Activity of dingoes (Canis familiaris) and their use of anthropogenic resources in the Strzelecki Desert, South Australia
Paul D. Meek


A
B
C
D
Abstract
Managing human–wildlife conflict where anthropogenic resources are provided is difficult. Providing food, water and shelter can result in over-abundant dingo populations, especially in Australian desert mine sites where managing dingoes, wildlife and humans around waste-management facilities and camps is problematic.
To measure and characterise the spatial activities of a population of arid-zone dingoes in relation to resources provided by a Cooper Basin (Strzelecki Desert, South Australia mining operation). The results were used to facilitate effective dingo management.
Free-roaming dingoes were captured, their morphometrics and ectoparasite presence recorded, and they were fitted with Iridium (GPS) radio collars. These were used to collect high-fidelity data about individual dingo activity and movements in relation to mine-site infrastructure and the Cooper Basin ecosystem.
A high density of dingoes (181 trapped in 2 km2 per 4 years) was associated with the mining operation. Home range/activity area sizes and usage of the anthropogenic landscape showed the following three categories of dingo: desert, peripatetic and tip dingoes. Dingoes reliant on food provisioning at the waste-management facility (WMF) displayed activity areas with a strong focus on the WMF (tip dingoes). Temporal activity patterns of another group of dingoes (peripatetic dingoes) were associated with regular waste-dumping times and normal nocturnal activity away from the WMF. Of the 27 dingoes collared, 30% (i.e. desert dingoes) were not dependent on the WMF, spending more time and a greater area of use in the desert dune system than in the mine-site area.
On the basis of the capture of 181 dingoes over 4 years and home-range analysis, it is likely that anthropogenic resource provisioning has caused an overabundance of dingoes in the Cooper Basin mine site. However, some of the dingo population remains reliant on native wildlife and resources in the surrounding desert. Managing food waste and excluding dingoes from food, water and shelter will result in a change in the prevalence of dingoes in the mine site, and subsequent reduction in the risk of disease transmission, native wildlife impacts, human conflicts and social pressures on dingoes, influencing them to revert to domestic-dog behaviours.
Waste-management facilities where food is dumped provide resources that lead to a change in wild-dingo behaviour, on the basis of their acceptance of human-provided resources, and high abundance. Managing access to anthropogenic resources will reduce the population as well as unwanted or aggressive encounters with humans. Dingoes reliant on food scraps will be encouraged to adjust their activity areas to desert habitat, thereby providing natural hunting opportunities and reduced contact rates with conspecifics, thus potentially reducing pathogen transmission.
Keywords: desert, ecology, food and water use, free roaming dog, home range, human–wildlife conflict, management, wild dog.
Introduction
Free-ranging dogs, including dingoes (Canis familiaris), are subject to conflict with humans throughout their range. Dingoes are an introduced canid from Asia that have become naturalised in Australia over the past ~5000 years (Jackson et al. 2017, 2019). Their ability to adapt to Australian environments, along with their co-occurrence with humans, has led to their widespread distribution. Following their introduction into Australia, the original canid ancestors became part of Aboriginal culture (Rose 1984), highlighting their domestication tendencies (Diamond 2002; Zeder 2006, 2012). These same tendencies continue today, with free-roaming dogs/dingoes exploiting the provisioning of food, water and shelter in remote areas, particularly near mine sites (Newsome et al. 2014, 2015; Déaux et al. 2018; Smith et al. 2020). In the Cooper Basin, management of dingo numbers is necessary to prevent human attacks such as those that have occurred on K’gari (Fraser Island) (Appleby et al. 2017; Tapply 2018; Behrendorff et al. 2023), disease spread and general nuisance issues. The provision of anthropogenic resources attracts large numbers of dingoes to occupy focal areas, and thus supports high densities (Becker and Hall 2014) around mining camps (Newsome et al. 2013a) and increases the risk of conflict with humans (Herrero and Higgins 2003). Dingoes are commensal with humans and, although they are wild animals, they exhibit many domestic behavioural traits in response to human interactions, including increased rest time (Altmann and Muruthi 1988; Déaux et al. 2018). This leads to individual dingoes living largely within human settlements, in some cases entering quarters and stealing food, human clothing and so on, which increases the occurrence of human–wildlife conflict (Newsome and Van Eeden 2017; Behrendorff et al. 2023). Further, a reliance on these resources can lead to development of anticipatory behaviour, where animals become reliant on and anticipate when food will be provided (Pini-Fitzsimmons et al. 2018). Similarly, provisioning of water in a landscape that was devoid of permanent water prior to European settlement (James et al. 1995) provides advantages to Australian wildlife (James et al. 1999).
A piosphere effect (Osborn et al. 1932) can be evident around anthropogenic food and, to a lesser degree, water-provisioning sites in arid/desert environments. This piosphere effect can even cause deep and obvious dingo walking pads (Eldridge 1996), linking these sites and the desert (P. Meek, pers. obs.). Provisioning of water in dams can help sustain larger numbers of dingoes than normal (Newsome and Corbett 1977) and dingo numbers in arid Australia are higher closer to water bodies (Brawata and Neeman 2011). In the central Australian arid zone, some water is available as either seasonal free water (ephemeral swamps) or agricultural and mining dams (settling ponds), although these are not common.
Managing dingo populations for human health and safety, as well as ecological and cultural goals, is required in the Cooper Basin mine area under management plans (Anon 2017a, 2017b). Controlling the number of dingoes living within waste-management facilities and camps also provides some disease-mitigation benefits for individual dingoes that suffer extreme sarcoptic mange (Sarcoptes scabiei) infection (Murray et al. 2015) because of high contact rates. The effect on the epidemiology of disease and pathogens of abnormally high animal densities of dingoes resulting from dependence on human resources (Becker and Hall 2014) is under-recognised in Australia. Provision of food and water can lead to an increase in wildlife population sizes and thus increase pathogen transmission (Becker and Hall 2014). Moreover, although providing food can reduce nutritional stress, this may cause prolonged infection (Becker and Hall 2014). In the case of dingoes, food and water provisioning in the arid zone leads to increased abundance, resting (Newsome et al. 2015), use of focal points, and tolerance of conspecifics (Newsome et al. 2013b), and probably has a negative effect on biodiversity through increased predation (Harrington et al. 1999; Davies et al. 2010). Whether undertaken for ecological, human-safety or dingo-welfare reasons, the management of over-abundant populations requires ecological knowledge of dingo ecology.
Understanding how dingoes use the resources available in mining camps and surrounding areas is an important first step in developing strategies for managing an over-abundant population. To this end, we studied a population of dingoes to evaluate their activity, movement patterns and use of the anthropomorphic resources provided in a mining camp. The knowledge gained from this study together with observations and data collected since 2015 will form the foundation of management recommendations for managing dingoes in desert mine sites.
Materials and methods
Study area
The GPS radio-tracking study was conducted in the gas fields of the Cooper Basin (28°07′25.91″S, 140°11′45.65″E) surrounded by the Strzelecki Desert in South Australia (Fig. 1). The Cooper Basin is located in north-eastern South Australia, north of Lyndhurst and south of Innamincka. The weather is predominantly hot and dry, with an annual rainfall of 200–300 mm, and temperature ranging from 0 to 50 degrees Celsius between winter and summer (10 September 2023, Bureau of Meteorology). The soils profile consists mainly of clay-rich vertosols and kandosols, with dune systems and low-lying wetlands. Vegetation is broadly sparse shrublands and scattered grasslands. The gas-field mine site has restricted access and consists of the mine camp which includes residential and industrial infrastructure (roads, airport, gas plant and living quarters) and a waste-management facility (WMF) where all food scraps are deposited daily (food pit), and rubbish is stored prior to recycling or burial (Fig. 1).
Data collection
Dingoes were trapped between 2015 and 2019 by using Victor Soft Catch #3 traps fitted with a short chain and a double-spring system; they were double-staked to the ground by using 40 cm pegs previously described in Meek et al. (2019a). A variety of lures was used, and traps were placed strategically around pads and tracks in the study site to optimise encounters by dingoes. All dingoes collared in this study were trapped around the mine site within a 2 km2 radius of the WMF. Capture success was improved over time by using a skunk lure (Skunkshot™ gel) from Connovation in New Zealand. The smell of this novel lure appeared to stand out above the cornucopia of dingo urine and faeces, and food that is in super high abundance at the site. All traps were checked and cleared throughout the day (sunrise) and early evening between 2000 hours and 2400 hours, depending on capture success. Trapping to fit GPS radio collars was peformed over 2 weeks in September 2019.
Animal handling and collaring were conducted consistent with standard practice (Fancourt et al. 2022; Morrant et al. 2022) and in accordance with animal ethics approval. Dingoes captured for collaring were restrained using a Ketch-All pole, then lifted onto a capture board and restrained with three straps and placed in the shade. The trapped foot was immediately massaged with Hirudoid® cream to reduce any swelling. Animals were offered water in the corner of the mouth and if a swallowing response was observed, this was continued until they stopped drinking. A fabric cover was placed over the dingo’s head to block visual stimuli and to assist with calming the animal. In accordance with animal ethics approval, morphological data were collected and a biopsy was collected from the ear with an Allflex sampling kit® and analysed by an independent laboratory Zoological Genetics (South Australia) by using microsatellites (Wilton 2001; Stephens et al. 2015). DNA analysis was undertaken to assess kin and subpopulation relationships within the population and elsewhere across Australia, so as to gain understanding of family relationships and lineage (Stephens et al. 2022). A small, coloured ear tag was fitted into the biopsy hole to aid identification at a distance and a microchip was inserted into the nape. A Lotek GPS radio collar (Litetrack/Pinpoint Iridium) with a time-release drop-off (TRD) was fitted to each animal where the collar was <5% bodyweight. The TRD was programmed to release after 12 months to preclude re-trapping each dingo. Transmitters were set to record at 30-min intervals for a settling period and then 5- and 15-min fixes were programmed over the life of the collar to produce high-frequency coordinates. Each collar was also programmed with a mortality function, which sent an email alert to one of the authors (PM) when a collar remained stationary for longer than 24 h.
Basic morphology data were recorded for each captured animal in this study, including data from dingoes trapped at the study site since 2015 by the authors (Meek and Brown 2017; Meek et al. 2019a, 2019b, 2020). All trapped dingoes were weighed, sexed and observations of reproductive physiology and pathology were recorded during 2015–2019, and visual observations during field work and camera-trap monitoring during a technology project until 2021. The extent of mange infection was scored as a proportion of the body infected, i.e. fur loss and ulceration, for each individual.
GPS-tracking fixes were monitored using the Lotek GPS Web Portal. Where a collar could not be recovered because of transmitter failure, fixes were downloaded from the portal. We programmed a high fix rate (5–15 min) to investigate the relationship between the dingoes and the facilities and resources provided by the mining operation. All collars producing a signal indicating mortality or drop-off because the TRD date had passed were located using a hand-held receiver and Yagi antenna. In cases of collar failure or where battery life had expired, the last known point from the portal was programmed into a GPS and a search was conducted in the vicinity. When collars were located, all fixes were downloaded directly from them by using the Lotek software package Pinpoint Host 2.15.19.0. Fix accuracy was calculated using repeated mortality fixes for each dingo to derive a value in metres.
Three Swift Enduro 3G camera-traps were placed on the road to the WMF on brackets attached to star pickets at a height of 1.5 m above ground, so as to avoid interference from dingoes. The cameras were attached to a solar panel to prolong battery life and programmed to take one photo per trigger and transmit data via the telecommunications network to the researchers. Photos were reviewed and tagged to collect date- and time-stamped records of movements of the food-waste trailer (FWT) to the WMF. On the basis of this imagery, we generated a data set of visits to the food pit inside the WMF, indicating when food was provided. We calculated from the camera-trap images that there was a 5–20 min gap between detection of the FWT on the road to the WMF and when the food was deposited. This was due to driver behaviour, gate access and food-pit activity. No data were available on the quantities of food scraps deposited each visit.
Data analysis
GIS files provided by the mining company allowed us to geo-reference human made structures such as roads, easements, buildings, settling ponds and the WMF, which enabled us to overlay dingo location data, and thus measure spatial and temporal use patterns.
Collar data were returned from the web portal or devices as CSV files. Data were pooled and relevant columns from the data imported into R (v. 4.2.1; RStudio 2022.07.1 + 554) (R Core Team 2022; RStudio Team 2022). Data were first filtered to remove fixes with incomplete records, before fixes with a dilution of precision (DOP) value greater than 10 were filtered out. The remaining fix timestamps were converted to Australian Central Standard Time from Greenwich Mean Time before a custom function was run to remove a single day’s worth of fixes from each end of the active dataset for each dingo (24 h post-capture and pre-mortality/collar drop off). Last, fixes were manually assessed to remove any that had escaped the filtering process, but were obviously erroneous; that is, too far away from the previous or subsequent fix to be a biologically plausible movement (hundreds of kilometres away) for a dingo. Only one fix across the entire collared population was removed in this final step.
Several home-range analyses were run to evaluate the best-fitting method for the dingo population, including minimum convex polygon (used for comparisons with historical studies), adaptive kernel estimate, kernel estimate, Brownian bridge, biased random bridges and convex hull. Because some of the dingoes at the WMF exhibited small activity areas associated with anthropomorphic resources, we extracted those animals for use in a Brownian bridge analysis to examine, in detail, areas of intense use associated with the food-waste pit. Hereafter, we refer to the area often described as a home range as an activity area because data collection did not span the entire year for the entire population and may not be a complete record of their use of the landscape throughout the year.
We refined the activity-area analysis using three analogous techniques, first 95%, and 50% minimum convex polygons (MCP), 95% and 50% kernel utilisation distribution (UD) and 95% kernel Brownian-bridge utilisation distribution (BB). MCPs, UD and BB were all calculated in R, using package adehabitatHR (v-0.4.19, mcp(), kernelUD() and kernelBB() functions respectfully). Simple kernel UD activity areas were produced with fixed smoothing values calculated within the function (‘href’ in adehabitatHR documentation). BB activity areas require the input of two smoothing variables (σ1 and σ2;). The first (σ1) relates to the speed of the animal between two GPS points and accounts for potential wandering by the animal between points. The second (σ2) is the error (in metres) of the GPS location produced by the collar. For our purposes, a σ2 of 5 m was rounded up from 3.79 m for ease of analysis on the basis of a fix accuracy calculated from repeated mortality fixes for each dog (3.76 m; s.d. = 2.83) (see Results). The values of σ1 were then calculated in R (adehabitatLT v-0.3.25, liker function). Outputs were exported to shapefiles (sf v-1.0.3, st_write function) and scrutinised in ArcMap. Figures were also produced in ArcMap (v-10.6.1).
Anthropogenic impacts were assessed by geofencing all animals’ fixes within a 150 m radius of the food pit at the WMF (Fig. 1) and by using the R (package circular) to identify the temporal distribution of food-pit visits by dingoes to examine whether these coincide with the temporal distribution of dumping events.
The percentage of days each animal attended the WMF (within 150 m radius) of all days recorded was also calculated. These values were correlated to activity-area sizes and movement for each animal in R (package geosphere v1.5-14, distm() function). Here, daily displacement was defined as the largest distance (calculated pairwise) between all fixes in a single day and daily distance as the length of the track taken over a 24-h period. Finally, the distance of every fix from the waste facility was calculated within ArcMap (Generate Near Table; Analysis Tools). From this analysis, the maximum, mean and minimum were averaged for each individual for each time of day and scrutinised. These individual values were then combined to assess the average distances from the WMF over time for the entire collared population. Outcomes of this analysis showed three potential types of dingoes, the results are presented cognisant of these findings. These groupings were validated using an ANOVA; proportion of days spent in the WMF, 95% utilisation distribution and average daily distance travelled).
Results
Dingo density estimates, genetics, morphology and reproduction
Between 2015 and 2019, 181 dingoes were trapped within a 2 km2 area associated with the mining operation, including 27 that were radio collared. This resulted in a high annual average density of dingoes (22.625 dingoes/km2.year). Analysis of 125 DNA samples was conducted for purity, including all collared animals (n = 27). Using the classification system of Stephens et al. (2015) and Wilton et al. (1999), 97.6% of tested animals were pure dingoes or possibly pure dingoes (i.e. 80–100% dingo genetics classification system), only three animals were dingo × modern dog hybrids and DNA could not be extracted from one sample.
The mean weight of adult dingoes (n = 162) was 15.9 kg, with female mean weight being 14.1 kg (range 9–20 kg) and males 15.9 kg (range 11–24.5 kg). Nineteen individuals were removed from the sample because they were pups or subadults, or weighed less than the 5% collar-to-body weight threshold required for collaring. The mean weight of the adult female dingoes collared in 2019 was 12.1 kg (range 9–16.5 kg); males were larger, with a mean weight of 17.1 kg (range 11–24 kg).
In total, 2 (7%) of the 27 collared animals were black or black and tan; the remaining animals had ginger coats, which is consistent with predominance of ginger coats in the larger population sample of the 181 dingoes from the Cooper Basin (Table 1).
Coat colour | Number | Percentage | |
---|---|---|---|
White | 7 | 4 | |
Brindle | 11 | 6 | |
Black/black and tan | 19 | 10 | |
Ginger | 144 | 80 | |
Total | 181 |
The reproductive physiology of the Cooper Basin dingoes (n = 181) was comparable to that of dingoes in other habitat types and arid-zone sites (Van Dyck and Strahan 2008; Baker and Gynther 2023); that is, they produced one litter per year, with pups being recorded mostly in the August–October period, lactating females seen from early August into September and whelping occurring from September until December. Litters of five to six were common, although observations (PM) suggested that survival was mostly one or two pups reaching maturity.
GPS radio tracking data
GPS collars were fitted to 20 males and seven females. Animal ethics approval allowed trapping of 30 dingoes. After filtering steps, collars returned an average of 15,014 fixes per dingo (min = 2399, max = 18,843; Table 2). Dingoes were radio tracked for 13–347 days (Table 2), with three collars failing and dropping off within 29 days (13–29 days). Dingoes were named for the purposes of engagement with mine workers and will be referred to hereafter by using those names. Summary data are provided in subsequent sections.
Name | Sex | Number of fixes | Number of days collared | Minimum convex polygon 95% (km2) | Utilisation distribution 95% (km2) | Brownian bridge kernel 95% (km2) | |
---|---|---|---|---|---|---|---|
Desert dingoes | |||||||
Julie | Female | 16,607 | 252 | 800.0 | 588.9 | 335.3 | |
Holly | Female | 17,047 | 253 | 740.8 | 607.2 | 124.4 | |
Dozer | Male | 17,364 | 340 | 2400.2 | 2526.1 | 1078.0 | |
Sunny | Male | 16,666 | 131 | 1830.6 | 1350.6 | 525.3 | |
Jamo | Male | 18,101 | 171 | 1470.6 | 902.4 | 500.5 | |
Sarge | Male | 18,580 | 280 | 801.9 | 741.1 | 489.7 | |
RikMan | Male | 18,845 | 338 | 703.1 | 677.5 | 403.4 | |
Yuley | Male | 8909 | 29 | 510.6 | 1014.5 | NA | |
Peripatetic dingoes | |||||||
CeeCee | Male | 17,373 | 341 | 485.7 | 211.9 | 238.1 | |
Durian | Male | 17,448 | 341 | 359.8 | 166.4 | 249.0 | |
Clearasil | Male | 9984 | 225 | 339.5 | 313.6 | 259.1 | |
Hurricane | Male | 17,423 | 340 | 271.4 | 258.5 | 234.3 | |
Shauno | Male | 17,331 | 341 | 232.0 | 123.3 | 229.2 | |
Leonardo | Male | 17,075 | 246 | 115.6 | 74.2 | 267.8 | |
Tip dingoes | |||||||
Mooka | Female | 16,536 | 131 | 230.5 | 212.1 | 86.7 | |
Demelza | Female | 10,237 | 167 | 90.1 | 50.5 | 71.3 | |
Mabel | Female | 16,561 | 133 | 64.5 | 61.0 | 39.4 | |
Shadow | Female | 16,460 | 125 | 58.2 | 34.2 | 20.4 | |
Heather | Female | 17,275 | 347 | 17.4 | 11.5 | 16.3 | |
Bernie | Male | 18,376 | 229 | 94.9 | 58.5 | 82.5 | |
MickTaylor | Male | 16,558 | 106 | 66.4 | 31.4 | 21.9 | |
Ibrahim | Male | 5029 | 18 | 62.6 | 99.6 | 17.8 | |
Wesley | Male | 9975 | 154 | 31.1 | 16.7 | 19.5 | |
Hun | Male | 16,955 | 218 | 21.8 | 13.4 | 16.4 | |
Obsidian | Male | 3536 | 13 | 19.3 | 15.0 | 7.0 | |
Capybara | Male | 16,433 | 133 | 16.3 | 18.1 | 25.0 | |
Schwob | Male | 16,305 | 130 | 9.0 | 7.4 | NA |
Dingo classifications based on activity-area analysis
The activity areas of dingoes in the Cooper Basin mine site ranged from 9 to 2400 km2 (95% MCP), with each of the dingoes using the WMF compared with the desert in different proportions. By analysing GPS fixes in relation to the distance that each dingo roamed from the WMF area, and by evaluating the proportion of location points in the landscape (i.e. WMF vs desert) used to generate activity areas (Table 2, Fig. 2), we separated dingoes into the following three classifications: tip, peripatetic and desert dingoes. Tip dingoes were those that focussed the largest proportion of their time (proportion of fixes) in the WMF or within a 20 km radius of it (Fig. 2). Peripatetic dingoes were those that showed a predisposition to share their activity between the desert and the WMF (<40 km), with a stronger association with the desert ecosystem than the food pit (Fig. 3). Desert dingoes were animals that utilised the WMF only rarely, with activity mostly occurring within 80 km of the mine site (Fig. 2). These classifications were validated further by testing the relationship among classifications and the amount of time spent in the WMF (F2,24 = 18.4, P < 0.05) and the size of individual dog-activity areas (F2,24−20.9, P < 0.05). Correlation of ‘percentage days spent at WMF’ and activity size and daily movement resulted in significant negative correlations (R2 = 0.40, F1,25 = 16.63, P < 0.05 and R2 = 0.16, F1,25 = 5.12, P < 0.05 respectively).
Activity areas of representatives of three dingo groups (tip, peripatetic and desert dingoes) using Brownian bridge 95% analysis to calculate activity areas in the Cooper Basin. Red dots represent the mine WMF.
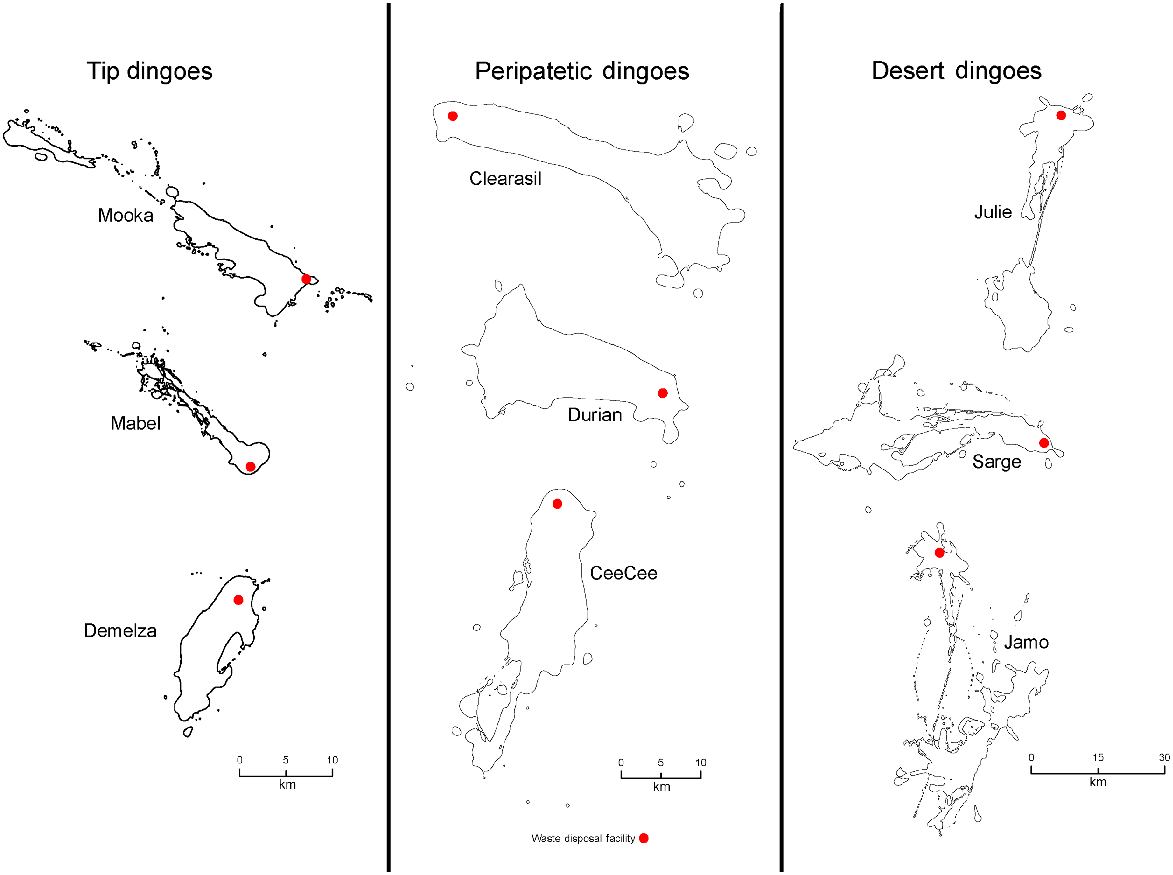
The activity area and movement by a male dingo (Sunny, desert dog) over 131 days from early spring to mid-summer from the mine camp towards Innamincka.
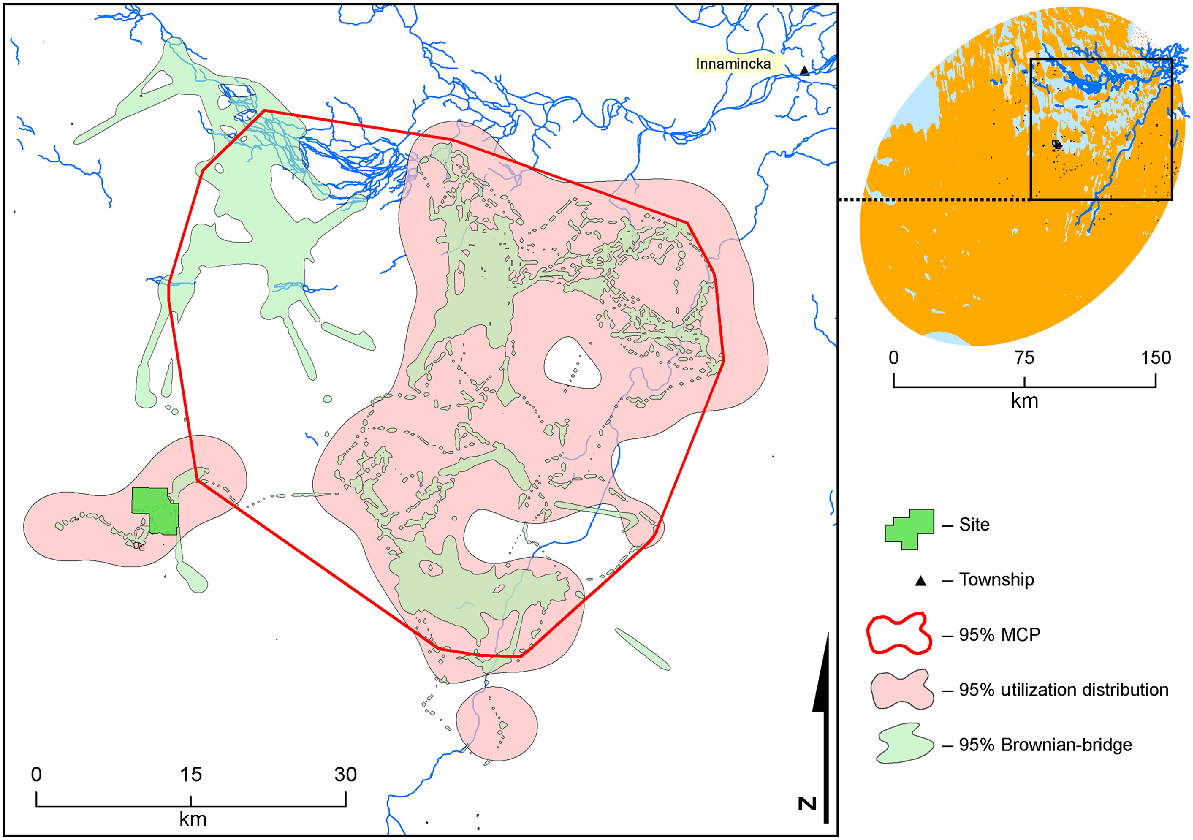
Desert dingoes had larger activity areas than did the other dingoes that utilised the WMF, regardless of the method used to calculate area (UD95%, F2,24 = 20.9, P < 0.05). We could not calculate a meaningful activity area for dingo Yuley by using BB95% because the distribution of his fixes did not fit the methodology assumptions. Likewise, this method was not accurate in calculating activity areas for Schwob, because he had a strong affinity with the WMF. Desert dingoes had activity areas ranging from 589 to 2526 km2 (UD95%)/125–1078 km2 (BB95%), compared with the activity areas of 74–314 km2 (UD95%)/229–268 km2 (BB95%) for peripatetic dingoes and of 12–212 km2 (UD95%)/7–87 km2 (BB95%) for tip dingoes (see Supplementary Material Table S1).
Waste management-facility use and associations with dingo activity
There was a relationship between the daily distance travelled and type of dingo on the basis of their use patterns of the WMF (F2,24 = 6.18, P < 0.05; Fig. 4). Tip dingo movement was mostly short-distance travel around the WMF and in the vicinity of established infrastructure and the food pit (Fig. 5). Peripatetic dingoes were recorded using more of the surrounding dune ecosystem, but spent a similar amount of time around the infrastructure of the WMF, which includes the food pit. The desert dingoes did not show any specific tendency to utilise infrastructure or the WMF and spent most of their time in the dune system (see Figs 2 and 3). Dozer and Sunny appeared not to have a strong use pattern around the WMF. Wesley was observed on camera-trap (data collected for a separate study) returning to the WMF periodically for months after his collar failed. Only one dingo used the camp infrastructure, whereas 10 dingoes visited water bodies on the outskirts of the camp boundary, using well-worn dingo pads connecting the two sites. Uncollared dingoes were common in the mine site camp throughout the day, but the highest activity was early morning and night.
The utilisation-distribution (UD) activity area, percentage of time spent in the waste-management facility (WMF) and average daily distance travelled by dingoes in the Cooper Basin. TD, tip dingo; PD is peripatetic dingo; and DD, desert dingo. 95% UD refers to the activity-analysis method utilisation distribution.
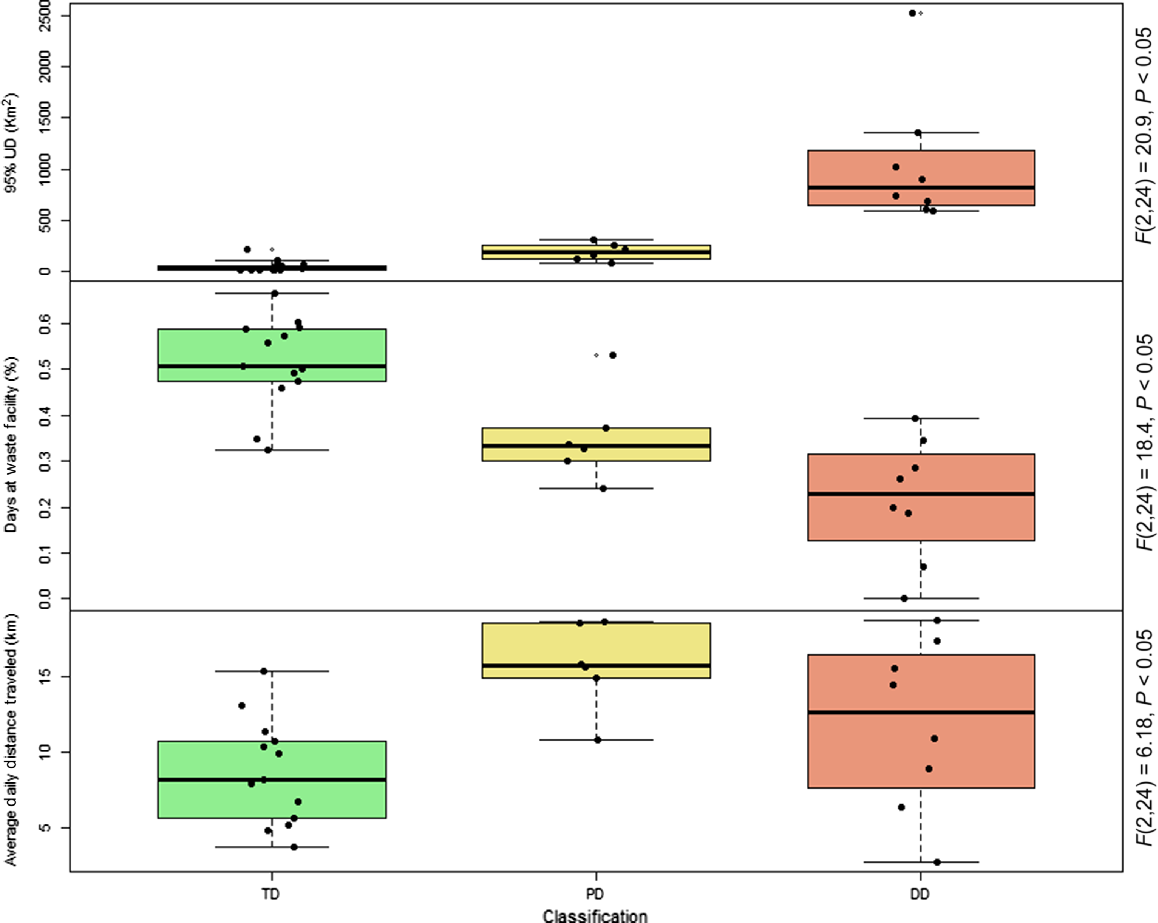
Long-range movements
Eight dingoes conducted long-distance forays (Fig. 6). A male dingo (Sunny) walked from his activity area towards Innamincka (Figs 3, 6), a linear distance of ~100 km from capture site to township. Seven dingoes displayed regular long-distance movements, travelling >45 km between the WMF and their preferred desert activity area (Fig. 6). A male dingo (Dozer) that was trapped in the WMF (but rarely seen in the area thereafter) occupied a largely desert home range (Fig. 6) where his collar dropped off ~125 km from the mine site in an extremely remote location only reachable by foot.
Food provisioning
On the basis of the output from kernel density estimates over time, dingoes most commonly accessed the WMF at night, despite the FWT depositing food waste from the mine camp mess hall twice daily. On average, the FWT entered the WMF at 0600 hours and 1200 hours, which corresponded with food-hall closure times (Fig. 7).
Temporal activity of four tip dingoes showing a strong relationship with their fixes within 150 m of the food pit when the food truck unloaded mess-hall food in the morning.
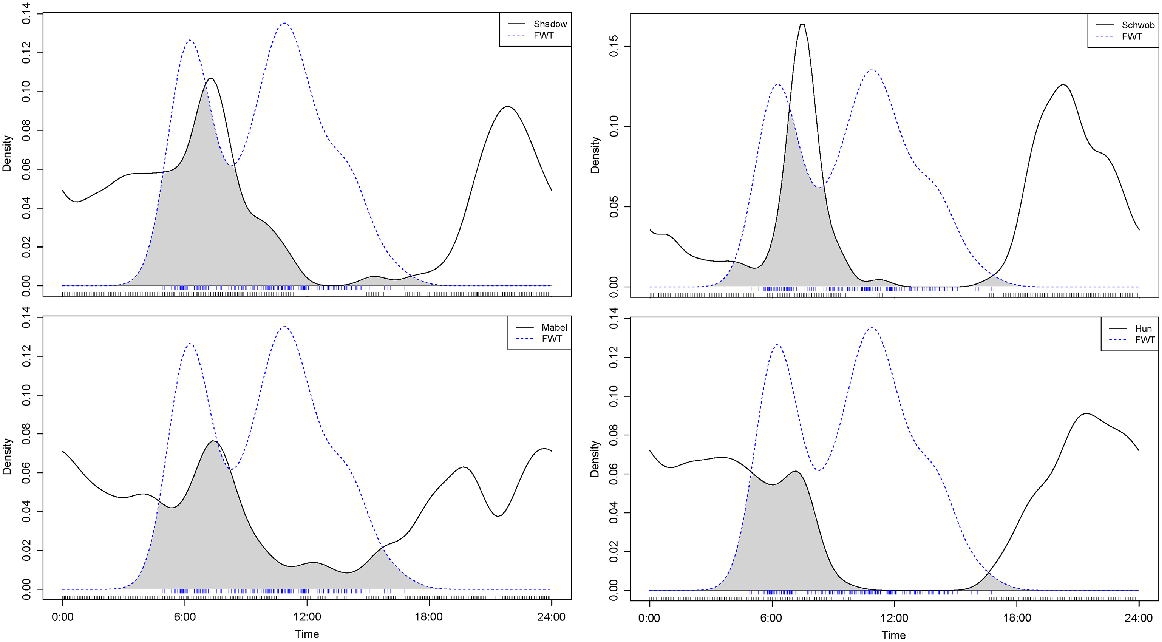
Once we accounted for the time delay of 5–20 min between when the camera-traps detected a FWT driving to the pit and when the food was actually dropped in the pit; four dingoes showed clear peaks in activity at the corresponding times (Fig. 7), especially in the early morning (breakfast). Visitation to the food pit was also high during the evening and night (sunset–sunrise) when dingoes are most active (Thomson 1992).
The WMF was used by all the collared dingoes to varying extents, with only one animal (Sunny) never returning to the WMF after being caught and released. Although the desert dingoes infrequently used the WMF (Fig. 2), they did make occasional sallies to the food pit. Analysis showed that the vast majority of dingoes were more likely to be closer to the WMF at night and further from the WMF in the early afternoon. These results were consistent throughout the population, and so were averaged across all dingoes and plotted to visualise the trend (Fig. 8).
The average minimum, maximum and mean distance (km) of the dingo population in the Cooper Basin from a waste-management facility (WMF) throughout a 24-h period.
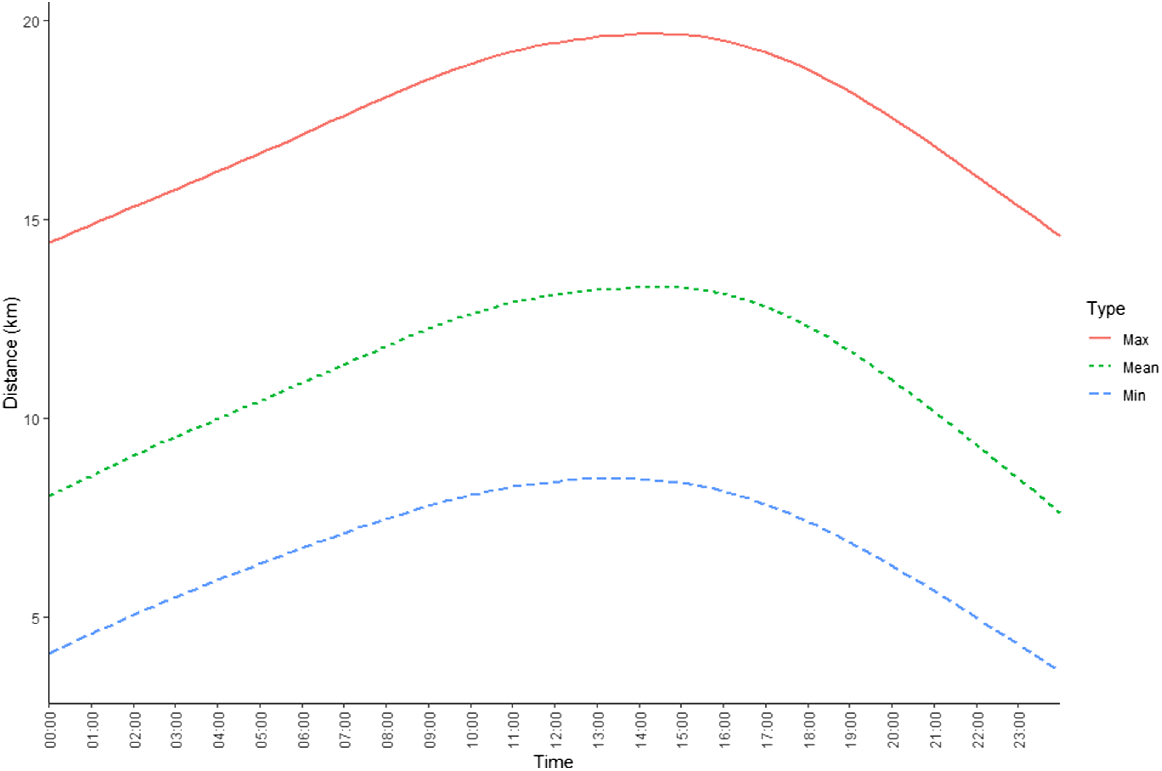
Despite a few permanent water bodies (settling ponds) being accessible to all the collared dingoes, 13 of them visited these sites infrequently. Eight dingoes visited water bodies multiple times, but there was no focus in activity associated with permanent water bodies. Uncollared dingoes were observed drinking from buckets of water and leaking garden taps at the WMF on occasions during summer.
Time-release drop-off (TRD) and GPS radio-collar failures
A failure of the time-release drop-offs (TRD) resulted in collars falling off prematurely or failing to fall off altogether. Wesley’s and Demelza’s collars both failed to drop-off and, despite extensive searching, VHF failure prevented uploading of data from the collars and had to be downloaded from the Lotek portal. This reduced the number of location data points we could analyse for those dingoes because we discovered there were more data stored on the collars than were uploaded to the Lotek portal server. The difference in the number of data points between the collar and the portal was likely a combination of the high frequency of data points being stored on board the collar and the low trajectory of the satellite, which reduced the upload time between the collar and the satellite (Ben McCleave, pers. comm. 2020). Recovery of collars was further complicated by battery failure of the VHF transmitter in the collar. Fortunately, the low-lying topography of the desert permitted quite accurate location data when the collars were in mortality mode (3.76 m, s.d. = 2.83 m), so walking to the site and visually finding collars was often possible. These issues are consistent with those reported elsewhere (Matthews et al. 2013), but highlighted the need for continued improvement in wildlife-research equipment to match costs with data quality.
Dingo pathology
Of the 27 dingoes trapped and collared, 43% showed evidence of sarcoptic mange; only two animals were affected over more than 50% of their body mass. Mange has been common in the Cooper Basin dingo population since the authors commenced research at this site in 2015. Severity of infection has resulted in serious disfigurement of some animals and the production of large joint lesions and disfigurement of the claw anatomy. The most likely cause of the cyst-like lesions are hygromas, which are connective tissue sacs of synovial fluid that often form around elbow joints as swollen bursae (Johnston 1975).
Discussion
In comparison to other dingo-density estimates (i.e. 0.01–0.7 individuals/km2; Gabriele-Rivet et al. 2019, 2020) and similarly to some other studies of arid-zone dingoes near mine sites (Newsome et al. 2013a; Smith et al. 2020), the density of our study dingo population was very high at the mine site. This anomalous high density was likely to be attributable to anthropogenic resourcing, as was found by Newsome et al. (2013a) in a similar central Australian situation. Our dingo population displayed high purity, as has been found elsewhere in central Australia (Newsome et al. 2013a; Stephens et al. 2015), which justifies direct comparisons with previous studies.
We classified the Cooper Basin dingoes into three types according to their use of the WMF and surrounding desert habitat (Figs 2, 4, 5). There were differences in the size of the activity areas of the three dingo groups, with desert dingoes occupying a much larger area (mean = 494 km2) than did tip dingoes (mean = 35 km2) and peripatetic dingoes (mean = 246 km2), being similar to area occupied by dingoes in the Tanami Desert (Newsome et al. 2013b) and wolves (Canis lupus) in North America (Petroelje et al. 2019). Our observed difference was also reflected in the WMF visitation rates of the different dingo classifications, which was much higher in the tip population than the other groups (Fig. 3).
A classification of Mine and Away dingoes has been used for Tanami Desert dingoes (Newsome et al. 2013b). Dingoes living close to the mine site in the Tanami Desert recorded kernel home ranges of 0.68–10.8 km2 (mean 10 km2); Cooper Basin dingoes had larger activity areas of 7–87 km2 (BB95%; Fig. 2). Cooper Basin dingoes that relied less on the WMF and more on the desert had a mean home-range/activity area of 494 km2 (range = 124–1078 km2), similar to the mean home range size of 403 km2 for Tanami dingoes (range = 79.5–999 km2). Dingoes in the Western Australian desert had larger average activity areas (seasonal home range using kernel density) of 480–1020 km2 (Wysong et al. 2020), whereas Allen (2012) estimated that dingo packs in the Strzelecki Desert had activity areas of only ~24 km2.
Several dingoes in the current study exhibited long movements into the desert, with distances covering >200 km (Figs 3, 6).
GPS data and observations of dingoes in the Cooper Basin (Fig. 8) were consistent with those in other studies in that they identified crepuscular–nocturnal peaks and inactivity mostly during the day (Tatler et al. 2021), especially in the summer. We found that dingoes were closer to and more likely to visit the food pit during the period from 2200 hours to 0200 hours. This corresponds with direct observations of increased numbers of dingoes and vocalisations occurring from sunset to sunrise, and is further supported by trapping records from previous studies (Meek and Brown 2017; Meek et al. 2019a, 2019b). On sunset, dingoes were consistently observed heading from within the WMF and the surrounding areas to the food pit during this study and in the 7 years we have been working at this site. During the day, some tip dingoes did display increased activity close to the food pit mid-morning and around midday when the FWT was dumping mess-hall food waste (Figs 7, 8). On the basis of collared dingo data and observations of uncollared dingoes close to the food pit, some dingoes displayed learned behaviour when the food truck approached the WMF. Competition to access freshly dumped food by other dingoes and an abundance of raptors and corvids could have influenced this behaviour. Even when machinery was used to cover freshly deposited food, dingoes and birds would immediately scavenge in the area, often at the risk of being run over and killed by the tracked excavator. This reliance on food (anticipatory behavioural development) by some dingoes was apparent by the focus of their activity around the WMF. However, although some of the study animals were recorded visiting settling ponds where water was freely accessible, their activity was not as focussed on water sites as it was food.
It was not within the scope of this study to analyse the diet of the dingoes in the Cooper Basin. However, the WMF was littered with dingo scats at an extremely high density throughout 2015–2021 and none had the appearance of normal, well-composed scat with digested flesh. Instead, a very high occurrence of rubbish (plastic bags) and litter in scats was evident These observations concur with dingo scats collected in the Tanami (Newsome et al. 2019). In 2021, one of the authors (PM) observed a lactating female successfully hunting burrowing dragon lizards 20 km away from the WMF. Also, like Newsome et al. (2019) and (Smith et al. 2020), who reported cannibalism among dingoes when food resources were provided, aggressive behaviours towards constrained (trapped) dingoes as well as cannibalism did occur at this site (Meek and Brown 2017). Nonetheless, given the high density of dingoes and high contact rates among individuals, aggressive behaviour was rare and was observed only in the food pit and when trapped.
Mange was common in the dingo population trapped and observed between 2015 and 2021. A study of coyotes (Canis latrans) in Canada showed that animals that relied on anthropogenic food subsidies tended to have higher levels of mange, and the resultant poorer health reduced their activity patterns and the size of their home range, which in turn facilitated their continued dependence on anthropogenic resources (Murray et al. 2015). Throughout the years 2015–2021, one of the authors (PM) made one or two field visits each year to the site for various research projects. Anecdotal data collected from trapping and camera trapping studies (Meek and Brown 2017; Meek et al. 2018, 2019a) suggested that dingoes that are almost hairless and ulcerated from severe mange and associated infection rarely moved far from the WMF. On several occasions, PM was required to euthanise dingoes with sever mange-related infection such that the animal’s spine was deformed, and large lesions developed on the legs affecting movement. There is a clear relationship between wildlife and anthropogenic resource provisioning on the health of wildlife populations. The additional resources often affect population demography by improving reproductive success and thus contact rates, resulting in amplified pathogen transmission (Becker and Hall 2014). Our sample size for each dingo classification was too small to enable any assessment of mange infection among dingo types with confidence. However, in terms of pathology, it might be expected that dingoes living in close contact with conspecifics would show a higher incidence of mange and animals with severe infection would not travel far from food and water.
The overabundance of dingoes at mine sites presents many management problems as discussed; however, the ecological consequences may also be problematic. Newsome et al. (2014) noted that the provisioning of resources at mine sites alters dingo diets and may result in higher predation on small mammals, but diet analysis (scat and stomach) would be required to confirm such increased predation. There is no evidence to suggest in the Cooper Basin that food provisioning will result in a decline in the abundance of dingoes as reported for other species that have declined as a result of ecological and behavioural changes (Peterson et al. 2005; Senigaglia 2020). In fact, the provision of easily accessible food and water to desert dingoes almost certainly causes a higher than normal abundance in the Cooper Basin (this study) and Tanami dingoes (Newsome et al. 2019). One explanation not previously explored is whether the provisioning of resources by humans and the safety afforded them by occupying a human settlement triggers an ancient domestic trait. Given dingoes are domestic dogs gone wild (Jackson et al. 2017, 2019, 2021), it is feasible that these dingoes revert to their domestic traits and behaviours of dependence, tolerance and acceptance of humans. Artificial food resources thus might essentially be triggering a re-taming of dingoes back to a more domestic type and re-igniting the artificial selection process that led to their domestication from wolf to dog approximately 17,000 years ago (Driscoll and Macdonald 2010). In the Cooper Basin, dingoes are commonly seen around people and some individuals become pet-like, hovering around doors to food halls and selected living quarters where mine workers feed them like pets. One of the authors (PM) has sat on the ground near one such dingo and photographed the animal licking his knee in anticipation of food. Many domestic-dog behavioural traits were recorded of dingoes in the mine site, such as playing with food wrappers and playing with humans in the manner of puppies. Similar behaviours are displayed by feral cats re-adjusting from wild-living to a stray and domestic existence (Serpell 2000), although this has not occurred in true wild introduced animals such as foxes (Vulpes vulpes), despite concerted efforts (Macdonald 1987; Trut 1999). If this is a valid proposition, then tip and peripatetic dingoes in the Cooper Basin, and similar types of dingoes in the Tanami (Newsome et al. 2013b), may have the genetic edge over desert dingoes as they revert to their domestic behaviours in response to anthropogenic food provisioning.
Analysis of the DNA from collared individuals confirmed that 97.6% of the collared population had a high percentage of dingo genes (Stephens et al. 2015), indicating that there has been little ingress from pastoralist domestic working dogs and that the isolation and security of the mine means that dingoes cannot inter-breed with any domestic dogs. Research is warranted to assess familial genetics among the three categories of dingo at the Cooper Basin, to determine whether behavioural traits such as dependency on anthropogenic resources are passed on to progeny within the tip and peripatetic dingoes.
Conclusions
Dingoes have adapted to and rely on anthropogenic food and other resources provided by human occupation in the arid zone, which has implications for population abundance, human–wildlife conflict and disease transmission (Newsome and Van Eeden 2017). This study found three types of dingoes in the Cooper Basin and a dependence by a proportion of the collared dingoes in using food, water and shelter provided by human presence. Excluding dingoes from food and water is a crucial first step in mitigating the consequences of providing resources to an animal that is highly susceptible to domestication. Exclusion fencing around waste facilities has proven to reduce dingo occupancy (Behrendorff et al. 2016; Newsome et al. 2019; Behrendorff et al. 2023) and should be a mandatory requirement in mine management planning. Ongoing efforts to process food waste to reduce the attraction of the food pit to wildlife should continue. Providing access to food, water and shelter, and the common practice of mine staff feeding dingoes will continue to encourage over-breeding, survival and behavioural attraction to mine workers, potentially leading to increasing levels of quasi-domestic behaviours that increase contact rates between humans and dingoes and causing over-abundant populations.
Declaration of funding
Funding for this research and previous projects was provided by the Department of Agriculture and Water Resources brokered via the Centre for Invasive Species Solutions. In-kind funding support was provided by Santos, project funding was also provided by NSW Department Primary Industries.
Acknowledgements
This research would not have been possible without the support of the Cooper Basin staff (too many to list); their assistance and friendship was paramount to the implementation of this project, and thanks go to Julie, Yuley, Wes, Rick, Shaun, Jamo and Andrew for field support. Thanks go to Donell Hole and Ben McCleave from Lotek for attempting to resolve our radio-tracking problems.
References
Allen BL (2012) Do desert dingoes drink daily? Visitation rates at remote waterpoints in the Strzelecki Desert. Australian Mammalogy 34, 251-256.
| Crossref | Google Scholar |
Altmann J, Muruthi P (1988) Differences in daily life between semiprovisioned and wild-feeding baboons. American Journal of Primatology 15, 213-221.
| Crossref | Google Scholar | PubMed |
Appleby R, Smith B, Bernede L, Jones D (2017) Utilising aversive conditioning to manage the behaviour of K’gari (Fraser Island) dingoes (Canis dingo). Pacific Conservation Biology 23, 335-358.
| Crossref | Google Scholar |
Becker DJ, Hall RJ (2014) Too much of a good thing: resource provisioning alters infectious disease dynamics in wildlife. Biology Letters 10, 20140309.
| Crossref | Google Scholar | PubMed |
Behrendorff L, Leung LK-P, McKinnon A, Hanger J, Belonje G, Tapply J, Jones D, Allen BL (2016) Insects for breakfast and whales for dinner: the diet and body condition of dingoes on Fraser Island (K’gari). Scientific Reports 6, 23469.
| Crossref | Google Scholar | PubMed |
Behrendorff L, King R, Allen BL (2023) Efficacy of management efforts to reduce food-related dingo–human interactions and conflict on K’gari (fraser island), Australia. Animals 13, 204.
| Crossref | Google Scholar | PubMed |
Brawata RL, Neeman T (2011) Is water the key? Dingo management, intraguild interactions and predator distribution around water points in arid Australia. Wildlife Research 38, 426-436.
| Crossref | Google Scholar |
Davies KF, Melbourne BA, James CD, Cunningham RB (2010) Using traits of species to understand responses to land use change: birds and livestock grazing in the Australian arid zone. Biological Conservation 143, 78-85.
| Crossref | Google Scholar |
Diamond J (2002) Evolution, consequences and future of plant and animal domestication. Nature 418, 700-707.
| Crossref | Google Scholar | PubMed |
Driscoll CA, Macdonald DW (2010) Top dogs: wolf domestication and wealth. Journal of Biology 9, 10.
| Crossref | Google Scholar | PubMed |
Déaux EC, Crowe T, Charrier I (2018) Recreational fishing alters dingo foraging behavior on Fraser Island. The Journal of Wildlife Management 82, 85-92.
| Crossref | Google Scholar |
Eldridge DJ (1996) Distribution and floristics of terricolous lichens in soil crusts in arid and semi-arid New South Wales, Australia. Australian Journal of Botany 44, 581-599.
| Crossref | Google Scholar |
Fancourt BA, Allen BL, Jackson SM, Meek PD, Zewe F, Ballard AG, Behrendorff L, Claridge AW, Fleming PJS (2022) Eutherian carnivores. In ‘Wildlife research in Australia: practical and applied method’. (Ed. BP Smith, HP Waudby, C Alberthsen, JO Hampton) pp. 428–436. (CSIRO Publishing: Melbourne, Vic., Australia)
Gabriele-Rivet V, Arsenault J, Wilhelm B, Brookes VJ, Newsome TM, Ward MP (2019) A scoping review of dingo and wild-living dog ecology and biology in australia to inform parameterisation for disease spread modelling. Frontiers in Veterinary Science 6, 47.
| Crossref | Google Scholar |
Gabriele-Rivet V, Arsenault J, Brookes VJ, Fleming PJS, Nury C, Ward MP (2020) Dingo density estimates and movements in equatorial Australia: spatially explicit mark–resight models. Animals 10, 865.
| Crossref | Google Scholar | PubMed |
Harrington R, Owen-Smith N, Viljoen PC, Biggs HC, Mason DR, Funston P (1999) Establishing the causes of the roan antelope decline in the Kruger National Park, South Africa. Biological Conservation 90, 69-78.
| Crossref | Google Scholar |
Herrero S, Higgins A (2003) Human injuries inflicted by bears in Alberta: 1960-98. Ursus 14, 44-54.
| Google Scholar |
Jackson SM, Groves CP, Fleming PJS, Aplin KP, Eldridge MDB, Gonzalez A, Helgen KM (2017) The wayward dog: is the Australian native dog or dingo a distinct species? Zootaxa 4317, 201-224.
| Crossref | Google Scholar |
Jackson SM, Fleming PJS, Eldridge MDB, Ingelby S, Flannery T, Johnson RN, Cooper SJB, MIitchel KJ, Souilmi Y, Cooper A, Wilson DE, Helgen KM (2019) The dogma of dingoes: taxonomic status of the dingo: a reply to smith et al. Zootaxa 4564, 198-212.
| Crossref | Google Scholar |
Jackson SM, Fleming PJS, Eldridge MDB, Archer M, Ingleby S, Johnson RN, Helgen KM (2021) Taxonomy of the dingo: it’s an ancient dog. Australian Zoologist 41, 347-357.
| Crossref | Google Scholar |
James CD, Landsberg J, Morton SR (1995) Ecological functioning in arid Australia and research to assist conservation of biodiversity. Pacific Conservation Biology 2, 126-142.
| Crossref | Google Scholar |
James CD, Landsberg J, Morton SR (1999) Provision of watering points in the Australian arid zone: a review of effects on biota. Journal of Arid Environments 41, 87-121.
| Crossref | Google Scholar |
Johnston DE (1975) Hygroma of the elbow in dogs. Journal of the American Veterinary Medical Association 167, 213-219.
| Google Scholar | PubMed |
Matthews A, Ruykys L, Ellis B, FitzGibbon S, Lunney D, Crowther MS, Glen AS, Purcell B, Moseby K, Stott J, Fletcher D, Wimpenny C, Allen BL, Van Bommel L, Roberts M, Davies N, Green K, Newsome T, Ballard G, Fleming P, Dickman CR, Eberhart A, Troy S, McMahon C, Wiggins N (2013) The success of GPS collar deployments on mammals in Australia. Australian Mammalogy 35, 65-83.
| Crossref | Google Scholar |
Meek PD, Brown SC (2017) It’s a dog eat dog world: observations of dingo (Canis familiaris) cannibalism. Australian Mammalogy 39, 92-94.
| Crossref | Google Scholar |
Meek PD, Shorter K, Falzon G (2018) Do lethal trap devices threaten foot-hold trap capture efficacy? International Journal of Pest Management 65, 66-71.
| Crossref | Google Scholar |
Meek PD, Brown SC, Wishart J, Milne H, Aylett P, Humphrys S, Ballard G, Fleming P (2019a) Efficacy of lethal-trap devices to improve the welfare of trapped wild dogs. Wildlife Research 46, 89-95.
| Crossref | Google Scholar |
Meek PD, Shorter K, Falzon G (2019b) Do lethal trap devices threaten foot-hold trap capture efficacy? International Journal of Pest Management 65, 66-71.
| Crossref | Google Scholar |
Meek PD, Ballard GA, Falzon G, Williamson J, Milne H, Farrell R, Stover J, Mather-Zardain AT, Bishop J, C. Ka-Wai Cheung E, Lawson CK, Munezero AM, Schneider D, Johnston BE, Kiani E, Shahinfar S, Sadgrove EJ, Fleming PJS (2020) Camera trapping technology and advances: into the new millennium. Australian Zoologist 40(3), 392-403.
| Google Scholar |
Murray M, Edwards MA, Abercrombie B, St. Clair CC (2015) Poor health is associated with use of anthropogenic resources in an urban carnivore. Proceedings of the Royal Society B: Biological Sciences 282, 20150009.
| Crossref | Google Scholar |
Newsome TM, Van Eeden LM (2017) The effects of food waste on wildlife and humans. Sustainability1269 9,.
| Crossref | Google Scholar |
Newsome TM, Ballard G-A, Dickman CR, Fleming PJS, Howden C (2013a) Anthropogenic resource subsidies determine space use by Australian arid zone dingoes: an improved resource selection modelling approach. PLoS ONE 8, e63931.
| Crossref | Google Scholar | PubMed |
Newsome TM, Ballard G-A, Dickman CR, Fleming PJS, van de Ven R (2013b) Home range, activity and sociality of a top predator, the dingo: a test of the Resource Dispersion Hypothesis. Ecography 36, 914-925.
| Crossref | Google Scholar |
Newsome TM, Ballard G-A, Fleming PJS, van de Ven R, Story GL, Dickman CR (2014) Human-resource subsidies alter the dietary preferences of a mammalian top predator. Oecologia 175, 139-150.
| Crossref | Google Scholar | PubMed |
Newsome TM, Dellinger JA, Pavey CR, Ripple WJ, Shores CR, Wirsing AJ, Dickman CR (2015) The ecological effects of providing resource subsidies to predators. Global Ecology and Biogeography 24, 1-11.
| Crossref | Google Scholar |
Newsome TM, Howden C, Wirsing AJ (2019) Restriction of anthropogenic foods alters a top predator’s diet and intraspecific interactions. Journal of Mammalogy 100, 1522-1532.
| Crossref | Google Scholar |
Osborn TGB, Wood JG, Paltridge TB (1932) On the growth and reaction to grazing of the perennial saltbush, Atriplex vesicarium. An ecological study of the biotic factor. Proceedings of the Linnean Society of New South Wales 57, 377-402.
| Google Scholar |
Peterson MN, Lopez RR, Laurent EJ, Frank PA, Silvy NJ, Liu J (2005) Wildlife loss through domestication: the case of endangered key deer. Conservation Biology 19, 939-944.
| Crossref | Google Scholar |
Petroelje TR, Belant JL, Beyer DE, Jr, Svoboda NJ (2019) Subsidies from anthropogenic resources alter diet, activity, and ranging behavior of an apex predator (Canis lupus). Scientific Reports 9, 13438.
| Crossref | Google Scholar | PubMed |
Pini-Fitzsimmons J, Knott NA, Brown C (2018) Effects of food provisioning on site use in the short-tail stingray Bathytoshia brevicaudata. Marine Ecology Progress Series 600, 99-110.
| Crossref | Google Scholar |
RStudio Team (2022) ‘Rstudio: integrated development environment for R.’ (RStudio PBC, Boston, MA, USA) Available at http://www.rstudio.com/
Smith BP, Morrant DS, Vague A-L, Doherty TS (2020) High rates of cannibalism and food waste consumption by dingoes living at a remote mining operation in the Great Sandy Desert, Western Australia. Australian Mammalogy 42, 230-234.
| Crossref | Google Scholar |
Stephens D, Wilton AN, Fleming PJS, Berry O (2015) Death by sex in an Australian icon: a continent-wide survey reveals extensive hybridization between dingoes and domestic dogs. Molecular Ecology 24, 5643-5656.
| Crossref | Google Scholar | PubMed |
Stephens D, Fleming PJS, Sawyers E, Mayr TP (2022) An isolated population reveals greater genetic structuring of the Australian dingo. Scientific Reports 12, 19105.
| Crossref | Google Scholar | PubMed |
Tapply J (2018) Contemporary dingo management on K’gari (Fraser Island, Great Sandy National Park) under the Queensland Parks and Wildlife Service. Australasian Journal of Environmental Management 25, 119-131.
| Crossref | Google Scholar |
Tatler J, Currie SE, Cassey P, Scharf AK, Roshier DA, Prowse TAA (2021) Accelerometer informed time-energy budgets reveal the importance of temperature to the activity of a wild, arid zone canid. Movement Ecology 9, 11.
| Crossref | Google Scholar | PubMed |
Thomson PC (1992) The behavioural ecology of dingoes in north-western Australia. II. Activity patterns, breeding season and pup rearing. Wildlife Research 19, 519-529.
| Crossref | Google Scholar |
Trut L (1999) Early canid domestication: the farm-fox experiment: foxes bred for tamability in a 40-year experiment exhibit remarkable transformations that suggest an interplay between behavioral genetics and development. American Scientist 87, 160-169.
| Crossref | Google Scholar |
Wilton AN, Steward DJ, Zafiris K (1999) Microsatellite variation in the Australian dingo. Journal of Heredity 90(1), 108-111.
| Crossref | Google Scholar |
Wysong ML, Hradsky BA, Iacona GD, Valentine LE, Morris K, Ritchie EG (2020) Space use and habitat selection of an invasive mesopredator and sympatric, native apex predator. Movement Ecology 8, 18.
| Crossref | Google Scholar | PubMed |
Zeder MA (2012) Pathways to animal domestication. In ‘Biodiversity in agriculture: domestication, evolution, and sustainability. Vol. 10’. (Eds P Gepts, T Famula, R Bettinger, S Brush, A Damania, P McGuire) pp. 227–259. (Cambridge University Press: Cambridge) doi:10.1017/CBO9781139019514.013