Habitat features important for the conservation of the endangered Sloane’s Froglet (Crinia sloanei) in peri-urban environments
Alexandra R. Knight


A
B
C
D
Abstract
Determining and quantifying habitat selection of endangered species in peri-urban environments assists planners and managers to develop strategies and alternative conservation measures in the face of urban expansion and development. Sloane’s Froglet (Crinia sloanei), listed nationally as endangered in Australia, is a little-known species distributed within peri-urban environments, where foundational ecological information and the development of adequate conservation responses has been lacking.
(a) To determine a core calling period for Sloane’s Froglet and detection probabilities for occupancy surveys. (b) To understand and characterise the habitat that Sloane’s Froglet uses at the wetland and microhabitat scale.
We used generalised linear modelling and the information-theoretic approach to model habitat preferences for this species at two scales: the waterbody scale, and the microhabitat scale. We quantified the habitat characteristics of waterbodies occupied by Sloane’s Froglet in winter, its peak breeding period, by measuring the biophysical characteristics of 54 occupied and 40 unoccupied waterbodies. The microhabitat and relative spatial positioning of Sloane’s Froglet within waterbodies was examined at 54 calling sites in an area of one m squared around individual male Sloane’s Froglets and 57 randomly selected unused sites. Wetlands were surveyed multiple times to determine detection probabilities.
Model selection indicated that Sloane’s Froglet is 450 times more likely to occupy a waterbody when an adjacent ephemeral shallow overflow is present; and are more likely to be present when there is greater cover of small stem-diameter emergent vegetation and less bare ground on the bank. The microhabitat investigation of one m squared sites showed that Sloane’s Froglet’s calling sites are predominantly inundated, and at significantly shallower water depths, than unused sites. Sloane’s Froglet was found to always call from within the waterbody, distinguishing them from other sympatric Crinia species.
The habitat characteristics detailed provide information necessary for the management of Sloane’s Froglet and its habitat.
Housing and industrial development is occurring rapidly in Sloane’s Froglet habitat. The information provided here can be used to refine local and state government planning and better design appropriate responses. Indeed, results from this study are currently being used by agencies and environmental consultants when developing conservation plans and in the design of stormwater retention ponds in rapidly urbanising environments.
Keywords: amphibian conservation, constructed wetlands, Eleocharis acuta, Box gum grassy woodland, habitat selection, Murray–Darling Basin, peri-urban biodiversity, Sloane’s Froglet.
Introduction
More than 40% of the world’s amphibians are threatened with imminent extinction (Luedtke et al. 2023). In Australia, and around the world, amphibian decline has predominantly been attributed to infection with chytridiomycosis (Scheele et al. 2019), climate change (Geyle et al. 2022) and habitat destruction and modification (Hero et al. 2006; Gardner et al. 2007). Gillespie et al. (2020) in their assessment of the conservation status of Australian frogs determined that habitat loss and alteration is contributing to the extinction threat for 22 of 44 threatened Australian frogs. Habitat modification is considered a threat in rural landscapes (e.g. Hazell et al. 2001; Hazell 2003) as well as in urban and peri-urban regions (e.g. Hamer and McDonnell 2008; Smallbone et al. 2011). Recently, habitat remediation has been recommended as an important way of battling frog disease (Heard et al. 2018; McKnight et al. 2019). Despite the acknowledged importance of understanding the role of habitat for threatened amphibian management, whether per se or for disease mitigation, better knowledge of ecological requirements is still needed for many Australian anurans (Gillespie et al. 2020).
Sloane’s Froglet (Crinia sloanei, Littlejohn 1958), a small ground-dwelling frog with a distribution in south-eastern Australia, is one species where knowledge of ecological requirements is lacking. First listed as a threatened species in 2008 as Vulnerable under the NSW Biodiversity Conservation Act 2016, and subsequently as ‘Endangered’ under the Australian Environment Protection and Biodiversity Conservation Act 1999, very little research has been undertaken on this species since its formal identification in 1958 (Littlejohn 1958). The available information on the species is considered ‘Data Deficient’ by the International Union for the Conservation of Nature (IUCN 2022). The listing of the species as threatened provided impetus for investigations into the distribution and habitat requirements to provide information to assist in the conservation of this species. No documented research had been undertaken into the perceived decline of Sloane’s Froglet until our work commenced in 2009 (Knight and Allan 2021).
Sloane’s Froglet is a member of the Myobatrachidae family containing approximately 91 species distributed only in the Australian region (Anstis 2013; Frost 2023). The Crinia genus contains 17 species and occurs over most of Australia except for very dry areas. They breed in water and lay a small number of eggs which are attached to vegetation or to the substrate (Anstis 2013). Some species of the Crinia genus are relatively well known; for instance there is considerable research into the common and sympatric Crinia signifera (Littlejohn 1964; Mac Nally 1979, 1985; Odendaal et al. 1982; Odendaal and Bull 1983; Lemckert and Shine 1993; Williamson and Bull 1995; Lemckert 2001; Wong et al. 2004; Lauck 2005) and into the similarly threatened Crinia tinnula (Letnic and Fox 1997; Meyer et al. 2004; Goldingay and Taylor 2006; Renwick 2006; Lowe et al. 2013; Simpkins 2013; Simpkins et al. 2014). In contrast, there is very limited research on Sloane’s Froglet.
We commenced research into Sloane’s Froglet ecology in 2009. From 2009 to 2011 we investigated the distribution of Sloane’s Froglet as before then it was rarely recorded across its range with only a few recordings from the 1970s until 2009. Surveying during the peak calling period of winter, showed the presence of an extant population in peri-urban areas of south-eastern Australia (Knight 2013). Research was undertaken into habitat use of Sloane’s Froglet presented here. Very little was known about the habitat requirements of Sloane’s Froglet, with the description by Littlejohn (1958) providing the major source of information. He described the habitat as ‘shallow temporary ponds in clay soil’ and ‘temporary ponds in the river valleys’ (Littlejohn 1958, p. 223). Anstis (2002) confirms the use of temporary ponds and adds that they occur in ‘flooded grassland, ditches and swamp areas’ (p. 176).
Knowledge of the habitat used by a species is essential for its management, and in the case of threatened species such as Sloane’s Froglet it may be critical for their conservation. On that basis we asked (a) what are the habitat characteristics of waterbodies occupied by calling Sloane’s Froglets? and (b) which parts of the waterbody do Sloane’s Froglets use during peak calling periods? Given their narrow range and rarity, we hypothesised that Sloane’s Froglet would require specific habitat features and a particular range of waterbody conditions. We predicted that factors influencing waterbody selection would include the following variables: (1) vegetation cover and structure within the waterbody and on the bank; (2) the hydroperiod of the waterbody; (3) the physical characteristics of the waterbody such as slope of the bank and depth, and; (4) the context of the waterbody with nearby waterbodies. We hypothesised that Sloane’s Froglet would exhibit strong habitat selection at the microhabitat level. Many anuran choruses exhibit spatial structuring with the aggregation of males attributed to reducing exposure to predation and increasing the opportunities for attracting females through group calling. Minimum distances within choruses are also often maintained by individual males (Wells 2007). Considering these behaviours, our hypothesis was also that Sloane’s Froglet would exhibit selection based on spatial separation with other frogs using the waterbody.
Materials and methods
Study area
The study area is within the Murray–Darling Basin, Australia. Aboriginal peoples have lived in the Murray–Darling Basin for at least 40,000 years (NSW National Parks and Wildlife Service 2003). The area was first explored by Europeans in 1817 and settlements were established in the 1830s (NSW National Parks and Wildlife Service 2003). The regional towns of Albury–Wodonga and Corowa in southern NSW, with human populations of 89,000 (Australian Bureau of Statistics 2016a) and 5500 (Australian Bureau of Statistics 2016b) respectively, were the centres of the focus areas of this study.
Our surveys focused on waterbodies located within farming, cropping, peri-urban and suburban lands near the Murray River (Fig. 1). The river has a broad floodplain up to 25 km wide and has many anabranches and wetlands (Thoms et al. 2000). The area is characterised as having flat to gently undulating topography with generally low elevations of between 150 and 300 m above sea level (NSW National Parks and Wildlife Service 2003). Within the area wetland habitats have been modified and lost through widespread and large-scale changes in the water regime, loss of floodplain connectivity, and broad-scale changes in land use particularly due to cropping and horticultural activities (Finlayson et al. 2013). Box gum grassy woodland was the predominant vegetation of the study area. It has been highly cleared and fragmented and is listed nationally as endangered, as less than 5% remains, much of it occurring in isolated patches within a predominantly agricultural landscape (Australian Government Department of the Environment and Heritage 2006).
Map showing the location of sampling sites in the Corowa and Albury study area of NSW that were used to compare the habitat characteristics of occupied and unoccupied waterbodies by calling Sloane’s Froglets in 2012 (closed circles) and those used for sampling the microhabitat characteristics of used and unused sites within waterbodies in 2011 (open circles).
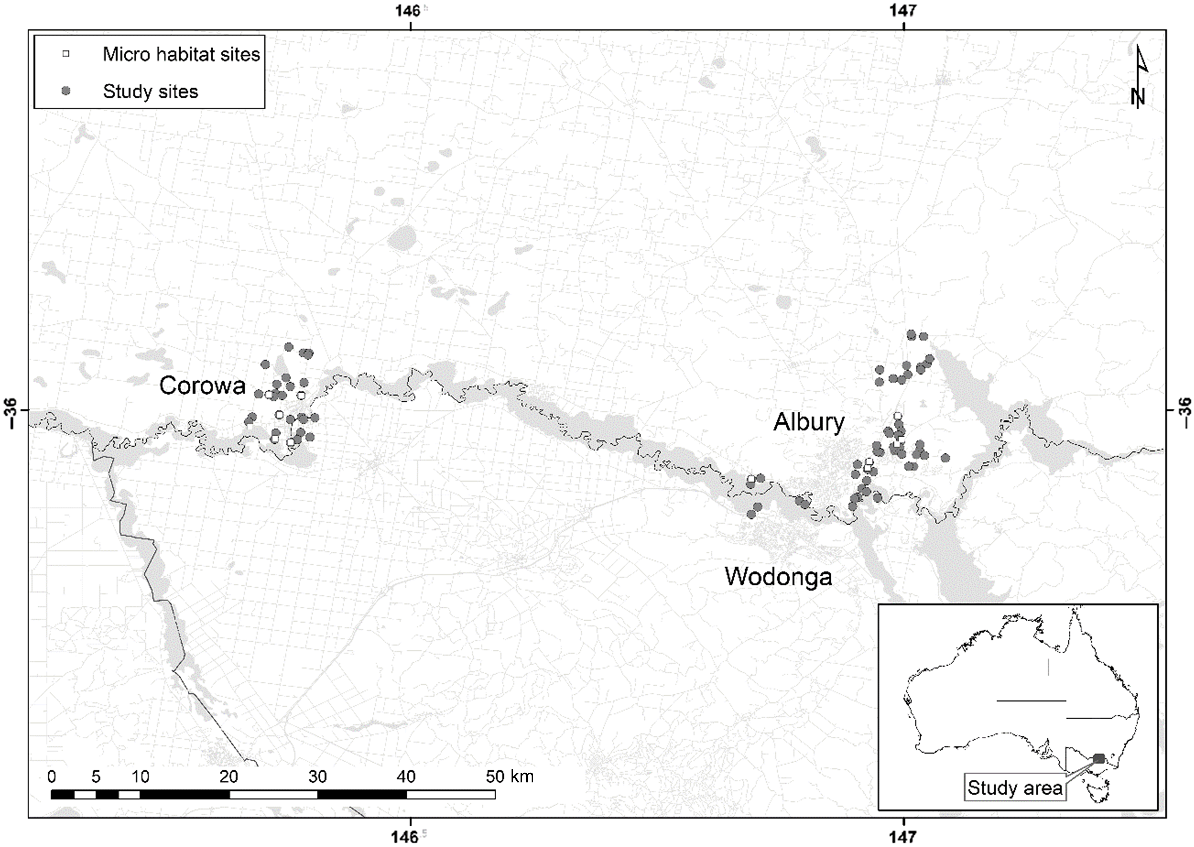
The study area experiences hot summers and mild winters. The mean maximum temperature ranges from 13.0°C in July to 31.2°C in January and mean minimum temperature ranges from 2.7°C in July to 15.5°C in January at Albury. Mean annual rainfall is 715 mm at Albury–Wodonga and 541 mm at Corowa (90 years of records) (Bureau of Meteorology 2015). The survey period followed a major drought and occurred during a La Niña event and associated flooding in south-eastern Australia. During the drought, annual rainfall was 12.4% below the 20th century mean (South Eastern Australian Climate Initiative 2011). Throughout the drought, in comparison with long-term averages, the most obvious shortfalls occurred during the autumn and winter months where higher rainfall generally occurs. In March 2010 the study area experienced a high-rainfall event and flooding occurred (Bureau of Meteorology 2023). During the La Niña event of 2010–2011 south-eastern Australia recorded its fourth highest annual rainfall on record (South Eastern Australian Climate Initiative 2011).
Detection
Where knowledge of detection parameters is limited, managing for detection probabilities in the analyses is important (MacKenzie 2006; Guillera-Arroita et al. 2014). To address the two research questions, we first ascertained the best time of year and time of day to survey for Sloane’s Froglet. We visited sites in winter and spring on non-consecutive nights. At least one site visit to each site occurred during winter. We analysed presence/absence data for three site visits using Program PRESENCE (Hines 2006) to establish a detection probability for the following survey season (MacKenzie et al. 2006). Single season and survey–specific detection probabilities were fitted, and the most parsimonious model selected using Akaike information criterion (AIC) (Burnham and Anderson 1998).
Wetland scale assessment
Presence/absence of Sloane’s Froglet was determined for each of 94 waterbodies using a standard survey approach that involved visiting each site a minimum of three times during winter and early spring from late July to early September 2012. The presence and abundance of calling males was recorded at each visit. If just one calling male was detected at the waterbody on any of the three visits, the waterbody was considered to be ‘occupied’ by Sloane’s Froglet. If no Sloane’s Froglets were heard calling at any of the three visits, the waterbody was considered as unoccupied. Site selection was limited by the proximity of the waterbody to a road, inaccessibility due to wet weather and inability to access landholders to seek permission (no landholders denied permission to undertake the survey work once approached). Limiting the possibility of spatial autocorrelation between nearby waterbodies when modelling occupancy patterns is important as local movements between waterbodies can change occupancy (Wassens et al. 2010). Hamer et al. (2002) found that waterbodies were more likely to be occupied if they were within 50 m of the next occupied waterbody for Litoria aurea. As there was no knowledge of the mobility of Sloane’s Froglet, a conservative approach was undertaken and any waterbody within 100 m of another waterbody was excluded from the study.
The type of waterbody and surrounding land use were recorded. The characteristics of the waterbody that were measured were grouped into two classes: physical features of the waterbody and vegetation within and adjacent to the waterbody. Waterbody physical features included an estimate of the size of the waterbody by taking measurements of length and width using a TruPulse 360B Laser series rangefinder (Welch and MacMahon 2005; Hunter et al. 2009); the hydroperiod (Otto et al. 2007; Wassens et al. 2010) gained from discussion with local landholders; an estimate of the bank slope; waterbody depth measured at the deepest part (Welch and MacMahon 2005; Hunter et al. 2009); the relationship with waterbodies within 100 m (Hamer et al. 2002; Houlahan and Findlay 2003); and an assessment of the size and depth of the overflow and any flooded or flood prone area adjacent to the waterbody (resulting from observations of the site during the preceding distribution studies).
Vegetation characteristics included a measure of the area of the waterbody that contained aquatic vegetation, once again using a TruPulse 360B Laser series rangefinder. Vegetation was categorised into structural categories of submerged (e.g. Potamogeton sp.), floating (e.g. Azolla sp.) and emergent small stem-diameter (e.g. Eleocharis acuta, Juncus usitatus) and large stem-diameter emergent vegetation (e.g. Juncus ingens, Phragmites australis) (Sainty 2003). As very little floating vegetation was found at any waterbody (<1% cover) it was pooled with the submerged vegetation category (Hazell et al. 2001). Each vegetation structure category was then further subdivided into the component of that vegetation type with less than 30% cover (sparse), 30–70% cover (sparse to medium) and greater than 70% cover (medium to dense). Each of the vegetation subcategories that occurred were visually estimated in percent cover for the entire waterbody. A Braun-Blanquet key table (Braun-Blanquet 1928) was used to ensure consistency of cover estimates across waterbodies. The aquatic vegetation was therefore described in such terms as, for example, ‘55% of the waterbody contained sparse cover of submerged vegetation’ or ‘25% of the waterbody contained medium to dense cover of small stem-diameter emergent reeds’.
Characteristics of the bank around each waterbody were measured within 2 m of the water’s edge (Hazell et al. 2004; Wassens et al. 2010). The bank was divided into 5 m areas and percent cover of vegetation, leaf litter and canopy cover estimated in each 2 m × 5 m quadrat using a Braun-Blanquet key table (Braun-Blanquet 1928) and averaged. The number of layers of vegetation was counted within 2 m of the water’s edge.
Microhabitat scale assessment
Microhabitat and relative spatial separation characteristics were sampled at 111 sites within 12 waterbodies in which Sloane’s Froglet was known to occur. Sampling for used calling sites and randomly selected unused sites was limited to a maximum water depth of 60 cm, as pilot work had indicated that Sloane’s Froglets were not found in depths greater than 60 cm.
Calling males were identified via triangulation by two observers. Triangulation is generally recommended when trying to trace a call for positive identification of a species (Department of Environment and Climate Change 2009). Two people are positioned around the calling frog, who are then able to ascertain the location of an individual. In this situation, as Sloane’s Froglet is highly cryptic, small (thus making it very difficult to see), and dives quickly when disturbed, the presence of a frog at a site was determined by identifying the spot it was heard calling from, using triangulation, rather than by necessarily visually observing it. Determining a frog’s position by seeing it may have biased the data towards those sites with habitat assemblages where individual frogs were more easily visible.
Microhabitat variables were measured within 1 m2 quadrats. The 1 m2 quadrat was placed around the calling position of each individual frog, with the estimated position of the frog at the centre of the quadrat. This 1 m2 area is referred to as a ‘site’. Assessing microhabitat in 1 m2 quadrats is consistent with other studies (Hollis 2004; Gorman and Haas 2011). The quadrat at sites where each individual Sloane’s Froglet was present is referred to as a ‘used site’. On the same night that the Sloane’s Froglet microhabitat was recorded, variables were also measured for randomly chosen ‘unused’ sites within each waterbody. In total 57 ‘unused’ sites were sampled across the 12 waterbodies.
Three types of variables were measured in each ‘used’ and ‘unused’ 1 m2 quadrat: physical characteristics including water depth and distance to bank; structural vegetation characteristics; and distances to the nearest other Sloane’s Froglet calling and to the nearest frog of another species calling (relative spatial separation). Measurements of the distance between the middle of the quadrats and other calling frogs were recorded as pond-breeding behaviours may influence spatial positioning (Mac Nally 1985; Wells 2007).
Water depth was measured in cm using a metric ruler in the centre and at each corner of each quadrat, and was averaged. The distance from the centre of the quadrat to the bank was measured with a metric tape in metres. The percentage of the quadrat that was above the water level (on the bank or in the littoral zone) was estimated where the quadrat placement covered both bank and aquatic areas of the waterbody.
Vegetation was categorised into functional categories of submerged and emergent vegetation as with the wetland scale assessment. Each of the vegetation categories that occurred in the quadrat were estimated in percent cover. A Braun-Blanquet key table (Braun-Blanquet 1928) was used to ensure consistency of cover estimates across sites. The percent cover was also estimated for the area where there was bare substrate (not obscured by vegetation or leaf litter) and the percentage of the quadrat where there was leaf litter visible on the bottom. In addition, the percent cover was recorded for any area within the quadrat and above the water level that was bare of vegetation. The percent cover of the canopy directly above the quadrat was estimated and recorded. For emergent vegetation the minimum and maximum height above the surface of the water was measured with a metric ruler in centimetres. As well as the structural characteristics of the vegetation, the dominant vegetation species present within each quadrat were recorded.
Three variables were measured at each quadrat to assess relative spatial separation in the waterbody. These variables were distance to another Sloane’s Froglet calling at that waterbody, and distance to a frog of another species calling at that waterbody (measured in metres using a tape), as well as the species of the nearest other frog calling (identified by call).
Statistical analysis
Models of both the waterbody characteristics and the microhabitat were developed to discriminate between occupied/used and unoccupied/unused sites using generalised linear modelling (GLM) and the information-theoretic approach (Burnham and Anderson 1998). The ‘all possible’ or ‘best-subsets’ routine was used as it is considered a powerful tool that conserves the principles of parsimony and is now widely available in most computer packages (King 2003). Modelling was undertaken using S-PLUS Ver. 8.2 (Tibco Software Inc., 2010).
The data were assessed for potential multicollinearity by generating a correlation matrix using Spearman’s Rank Correlation Coefficients (r). Variables with strong correlations (r > 0.7), indicating pseudo replication, were excluded from further analysis (Tabachnick and Fidell 2013). Variables showing very limited or no variability were also excluded from the modelling process.
In acknowledgement that several models may make good predictions (e.g. Van Sickle et al. 2006; Claeskens and Hjort 2008), Akaike Information Criterion (AIC) was used to identify the most parsimonious models. AIC is calculated as:
where k is the number of model parameters and RSS is the Residual Sum of Squares and is used to select between multivariate models where (k < 1).
The second order derivative AICc was used to select between models for discussion as it contains a bias correction term for small sample size, and is recommended by Burnham and Anderson (2004) for use in practice because it converges to AIC as n gets large. Models with an AICc score that is within two of the AICc score for the first model were selected for discussion in the first instance.
Akaike weights (ωi) were also calculated as these can be interpreted as ‘the probability that any given model is the best model for the observed data in the suite of candidate models’ (Johnson and Omland 2004), and as such ωi provides a measure of the strength of evidence for each model. Akaike weights can also be used to indicate the relative importance of predictor variables (Johnson and Omland 2004) and so the sum of the Akaike weights over all of the models in which the parameter appears was calculated.
Results
Detection
Sloane’s Froglet commenced calling in early to mid-April and continued to call into August, September and early October throughout the study period of 2010–2014. Sloane’s Froglet consistently called during the day as well as into the evening in winter and early spring. Detection probabilities analysed for the 2012 data collection period for the presence/absence data show there is a very high confidence that waterbodies identified as unoccupied were not falsely identified, and were in fact unoccupied. The AIC (Burnham and Anderson 1998) suggested that survey-specific detection probability for Sloane’s Froglet was a better fit for the data than using a constant detection probability across surveys (Table 1). The survey-specific detection probability revealed there was a 0.17% chance of a waterbody being classified falsely as unoccupied. As the detection probability analysis showed that the probability of detecting a false absence was extremely low, it was not necessary to consider detectability in subsequent analyses exploring habitat associations.
AIC | Naive occupancy estimate | Detection probability | Cumulative likelihood of reporting a false absence (1 − P1) × (1 − P2) × (1 − P3) | ||
---|---|---|---|---|---|
Survey – specific | 262.99 | 0.5745 | P1 = 0.6101 P2 = 0.8874 P3 = 0.9613 | 0.0016 (0.17%) | |
Constant | 283.82 | 0.5745 | 0.8158 |
Waterbody features
Sloane’s Froglets were present in waterbodies with varying characteristics (Table 2). Sloane’s Froglets occurred in waterbodies with gentle, moderate and steeply sloping banks but showed a preference for waterbodies with gently sloping banks (P = 0.001). Sloane’s Froglets occurred in waterbodies with ephemeral, seasonal and permanent hydroperiods. Waterbodies that were isolated and those that were on a watercourse (for instance a dam or a creek) as well as waterbodies that were part of larger complex lentic systems were occupied by Sloane’s Froglets. Sloane’s Froglets occupied waterbodies that were either shallow, of medium depth or deep. They also occupied waterbodies where the water spilled over and flowed directly away from the waterbody. However, they showed a preference for waterbodies where water pooled in adjacent shallow ephemeral overflows. Sloane’s Froglets occurred in both constructed and ‘natural’ waterbodies. Of the 54 occupied waterbodies surveyed, 41% were classified as ‘natural’, that is, they were natural open wetlands, natural depressions or gilgais. Constructed waterbodies (farm dams, constructed wetlands including stormwater and grey water ponds and drains) were actively used for breeding. The only two instances where Sloane’s Froglets were located in amplexus were in constructed waterbodies – once in a constructed grey water treatment wetland and the second time in a dam on a watercourse.
Waterbody characteristic | Occupied waterbodies (%) n = 54 | Unoccupied waterbodies (%) n = 40 | ||
---|---|---|---|---|
Bank slope | Gentle | 72 | 25 | |
Moderate | 20 | 55 | ||
Steep | 8 | 20 | ||
Waterbodies within 50 m | Isolated | 35 | 40 | |
On a watercourse | 21 | 22 | ||
Part of a complex | 44 | 38 | ||
Hydroperiod | Ephemeral | 24 | 20 | |
Seasonal | 37 | 35 | ||
Permanent | 39 | 45 | ||
Waterdepth | Shallow | 39 | 15 | |
Moderate | 48 | 67 | ||
Deep | 13 | 18 | ||
Presence of an ephemeral adjacent shallow overflow | Present | 67 | 73 | |
Absent | 33 | 27 | ||
Waterbody type | Constructed | 59 | 75 | |
Natural | 41 | 25 |
Waterbodies were considered ‘occupied’ if Sloane’s Froglet was present at any of a minimum of three visits, and ‘unoccupied’ if Sloane’s Froglet was not detected during any of the visits.
Wetland scale modelling
Seven variables were included in the modelling process (Table 3).
Variable abbreviation | Definition | |
---|---|---|
Percent cover of emergent vegetation | The total percent cover of the waterbody with small stem-diameter emergent vegetation | |
Percent cover of submerged vegetation | The total percent cover of the waterbody with any completely submerged vegetation | |
Slope of the bank | The slope of the waterbody bank, gentle, moderate, steep | |
Water depth | The depth of the water in the waterbody, shallow, moderate or deep | |
Overflow | The presence or absence of an adjacent ephemeral shallow overflow | |
Bank vegetation | The number of layers of vegetation on the bank | |
Bank bare ground | The percent of the bank that was bare of vegetation |
By using GLM and the information-theoretic approach (Burnham and Anderson 1998) we identified four models with an AICc difference of <2 which are explanatory of waterbody selection by Sloane’s Froglet. The percent of the waterbody with small stem-diameter emergent vegetation was included in all four models as was the presence or absence of an adjacent ephemeral shallow overflow. The percent of the bank that was bare of vegetation was present in three of the four models, and the slope of the bank and the number of layers of vegetation on the bank in two of the models.
Assessing the relative importance of the individual models established that the presence/absence of an adjacent ephemeral shallow overflow had the highest summed Akaike weight (Σ ωi = 0.8642), followed by the percent cover of emergent small stem-diameter vegetation (Σ ωi = 0.8088), and the percent cover of the bank bare of vegetation (Σ ωi = 0.8007), while the slope of the bank, the number of layers of vegetation on the bank, the percent cover of submerged vegetation and the water depth had lower Akaike weights. The model selected for further detailed investigation contained only the three variables with the highest summed Akaike weights of percent cover of small stem-diameter emergent vegetation, the presence or absence of an overflow and the percent cover of bare ground. While not having the highest overall AICc, the model contained the lowest number of variables and exhibited the highest percent correctly predicted classification table of the top four models. The beta values and odds ratio for these three variables (Table 4) show that Sloane’s Froglet is 450 times more likely to occupy the waterbody when an adjacent ephemeral shallow overflow is present, and that Sloane’s Froglet is more likely to be present with greater cover of small stem-diameter emergent vegetation, and less bare ground on the bank. A combination of these variables was the best explanation for the selection of waterbodies by calling male Sloane’s Froglets (Fig. 2).
Variable | Beta value | s.e. | Significance | Odds ratio | |
---|---|---|---|---|---|
Overflow | 1.503 | 0.515 | 0.004 | 4.494 | |
Percent cover of emergent vegetation | 0.032 | 0.012 | 0.008 | 1.032 | |
Bare ground on the bank | −0.021 | 0.011 | 0.054 | 0.980 | |
Constant | −0.596 | 0.436 | 0.171 | 0.551 |
Fitted curves for the relationship between expected occupancy and the two significant habitat variables ((a) Percent cover of emergent small stem diameter vegetation and (b) Percent of bareground on the bank of the waterbody) identified in the GLM (Presence of an adjacent ephemeral overflow held at 1 and 0). Dotted lines demark upper and lower confidence intervals. (c) Bar chart for the expected occupancy with upper and lower confidence intervals for the binomial factor ‘Presence of an adjacent empheral’ overflow identified in the GLM (other variables held at their means).
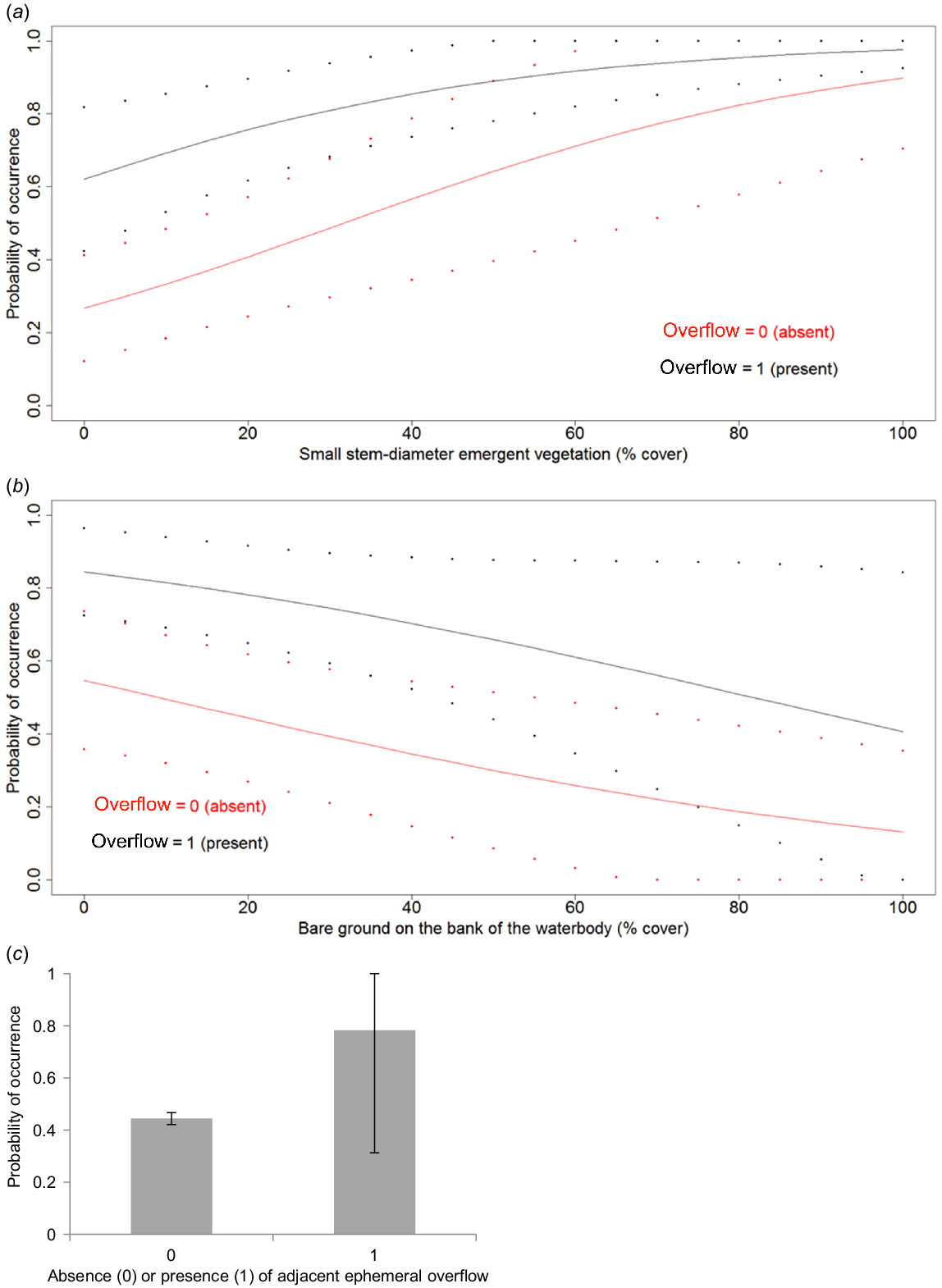
Microhabitat scale features
All Sloane’s Froglets detected in this survey were calling from within a waterbody and not on the surrounding bank or riparian area. Sloane’s Froglets were located in those parts of the waterbody with very shallow water having a median water depth of 8 cm (range 0–32 cm). The median water depth was significantly shallower at used sites than the randomly selected unused sites (P = 0.013). Vegetation at the wetlands consisted predominantly of low reeds such as E. acuta and inundated grasses including Paspalum cynodon and Paspalum distichum. Submerged water plants such as Potamogeton spp. (pondweeds) as well floating vegetation such as Azolla sp. occurred less frequently. For nine of the sites that Sloane’s Froglet used there was no frog of another species calling (i.e. Sloane’s Froglet was the only species of frog calling at that waterbody at the time of sampling). At 30 of the remaining 45 sites the closest other frog calling was a Crinia parinsignifera. At the remaining 15 sites the nearest neighbour was Litoria paraewingi at one site, C. signifera at nine sites and Limnodynastes tasmaniensis at five sites.
Microhabitat scale assessment
Preparation of the data for modelling resulted in six variables being included in the modelling process for sites used or unused by Sloane’s Froglet (Table 5).
Variable | Definition | |
---|---|---|
Water depth | The average depth of the water within the 1 m2 quadrat | |
Area of quadrat above bank | The percent of the quadrat that was on the bank above the water level | |
Percent cover of submerged vegetation | The total percent cover of any completely submerged vegetation | |
Height of emergent reeds | The maximum height of any emergent reeds in the quadrat | |
Distance to Sloane’s Froglet | The distance to another Sloane’s Froglet calling at the time of sampling | |
Distance to other frog | The distance to a frog of any other species calling at the time of sampling |
Two models with an AIC difference of <2 that are explanatory of site selection by Sloane’s Froglet were identified. The average depth of the water, the percent of the quadrat that was on the bank above the water level, the distance to another calling Sloane’s Froglet and the distance to another calling frog of another species were present in each of the two top ranked models, while the percent cover of submerged vegetation occurred only in the second model.
The distance to another calling Sloane’s Froglet and another calling frog of another species had the highest summed Akaike weights (Σ ωi = 0.9999), followed by the area of the quadrat above the bank (Σ ωi = 0.8118), and the average water depth (Σ ωi = 0.7742), while the two vegetation categories, the cover of submerged vegetation and the maximum height of emergent vegetation were of less importance.
The model selected for further detailed investigation contained only the four variables with the highest summed Akaike weights of average water depth, the percent of the quadrat that was on the bank above the water level, the distance to another Sloane’s Froglet calling at the time of sampling and the distance to a frog of another species calling at the time of sampling. This model had the highest overall AICc, as well as containing the lowest number of variables and it exhibited the highest percent correctly predicted classification table of the top two models.
The beta values and odds ratio for these four variables (Table 6) and the probability plots (Fig. 3) show that Sloane’s Froglet is more likely to occupy sites within a waterbody with decreasing distances to another calling Sloane’s Froglet and to another species of calling frog, as well as having less area of the site occurring above the water level and with shallower water depths.
Variable | Beta value | s.e. | Significance | Odds ratio | |
---|---|---|---|---|---|
Water depth | −0.064 | 0.030 | 0.035 | 0.938 | |
Area of quadrat above bank | −0.102 | 0.056 | 0.069 | 0.903 | |
Distance to Sloane’s Froglet | −0.235 | 0.078 | 0.003 | 0.791 | |
Distance to other frog | −0.142 | 0.064 | 0.027 | 0.867 | |
Constant | 2.985 | 0.698 | 0.000 | 19.796 |
Fitted curves for the relationship between expected occupancy and the four significant variables (a) average depth of the water, (b) Distance to another calling male Sloane’s Froglet, (c) distance to another calling male frog of another species and (d) Percent of the calling site above the water level, identified in the GLM for Sloane’s Froglet microhabitat study undertaken in the Albury and Corowa regions in 2011. Dotted lines demark upper and lower confidence intervals.
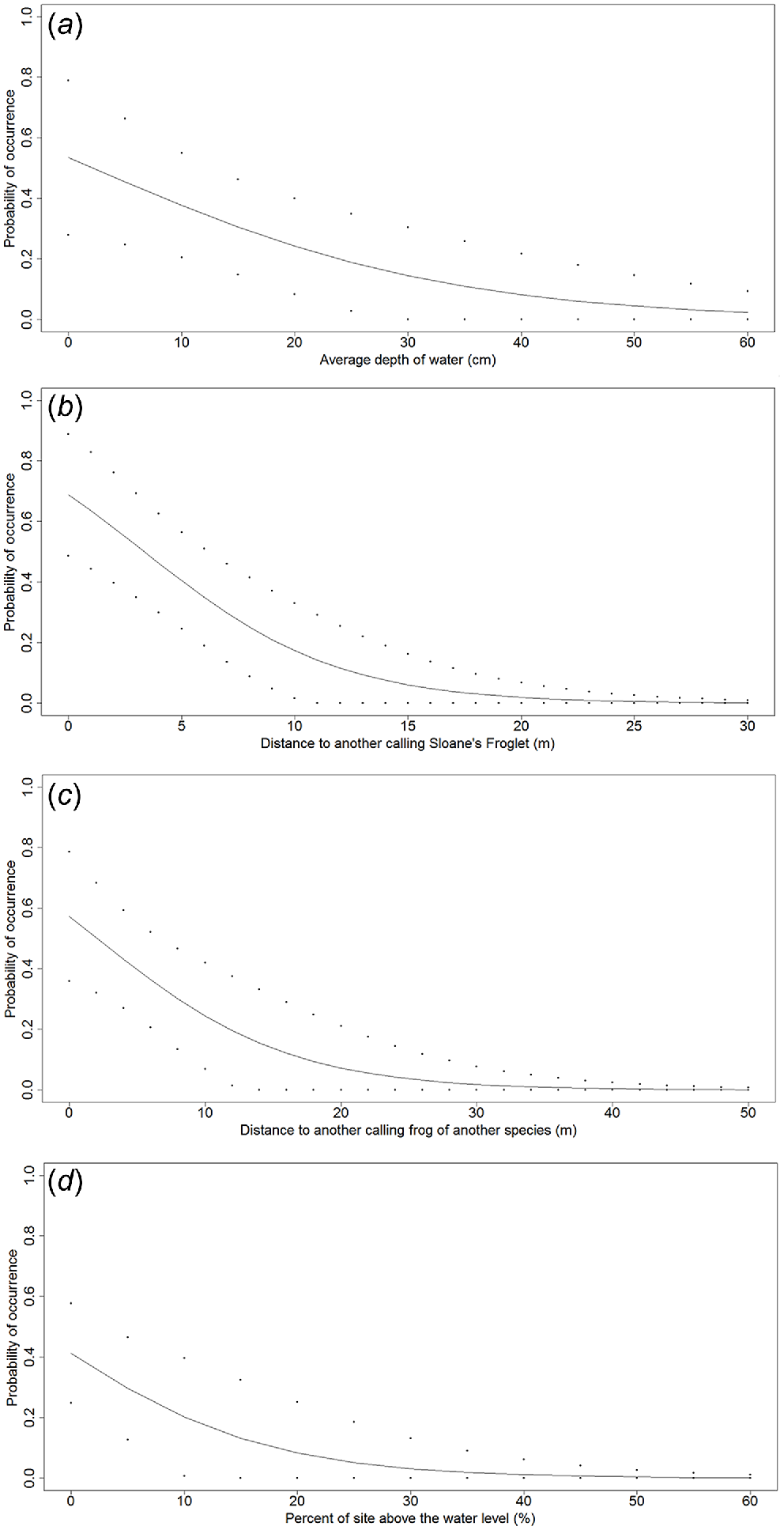
Discussion
Sloane’s Froglets surveyed in this study occupied a wide range of waterbody types, both natural and constructed, suggesting that Sloane’s Froglet is more of a generalist than previously hypothesised. However, further analysis revealed that both niche and behavioural characteristics, particularly spatial separation, influenced their use of waterbodies. While the waterbody scale assessment suggested that Sloane’s Froglet is a generalist, the microhabitat assessment refined this picture. In peak breeding season Sloane’s Froglets were using only specific parts of waterbodies. Gently sloping banks, together with emergent small-stem diameter vegetation, a bank area vegetated with understorey species and the presence of an adjacent ephemerally inundated overflow, all influenced the use of waterbodies by Sloane’s Froglet.
Wetlands within the study region have been affected by the landscape scale changes in wetland health, hydrology and floodplain connectivity prevalent across the Murray–Darling Basin (Finlayson et al. 2013); loss, fragmentation and modification of habitat widespread throughout the box gum grassy woodlands in eastern Australia (Lindenmayer et al. 2005); and through the development of peri-urban, industrial and suburban areas currently occurring on previous agricultural and environmental land. As the study occurred in such a massively altered landscape (Mac Nally et al. 2009), there is the potential that sub-optimal habitat for this species is being described (Hazell 2003).
Detection
The choice of mid-winter surveys was vindicated by the detection probability analysis undertaken for this study. The survey-specific detection probability revealed that the probability of detecting Sloane’s Froglet at least once, if it was present on all three surveys, was 0.998, and so there was a very low chance of falsely recording the species as absent from the site. This suggests that a standard survey method founded upon a minimum of three site visits during the afternoon and evening of the peak calling season is adequate to detect Sloane’s Froglet by call for an experienced observer. This finding is consistent with the findings of MacKenzie and Royle (2005) who state that ‘sampling units should be surveyed a minimum of three times when detection probability is high (>0.5 survey−1)’ (p. 1105). A note of caution is that inexperienced surveyors may have difficulty distinguishing Sloane’s Froglet from Plains Froglet (C. parinsignifera), especially when the call pitch changes in late spring and summer.
Advice on detection based on our recommendations is now available in Anstis (2013) and in State of NSW and Department of Planning, Industry and Environment (2020). In following this advice, surveyors should note that this analysis was based on non-consecutive survey nights with at least one, and most often two or three surveys undertaken in the winter months. Surveys undertaken on three consecutive nights and outside of key calling periods may result in false identification of absent sites.
Wetland scale assessment
In this study Sloane’s Froglets occupied ephemeral, seasonal and permanent wetlands with a range of water depths. The context of the waterbody was also variable, as some were isolated and others were part of complex wetland systems, incorporating dams, drains, natural watercourses and overflows. Our research, while confirming Littlejohn’s (1958) and Anstis’ (2013) observations of habitat, shows that Sloane’s Froglets also use other types of waterbodies that are moderately to relatively deep and with seasonal and permanent hydroperiods. Like the sympatric C. signifera that breeds in ‘temporary to semi-permanent flooded ditches, streams, grassland, or permanent ponds and dams’ (Anstis 2013, p. 576) and C. parinsignifera which is associated with ‘ponds or swamps or flooded grassland’ (Anstis 2013, p. 563), Sloane’s Froglet appears to occupy a variety of sites.
Two physical characteristics were significantly different between waterbodies occupied and unoccupied by Sloane’s Froglet: the slope of the bank, and the presence or absence of an adjacent ephemerally inundated shallow overflow. There was a correlation between gently sloping banks and the presence of Sloane’s Froglet. Gently sloping banks may provide larger areas of shallow water in a waterbody and also provide an area where small stem-diameter vegetation that responds to both inundation and drying periods is able to establish (Roberts and Marston 2011; Webb et al. 2012). The presence of ephemerally inundated shallow overflows adjacent to the waterbody which Sloane’s Froglet was located in is of interest. In periods of heavy rain when these areas were flooded, Sloane’s Froglets actively called from them. However the period of time that these areas retained water is expected to be short, and the duration Sloane’s Froglet stay in them (and their use of these areas) have not been studied. These areas may be analogous with the rain-fed wetlands examined by Wassens et al. (2013) in their study of tadpole response to rainfall events. Shallow ephemeral overflows may also exhibit similarities with the well-studied vernal ponds of the northern hemisphere (Paton and Crouch 2002; Gamble and Mitsch 2009; Green et al. 2013; Calhoun et al. 2014a, 2014b) that provide seasonal opportunities for amphibian breeding. Further research into the relevance of ephemeral shallow overflows for Sloane’s Froglet lifecycle is required.
In addition to using a variety of natural wetland types, Sloane’s Froglets occupy farm dams, roadside drains, irrigation drains, stormwater retention ponds and other constructed wetlands where appropriate habitat is present. The use of constructed and ‘natural’ waterbodies by Sloane’s Froglet is relevant to the context of its distribution in highly modified landscapes. Sloane’s Froglets were observed not only calling in constructed waterbodies, but also in amplexus and laying eggs. Many international studies have investigated the use of constructed waterbodies by anurans (Simon et al. 2009; Shulse et al. 2010; Denton and Richter 2013). Constructed waterbodies continue to provide important habitat for many frog species across south-east Australia in both agricultural (Hazell et al. 2001) and urban environments (Sievers et al. 2019). In this study there were several stormwater ponds occupied by Sloane’s Froglets. It seems likely that the use of stormwater ponds by Sloane’s Froglet is dependent on suitable habitat characteristics (such as the presence of emergent vegetation) which may also be correlated with untested variables of stormwater ponds such as age and substrate. The use of stormwater retention ponds by amphibians has also been investigated both internationally (Scheffers and Paszkowski 2013) and in the Australian context (Hamer et al. 2012; Sievers et al. 2018, 2019). In these cases, the habitat quality of stormwater ponds remains in question, with concerns that constructed ponds may be providing limited or poor-quality habitat compared with natural ponds (Scheffers and Paszkowski 2013) and that they may in the longer term be ecological traps (Sievers et al. 2018).
In the case of Sloane’s Froglet, it is highly likely that frogs use roadside and irrigation ditches and drains with appropriate vegetation for breeding as well as refuge, foraging and dispersal. Male froglets actively called from drains during peak breeding times. Few studies focus on the use of drains as anuran breeding sites. Mazerolle (2005) investigated the use of constructed ditches by pond breeding amphibians. He found that many individuals occurred in ditches and survival in these structures was high. He found that no breeding occurred in the structures as calling males, eggs and tadpoles were not detected (2005, p. 584). Rather, Mazerolle concluded that ditches provide temporary habitat for individuals and function as corridors as they move around the landscape.
Sloane’s Froglets occupied waterbodies with significantly greater percent cover of in-waterbody vegetation, particularly small stem-diameter emergent vegetation, than that in unoccupied waterbodies. Small stem-diameter emergent vegetation typically grows in shallow water and its presence may reflect a range of hydrological conditions, such as water depth and stability of water levels as well as productivity (Casanova and Brock 2000; Barrett et al. 2010). The presence of ground-storey vegetation on the bank at sites with greater covers of emergent vegetation is likely to represent a continuity of habitat across the land–water interface. Aquatic and fringing vegetation characteristics have been shown to be important both for individual species and in relation to frog diversity (Lane et al. 2007; Popescu et al. 2013; Scheffers and Paszkowski 2013). Several Australian studies have discussed the importance of vegetation structure, particularly at the land–water interface for pond-dwelling species (Healey et al. 1997; Hazell et al. 2004).
This study focussed on the habitat characteristics of waterbodies occupied by Sloane’s Froglet during peak calling periods, however aquatic vegetation structure can influence multiple life-history stages of frogs (Egan and Paton 2004; Purrenhage and Boone 2009; Scheffers and Paszkowski 2013). The presence of small-stem diameter emergent vegetation may be useful for Sloane’s Froglet activities throughout its complex life cycle including for ovipositioning, foraging, metamorph growth, and predator avoidance by tadpoles, metamorphs and adults. For instance, the Anstis (2013) observation that Sloane’s Froglet eggs are ‘laid singly, mostly attached to submerged vegetation’ (p. 580) accord with our observation that eggs are laid on submerged stems of E. acuta or other small-stem diameter grass and reed species. While Sloane’s Froglet tadpoles are unable to be distinguished from those of the sympatric C. parinsignifera (Anstis 2002), it is likely that they feed on ‘fine particles and algae in sediments and on vegetation’ (Anstis 2013, p. 582) once again reinforcing the need for aquatic vegetation at different life stages.
Microhabitat scale assessment
All calling male Sloane’s Froglets in this study were located within the waterbody and not on the edge or bank of the waterbody. Those that were located visually were found to be floating or gripping small pieces of vegetation on the water surface. Throughout the study period Sloane’s Froglets were infrequently observed out of water, only when moving from one place to another and when calling from the floor of an empty pond prior to rain. Mac Nally (1979, 1985) in his studies of the sympatric and related C. parinsignifera and signifera found that their preferred calling stations were located on land (1985, p. 335).
The depth of the water in which Sloane’s Froglets were floating or sitting was a significant variable for site selection. All males in the microhabitat study were calling from very shallow water. At times, the shallowness of the water reflected the nature of the entire waterbody. However, several of the waterbodies in the microhabitat study had deeper areas of water that were not used by Sloane’s Froglet. Shallow water warms quickly and stays warmer than surrounding air temperatures after dark. For a small frog such as Sloane’s Froglet that is using energy for breeding at the coldest time of the year, shallow water provides important opportunities for thermoregulation. Warmer body temperatures may enhance the ability to breed. For instance, there have been many studies into the relationship between body temperature and calling energetics (Lemckert and Shine 1993; Wells et al. 1996). Shallow water also often contains littoral wetland vegetation such as inundated grasses and reeds suitable for ovipositioning, foraging and tadpole shelter (Casanova and Brock 2000).
Sloane’s Froglets were found to cluster or aggregate in the sampled waterbodies. Recent microhabitat studies of pond-breeding frogs tend to focus on vegetation or other habitat characteristics rather than relationships with positions of other individual frogs (Gillespie et al. 2004; Gorman and Haas 2011; Popescu et al. 2013). For Sloane’s Froglet, relative spatial separation was a significant component influencing site use. Wells (2007) points out that most anuran choruses show spatial structuring. Generally, spatial structuring is attributed to male gatherings to compete during peak calling periods (Wells 2007), or the positioning of males at sites suitable for female oviposition (Gillespie et al. 2004; Hollis 2004). Mac Nally (1979, 1985) in his research on the two Crinia spp. sympatric with Sloane’s Froglet discusses how territories are maintained between both conspecific and heterospecific individuals when the sites are largely homogenous environments. He asserts that ‘behaviour primarily determines spacing, rather than topography or vegetation patterns’ (Mac Nally 1979, p. 301). Mac Nally (1985) further points out that habitat preferences or selectivities are not the only driver allowing different species to coexist. Other factors include predation, physiological tolerances and interspecific interaction.
Conclusions
The wetlands surveyed in this study occurred in southern NSW in box gum grassy woodlands in Australia’s Murray–Darling Basin. This landscape, well documented as over-cleared (Australian Government Department of the Environment and Heritage 2006), also has modified water availability and connectivity (Finlayson et al. 2013). Adding to the pressures on Sloane’s Froglet is that the extant population occurs predominantly in peri-urban areas that are currently undergoing more intensive settlement and urbanisation. It is likely that the documented decline of Sloane’s Froglet is a real reflection of the condition of the population and that the decline is related to landscape-scale threats. Habitat protection and construction will play an important role in conserving this endangered species.
We chose to focus on the habitat used during the breeding period (Knight and Allan 2021), as management of breeding habitat is a core requirement for the ongoing survival of a threatened species (Lind et al. 1996; Ferreira and Beja 2013; Thomas et al. 2014). Habitat variables for the waterbodies surveyed in this study were collected in one season only, in the peak calling period, winter and early spring. There is currently no information on the refuges Sloane’s Froglets use during dry periods. The use of clay cracks may be common for some species (Wassens et al. 2008), others use leaf litter and coarse woody debris (Heard et al. 2008) or deep refuge ponds.
Many of the areas where Sloane’s Froglet occurred were part of complex wetland systems that include multiple ponds of varying depths as well as drains and creek overflows. It is well established that frogs with their complex life cycles depend on the linking of complementary habitats at multiple spatial scales and that populations are structured as patchy networks or metapopulations (Pope et al. 2000; Marsh and Trenham 2001; Hale et al. 2013). As such, connections across the landscape and between waterbodies are just as important for frog survival as the characteristics of the waterbodies used for breeding (Cushman 2006; Cushman et al. 2013). Even when the classical interpretation of metapopulation theory (local extinction and recolonisation) is not applied to frog populations, frogs have been shown to depend on the spatial arrangement of ponds as a best recruitment model (Marsh et al. 1999).
Preliminary findings from this study, prior to the detailed modelling reported here, were published in popular format as Sloane’s Froglet interim habitat guide and management recommendations (Knight 2014), a publication distributed to developers and local government agencies where development is likely to impact upon Sloane’s Froglet habitat. The research presented here provides foundational information regarding the habitats used by this small and little-known endangered species within a context of immediate threat of habitat loss. The focus of the study is on habitat requirements due to the species’ occurrence in areas which continue to be under pressure from urban development. As Sloane’s Froglet has been identified as one of the 26 most threatened species in Australia (Geyle et al. 2022), it is hoped that the management and ongoing conservation of their populations and habitats can be further informed by the detailed information presented here.
Data availability
The data that supports this study will be shared upon reasonable request to the corresponding author.
Declaration of funding
The work was undertaken as part of a postgraduate project at Charles Sturt University and funded through an APA scholarship. This project was partially funded by the Albury Conservation Company.
Acknowledgements
Dr David Hunter provided invaluable input and guidance for the research. Dr Wayne Robinson assisted with detection statistics. Fieldwork was undertaken with the help of Cassie Douglas, Beryl Derwent, James Maguire, Kate Hill, Marion Anstis, and Joanne Diver. This paper includes part of the research undertaken for the PhD thesis of Alexandra Knight (2015).
References
Australian Bureau of Statistics (2016a) 2016 Census QuickStats Albury-Wodonga. Available at https://www.abs.gov.au/census/find-census-data/quickstats/2016/1001#:~:text=In%20the%202016%20Census%2C%20there,up%202.7%25%20of%20the%20population.&text=The%20median%20age%20of%20people%20in%20Albury%20%2D%20Wodonga%20was%2038%20years [accessed 1 January 2022]
Australian Bureau of Statistics (2016b) 2016 Census QuickStats Corowa. Available at https://www.abs.gov.au/census/find-census-data/quickstats/2016/SSC11080#:~:text=In%20the%202016%20Census%2C%20there,up%201.8%25%20of%20the%20population.&text=The%20median%20age%20of%20people%20in%20Corowa%20was%2049%20years [accessed 1 January 2022]
Barrett R, Nielsen DL, Croome R (2010) Associations between the plant communities of floodplain wetlands, water regime and wetland type. River Research and Applications 26, 866-876.
| Crossref | Google Scholar |
Bureau of Meteorology (2015) Climate statistics for Australian locations. Monthly Climate Statistics. Available at Http://Www.Bom.Gov.Au/Climate/Averages/Tables/Cw_074034.Shtml [accessed 11 February 2015]
Bureau of Meteorology (2023) Australia in March 2010. Available at http://www.bom.gov.au/climate/current/month/aus/archive/201003.summary.shtml [accessed 29 November 2023]
Burnham KP, Anderson DR (2004) Multimodel inference: understanding AIC and BIC in model selection. Sociological Methods & Research 33, 261-304.
| Crossref | Google Scholar |
Calhoun AJK, Arrigoni J, Brooks RP, Hunter ML, Richter SC (2014a) Creating successful vernal pools: a literature review and advice for practitioners. Wetlands 34, 1027-1038.
| Crossref | Google Scholar |
Calhoun AJK, Jansujwicz JS, Bell KP, Hunter ML, Jr. (2014b) Improving management of small natural features on private lands by negotiating the science-policy boundary for Maine vernal pools. Proceedings of the National Academy of Sciences 111, 11002-11006.
| Crossref | Google Scholar |
Casanova MT, Brock MA (2000) How do depth, duration and frequency of flooding influence the establishment of wetland plant communities? Plant Ecology 147, 237-250.
| Crossref | Google Scholar |
Cushman SA (2006) Effects of habitat loss and fragmentation on amphibians: a review and prospectus. Biological Conservation 128, 231-240.
| Crossref | Google Scholar |
Cushman SA, Landguth EL, Flather CH (2013) Evaluating population connectivity for species of conservation concern in the American Great Plains. Biodiversity and Conservation 22, 2583-2605.
| Crossref | Google Scholar |
Denton RD, Richter SC (2013) Amphibian communities in natural and constructed ridge top wetlands with implications for wetland construction. The Journal of Wildlife Management 77, 886-896.
| Crossref | Google Scholar |
Egan RS, Paton PWC (2004) Within-pond parameters affecting oviposition by wood frogs and spotted salamanders. Wetlands 24, 1-13.
| Crossref | Google Scholar |
Ferreira M, Beja P (2013) Mediterranean amphibians and the loss of temporary ponds: are there alternative breeding habitats? Biological Conservation 165, 179-186.
| Crossref | Google Scholar |
Finlayson CM, Davis JA, Gell PA, Kingsford RT, Parton KA (2013) The status of wetlands and the predicted effects of global climate change: the situation in Australia. Aquatic Sciences 75, 73-93.
| Crossref | Google Scholar |
Frost DR (2023) Amphibian species of the world: an online reference. Version 6.1. American Museum of Natural History, New York, New York. Available at https://amphibiansoftheworld.amnh.org/ [accessed 29 November 2023]
Gamble DL, Mitsch WJ (2009) Hydroperiods of created and natural vernal pools in central Ohio: a comparison of depth and duration of inundation. Wetlands Ecology and Management 17, 385-395.
| Crossref | Google Scholar |
Gardner TA, Barlow J, Peres CA (2007) Paradox, presumption and pitfalls in conservation biology: the importance of habitat change for amphibians and reptiles. Biological Conservation 138, 166-179.
| Crossref | Google Scholar |
Geyle HM, Hoskin CJ, Bower DS, Catullo R, Clulow S, Driessen M, Daniels K, Garnett ST, Gilbert D, Heard GW, Hero J-M, Hines HB, Hoffmann EP, Hollis G, Hunter DA, Lemckert F, Mahony M, Marantelli G, McDonald KR, Mitchell NJ, Newell D, Roberts JD, Scheele BC, Scroggie M, Vanderduys E, Wassens S, West M, Woinarski JCZ, Gillespie GR (2022) Red hot frogs: identifying the Australian frogs most at risk of extinction. Pacific Conservation Biology 28, 211-223.
| Crossref | Google Scholar |
Gillespie GR, Lockie D, Scroggie MP, Iskandar DT (2004) Habitat use by stream-breeding frogs in south-east Sulawesi, with some preliminary observations on community organization. Journal of Tropical Ecology 20, 439-448.
| Crossref | Google Scholar |
Gillespie GR, Roberts JD, Hunter D, Hoskin CJ, Alford RA, Heard GW, Hines H, Lemckert F, Newell D, Scheele BC (2020) Status and priority conservation actions for Australian frog species. Biological Conservation 247, 108543.
| Crossref | Google Scholar |
Goldingay R, Taylor B (2006) How many frogs are killed on a road in North-east New South Wales? Australian Zoologist 33, 332-336.
| Crossref | Google Scholar |
Gorman TA, Haas CA (2011) Seasonal microhabitat selection and use of syntopic populations of Lithobates okaloosae and Lithobates clamitans clamitans. Journal of Herpetology 45, 313-318.
| Crossref | Google Scholar |
Green AW, Hooten MB, Grant EHC, Bailey LL (2013) Evaluating breeding and metamorph occupancy and vernal pool management effects for wood frogs using a hierarchical model. Journal of Applied Ecology 50, 1116-1123.
| Crossref | Google Scholar |
Guillera-Arroita G, Lahoz-Monfort JJ, MacKenzie DI, Wintle BA, McCarthy MA (2014) Ignoring imperfect detection in biological surveys is dangerous: a response to ‘fitting and interpreting occupancy models’. PLoS ONE 9, e99571.
| Crossref | Google Scholar |
Hale JM, Heard GW, Smith KL, Parris KM, Austin JJ, Kearney M, Melville J (2013) Structure and fragmentation of growling grass frog metapopulations. Conservation Genetics 14, 313-322.
| Crossref | Google Scholar |
Hamer AJ, McDonnell MJ (2008) Amphibian ecology and conservation in the urbanising world: a review. Biological Conservation 141, 2432-2449.
| Crossref | Google Scholar |
Hamer AJ, Lane SJ, Mahony MJ (2002) Management of freshwater wetlands for the endangered Green and Golden Bell Frog (Litoria aurea): roles of habitat determinants and space. Biological Conservation 106, 413-424.
| Crossref | Google Scholar |
Hamer AJ, Smith PJ, McDonnell MJ (2012) The importance of habitat design and aquatic connectivity in amphibian use of urban stormwater retention ponds. Urban Ecosystems 15, 451-471.
| Crossref | Google Scholar |
Hazell D (2003) Frog ecology in modified Australian landscapes: a review. Wildlife Research 30, 193-205.
| Crossref | Google Scholar |
Hazell D, Cunnningham R, Lindenmayer D, Mackey B, Osborne W (2001) Use of farm dams as frog habitat in an Australian agricultural landscape: factors affecting species richness and distribution. Biological Conservation 102, 155-169.
| Crossref | Google Scholar |
Hazell D, Hero J-M, Lindenmayer D, Cunningham R (2004) A comparison of constructed and natural habitat for frog conservation in an Australian agricultural landscape. Biological Conservation 119, 61-71.
| Crossref | Google Scholar |
Healey M, Thompson D, Robertson A (1997) Amphibian communities associated with billabong habitats on the Murrumbidgee floodplain, Australia. Australian Journal of Ecology 22, 270-278.
| Crossref | Google Scholar |
Heard G, Robertson P, Scroggie M (2008) Microhabitat preferences of the endangered growling grass Frog Litoria raniformis in southern Victoria. Australian Zoologist 34, 414-425.
| Crossref | Google Scholar |
Heard GW, Scroggie MP, Ramsey DSL, Clemann N, Hodgson JA, Thomas CD (2018) Can habitat management mitigate disease impacts on threatened Amphibians? Conservation Letters 11, e12375.
| Crossref | Google Scholar |
Hero J-M, Morrison C, Gillespie G, Dale Roberts J, Newell D, Meyer E, McDonald K, Lemckert F, Mahony M, Osborne W, Hines H, Richards S, Hoskin C, Clarke J, Doak N, Shoo L (2006) Overview of the conservation status of Australian frogs. Pacific Conservation Biology 12, 313-320.
| Crossref | Google Scholar |
Houlahan JE, Findlay CS (2003) The effects of adjacent land use on wetland amphibian species richness and community composition. Canadian Journal of Fisheries and Aquatic Sciences 60, 1078-1094.
| Crossref | Google Scholar |
Hunter D, Osborne W, Smith M, McDougall K (2009) Breeding habitat use and the future management of the critically endangered Southern Corroboree Frog. Ecological Management & Restoration 10, S103-S109.
| Crossref | Google Scholar |
IUCN (2022) The IUCN Red List of Threatened Species Version 2022.2. Available at https://www.iucnredlist.org.
Johnson JB, Omland KS (2004) Model selection in ecology and evolution. Trends in Ecology & Evolution 19(2), 101-108.
| Crossref | Google Scholar |
King JE (2003) Running a best-subsets logistic regression: an alternative to stepwise methods. Education and Psychological Measurement 63, 392-403.
| Google Scholar |
Knight AR, Allan C (2021) Intentional ecology: integrating environmental expertise through a focus on values, care and advocacy. Humanities and Social Sciences Communications 8, 290.
| Crossref | Google Scholar |
Lane SJ, Hamer AJ, Mahony MJ (2007) Habitat correlates of five amphibian species and of species-richness in a wetland system in New South Wales, Australia. Applied Herpetology 4, 65-82.
| Crossref | Google Scholar |
Lauck B (2005) The impact of recent logging and pond isolation on pond colonization by the frog Crinia signifera. Pacific Conservation Biology 11, 50-56.
| Crossref | Google Scholar |
Lemckert F (2001) The influence of micrometeorological factors on the calling activity of the frog Crinia signifera (Anura: Myobatrachidae). Australian Zoologist 31, 625-631.
| Crossref | Google Scholar |
Lemckert FL, Shine R (1993) Costs of reproduction in a population of the frog Crinia signifera (Anura: Myobatrachidae) from Southeastern Australia. Journal of Herpetology 27, 420-425.
| Crossref | Google Scholar |
Letnic M, Fox BJ (1997) The impact of industrial fluoride fallout on faunal succession following sand-mining of dry sclerophyll forest at Tomago, NSW, II. Myobatrachid frog recolonization. Biological Conservation 82, 137-146.
| Crossref | Google Scholar |
Lind AJ, Welsh HH, Jr, Wilson RA (1996) The effects of a dam on breeding habitat and egg survival of the foothill yellow-legged frog (Rana boylii) in Northwestern California. Herpetological Review 27, 62-67.
| Google Scholar |
Littlejohn MJ (1958) A new species of frog of the genus Crinia Tschudi from South-eastern Australia. Proceedings of the Linnean Society of New South Wales 83, 222-226.
| Google Scholar |
Littlejohn MJ (1964) Geographic isolation and mating call differentiation in Crinia signifera. Evolution 18, 262-266.
| Crossref | Google Scholar |
Lowe K, Castley JG, Hero J-M (2013) Acid frogs can stand the heat: amphibian resilience to wildfire in coastal wetlands of eastern Australia. International Journal of Wildland Fire 22, 947-958.
| Crossref | Google Scholar |
Luedtke JA, Chanson J, Neam K, Hobin L, Maciel AO, Catenazzi A, Borzée A, Hamidy A, Aowphol A, Jean A, Sosa-Bartuano Á, Fong G A, de Silva A, Fouquet A, Angulo A, Kidov AA, Muñoz Saravia A, Diesmos AC, Tominaga A, Shrestha B, Gratwicke B, Tjaturadi B, Martínez Rivera CC, Vásquez Almazán CR, Señaris C, Chandramouli SR, Strüssmann C, Cortez Fernández CF, Azat C, Hoskin CJ, Hilton-Taylor C, Whyte DL, Gower DJ, Olson DH, Cisneros-Heredia DF, Santana DJ, Nagombi E, Najafi-Majd E, Quah ESH, Bolaños F, Xie F, Brusquetti F, Álvarez FS, Andreone F, Glaw F, Castañeda FE, Kraus F, Parra-Olea G, Chaves G, Medina-Rangel GF, González-Durán G, Ortega-Andrade HM, Machado IF, Das I, Dias IR, Urbina-Cardona JN, Crnobrnja-Isailović J, Yang J-H, Jianping J, Wangyal JT, Rowley JJL, Measey J, Vasudevan K, Chan KO, Gururaja KV, Ovaska K, Warr LC, Canseco-Márquez L, Toledo LF, Díaz LM, Khan MMH, Meegaskumbura M, Acevedo ME, Napoli MF, Ponce MA, Vaira M, Lampo M, Yánez-Muñoz MH, Scherz MD, Rödel M-O, Matsui M, Fildor M, Kusrini MD, Ahmed MF, Rais M, Kouamé NGG, García N, Gonwouo NL, Burrowes PA, Imbun PY, Wagner P, Kok PJR, Joglar RL, Auguste RJ, Brandão RA, Ibáñez R, von May R, Hedges SB, Biju SD, Ganesh SR, Wren S, Das S, Flechas SV, Ashpole SL, Robleto-Hernández SJ, Loader SP, Incháustegui SJ, Garg S, Phimmachak S, Richards SJ, Slimani T, Osborne-Naikatini T, Abreu-Jardim TPF, Condez TH, De Carvalho TR, Cutajar TP, Pierson TW, Nguyen TQ, Kaya U, Yuan Z, Long B, Langhammer P, Stuart SN (2023) Ongoing declines for the world’s amphibians in the face of emerging threats. Nature 622(7982), 308-314.
| Crossref | Google Scholar |
Mac Nally R (1979) Social organisation and interspecific interactions in two sympatric species of Ranidella (Anura). Oecologia 42(3), 293-306.
| Google Scholar |
Mac Nally R (1985) Habitat and microhabitat distributions in relation to ecological overlap in two species of Ranidella (Anura). Australian Journal of Zoology 33, 329-338.
| Google Scholar |
Mac Nally R, Horrocks G, Lada H, Lake PS, Thomson JR, Taylor AC (2009) Distribution of anuran amphibians in massively altered landscapes in south-eastern Australia: effects of climate change in an aridifying region. Global Ecology and Biogeography 18(5), 575-585.
| Crossref | Google Scholar |
MacKenzie DI (2006) Modeling the probability of resource use: the effect of, and dealing with, detecting a species imperfectly. Journal of Wildlife Management 70, 367-374.
| Crossref | Google Scholar |
MacKenzie DI, Royle JA (2005) Designing occupancy studies: general advice and allocating survey effort. Journal of Applied Ecology 42, 1105-1114.
| Crossref | Google Scholar |
Marsh DM, Trenham PC (2001) Metapopulation dynamics and amphibian conservation. Conservation Biology 15, 40-49.
| Crossref | Google Scholar |
Marsh DM, Fegraus EH, Harrison S (1999) Effects of breeding pond isolation on the spatial and temporal dynamics of pond use by the tungara frog, Physalaemus pustulosus. Journal of Animal Ecology 68, 804-814.
| Crossref | Google Scholar |
Mazerolle MJ (2005) Drainage ditches facilitate frog movements in a hostile landscape. Landscape Ecology 20, 579-590.
| Crossref | Google Scholar |
McKnight DT, Lal MM, Bower DS, Schwarzkopf L, Alford RA, Zenger KR (2019) The return of the frogs: the importance of habitat refugia in maintaining diversity during a disease outbreak. Molecular Ecology 28, 2731-2745.
| Crossref | Google Scholar | PubMed |
Meyer EA, Hines HB, Clarke JM, Hodgon J, Gynther I (2004) Occurrence of the Wallum Froglet (Crinia tinnula) at Littabella National Park, southeastern Queensland. Memoirs of the Queensland Museum 49, 691-692.
| Google Scholar |
Odendaal FJ, Bull CM (1983) Water movements, tadpole competition and limits to the distribution of the frogs Ranidella riparia and R. signifera. Oecologia 57, 361-367.
| Crossref | Google Scholar | PubMed |
Odendaal FJ, Bull CM, Nias RC (1982) Habitat selection in tadpoles of Ranidella signifera and R. riparia (Anura: Leptodactylidae). Oecologia 52, 411-414.
| Crossref | Google Scholar | PubMed |
Otto CRV, Forester DC, Snodgrass JW (2007) Influences of wetland and landscape characteristics on the distribution of carpenter frogs. Wetlands: Journal of the Society of Wetland Scientists 27, 261-269.
| Crossref | Google Scholar |
Paton PWC, Crouch WB, III (2002) Using the phenology of pond-breeding amphibians to develop conservation strategies. Conservation Biology 16, 194-204.
| Crossref | Google Scholar | PubMed |
Pope SE, Fahrig L, Merriam HG (2000) Landscape complementation and metapopulation effects on leopard frog populations. Ecology 81, 2498-2508.
| Crossref | Google Scholar |
Popescu VD, Kissel AM, Pearson M, Palen WJ, Govindarajulu P, Bishop CA (2013) Defining conservation-relevant habitat selection by the highly imperiled Oregon Spotted Frog, Rana pretiosa. Herpetological Conservation and Biology 8, 688-706.
| Google Scholar |
Purrenhage JL, Boone MD (2009) Amphibian community response to variation in habitat structure and competitor density. Herpetologica 65, 14-30.
| Google Scholar |
Scheele BC, Pasmans F, Skerratt LF, Berger L, Martel A, Beukema W, Acevedo AA, Burrowes PA, Carvalho T, Catenazzi A, De la Riva I, Fisher MC, Flechas SV, Foster CN, Frías-Álvarez P, Garner TWJ, Gratwicke B, Guayasamin JM, Hirschfeld M, Kolby JE, Kosch TA, La Marca E, Lindenmayer DB, Lips KR, Longo AV, Maneyro R, McDonald CA, Mendelson J, III, Palacios-Rodriguez P, Parra-Olea G, Richards-Zawacki CL, Rödel M-O, Rovito SM, Soto-Azat C, Toledo LF, Voyles J, Weldon C, Whitfield SM, Wilkinson M, Zamudio KR, Canessa S (2019) Amphibian fungal panzootic causes catastrophic and ongoing loss of biodiversity. Science 363, 1459.
| Crossref | Google Scholar | PubMed |
Scheffers BR, Paszkowski CA (2013) Amphibian use of urban stormwater wetlands: the role of natural habitat features. Landscape and Urban Planning 113, 139-149.
| Crossref | Google Scholar |
Shulse CD, Semlitsch RD, Trauth KM, Williams AD (2010) Influences of design and landscape placement parameters on amphibian abundance in constructed wetlands. Wetlands 30, 915-928.
| Crossref | Google Scholar |
Sievers M, Parris KM, Swearer SE, Hale R (2018) Stormwater wetlands can function as ecological traps for urban frogs. Ecological Applications 28, 1106-1115.
| Crossref | Google Scholar | PubMed |
Sievers M, Hale R, Swearer SE, Parris KM (2019) Frog occupancy of polluted wetlands in urban landscapes. Conservation Biology 33, 389-402.
| Crossref | Google Scholar | PubMed |
Simon JA, Snodgrass JW, Casey RE, Sparling DW (2009) Spatial correlates of amphibian use of constructed wetlands in an urban landscape. Landscape Ecology 24, 361-373.
| Crossref | Google Scholar |
Simpkins CA, Shuker JD, Lollback GW, Castley JG, Hero J-M (2014) Environmental variables associated with the distribution and occupancy of habitat specialist tadpoles in naturally acidic, oligotrophic waterbodies. Austral Ecology 39, 95-105.
| Crossref | Google Scholar |
Smallbone LT, Luck GW, Wassens S (2011) Anuran species in urban landscapes: relationships with biophysical, built environment and socio-economic factors. Landscape and Urban Planning 101, 43-51.
| Crossref | Google Scholar |
South Eastern Australian Climate Initiative (2011) The millennium drought and 2010/11 floods. Available at https://www.seaci.org/publications/documents/SEACI-2Reports/SEACI2_Factsheet2of4_WEB_110714.pdf [accessed 12 November 2023]
State of NSW and Department of Planning, Industry and Environment (2020) NSW survey guide for threatened frogs. A guide for the survey of threatened frogs and their habitats for the biodiversity assessment method. Available at https://www.environment.nsw.gov.au/-/media/OEH/Corporate-Site/Documents/Animals-and-plants/Threatened-species/nsw-survey-guide-for-threatened-frogs-200440.pdf [accessed 24 February 2024]
Thomas RL, Owen-Smith L, Drake DC, Alexander GJ (2014) Restoring breeding habitat for Giant Bullfrogs (Pyxicephalus adspersus) in South Africa. African Journal of Herpetology 63, 13-24.
| Crossref | Google Scholar |
Van Sickle J, Huff DD, Hawkins CP (2006) Selecting discriminant function models for predicting the expected richness of aquatic macroinvertebrates. Freshwater Biology 51, 359-372.
| Google Scholar |
Wassens S, Watts RJ, Jansen A, Roshier D (2008) Movement patterns of southern bell frogs (Litoria raniformis) in response to flooding. Wildlife Research 35, 50-58.
| Crossref | Google Scholar |
Wassens S, Hall A, Osborne W, Watts RJ (2010) Habitat characteristics predict occupancy patterns of the endangered amphibian Litoria raniformis in flow-regulated flood plain wetlands. Austral Ecology 35, 944-955.
| Crossref | Google Scholar |
Wassens S, Walcott A, Wilson A, Freire R (2013) Frog breeding in rain-fed wetlands after a period of severe drought: implications for predicting the impacts of climate change. Hydrobiologia 708, 69-80.
| Crossref | Google Scholar |
Webb JA, Wallis EM, Stewardson MJ (2012) A systematic review of published evidence linking wetland plants to water regime components. Aquatic Botany 103, 1-14.
| Crossref | Google Scholar |
Welch NE, MacMahon JA (2005) Identifying habitat variables important to the Rare Columbia Spotted Frog in Utah (U.S.A.): an information-theoretic approach. Conservation Biology 19, 473-481.
| Crossref | Google Scholar |
Wells KD, Taigen TL, O’Brien JA (1996) The effect of temperature on calling energetics of the spring peeper (Pseudacris crucifer). Amphibia-Reptilia 17, 149-158.
| Crossref | Google Scholar |
Williamson I, Bull CM (1995) Life-history variation in a population of the Australian Frog Ranidella signifera: seasonal changes in clutch parameters. Copeia 1995, 105-113.
| Crossref | Google Scholar |
Wong BBM, Cowling ANN, Cunningham RB, Donnelly CF (2004) Do temperature and social environment interact to affect call rate in frogs (Crinia signifera)? Austral Ecology 29, 209-214.
| Crossref | Google Scholar |