MuMIn), where each possible model was ranked according to its value of Akaike information criterion corrected for small sample size (AICc) and with model averaging using Akaike weights to estimate each conditional parameter estimate and standard error (Burnham and Anderson 2002). Visualisations of the most influential parameters of each averaged model were produced using the R package ‘ggplot2’ (ver. 3.4.2; https://ggplot2.tidyverse.org; Wickham 2016). To determine how divergent dens were from their neighbouring trees and logs, we undertook a principal-component analysis (PCA) of all trees and all logs by using the R package ‘stats’ (ver. 3.6.2; R Core Team 2023). Each PCA used the same variables as did each respective GLM, with all variables being centred and scaled. Visualisation of each two-dimensional PCA was undertaken using the R package ‘factoextra’ (ver. 1.0.7; http://www.sthda.com/english/wiki/factoextra-r-package-easy-multivariate-data-analyses-and-elegant-visualization).
GPS fixes for each northern quoll were used to estimate a home range. GPS fixes with a dilution of precision value of >10 or with less than four recorded satellite signals were removed to improve the accuracy of each home-range estimate (Langley 1999). Home-range size was estimated using 95% and 50% minimum-convex polygons (MCP) and 95% kernel-density estimates (KDE) for each northern quoll across the 4-week survey period, with the R package ‘rhr’ (ver. 1.2.907; https://jmsigner.github.io/rhrman/; Signer and Balkenhol 2015). These home-range metrics were calculated to gauge a variety of movement patterns and to ensure straightforward comparison with previously published studies.
Results
Home range
Breeding season home ranges for males were twice the size of those for females, with mild intra-sex range overlap and considerable inter-sex range overlap (Fig. 2). The mean 95% MCP estimate and mean 95% KDE estimate for males were 47.2 ± 25.1 ha (mean ± s.d.) and 68.8 ± 44.0 ha respectively (Table 1). The mean 95% MCP estimate and mean 95% KDE estimate for females were 25.3 ± 13.7 ha and 35.4 ± 13.6 ha respectively (Table 1). However, male home ranges were greatly influenced by one male, Male 5, which had a home range three times the size of those for the other males. After excluding Male 5, the mean male home range dropped to only 34.7 ha (95% MCP) and 47.2 ha (95% KDE), which were only one and a half times larger than the mean female home ranges. Core home-range estimates (50% MCP) for both sexes were also greatly reduced compared with their full home range, with both males and females having similar estimates (males = 9.6 ± 5.4 ha, females = 6.2 ± 0.2 ha). None of our home-range estimates reached an asymptote during the survey period (Supplementary material Fig. S1), so our estimates of mean home-range size should be considered conservative.
The estimated home ranges of all seven successful GPS-tracked northern quoll individuals by using 95% minimum-convex polygons (MCP). Single points represent each GPS fixes for each northern quoll. Solid and dotted polygons represent the 95% MCP for each individual northern quoll, with solid polygons representing male northern quolls and dotted polygons representing female northern quolls. The smooth shaded line represents the edge of the plateau, with the darker side denoting the plateau and the lighter side denoting the low-lying savanna.
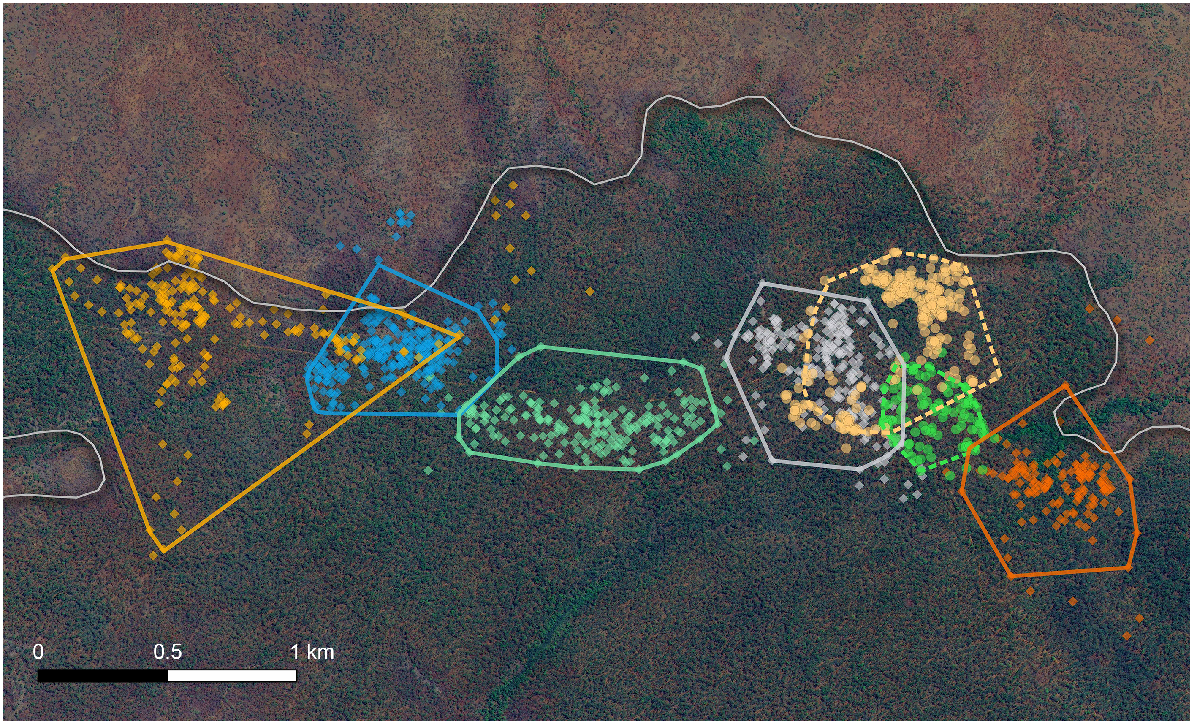
Individual | Duration of tracking (nights) | Number of fixes | 95% MCP (ha) | 50% MCP (ha) | 95% KDE (ha) | |
---|---|---|---|---|---|---|
Male 1 | 16 | 156 | 34.4 | 4.9 | 49.4 | |
Male 2 | 17 | 216 | 36.5 | 9.7 | 57.1 | |
Male 3 | 19 | 333 | 30.1 | 4.5 | 34.1 | |
Male 4 | 16 | 258 | 37.8 | 9.4 | 47.7 | |
Male 5 | 12 | 228 | 97.1 | 19.5 | 155.6 | |
Male 6 | Failed | |||||
Mean ± s.d.: | 47.2 ± 25.1 | 9.6 ± 5.4 | 68.8 ± 44.0 | |||
Female 1 | 11 | 114 | 11.6 | 6.5 | 21.8 | |
Female 2 | 19 | 314 | 39.0 | 6.0 | 49.0 | |
Female 3 | Failed | |||||
Mean ± s.d.: | 25.3 ± 13.7 | 6.2 ± 0.3 | 35.4 ± 13.6 |
s.d. is the standard deviation of the mean. No home-range estimates reached asymptote.
Den tracking
The number of dens recorded for each northern quoll ranged from 5 to 11. The male tracked for the longest duration, Male 3, visited 11 dens over 19 days, whereas the shortest-tracked male, Male 5, visited eight dens over 12 days. Similarly, the longest-tracked female, Female 2, visited seven dens over 19 days, whereas the shortest-tracked female, Female 1, visited five dens over 11 days. In total, we identified 53 unique den sites used by the eight collared northern quolls, including 34 tree hollows and 19 fallen logs.
We found no evidence of den sharing by collared northern quolls, and 40 den sites were used only once during the radio-tracking period. Of the 13 dens used more than once, nine were trees and four were logs. Of the nine trees, seven were E. tetrodonta, one was C. nesophila and one was unidentified. All dens were located on top of the bauxite plateau. Den trees were also consistently found to be larger and have more hollows across all size classes and all tree species than were neighbouring trees (Table 2).
Tree species | Number of tracked | Average DBH (cm) | Average number of small hollows | Average number of medium hollows | Average number of large hollows | Average number of total hollows | |
---|---|---|---|---|---|---|---|
Den trees | |||||||
Eucalyptus tetrodonta | 25 | 47 | 0.9 | 1.4 | 0.5 | 2.8 | |
Dead | 5 | 38 | 0.6 | 1.6 | 0.6 | 2.8 | |
Corymbia nesophila | 2 | 45 | 1.5 | 0 | 0 | 1.5 | |
Erythrophleum chlorostachys | 1 | 53 | 0 | 1 | 1 | 2 | |
Other | 1 | 41 | 1 | 1 | 1 | 3 | |
Total | 34 | 45 | 0.8 | 1 | 0.6 | 2.4 | |
Neighbouring trees | |||||||
E. tetrodonta | 92 | 35 | 0.2 | 0.3 | 0.1 | 0.6 | |
Dead | 15 | 32 | 0.1 | 0.1 | 0.3 | 0.5 | |
C. nesophila | 57 | 43 | 0.3 | 0.2 | 0.02 | 0.5 | |
E. chlorostachys | 10 | 32 | 0 | 0.04 | 0.04 | 0.08 | |
C. stockerii | 24 | 34 | 0.4 | 0.1 | 0 | 0.5 | |
Other | 2 | 20 | 0 | 0 | 0 | 0 | |
Total | 200 | 22 | 0.2 | 0.1 | 0.08 | 0.4 |
Den trees were identified during radio-tracking surveys of the northern quoll undertaken in Weipa, Queensland, between April and May 2019. Neighbouring trees were the closest tree in each of four quadrants around a central den tree. Only neighbouring trees larger than 15 cm DBH were measured as per Oakwood (1997). For analysis, hollow sizes were separated into the following size classes: small = 5–10 cm, medium = 10–20 cm, and large = >20 cm.
Den selection
Generalised linear modelling showed that the frequency of use of trees was correlated with three variables, namely, larger DBH and more small–medium hollows (Fig. 3). The largest trees (DBH > 70 cm) were used nearly 20 times more often than were the smallest trees (DBH < 20 cm). Trees with the highest number of small hollows (four 5–10 cm hollows per tree) were used about six times as often as were trees without any small hollows (regardless of other hollows present) and trees with the most medium hollows (four 10–20 cm hollows per tree) were used about three times as often as were trees without any medium hollows (regardless of other hollows present). These variables were present in all well-supported models (ΔAICc ≤ 2) (Supplementary material Table S1).
The predicted relationship between the frequency of nightly den-tree use by the northern quoll and the most influential predictor variables from the model-averaged generalised linear model: (a) DBH (cm), (c) number of 5–10 cm hollows and (e) number of 10–20 cm hollows, alongside their respective box plots showing the frequency of nightly den-tree use and neighbouring trees against the predictive variables: (b) DBH (cm), (d) number of 5–10 cm hollows and (f) number of 10–20 cm hollows. For the regression plots, the black line represents model fit with the grey bands representing 95% confidence intervals. The box plots show the median (bold line), first and third quartiles (boxes) and range (whiskers) of measurements, with the black circles representing the raw data. Note that the raw data have been ‘jittered’ (a small amount of random variation added) to aid visualisation.
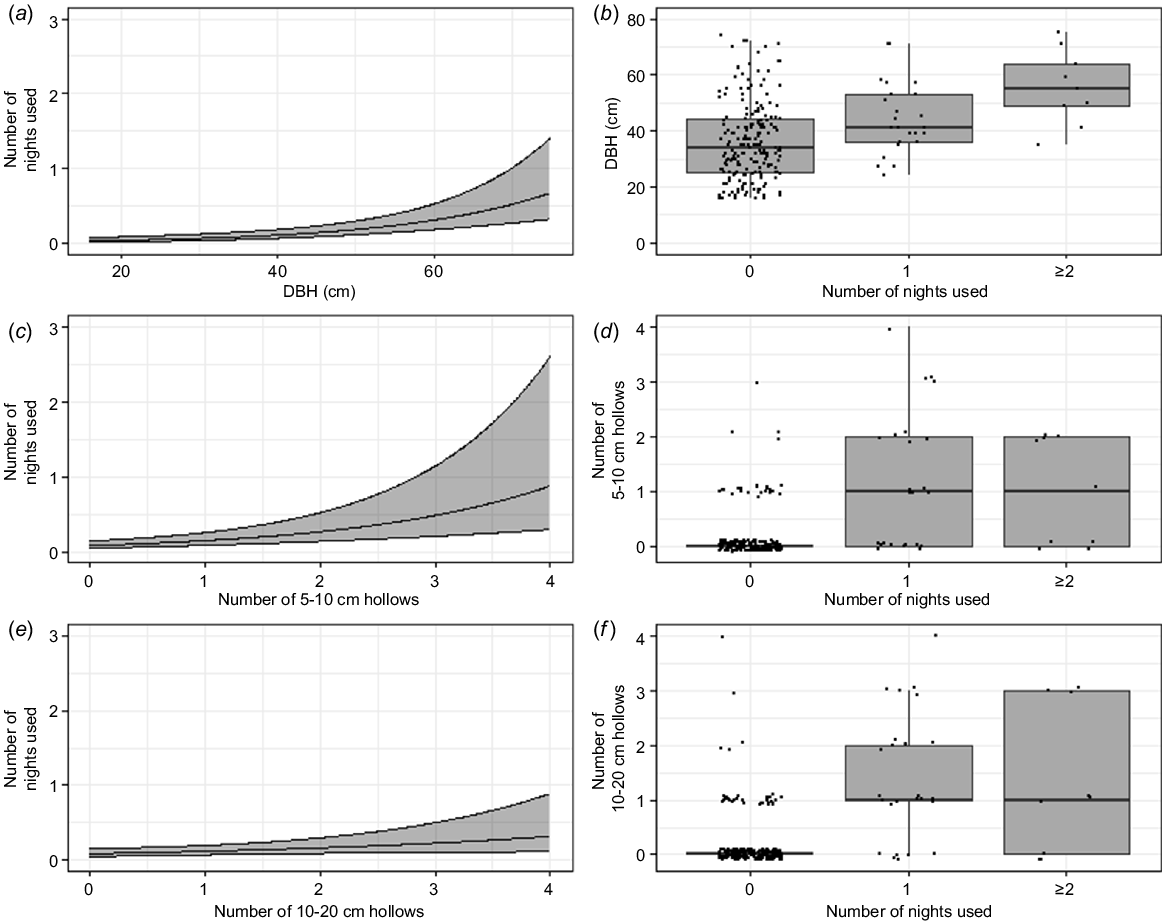
Generalised linear modelling showed that the frequency of use of logs was correlated with two variables, namely, longer length and more medium hollows (Fig. 4). The longest logs (up to 30 m) were used about 12 times more often than were the shortest logs (<4 m) and the logs with the most medium hollows (four 10–20 cm hollows per log) were used about six times as often as were logs without any medium hollows (regardless of other hollows present). These variables were present in all well-supported models (ΔAICc ≤ 2) (Table S1).
The predicted relationship between the frequency of nightly den-log use by the northern quoll and the most influential predictor variables from the model-averaged generalised linear model: (a) length of log (m) and (c) number of 10–20 cm hollows, alongside their respective box plots showing the frequency of nightly den-log use and neighbouring log against the predictive variables: (b) length of log (m) and (d) number of 10–20 cm hollows. For the regression plots, the black line represents model fit, with the grey bands representing 95% confidence intervals. The box plots show the median (bold line), first and third quartiles (boxes) and range (whiskers) of measurements, with the black circles representing the raw data. Note that the raw data have been ‘jittered’ (a small amount of random variation added) to aid visualisation.

Principal-component analysis
The principal-component analysis identified a moderate degree of overlap between den-resource characteristics and the characteristics of the surrounding available trees and logs, but also identified important differences. Den-tree characteristics showed a minor overlap with the neighbouring trees, but ultimately were found to exhibit significant differences from neighbouring trees in relation to all explanatory variables, with the centroid of each group being distinct from the 95% ellipses of the other group (Fig. 5). Den trees were also found to have a greater diversity in characteristics among themselves than were the neighbouring trees which were much more homogenous. Den logs were found to overlap more with neighbouring logs, with centroids of both groups being contained within 95% ellipses of each group, but did show significant differences in relation to medium and large hollows, total log length and largest and smallest diameters of each log (Fig. 5). Both den and neighbouring logs displayed high variability, with den logs showing greater diversity in characteristics among themselves. However, the most divergent neighbouring logs still shared similar characteristics with the most divergent den logs.
Principal-component biplots of (a) den-tree and neighbouring-tree variables and (b) den-log and neighbouring-log variables. Pale circles represent individual den resources, with the pale ellipse containing 95% of all den trees or logs. The large pale circle represents the group centroids of all den-tree and den-log variables. Red circles represent individual neighbouring resources, with the red ellipsis containing 95% of all neighbouring trees or logs. The large red circle represents the group centroids of all neighbouring-tree and neighbouring-log variables.
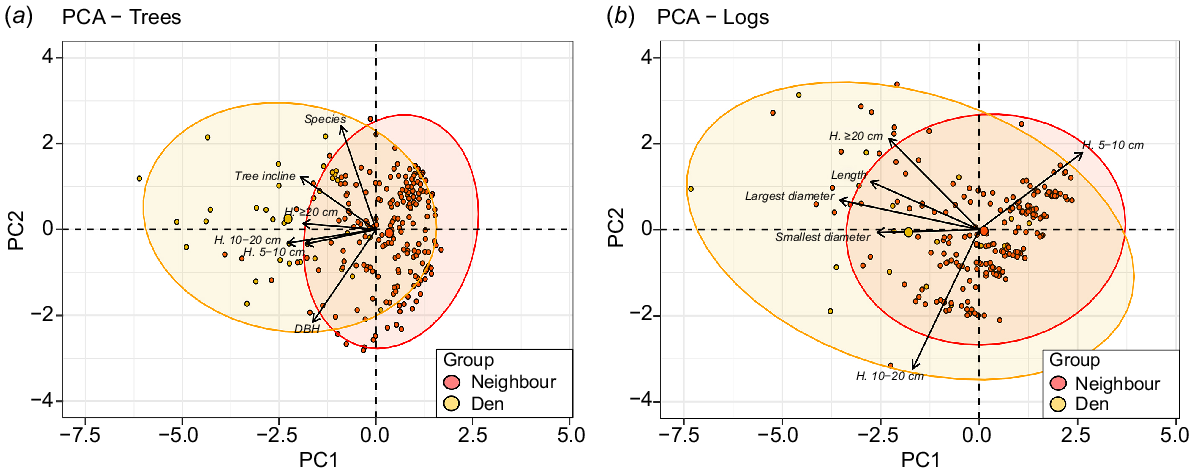
Discussion
Hollow-dependent mammals in the tropical savannas of northern Australia have undergone significant declines in recent decades despite minimal large-scale land-clearing (Woinarski et al. 2011; Woinarski et al. 2014; Davies et al. 2018; Stobo-Wilson et al. 2019). Using the northern quoll as a case study, we sought to identify resources that are critical to the persistence of a declining scansorial mammal. Our results suggest that den availability for our northern quoll population is restricted to the productive E. tetrodonta-dominated savanna on bauxite plateaus. We found that the northern quoll preferentially denned in large trees with numerous small and medium hollow entrances (Fig. 6). It also preferentially denned in large fallen logs with numerous medium hollow entrances. These findings are consistent with those of the current research, suggesting that large hollow-bearing trees, particularly eucalypt species, are a critical resource for a range of declining scansorial mammals, such as the northern quoll, in Australia’s tropical savannas (Geyle et al. 2020; Penton et al. 2020b; Stobo-Wilson et al. 2021).
Published home-range estimates for northern quolls are highly variable across populations and individuals, particularly for breeding males that roam extensively in search of females (Cook 2010; Heiniger et al. 2020a; Hernandez-Santin et al. 2020). Males appear to be greatly influenced by habitat structure, with tropical savanna populations exhibiting smaller home ranges than semi-arid shrubland ecosystems of the Pilbara region of north-western Australia (Moore et al. 2021a). We observed an average male home range of 69 ha (95% KDE), which is comparable to the exceptionally varied male home-range estimates of 2–1751 ha from other savanna populations (Heiniger et al. 2020a; Moore et al. 2021a). On Groote Eylandt, regarded as a safe haven for the northern quoll, Heiniger et al. (2020a) observed highly varied home-range estimates for males across the island’s rugged tropical savannas (up to 1751 ha for one male), but still averaged a breeding male home-range estimate of 128 ha (95% KDE). Our average male home-range estimates were also much smaller than the average male home-range estimates from Pilbara populations of between 301 and 931 ha by using VHF den tracking (King 1989; Cowan et al. 2020a) and 193 ha using GPS tracking (Hernandez-Santin et al. 2020). These inconsistent home-range metrics suggest that the movement patterns of the northern quoll are likely to be a response to the varying spatial arrangements of critical resources across the different environments they inhabit. Males are, therefore, forced to expose themselves to greatly increased energetic costs and predation risk to access females during the breeding season (Fisher et al. 2013).
Female home ranges appear to be much smaller than those of their male counterparts across all environments and appear to be less influenced by habitat type. Our average female home-range estimate of 35 ha (95% KDE) is smaller than those in other tropical savanna populations, i.e. 35 ha (Oakwood 2002) and 53 ha (Heiniger et al. 2020a), but is roughly comparable to Pilbara estimates, which range from 2 ha (Hernandez-Santin et al. 2020) up to 168 ha (King 1989). Males having access to multiple females that they can visit in quick succession is an important aspect of northern quoll breeding (Oakwood 2002). As such, female home-range sizes are likely to play a significant role in male survivorship during the breeding season. Our two tracked females had only a mild overlap in their home ranges, suggesting that females with large home ranges, such as those seen in the Pilbara, may be exacerbating the energetic demands of males. These findings highlight the importance of having abundant critical resources, such as dens, that can maintain healthy connectivity between males and females to allow the population to persist within their given environments.
We found evidence that the spatial arrangement of den availability influences northern quoll movement. Over the 30-day duration of our study, we recorded very few GPS fixes beyond the plateau boundary, with only a few observations of males moving within the ecotonal zone between the plateau and the adjacent low-lying savanna (Fig. 2). This suggests that the less productive, low-lying savanna may lack sufficient denning resources for northern quolls to naturally roam through and inhabit, without being exposed to significant predation risk. All northern quolls tracked during this study were captured and remained in the plateau, consistent with camera-trapping observations in the region (Trewella et al. 2023). Both studies in our area suggest that this northern quoll population is restricted to the structurally complex vegetation of the bauxite plateau. However, we cannot rule out the use of open habitats in the study area because of the limited duration of our study. The restriction to structurally complex habitats may hinder their ability to recover to pre-cane toad levels and may threaten the population persistence by making them more vulnerable to stochastic events, as has been observed in arid-zone populations of northern quolls (Hernandez-Santin et al. 2019).
Over the duration of our study, northern quolls were observed using only hollow trees or fallen logs as den sites. The northern quoll is adapted to a predominantly terrestrial lifestyle but has some climbing adaptations (Henderson et al. 2017; Clemente et al. 2019), enabling it to access a variety of den resources. We identified a preference for larger trees, which are more likely to have developed hollows larger than 10 cm (Woolley et al. 2018), but we also identified preferences for small (5–10 cm) and medium (10–20 cm) hollow entrances rather than large (>20 cm) hollow entrances. Penton et al. (2021b) identified medium–large hollow entrances (>10 cm) as the most frequently visited entrance size by a range of arboreal mammals in similar high-rainfall savanna on Melville Island, Australia. This potential competition for larger hollows with similarly sized mammals in the region, such as the black-footed tree-rat (Mesembriomys gouldii) and the common brushtail possum (Trichosurus vulpecula), may discourage the use of these hollows by the northern quoll. This is particularly true when hollows larger than 10 cm are uncommon in frequently burnt savannas (≥0.5 fires per year; Woolley et al. 2018), and the northern quoll can use alternative options such as fallen logs. Because our study was conducted in the cooler early dry season, hollows larger than 20 cm, which provide cooler microclimates (Isaac et al. 2008), may also not have been as preferentially selected compared with the hotter, more humid late dry-season months. However, physical properties such as hollow depth and tree size, which both cool internal temperatures and stabilise daytime temperature fluctuations (Isaac et al. 2008), are likely to become an increasingly important survival factor as the impacts of climate change become more apparent in tropical savannas. Research by Cowan et al. (2020b) identified cooler and more stable microclimate temperatures as important den-selection factors for rock crevices by northern quolls in semi-arid habitats (Cowan et al. 2020b). As such, microclimatic conditions in tree hollows and fallen logs should be a focus for future research in northern quoll arboreal den selection. Considering the importance of arboreal den use by the northern quoll in savannas and the high energetic costs associated with the mating season and rearing young, future research should also consider the energetic demands of arboreal den use and potential trade-offs in survivorship of individuals, particularly in comparison to populations residing in rocky escarpments where northern quoll movement is more energetically efficient (Clemente et al. 2019).
Trade-offs in survivorship may also play an important role in the use of alternative dens in savannas. The results of our principal-component analysis showed that den trees are a distinct subset of all trees in the study area. As such, alternative ground-based den resources, such as fallen logs, may be crucial for the northern quoll in savannas. We found that many available fallen logs in our study area were similar in structure to the den logs used by northern quolls and may therefore not be a limiting factor for the northern quoll compared with hollow-bearing trees. Grasping strength by the northern quoll has been found to be correlated with body size, with males displaying greater grasp strength than do females (Charters et al. 2018). As a result, ground-based den resources may be of greater importance for the smaller-sized female northern quolls (Oakwood 1997), but may also leave them at a greater risk of predation by terrestrial predators (Oakwood 2000; Hernandez-Santin et al. 2016; Cremona et al. 2017). Thomas et al. (2021) found that on Groote Eylandt, where feral cat populations are in very low densities (0.07 cats/km2; Heiniger et al. 2020b), northern quolls from savanna populations were healthier than those from rocky escarpment populations. However, in Kakadu National Park, where cat densities are higher (0.19–0.68 cats/km2; Watson and Woinarski 2004), Oakwood (2000) found that rocky escarpment populations have higher survival rates than do their savanna conspecifics. The bauxite plateaus of the Weipa region have very high cat densities (0.8 cats/km2; G. J. Trewella, unpubl. data) and, as such, this may be a significant factor driving the absence of northern quolls from the simplified, low-lying savannas surrounding the plateaus, similar to the findings of Oakwood (2000). This may also explain the preferential use of rock crevices by female northern quolls in mainland mixed savanna habitat, because rugged terrain tends to reduce the occurrence of terrestrial predators, such as feral cats (Hohnen et al. 2016) and fire (Fisher et al. 2022), creating a safer environment to rear young, thus promoting population persistence (Cremona et al. 2017; Moro et al. 2019).
The importance of trees and logs as den sites for the northern quoll highlights the potential importance of fire management for preserving critical denning resources across northern Australia’s savannas (Andersen et al. 2012; Radford et al. 2020). Frequent, high-intensity fires kill and incinerate hollow-bearing trees and ground-level den resources such as fallen logs (Williams et al. 1999; Woolley et al. 2018; Radford et al. 2021). Low fire frequency (and most likely intensity) on top of our study plateau is likely to benefit the northern quoll population by maintaining a diversity of den resources in the landscape, whereas higher fire frequencies in the low-lying savannas may play a role in reducing the suitability of this habitat by limiting den availability and understorey vegetation density. Feral cats are known to be attracted to recently burnt areas, where they enjoy increased hunting efficiency in a simplified, open habitat (McGregor et al. 2016a, 2016b). Hence, the predation pressure exerted by cats on mammal populations is thought to be greatly amplified in the post-fire environment (Frank et al. 2014; Leahy et al. 2015; Tuft et al. 2021). Reducing the frequency and intensity of fires in savannas is likely to benefit the northern quoll, by allowing the persistence of ground-based den resources, such as fallen logs, and maintaining ground cover to avoid predation, while also reducing mortality of hollow-bearing trees. However, because of the varied threats that are believed to be driving northern quoll decline, such as cane toad-related mortality, reducing fire frequency alone may not be sufficient to facilitate the persistence of northern quoll populations in regions of low-rainfall, less productive savannas that lack large, hollow-bearing trees. Overall, the preservation of infrequently burnt savanna habitat is likely to have benefits for a wide variety of small mammal species (Andersen et al. 2012; von Takach et al. 2022), but the management of fire in northern Australian savannas should be viewed as a component of a holistic management approach to avoid unintended outcomes (Murphy and Russell-Smith 2010; Legge et al. 2019).
Reductions in the availability of den resources in northern Australia’s tropical savannas may be an important factor driving the declines of the northern quoll and other hollow-dependent small mammals (Ziembicki et al. 2015; Stobo-Wilson et al. 2020a). Hollow-dependent mammals are contracting away from lower-rainfall, less-productive savannas and to regions of higher-rainfall, productive savannas that retain abundant large hollow-bearing trees (McKenzie et al. 2007; Stobo-Wilson et al. 2019; von Takach et al. 2020). A similar pattern has emerged for the northern quoll across northern Australia (Start et al. 2012; Radford et al. 2014; Moore et al. 2019). Our results suggest that high-rainfall, high-biomass savannas, such as those occurring on the bauxite plateau near Weipa, can support remnant northern quoll populations, even if the cane toad is present. The ability of such areas to support remnant northern quoll populations is likely to be due to the availability of large eucalypt trees that have a diversity of hollows, and an abundance of fallen logs. Management strategies to conserve critical den resources include preventing logging and land-clearing in high-biomass savannas (Ward et al. 2019), and limiting the frequency and intensity of savanna fires, so as to support the accumulation of ground-based den resources and the natural development of large, hollow-bearing trees (Andersen et al. 2012; Radford et al. 2015; von Takach et al. 2022).
Data availability
The data that support this study will be shared upon reasonable request to the corresponding author.
Declaration of funding
This study was funded by Rio Tinto Weipa (RTA Weipa Pty Ltd) and an Australian Government Research Training Program (RTP) Scholarship.
Acknowledgements
We acknowledge the Traditional Owners of the land upon which these data were collected. We thank the staff of the RTA Weipa Land and Rehabilitation team for their logistical and safety support. We thank Ecotone Flora and Fauna Consultants for their on-site assistance and data sharing. We also thank the many hard-working volunteers that allowed this entire project to happen: Claire Bowry, Lucinda Auslebrook, Justin Wright, Kevin Sibbison, Shoshana Rapley, Grant Trewella, Dimpu Dhar, Alex Williams, Anna Signorini, Kristin Semmens, Amoi Stubbs-Oliver and Matthew Brun. We also thank our anonymous reviewers for their thoughtful comments and improvements made to the paper.
References
Andersen AN, Woinarski JCZ, Parr CL (2012) Savanna burning for biodiversity: fire management for faunal conservation in Australian tropical savannas. Austral Ecology 37, 658-667.
| Crossref | Google Scholar |
Australian Bureau of Meteorology (2024) Average annual, seasonal and monthly rainfall. Available at http://www.bom.gov.au/climate/data/index.shtml [Accessed 20 January 2024]
Barton RA, Whiten A, Strum SC, Byrne RW, Simpson AJ (1992) Habitat use and resource availability in baboons. Animal Behaviour 43, 831-844.
| Crossref | Google Scholar |
Berry LE, L’ Hotellier FA, Carter A, Kemp L, Kavanagh RP, Roshier DA (2019) Patterns of habitat use by three threatened mammals 10 years after reintroduction into a fenced reserve free of introduced predators. Biological Conservation 230, 1-9.
| Crossref | Google Scholar |
Bradshaw CJA (2012) Little left to lose: deforestation and forest degradation in Australia since European colonization. Journal of Plant Ecology 5, 109-120.
| Crossref | Google Scholar |
Braithwaite RW, Griffiths AD (1994) Demographic variation and range contraction in the northern quoll, Dasyurus hallucatus (MArsupialia: Dasyuridae). Wildlife Research 21, 203-217.
| Crossref | Google Scholar |
Cameron GN, Spencer SR (1985) Assessment of space-use patterns in the hispid cotton rat (Sigmodon hispidus). Oecologia 68, 133-139.
| Crossref | Google Scholar | PubMed |
Charters JE, Heiniger J, Clemente CJ, Cameron SF, Amir Abdul Nasir AF, Niehaus AC, Wilson RS (2018) Multidimensional analyses of physical performance reveal a size-dependent trade-off between suites of traits. Functional Ecology 32, 1541-1553.
| Crossref | Google Scholar |
Clemente CJ, Dick TJM, Wheatley R, Gaschk J, Nasir AFAA, Cameron SF, Wilson RS (2019) Moving in complex environments: a biomechanical analysis of locomotion on inclined and narrow substrates. Journal of Experimental Biology 222, jeb189654.
| Crossref | Google Scholar |
Connor SE, Schneider L, Trezise J, Rule S, Barrett RL, Zawadzki A, Haberle SG (2018) Forgotten impacts of European land-use on riparian and savanna vegetation in northwest Australia. Journal of Vegetation Science 29, 427-437.
| Crossref | Google Scholar |
Cowan M, Moro D, Anderson H, Angus J, Garretson S, Morris K (2020a) Aerial baiting for feral cats is unlikely to affect survivorship of northern quolls in the Pilbara region of Western Australia. Wildlife Research 47, 589-598.
| Crossref | Google Scholar |
Cowan MA, Dunlop JA, Turner JM, Moore HA, Nimmo DG (2020b) Artificial refuges to combat habitat loss for an endangered marsupial predator: how do they measure up? Conservation Science and Practice 2(6), e204.
| Crossref | Google Scholar |
Cremona T, Crowther MS, Webb JK (2017) High mortality and small population size prevent population recovery of a reintroduced mesopredator. Animal Conservation 20, 555-563.
| Crossref | Google Scholar |
Davies HF, McCarthy MA, Firth RSC, Woinarski JCZ, Gillespie GR, Andersen AN, Rioli W, Puruntatameri J, Roberts W, Kerinaiua C, Kerinauia V, Womatakimi KB, Murphy BP (2018) Declining populations in one of the last refuges for threatened mammal species in northern Australia. Austral Ecology 43, 602-612.
| Crossref | Google Scholar |
Doherty TS, Dickman CR, Johnson CN, Legge SM, Ritchie EG, Woinarski JCZ (2017) Impacts and management of feral cats Felis catus in Australia. Mammal Review 47, 83-97.
| Crossref | Google Scholar |
Doody JS, McHenry C, Rhind D, Gray C, Clulow S (2021) Impacts of invasive cane toads on an endangered marsupial predator and its prey. Endangered Species Research 46, 269-277.
| Crossref | Google Scholar |
Fisher DO, Dickman CR, Jones ME, Blomberg SP (2013) Sperm competition drives the evolution of suicidal reproduction in mammals. Proceedings of the National Academy of Sciences 110, 17910-17914.
| Crossref | Google Scholar |
Fisher R, Lewis B, Price O, Pickford A (2022) Barriers to fire spread in northern Australian tropical savannas, deriving fire edge metrics from long term high-frequency fire histories. Journal of Environmental Management 301, 113864.
| Crossref | Google Scholar | PubMed |
Frank ASK, Johnson CN, Potts JM, Fisher A, Lawes MJ, Woinarski JCZ, Tuft K, Radford IJ, Gordon IJ, Collis M-A, Legge S, Frid C (2014) Experimental evidence that feral cats cause local extirpation of small mammals in Australia’s tropical savannas. Journal of Applied Ecology 51, 1486-1493.
| Crossref | Google Scholar |
Franklin JF, Van Pelt R (2004) Spatial aspects of structural complexity in old-growth forests. Journal of Forestry 102, 22-28.
| Crossref | Google Scholar |
Freeland WJ (1990) Large herbivorous mammals: Exotic species in northern Australia. Journal of Biogeography 17, 445-449.
| Crossref | Google Scholar |
Gaschk JL, Del Simone K, Wilson RS, Clemente CJ (2023) Resting disparity in quoll semelparity: examining the sex-linked behaviours of wild roaming northern quolls (Dasyurus hallucatus) during breeding season. Royal Society Open Science 10, 221180.
| Crossref | Google Scholar | PubMed |
Gelman A (2008) Scaling regression inputs by dividing by two standard deviations. Statistics in Medicine 27, 2865-2873.
| Crossref | Google Scholar | PubMed |
Geyle HM, Woolley L-A, Davies HF, Woinarski JCZ, Murphy BP (2020) Targeted sampling successfully detects the cryptic and declining arboreal marsupial (Phascogale pirata) in northern Australia. Pacific Conservation Biology 26, 395-403.
| Crossref | Google Scholar |
Goldingay RL (2011) Characteristics of tree hollows used by Australian arboreal and scansorial mammals. Australian Journal of Zoology 59, 277-294.
| Crossref | Google Scholar |
Gorini L, Linnell JDC, May R, Panzacchi M, Boitani L, Odden M, Nilsen EB (2012) Habitat heterogeneity and mammalian predator–prey interactions. Mammal Review 42, 55-77.
| Crossref | Google Scholar |
Heiniger J, Cameron SF, Madsen T, Niehaus AC, Wilson RS (2020a) Demography and spatial requirements of the endangered northern quoll on Groote Eylandt. Wildlife Research 47, 224-238.
| Crossref | Google Scholar |
Heiniger J, Davies HF, Gillespie GR (2020b) Status of mammals on Groote Eylandt: safe haven or slow burn? Austral Ecology 45, 759-772.
| Crossref | Google Scholar |
Henderson K, Pantinople J, McCabe K, Richards HL, Milne N (2017) Forelimb bone curvature in terrestrial and arboreal mammals. PeerJ 5, e3229.
| Crossref | Google Scholar | PubMed |
Hernandez-Santin L, Goldizen AW, Fisher DO (2016) Introduced predators and habitat structure influence range contraction of an endangered native predator, the northern quoll. Biological Conservation 203, 160-167.
| Crossref | Google Scholar |
Hernandez-Santin L, Dunlop JA, Goldizen AW, Fisher DO (2019) Demography of the northern quoll (Dasyurus hallucatus) in the most arid part of its range. Journal of Mammalogy 100, 1191-1198.
| Crossref | Google Scholar |
Hernandez-Santin L, Henderson M, Molloy SW, Dunlop JA, Davis RA (2020) Spatial ecology of an endangered carnivore, the Pilbara northern quoll. Australian Mammalogy 43, 235-242.
| Crossref | Google Scholar |
Hernandez-Santin L, Goldizen AW, Fisher DO (2022) Northern quolls in the Pilbara persist in high-quality habitat, despite a decline trajectory consistent with range eclipse by feral cats. Conservation Science and Practice 4, e12733.
| Crossref | Google Scholar |
Hohnen R, Tuft K, McGregor HW, Legge S, Radford IJ, Johnson CN (2016) Occupancy of the invasive feral cat varies with habitat complexity. PLoS ONE 11, e0152520.
| Crossref | Google Scholar | PubMed |
Horne JS, Garton EO, Rachlow JL (2008) A synoptic model of animal space use: simultaneous estimation of home range, habitat selection, and inter/intra-specific relationships. Ecological Modelling 214, 338-348.
| Crossref | Google Scholar |
Incoll RD, Loyn RH, Ward SJ, Cunningham RB, Donnelly CF (2001) The occurrence of gliding possums in old-growth forest patches of mountain ash (Eucalyptus regnans) in the central highlands of victoria. Biological Conservation 98, 77-88.
| Crossref | Google Scholar |
Isaac JL, De Gabriel JL, Goodman BA (2008) Microclimate of daytime den sites in a tropical possum: implications for the conservation of tropical arboreal marsupials. Animal Conservation 11, 281-287.
| Crossref | Google Scholar |
King DR (1989) An assessment of the hazard posed to northern quolls (Dasyurus hallucatus) by aerial baiting with 1080 to control dingoes. Wildlife Research 16, 569-574.
| Crossref | Google Scholar |
Kutt AS, Woinarski JCZ (2007) The effects of grazing and fire on vegetation and the vertebrate assemblage in a tropical savanna woodland in north-eastern Australia. Journal of Tropical Ecology 23, 95-106.
| Crossref | Google Scholar |
Kutt AS, Vanderduys EP, Ferguson D, Mathieson M (2012) Effect of small-scale woodland clearing and thinning on vertebrate fauna in a largely intact tropical savanna mosaic. Wildlife Research 39, 366-373.
| Crossref | Google Scholar |
Kyle G, Duncan DH (2012) Arresting the rate of land clearing: change in woody native vegetation cover in a changing agricultural landscape. Landscape and Urban Planning 106, 165-173.
| Crossref | Google Scholar |
Langley RB (1999) Dilution of precision. GPS World 10, 52-59.
| Google Scholar |
Leahy L, Legge SM, Tuft K, McGregor HW, Barmuta LA, Jones ME, Johnson CN (2015) Amplified predation after fire suppresses rodent populations in Australia’s tropical savannas. Wildlife Research 42, 705-716.
| Crossref | Google Scholar |
Legge S, Smith JG, James A, Tuft KD, Webb T, Woinarski JCZ (2019) Interactions among threats affect conservation management outcomes: livestock grazing removes the benefits of fire management for small mammals in Australian tropical savannas. Conservation Science and Practice 1, e52.
| Crossref | Google Scholar |
Liedloff AC, Cook GD (2007) Modelling the effects of rainfall variability and fire on tree populations in an Australian tropical savanna with the flames simulation model. Ecological Modelling 201, 269-282.
| Crossref | Google Scholar |
Lindenmayer DB, Incoll RD, Cunningham RB, Donnelly CF (1999) Attributes of logs on the floor of Australian mountain ash (Eucalyptus regnans) forests of different ages. Forest Ecology and Management 123, 195-203.
| Crossref | Google Scholar |
Lindenmayer DB, Cunningham RB, Donnelly CF, Franklin JF (2000) Structural features of old-growth Australian montane ash forests. Forest Ecology and Management 134, 189-204.
| Crossref | Google Scholar |
Lindenmayer DB, Blanchard W, Blair D, McBurney L, Banks SC (2017) Relationships between tree size and occupancy by cavity-dependent arboreal marsupials. Forest Ecology and Management 391, 221-229.
| Crossref | Google Scholar |
Macdonald DW, Rushton S (2003) Modelling space use and dispersal of mammals in real landscapes: a tool for conservation. Journal of Biogeography 30, 607-620.
| Crossref | Google Scholar |
McElhinny C, Gibbons P, Brack C, Bauhus J (2006) Fauna-habitat relationships: a basis for identifying key stand structural attributes in temperate Australian eucalypt forests and woodlands. Pacific Conservation Biology 12, 89-110.
| Crossref | Google Scholar |
McGregor HW, Legge S, Jones ME, Johnson CN (2014) Landscape management of fire and grazing regimes alters the fine-scale habitat utilisation by feral cats. PLoS ONE 9, e109097.
| Crossref | Google Scholar | PubMed |
McGregor H, Legge S, Jones ME, Johnson CN (2015) Feral cats are better killers in open habitats, revealed by animal-borne video. PLoS ONE 10, e0133915.
| Crossref | Google Scholar | PubMed |
McGregor HW, Cliff HB, Kanowski J (2016a) Habitat preference for fire scars by feral cats in Cape York Peninsula, Australia. Wildlife Research 43, 623-633.
| Crossref | Google Scholar |
McGregor HW, Legge S, Jones ME, Johnson CN (2016b) Extraterritorial hunting expeditions to intense fire scars by feral cats. Scientific Reports 6, 22559.
| Crossref | Google Scholar | PubMed |
McKenzie NL, Burbidge AA, Baynes A, Brereton RN, Dickman CR, Gordon G, Gibson LA, Menkhorst PW, Robinson AC, Williams MR, Woinarski JCZ (2007) Analysis of factors implicated in the recent decline of Australia’s mammal fauna. Journal of Biogeography 34, 597-611.
| Crossref | Google Scholar |
Miritis V, Rendall AR, Doherty TS, Coetsee AL, Ritchie EG (2020) Living with the enemy: a threatened prey species coexisting with feral cats on a fox-free island. Wildlife Research 47, 633-642.
| Crossref | Google Scholar |
Moore HA, Dunlop JA, Valentine LE, Woinarski JCZ, Ritchie EG, Watson DM, Nimmo DG (2019) Topographic ruggedness and rainfall mediate geographic range contraction of a threatened marsupial predator. Diversity and Distributions 25, 1818-1831.
| Crossref | Google Scholar |
Moore HA, Dunlop JA, Jolly CJ, Kelly E, Woinarski JCZ, Ritchie EG, Burnett S, van Leeuwen S, Valentine LE, Cowan MA, Nimmo DG (2021a) A brief history of the northern quoll (Dasyurus hallucatus): a systematic review. Australian Mammalogy 44, 185-207.
| Crossref | Google Scholar |
Moore HA, Michael DR, Ritchie EG, Dunlop JA, Valentine LE, Hobbs RJ, Nimmo DG (2021b) A rocky heart in a spinifex sea: occurrence of an endangered marsupial predator is multiscale dependent in naturally fragmented landscapes. Landscape Ecology 36, 1359-1376.
| Crossref | Google Scholar |
Moro D, Dunlop J, Williams MR (2019) Northern quoll persistence is most sensitive to survivorship of juveniles. Wildlife Research 46, 165-175.
| Crossref | Google Scholar |
Murphy BP, Russell-Smith J (2010) Fire severity in a northern Australian savanna landscape: the importance of time since previous fire. International Journal of Wildland Fire 19, 46-51.
| Crossref | Google Scholar |
North Australian Fire Information (2023) North Australian 250m fire frequency mapping 2000 to 2022. Available at https://firenorth.org.au/nafi3/ [Accessed 20 January 2024]
Oakwood M (2000) Reproduction and demography of the northern quoll, Dasyurus hallucatus, in the lowland savanna of northern Australia. Australian Journal of Zoology 48, 519-539.
| Crossref | Google Scholar |
Oakwood M (2002) Spatial and social organization of a carnivorous marsupial Dasyurus hallucatus Marsupialia: Dasyuridae). Journal of Zoology 257, 237-248.
| Crossref | Google Scholar |
Oakwood M, Bradley AJ, Cockburn A (2001) Semelparity in a large marsupial. Proceedings: Biological Science 268, 407-411.
| Crossref | Google Scholar |
Oakwood M, Woinarski JCZ, Burnett S (2016) Dasyurus hallucatus. The IUCN Red List of Threatened Species 2016 [Online]. Available at https://dx.doi.org/10.2305/IUCN.UK.2016-2.RLTS.T6295A21947321.en
Pavey CR, Addison J, Brandle R, Dickman CR, McDonald PJ, Moseby KE, Young LI (2017) The role of refuges in the persistence of Australian dryland mammals. Biological Reviews 92, 647-664.
| Crossref | Google Scholar | PubMed |
Penton CE, Woolley L-A, Radford IJ, Murphy BP (2020a) Blocked-off: Termitaria cause the overestimation of tree hollow availability by ground-based surveys in northern Australia. Forest Ecology and Management 458, 117707.
| Crossref | Google Scholar |
Penton CE, Woolley L-A, Radford IJ, Murphy BP (2020b) Overlapping den tree selection by three declining arboreal mammal species in an Australian tropical savanna. Journal of Mammalogy 101, 1165-1176.
| Crossref | Google Scholar | PubMed |
Penton CE, Davies HF, Radford IJ, Woolley L-A, Rangers TL, Murphy BP (2021a) A hollow argument: understory vegetation and disturbance determine abundance of hollow-dependent mammals in an Australian tropical savanna. Frontiers in Ecology and Evolution 9, 739550.
| Crossref | Google Scholar |
Penton CE, Radford IJ, Woolley L-A, von Takach B, Murphy BP (2021b) Unexpected overlapping use of tree hollows by birds, reptiles and declining mammals in an Australian tropical savanna. Biodiversity and Conservation 30, 2977-3001.
| Crossref | Google Scholar |
Powell RA, Mitchell MS (2012) What is a home range? Journal of Mammalogy 93, 948-958.
| Crossref | Google Scholar |
R Core Team (2023) R: a language and environment for statistical computing. (R Foundation for Statistical Computing: Vienna, Austria) Available at https://www.R-project.org/ [Accessed 20 January 2024]
Radford IJ, Dickman CR, Start AN, Palmer C, Carnes K, Everitt C, Fairman R, Graham G, Partridge T, Thomson A (2014) Mammals of Australia’s tropical savannas: a conceptual model of assemblage structure and regulatory factors in the kimberley region. PLoS ONE 9, e92341.
| Crossref | Google Scholar | PubMed |
Radford IJ, Gibson LA, Corey B, Carnes K, Fairman R (2015) Influence of fire mosaics, habitat characteristics and cattle disturbance on mammals in fire-prone savanna landscapes of the northern Kimberley. PLoS ONE 10, e0130721.
| Crossref | Google Scholar | PubMed |
Radford IJ, Woolley L-A, Corey B, Vigilante T, Wunambal Gaambera Aboriginal Corporation, Hatherley E, Fairman R, Carnes K, Start AN (2020) Prescribed burning benefits threatened mammals in northern Australia. Biodiversity and Conservation 29, 2985-3007.
| Crossref | Google Scholar |
Radford IJ, Corey B, Carnes K, Shedley E, McCaw L, Woolley L-A (2021) Landscape-scale effects of fire, cats, and feral livestock on threatened savanna mammals: unburnt habitat matters more than pyrodiversity. Frontiers in Ecology and Evolution 9, 739817.
| Crossref | Google Scholar |
Remm J, Lõhmus A (2011) Tree cavities in forests – the broad distribution pattern of a keystone structure for biodiversity. Forest Ecology and Management 262, 579-585.
| Crossref | Google Scholar |
Ritchie EG, Johnson CN (2009) Predator interactions, mesopredator release and biodiversity conservation. Ecology Letters 12, 982-998.
| Crossref | Google Scholar | PubMed |
Russell-Smith J (2001) Pre-contact aboriginal, and contemporary fire regimes of the savanna landscapes of northern Australia: patterns, changes and ecological responses. Ngoonjook 20, 6-32.
| Google Scholar |
Russell-Smith J, Edwards AC, Price OF (2012) Simplifying the savanna: the trajectory of fire-sensitive vegetation mosaics in northern Australia. Journal of Biogeography 39, 1303-1317.
| Crossref | Google Scholar |
Sharp BR, Whittaker RJ (2003) The irreversible cattle-driven transformation of a seasonally flooded Australian savanna. Journal of Biogeography 30, 783-802.
| Crossref | Google Scholar |
Signer J, Balkenhol N (2015) Reproducible home ranges (rhr): a new, user-friendly r package for analyses of wildlife telemetry data. Wildlife Society Bulletin 39, 358-363.
| Crossref | Google Scholar |
Sih A (1984) The behavioral response race between predator and prey. The American Naturalist 123, 143-150.
| Crossref | Google Scholar |
Sikes RS, Animal Care and Use Committee of the American Society of Mammalogists (2016) Guidelines of the American Society of Mammalogists for the use of wild mammals in research and education. Journal of Mammalogy 97, 663-688.
| Crossref | Google Scholar | PubMed |
Simberloff D, Cox J (1987) Consequences and costs of conservation corridors. Conservation Biology 1, 63-71.
| Crossref | Google Scholar |
South A (1999) Extrapolating from individual movement behaviour to population spacing patterns in a ranging mammal. Ecological Modelling 117, 343-360.
| Crossref | Google Scholar |
Start AN, Burbidge AA, McDowell MC, McKenzie NL (2012) The status of non-volant mammals along a rainfall gradient in the south-west Kimberley, Western Australia. Australian Mammalogy 34, 36-48.
| Crossref | Google Scholar |
Stobo-Wilson AM, Murphy BP, Cremona T, Carthew SM (2019) Contrasting patterns of decline in two arboreal marsupials from northern Australia. Biodiversity and Conservation 28, 2951-2965.
| Crossref | Google Scholar |
Stobo-Wilson AM, Stokeld D, Einoder LD, Davies HF, Fisher A, Hill BM, Mahney T, Murphy BP, Scroggie MP, Stevens A, Woinarski JCZ, Bawinanga Rangers, Warddeken Rangers, Gillespie GR (2020a) Bottom-up and top-down processes influence contemporary patterns of mammal species richness in Australia’s monsoonal tropics. Biological Conservation 247, 108638.
| Crossref | Google Scholar |
Stobo-Wilson AM, Stokeld D, Einoder LD, Davies HF, Fisher A, Hill BM, Mahney T, Murphy BP, Stevens A, Woinarski JCZ, Bawinanga Rangers, Warddeken Rangers, Gillespie GR (2020b) Habitat structural complexity explains patterns of feral cat and dingo occurrence in monsoonal Australia. Diversity and Distributions 26, 832-842.
| Crossref | Google Scholar |
Stobo-Wilson AM, Murphy BP, Cremona T, Carthew SM, Levick SR, Pettorelli N, Carter A (2021) Illuminating den-tree selection by an arboreal mammal using terrestrial laser scanning in northern Australia. Remote Sensing in Ecology and Conservation 7, 154-168.
| Crossref | Google Scholar |
Thomas H, Cameron SF, Campbell HA, Micheli-Campbell MA, Kirke EC, Wheatley R, Wilson RS (2021) Rocky escarpment versus savanna woodlands: comparing diet and body condition as indicators of habitat quality for the endangered northern quoll (Dasyurus hallucatus). Wildlife Research 48, 434-443.
| Crossref | Google Scholar |
Thornton DH, Branch LC, Sunquist ME (2011) The relative influence of habitat loss and fragmentation: do tropical mammals meet the temperate paradigm? Ecological Applications 21, 2324-2333.
| Crossref | Google Scholar | PubMed |
Trewella GJ, Cremona T, Nevard H, Murphy BP (2023) Habitat structure facilitates coexistence of native and invasive mesopredators in an Australian tropical savanna. Wildlife Research 50, 1058-1070.
| Crossref | Google Scholar |
Tuft K, Legge S, Frank ASK, James AI, May T, Page E, Radford IJ, Woinarski JCZ, Fisher A, Lawes MJ, Gordon IJ, Johnson CN (2021) Cats are a key threatening factor to the survival of local populations of native small mammals in Australia’s tropical savannas: evidence from translocation trials with Rattus tunneyi. Wildlife Research 48, 654-662.
| Crossref | Google Scholar |
von Takach B, Scheele BC, Moore H, Murphy BP, Banks SC (2020) Patterns of niche contraction identify vital refuge areas for declining mammals. Diversity and Distributions 26, 1467-1482.
| Crossref | Google Scholar |
von Takach B, Jolly CJ, Dixon KM, Penton CE, Doherty TS, Banks SC (2022) Long-unburnt habitat is critical for the conservation of threatened vertebrates across australia. Landscape Ecology 37, 1469-1482.
| Crossref | Google Scholar |
Ward MS, Simmonds JS, Reside AE, Watson JEM, Rhodes JR, Possingham HP, Trezise J, Fletcher R, File L, Taylor M (2019) Lots of loss with little scrutiny: the attrition of habitat critical for threatened species in Australia. Conservation Science and Practice 1, e117.
| Crossref | Google Scholar |
Warfe DM, Barmuta LA (2004) Habitat structural complexity mediates the foraging success of multiple predator species. Oecologia 141, 171-178.
| Crossref | Google Scholar | PubMed |
Williams RJ, Cook GD, Gill AM, Moore PHR (1999) Fire regime, fire intensity and tree survival in a tropical savanna in northern Australia. Australian Journal of Ecology 24, 50-59.
| Crossref | Google Scholar |
Woinarski JCZ, Armstrong M, Brennan K, Fisher A, Griffiths AD, Hill B, Milne DJ, Palmer C, Ward S, Watson M, Winderlich S, Young S (2010) Monitoring indicates rapid and severe decline of native small mammals in Kakadu National Park, northern Australia. Wildlife Research 37, 116-126.
| Crossref | Google Scholar |
Woinarski JCZ, Legge S, Fitzsimons JA, Traill BJ, Burbidge AA, Fisher A, Firth RSC, Gordon IJ, Griffiths AD, Johnson CN, McKenzie NL, Palmer C, Radford I, Rankmore B, Ritchie EG, Ward S, Ziembicki M (2011) The disappearing mammal fauna of northern Australia: context, cause, and response. Conservation Letters 4, 192-201.
| Crossref | Google Scholar |
Woinarski JCZ, Braby MF, Burbidge AA, Coates D, Garnett ST, Fensham RJ, Legge SM, McKenzie NL, Silcock JL, Murphy BP (2019) Reading the black book: the number, timing, distribution and causes of listed extinctions in Australia. Biological Conservation 239, 108261.
| Crossref | Google Scholar |
Woolley L-A, Murphy BP, Radford IJ, Westaway J, Woinarski JCZ (2018) Cyclones, fire, and termites: The drivers of tree hollow abundance in northern Australia’s mesic tropical savanna. Forest Ecology and Management 419–420, 146-159.
| Crossref | Google Scholar |
Ziembicki MR, Woinarski JCZ, Webb JK, Vanderduys E, Tuft K, Smith J, Ritchie EG, Reardon TB, Radford IJ, Preece N, Perry J, Murphy BP, McGregor H, Legge S, Leahy L, Lawes MJ, Kanowski J, Johnson CN, James A, Griffiths AD, Gillespie G, Frank ASK, Fisher A, Burbidge AA (2015) Stemming the tide: progress towards resolving the causes of decline and implementing management responses for the disappearing mammal fauna of northern Australia. Therya 6, 169-226.
| Crossref | Google Scholar |
Zuur AF, Ieno EN, Elphick CS (2010) A protocol for data exploration to avoid common statistical problems. Methods in Ecology and Evolution 1, 3-14.
| Crossref | Google Scholar |